- 1Faculty of Veterinary Medicine, Chiang Mai University, Chiang Mai, Thailand
- 2Center of Elephant and Wildlife Health, Chiang Mai University Animal Hospital, Chiang Mai University, Chiang Mai, Thailand
- 3Elephant, Wildlife, and Companion Animals Research Group, Chiang Mai University, Chiang Mai, Thailand
- 4Smithsonian Conservation Biology Institute, Center for Species Survival, Front Royal, VA, United States
- 5Department of Medical Technology, Faculty of Associated Medical Sciences, Chiang Mai University, Chiang Mai, Thailand
- 6Small Animal Hospital, Faculty of Veterinary Medicine, Chiang Mai University, Chiang Mai, Thailand
The international travel ban instituted by the Thai government in March 2020 in response to the COVID-19 pandemic greatly affected how tourist camp elephants were managed, with reductions in exercise opportunities, longer chaining hours, and diminished food provisioning. This study was conducted to determine how those changes affected health and welfare biomarkers in individual elephants over the 2 years of the countrywide lockdown (April 2020–April 2022). Blood and fecal samples were collected from 58 elephants at six camps (monthly in Year 1, quarterly in Year 2) and analyzed for stress biomarkers — fecal glucocorticoid metabolites (fGCM), serum oxidative stress [malondialdehyde (MDA) and 8-hydroxy-2′-deoxyguanosine (8-OHdG)], and stress leukograms. Overall, fGCM concentrations increased within the first few months and remained higher than pre-COVID levels, as did the H/L ratio, a measure affected by cortisol. Serum 8-OHdG, an indicator of DNA oxidative damage, also increased over time, while monocytosis and lymphopenia further suggested alterations in immune function as a result of stress. By contrast, another marker of oxidative stress, serum MDA, declined, possibly in response to reduced roughage and supplement intake. A notable finding was a seasonal pattern of fGCM that was significantly different from previous studies. Whereas higher fGCM during the rainy season were observed in this study, previously, concentrations were highest during the winter, high tourist season. Thus, ironically, both the presence and absence of tourists have been associated with increased fGCM concentrations, albeit for different reasons. Camp management factors negatively affecting stress outcomes included shorter chain lengths, longer chain hours, lack of exercise, and reduced roughage and supplements. Overall, it was clear that camps struggled to maintain adequate care for elephants during the COVID-19 pandemic, highlighting the importance of tourist income and need for contingency plans to cope with potential future disruptions to tourism.
Introduction
The Asian elephant (Elephas maximus) is classified as endangered on the International Union for Conservation of Nature (IUCN) Red List of Threatened Species™ (2016) and listed under Appendix I by the Convention on International Trade in Endangered Species of Wild Fauna and Flora (1). In Thailand, the elephant is revered as a national symbol and plays a crucial role in Thai cultural traditions and Buddhist practices, while also significantly contributing to the country’s economic landscape. Presently, Thailand is home to approximately 3,500 captive elephants, with the majority (~95%) under private ownership and engaged primarily in tourism (2). Most (~60%) are in the northern and northeastern regions of the country, predominantly in Chiang Mai province (3). Tourist activities vary and include hands-off opportunities like observation from afar or walking alongside to more interactive activities like feeding, bathing, riding with a saddle or bareback, and elephant shows (4). Our previous studies of how tourism affects elephant health and welfare found that feeding high energy foods (banana and sugar cane) was associated with obesity and alterations in lipid profiles and metabolic function (insulin, glucose, fructosamine, glucose to insulin ratio), while fecal glucocorticoid metabolite (fGCM) concentrations as a proxy for stress were actually lower in riding elephants in relation to more exercise and better body condition (5–7), similar to findings in U.S. zoos (8–10). Poor foot scores were associated with longer work hours and walking distances, and being on concrete, while skin wounds were related to improper restrain equipment used by mahouts (e.g., ankus/bullhook, chains) and higher fGCM (11). Thus, while some tourist activities may be a benefit to elephant health (5–7), others can contribute to poor welfare through long work hours, misuse of the ankus, stress associated with being too close to tourists, and harsh training to allow hands-on interactions (11, 12). Based on these empirical findings, a set of welfare guidelines was developed for the Asian Elephant Specialist Group (13) and adapted by Captive Elephant Standards1 that conducts on-demand welfare audits of elephant camps throughout southeast Asia. As a result, improvements in tourist camp management based on these research findings were beginning to take hold (14). Then the pandemic hit.
When SARS-CoV-2, the virus that causes COVID-19, was recognized by Thailand in March 2020 (12), the tourism landscape changed drastically. The Thai government banned all international travel, severely reducing foreign tourism and associated income (15). Consequently, all tourist camps closed, leading to further concerns over welfare of elephants and mahouts (elephant keepers). In a previous study, surveys were conducted throughout the first 2 years of the country-wide lockdown (15), with data compared to before COVID-19 (4) and identified significant changes in camp structure and elephant management. Elephant activities, distance walked, and amounts of food were reduced, while chain hours were increased. Thus, effects on animal health were expected. Indeed, changes in several health biomarkers were observed, including lower serum creatine kinase and increased liver enzyme, AST and ALP, concentrations possibly due to physical inactivity or altered nutrition. By contrast, reduced feeding of bananas and sugar cane was associated with lower body condition scores (BCS), less obesity, and lower TG concentrations (16) suggesting some physical improvements. This companion study further examines patterns of several stress biomarkers throughout the 2-year COVID-19 tourism ban to examine effects on health and welfare.
Exposure to harmful stimuli over time can lead to adverse effects on animal well-being (17), generally through activation of the hypothalamo-pituitary–adrenal (HPA) axis and resulting secretion of glucocorticoids (GC) from the adrenal cortex (18). Downstream effects of increased GC production include suppression of immune function (19, 20), reproduction (21–23), increased disease susceptibility (19, 24, 25), and altered carbohydrate metabolism (26, 27). Hematology changes also can occur with stress, including an increase in the neutrophil to lymphocyte (N/L) ratio (28, 29). Neutrophils (or heterophils, depending on the species) and lymphocytes are involved in immunological processes and constitute almost 80% of all white blood cells in mammals (30). Interestingly, in elephants and other Afrotheria like hyraxes and manatees, heterophils are produced instead of neutrophils (31). Thus, in those species, heterophil to lymphocyte (H/L) ratios are calculated instead. Leukocyte numbers react to stress, but in different directions: e.g., heterophils increase, while lymphocyte numbers decrease during a stressful event (32, 33). Studies further show that an increase in GC can drive changes in heterophil and lymphocyte numbers (32–34). Indeed, one study found a positive relationship between the H/L ratio and fecal GC concentrations (fGCM) in logging Asian elephants in Myanmar, irrespective of sex, age, or environmental context (35). Stress leukograms vary among species but are typically characterized by lymphopenia and neutrophilia, with occasional monocytosis and eosinopenia (36). Serum malondialdehyde (MDA), a byproduct of lipid peroxidation also has been associated with high levels of GC hormones (37, 38). An increase in free radicals causes overproduction of MDA, so it is commonly used as a marker of oxidative stress and antioxidant status (39) and could be measured in addition to GCs to assess welfare in working elephants. Finally, 8-hydroxy-2′-deoxyguanosine (8-OHdG) is a biomarker of DNA oxidative damage and formed when oxygen radicals damage DNA. It can be measured in blood, as well as noninvasively in urine and feces, and so has become another potential marker of welfare (40–43).
Given that changes in metabolic health were observed in tourist elephants during the COVID-induced travel ban (16), the goals of this study were to evaluate how stress biomarkers (GCs, serum MDA, serum 8-OHdG, stress leukograms, and H/L ratio) were affected in these same elephants. As in the previous study, some biomarker and BCS data were compared to earlier studies on tourist elephants before the pandemic (5–7, 12, 34, 44–47). Ultimately, it is hoped that this information will be used to develop management plans that can ensure good welfare of elephants with or without tourists, and contribute to strategies that improve overall health, welfare and population sustainability within the tourism paradigm.
Materials and methods
Human and animal ethics statements
The research received approval from the Human Research Ethics Committee (HS1/2564) and the Institutional Animal Care and Use Committee at the Faculty of Veterinary Medicine (FVM), Chiang Mai University (CMU), located in Chiang Mai, Thailand (FVM-ACUC; S4/2564).
Study population
A total of 58 adult elephants (14 males and 44 females) from six elephant camps in the Mae Taeng district of Chiang Mai province was studied and initially described by Supanta et al. (15, 16) (Table 1). Preceding the nationwide lockdown, elephants participated in five tourist-related activities: riding with a saddle, riding bareback, elephant shows, feeding, bathing or observation only. As a result of the lockdown, all tourist-related activities ceased. The inclusion criteria for elephants in this study were: adult age (18–50 years); deemed healthy by camp veterinarians or veterinary consultants; females were not pregnant. Samples were not collected from males during musth for safety reasons. These are the same elephants included in the two companion papers (15, 16).
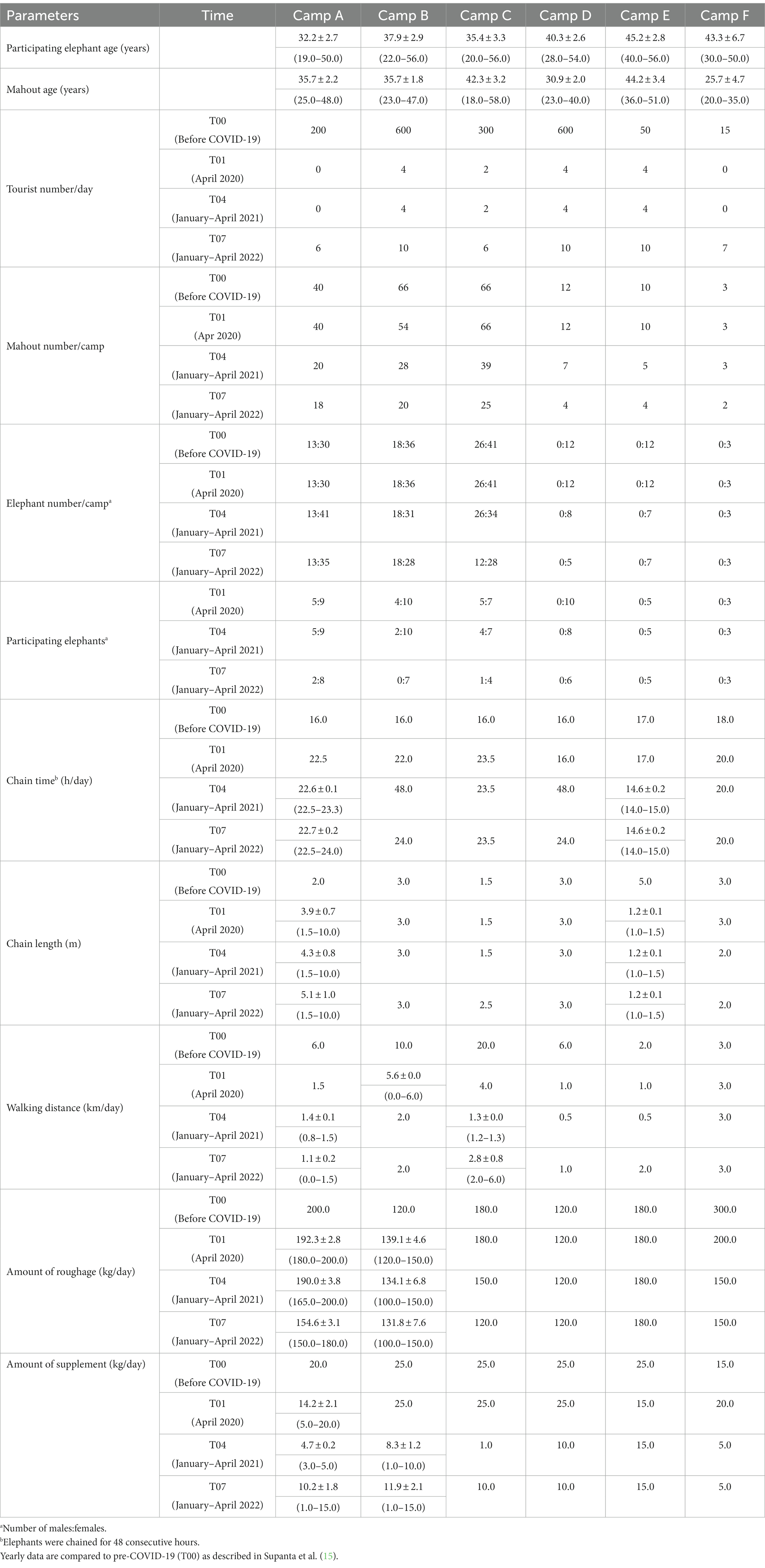
Table 1. Summary (mean ± SEM, range) of tourist, elephant and mahout numbers, and elephant management at six Thailand tourist camps during the COVID-19 pandemic and international travel ban between April 2020 and April 2022.
Data collection
Data collection for this prospective study began in April 2020 (T01), a month after the implementation of a travel ban due to the COVID-19 pandemic, and continued through April 2022 (T07) as camps began to open again to tourists. The study consisted of in-person interviews with camp owners, veterinarians and/or mahouts (elephant keepers) to gather information about the numbers of tourists, elephants, and mahouts, tourist activities (riding, walking, shows, feeding), chaining duration and length, and provisioning of roughage and supplements (14). The questionnaire is shown in Table 1. Interviews were conducted, and samples were collected monthly for the first year [April 2020 to April 2021 (T01-T04)] and then every 4 months during the second year [May 2021 to April 2022 (T05-T07)]. This methodology led to a total of 16 on-site visits that were subsequently segmented into seven time periods for analysis [April 2020 (T01), May–August 2020 (T02), September–December 2020 (T03), January–April 2021 (T04), May–August 2021 (T05), September–December 2021 (T06), January–April 2022 (T07)] as described previously (15, 16). Queries about camp management before COVID-19 were included in the first interview to capture data on operations in 2019 (16). A total of seven camp managers, 50 mahouts, and two veterinarians were interviewed across the 16 data collection periods. Supplementary information on camp management and elephant activities pre-COVID-19 was sourced from Bansiddhi et al. (4, 11, 12) and Norkaew et al. (5–7).
Fecal collection
Fecal samples were collected monthly in the morning between 08.00 to 12.00 h to avoid variation in the circadian rhythm of cortisol secretion (23, 48, 49). Defecation was either observed or indicated to have occurred within the hour by the mahout. Fecal boluses were broken open and approximately 50 grams of material taken from the middle and placed into zip lock plastic bags labeled with the elephant name, time of collection and date. Samples were transported at 2–8°C in a cool box to the Faculty of Veterinary Medicine, Chiang Mai University and then stored at −20°C until fecal extraction and hormonal analysis.
Blood collection
Blood (~8 mL) was collected from a caudal auricular vein and placed into two tubes: serum separator tubes (SST) (~7 mL) for analysis of oxidative stress (MDA, 8-OHdG), and ethylenediaminetetraacetic acid tubes (EDTA) (~1 mL) for the stress leukogram (heterophils, lymphocytes, monocytes and eosinophils). Blood in SST tubes was centrifuged at 1,200×g for 15 min within 6 h of collection (50), while whole EDTA blood was analyzed within 24 h of blood smear preparation. Serum was stored at −20°C until processing and analysis.
Fecal extraction and glucocorticoid analysis
Fecal samples were extracted as described by Norkaew et al. (5). Frozen feces were thawed at room temperature (RT) and dried in a conventional oven (60°C) for 24–48 h. Mixed powdered feces (~0.1 g) were placed into glass tubes, 4.5 mL of EtOH and 0.5 mL of distilled water was added, and tubes were vortexed briefly. Samples were extracted by boiling in a water bath (90°C) for 20 min, with 95% EtOH added to keep the volume at 5 mL, and then centrifuged at 1,200×g for 20 min. The fecal extracts were combined, dried in a 90°C water bath, re-suspended in 3 mL of 95% EtOH, dried down again, and finally re-suspended in 1 mL of 50% methanol. Samples were stored at −20°C until analysis.
Concentrations of fGCM were measured in extracts diluted 1:3 in assay buffer (0.0137 M Trizma base, 0.2 M Tris–HCl, 0.2 M NaCl, 0.2 M EDTA, 0.001% BSA, and 0.001% Tween 20; pH 7.5) using a double-antibody EIA with a polyclonal rabbit anti-corticosterone antibody (CJM006, Coralie Munro, UC Davis, CA) previously used in Thailand (5). Samples and corticosterone standards (50 μL) were added to wells in duplicate followed by corticosterone-HRP (25 μL; 1:30,000) and anti-corticosterone antibody (25 μL, 1:100,000). Plates were incubated in the dark at RT for 2 h, followed by 100 μL of TMB solution and incubation for 20–35 min. Stop solution was added (50 μL, 1 N HCl) and absorbance measured at 450 nm (TECAN, Männedorf, Switzerland). Assay sensitivity was 0.12 ng/mL. Intra- (at 90% binding) and inter-assay coefficients of variation (CV, based on dose values) were < 10% (all duplicates over 10% were reanalyzed) and 12.28%, respectively.
Oxidative stress
MDA was quantified by a thiobarbituric acid (TBA)-reacting substances (TBARS) assay described previously (51, 52). Briefly, 50 μL of serum samples and standards were mixed with 750 μL of 0.44 M phosphoric acid, 250 μL of 42 mM TBA and 450 μL of distilled water. Tubes were placed into a boiling water bath for 15 min, cooled on ice for 5 min, and then centrifuged at 900×g for 5 min. Absorbance of the supernatant was measured at 532 nm using a UV–VIS spectrophotometer (Shimadzu, Japan). Serum MDA concentrations were calculated from standard curves of MDA equivalents generated by the acid-catalyzed hydrolysis of 1,1,3,3-tetramethoxypropane (TMP) within the range of 1.25–80.00 μM. Assay sensitivity was 0.15 μM. Intra- and inter-assay coefficients of variation (CV) were 1.1 and 2.3%, respectively.
Serum 8-OHdG was measured using a DetectX® DNA Damage Enzyme Immunoassay Kit (Cat #K059-H5, Arbor Assays) validated for Asian elephants (52, 53). Assay sensitivity was 0.072 ng/mL and intra-assay and inter-assay CVs were < 10 and 6.3%, respectively.
Stress leukogram
White blood cell count differentials were done manually on blood smears stained with Wright–Giemsa (RVL Supply, Pathumthani, Thailand) (45). A ratio of heterophils to lymphocytes was calculated for each sample.
Statistical analyses
Elephant data from management surveys are presented as mean ± standard error of the mean (SEM) and a range depending on the type of data. Statistical analyses were conducted using R version 4.3.1 (54) package geepack, function geeglm, under the optimal autoregressive order 1 correlation structure (55). Repeated measures data were analyzed using Generalized Estimating Equations (GEE) to determine the effect of camp management practices on stress parameters. A univariate analysis was performed, and any variable with a significance level of p < 0.15 was included in the subsequent multivariate analysis. Post hoc tests were conducted using Tukey’s multiple comparisons of least-squares mean (LS-mean) to identify differences between variable categories. For the multivariate model, Sex and Age were incorporated as covariate factors. The selection of the final model in the GEE analysis was determined based on the smallest quasi-likelihood under the independence model criterion (QIC) values (56) using package MuMIn, function dredge (57) with the significance set at α = 0.05. Reference categories were chosen based on specific questions as described previously (11, 12), including Sex = male; Time = T01 (April 2020, the beginning of the travel ban); and Camp = F (the smallest camp). Differences in mean fGCM, oxidative stress markers, stress leukograms and H/L ratio among three seasons (summer: February 16–May 15; rainy: May 16–October 15; winter: October 16–February 15) (53, 58, 59) were analyzed by Tukey’s post-hoc tests following GEE analyses.
Results
Descriptive statistics of camp management variables
Camp differences in management practices before the COVID-19 travel ban (T00) compared to the start of the lockdown (T01) and each subsequent year (T04, T07) are described in Supanta et al. (15) and Table 1. A summary of Time and Camp differences in biomarkers of adrenal activity, oxidative stress, DNA damage, stress leukogram, and the heterophil/lymphocyte ratio are shown in Supplementary Table S1. Univariate and multivariate GEE results are presented in Supplementary Tables S1–S9. Table 2 summarizes the results of multivariate GEE analyses of category and continuous camp management variables associated with stress biomarkers, with LS-mean data from the final GEE models presented in Table 3. Stress biomarkers were compared to published reference ranges before COVID-19 in Table 4. Moreover, seasonal effects are presented in Supplementary Table S10.
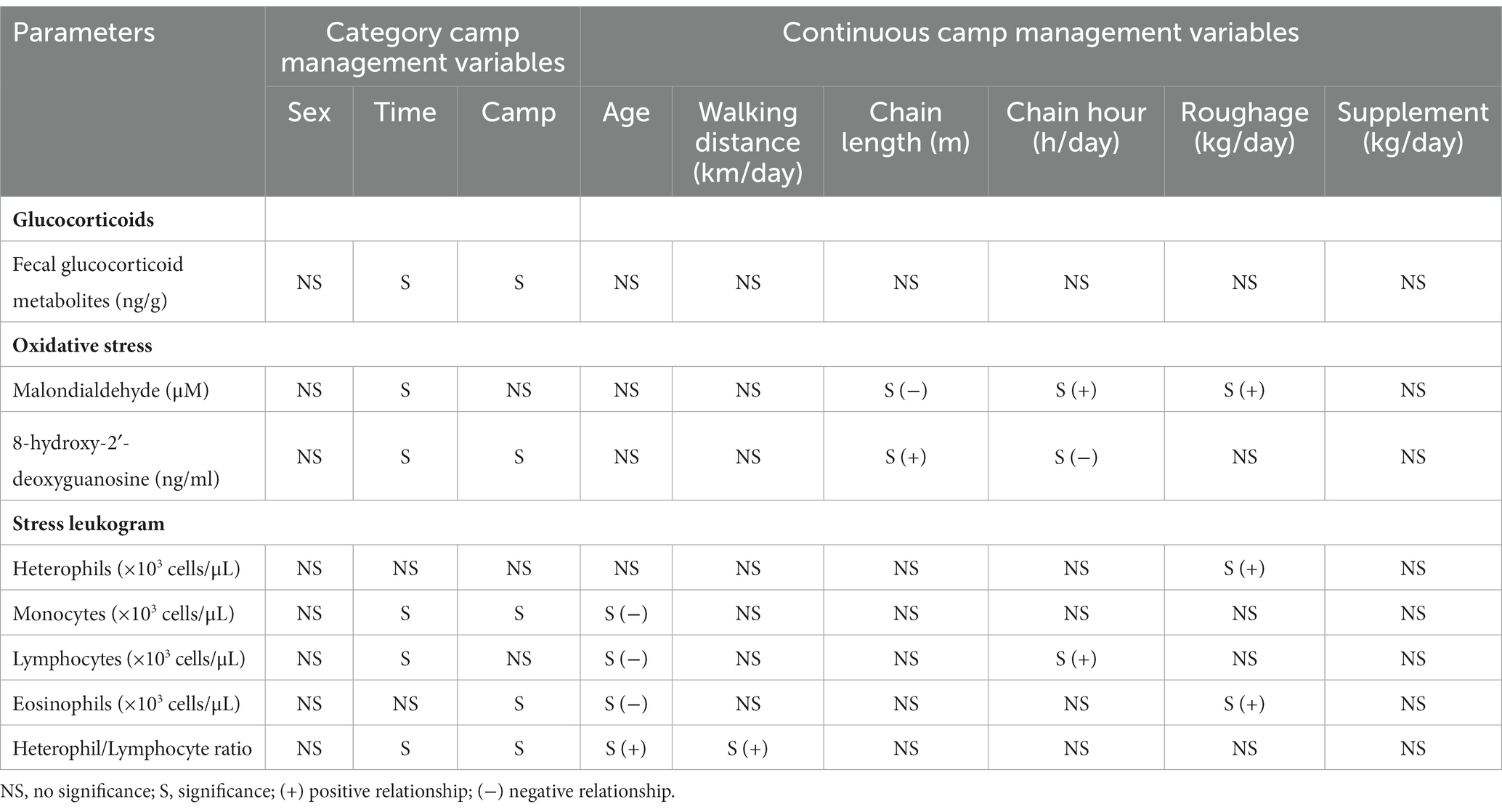
Table 2. Summary of significant relationships from multivariate GEE analyses of category and continuous camp management variables associated with stress biomarkers.
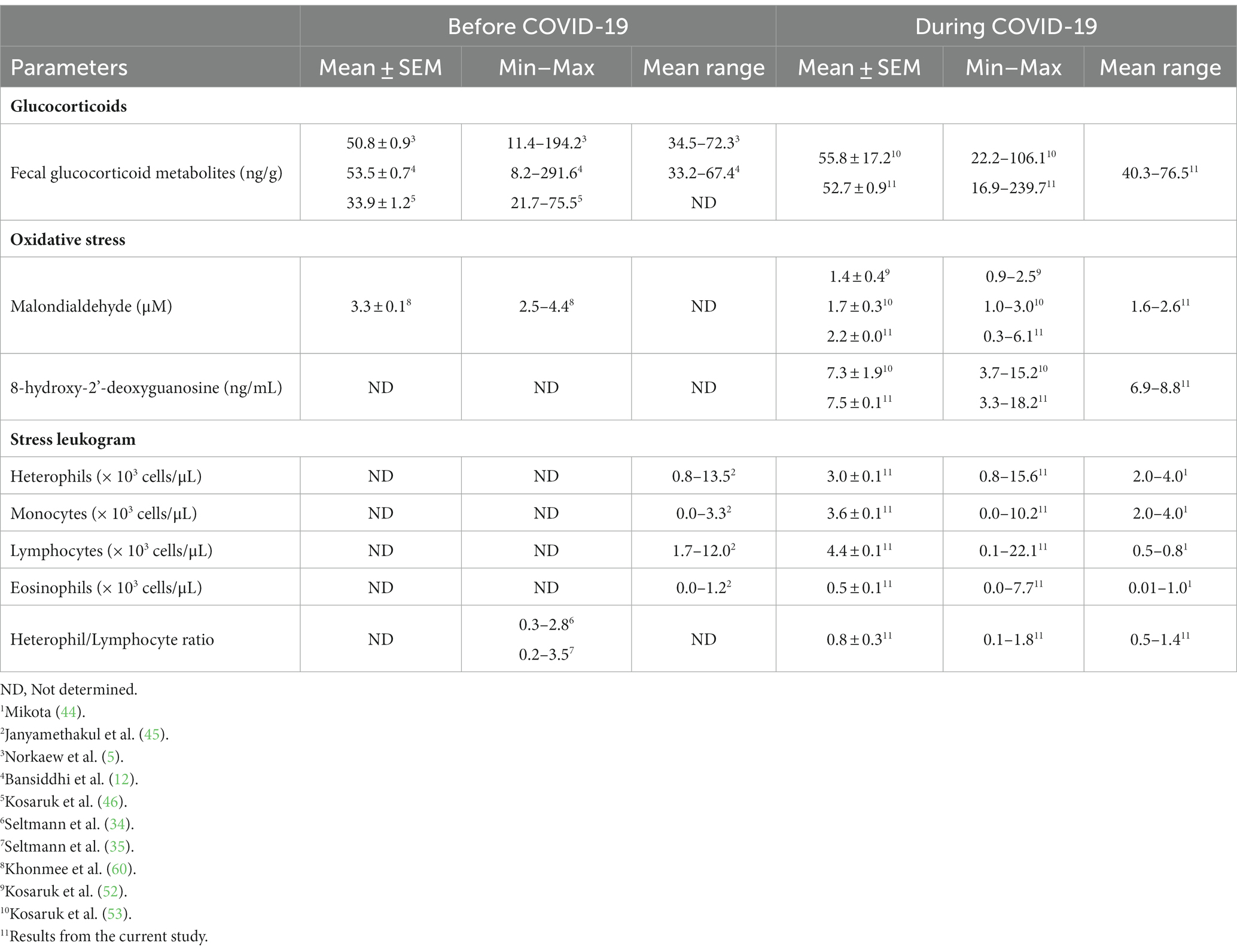
Table 3. Mean (± SEM) and range values for biomarkers of adrenal activity, oxidative stress, DNA damage, stress leukogram, and the heterophil/lymphocyte ratio in captive Asian elephants (n = 58) in six Thailand tourist camps during the COVID-19 pandemic and international travel ban between April 2020 and April 2022.
Fecal glucocorticoid metabolites
Overall average fGCM concentration was 52.9 ± 1.1 ng/g (21.2–147.4 ng/g), with lower overall concentrations observed in T01 (p < 0.001) (Figure 1 and Table 4). Variables significantly affecting fGCM concentrations in the multivariate model were Time and Camp (Tables 2, 4 and Supplementary Table S2), with a rising trend in three of six camps (Camps A, C, E) when data were compared across T01, T04 and T07 time periods (Supplementary Table S1). Elephants at Camps B and F exhibited the highest fGCM concentrations, while those at Camp C had the lowest overall. There was a seasonal effect on fGCM, with the lowest concentrations in the summer and highest values in the rainy season (Supplementary Table S10).
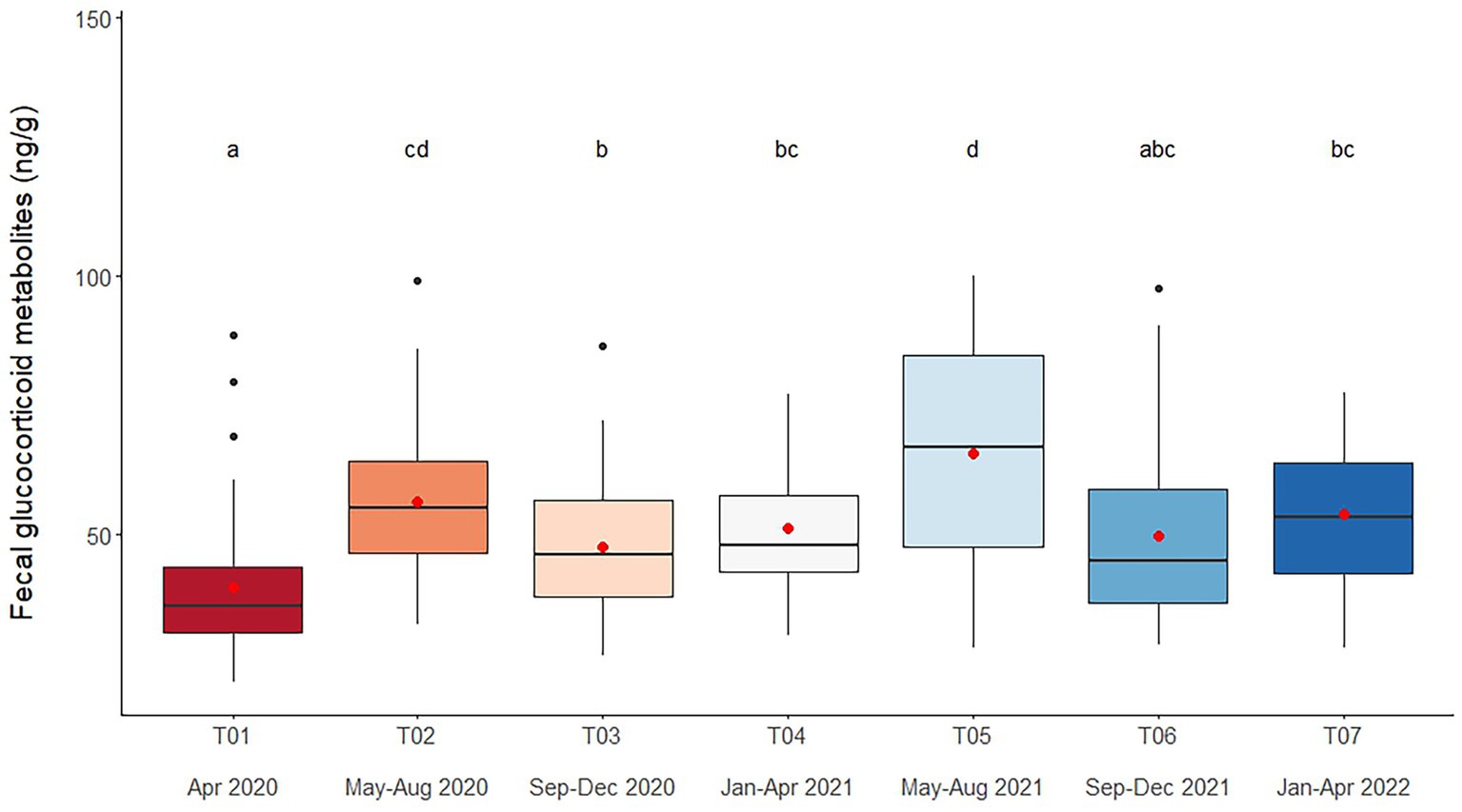
Figure 1. Boxplots of fecal glucocorticoid metabolite concentrations in captive Asian elephants (n = 58) over a 2-year period during the COVID-19 pandemic and international travel ban. Each plot shows the median (horizontal line), mean (red dot), 25 and 75% quartile ranges, and minimum and maximum ranges (whiskers). Different letters indicate significant differences between time periods (p < 0.001).
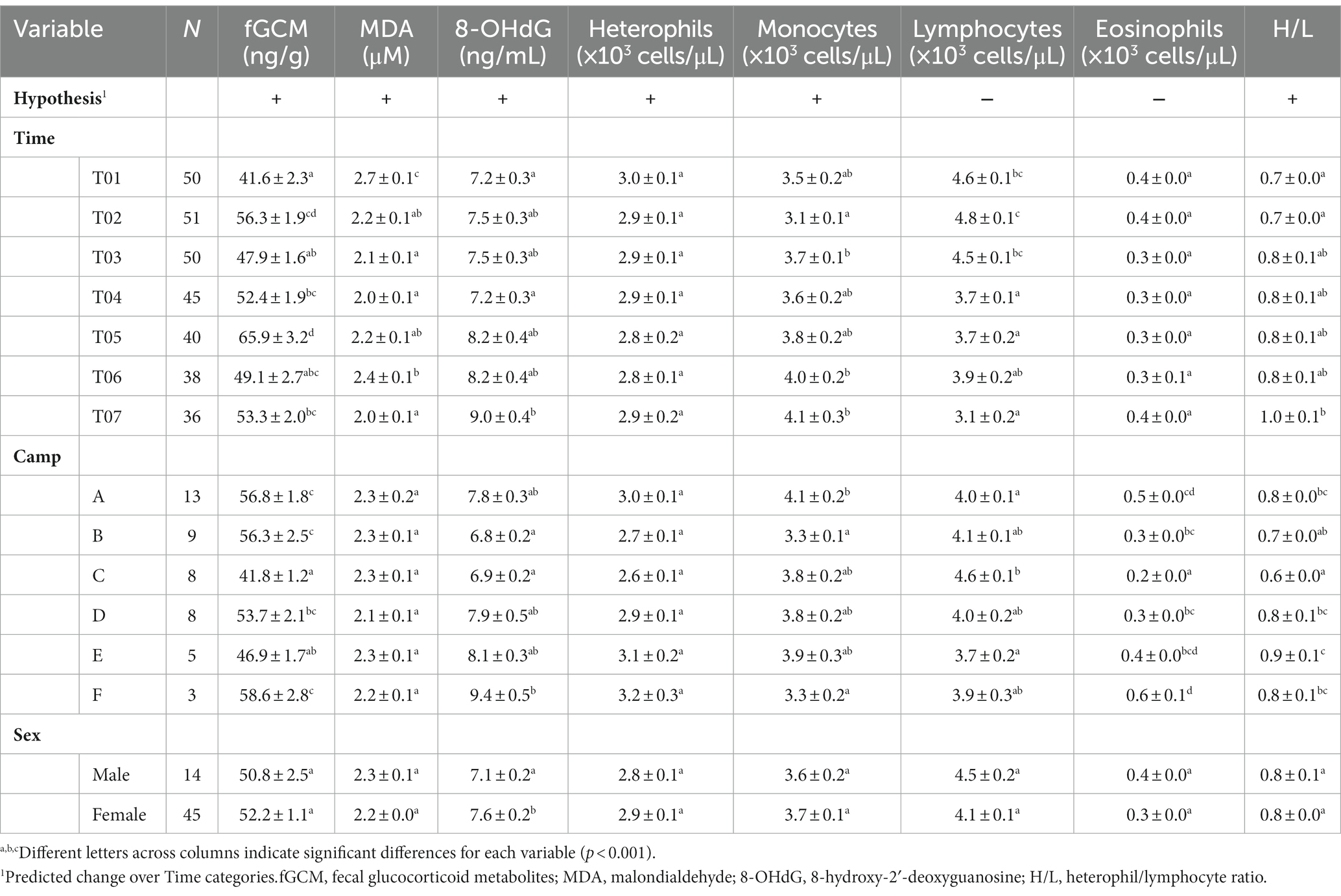
Table 4. LS-mean (± SEM) glucocorticoids, oxidative stress, stress leukogram and heterophil/lymphocyte ratio of Asian elephants (n = 58) in relation to categorical demographic and management variables from final GEE models.
Oxidative stress
Serum MDA concentrations averaged 2.2 ± 0.5 μM (1.3–3.8 μM), and were affected by Time, Chain length, Chain hour and Roughage in the multivariate analysis (Tables 2, 4 and Supplementary Table S3). Chain hour and Roughage were positive relationships, while Chain hour was negative (Table 1 and Supplementary Table S3). There was a declining trend over time (Figure 2A and Table 4) in all six camps (Supplementary Table S1), but no seasonal effect (Supplementary Table S10).
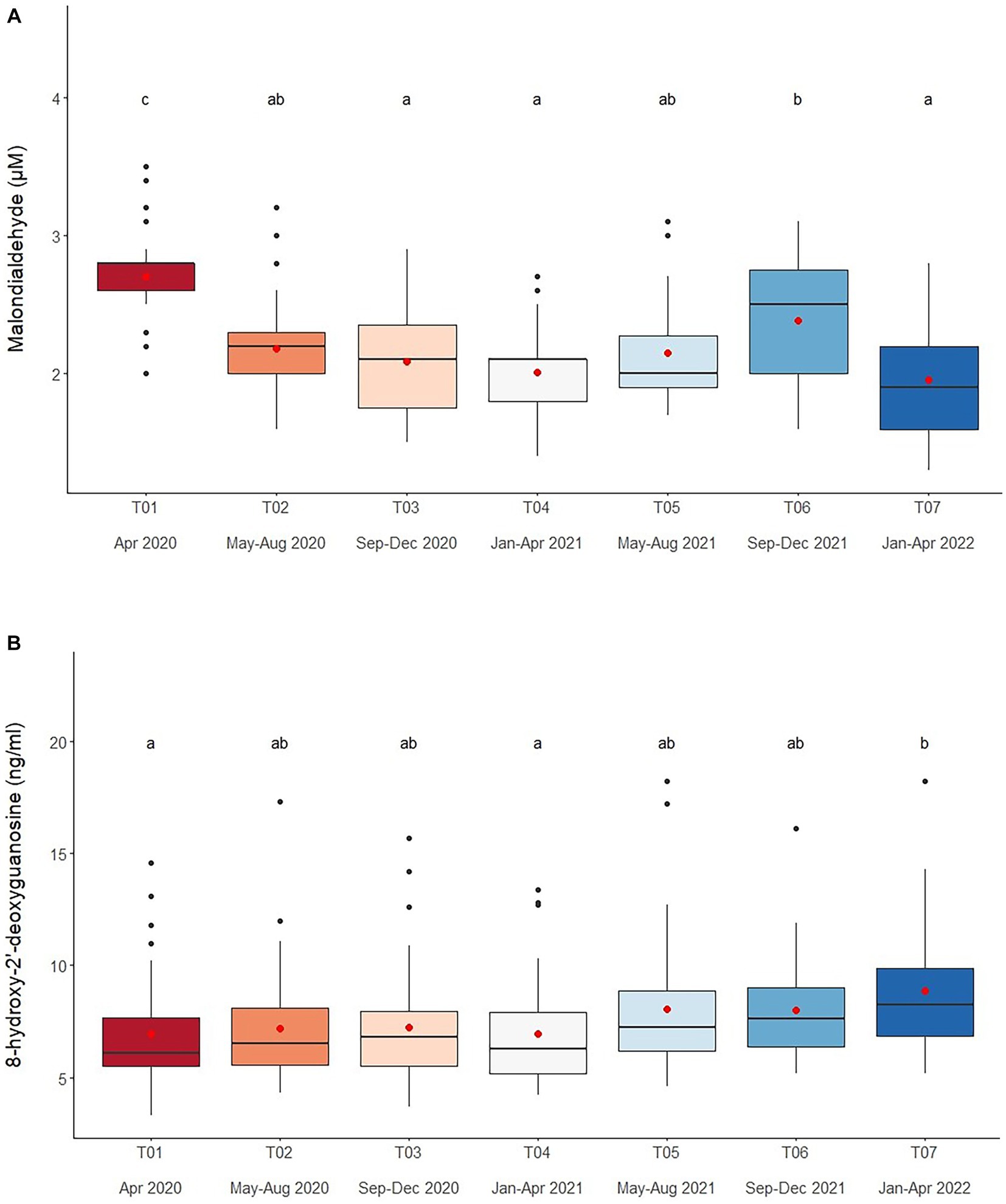
Figure 2. Boxplots of oxidative stress markers (A) malondialdehyde and (B) 8-hydroxy-2′-deoxyguanosine in captive Asian elephants (n = 58) over a 2-year period during the COVID-19 pandemic and international travel ban. Each plot shows the median (horizontal line), mean (red dot), 25 and 75% quartile ranges, and minimum and maximum ranges (whiskers). Different letters indicate significant differences between time periods (p < 0.001).
Overall average serum 8-OHdG concentration was 7.5 ± 1.4 mg/mL (3.3–18.2 mg/mL) and related to Time, Camp, Chain length and Chain hour in the final multivariable model (Tables 2, 4 and Supplementary Table S4). Chain length was positively associated with serum 8-OHdG concentration, while a negative relationship with Chain hour approached significance (Table 2 and Supplementary Table S4). There was an increasing trend over the 2-year period (Figure 2B and Table 4), albeit significant in only two camps (Camp C and E) (Supplementary Table S1). Overall, Camp F had consistently higher 8-OHdG values, while Camp B had among the lowest concentrations. There was no seasonal effect on serum 8-OHdG concentrations (Supplementary Table S10).
Stress leukogram
Overall average heterophil counts were 2.9 ± 0.1 × 103 cells/μL (1.1–8.9 × 103 cells/μL) and affected only by Roughage (positive) in the multivariate analysis (Tables 2, 4 and Supplementary Table S5). There were no differences among Time periods (Figure 3A and Table 4), and counts were not affected by season (Supplementary Table S10).
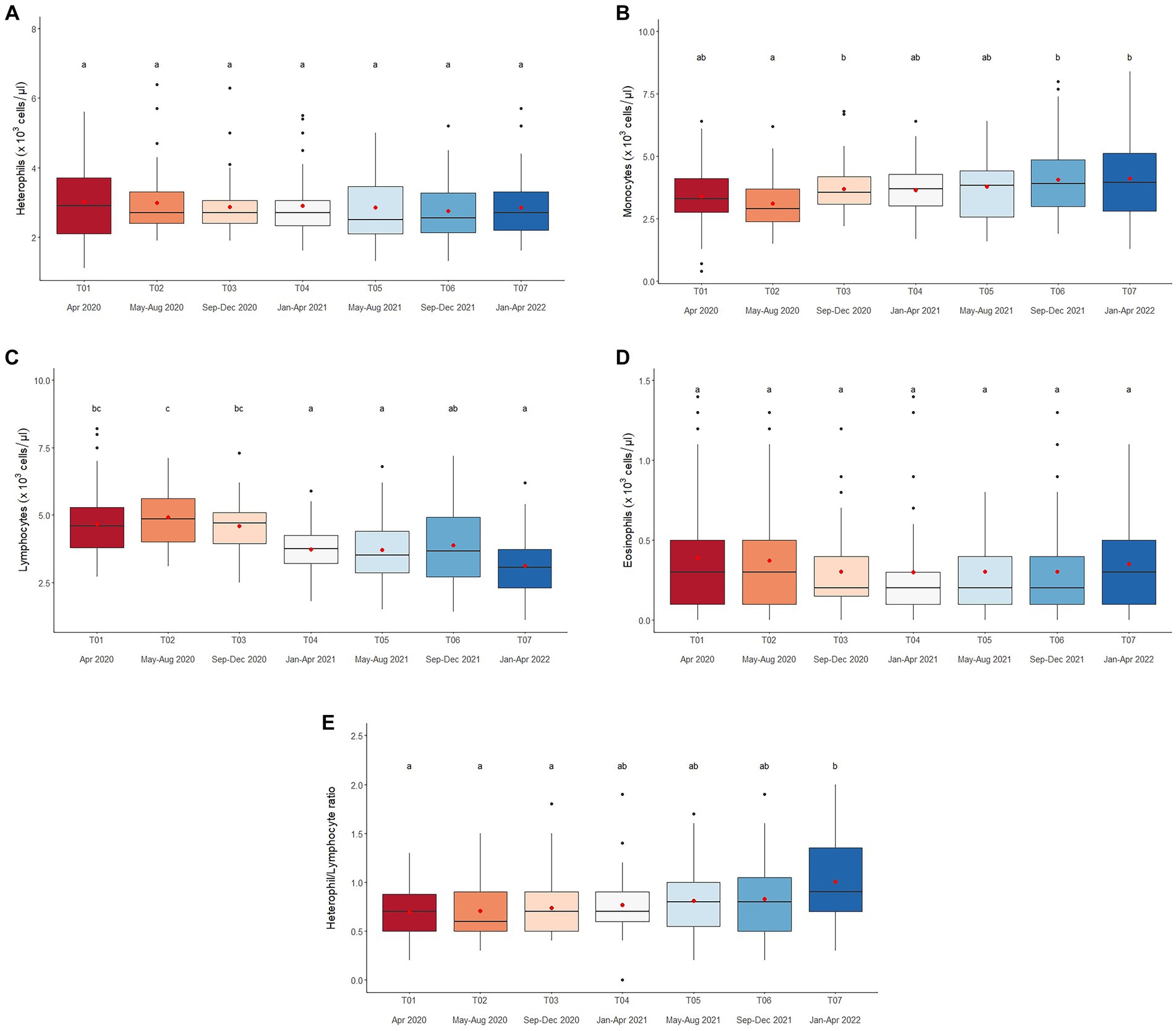
Figure 3. Boxplots of white blood cell counts in the stress leukogram for (A) heterophils, (B) monocytes, (C) lymphocytes, (D) eosinophils, and the (E) heterophil/lymphocyte ratio in captive Asian elephants (n = 58) over a 2-year period during the COVID-19 pandemic and international travel ban. Each plot shows the median (horizontal line), mean (red dot), 25 and 75% quartile ranges, and minimum and maximum ranges (whiskers). Different letters indicate significant differences between time periods (p < 0.001).
Monocyte counts averaged 3.7 ± 0.1 × 103 cells/μL (0.0–8.7 × 103 cells/μL). From the multivariate analysis, factors that had the greatest influence were Age, Time, and Camp (Tables 2, 4 and Supplementary Table S6). Monocyte counts were higher in younger elephants (Table 1 and Supplementary Table S6) and exhibited an increasing trend over the 2-year period (Figure 3B and Table 4). At each time period, Camp A elephants consistently had lower monocyte counts (Supplementary Table S1). There was no seasonal effect (Supplementary Table S10).
Mean lymphocyte counts overall were 4.2 ± 0.1 × 103 cells/μL (1.1–9.9 × 103 cells/μL). From the multivariate analysis, Age, Time, and Chain hour had the greatest influence (Tables 2, 4 and Supplementary Table S7), with a negative relationship to Age and positive relationship to Chain hour (Table 2 and Supplementary Table S7). An overall declining trend over time was observed (Figure 3C and Table 2) that was significant in four camps (Camps A, C, E, F) (Supplementary Table S1). There was no effect of season (Supplementary Table S10).
Eosinophil counts averaged 0.3 ± 0.0 × 103 cells/μL (0.0–1.7 × 103 cells/μL), and from the multivariate analysis, Age, Time, Camp and Roughage had significant effects. Eosinophil counts were lower in older elephants, and there was a positive relationship with Roughage (Table 2 and Supplementary Table S8). However, changes over time revealed no discernable pattern (Figure 3D and Table 4), and neither were their seasonal effects (Supplementary Table S10). Elephants at Camp C had the lowest eosinophil counts, while those at Camp F were generally the highest (Table 4).
For the overall H/L ratio (0.8 ± 0.01; range, 0.2–3.4) Age, Time, Camp and Walking distance had significant effects. H/L ratio was higher in older elephants, with a positive relationship to Walking distance (Table 2 and Supplementary Table S9) and increasing trend over the 2-year period (Figure 3E and Table 4) for four camps (Camps A, C, E, F) (Supplementary Table S1). Camp E had the highest H/L ratio, while Camp C was the lowest. There were no seasonal effects (Supplementary Table S10).
Discussion
After the closure of Thailand’s borders in response to the COVID-19 pandemic in March 2020, a study was undertaken to determine the impact of the COVID-19 pandemic-induced international travel ban on the management, health, and welfare of captive elephants. In the first paper, we describe marked changes in physical activity and access to nourishment, with heightened durations of chaining, and decreases in numbers of mahouts as camps closed due to the lack of tourists (15). We then showed how significant alterations in muscle and liver enzymes, lipid profiles, and body condition occurred in relation to these management changes, indicating potential adverse effects on elephant health (16). The current investigation provides additional evidence of physiological shifts based on measures of stress biomarkers in these same elephants, specifically those associated with adrenal GC activity, oxidative stress, and hematology, that suggests these biological changes may be caused by chronic stress. Overall, concentrations of fGCM and serum 8-OHdG increased, as did the H/L ratio, and remained elevated throughout the travel ban, potentially in relation to prolonged inactivity. By contrast, MDA concentrations decreased and remained low, possibly as a result of dietary modifications. Based on hematology, there was evidence of monocytosis and lymphopenia, possibly due to stress-related increases in corticosteroids. Camp management factors negatively affecting stress outcomes included shorter chain lengths, longer chain hours, lack of exercise, and restricted food resources. Altogether, these studies exposed the struggles that camps experienced in trying to maintain adequate care for elephants in the absence of tourists and associated income, and the need for contingency plans to better prepare for any future disruptions to tourism.
Fecal glucocorticoid metabolites
Concentrations of fGCM increased over time and were highest at T05 (May–August 2021), a year into the lockdown. The range in this study (16.9–239.7 ng/g) aligns with previous values in Asian elephants in northern Thailand [37.4–98.3 ng/g (5–7); 39.2–69.0 ng/g/feces (12); 25.8–39.3 ng/g (46); 22.2–106.1 ng/g (53)], albeit at the higher end as the travel ban progressed. While there are limited studies on how tourist or logging activities impact stress hormone responses, there are indications that yearly patterns of GCs may be related more to work activities than environmental influences (52, 53). In India, fGCM concentrations were higher in elephants involved in an intensive and crowded public festival, but not other tourist activities (61). In Myanmar, elephants have the best body condition, but also experience the highest fGCM concentrations as they enter the more stressful logging and monsoon seasons (62). By contrast, a different study of the Myanmar population found the highest fGCM concentrations in the winter and lowest in the monsoon season, with intermediate values in the summer (63). That study was conducted on non-working elephants only; thus, it appears to demonstrate a true seasonal pattern.
In Thailand, a series of correlational studies aimed at identifying captive management factors that exert significant influences on welfare outcomes have been conducted by our group over the past 6 years (4–7, 11, 12, 14–16, 45–47, 49, 52, 53, 64). Lower fGCM concentrations were found to be associated with riding or walking activities, being kept in open areas during the day, and in the forest or under a tree at night (12). High BCSs were linked to limited exercise and feeding of high-calorie treats like banana and sugarcane, and also higher fGCM concentrations (5–7); all these were similar to findings observed in U.S. zoo elephants (8, 9). However, the relationship between fGCM and BCS during COVID-19 differed, as there was a slight, but significant negative relationship between fGCM and BCS (Spearman’s rank correlation; rho = −0.14; p = 0.0172). So, as body condition decreased, presumably due to limited roughage and supplements, fGCM increased. The reason for this is not clear, as there were no clear relationships between fGCM and management factors found to be important in earlier studies, like Walking distance, Chain hours, Chain length, Roughage or Supplements. Rather it could simply be due to the fact that elephants during the COVID-19 pandemic were chained most, if not all, of the time and had nothing to do, and body condition was reduced to levels not seen in earlier studies. In a study of wild elephants in India, fGCM were highest in individuals with the lowest BCSs, lower than those observed in this study (65). So, it might not be surprising there were little differences among camps in elephant adrenal responses to management, as it was all inadequate. Follow up studies are now planned as tourism begins to return normal in Thailand, although as of the end of 2023 it had not.
A significant new finding was related to season, where the pattern in this study was opposite to that found earlier. Previously, higher fGCM concentrations during the winter months suggested a possible link between colder temperatures and increased adrenal activity (5, 6, 46). However, those months (November–February) also correspond to the high tourist season (5, 6), so until now, it has been impossible to tease apart tourist activity versus climate conditions on adrenal activity. But this and another study (53) conducted during the COVID-19 lockdown strongly suggest that tourist activities are the most likely cause of increased fGCM excretion during the winter, high tourist season months. Thus, further studies are needed to better understand not only what tourist activities affect elephant health and welfare, but how. That said, it is critical to consider context, as increases in adrenal GC activity can occur in response to both positive and negative stimuli, so higher concentrations do not necessarily equate to poor welfare, nor do lower levels indicate good welfare (66).
Oxidative stress
Serum MDA concentrations (1.3–3.8 μM) were similar to previous ranges [0.93–3.0 μM (44, 45)], but showed a declining trend as the study progressed, with T01 (April 2020) being the highest and T07 (January–April 2020) the lowest. There was no obvious seasonal effect, which agrees with another study conducted during the same time period in Thai elephants (53), although a follow-up study did find somewhat lower concentrations during the rainy season (52). Polyunsaturated fatty acids are major substrates for lipid peroxidation (59, 61, 62), and MDA is an end-product produced by the decomposition of the lipid peroxidation process (61). We recently found that elephants during COVID-19 received reduced high-energy diets from a lack of tourists (16), resulting in lower lipid concentrations and BCSs (16). Thus, one possible explanation is a reduction in fat consumption due to dietary changes, as the factor, Roughage was positively associated with MDA concentrations and generally contains 4.1–5.5% crude fat (64). During COVID-19, amounts of roughage decreased, leading to possible reductions in lipid intake. Moreover, roughage, especially young Napier grass, has antioxidant properties (67) known to inhibit the activity of free radicals (68, 69), which might also explain the observed lower MDA concentrations during the lockdown.
Several studies have suggested monitoring serum 8-OHdG as an indicator of DNA damage might be a useful biomarker for both acute (70, 71) and chronic stress (72). In this study, serum 8-OHdG concentrations (3.7–15.2 ng/mL) were similar to a previous study in elephants (3.3–18.2 ng/mL, (52, 53), but with an increasing trend over time, being lowest at the beginning and highest at the end of the study. A previous study found inactive mice had higher oxidative stress markers (lipid hydroperoxides, vascular superoxide release and nicotinamide adenine dinucleotide phosphate oxidase) than active mice (42). In humans, positive correlations have been observed between urinary 8-OHdG and average working hours and serum cortisol concentrations (69), suggesting that increased GC activity may lead to higher levels of oxidative DNA damage (37). In zoo-housed grizzly bears (Ursus arctos horribilis), fecal 8-OHdG concentrations were positively related to fGCM concentrations (71). Thus, it is possible that the increased GCs noted in this study may have contributed to increased DNA damage (73), although other factors may certainly have been involved.
Stress leukogram and H/L ratio
In human and animal medicine, a stress leukogram is characterized by neutrophilia, lymphopenia, eosinopenia, and monocytosis, and specifically refers to the effects of corticosteroids on white blood cell populations (36). While the hematology values during COVID-19 were generally within the range of healthy elephants (45, 74, 75), comparatively, data point to the presence of monocytosis and lymphopenia, two of four criteria in a stress leukogram. Monocytosis was most likely the result of a shift from a marginalized to a circulating population of monocytes. Changes in the expression of adhesion molecules and chemotactic cytokines that interfere with the trafficking of leukocytes into tissues (76) could play a role in changes in WBC populations. Lymphopenia is the result of an immediate shift of lymphocytes from the circulating blood to other tissues, as well as decreased lymphopoeisis (77). In addition, GCs can induce apoptosis of lymphoid cells in mammals (78) and thus interfere with immune functionality. Although no elephants became visibly sick during the study, we cannot rule out subclinical changes as a result of long-term stress associated with restrictive management changes.
The H/L values in this study ranged from 0.1 to 2.5, which agrees with earlier reports of 0.30 to 2.8 (34) and 0.2 to 3.5 (63) in captive Asian timber elephants. The H/L ratio reflects the ability of an animal to cope with infection through injury (via heterophils) or disease (via lymphocytes) (79). While heterophils form the first line of immune defense against bacterial pathogens in inflammatory lesions, lymphocytes are involved in host defense through cytokine production and immune responses (80). As early as 1989, the H/L ratio has been used as an indicator of welfare and how stress affects immune function in response to adrenal-corticoid hyperactivity (81, 82), with lower ratios suggesting better immune function or as a proxy of lower GC responses. During the travel ban, H/L ratios increased over time, which agrees with concomitant increases in GCs that are known to affect the H/L. In elephants, Ukonaho et al. (63) found elephants in Myanmar had higher fGCM concentrations and H/L ratios during the cold season, indicating increased stress. Heterophilia occurs only under stressful conditions, similar to the situation with monocytes, but in this study was not enough to be statistically different. In relation to management factors, there was a positive correlation between lymphocyte numbers and Chain Time, which is consistent with a report showing captive rodents presented higher lymphocyte counts than free-ranging ones (83). Thus, results further suggest elephants in Thailand were exposed to chronic stress during the COVID-19 lockdown.
Conclusion
This and our two companion studies (15, 16) are the first to reveal how a disruption to the tourist industry had significant negative effects on captive elephant health and welfare in Thailand. Throughout the 2-year international travel ban, there were clear effects of management changes because of the lack of tourists on several stress biomarkers reflecting adrenal activity, oxidative stress, and leukograms. Serum MDA as a measure of oxidative stress declined during the COVID-19 countrywide lockdown in relation to dietary changes. Serum 8-OHdG concentrations were increased, suggesting stressors (via glucocorticoid activity) can physically damage DNA. Higher H/L ratio, along with monocytosis and lymphopenia were two criteria of the stress leukogram that appear to reflect increased glucocorticoid activity due to physical inactivity. One aim was to identify what camps adapted to still meet elephant welfare needs in the absence of tourists that could serve as models for other camps. However, only Camp C had lower stress biomarker profiles, including fGCM, serum 8-OHdG, monocyte counts, and H/L ratios, potentially suggesting elephants were less stressed. Still, those elephants were chained all but 30 min a day, with short chain lengths of 2.5 m and < 3 km/d of walking time - not drastically different from the other camps (40). Thus, it was strikingly clear that camps struggled to care for elephants during the COVID-19 lockdown when there was little incentive to provide exercise or other activities, including socialization, to the elephants. It is hoped that elephant owners will recognize the seriousness of these inadequacies and develop contingency plans to avoid such problems in the future should other events cause disruptions to the tourist industry.
Data availability statement
The original contributions presented in the study are included in the article/Supplementary material, further inquiries can be directed to the corresponding author.
Ethics statement
The research received approval from the Human Research Ethics Committee (HS1/2564) and the Institutional Animal Care and Use Committee at the Faculty of Veterinary Medicine (FVM), Chiang Mai University (CMU), located in Chiang Mai, Thailand (FVM-ACUC; S4/2564). The study was conducted in accordance with the local legislation and institutional requirements.
Author contributions
JS: Conceptualization, Formal analysis, Investigation, Methodology, Validation, Writing – review & editing, Visualization, Writing – original draft. JB: Formal analysis, Methodology, Validation, Writing – review & editing, Conceptualization, Funding acquisition, Resources. PB: Conceptualization, Formal analysis, Investigation, Methodology, Writing – review & editing. CT: Conceptualization, Funding acquisition, Resources, Writing – review & editing. VP: Methodology, Software, Validation, Writing – review & editing. KP: Methodology, Validation, Writing – review & editing. PT: Methodology, Validation, Writing – review & editing. NS: Methodology, Validation, Writing – review & editing. JK: Conceptualization, Data curation, Formal analysis, Funding acquisition, Investigation, Methodology, Resource, Project administration, Supervision, Validation, Visualization, Writing original draft, Writing – review & editing.
Funding
The author(s) declare financial support was received for the research, authorship, and/or publication of this article. This study was supported in part from the Smithsonian Conservation Biology Institute (SCBI, United States) through a grant from the Shared Earth Foundation as part of a Memorandum of Understanding with Faculty of Veterinary Medicine, Chiang Mai University (grant number R000032244) and the Chiang Mai University (grant number R66IN00349). The funders had no role in study design, data collection and analysis, decision to publish, or preparation of the manuscript.
Acknowledgments
We would like to acknowledge elephant tourist camps in Chiang Mai province, Thailand for kind cooperation and support. A special thanks to Khajohnpat Boonprasert, Sarisa Klinhom, Panida Muanghong, Wachiraporn Toonrongchang, Worapong Kosaruk, Siripat Khammesri, Pratthana Inthawong and Teepakorn Lertwichaikul for help with sample collections and suggestions. Thanks also to Caroline Gabel and Suzanne Engel for generously supporting our research in Asia.
Conflict of interest
The authors declare that the research was conducted in the absence of any commercial or financial relationships that could be construed as a potential conflict of interest.
Publisher’s note
All claims expressed in this article are solely those of the authors and do not necessarily represent those of their affiliated organizations, or those of the publisher, the editors and the reviewers. Any product that may be evaluated in this article, or claim that may be made by its manufacturer, is not guaranteed or endorsed by the publisher.
Supplementary material
The Supplementary material for this article can be found online at: https://www.frontiersin.org/articles/10.3389/fvets.2024.1351361/full#supplementary-material
Footnotes
References
1. Sakamoto, M . Recent topics on CITES related to Asian elephants in particular. Gajah (2017) 47:42–4.
2. Asian Elephant Specialist Group (2017) Asian elephant range states meeting, final report. Available online at: http://www.asesg.org/PDFfiles/2017/AsERSM2017FinalReport.pdf (Accessed September 6, 2023).
3. Godfrey, A, and Kongmuang, C. Distribution, demography and basic husbandry of the Asian elephant in the tourism industry in northern Thailand. Gajah (2009) 30:13–8.
4. Bansiddhi, P, Brown, J, Thitaram, C, Punyapornwithaya, V, Somgird, C, Edwards, K, et al. Changing trends in elephant camp management in northern Thailand and implications for welfare. PeerJ (2018) 6:e5996. doi: 10.7717/peerj.5996
5. Norkaew, T, Brown, J, Bansiddhi, P, Somgird, C, Thitaram, C, Punyapornwithaya, V, et al. Body condition and adrenal glucocorticoid activity affects metabolic marker and lipid profiles in captive female elephants in Thailand. PLoS One (2018) 13:e0204965. doi: 10.1371/journal.pone.0204965
6. Norkaew, T, Brown, J, Bansiddhi, P, Somgird, C, Thitaram, C, Punyapornwithaya, V, et al. Influence of season, tourist activities and camp management on body condition, testicular and adrenal steroids, lipid profiles, and metabolic status in captive Asian elephant bulls in Thailand. PLoS One (2019) 14:e0210537. doi: 10.1371/journal.pone.0210537
7. Norkaew, T, Brown, J, Thitaram, C, Bansiddhi, P, Somgird, C, Punyapornwithaya, V, et al. Associations among tourist camp management, high and low tourist seasons, and welfare factors in female Asian elephants in Thailand. PLoS One (2019) 14:e0218579. doi: 10.1371/journal.pone.0218579
8. Morfeld, KA, and Brown, JL. Metabolic health assessment of zoo elephants: management factors predicting leptin levels and the glucose-to-insulin ratio and their associations with health parameters. PLoS One (2017) 12:e0188701. doi: 10.1371/journal.pone.0188701
9. Morfeld, KA, Meehan, CL, Hogan, JN, and Brown, JL. Assessment of body condition in African (Loxodonta africana) and Asian (Elephas maximus) elephants in North American zoos and management practices associated with high body condition scores. PLoS One (2016) 11:e0155146. doi: 10.1371/journal.pone.0155146
10. Brown, J, Bansiddhi, P, Khonmee, J, and Thitaram and., Commonalities in management and husbandry factors important for health and welfare of captive elephants in North America and Thailand. Animals (2020) 10:737. doi: 10.3390/ani10040737
11. Bansiddhi, P, Nganvongpanit, K, Brown, J, Punyapornwithaya, V, Pongsopawijit, P, and Thitaram, C. Management factors affecting physical health and welfare of tourist camp elephants in Thailand. PeerJ (2019) 7:e6756. doi: 10.7717/peerj.6756
12. Bansiddhi, P, Brown, J, Khonmee, J, Norkaew, T, Nganvongpanit, K, Punyapornwithaya, V, et al. Management factors affecting adrenal glucocorticoid activity of tourist camp elephants in Thailand and implications for elephant welfare. PLoS One (2019) 14:e0221537. doi: 10.1371/journal.pone.0221537
13. Roberts, J, Thitaram, C, Luz, S, Brown, JL, Mikota, S, Mar, K, et al.Asian Elephant Specialist Group. (2017). Management and welfare of captive elephants used in tourism. Available at: https://www.asesg.org/PDFfiles/WGReport-Tourism.pdf (Accessed December 4, 2023).
14. Bansiddhi, P, Brown, JL, Thitaram, C, Punyapornwithaya, V, and Nganvongpanit, K. Elephant tourism in Thailand: a review of animal welfare practices and needs. J Appl Anim Welf Sci (2020) 23:164–77. doi: 10.1080/10888705.2019.1569522
15. Supanta, J, Brown, JL, Bansiddhi, P, Thitaram, C, Punyapornwithaya, V, and Khonmee, J. Effect of the COVID-19 pandemic and international travel ban on elephant tourist camp management in northern Thailand. Front Vet Sci (2022) 9:1038855. doi: 10.3389/fvets.2022.1038855
16. Supanta, J, Brown, JL, Bansiddhi, P, Thitaram, C, Punyapornwithaya, V, Punturee, K, et al. Physiological changes in captive elephants in northern Thailand as a result of the COVID-19 tourism ban -muscle, liver, metabolic function, and body condition. Front Vet Sci (2023) 10:1303537. doi: 10.3389/fvets.2023.1303537
17. Romero, LM . Physiological stress in ecology: lessons from biomedical research. Trends Ecol Evol (2004) 19:249–55. doi: 10.1016/j.tree.2004.03.008
18. Herman, JP, McKlveen, JM, Ghosal, S, Kopp, B, Wulsin, A, Makinson, R, et al. Regulation of the hypothalamic-pituitary-adrenocortical stress response. Compr Physiol (2016) 6:603–21. doi: 10.1002/cphy.c150015
19. McKay, LI, and Cidlowski, JA. Corticosteroids in the treatment of neoplasms. In: DW Kufe, RE Pollock, and RR Weichselbaum, et al., editors. Holland-Frei cancer medicine. 6th edition. Hamilton (ON): BC Decker (2003).
20. Strehl, C, Ehlers, L, Gaber, T, and Buttgereit, F. Glucocorticoids—all-rounders tackling the versatile players of the immune system. Front Immunol (2019) 10:1744. doi: 10.3389/fimmu.2019.01744
21. Glaeser, SS, Edwards, KL, Wielebnowski, N, and Brown, JL. Effects of physiological changes and social life events on adrenal glucocorticoid activity in female zoo-housed Asian elephants (Elephas maximus). PLoS One (2020) 15:e0241910. doi: 10.1371/journal.pone.0241910
22. Whirledge, S, and Cidlowski, JA. Glucocorticoids and reproduction: traffic control on the road to reproduction. Trends Endocrinol Metab (2017) 28:399–415. doi: 10.1016/j.tem.2017.02.005
23. Fanson, KV, Keeley, T, and Fanson, BG. Cyclic changes in cortisol across the estrous cycle in parous and nulliparous Asian elephants. Endocr Connect (2014) 3:57–66. doi: 10.1530/EC-14-0025
24. Cohen, S, Tyrrell, DAJ, and Smith, AP. Psychological stress and susceptibility to the common cold. N Engl J Med (1991) 325:606–12. doi: 10.1056/NEJM199108293250903
25. Cohen, S, Frank, E, Doyle, WJ, Skoner, DP, Rabin, BS, and Gwaltney, JM Jr. Types of stressors that increase susceptibility to the common cold in healthy adults. Health Psychol (1998) 17:214–23. doi: 10.1037/0278-6133.17.3.214
26. Olefsky, JM, and Kimmerling, G. Effects of glucocorticoids on carbohydrate metabolism. Am J Med Sci (1976) 271:202–10. doi: 10.1097/00000441-197603000-00009
27. Kuo, T, McQueen, A, Chen, T-C, and Wang, J-C. Regulation of glucose homeostasis by glucocorticoids. Adv Exp Med Biol (2015) 872:99–126. doi: 10.1007/978-1-4939-2895-8_5
28. Zahorec, R . Ratio of neutrophil to lymphocyte counts--rapid and simple parameter of systemic inflammation and stress in critically ill. Bratisl Lek Listy (2001) 102:5–14. doi: 10.4149/BLL_2014_076
29. Wang, Y, Ju, M, Chen, C, Yang, D, Hou, D, Tang, X, et al. Neutrophil-to-lymphocyte ratio as a prognostic marker in acute respiratory distress syndrome patients: a retrospective study. J Thorac Dis (2018) 10:273–82. doi: 10.21037/jtd.2017.12.131
30. Rosales, C . Neutrophil: a cell with many roles in inflammation or several cell types? Front Physiol (2018) 9:113. doi: 10.3389/fphys.2018.00113
31. Harr, KE, Isaza, R, and Blue, JT. Hematology of elephants In: DJ Weiss and KJ Wardrop, editors. Schalm's veterinary hematology. 6th ed. Ames, IA: Blackwell (2010). 942–9.
32. Davis, AK, Maney, DL, and Maerz, JC. The use of leukocyte profiles to measure stress in vertebrates: a review for ecologists. Funct Ecol (2008) 22:760–72. doi: 10.1111/j.1365-2435.2008.01467.x
33. Grzelak, AK, Davis, DJ, Caraker, SM, Crim, MJ, Spitsbergen, JM, and Wiedmeyer, CE. Stress leukogram induced by acute and chronic stress in zebrafish (Danio rerio). Comp Med (2017) 67:263–9.
34. Seltmann, MW, Ukonaho, S, Reichert, S, Dos Santos, D, Nyein, UK, Htut, W, et al. Faecal glucocorticoid metabolites and H/L ratio are related markers of stress in semi-captive Asian timber elephants. Animals (2020) 10:94. doi: 10.3390/ani10010094
35. Seltmann, MW, Öst, M, Jaatinen, K, Atkinson, S, Mashburn, K, and Hollmén, T. Stress responsiveness, age and body condition interactively affect flight initiation distance in breeding female eiders. Anim Behav (2012) 84:889–96. doi: 10.1016/j.anbehav.2012.07.012
36. Stockham, SL, and Scott, MA. Fundamentals of veterinary clinical pathology, 2nd edition. Ames, IA, Blackwell (2008) pp. 70–88.
37. Cordiano, R, Di Gioacchino, M, Mangifesta, R, Panzera, C, Gangemi, S, and Minciullo, PL. Malondialdehyde as a potential oxidative stress marker for allergy-oriented diseases: an update. Mol Basel Switz (2023) 28:5979. doi: 10.3390/molecules28165979
38. Islam, MR, Ahmed, I, Moktadir, AA, Nahar, Z, Islam, MS, Shahid, SFB, et al. Elevated serum levels of malondialdehyde and cortisol are associated with major depressive disorder: a case-control study. SAGE Open Med (2018) 6:2050312118773953. doi: 10.1177/2050312118773953
39. Gaweł, S, Wardas, M, Niedworok, E, and Wardas, P. Malondialdehyde (MDA) as a lipid peroxidation marker. Wiadomosci Lek Wars Pol 1960 (2004) 57:453–5.
40. Beckman, KB, and Ames, BN. Oxidative decay of DNA. J Biol Chem (1997) 272:19633–6. doi: 10.1074/jbc.272.32.19633
41. Kasai, H . Analysis of a form of oxidative DNA damage, 8-hydroxy-2′-deoxyguanosine, as a marker of cellular oxidative stress during carcinogenesis. Mutat Res Mutat Res (1997) 387:147–63. doi: 10.1016/S1383-5742(97)00035-5
42. Fuller, G, Hamilton, J, and Allard, S. Damage as a potential non-invasive indicator of welfare: a preliminary study in zoo-housed grizzly bears (Ursus arctos horribilis). J Zool Bot Gard (2021) 2:316–34. doi: 10.3390/jzbg2030022
43. Graille, M, Wild, P, Sauvain, J-J, Hemmendinger, M, Guseva Canu, I, and Hopf, NB. Urinary 8-OHdG as a biomarker for oxidative stress: a systematic literature review and meta-analysis. Int J Mol Sci (2020) 21:3743. doi: 10.3390/ijms21113743
44. Mikota, S . Hemolymphatic system In: ME Fowler and S Mikota, editors. Biology, medicine, and surgery of elephants. Ames, Iowa: Blackwell Publishing (2006). 325–45.
45. Janyamethakul, T, Sripiboon, S, Somgird, C, Pongsopawijit, P, Panyapornwithaya, V, and Klinhom, S. Hematologic and biochemical reference intervals for captive Asian elephants (Elephas maximus) in Thailand. Kafkas Univ Vet Fak Derg (2017) 23:665–8. doi: 10.9775/kvfd.2017.17380
46. Kosaruk, W, Brown, JL, Plangsangmas, T, Towiboon, P, Punyapornwithaya, V, Silva-Fletcher, A, et al. Effect of tourist activities on fecal and salivary glucocorticoids and immunoglobulin a in female captive Asian elephants in Thailand. Animals (2020) 10:1928. doi: 10.3390/ani10101928
47. Brown, JL, Walker, SL, and Moeller, T. Comparative endocrinology of cycling and non-cycling Asian (Elephas maximus) and African (Loxodonta africana) elephants. Gen Comp Endocrinol (2004) 136:360–70. doi: 10.1016/j.ygcen.2004.01.013
48. Menargues, A, Urios, V, Limiñana, R, and Mauri, M. Circadian rhythm of salivary cortisol in Asian elephants (Elephas maximus): a factor to consider during welfare assessment. J Appl Anim Welf Sci JAAWS (2012) 15:383–90. doi: 10.1080/10888705.2012.709157
49. Plangsangmas, T, Brown, JL, Thitaram, C, Silva-Fletcher, A, Edwards, KL, Punyapornwithaya, V, et al. Circadian rhythm of salivary immunoglobulin a and associations with cortisol as a stress biomarker in captive Asian elephants (Elephas maximus). Animals (2020) 10:157. doi: 10.3390/ani10010157
50. Hewage, NI, Dassanayaka, LY, and Uluwaduge, DI. Sodium fluoride tubes versus plain tubes for in vitro blood glucose analysis. Ceylon J Med Sci (2018) 55:46. doi: 10.4038/cjms.v55i2.4936
51. Satitmanwiwat, S, Promthep, K, Buranaamnuay, K, Mahasawangkul, S, and Saikhun, K. Lipid and protein oxidation levels in spermatozoa and seminal plasma of Asian elephants (Elephas maximus) and their relationship with semen parameters. Reprod Domest Anim (2017) 52:283–8. doi: 10.1111/rda.12900
52. Kosaruk, W, Brown, JL, Towiboon, P, Punyapornwithaya, V, Pringproa, K, and Thitaram, C. Measures of oxidative status markers in relation to age, sex, and season in sick and healthy captive Asian elephants in Thailand. Animals (2023) 13:1548. doi: 10.3390/ani13091548
53. Kosaruk, W, Brown, JL, Towiboon, P, Pringproa, K, Punyapornwithaya, V, Tankaew, P, et al. Seasonal patterns of oxidative stress markers in captive Asian elephants in Thailand and relationships to elephant endotheliotropic herpesvirus shedding. Front Vet Sci (2023) 10:1263775. doi: 10.3389/fvets.2023.1263775
54. R: The R project for statistical computing (2023). Available online at: https://www.r-project.org (Accessed September 6, 2023).
55. Højsgaard, S, Yan, J, and Halekoh, U. The R package GEEPACK for generalized estimating equations. J Stat Softw (2005) 15:1–11. doi: 10.18637/jss.v015.i02
56. Pan, W . Akaike’s information criterion in generalized estimating equations. Biometrics (2001) 57:120–5. doi: 10.1111/j.0006-341x.2001.00120.x
57. Barton, K. Package “MuMIn”. (2023). Available online at: https://cran.rproject.org/web/packages/MuMIn/MuMIn.pdf (Accessed September 6, 2023).
58. Khonmee, J, Brown, JL, Rojanasthien, S, Aunsusin, A, Thumasanukul, D, Kongphoemphun, A, et al. Gender, season and management affect fecal glucocorticoid metabolite concentrations in captive goral (Naemorhedus griseus) in Thailand. PLoS One (2014) 9:e91633. doi: 10.1371/journal.pone.0091633
59. Khonmee, J, Brown, JL, Rojanasthien, S, Thumasanukul, D, Kongphoemphun, A, Siriaroonrat, B, et al. Seasonality of fecal androgen and glucocorticoid metabolite excretion in male goral (Naemorhedus griseus) in Thailand. Anim Reprod Sci (2014) 146:70–8. doi: 10.1016/j.anireprosci.2014.02.008
60. Khonmee, J, Brown, JL, Jinawong, T, Sathanawongs, A, Panasophonkul, S, et al. Relationship between malondialdehyde, cortisol, testosterone and semen quality in captive Asian elephant (Elephas maximus) bulls: Proceedings of the 15th Asian Society of Conservation Medicine, Chiang Mai University, Chiang Mai, Thailand (2022), p. 48
61. Kumar, V, Pradheeps, M, Kokkiligadda, A, Niyogi, R, and Umapathy, G. Non-invasive assessment of physiological stress in captive Asian elephants. Animals (2019) 9:553. doi: 10.3390/ani9080553
62. Mumby, HS, Mar, KU, Hayward, AD, Htut, W, Htut-Aung, Y, and Lummaa, V. Elephants born in the high stress season have faster reproductive ageing. Sci Rep (2015) 5:13946. doi: 10.1038/srep13946
63. Ukonaho, S, Berger, V, Franco dos Santos, DJ, Htut, W, Aung, HH, Nyeing, UK, et al. Seasonal variation in molecular and physiological stress markers in Asian elephants. Conserv Physiol (2023) 11:coad029. doi: 10.1093/conphys/coad029
64. Suriyong, S, Sringarm, K, Krittigamas, N, and Bansiddhi, P. A study of the feed management practices of private elephant camps in Chiangmai province. Vet Integr Sci (2023) 21:945–58. doi: 10.12982/VIS.2023.068
65. Pokharel, SS, Seshagiri, PB, and Sukumar, R. Assessment of season-dependent body condition scores in relation to faecal glucocorticoid metabolites in free-ranging Asian elephants. Conserv Physiol (2017) 5:cox039. doi: 10.1093/conphys/cox039
66. Pokharel, SS, and Brown, JL. Physiological plasticity in elephants: highly dynamic glucocorticoids in African and Asian elephants. Conserv Physiol (2023) 11:coad088. doi: 10.1093/conphys/coad088
67. Onjai-uea, N, Paengkoum, S, Taethaisong, N, Thongpea, S, Sinpru, B, Surakhunthod, J, et al. Effect of cultivar, plant spacing and harvesting age on yield, characteristics, chemical composition, and anthocyanin composition of purple Napier grass. Animals (2023) 13:10. doi: 10.3390/ani13010010
68. Lü, J-M, Lin, PH, Yao, Q, and Chen, C. Chemical and molecular mechanisms of antioxidants: experimental approaches and model systems. J Cell Mol Med (2010) 14:840–60. doi: 10.1111/j.1582-4934.2009.00897.x
69. Su, L-J, Zhang, J-H, Gomez, H, Murugan, R, Hong, X, Xu, D, et al. Reactive oxygen species-induced lipid peroxidation in apoptosis, autophagy, and ferroptosis. Oxidative Med Cell Longev (2019) 2019:1–13. doi: 10.1155/2019/5080843
70. Irie, M, Tamae, K, Iwamoto-Tanaka, N, and Kasai, H. Occupational and lifestyle factors and urinary 8-hydroxydeoxyguanosine. Cancer Sci (2005) 96:600–6. doi: 10.1111/j.1349-7006.2005.00083.x
71. Gormally, BMG, Estrada, R, McVey, M, and Romero, LM. Beyond corticosterone: the acute stress response increases DNA damage in house sparrows. J Exp Zool Part Ecol Integr Physiol (2020) 333:595–606. doi: 10.1002/jez.2405
72. Laufs, U, Wassmann, S, Czech, T, Münzel, T, Eisenhauer, M, Böhm, M, et al. Physical inactivity increases oxidative stress, endothelial dysfunction, and atherosclerosis. Arterioscler Thromb Vasc Biol (2005) 25:809–14. doi: 10.1161/01.ATV.0000158311.24443.af
73. Flaherty, RL, Owen, M, Fagan-Murphy, A, Intabli, H, Healy, D, Patel, A, et al. Glucocorticoids induce production of reactive oxygen species/reactive nitrogen species and DNA damage through an iNOS mediated pathway in breast cancer. Breast Cancer Res BCR (2017) 19:35. doi: 10.1186/s13058-017-0823-8
74. Pandit, A, Dhakal, IP, Gairhe, KP, and Rana, HB. Serum biochemical profile of the elephants in Chitwan district, Nepal. Int J Rec Sci Res (2015) 6:3130–5.
75. Cronstein, BN, Kimmel, SC, Levin, RI, Martiniuk, F, and Weissmann, G. A mechanism for the anti-inflammatory effects of corticosteroids: the glucocorticoid receptor regulates leukocyte adhesion to endothelial cells and expression of endothelial-leukocyte adhesion molecule 1 and intercellular adhesion molecule 1. Proc Natl Acad Sci USA (1992) 89:9991–5. doi: 10.1073/pnas.89.21.9991
76. Mendelsohn, J, Multer, MM, and Bernheim, JL. Inhibition of human lymphocyte stimulation by steroid hormones: cytokinetic mechanisms. Clin Exp Immunol (1977) 27:127–34.
77. Schwartzman, RA, and Cidlowski, JA. Glucocorticoid-induced apoptosis of lymphoid cells. Int Arch Allergy Immunol (1994) 105:347–54. doi: 10.1159/000236781
78. Steyrer, C, Miller, M, Hewlett, J, Buss, P, and Hooijberg, EH. Reference intervals for hematology and clinical chemistry for the African elephant (Loxodonta africana). Front Vet Sci (2021) 8:599387. doi: 10.3389/fvets.2021.599387
79. Johnstone, CP, Reina, RD, and Lill, A. Interpreting indices of physiological stress in free-living vertebrates. J Comp Physiol B (2012) 182:861–79. doi: 10.1007/s00360-012-0656-9
80. Chaplin, DD . Overview of the immune response. J Allergy Clin Immunol (2003) 111:S442–59. doi: 10.1067/mai.2003.125
81. Davison, TF, Rowell, JG, and Rea, J. Effects of dietary corticosterone on peripheral blood lymphocyte and granulocyte populations in immature domestic fowl. Res Vet Sci (1983) 34:236–9. doi: 10.1016/S0034-5288(18)32266-5
82. Gross, WB . Factors affecting chicken thrombocyte morphology and the relationship with heterophil:lymphocyte ratios. Br Poult Sci (1989) 30:919–25. doi: 10.1080/00071668908417218
Keywords: Asian elephant, welfare, glucocorticoids, stress leukogram, oxidative stress, malondialdehyde, 8-hydroxy-2′-deoxyguanosine
Citation: Supanta J, Brown JL, Bansiddhi P, Thitaram C, Punyapornwithaya V, Punturee K, Towiboon P, Somboon N and Khonmee J (2024) Physiological changes in captive elephants in northern Thailand as a result of the COVID-19 tourism ban—stress biomarkers. Front. Vet. Sci. 11:1351361. doi: 10.3389/fvets.2024.1351361
Edited by:
Ana Molina-López, University of Cordoba, SpainReviewed by:
Supaphen Sripiboon, Kasetsart University, ThailandJames Edward Brereton, Sparsholt College, United Kingdom
Ismael Hernández Avalos, National Autonomous University of Mexico, Mexico
Copyright © 2024 Supanta, Brown, Bansiddhi, Thitaram, Punyapornwithaya, Punturee, Towiboon, Somboon and Khonmee. This is an open-access article distributed under the terms of the Creative Commons Attribution License (CC BY). The use, distribution or reproduction in other forums is permitted, provided the original author(s) and the copyright owner(s) are credited and that the original publication in this journal is cited, in accordance with accepted academic practice. No use, distribution or reproduction is permitted which does not comply with these terms.
*Correspondence: Jaruwan Khonmee, amFydXdhbi5raG9ubWVlQGNtdS5hYy50aA==
†These authors have contributed equally to this work and share senior authorship