- 1Department of Microbiology, College of Veterinary Medicine, King Faisal University, Al-Hofuf, Saudi Arabia
- 2Department of Veterinary Medicine, Faculty of Agriculture and Veterinary Medicine, Thamar University, Dhamar, Yemen
- 3Camel Research Center, King Faisal University, Al Hofuf, Saudi Arabia
- 4Faculty of Veterinary Medicine, Veterinary Educational Hospital, Zagazig University, Zagazig, Egypt
- 5Veterinary Diagnostic Laboratory, Ministry of Environment, Water and Agriculture, Al-Ahsa, Saudi Arabia.
- 6Department of Biomedical Sciences, College of Veterinary Medicine, King Faisal University, Al-Hofuf, Saudi Arabia
- 7Department of Pharmacology, Faculty of Veterinary Medicine, Kafrelsheikh University, Kafrelsheikh, Egypt
- 8Department of Veterinary Biomedical Sciences, College of Veterinary Medicine, Long Island University, Brookville, NY, United States
Introduction: Avian orthoavulavirus-1 (AOAV1) has a wide host range, including domestic and wild birds. The present study aimed to identify the currently circulating AOAV1 strains from some outbreaks in some backyard pigeons in the eastern region of Saudi Arabia (ERSA).
Methods: Tracheal/cloacal swabs and tissue specimens were collected from eight backyards in Al-Ahsa, ERSA, between January 2021 and March 2023. Samples were tested for the presence of AOAV1 using commercial real-time RT-PCR. Part of the fusion gene was also amplified by gel-based RT-PCR, and the obtained amplicons were sequenced.
Results and discussion: AOAV1 was detected in samples from the eight flocks. The retrieved sequences from samples of 6/8 pigeon backyards are reported. Phylogenetic analysis based on the obtained sequences from these backyard pigeons showed the segregation of the obtained sequences in AOAV1 genotypes VI.2.1 and VII.1.1. Clinically, nervous manifestations were dominant in pigeons infected with both genotypes. Respiratory manifestations and significantly higher overall mortality rate were induced by genotype VI.2.1. The deduced amino acid sequences of the fusion protein cleavage site (FPCS) showed that all the detected isolates belong to velogenic strains. Differences in clinical profiles induced by the natural infection of pigeons with AOAV1 genotypes VI.2.1 and VII.1.1 were reported. The present findings highlight the potential roles of some backyard pigeons in the long-distance spread and cross-species transmission of the reported AOAVI genotypes. Further research is required to perform biotyping and pathotyping of the reported strains.
1 Introduction
Avian Orthoavulavirus-1 (AOAV1), formerly termed Avian Paramyxovirus 1 (APMV1), belongs to the genus Orthoavulavirus of the subfamily Avulavirinae, family Paramyxoviridae. AOAV1 possesses a single-stranded negative-sense RNA genome of about 15 kb in length that encodes six genes (3′-N-P-M-F-HN-L-5′) (1). AOAV1 can be classified according to their sequence similarity (genotyping), according to their ability to induce death and/or nervous signs in inoculated embryonated eggs or chicks [mean death time (MDT) and intracerebral pathogenicity index (ICPI)], and according to the clinical signs they induce in naturally infected chickens. The fourth gene, Fusion (F), is frequently targeted by molecular diagnostic techniques for detection, sequencing, and genotyping of the AOAV1. Based on complete F gene sequences, AOAV1 has been classified into two major classes: I and II. Class II includes virulent and avirulent viruses, and it is subclassified into 21 genotypes (I to XXI) and many subgenotypes (2). AOAV1 may also be classified according to the score of the ICPI into lentogenic (< 0.7), mesogenic (0.7-1.5), and velogenic (>1.5) strains (3). Alternatively, the World Organization of Animal Health (WOAH) recommended the use of the deduced amino acid sequence of the F protein cleavage site (FPCS) as a non-invasive technique to assess the virulence of the AOAV1 isolates (4). Based on the clinical signs, AOAV1 infection in chickens was classified into five types: asymptomatic enteric, lentogenic, mesogenic, velogenic viscerotropic, and velogenic neurotropic (4). Differentiation between velogenic viscerotropic and velogenic neurotropic strains demands evaluation of the clinical disease or an intravenous test in adult birds (3).
AOAV1 affects a wide range of bird species, such as chickens, turkeys, pigeons, and wild bird species (2, 5). This virus induces considerable economic losses to the poultry industry worldwide (6). Additionally, AOAV1 can affect humans and cause mild, transient conjunctivitis, though rare, severe infections were also reported in immunocompromised patients (7, 8). The virus remains endemic in a wide part of the world, and some of the affected birds were implicated in the maintenance of the virus (9). Virulent AOAV1 was reported in migratory waterfowl (10). In fact, it has been demonstrated that similar AOAV1 strains are circulating simultaneously in both domestic and wild birds (11–13), suggesting a potential role in the dissemination of virulent AOAV1 (5, 14, 15). The role of some free-ranging birds, like pigeons, in the transmission of virulent NDV to poultry is also a major concern (16–18).
AOAV1 has been reported in Saudi Arabia in the 1980s (19). The AOAV1 genotypes II, VI, VII, XII, XIII, XX, and XXI have been reported in Saudi Arabia and many surrounding countries (2, 20–23). The diversity of the reported genotypes implies the high vulnerability of this region to the spread of AOAV1 and dictates continuous monitoring of this virus. Limited data is available on the circulating AOAV1 genotypes in non-chicken flocks, such as pigeons, in Saudi Arabia. Pigeon flocks in Al-Ahsa, ERSA, have experienced disease with manifestations suggestive of AOAV1 infection during the years 2021 and 2022. Swabs and tissue samples were collected from affected flocks; nucleic acid was extracted from the collected samples and used in an RT-PCR assay to detect AOAV1. The F-gene was also targeted for sequencing and genotyping of the detected AOAV1. Herein, we report on findings from disease investigation, detection, and genotyping of AOAV1 in some affected pigeon flocks in ERSA.
2 Materials and methods
2.1 Ethical approval
All management conditions and experimental procedures used in the present study were approved by the Ethics Committee at King Faisal University, Saudi Arabia (KFU-REC-2022-MAR-EA000541). Additionally, the experimental protocols and all management conditions in the study were conducted following the regulations, husbandry, ethics, and approved guidelines of the Institutional Animal Care and Use Committee of the Faculty of Veterinary Medicine, Zagazig University, Egypt under the reference number of ZU-IACUC/2/F/444/2023.
2.2 Sample collection and processing
The study was conducted in Al-Ahsa governorate in the ERSA during the period from January 2021 to March 2023. Pigeons flocks showing signs suggestive of AOAV1 infection were targeted in the present study. Epidemiological and clinical data were reported. The birds showing nervous manifestations were euthanized by cervical dislocation following standard procedures according to Anon (24). A diagnostic postmortem inspection was conducted, and the main lesions were systematically reported. A total of 64 cloacal/tracheal swabs and 147 tissue/organ specimens were collected from eight backyard pigeon flocks in ERSA at this time window. Tracheal and cloacal swabs were collected on viral transport media prepared from Dulbecco's Modified Eagle's Medium, and antibiotics (2,000 U/ml penicillin, 2 mg/ml streptomycin, 50 μg/ml gentamycin), anti-mycotic (50 U/ml nystatin) and bovine serum albumin (0.5%) (Sigma-Aldrich, USA). Swabs were thoroughly vortexed for 2 min and then stored at (−80°C). Ten percent of tissue homogenates were prepared from collected tissue samples (trachea, lung, glandular stomach, spleen, liver, and brain) using silica beads and Tissue-Lyser (Qiagen, Hilden, Germany). The obtained supernatants were then stored at (−80°C) for further analysis.
2.3 Detection of AOAV1 by real-time RT-PCR
Viral RNA was extracted from the processed swabs and tissue suspensions using the MagNA Pure Compact Nucleic Acid Isolation Kit (Cat # 03 730 964 001, Roche Diagnostic Gmbh, Mannheim, Germany) according to the manufacturer's instructions. Extracted viral RNAs were stored at (−20°C) until used in a subsequent molecular procedure. For the detection of the AOAV1 nucleic acid, the commercially-available real time RT-PCR reagents developed and manufactured by TIB MOLBIOL Gmbh berline, Germany were used. Forward and reverse primers and probes used according to the method of Wise et al. (25) and produced by TIB MOLBIOL Gmbh berline, Germany. Briefly, the provided specific primers were used to amplify a 99-bp fragment of the AOAV1-fusion gene, and the amplified fragment was detected using a hybridization probe labeled with Light Cycler Red 640. The test was considered valid only if the negative control showed no signal, the low concentration NDV DNA samples should show an amplification curve for IC with Cp at approximately cycle 28–30. The kit provides standard row of clone and purified DNA with concentration in the range from 105 to 101 copies/rxn of NDV should have Cp value (19–23, 26–38).
2.4 Genotyping of the detected AOAV1 amplicons
According to the manufacturer's instructions, the Reverse Transcription System (Cat # A3500, Promega, USA) was used to reverse transcribe of the extracted viral RNA. The obtained cDNA was then stored at (−80°C) until used in a PCR test. The previously described primers (Table 1) and thermal protocols were used to amplify the fusion gene of the AOAV1 (27, 28). The PCR assay was performed using the Go-Taq Green Master Mix (Cat #M7122, Promega, USA) in a final volume of 25 μl according to the manufacturer's procedure. Thermal manipulation was performed using the Bio-Rad T-100 thermal cycler. PCR products were separated by electrophoresis in a 1% agarose gel in Tris-borate-EDTA buffer with red-safe nucleic acid staining dye (Cat #21141, iNtRON, Biotechnology, Burlington, USA). Targeted bands of correct size were excised, and amplicons were purified using the Wizard SV Gel and PCR Clean-up System (Cat # A1460, Promega) according to the manufacturer's instructions. Purified amplicons were sequenced at Macrogen Sequencing Service, South Korea, using the same primers as for the PCR.
For both PCR assays, internal extraction control were used in parallel to the tested samples to evaluate the RNA extraction process. Additionally, positive internal control were used for evaluation of amplification of target gene as well as negative control to exclude cross contamination. The negative control was setup with all the ingredients except the cDNA which was replaced with nuclease free water.
2.5 Sequence analysis and phylogenetic analysis
Sequences were handled using the Molecular Evolutionary Genetics Analysis (MEGA) 11 software (29). Sequences were aligned using the ClustalW method with default setting. Genbank BLAST search was used to retrieve most similar sequences to those reported in the present study. Sequences with identity of ≥95% were involved in the analysis after removal of those showing high identity to each other (≥99). Sequences from Saudi Arabia and gulf region were also involved in the analysis. The phylogenetic analysis was performed according to the genotyping and nomenclature system of the AOAV1 developed by (2). Phylogenetic trees were prepared using the Neighbor-Joining method in MEGA11 with a bootstrap value of 1,000 replicates. Sequence identity was calculated by dividing the number of matched nucleotides by the total length of the aligned pairs of sequences.
2.6 Data analysis
Data was handled using MS Excel. The Fisher's exact test was used to reveal the significance of the differences in morbidity and mortality rates induced by detected genotypes. Significance was defined as a p < 0.05.
2.7 Genbank accession number (GB#)
The reported pigeon-AOAV1 sequences in the present study were deposited in the Genbank under the GB# shown in Table 4.
3 Results
3.1 Molecular detection and genotyping
AOAV1 was detected by real-time RT-PCR in the samples collected from the investigated eight pigeon flocks. Regarding the genotyping RT-PCR, partial F gene sequences were successfully recovered from samples of 4/8 pigeon flocks (F1 to F4) based on the F-primers (Table 1). Subsequently, the I and N primers were also used in attempts to obtain sequences from samples of the remaining pigeon flocks. Sequences were recovered from samples of two additional pigeon flocks using primers I (I2 and I3).
Phylogenetic analysis showed the segregation of 3/6 sequences (F1, F3, and F4) with AOAV1-subgenotype VII.1.1 (VII. l). These sequences showed an internal identity of 100%. The most similar (97.2%) published sequence (GB# KX268351) was recovered from chickens in Iran in 2015 (Figure 1). The remaining three sequences (F2, I2, and I3) showed an overall similarity of 97.8%-100% and segregated with subgenotype VI.2.1. These sequences clustered with the reference sequence (GB# JX518532) that was recovered from a laughing dove in Kenya in 2012 with (95.1%−95.2%) similarities. Additionally, it showed 94.1% to 95.4% similarity with the published sequence (GB#HG326604) that was recovered from pigeons in Nigeria in 2009. The sequence identity of the two groups with published sequences of the AOAV1 genotypes and subgenotypes is shown in Table 2.
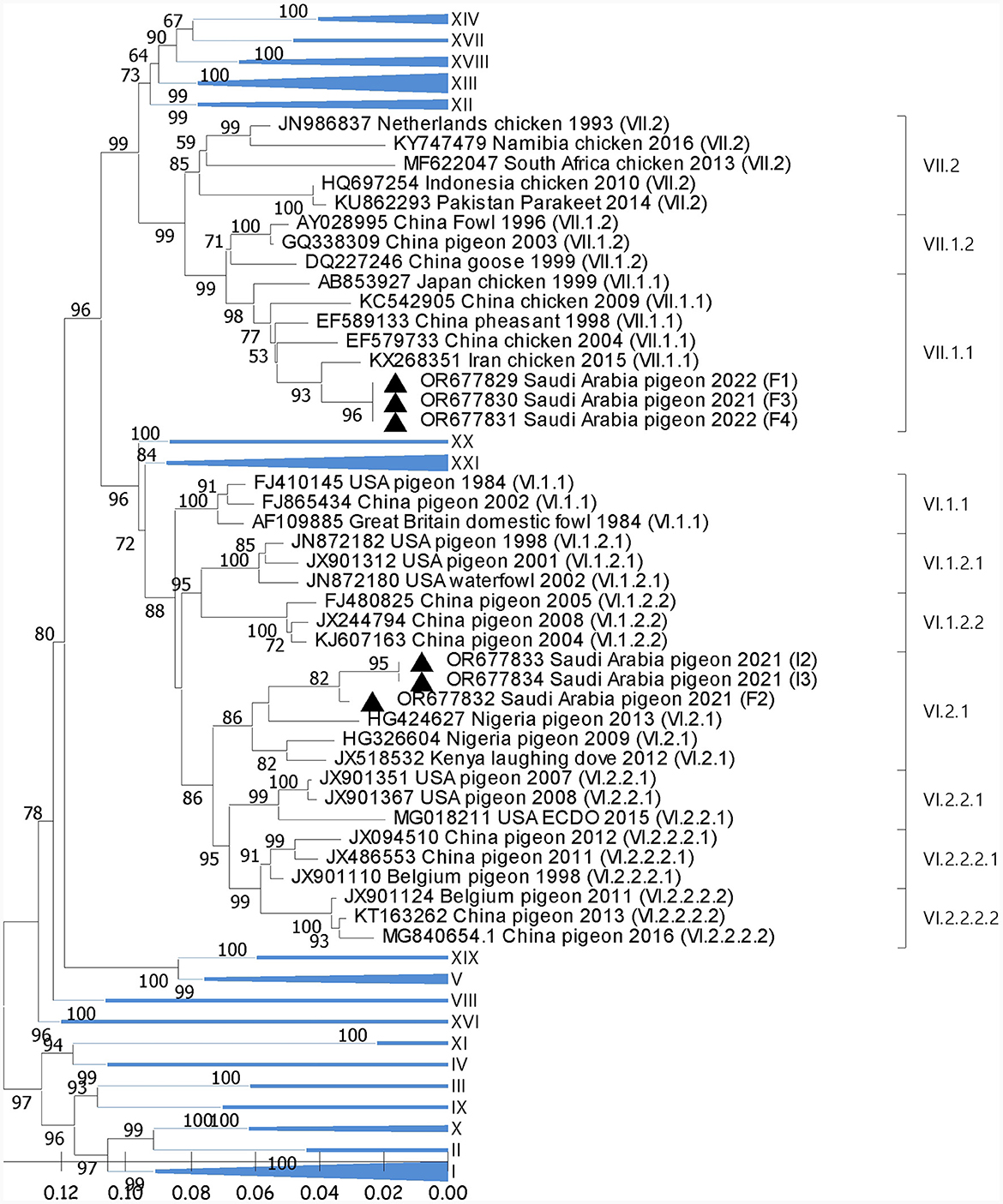
Figure 1. Phylogenetic analysis of the reported AOAV1 sequences from pigeons. The tree was constructed using the NJ alignment with a bootstrap value (=1,000). The black triangle indicates the present detections of AOAV1. GB# of sequences are shown, followed by country, host, and collection year of the sequenced samples. Genotypes of the published sequences are shown in brackets.
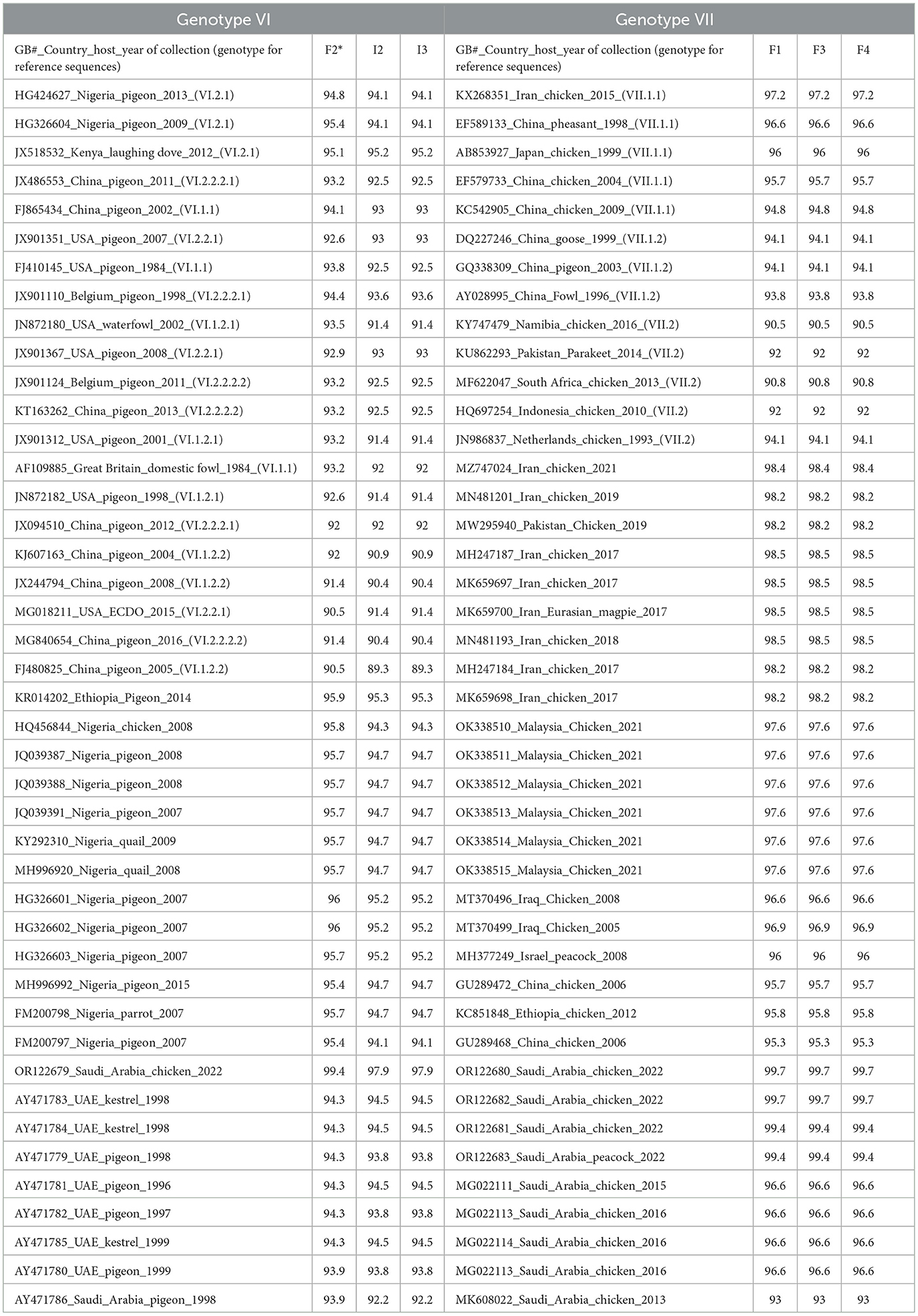
Table 2. Averaged identity of recovered AOAV1 sequences of each genotype with reference sequences and most similar local and regional sequences according to BLAST search.
3.2 Comparison of the similarity of the reported sequences with local and regional AOAV1 sequences
Reported sequences in the subgenotype VI.2.1 showed (97.9%-99.4%) similarity with the (GB# OR122679; SA/Chicken/NDV1/2022) sequence isolated from backyard chickens in Al-Ahsa in 2022. Regionally, these sequences showed (94.4%) similarity with (GB# AY471783), isolated from Kestrel in the UAE in 1998. It also showed (95.3%-95.9%) identity with the (GB# KR014202) sequence that was recovered from pigeons in Ethiopia in 2014 (Figure 2). The BLAST analysis showed that sequences with ≥95% similarity were mainly isolated from pigeons and rarely from quail, parrots, and laughing doves through the period from 2004 to 2019 from Nigeria, Kenya, Ethiopia, Iran, Iraq, Turkey, Russia, and China.
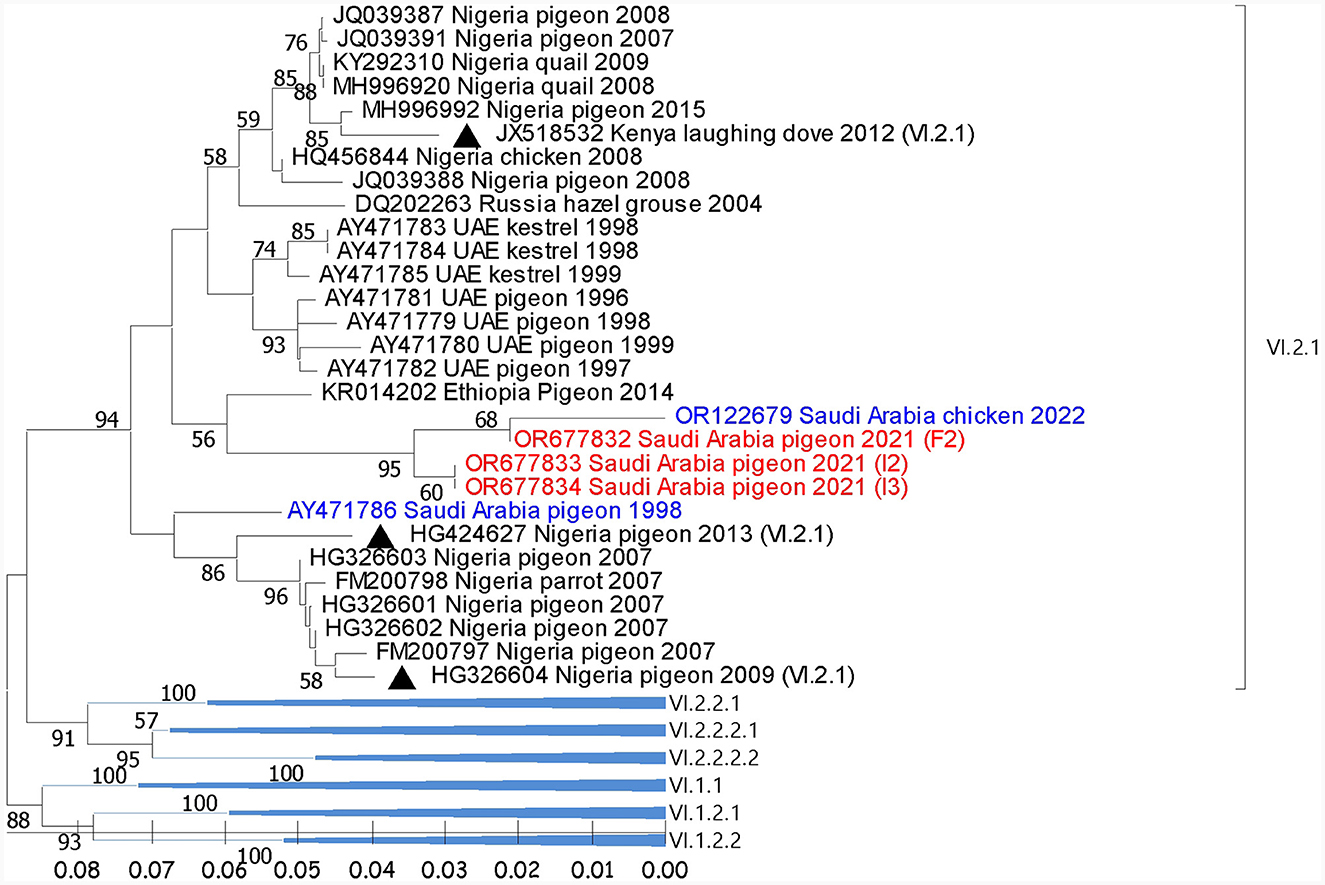
Figure 2. Phylogenetic analysis of the reported AOAV1-VI.2.1 sequences from pigeons and most similar sequences. The tree was constructed using the NJ alignment with a bootstrap value of 1,000. The black triangles indicate the reference sequence; the blue ones are the previous detections from Saudi Arabia; the red ones are the present detections of AOAV1. GB# of sequences are shown, followed by country, host, and collection year of the sequenced samples. Genotypes of the reference sequences are shown in brackets.
Reported sequences in the subgenotype VII.1.1 showed 99.4% to 99.7% similarity with sequences recovered from chickens and peacocks in Al-Ahsa in 2022. A lower (93% to 96.6%) similarity was shared with sequences that were isolated from chickens in Saudi Arabia in 2013 to 2015. Regionally, these sequences showed (98.4%) similarity with the (GB# MZ747024) sequence that was isolated from chickens in Iran in 2021 (Figure 3).
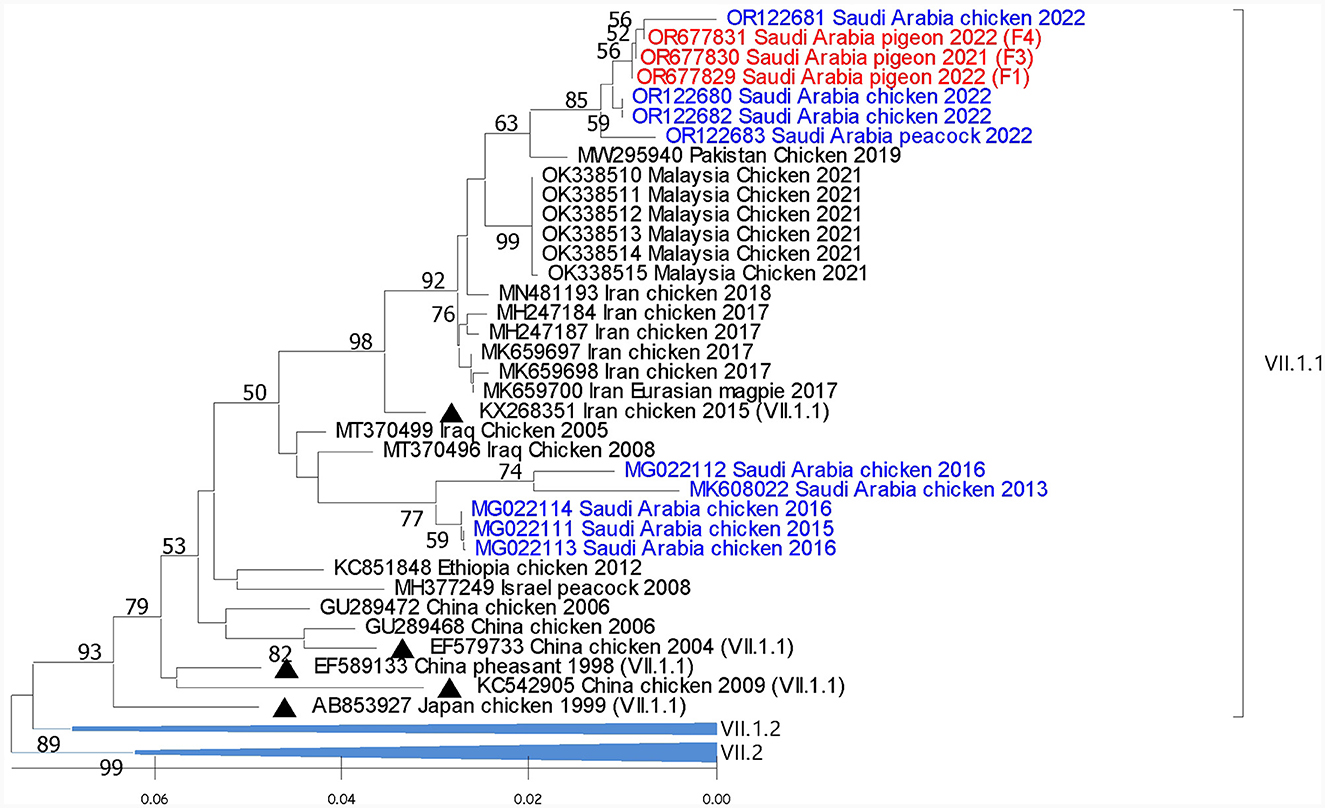
Figure 3. Phylogenetic analysis of the reported AOAV1-VII.1.1 sequences from pigeons and most similar sequences. The tree was constructed using the NJ alignment with a bootstrap value of 1,000. The black triangles indicate the reference sequence; the blue ones are the previous detections from Saudi Arabia, and the red ones are the present detections of AOAV1. GB# of sequences are shown, followed by country, host, and collection year of the sequenced samples. The genotypes of the reference sequences are shown in brackets.
3.3 Epidemiological and clinical data of the investigated pigeon flocks
Infected pigeon flocks showed morbidity rates ranging between 25% and 50%, while mortality rates ranged between 8.3% and 21.3% (Table 3). Some pigeons or squabs were found dead without any clinical signs. In others, the disease started with depression and loss of appetite, mild respiratory signs, and greenish diarrhea. These were followed by the appearance of nervous manifestations that commence with wing and leg paralysis, recumbency, or inability to walk and fly. In some cases, severe nervous signs follow and include circling, loss of balance, torticollis, neck paralysis, or twisting (Figure 4), and then finally death. Necropsy of recently died pigeons or euthanized diseased pigeons revealed the presence of congestion/hemorrhages in the trachea, lungs, liver, spleen, and brain. Petechial hemorrhages were also observed on proventriculus and cecal tonsils.
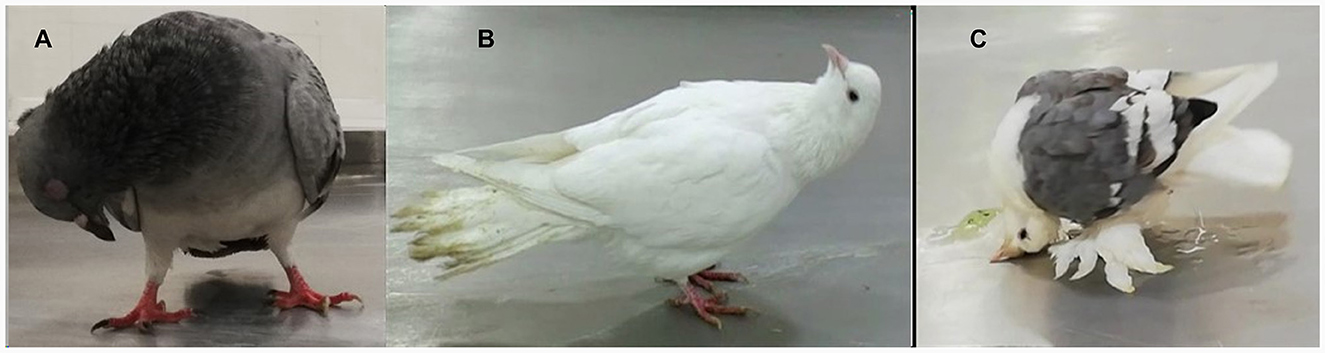
Figure 4. Nervous signs (head and neck twist) (A–C) and greenish diarrhea (B, C) with a soiled tail feather (B) in pigeons infected with AOAV1 in the present study.
There were some differences in the clinical profiles and mortality rates of pigeons naturally infected with either genotype VI.2.1 or genotype VII.1.1. Pigeons infected with genotype VI.2.1 showed signs and lesions consistent with respiratory, digestive, and nervous involvements. Similar signs and lesions were observed in pigeons infected with genotype VII.1.1, except that respiratory involvement was less obvious. Both genotypes induce an almost identical overall morbidity rate of approximately 30% (156 birds out of 505 birds for genotype VI.2.1 and 178 birds out of 592 birds for genotype VII.1.1). However, genotype VI.2.1 induced a significant (P = 0.0009) higher overall mortality rate of 18.82% (95 birds out of 505 birds) in comparison with the 11.49% induced by genotype VII.1.1 (68 birds out of 592 birds).
3.4 Amino acid substitution
Based on the deduced amino acid sequences of the FPCS (112RRR/QKR↓F117), all the reported sequences belonged to the velogenic AOAV1 strains (Table 4). The deduced amino acids for the reported sequences were compared with the most similar sequences, those recently reported from Al-Ahsa, ERSA (GB# OR122679 and OR122681). The reported VI.2.1 sequences have a single substitution (G123S), while the reported VII.1.1 sequences have two substitutions (I50V and L67P) (Figure 5). Comparison of the deduced amino acids from reported sequences with those of the most similar published sequences revealed I50V, V79A, G110V, G123S, and S124G substitutions in the reported VI.2.1 sequences and K78R and A79V substitutions in the reported VII.1.1 sequences. Of note is the tendency of the amino acid substitutions in the reported VI.2.1 sequences to locate around the FPCS.
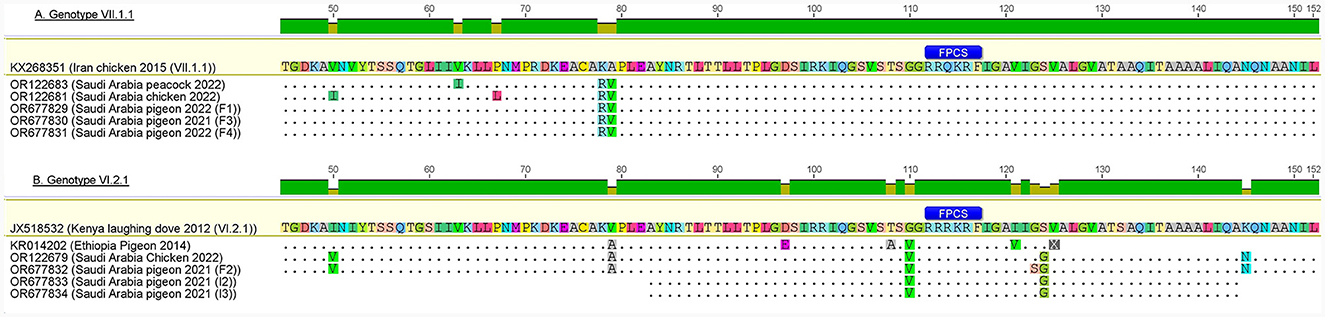
Figure 5. Amino acid substitutions in reported AOAV1-VI.2.1 and VII.1.1 sequences in comparison with reference and most similar sequences. (A) Genotype VII.1.1. (B) Genotype VI.2.1. The figure was prepared using Geneious 6.0.4 software (https://www.geneious.com).
4 Discussion
The poultry industry is a growing sector in Saudi Arabia, with an estimated production of 910,000 metric tons in 2021. It covered 60% of national market demand in 2020 and targets coverage of 85% by 2030. In 2021, mortalities of up to 8% of chickens were attributed to outbreaks of Newcastle disease (ND), that induced by AOAV1, and a few other viral diseases (30). AOAV1 has been known in Saudi Arabia for about four decades (19). The main goals of the current study were to investigate several outbreaks in some backyard pigeon flocks in the ERSA. It presents a detailed description of the clinical and postmortem records as well as the molecular detection and characterization of AOAV1 from naturally infected domestic pigeons in the ERSA. The phylogenetic analysis revealed the circulation of at least two AOAV1 genotypes (VI.2.1 and VII.1.1) in the investigated flocks. The present study showed the dominance of circulation of some neurotropic genotypes of AOAV1 (VI.2.1 and VII.1.1) in the investigated backyard pigeons.
Phylogenetic analysis of the reported sequences indicates that 3/6 sequences belong to genotype VII.1.1. These sequences clustered with previous detections from chickens in Iran, Pakistan, and Malaysia (32–34). Genotype VII was reported in commercial poultry in ERSA between 2012 and 2016 (22, 23). Genotype VII contains viruses responsible for the 4th ND panzootic (2) that started in the 1980s and affected Asia, Europe, Africa, and South America (35). As in many other regions of the world, viruses of genotype VII remain the leading cause of ND outbreaks in parts of the Middle East (5, 26, 36–38). Viruses of this genotype is well adapted to and maintained in chickens. It is also adapted to domestic waterfowl (35). Additionally, pigeons have been shown to be susceptible to VII.1.1 (VII d)-infection (18).
The other genotype reported in the present study, genotype VI.2.1, contains three sequences from backyard pigeons. These sequences clustered with those previously reported in backyard flocks in this region and those reported from pigeons in Ethiopia (39) in a separate cluster. Related isolates were detected in pigeons from Kenya and Nigeria in 2007–2013 (26). Similar sequences were also reported from China (GB# MN422262) and Iran (40). Genotype VI, otherwise termed Pigeon Paramyxovirus 1 (PPMV1), was reported in Saudi Arabia, the UAE, and Iran more than two decades ago (21). It was reported again in Iran in 2012 and thereafter (40). Viruses of this group are part of the 3rd ND panzootic that started in the Middle East, possibly in Iraq in 1978, and then spread to other parts of the world (3, 41). It is speculated that PPMV1 evolved from the chicken AOAV1 (3) and became adapted to columbiform (16). While circulating in the pigeon population, PPMV1 adaptation and virulence increase for pigeons and decrease for chickens (42). Beside columbiform and chickens, PPMV1 may infect many other wild birds and gallinaceous species (43, 44).
Though not detected in the present study, genotype XX was previously reported in Saudi Arabia, Iraq, Kuwait, and the UAE (21), as well as in other Asian and European countries (2). Similarly, genotypes XII, and XIII, and XXI were previously reported in the UAE and Iran (21, 40, 45) and were also reported from different countries in Asia, Europe, and Africa (2, 46).
The risk of disseminating virulent AOAV1 by wild pigeons and cormorants to poultry remains a major concern for a long time (43, 47). In the US, columbiform, especially rock doves, were involved in the maintenance of the PPMV1 (16). After infection, pigeons and cormorants may shed virulent AOAV1 for a long period, especially in faces, with no apparent clinical signs (43). PPMV1 is transmissible from infected pigeons to in-contact susceptible chickens (16, 48, 49). On infection with low-virulent PPMV1, chickens may shed the virus for up to 60 days (50). Regarding genotype VII.1.1 (VIId), it has been shown that pigeons are an efficient source of infection with this virus for in-contact chickens. Similarly, the mallard-originated VII.2 strain was shown to be readily transmissible to and between chickens (51). Recently, Hicks and colleagues conducted a phylodynamic analysis of the complete F gene sequences of AOAV1. Findings support the inter-species transmission of AOAV1-class II viruses, including those between domestic chicken and columbiform. Findings also support the intercontinental dispersion of the AOAV1-class II viruses, especially from South Asia to the Middle East (52). However, pigeons are not migratory birds and the way in which PPMV1 spreads over long distances is yet unknown. In this regard, it has been reported that closely related PPMV1 viruses were detected over the past few years from long-distant countries in Asia and Africa, including Nigeria, Egypt, Pakistan, Ukraine, Kazakhstan, and Russia (53).
PPMV1 usually shows low to moderate virulence in infected chickens (3, 16). In this regard, it has been reported that chickens near the AOAV1-VI-infected pigeons remained apparently healthy (54). After a few passages in chickens, PPMV1 eventually acquires some mutations that increase its adaptation and virulence in chickens, possibly by increasing its replication efficiency (48). However, some PPMV1 isolates were readily virulent for chickens (ICPI=1.51) with no need for prior adaptation (42). Variable ICPI scores were reported for pigeon derived PPMV1. A score mean of 1.44 and a range of 1.06 to 1.79 were reported (55). Nevertheless, lower score ranges were reported (3, 56) and wider score ranges were also reported (57). Regarding the pathogenicity of the pigeon-derived strains of the subgenotype VII.1.1, ICPI scores ranging between 1.34 and 1.96 were reported (58–60). Likewise, the previous detection of this genotype from Al-Ahsa showed ICPI score of 1.54 (22).
The present findings show that the natural infection of pigeons with AOAV1 genotype VII.1.1 induces nervus signs with greenish diarrhea and a mortality rate of 11.49%. This is in agreement with reported severe nervous signs and greenish diarrhea, along with mild respiratory signs and a mortality rate of 6.66% in pigeons after intranasal infection with the VII.1.1 virus (18). Genotype VII.1.1 strain (GB# DQ486859) replicated efficiently in the brain, cecal tonsil, trachea, and lungs of experimentally infected pigeons (61). This is also in agreement with findings showing that natural infection of pigeons with non-PPMV1 is associated with nervus signs and diarrhea (57). Nevertheless, experimental infection of pigeons with subgenotype VII.2 resulted in a 100% mortality rate. Clinical signs started with mild respiratory distress, followed by the development of nervous signs. Reported postmortem lesions include hemorrhage in proventriculus, lung, hepatic, and renal congestion (62).
The present findings showed the dominance of nervous signs and the presence of greenish diarrhea and respiratory symptoms in pigeons infected with genotype VI.2.1, with a significantly higher overall mortality rate (18.82%) than genotype VII.1.1 (11.49%). Natural infection of pigeons and doves with viruses of genotype VI.2.1 (nucleotide identity of >95%) was reported to induce Additionally, comparable nervous signs and mortality rates were observed on birds from which similar VI.2.1 sequences (> 95%) were recovered (56, 63, 64). Likewise, similar nervous signs were reported in pigeons infected with less similar isolates of genotype VI.2.1 (54, 65) and genotype VI.2.2.2.2 (66). Inconsistent clinical signs were reported after the experimental infection of pigeons with viruses of genotype VI. Pigeons infected with AOAV1-genotype VI.2.2.2.1 (GB# FJ766530) showed signs of general illness, like depression and anorexia, along with nervous symptoms and green diarrhea, with a mortality rate of 33% (67). Likewise, signs of general illness with greenish diarrhea but no mortality were also reported in pigeons infected with genotype VI.2.2.2.2 (68). On the other hand, depression and greenish diarrhea were reported in pigeons infected with strains of genotypes VI.2.1.1.2.1 and VI.2.2.2 with mortality rates of 80% and 60%, respectively (31). Nevertheless, signs of respiratory involvement in pigeons were observed following a natural infection with genotype VI.1.1 (69). Additionally, efficient viral replication in the brain, trachea, and intestine of pigeons experimentally infected with strains of genotypes VI.2.2.2.1 and VI.1.1 was reported (61, 70).
RT-PCR, followed by sequencing of the F gene, has been widely used to detect virulence and to connect AOAVs recovered from different outbreaks (65). The present study's genotyping RT-PCR failed to detect AOAV1 in samples from 2 out of the eight pigeon flocks. The false negativity of the F-based RT-PCR is well documented (71). High mutation rates and genetic variability, especially of the F gene, and the low viral RNA amount in clinical samples were reported as a possible explanations for false negative PCR results (50, 71). It has been reported that out of four validated RT-PCR assays, one was able to detect PPMV1 in all tested samples, while three assays failed to detect PPMV1 in at least one out of eight samples. PPMV1 detectability was lower in RT-PCR targeting the F gene than in those targeting the M gene. The main reason was the primer mismatching (50). A false negative result may induced by the mismatching of three nucleotides or more in a primer or probe (72, 73). On the other hand, a complete F gene sequence was recommended for the characterization of AOAV1. Dimitrov and others (2) emphasize the use of the complete F gene sequence, as well as other criteria, for the establishment of a new genotype or subgenotype. Diel and colleagues (74) propose the use of the complete F gene sequence for phylogenetic analysis. Using short F gene sequences but not the complete F gene sequences, may result in inconsistency in the topology of the phylogenetic tree (74). However, difficulties in amplifying the complete F gene of PPMV1 were previously reported (75). Additionally, Dimitrov-dataset-based phylogeny of partial F gene sequences with the right topology was previously achieved (76–82). In the present study, the topology of the obtained phylogenetic tree was maintained as that presented by Dimitrov and colleagues (2) with no out-grouping or relocation for the genotypes or subgenotypes. Additionally, each of the presented sequences aligned unambiguously and showed a similarity of >95% with reference sequences of only one subgenotype, according to the Dimitrov sequence dataset.
The deduced amino acid from reported sequences revealed the presence of polybasic amino acids (112RRR/QKR↓F117) in the FPCS, suggesting that the detected isolates are velogenic strains. Such motifs have been previously reported (83). Neutralizing epitopes at residues D72, E74, A75, K78, A79, 157SIAATNEAVHEVTDG171, and L343 were reported in the AOAV1 F protein (84–86). Two substitutions, K78R and A79V in genotype VII.1.1 and V79A in genotype VI.2.1, were reported in the present study. Similar substitutions were previously detected (GB# MH371035) in Israel and (GB# OR924274) in Bangladesh. Substitutions G123S and S124G in the fusion peptide and substitutions I50V and G110V in the F2 subunit were also observed in sequences of genotype VI.2.1 (87). Substitutions I50V and S124G were previously reported (88), as well as substitution G110V (GB#KR014202) and substitution G123S (GB#AF257759). Further research is required to identify the impact of these substitutions on the vaccine competence and virulence of the reported isolates.
AOAV1 represents a threat to domestic birds, especially commercial chickens, and wild birds, emphasizing the need to take steps to prevent the spread of the virus. Intervention may include increasing awareness among pigeon owners, implementing biosecurity measures, and vaccination. In Saudi Arabia, pigeons are not regularly vaccinated against AOAV1. Vaccination was not applied in the studied pigeon flocks, and vaccine-related strains were not detected. In contrast, vaccine-related strains were detected in commercial chicken farms (22) and wild birds, including pigeons (12). Nevertheless, viruses of genotype VI have shown some antigenic variance (2), while vaccination with genotype II strains and challenge with genotype VII.1.1 strain has shown incomplete protection (89). Inactivated PPMV1-based vaccines are recommended for pigeons to minimize their shedding for virulent AOAV1 (43). Sustainable monitoring of AOAV1 is necessary to elucidate epidemiology and take the necessary control steps. Measures to separate raised backyard species and to prevent Columbiformes from mixing or accessing water and food dedicated to poultry are also indicated to minimize inter-species transmission.
5 Conclusions
The present finding showed cocirculation of genotypes VI.2.1 and VII.1.1 in pigeons in Al-Ahsa, ERSA, with dominance of the neurological manifestations. It highlighted the differences in the clinical picture and mortality rates induced by AOAV1-genotypes VI.2.1 and VII.1.1 in naturally infected pigeons. These findings emphasize the need to evaluate and revise the vaccine schedules. Further research is required to understand the ecology and the different birds' roles in transmitting this virus.
Data availability statement
The original contributions presented in the study are included in the article, further inquiries can be directed to the corresponding authors.
Ethics statement
The animal studies were approved by King Fasial University Research Ethics Committee. The studies were conducted in accordance with the local legislation and institutional requirements. Written informed consent was obtained from the owners for the participation of their animals in this study.
Author contributions
AA-M: Conceptualization, Data curation, Formal analysis, Funding acquisition, Investigation, Methodology, Project administration, Resources, Software, Supervision, Validation, Visualization, Writing – original draft, Writing – review & editing. AA-K: Conceptualization, Data curation, Formal analysis, Investigation, Methodology, Software, Validation, Writing – original draft, Writing – review & editing. AS: Data curation, Formal analysis, Investigation, Methodology, Software, Validation, Writing – original draft. AA: Data curation, Formal analysis, Investigation, Methodology, Validation, Writing – original draft, Conceptualization, Writing – review & editing. JH: Conceptualization, Data curation, Formal analysis, Investigation, Methodology, Validation, Writing – original draft, Writing – review & editing, Software, Supervision. MK: Conceptualization, Data curation, Formal analysis, Investigation, Methodology, Software, Supervision, Validation, Writing – original draft, Writing – review & editing, Resources, Visualization. BF: Software, Writing – review & editing. MH: Conceptualization, Data curation, Formal analysis, Investigation, Methodology, Resources, Software, Supervision, Validation, Visualization, Writing – original draft, Writing – review & editing.
Funding
The author(s) declare financial support was received for the research, authorship, and/or publication of this article. This work was supported by King Abdul-Aziz City for Science and Technology [grant number 14-AGR1508-06].
Conflict of interest
The authors declare that the research was conducted in the absence of any commercial or financial relationships that could be construed as a potential conflict of interest.
The author(s) declared that they were an editorial board member of Frontiers, at the time of submission. This had no impact on the peer review process and the final decision.
Publisher's note
All claims expressed in this article are solely those of the authors and do not necessarily represent those of their affiliated organizations, or those of the publisher, the editors and the reviewers. Any product that may be evaluated in this article, or claim that may be made by its manufacturer, is not guaranteed or endorsed by the publisher.
Supplementary material
The Supplementary Material for this article can be found online at: https://www.frontiersin.org/articles/10.3389/fvets.2024.1352636/full#supplementary-material
References
1. Rima B, Balkema-Buschmann A, Dundon WG, Duprex P, Easton A, Fouchier R, et al. ICTV virus taxonomy profile: paramyxoviridae. J Gen Virol. (2019) 100:1593. doi: 10.1099/jgv.0.001328
2. Dimitrov KM, Abolnik C, Afonso CL, Albina E, Bahl J, Berg M, et al. Updated unified phylogenetic classification system and revised nomenclature for Newcastle disease virus. Infect Genet Evol. (2019) 74:103917. doi: 10.1016/j.meegid.2019.103917
3. Afonso CL. Virulence during Newcastle disease viruses cross species adaptation. Viruses. (2021) 13:110. doi: 10.3390/v13010110
4. WOAH. Chapter 2.3.14 newcastle disease (Infection with Newcastle Disease virus). In: Manual of Diagnostic Tests and Vaccines for Terrestrial Animals. Paris, France: World Organisation for Animal Health. (2012) p. 555–74.
5. Eid AA, Hussein A, Hassanin O, Elbakrey RM, Daines R, Sadeyen J-R, et al. Newcastle disease genotype VII prevalence in poultry and wild birds in Egypt. Viruses. (2022) 14:2244. doi: 10.3390/v14102244
6. Alexander DJ, Bell JG, Alders RG. A technology review: Newcastle disease. In: With Special Emphasis On Its Effect On Village Chickens. Rome: Food and Agriculture Organization of the United Nations (FAO). (2004).
7. Goebel SJ, Taylor J, Barr BC, Kiehn TE, Castro-Malaspina HR, Hedvat CV, et al. Isolation of avian paramyxovirus 1 from a patient with a lethal case of pneumonia. J Virol. (2007) 81:12709–14. doi: 10.1128/JVI.01406-07
8. Kuiken T, Breitbart M, Beer M, Grund C, Höper D, van den Hoogen B, et al. Zoonotic infection with pigeon paramyxovirus type 1 linked to fatal pneumonia. J Infect Dis. (2018) 218:1037–44. doi: 10.1093/infdis/jiy036
9. Alexander DJ. Ecology and epidemiology of Newcastle disease. In:Capua I, Alexander DJ, , editors. Avian Influenza and Newcastle Disease. Cham: Springer. (2009) p. 19–26.
10. Takakuwa H, ITO T, Takada A, Okazaki K, Kida H. Potentially virulent Newcastle disease viruses are maintained in migratory waterfowl populations. Jap J Vet Res. (1998) 45:207–15.
11. Cappelle J, Caron A, Almeida RSD, Gil P, Pedrono M, Mundava J, et al. Empirical analysis suggests continuous and homogeneous circulation of Newcastle disease virus in a wide range of wild bird species in Africa. Epidemiol Infect. (2015) 143:1292–303. doi: 10.1017/S095026881400185X
12. Ayala AJ, Dimitrov KM, Becker CR, Goraichuk IV, Arns CW, Bolotin VI, et al. Presence of vaccine-derived newcastle disease viruses in wild birds. PLoS ONE. (2016) 11:e0162484. doi: 10.1371/journal.pone.0162484
13. Zhu W, Dong J, Xie Z, Liu Q, Khan MI. Phylogenetic and pathogenic analysis of Newcastle disease virus isolated from house sparrow (Passer domesticus) living around poultry farm in southern China. Virus Genes. (2010) 40:231–5. doi: 10.1007/s11262-009-0436-0
14. Munir T, Aslam A, Zahid B, Ahmed I, Imran M, Ijaz M. Potential of commonly resident wild birds towards newcastle disease virus transmission. Pak Vet J. (2015) 35:106–7.
15. Rehan MA, Aslam MR, Khan M, Abid S, Hussain J, Amber, et al Potential economic impact of Newcastle disease virus isolated from wild birds on commercial poultry industry of Pakistan: A review. Hosts Virus. (2019) 6:1–15. doi: 10.17582/journal.hv/2019/6.1.1.15
16. Brown VR, Bevins SN, A. review of virulent Newcastle disease viruses in the United States and the role of wild birds in viral persistence and spread. Vet Res. (2017) 48:1–15. doi: 10.1186/s13567-017-0475-9
17. Ross CS, Sutton D, Skinner P, Mahmood S, Wynne F, Londt B, et al. Comparative pathogenesis of two genotype VI.2 avian paramyxovirus type-1 viruses (APMV-1) in pheasants, partridges and chickens. Avian Pathol. (2023) 52:36–50 doi: 10.1080/03079457.2022.2133680
18. Ellakany HF, Elbestawy AR, El-Hamid HSA, Zedan RE, Gado AR, Taha AE, et al. Role of pigeons in the transmission of avian avulavirus (newcastle disease-genotype VIId) to chickens. Animals. (2019) 9:338. doi: 10.3390/ani9060338
19. el-Zein A. Characterization of a velogenic Newcastle disease virus isolated from broilers in Saudi Arabia. Avian Dis. (1986) 30:825–8. doi: 10.2307/1590593
20. Aldous E, Fuller C, Mynn J, Alexander D, A. molecular epidemiological investigation of isolates of the variant avian paramyxovirus type 1 virus (PPMV-1) responsible for the 1978 to present panzootic in pigeons. Avian Pathol. (2004) 33:258–69. doi: 10.1080/0307945042000195768
21. Aldous EW, Mynn JK, Banks J, Alexander DJ, A. molecular epidemiological study of avian paramyxovirus type 1 (Newcastle disease virus) isolates by phylogenetic analysis of a partial nucleotide sequence of the fusion protein gene. Avian Pathol. (2003) 32:239–57. doi: 10.1080/030794503100009783
22. Almubarak AIA. Molecular and biological characterization of some circulating strains of Newcastle disease virus in broiler chickens from Eastern Saudi Arabia in 2012-2014. Veterinary World. (2019) 12:1668–76. doi: 10.14202/vetworld.2019.1668-1676
23. Al-Ali AM, El-Sabagh IM, Mohamed MH, Alluwaimi AM, Arif IA. Molecular characterization of common respiratory viral infections in broilers in Al-Hassa, Eastern Province, Saudi Arabia. Thai J Vet Med. (2018) 48:235–45. doi: 10.56808/2985-1130.2907
24. Anon. Guidelines for the Euthanasia of Animals: 2013 Edition. American Veterinary Medical Association (2013). Available online at: https://www.avma.org/KB/Policies/Documents/euthanasia.pdf (accessed June 3, 2018).
25. Wise MG, Suarez DL, Seal BS, Pedersen JC, Senne DA, King DJ, et al. Development of a real-time reverse-transcription PCR for detection of Newcastle disease virus RNA in clinical samples. J Clin Microbiol. (2004) 42:329–38.
26. Dimitrov KM, Ramey AM, Qiu X, Bahl J, Afonso CL. Temporal, geographic, and host distri-bution of avian paramyxovirus 1 (Newcastle disease virus). Infect Genet Evol. (2016) 39:22–34. doi: 10.1016/j.meegid.2016.01.008
27. Nanthakumar T, Kataria RS, Tiwari AK, Butchaiah G, Kataria JM. Pathotyping of Newcastle disease viruses by RT-PCR and restriction enzyme analysis. Vet Res Commun. (2000) 24:275–86. doi: 10.1023/A:1006403017578
28. Stäuber N, Brechtbühl K, Bruckner L, Hofmann MA. Detection of Newcastle disease virus in poultry vaccines using the polymerase chain reaction and direct sequencing of amplified cDNA. Vaccine. (1995) 13:360–4. doi: 10.1016/0264-410X(95)98257-B
29. Tamura K SG, Kumar S. MEGA11: Molecular evolutionary genetics analysis version 11. Mol Biol Evol. (2021) 38:3022–7. doi: 10.1093/molbev/msab120
30. Mousa H. Saudi Arabia: Poultry and Products Annual. Washington DC: United States Department of Agriculture. (2021) 09:2021.
31. Qian J, Liu X, Tang Z, Wang J, Wang Y, Wang W, et al. Genetic Characterization and Pathotypical Analysis of Two Strains of Pigeon Newcastle Disease Virus of Jiangsu in China. (2022). Available online at: https://assets.researchsquare.com/files/rs-1529999/v1/21d710a5-1479-4f44-b2f3-34181402db99.pdf?c=1649774803 (accessed February 13, 2024).
32. Molouki A, Mehrabadi MHF, Bashashati M, Akhijahani MM, Lim SHE, Hajloo SA, et al. subgenotype VII (L) is currently circulating in commercial broiler farms of Iran, 2017–2018. Trop Anim Health Prod. (2019) 51:1247–52. doi: 10.1007/s11250-019-01817-1
33. Syamsiah Aini S, Leow B, Faizul Fikri M, Muhammad Redzwan S, Faizah Hanim M. Identification of Newcastle Disease Virus sub-genotype VII 1. 1 isolated from chickens in Sabah, Malaysia. Trop Biomed. (2022) 39:579–86. doi: 10.47665/tb.39.4.015
34. Ali M, Khan M, Aslam A, Rehman H, Anjum A, Waheed S, et al. Molecular characterization and pathology of virulent newcastle disease virus isolated from broiler chickens in District Lahore, Pakistan. Indian J Animal Res. (2021) BF-1420:1–8. doi: 10.18805/IJAR.BF-1420
35. Xiang B, Chen L, Cai J, Liang J, Lin Q, Xu C, et al. Insights into genomic epidemiology, evolution, and transmission dynamics of genotype VII of class II newcastle disease virus in China. Pathogens. (2020) 9:837. doi: 10.3390/pathogens9100837
36. Shahsavandi S. Mohammad Majid Ebrahimi, Tebianian M. The predominance of newcastle disease virus genotype VII: genome diversity or poor cross-immunity of non-matched vaccines. Vaccine Res. (2021) 8:1–16. doi: 10.52547/vacres.8.2.4
37. Ababneh MM, Dalab AE, Alsaad SR, Al-Zghoul MB, Al-Natour MQ. Molecular characterization of a recent Newcastle disease virus outbreak in Jordan. Res Vet Sci. (2012) 93:1512–4. doi: 10.1016/j.rvsc.2012.03.004
38. Molouki A, Sotani M, Fallah Mehrabadi MH, Shoushtari A, Abtin A, Mahmoudzadeh Akhijahani M, et al. Predominance of fourth panzootic Newcastle disease virus subgenotype VII. 11 in Iran and its relation to the genotypes circulating in the region. Curr Microbiol. (2021) 78:3068–78. doi: 10.1007/s00284-021-02572-z
39. Damena D, Fusaro A, Sombo M, Belaineh R, Heidari A, Kebede A, et al. Characterization of Newcastle disease virus isolates obtained from outbreak cases in commercial chickens and wild pigeons in Ethiopia. Springerplus. (2016) 5:1–8. doi: 10.1186/s40064-016-2114-8
40. Molouki A, Soltani M, Akhijahani MM, Merhabadi MHF, Abtin A, Shoushtari A, et al. Circulation of at Least Six Distinct Groups of Pigeon-Derived Newcastle Disease Virus in Iran Between 1996 and 2019. Curr Microbiol. (2021) 78:2672–81. doi: 10.1007/s00284-021-02505-w
41. Pestka D, Stenzel T, Koncicki A. Occurrence, characteristics and control of pigeon paramyxovirus type 1 in pigeons. Polish J Vet Sci. (2014) 17:2. doi: 10.2478/pjvs-2014-0056
42. Nooruzzaman M, Barman LR, Mumu TT, Chowdhury EH, Dimitrov KM, Islam MR, et al. pigeon-derived sub-genotype XXI. 12 Newcastle disease virus from Bangladesh induces high mortality in chickens. Viruses. (2021) 13:1520. doi: 10.3390/v13081520
43. Miller PJ, Koch G. Newcastle Disease. In:Swayne DE, , editor. Diseases of Poultry Fourteenth ed. Hoboken, NJ: John Wiley & Sons, Inc. (2020).
44. Irvine RM E. W., Aldous R. J., Manvell W. J., Cox V., Ceeraz C. M., Fuller, et al. Outbreak of Newcastle disease due to pigeon paramyxovirus type 1 in grey partridges (Perdix perdix) in Scotland in October 2006. Vet Rec. (2009) 165:531–5. doi: 10.1136/vr.165.18.531
45. Hirschinger J, Munoz MC, Hingrat Y, Vergne T, Guerin J-L. Le Loc'h G. Exposure to and circulation of avian influenza and newcastle disease viruses in peridomestic wild birds in the United Arab Emirates. J Wildlife Dis. (2020) 56:437–42. doi: 10.7589/2019-06-164
46. Moustapha A, Talaki E, Akourki A, Ousseini M. Newcastle disease virus in poultry: current status and control prospects. World's Vet J. (2023) 13:240–9. doi: 10.54203/scil.2023.wvj26
47. Liao YS. Studies on the Nature of Newcastle Disease Virus. Kansas: Kansas State University. (1951).
48. Dortmans JCFM. Virulence Determinants of Newcastle Disease Virus (Doctoral dissertation, Utrecht University) (2011). Available online at: https://dspace.library.uu.nl/bitstream/handle/1874/194128/dortmans.pdf;sequence=1 (accessed February 13, 2024).
49. Kommers G, King D, Seal B, Carmichael K, Brown C. Pathogenesis of six pigeon-origin isolates of Newcastle disease virus for domestic chickens. Vet Pathol. (2002) 39:353–62. doi: 10.1354/vp.39-3-353
50. Hüppi L, Ruggli N, Python S, Hoop R, Albini S, Grund C, et al. Experimental pigeon paramyxovirus-1 infection in chicken: Evaluation of infectivity, clinical and pathological manifestations and diagnostic methods. J General Virol. (2020) 101:156–67. doi: 10.1099/jgv.0.001364
51. Habib M, Yaqub T, Sohail T, Shahbaz M, Shehzad W, Munir M, et al. Evaluation of transmission potential and pathobiological characteristics of mallard originated Avian orthoavulavirus 1 (sub-genotype VII. 2) in commercial broilers. Microbial Pathog. (2019) 137:103785. doi: 10.1016/j.micpath.2019.103785
52. Hicks JT, Dimitrov KM, Afonso CL, Ramey AM, Bahl J. Global phylodynamic analysis of avian paramyxovirus-1 provides evidence of inter-host transmission and intercontinental spatial diffusion. BMC Evol Biol. (2019) 19:1–15. doi: 10.1186/s12862-019-1431-2
53. Sabra M, Dimitrov KM, Goraichuk IV, Wajid A, Sharma P, Williams-Coplin D, et al. Phylogenetic assessment reveals continuous evolution and circulation of pigeon-derived virulent avian avulaviruses 1 in Eastern Europe, Asia, and Africa. BMC Vet Res. (2017) 13:291. doi: 10.1186/s12917-017-1211-4
54. Thomazelli LM, Sinhorini JA, Oliveira DB, Knöbl T, Bosqueiro TC, Sano E, et al. An outbreak in pigeons caused by the subgenotype VI. 21 2 of Newcastle disease virus in Brazil. Viruses. (2021) 13:2446. doi: 10.3390/v13122446
55. Alexander DJ, Russell PH, Parsons G, Abu Elzein EME, Ballouh A, Cernik K, et al. Antigenic and biological characterisation of avian paramyxovirus type I isolates from pigeons - an international collaborative study. Avian Pathol. (1985) 14:365–76. doi: 10.1080/03079458508436238
56. Meulemans G, van den Berg TP, Decaesstecker M, Boschmans M. Evolution of pigeon Newcastle disease virus strains. Avian Pathol. (2002) 31:515–9. doi: 10.1080/0307945021000005897
57. O. Werner A., Römer-Oberdörfer B., Köllner, Manvell RJ, Alexander DJ. Characterization of avian paramyxovirus type 1 strains isolated in Germany during 1992 to 1996. Avian Pathol. (1999) 28:79–88. doi: 10.1080/03079459995082
58. Liu H, Wang Z, Song C, Wang Y, Yu B, Zheng D, et al. Characterization of pigeon-origin Newcastle disease virus isolated in China. Avian Dis. (2006) 50:636–40. doi: 10.1637/7618-042606R1.1
59. Qin Z-M, Tan L-T, Xu H-Y, Ma B-C, Wang Y-L, Yuan X-Y, et al. Pathotypical characterization and molecular epidemiology of newcastle disease virus isolates from different hosts in China from 1996 to 2005. J Clin Microbiol. (2008) 46:601–11. doi: 10.1128/JCM.01356-07
60. Lebdah M TL, Elgamal AM, Mohamed M, Elsafty MM, Elhusseiny MH, Mohamed ME. Molecular detection and characterization of virulent newcastle disease viruses from different avian species in Egypt. Int J Vet Sci. (2022) 11:189–95. doi: 10.47278/journal.ijvs/2021.084
61. Kang Y, Xiang B, Yuan R, Zhao X, Feng M, Gao P, et al. Phylogenetic and pathotypic characterization of Newcastle disease viruses circulating in South China and transmission in different birds. Front Microbiol. (2016) 7:119. doi: 10.3389/fmicb.2016.00119
62. Aziz-ul-Rahman, Mohammed A, Rohaim Rania F., El Naggar, Ghulam Mustafa, Chaudhry U, Shabbir MZ. Comparative clinicopathological assessment of velogenic (sub-genotype VIIi) and mesogenic (sub-genotype VIm) Avian avulavirus 1 in chickens and pigeons. Avian Pathol. (2019) 48:610–21. doi: 10.1080/03079457.2019.1648751
63. Borm SV, Obishakin E, Joannis T, Lambrecht B. Berg Tvd. Further evidence for the widespread co-circulation of lineages 4b and 7 velogenic Newcastle disease viruses in rural Nigeria. Avian Pathol. (2012) 41:377–82. doi: 10.1080/03079457.2012.696311
64. Obanda V, Michuki G, Jowers MJ, Rumberia C, Mutinda M, Lwande OW, et al. Complete genomic sequence of virulent pigeon paramyxovirus in laughing doves (Streptopelia senegalensis) in Kenya. J Wildl Dis. (2016) 52:599–608. doi: 10.7589/2015-07-199
65. Souza SO, Fredo G, Dupont PM, Leite-Filho RV, Teifke JP, Pavarini SP, et al. Pathological and molecular findings of avian avulavirus type 1 outbreak in pigeons (Columba livia) of southern Brazil. Pesquisa Veterinária Brasileira. (2018) 38:2254–61. doi: 10.1590/1678-5150-pvb-5528
66. Mansour SM, ElBakrey RM, Mohamed FF, Hamouda EE, Abdallah MS, Elbestawy AR, et al. Avian paramyxovirus type 1 in Egypt: epidemiology, evolutionary perspective, and vaccine approach. Front Vet Sci. (2021) 8:647462. doi: 10.3389/fvets.2021.647462
67. Zhan T, Hu S, Chen Y, Lu X, Liao T, Guo L, et al. Comparative pathogenicity of two closely related Newcastle disease virus isolates from chicken and pigeon respectively. Virus Res. (2020) 286:198091. doi: 10.1016/j.virusres.2020.198091
68. Xue C, Xu X, Yin R, Qian J, Sun Y, Wang C, et al. Identification and pathotypical analysis of a novel VIk sub-genotype Newcastle disease virus obtained from pigeon in China. Virus Res. (2017) 238:1–7. doi: 10.1016/j.virusres.2017.05.011
69. Guo H, Liu X, Han Z, Shao Y, Chen J, Zhao S, et al. Phylogenetic analysis and comparison of eight strains of pigeon paramyxovirus type 1 (PPMV-1) isolated in China between 2010 and 2012. Arch Virol. (2013) 158:1121–31. doi: 10.1007/s00705-012-1572-8
70. Chang H, Feng S, Wang Y, Li F, Su Q, Wang B, et al. Isolation and pathogenic characterization of pigeon paramyxovirus type 1 via different inoculation routes in pigeons. Front Vet Sci. (2021) 7:569901. doi: 10.3389/fvets.2020.569901
71. Cattoli G, Susta L, Terregino C, Brown C. Newcastle disease: a review of field recognition and current methods of laboratory detection. J Vet Diagn Investigat. (2011) 23:637–56. doi: 10.1177/1040638711407887
72. Cattoli G, Fusaro A, Monne I, Molia S, Le Menach A, Maregeya B, et al. Emergence of a new genetic lineage of Newcastle disease virus in West and Central Africa implications for diagnosis and control. Vet Microbiol. (2010) 142:168–76. doi: 10.1016/j.vetmic.2009.09.063
73. Wiseman A, Shabbat M, Cohen A, From I, Almog Samina Y, Sade Levy E, et al. Point mutation revealed the resurgence of sub-genotype VII. 2 Newcastle disease virus in Israel. Avian Pathol. (2022) 51:236–43. doi: 10.1080/03079457.2022.2040730
74. Diel D, Silva L, Liu H, Wang Z, Miller P, Afonso C. Genetic diversity of avian paramyxovirus Type 1: Proposal for a unified nomenclature and classification system of Newcastle disease virus genotypes. Infect Genet Evol. (2012) 12:1770–9. doi: 10.1016/j.meegid.2012.07.012
75. Goraichuk IV, Gerilovych A, Bolotin V, Solodiankin O, Dimitrov KM, Rula O, et al. Genetic diversity of Newcastle disease viruses circulating in wild and synanthropic birds in Ukraine between 2006 and 2015. Front Vet Sci. (2023) 10:1026296. doi: 10.3389/fvets.2023.1026296
76. Al-Shammari AM, Hamad MA, AL-Mudhafar MA, Raad K, Ahmed A. Clinical, molecular and cytopathological characterization of a Newcastle disease virus from an outbreak in Baghdad, Iraq. Vet Med Sci. (2020) 6:477–84. doi: 10.1002/vms3.262
77. Msoffe PL, Chiwanga GH, Cardona CJ, Miller PJ, Suarez DL. Isolation and characterization of Newcastle disease virus from live bird markets in Tanzania. Avian Dis. (2019) 63:634–40. doi: 10.1637/aviandiseases-D-19-00089
78. Abd Elfatah KS, Elabasy MA, El-Khyate F, Elmahallawy EK, Mosad SM, El-Gohary FA, et al. Molecular characterization of velogenic Newcastle disease virus (sub-genotype VII. 11) from wild birds, with assessment of its pathogenicity in susceptible chickens. Animals. (2021) 11:505. doi: 10.3390/ani11020505
79. Kabir H, Hakim H, Alizada MN, Hasan A, Miyaoka Y, Yamaguchi M, et al. Isolation, identification, and molecular characterization of newcastle disease virus from field outbreaks in chickens in Afghanistan. Avian Dis. (2022) 66:176–80. doi: 10.1637/aviandiseases-D-22-00002
80. Turan N, Ozsemir C, Yilmaz A, Cizmecigil UY, Aydin O, Bamac OE, et al. Identification of Newcastle disease virus subgenotype VII. 2 in wild birds in Turkey. BMC Vet Res. (2020) 16:1–8. doi: 10.1186/s12917-020-02503-3
81. Henriques AM, Neto A, Fagulha T, Almeida V, Fevereiro M. Molecular characterization and phylogenetic analysis of Newcastle disease viruses isolated in southern Angola, 2016-2018. Inf Genet Evolut. (2023) 113:105481. doi: 10.1016/j.meegid.2023.105481
82. Rtishchev A, Treshchalina A, Shustova E, Boravleva E, Gambaryan A. An outbreak of Newcastle disease virus in the moscow region in the summer of 2022. Vet Sci. (2023) 10:404. doi: 10.3390/vetsci10060404
83. Bello MB, Yusoff KM, Ideris A, Hair-Bejo M, Peeters BP, Jibril AH, et al. Genotype diversity of Newcastle disease virus in Nigeria: Disease control challenges and future outlook. Adv Virol. (2018) 2018:6097291. doi: 10.1155/2018/6097291
84. Yusoff K, Nesbit M, McCartney H, Meulemans G, Alexander D, Collins M, et al. Location of neutralizing epitopes on the fusion protein of Newcastle disease virus strain Beaudette C. J Gen Virol. (1989) 70:3105–9. doi: 10.1099/0022-1317-70-11-3105
85. Neyt C, Geliebter J, Slaoui M, Morales D, Meulemans G, Burny A. Mutations located on both F1 and F2 subunits of the Newcastle disease virus fusion protein confer resistance to neutralization with monoclonal antibodies. J Virol. (1989) 63:952–4. doi: 10.1128/jvi.63.2.952-954.1989
86. Toyoda T, Gotoh B, Sakaguchi T, Kida H, Nagai Y. Identification of amino acids relevant to three antigenic determinants on the fusion protein of Newcastle disease virus that are involved in fusion inhibition and neutralization. J Virol. (1988) 62:4427–30. doi: 10.1128/jvi.62.11.4427-4430.1988
87. Abd El-Hamid HS, Shafi ME, Albaqami NM, Ellakany HF, Abdelaziz NM, Abdelaziz MN, et al. Sequence analysis and pathogenicity of Avian Orthoavulavirus 1 strains isolated from poultry flocks during 2015–2019. BMC Vet Res. (2020) 16:1–15. doi: 10.1186/s12917-020-02470-9
88. Castro ER, Zanetti F, Arbiza J. Genetic characterization of a pigeon paramyxovirus type 1 isolated from Columba livia in Uruguay. Aviand Isease5. (2012) 56:243–8. doi: 10.1637/9835-061611-Case.1
Keywords: Avian Orthoavulavirus-1 (AOAV1), pigeons, natural infection, RT-PCR, genotype VI, genotype VII, neurotropic, viscerotropic
Citation: Al-Mubarak AIA, Al-Kubati AAG, Sheikh A, Abdelaziz AM, Hussen J, Kandeel M, Falemban B and Hemida MG (2024) Detection of Avian Orthoavulavirus-1 genotypes VI.2.1 and VII.1.1 with neuro-viscerotropic tropism in some backyard pigeons (Columbidae) in Eastern Saudi Arabia. Front. Vet. Sci. 11:1352636. doi: 10.3389/fvets.2024.1352636
Received: 08 December 2023; Accepted: 06 February 2024;
Published: 27 February 2024.
Edited by:
Yasser Mahmmod, Higher Colleges of Technology, United Arab EmiratesReviewed by:
Denys Muzyka, Institute of Experimental and Clinical Veterinary Medicine, UkraineMieke Steensels, Sciensano, Belgium
Copyright © 2024 Al-Mubarak, Al-Kubati, Sheikh, Abdelaziz, Hussen, Kandeel, Falemban and Hemida. This is an open-access article distributed under the terms of the Creative Commons Attribution License (CC BY). The use, distribution or reproduction in other forums is permitted, provided the original author(s) and the copyright owner(s) are credited and that the original publication in this journal is cited, in accordance with accepted academic practice. No use, distribution or reproduction is permitted which does not comply with these terms.
*Correspondence: Abdullah I. A. Al-Mubarak, aialmubark@kfu.edu.sa; Maged Gomaa Hemida, maged.hemida@liu.edu
†ORCID: Abdullah I. A. Al-Mubarak orcid.org/0000-0003-3773-7447
Anwar A. G. Al-Kubati orcid.org/0000-0001-9681-0777
Abdullah Sheikh orcid.org/0000-0002-0482-503X
Adel M. Abdelaziz orcid.org/0000-0001-7874-5764
Jamal Hussen orcid.org/0000-0001-8942-005X
Mahmoud Kandeel orcid.org/0000-0003-3668-5147
Baraa Falemban orcid.org/0000-0001-5591-2689
Maged Gomaa Hemida orcid.org/0000-0002-5986-7237