- Bristol Veterinary School, University of Bristol, Bristol, United Kingdom
Introduction: Depression is a human mental disorder that can also be inferred in non-human animals. This study explored whether time spent inactive but awake (“IBA”) in the home-cage in mice was further triggered by risk factors similar to those increasing vulnerability to depression in humans (early life stress, genetic predispositions, adulthood stress).
Methods: Eighteen DBA/2 J and 18 C57BL/6 J females were tested, of which half underwent as pups a daily maternal separation on post-natal days 2–14 (early-life stress “ELS”) (other half left undisturbed). To assess the effect of the procedure, the time the dams from which the 18 subjects were born spent active in the nest (proxy for maternal behavior) was recorded on post-natal days 2, 6, 10 and 14 for 1 h before separation and following reunion (matched times for controls), using live instantaneous scan sampling (total: 96 scans/dam). For each ELS condition, about half of the pups were housed post-weaning (i.e., from 27 days old on average) in either barren (triggering IBA and depression-like symptoms) or larger, highly enriched cages (n = 4–5 per group). Time mice spent IBA post-weaning was observed blind to ELS treatment using live instantaneous scan sampling in two daily 90-min blocks, two days/week, for 6 weeks (total: 192 scans/mouse). Data were analyzed in R using generalized linear mixed models.
Results: The dams were significantly more active in the nest over time (p = 0.016), however with no significant difference between strains (p = 0.18), ELS conditions (p = 0.20) and before/after separation (p = 0.83). As predicted, post-weaning barren cages triggered significantly more time spent IBA in mice than enriched cages (p < 0.0001). However, neither ELS (p = 0.4) nor strain (p = 0.84) significantly influenced time mice spent IBA, with no significant interaction with environmental condition (ELS × environment: p = 0.2861; strain × environment: p = 0.5713).
Discussion: Our results therefore only partly support the hypothesis that greater time spent IBA in mice is triggered by risk factors for human depression. We discuss possible explanations for this and further research directions.
1 Introduction
Clinical depression1 is a mental disorder characterized by the co-existence of several debilitating symptoms (depressed mood, loss of pleasure or interest in activities, poor concentration, feelings of excessive guilt or low self-worth, hopelessness, suicidal thoughts, changes in sleep and appetite/weight, and/or fatigue) for at least two weeks (1, 2). Clinical depression affects about 5% of the adult human population worldwide (3) and has a higher prevalence in women (1–8). As stated by the diathesis-stress model (a common framework modelling the etiology of complex mental disorders), individuals made vulnerable by genetic predisposition and/or exposure to early-life stressors (e.g., childhood neglect and abuse) are more prone to develop the symptoms of depression when subjected to stressors later in life, compared to individuals not exposed to these risks factors (9, 10).
Like humans, captive and domestic animals can be exposed to these risk factors, i.e., early life stress [e.g., mother-infant early separation, (e.g., 11, 12)], their stress-mediated responses can be influenced by genetic factors [(e.g., 13, 14)], and they can be exposed to stress during adulthood. The latter includes exposure to a variety of short-, mid- and longer-term environmental stressors (e.g., confinement, unoptimized feeding regimes), social stressors (e.g., mixing, crowding, social restrictions), and pain due to husbandry practices (e.g., disbudding, castration) or health issues (e.g., keel bone damage, mastitis, lameness) [reviewed in Lecorps et al. (15) and Appleby et al. (16)]. Among the animals’ reactions to such impoverished/chronically stressful environments are elevated levels of waking inactivity, i.e., being inactive but awake “IBA” (motionless, eyes open), in the home environment [reviewed in Fureix and Meagher (17) and MacLellan et al. (18)]. Increased inactivity is a feature of human clinical depression (2). We therefore propose that, in animals exposed to chronically barren/restricted environments, greater levels of IBA could indicate depression-like states (19); and that, like in humans, the vulnerability to develop these states are increased by genetic and early life risk factors.
In mice (Mus musculus), elevated IBA was first mentioned as a “pathological form of behavior” and “posture of depression” 30 years ago, in a social defeat study where half of the defeated mice displayed “nose in the corner” behavior accompanied by unresponsiveness to the attacker’s movements or manipulation of the cage by the experimenter (20). Since then, it has been demonstrated that IBA levels are greater in comparatively non-enriched (standard) home-cage environments versus larger, highly enriched preferred cages (21–25). Furthermore, greater time spent IBA in the home cage tends to predict greater time spent immobile in the forced swim test [Fureix et al. (21), see in MacLellan et al. (18) for replication], and has been associated with three other symptoms of human clinical depression: anhedonia (assessed by reduced preference for sucrose, Trevarthen et al., in prep) and changes in weight and sleep [MacLellan et al. (18), replicated in Trevarthen et al., in prep]. Moreover, chronic administration of Venlafaxine (a serotonin-norepinephrine reuptake inhibitor antidepressant), exposure to housing conditions promoting better health and well-being, and their combinations alleviate IBA levels (24), therefore showing common curative factors between IBA in mice and human clinical depression. Altogether, the results suggest a depressive-like state in laboratory mice displaying greater levels of IBA in their home-cage. Here we explored this hypothesis further by investigating whether combining genetic predispositions and/or aversive early life experiences to environmental stress in adulthood triggers further IBA in mice, just as it can do in humans.
We tested genetic predispositions by using two common laboratory strains of mice, C57BL/6 J and DBA/2 J, known to differ in their tendencies to display depression-like profiles under tests, with C57BL/6Js being more prone to display depression-like profiles [(e.g., 26, 27)] although phenotypes may vary between laboratories [(e.g., 28)]. These strains also differ in their IBA levels, and even more so when housed in relatively barren ‘shoebox’ cages, although the direction of the difference can vary between studies. Indeed, C57BL/6 s have been shown to display more IBA than DBA/2Js in Fureix et al. (21) and Nip et al. (23) (Canadian lab, mice sourced from US Charles River), but DBA/2Js displayed more IBA than C57BL/6 s in Trevarthen et al. (25) (British lab, mice sourced from European Charles River), and strains did not differ in Fureix et al. (24). That behavioral strain phenotype may be sensitive to supplier and/or unidentified ‘local environment’ effects is not uncommon [(e.g., 28–30)].
To manipulate the early life experience of mice, maternal separation was applied pre-weaning to half of the mice for each strain (other half undisturbed: control group). Maternal separation is a form of early life stress, which is designed to simulate parental neglect by separating dams and pups for an amount of time (variable between studies). Applying maternal separation to induce depressive features in animals shares construct validity with the human illness (31), since people exposed to early life stressors are at higher risk of developing depression, (e.g., 32, 33). Moreover, maternal separation results in long-lasting behavioral and neuroendocrine alterations in animals similar to behavioral and neuroendocrine features that can be observed in depressed people [face and construct validity, (31)]. For instance, female CD1 mice submitted to maternal separation showed an imbalance in the tryptophan-kynurenine pathway in brain areas associated with emotional processes (pre-frontal cortex and hypothalamus) (34). In humans, major depression is associated with increased inflammatory drive, which causes alterations in the tryptophan-kynurenine pathway and thus the production of neurotransmitters regulating mood such as serotonin (35). Female CD1 mice submitted to maternal separation also show greater depressive-like behavior in tail suspension (greater amount of time spent immobile) and saccharin preference (lower preference at 24 h) tests (34), respectively used as proxies for two symptoms of depression in humans [hopelessness and anhedonia (2)]. Maternal separation in mice also shares predictive validity with human depression since treatment with fluoxetine attenuates the effects of maternal separation (36, 37). Finally, variations in environmental stress at adulthood were induced by housing the mice post-weaning in either barren ‘shoebox’ cages [i.e., validated as inducing depression-like features (38) and greater levels of IBA (21, 22) in rodents], or larger, highly enriched preferred cages.
Our first prediction is primarily methodological: that maternal separation would reduce the maternal behavior of the dams (assessed here using time spent active in the nest as a proxy), therefore confirming a differential early life condition treatment for the pups, which would subsequently influence their susceptibility to depression-like states in adulthood. Since chronic stress commonly triggers depression in humans, particularly in people with genetic predisposition and/or exposure to early life stress, our second prediction is that relatively barren, non-preferred small cages (‘non-enriched’) would trigger greater levels of IBA than larger, highly enriched preferred cages, and even more so in mice exposed to early life maternal separation stress and/or one of the two strains (39, 40). Due to the variation in strain differences between above mentioned study, we predict a strain-related difference exacerbated in non-enriched cages, rather than one strain specifically displaying more IBA than the other.
2 Materials and methods
2.1 Ethical approval
This study was conducted under the UK Home Office Licences PPL P2556FBFE and P10DC2972, in accordance with the Animals (Scientific Procedures) Act 1986 (ASPA), the EU directive 2010/63/EU and the UK Home Office code of practice for the housing and care of animals bred, supplied, or used for scientific purposes. The mice were handled using a polycarbonate tunnel from their home cage, following a validated method shown to reduce stress in laboratory mice (41), at all times unless stated otherwise. Animals were monitored daily throughout the study for any health issues; none were observed at any point. At the end of the study, the mice were either euthanized by skilled technicians (using concussion, immediately followed by cervical dislocation and confirmation of death; the use of both methods ensured rapid loss of consciousness), or released from the ASPA and rehomed to private owners subject to the conditions stated in the PPL P2556FBFE (n rehomed = 33 out of 128 mice used) (Figure 1).
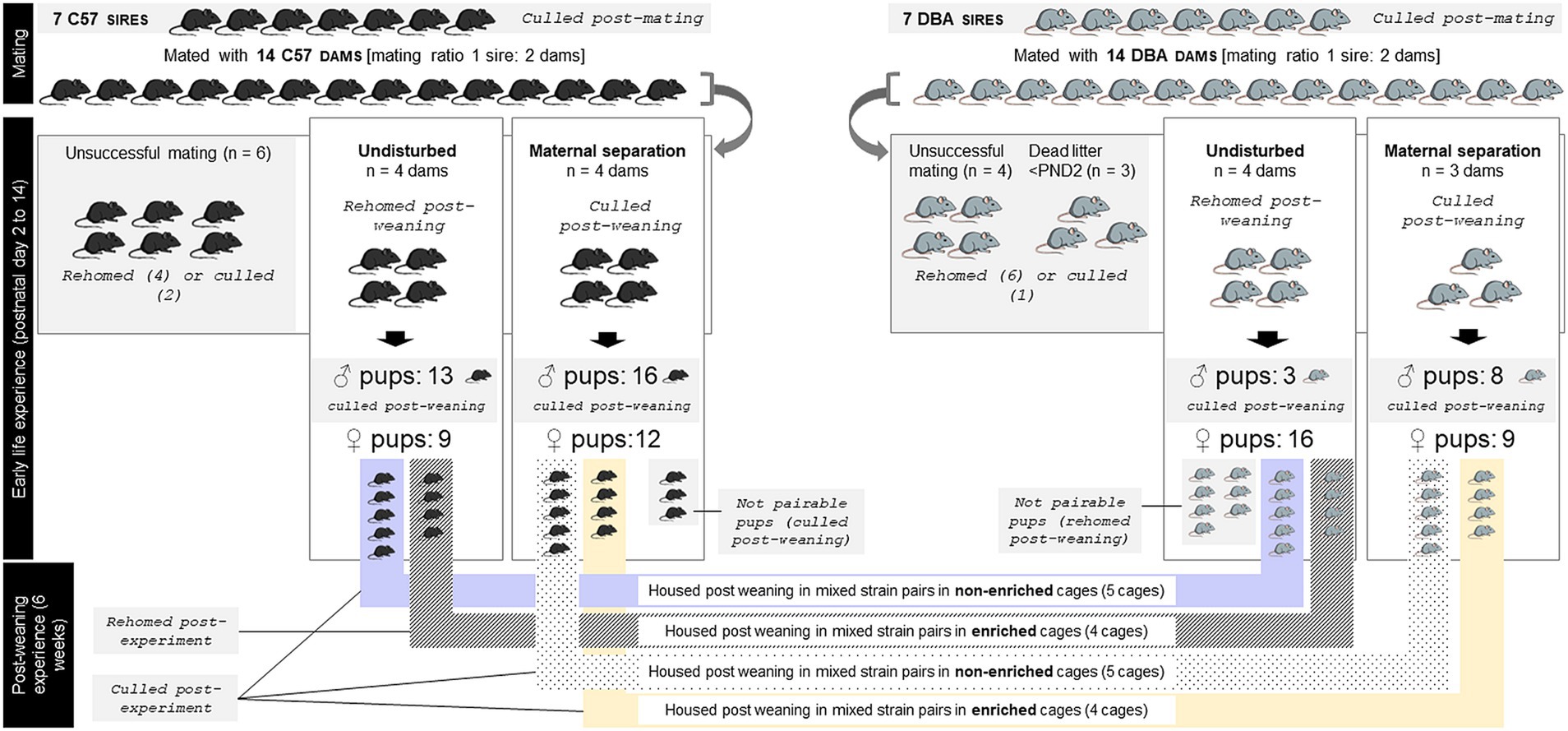
Figure 1. Overview of the experimental design, and number and fate of mice used at every step of the experiment. C57 = C57BL/6 J mice, DBA = DBA/2 J mice.
2.2 Animals and overview of experimental treatments
This experiment was conducted at the research facilities of the University of Bristol from January to March 2020. Estimates of animal numbers were based on power analyses using data from Trevarthen et al. (in prep) (power 80%, significance criterion 2-tailed 0.05, Cohen’s d effect size 0.7 (minimum expected means difference 0.011 and estimated standard deviation 0.016), replicated using several calculators). The Covid-19 pandemic situation, together with funding completion at the time the facilities re-opened post-pandemic, however prevented us from completing the experiment on the last batch of mice. In total, 71 C57BL/6Js (‘C57’) and 57 DBA/2Js (“DBA”) were used: 14 dams and 7 sires of each strain (Charles River, France, eight weeks old at arrival), and their 50 C57 and 36 DBA offspring (bred at our facility). At birth, dams of each strain and their pups were randomly split between two treatments: either maternal separation or left undisturbed (Figure 1). At weaning, the pups were pseudo-randomly (matching cage mates for weight) assigned into mixed strain pairs randomly split between two different environment conditions: either in standard ‘shoebox’, relatively barren cages, or in larger highly enriched cages (Figure 1). Only female pups that could be paired by ELS condition at weaning (n = 18 of each strain) were exposed to the post-weaning differential environment condition. Indeed, depression is more prevalent in women (3) (although we would predict greater level of IBA to reflect depression-like states in males as well), and our mixed-strain housing promoting Refinement (see below) has been investigated and validated in females, not in males (42, 43). The remaining 10 female offspring which could not be paired at weaning were either culled following Schedule 1 killing method (n = 3) or rehomed (n = 7). All the male pups (n = 40) were culled following Schedule 1 killing method, out of which 16 were first released from The Act, kept alive at the licensed establishment and used in non-procedural (reward acquisition based) behavioral work piloting for a different study.
2.3 Breeding conditions
Male breeders were housed in randomly allocated single-strain groups of three to four individuals, and females in single-strain pairs. Each cage had its own ventilation system regulated by an airflow system (IVC cages, 30 cm L × 20 cm W × 17 cm H; Model EFS120PR00; Allentown Inc., New Jersey, United States) and contained nesting material, a cardboard igloo and a Perspex tunnel. All cages were housed within a single rack in the same colony room (room temperature: 21°C, humidity level: 37–45%). The light–dark cycle was 12/12 h with lights on 0800–2000. Two weeks after arrival, a single male (randomly chosen) was introduced in a females’ home cage for 7 days, allowing both females to mate. The onset of gestation was determined by daily observation of the females’ genital area (presence of a vaginal plug) conducted by experienced animal care staff. Females remained housed in pairs until 5–8 days before parturition date, then they were singly housed in the IVC cages above described to allow better parturition and maternal care [cf. (44)].
2.4 Early life experience treatment
Litters were equally split between either the maternal separation or the undisturbed (control) treatment, balancing assignment for each of birth date (post-natal day 0; PND0), litter size (number of pups born alive) and sire (paternal origin) following a randomization schedule (Figure 1). The housing of the cages within the rack was reorganized in a pseudo-random order that ensured an equal spreading of treatments (strain and early life experience) within shelves. Maternal separation happened daily for 180 min from post-natal day (PND) 2 to 14 (Figure 1). Applying 180 min of daily maternal separation has been shown in the literature to induce neuro-physiological (e.g., decreased Brain-Derived Neurotrophic Factor mRNA in the hippocampus, increased 5-HTT methylation) and behavioral (e.g., forced swim test, sucrose consumption) changes reflecting depressive phenotypes in the pups post-weaning (45). The time of the day at which the litters underwent the maternal separation was randomized (Table 1) to increase unpredictability of the event. During maternal separation, the home cages were removed from the rack and placed on heat pads (temperature controlled at 21°C) for 3 h, during which the dams were removed from their home cage and placed alone in another IVC cage back into the rack, in the same room as the pups. Each dam had her own ‘separation cage’ to avoid extra stress due to mixing smells, with fresh bedding added in the dam separation cage on the first separation day and not changed afterwards. Food and water were provided but no enrichment was added. Control litters were checked daily for the pups’ and dams’ health condition, but no disturbance was made to the nest besides lifting the house/food hopper. For both treatment groups, the pups remained undisturbed until they were sexed (PND 20), and the cages were not cleaned until weaning, unless necessary (i.e flooding, one cage).
2.5 Post-weaning differential environment condition
Offspring were weaned at 26.9 ± 2.90 day-old (range: 25–28 days-old). At weaning, the dams that did not undergo the maternal separation (n = 8 mice) and females for which mating was not successful (n = 10; C57: n = 6; DBA: n = 4) or which litters died before PND2 (n = 3; DBA: n = 3) were rehomed (n = 10) or culled (n = 3). The dams exposed to the maternal separation treatment (n = 7 mice) and sires (n = 14) were culled following Schedule 1 killing method. Offspring were moved at weaning to a different colony room (temperature: 21°C, humidity: 37–45%). Each mouse was weighed, and one C57 and one DBA female mouse of the same early life stress treatment and of similar weight (mean weight difference across all pairs = 1.0 g [range 0–3.7 g]) was pseudo-randomly allocated to each cage (mouse selected at random, but matched with cage mate based on weight). Mixed-strain pair-housing allows non-invasive identification of the individuals within pairs, removing the need to conduct invasive identification during behavioral observations (42). For each of the early life conditions, the cages were pseudo-randomly split (i.e., counterbalancing full- and half-siblings across post-weaning environment conditions) between two post-weaning environmental conditions: either in standard, relatively barren cages (“NE”, 10 cages), or in larger highly enriched cages (‘EE’, 8 cages)2 (Figure 1) in which they were housed for 6-weeks.
The NE cages were conventional laboratory cages (37 cm L × 21 cm W × 14 cm, Techniplast) equipped with basic enrichments: sawdust (IPS), a handful of nesting material (Datesand Bed-R’Nest), a Polycarbonate handling tunnel (13 cm L, ∅ 5 cm, Datesand) and a small piece of cardboard. The EE cages were larger (44 cm L × 34 cm W × 20 cm H; Techniplast), and equipped with sawdust, a larger amount (three handfuls) of nesting material (Datesand Bed-R’Nest), the polycarbonate handling tunnel, two small pieces of cardboard, a running wheel with a red igloo (fast-trac, Datesand), one plastic transparent shelter (∅ 15 cm, 5.5 cm H, Biopac UK), three wooden blocks (two small: 5 cm L × 1 cm W × 1 cm H, one large: 10 cm L × 2 cm W × 2 cm H, Datesand), a pinecone (autoclaved before being placed in the cage), additional nesting material such as tissue (Waitrose Basics), two Nestlets (Ancare, United States), two Cocoon nestlets (Datesand) and Sizzle Nest (Datesand), as well as (attached to the cage lid using cable ties): one flexible plastic tunnel (Merlett Superflex L Hose: 30 cm L × ∅ 6.3 cm, RS Components), one half of a coconut shell (approximate dimensions: 12.7 cm L × 7.6 cm W × 5 cm H, Little Cherry Ltd), a hammock made from pillowcases (approximately 15 cm × 8 cm), a sisal rope ladder, and a single spray of Millet (Pets at Home).
All cages were housed within two scantainers (Scanbur, Karlslunde, Denmark). Cages ID and location within the scantainers were allocated by an assistant (naïve to the study and not involved in behavioral observations later), in a pseudo-random order that ensured an equal spreading of experimental treatments (early life experience and post-weaning environment conditions) between the two scantainers, and within shelves for each scantainer. This ensured behavioral observers remained blind to the early-life treatment each cage of mice had been exposed to. NE cages were cleaned every week and larger EE cages were cleaned every three weeks. During cleaning, mice from NE cages were tail-handled [as part of the stress at adulthood treatment, see, (e.g., 41)], while EE mice were tunnel-handled. Food (LabDiet) and water were available ad-libitum for all, and all mice were kept under a 12 h reversed light–dark cycle (lights on 1900–0700) to facilitate post-weaning in-cage behavioral observations. At the end of the experiment, the mice that did not undergo the maternal separation and were housed in an enriched environment (n = 8) were rehomed and the mice in the other experimental conditions (n = 28) were culled following Schedule 1 killing method.
2.6 Measures
Live behavioral observations (following habituation to the presence of the experimenter in the room) were conducted by two trained experimenters (OS and EF, Cohen’s Kappa overall interrater agreement during training = 94.45%, ranging from 87 to 97% according to the behavior considered, splitting equally the sessions between the two observers). Observation sessions took place both during the early life experience (to quantify the time dams spent active in their nest), and during the post-weaning differential environment condition phases (to quantify the behavior related to hypothesis under test, i.e., IBA). Inter-observer reliability was assessed during training of live observations sessions, where the two observers independently observed the same mice at the same time, and later compared their ratings. There were 4 training sessions (of 1 h each) in total. Two sessions were performed on non-experimental litters (i.e., not part of this study) to assess inter-observer reliability with regards to quantifying the dams’ maternal behavior. Two other training sessions were conducted post-weaning on the mice included in the experiment (data not included in the current study analyses), before the actual observation period started.
2.6.1 Behavioral observations conducted during the early life experience phase
On PND2 (first day of maternal separation protocol), PND6, PND10 and PND14 (last day of maternal separation protocol), the time dams spent active in their nest (proxy for maternal behavior) was observed live every 5 min for 1 h before separation and 1 h following reunion, using instantaneous scan sampling method (12 scans/mouse/observation) (46). Control (undisturbed) mice were simultaneously observed to allow comparison of the activity in the nest between the two treatments. Mice were scored as ‘Active in the nest’ when grooming pups, moving nesting material, nursing pups, or performing rapid movements inside the nest [adapted from Brajon et al. (44)]. The dams and their litter were not yet allocated to their future post-weaning environment conditions, which ensured that the observers recording the dams’ behavior were blinded to this aspect of their treatment.
2.6.2 Behavioral observations conducted during the post-weaning differential environment condition phase
The behavior relevant to the hypothesis under test (IBA) was defined following (23, 24) as ‘mouse motionless, muzzle in sight and eyes open, for at least 3 s’. Live behavioral observations of the mice in their home cage began after four days of acclimatization to the new colony room. All observations were conducted during the dark (active) phase under red ambient light, for six weeks (PND 30–33 to PND 67–70), two days per week (Tuesdays and Thursdays), over two 90-min time blocks per day (0930–1,100 and 1,100–1,230). The sampling timetable was chosen after analyzing data from previous experiments, indicating that the proportion of IBA observed weekly when sampling as described above predicted the proportion of IBA observed weekly when sampling more extensively on 4 daily blocks, 4 days per week (assessed and replicated independently across two laboratories; Trevarthen et al., MacLellan et al., unpublished data). Behavior was recorded via live instantaneous scan-sampling (46), switching from scan to 3 s focal sampling to allow for differentiation between behaviors characterized by a lack of movement (e.g., IBA versus sleeping) as in Fureix et al. (21). In total, 8 scan samples were taken per mouse each time block (i.e., scan performed every 10 min), totaling 32 scan samples/mouse/week, and 192 scan samples/mouse in total. The observers (EF and OS) were blind to the mice’s early life experiences, since they were not involved in allocating the post-weaning cages ID and location within the scantainers (as described in 2.5. Post-weaning differential environment conditions section).
2.7 Statistical analyses
Statistical analyses were performed using R software [version 3.6.1 with RStudio 1.2.1578; (47)], using the mice as experimental units and generalized linear mixed models. Blinding to treatments during the analysis was done by CF assigning new (temporary) codes to the experimental conditions in data files before the analysis (experimental conditions were ‘de-blinded’ to the other investigators post-analyses). The normality of data was checked by Shapiro–Wilk tests, and the analyses were performed using lme4 (48) and lmerTest (49) packages. Least square means for post-hoc pairwise comparisons were extracted using the lsmeans package (50).
In order to evaluate the effect of the maternal separation on the amount of maternal care received by the pups, the proportion of time spent active in the nest (proxy for maternal behavior) was analyzed using generalized linear mixed models, with pups’ age (i.e., PND2, PND6, PND10 and PND14), time (i.e., before or after separation), ELS (i.e., maternal separation or undisturbed), strain (i.e., DBA or C57), and their interactions as fixed effects, and cage as a random effect. The significance of explanatory variables and their interactions was determined via stepwise reduction of the model, using Log-likelihood ratio tests (LRTs), starting from the most complex model with all possible pairwise interactions to the simplest one that explained best the response variables, using a significance level of 0.05. The final model only included post-natal day as a fixed factor and the cage as a random effect. All models ran without convergence issue. The effect size of the full model was 0.42, with an associated analysis power of 0.3. The final model explained more variance than a null model, which included only the random effects (p < 0.05).
To explore whether early life stress and/or genetic predispositions triggers further IBA in mice exposed to post-weaning environmental stress, the proportion of IBA whilst in view during scan sampling performed by each individual mouse (averaged across a week for each of the six weeks of observation) was used as the dependent variable. The initial model included week (i.e., 1 to 6), strain (i.e., DBA and C57), ELS (i.e., maternal separation or undisturbed) and post-weaning environment (i.e., enriched EE or non-enriched NE cages), and their interactions as fixed effects, and mouse ID as a random effect. The final model included week, environment and their interaction as fixed effects, and mouse as a random effect. All models ran without convergence issue. The effect size of the final model was 0.6, with an associated analysis power of 0.55. The final model explained more variance than a null model, which included only the random effects (p < 0.0001).
Time spent active in nest and time spent IBA during scan sampling reported in the text are Least Square Means (LSM) ± standard error (SE).
3 Results
3.1 Maternal behavior (pre-weaning observations)
The proportion of scans the dams spent active in the nest significantly increased with pups’ age (X23 = 10.302; p = 0.016), although only PND6 significantly differed from PND14 in post-hoc comparisons (t91.9 = −3.186; p = 0.0105; Figure 2). Contrary to the prediction, the time dams spent active in the nest was not significantly influenced by the early life stress treatment (maternal separation = 0.29 ± 0.061; undisturbed = 0.38 ± 0.059; X21 = 1.6553; p = 0.2), time of observation (before/after separation) (before = 0.33 ± 0.051; after = 0.33 ± 0.051; X21 = 0.047; p = 0.83), or by strain (C57BL/6 J = 0.28 ± 0.058; DBA/2 J = 0.38 ± 0.062; X21 = 1.7641; p = 0.18). None of the 2-way, 3-way and 4-way interactions were significant (Table 2).
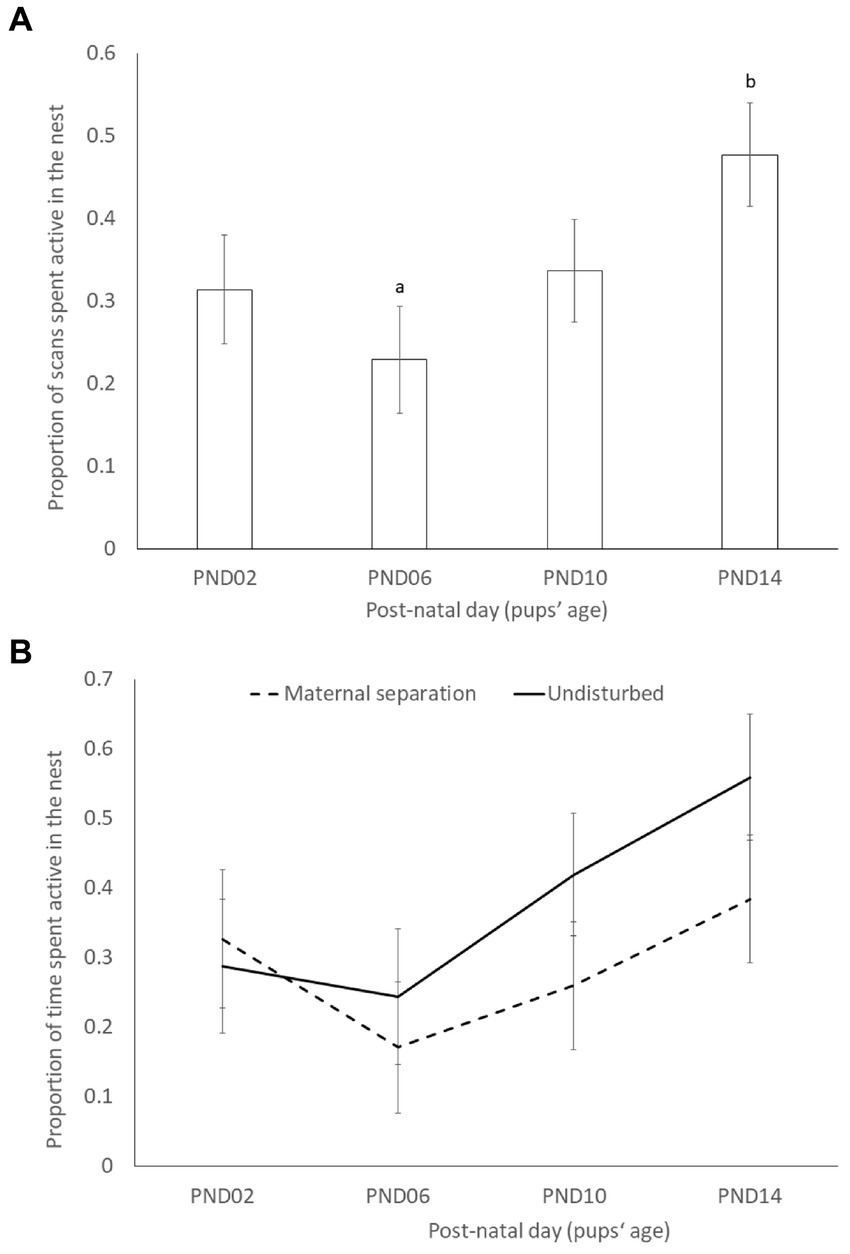
Figure 2. Least square means and standard errors of the proportion of scans spent active in the nest across pups’ age (PND = post-natal day; PND0 = birth and PND02 = start of maternal separation). (A) Pooled data (pups’ age effect: X23 = 10.302; P = 0.016); (B) data split by early life stress treatment (pups’ age × ELS effect: X23 = 4.3294; p = 0.228). Different superscript letters indicate significant differences between post-natal days at p < 0.05.
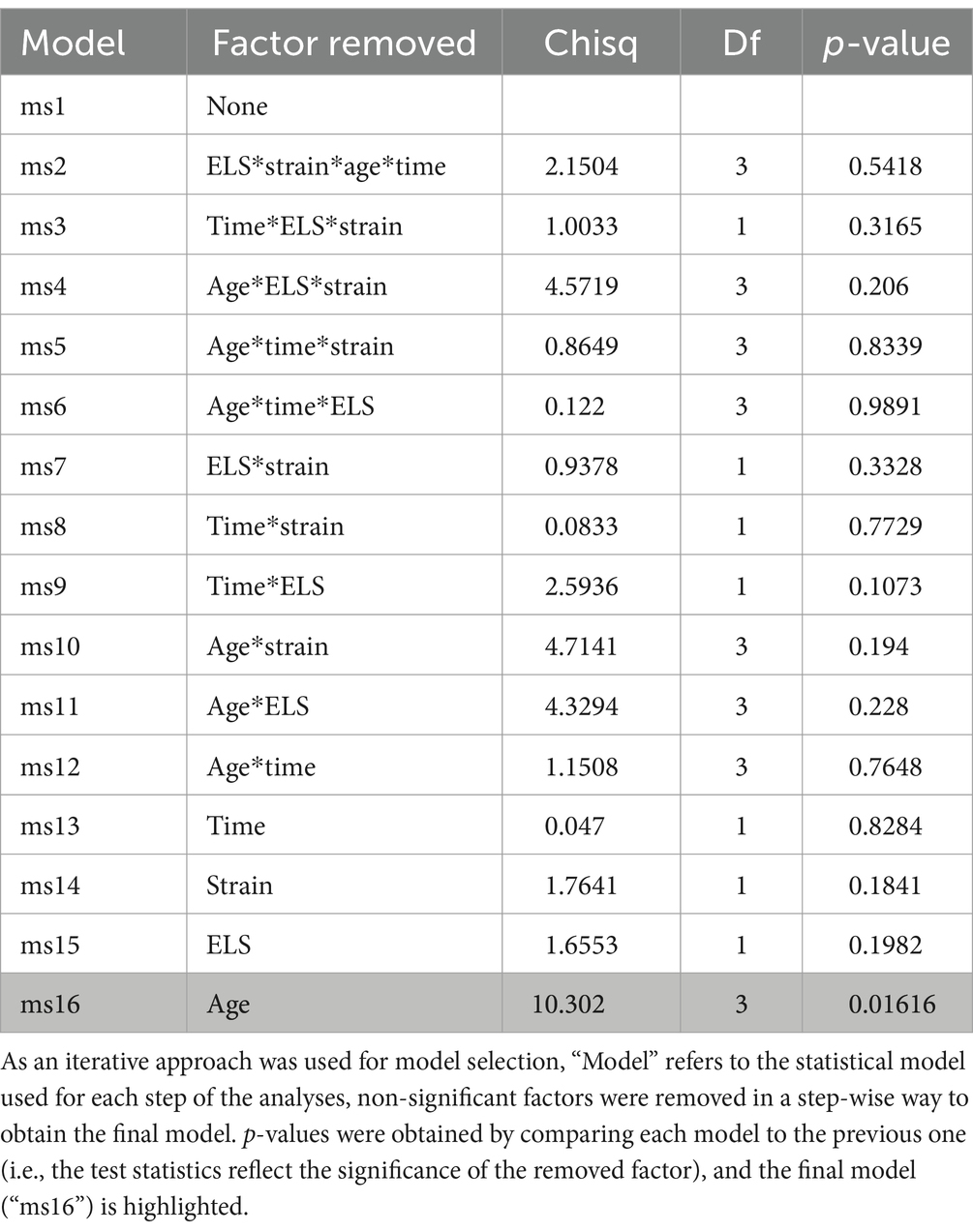
Table 2. Test statistics (Chi-Square), degrees of freedom (Df) and significance (p-value) of each fixed factor, and of the interaction between these factors, for the dams’ proportion of visible scans spent “active in nest”.
3.2 Inactive but awake behavior (post-weaning observations)
As predicted, the proportion of visible scans displaying IBA across the 6 weeks was significantly lower in the mice housed in EE cages than in those housed in NE cages (X21 = 39.615; p < 0.0001) (Figure 3A). Neither the early life experience (undisturbed: 0.045 ± 0.0045; maternal separation: 0.050 ± 0.0045) or strain (DBA: 0.048 ± 0.0045; C57: 0.047 ± 0.0045) significantly predicted the average proportion of visible scans displaying IBA (respectively X21 = 0.6883, p = 0.4 and X21 = 0.0404, p = 0.84). Contrary to the prediction, we also found non-significant 2-way and 3-way interactions (Table 3), i.e., no significant strain or maternal separation effects were observed when the mice were specifically housed in the NE (non-preferred), comparatively more stressful cages.
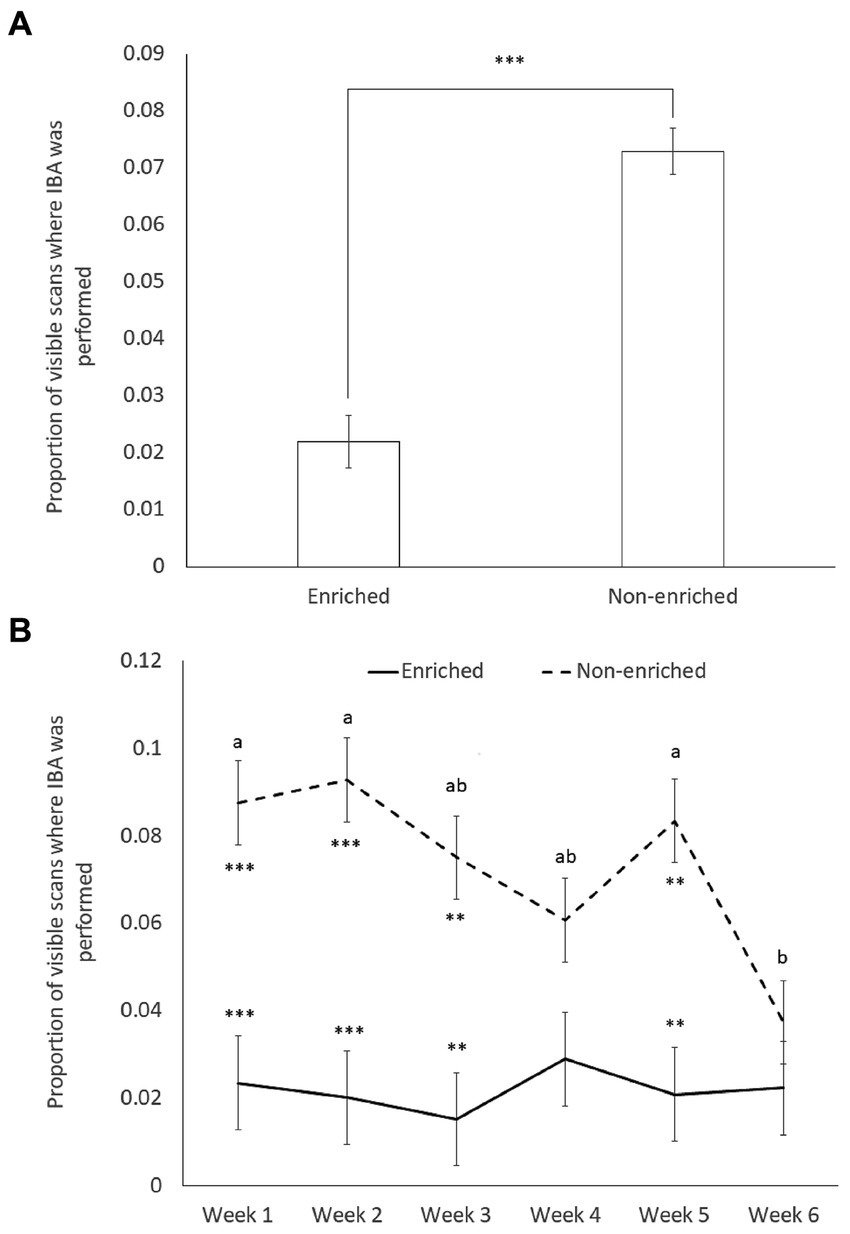
Figure 3. Least square means and standard errors of the proportion of visible scans displaying “inactive but awake” (IBA) behavior by mice housed in different post-weaning environments (enriched environment, n = 16; Non-enriched environment, n = 20). (A) Data averaged over the 6 weeks of experimentation (X21 = 39.615; p < 0.0001); (B) data by week (week × environment: X25 = 12.68; p = 0.027). Superscript letters indicate significant week differences within each environment at p < 0.05; environment differences are represented by ** for significance at p < 0.01 and *** for significance at p < 0.001.
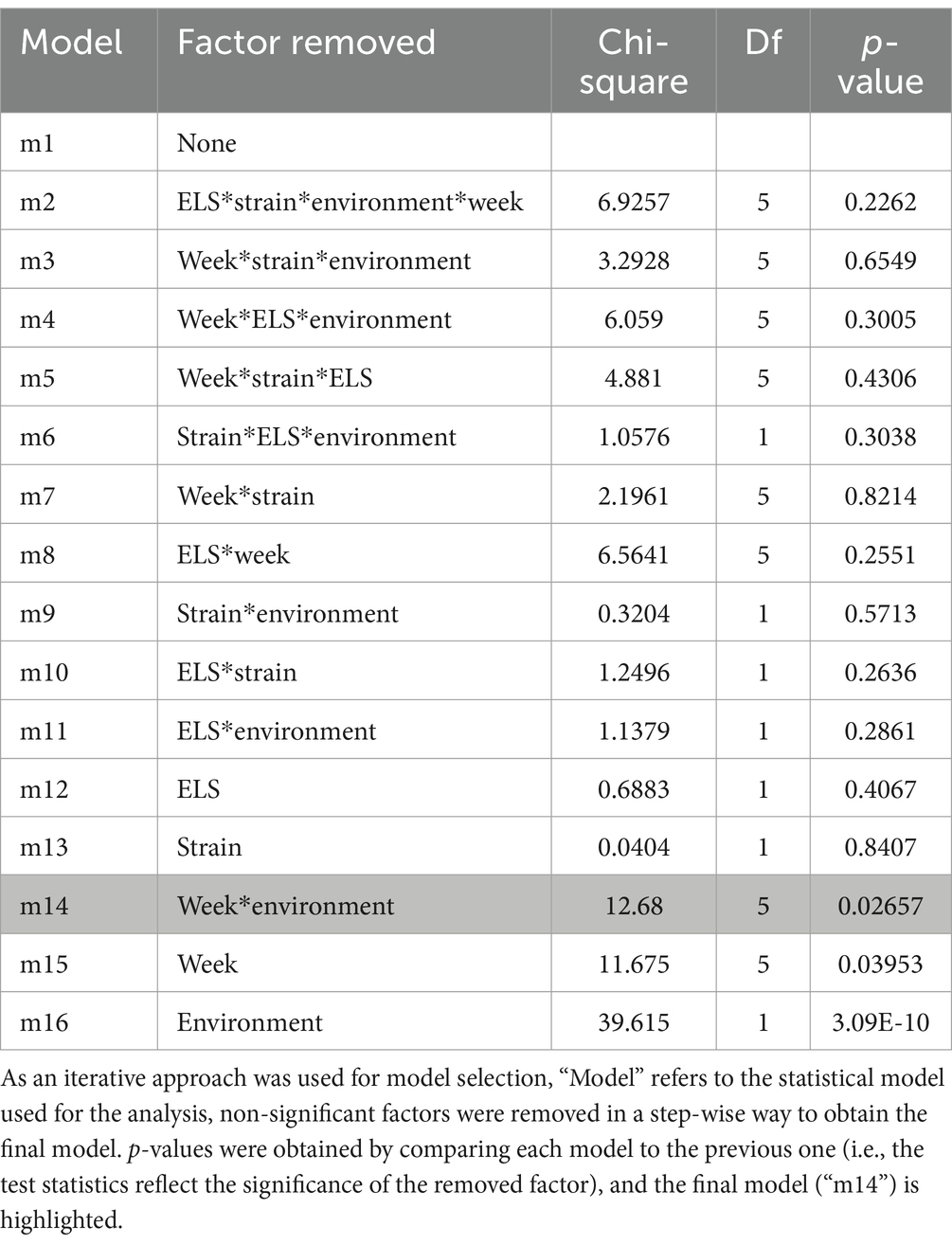
Table 3. Test statistics (Chi-Square), degrees of freedom (Df) and significance (p-value) of each fixed factor, and of the interaction between these factors, on the mice’ proportion of visible scans displaying IBA (averaged across the 6 weeks post-weaning).
Finally, although no specific predictions were made about the week factor, week (X25 = 11.675; p = 0.040) and its interaction with environment (X25 = 12.68; p = 0.027) significantly predicted the proportion of visible scans in which mice performed IBA (Table 3). Hence levels of IBA were significantly lower in enriched than in non-enriched mice for most observation periods (week 1: t204 = −4.45, p = 0.0008; week 2: t204 = −5.04, p = 0.0001; week 3: t204 = −4.16, p = 0.0027; week 5: t204 = −4.38, p = 0.0013), except during week 4 (t204 = −2.211, p = 0.5435) and week 6 (t204 = −1.04, p = 0.9966). During week 6 the levels of IBA in NE mice dropped significantly compared to week 5 (week 5 vs. week 6: t170 = −3.44, p = 0.0345) (Figure 3B).
4 Discussion
The present study investigated whether early life stress and/or genetic predispositions (i.e., two risk factors increasing vulnerability to depression in humans exposed to stressful life events) also trigger greater time spent IBA in mice exposed to stress at adulthood. As predicted, barren post-weaning environment significantly enhanced IBA levels, which confirmed previous findings, (e.g., 21–23). However, exposure to early-life stress via maternal separation did not significantly influence the likelihood of displaying IBA when exposed to a barren post-weaning environment. Furthermore, although the studied strains of mice (i.e., C57/6 J and DBA/2 J) significantly differed in their likelihood of displaying IBA in other studies (21, 23, 25), the present study did not find such an effect of genetic predisposition, as in Fureix et al. (24). Therefore, the results provide only partial support to our hypothesis that greater levels of IBA in mice are triggered by similar risk factors to those causing depression in humans. Several reasons could explain the lack of significant effects of maternal separation and strain on the level of IBA expressed in barren environments, which we discuss below.
First, this study started January 2020 and had to be interrupted due to the Covid-19 pandemic and could not be resumed after interruption (due to grant ending). Consequently, the sample sizes are lower than those originally planned from the power analysis, reducing the statistical power. Despite our reduction in statistical power, we still observed the differential housing treatment effect, which here was robust enough to reach significance regardless of the lack of power. That non-enriched cages trigger greater levels of IBA has indeed been replicated both across and within research institutes [Guelph, Canada: (21, 23); Bristol, UK: (24, 25)]. Such impoverished housing is also known to drastically impact mice welfare [e.g., increased stereotypic behaviors and aggression, weakened immunity, poorer breeding performances, and shortened life spans (22, 51–55)], and its detrimental effect might be too strong to be protected by the undisturbed early life condition. In contrast, the effects on IBA/ depressive-like phenotypes of genetic predispositions and exposure to early life stress might be less strong (as can be the effect of early life stress and genetic factors on human clinical depression) [(e.g., 5, 8, 56) as discussed below]. Therefore, their statistical investigation may have been more likely to be jeopardized by the decreased statistical power. This study must therefore be replicated with originally planned (larger) sample sizes (i.e., 11 mice per strain × ELS × enrichment condition, i.e., 88 individuals in total).
With regards to genetic factors, human depression is only moderately heritable (~40%), and a highly polygenic disease, i.e., influenced by very high numbers of gene variants of very small effects, rather than being triggered by a few gene variants of large effects [(e.g., 5–7)]. While genetic animal models of depression such as knockout mice (generated by single gene deletion) have provided important information about the exact role of specific genes in the pathophysiology of the illness, they have also shown limited applicability to the complex processes that occur within human depression, since they do not encompass the highly polygenic feature of the illness, (e.g., 57). Therefore, while genetic factors linked to neuronal growth, synaptic function and inflammation are at stake in triggering susceptibility to clinical depression, the exact genetic risk factors predisposing some people to the illness are not yet fully understood, (e.g., 6, 7). Moving away from testing the effect of specific gene(s) and conducting genome-wide association studies (a hypothesis free or ‘unbiased’ approach to detecting gene variants involved in an illness) is recognized as a way to move forward for animal models and human investigation. Such approaches are however not free from difficulties [e.g., very large sample sizes are required to have sufficient power to detect effects at a statistical threshold, as discussed in detail in, (e.g., 8)]. Moving towards interdisciplinary ethological x genome-wide association studies could nevertheless be a way to take the IBA investigation further.
The effect of the second predisposing risk factor to clinical depression we investigated here, i.e., exposure to early life stress, can be sensitive to the research design [(e.g., 5, 58)]. For instance, in humans, variations in defining and measuring early life stressors [(e.g., 32, 59, 60)] and in methods used to assess the depressive outcomes [(e.g., 5, 7, 8)] can result in different findings between studies. In animal models investigating how maternal separation impacts the development of depressive-like phenotypes, variations in the maternal separation procedure (61–63), housing and caring procedures, early life experience of the dam, sex of the pups and sample sizes can also influence the results (64). Creating a single maternal separation paradigm that would be fully identical and replicable across laboratories and within studies seems (realistically) unachievable. Combining results from investigations, each using different early life stressors (e.g., reduced bedding and nesting material (64), early weaning) may be an approach to target this replication difficulty. Alternatively, a potentially Refined approach with regards to the 3Rs (Mason, personal communication) to study the effect of early life stress on IBA development could be to create a contrast between early life conditions by making the control treatment much more positive than what it is normally (e.g., house the dams in highly enriched cages, allow natural weaning from the dams), rather than by making the early life environment more negative to create the early life stress. Studying the effect of maternal separation as an early life stressor nevertheless remain relevant for the study of welfare of captive species [especially dairy, (e.g., 65, 66)], which are often weaned much earlier than they would be under natural conditions (67).
Any one (or all) of the explanations discussed above may be responsible for our partially supported hypothesis that greater levels of IBA are triggered in mice by similar risk factors to those causing depression in humans. We can also question whether the failure to induce a significant difference in actual maternal attendance at the nest/offspring may also contribute to the lack of effect of maternal separation observed in our study, as it was suggested that the amount of maternal care would influence the emotional response of offspring, independently of the maternal separation treatment (68). Furthermore, maternal separation was conducted in the colony room for practical reason (space availability). We therefore cannot rule out the possibility that mice from the control undisturbed group may have been affected by maternal separation by being exposed to the treatment groups’ vocalizations. A further replicating study would need the maternal separation treatment to be applied in a different room from the room housing the undisturbed mice. Another possibility could be that genetic background and early life stress do not increase IBA levels when exposed to stress later in life because elevated IBA does not reflect a depression-like state. We must for instance cautiously acknowledge the possibility that greater levels of IBA in the home environment can be associated with a different affective state, such as tentatively putative boredom-like states in dogs (Canis lupus familiaris) (69). Greater levels of IBA in mice nevertheless covaries with other symptoms of depression [i.e., immobility in the forced swim test (21, 23), changes in sleep and weight (18), Trevarthen et al. in prep] and are reduced by administration of antidepressants (24), making testing further the depression-like state hypothesis worthwhile. Therefore, future research replicating the current study should be conducted using larger sample sizes and using several paradigms of early life stress. Furthermore, co-existence and co-variation of greater levels of IBA with the range of the human illness symptoms that can be operationalized in animals should be investigated, since co-existence of symptoms is of crucial relevance to the diagnosis of the condition in humans (18, 70).
5 Conclusion
This study further supports that barren ‘shoebox’ cages trigger a specific form of waking inactivity (IBA) in laboratory mice. Although no significant effect of early-life stress and genetic factors could be evidenced in this study, we encourage further research on this topic, especially studies using larger sample sizes, several paradigms of early life stress, and investigating the co-existence and co-variation of greater levels of IBA with the range of human depression symptoms that can be operationalized in animals.
Data availability statement
The datasets presented in this study can be found in online repositories. The names of the repository/repositories and accession number(s) can be found at: https://osf.io/94bf6/?view_only=b2dfebf15c5e470bbb8a85c5921c83f7.
Ethics statement
The animal study was approved by Home Office User Research Ethics Board (Licence number PPL P2556FBFE and P10DC2972). The study was conducted in accordance with the local legislation and institutional requirements (Animals Scientific Procedures Act 1986 (ASPA), the EU directive 2010/63/EU and the UK Home Office code of practice for the housing and care of animals bred, supplied, or used for scientific purposes).
Author contributions
OS: Data curation, Formal analysis, Investigation, Methodology, Visualization, Writing – original draft, Writing – review & editing. EF: Investigation, Methodology, Writing – review & editing. AT: Formal analysis, Methodology, Project administration, Supervision, Writing – review & editing. CW: Formal analysis, Writing – review & editing. EP: Methodology, Writing – review & editing. MM: Conceptualization, Funding acquisition, Project administration, Supervision, Writing – review & editing. CF: Conceptualization, Data curation, Funding acquisition, Methodology, Project administration, Supervision, Writing – review & editing.
Funding
The author(s) declare financial support was received for the research, authorship, and/or publication of this article. This project was funded by a BBSRC grant to CF and MM (grant number BB/P019218/1). The funders had no role in the study design, data collection, analyses and interpretation, decision to publish or preparation of the manuscript.
Acknowledgments
The authors would like to thank the Bristol University Animal Service Unit staff for caring for the animals and Louise Phelon for providing invaluable guidance during the breeding period.
Conflict of interest
The authors declare that the research was conducted in the absence of any commercial or financial relationships that could be construed as a potential conflict of interest.
Publisher’s note
All claims expressed in this article are solely those of the authors and do not necessarily represent those of their affiliated organizations, or those of the publisher, the editors and the reviewers. Any product that may be evaluated in this article, or claim that may be made by its manufacturer, is not guaranteed or endorsed by the publisher.
Footnotes
1. ^A term by which we mean “major depressive disorder” or having “depressive episodes”, to encompass Diagnostic Manual of Mental Disorders 5th edition & International Statistical Classification of Diseases & Related Health Problems ICD-11 terminologies.
2. ^At weaning, we obtained 9 pairs of mice (one C57 + one DBA) for each ELS condition (MS and UND), of which 4 pairs were assigned to “NE” and 5 were assigned to “EE.”
References
1. World Health Organisation . ICD-11 for mortality and morbidity statistics—depressive disorders. [internet]. ICD-11 for mortality and morbidity statistics. (2022) [cited 2022 Jun 21]. Available at: https://icd.who.int/browse11/l-m/en
2. American Psychiatric Association . Diagnostic and statistical manual of mental disorders (DSM-5). 5th ed. Arlington, VA, USA: American Psychiatric Publishing (2013).
3. Institute of Health Metrics and Evaluation . Global Health data exchange (GHDx) [internet]. [cited 2021 Dec 11]. Available at: http://ghdx.healthdata.org/gbd-results-tool?params=gbd-api-2019-permalink/d780dffbe8a381b25e1416884959e88b
4. Angst, J, Gamma, A, Gastpar, M, Lépine, JP, Mendlewicz, J, and Tylee, A. Gender differences in depression: epidemiological findings from the European DEPRES I and II studies. Eur Arch Psychiatry Clin Neurosci. (2002) 252:201–9. doi: 10.1007/s00406-002-0381-6
5. Assary, E, Vincent, JP, Keers, R, and Pluess, M. Gene-environment interaction and psychiatric disorders: review and future directions. Semin Cell Dev Biol. (2018) 77:133–43. doi: 10.1016/j.semcdb.2017.10.016
6. Musci, RJ, Augustinavicius, JL, and Volk, H. Gene-environment interactions in psychiatry: recent evidence and clinical implications. Curr Psychiatr Rep. (2019) 21:81. doi: 10.1007/s11920-019-1065-5
7. Kendall, KM, Van Assche, E, Andlauer, TFM, Choi, KW, Luykx, JJ, Schulte, EC, et al. The genetic basis of major depression. Psychol Med. (2021) 51:2217–30. doi: 10.1017/S0033291721000441
8. Dunn, EC, Brown, RC, Dai, Y, Rosand, J, Nugent, NR, Amstadter, AB, et al. Genetic determinants of depression: recent findings and future directions NIH public access. Harv Rev Psychiatry. (2015) 23:1–18. doi: 10.1097/HRP.0000000000000054
9. Sullivan, PF, Michael Neale, FC, and Kendler, KS. Reviews and overviews genetic epidemiology of major depression: review and Meta-analysis. Am J Psychiatry. (2000) 157:1552–62. doi: 10.1176/appi.ajp.157.10.1552
10. Norman, RE, Byambaa, M, De, R, Butchart, A, Scott, J, and Vos, T. The long-term health consequences of child physical abuse, emotional abuse, and neglect: a systematic review and Meta-analysis. PLoS Med. (2012) 9:e1001349. doi: 10.1371/journal.pmed.1001349
11. Weary, DM, Jasper, J, and Hötzel, MJ. Understanding weaning distress. Appl Anim Behav Sci. (2008) 110:24–41. doi: 10.1016/j.applanim.2007.03.025
12. Newberry, RC, and Swanson, JC. Implications of breaking mother-young social bonds. Appl Anim Behav Sci. (2008) 110:3–23. doi: 10.1016/j.applanim.2007.03.021
13. Ilska, J, Haskell, MJ, Blott, SC, Sánchez-Molano, E, Polgar, Z, Lofgren, SE, et al. Genetic characterization of dog personality traits. Genetics. (2017) 206:1101–11. doi: 10.1534/genetics.116.192674
14. Piovezan, U, Cyrillo, JNDSC, and Costa, MJ. Breed and selection line differences in the temperament of beef cattle. Acta Sci Anim Sci. (2013) 35:207–12. doi: 10.4025/actascianimsci.v35i2.16426
15. Lecorps, B, Weary, DM, and von Keyserlingk, MAG. Captivity-induced depression in animals. Trends Cogn Sci. (2021) 25:539–41. doi: 10.1016/j.tics.2021.03.010
16. Appleby, MC, Olsson, AS, and Galindo, F eds. Animal Welfare. 3rd ed. Wallingford, UK: CABI (2018). 408 p.
17. Fureix, C, and Meagher, RK. What can inactivity (in its various forms) reveal about affective states in non-human animals? A review. Appl Anim Behav Sci. (2015) 171:8–24. doi: 10.1016/j.applanim.2015.08.036
18. MacLellan, A, Fureix, C, Polanco, A, and Mason, G. Can animals develop depression? An overview and assessment of “depression-like” states. Behaviour. (2021) 158:1303–53. doi: 10.1163/1568539X-bja10132
19. Fureix, C, Jego, P, Henry, S, Lansade, L, and Hausberger, M. Towards an ethological animal model of depression? A study on horses. PLoS One. (2012) 7:1–9. doi: 10.1371/journal.pone.0039280
20. Kudryavtseva, NN, Bakshtanovskaya, IV, and Koryakina, LA. Social model of depression in mice of C57BL/6J strain. Pharmacol Biochem Behav. (1991) 38:315–20. doi: 10.1016/0091-3057(91)90284-9
21. Fureix, C, Walker, M, Harper, L, Reynolds, K, Saldivia-Woo, A, and Mason, G. Stereotypic behaviour in standard non-enriched cages is an alternative to depression-like responses in C57BL/6 mice. Behav Brain Res. (2016) 305:186–90. doi: 10.1016/j.bbr.2016.02.005
22. Tilly, SLC, Dallaire, J, and Mason, GJ. Middle-aged mice with enrichment-resistant stereotypic behaviour show reduced motivation for enrichment. Anim Behav. (2010) 80:363–73. doi: 10.1016/j.anbehav.2010.06.008
23. Nip, E, Adcock, A, Nazal, B, MacLellan, A, Niel, L, Choleris, E, et al. Why are enriched mice nice? Investigating how environmental enrichment reduces agonism in female C57BL/6, DBA/2, and BALB/c mice. Appl Anim Behav Sci. (2019) 217:73–82. doi: 10.1016/j.applanim.2019.05.002
24. Fureix, C, Trevarthen, AC, Finnegan, EM, Bu, K, Paul, ES, and Mendl, MT. Do greater levels of in-cage waking inactivity in laboratory mice reflect a spontaneous depression-like symptom? A pharmacological investigation. Pharmacol Biochem Behav. (2022) 212:173311. doi: 10.1016/j.pbb.2021.173311
25. Trevarthen, A, Mendl, M, Finnegan, E, Paul, E, Resasco, A, and Fureix, C. Inactive but awake behaviour as a potential indicator of a housing-induced depressive-like state in mice In: Universities Federation for Animal Welfare international symposium. eds. S. Wickens, R. Hubrecht, and H. Golledge Bruges, Belgium: Universities Federation for Animal Welfare (2019)
26. Filiou, MD, Nussbaumer, M, Teplytska, L, and Turck, CW. Behavioral and metabolome differences between c57bl/6 and dba/2 mouse strains: implications for their use as models for depression-and anxiety-like phenotypes. Meta. (2021) 11:1–12. doi: 10.3390/metabo11020128
27. de Sá-Calçada, D, Roque, S, Branco, C, Monteiro, S, Cerqueira-Rodrigues, B, Sousa, N, et al. Exploring female mice interstrain differences relevant for models of depression. Front Behav Neurosci. (2015) 9:1–12. doi: 10.3389/fnbeh.2015.00335
28. Wahlsten, D, Bachmanov, A, Finn, DA, and Crabbe, JC. Stability of inbred mouse strain differences in behavior and brain size between laboratories and across decades. Proc Natl Acad Sci USA. (2006) 103:16364–9. doi: 10.1073/pnas.0605342103
29. Richter, SH, Garner, JP, and Wurbel, H. Environmental standardization: cure or cause of poor reproducibility in animal experiments? Nat Methods. (2009) 6:257–61. doi: 10.1038/nmeth.1312
30. Antoniuk, S, Bijata, M, Ponimaskin, E, and Wlodarczyk, J. Chronic unpredictable mild stress for modeling depression in rodents: Meta-analysis of model reliability. Neurosci Biobehav Rev. (2019) 99:101–16. doi: 10.1016/j.neubiorev.2018.12.002
31. Mul, JD . Voluntary exercise and depression-like behavior in rodents: are we running in the right direction? J Mol Endocrinol. (2018) 60:R77–95. doi: 10.1530/JME-17-0165
32. Infurna, MR, Reichl, C, Parzer, P, Schimmenti, A, Bifulco, A, and Kaess, M. Associations between depression and specific childhood experiences of abuse and neglect: a meta-analysis. J Affect Disord. (2016) 190:47–55. doi: 10.1016/j.jad.2015.09.006
33. Van Veen, T, Wardenaar, KJ, Carlier, IVE, Spinhoven, P, Penninx, BWJH, and Zitman, FG. Are childhood and adult life adversities differentially associated with specific symptom dimensions of depression and anxiety? Testing the tripartite model. J Affect Disord. (2013) 146:238–45. doi: 10.1016/j.jad.2012.09.011
34. Gracia-Rubio, I, Moscoso-Castro, M, Pozo, OJ, Marcos, J, Nadal, R, and Valverde, O. Maternal separation induces neuroinflammation and long-lasting emotional alterations in mice. Prog Neuropsychopharmacology Biol Psychiatry. (2016) 65:104–17. doi: 10.1016/j.pnpbp.2015.09.003
35. Christmas, DM, Potokar, JP, and Davies, SJ. A biological pathway linking inflammation and depression: activation of indoleamine 2,3-dioxygenase. Neuropsychiatr Dis Treat. (2011) 7:431–9. doi: 10.2147/NDT.S17573
36. Mizoguchi, H, Fukumoto, K, Sakamoto, G, Jin, S, Toyama, A, Wang, T, et al. Maternal separation as a risk factor for aggravation of neuropathic pain in later life in mice. Behav Brain Res. (2018) 359:942–9. doi: 10.1016/j.bbr.2018.06.015
37. Yu, X, Yao, H, Zhang, X, Liu, L, Liu, S, and Dong, Y. Comparison of LPS and MS-induced depressive mouse model: behavior, inflammation and biochemical changes. BMC Psychiatry. (2022) 22:1–11. doi: 10.1186/s12888-022-04233-2
38. Chourbaji, S, Zacher, C, Sanchis-Segura, C, Spanagel, R, and Gass, P. Social and structural housing conditions influence the development of a depressive-like phenotype in the learned helplessness paradigm in male mice. Behav Brain Res. (2005) 164:100–6. doi: 10.1016/j.bbr.2005.06.003
39. Belzung, C, and Lemoine, M. Criteria of validity for animal models of psychiatric disorders: focus on anxiety disorders and depression. Biol Mood Anxiety Disord. (2011) 1:1–14. doi: 10.1186/2045-5380-1-9
40. Caspi, A, Sugden, K, Moffitt, TE, Taylor, A, Craig, IW, Harrington, HL, et al. Influence of life stress on depression: moderation by a polymorphism in the 5-HTT gene. Science. (2003) 301:386–9. doi: 10.1126/science.1083968
41. Hurst, JL, and West, RS. Taming anxiety in laboratory mice. Nat Methods. (2010) 7:825–6. doi: 10.1038/nmeth.1500
42. Walker, M, Fureix, C, Palme, R, and Mason, G. Co-housing rodents with different coat Colours as a simple, non-invasive means of individual identification: validating mixed-strain housing for C57BL/6 and DBA/2 mice. PLoS One. (2013) 8:1–12. doi: 10.1371/journal.pone.0077541
43. Walker, M, Fureix, C, Palme, R, Newman, JA, Ahloy Dallaire, J, and Mason, G. Mixed-strain housing for female C57BL/6, DBA/2, and BALB/c mice: validating a split-plot design that promotes refinement and reduction study design. BMC Med Res Methodol. (2016) 16:1–13. doi: 10.1186/s12874-016-0113-7
44. Brajon, S, Munhoz Morello, G, Teixeira, MS, Hultgren, J, Gilbert, C, and Olsson, IAS. Social environment as a cause of litter loss in laboratory mouse: a behavioural study. Appl Anim Behav Sci. (2019) 218:104827. doi: 10.1016/j.applanim.2019.06.008
45. Alves, RL, Portugal, CC, Summavielle, T, Barbosa, F, and Magalhães, A. Maternal separation effects on mother rodents’ behaviour: a systematic review. Neurosci Biobehav Rev. (2019) 117:98–109. doi: 10.1016/j.neubiorev.2019.09.008
46. Martin, P, and Bateson, M. Measuring behaviour: An introductory guide. 4th ed. Cambridge, UK: Cambridge university press (2021).
47. R Core Team . R: A language and environment for statistical computing. Vienna, Austria: R Foundation for Statistical Computing (2020) Available at: https://www.r-project.org/.
48. Bates, D, Maechler, M, and Bolker, BW. Fitting linear mixed-effects models using lme4. J Stat Softw. (2015) 67:1–48. doi: 10.18637/jss.v067.i01
49. Kuznetsova, A, Brockhoff, PB, and Christensen, RHB. lmerTest package: tests in linear mixed effects models. J Stat Softw. (2017) 82:1–26. doi: 10.18637/jss.v082.i13
50. Lenth, RV . Least-squares means: the R package lsmeans. J Stat Softw. (2016) 69:1–33. doi: 10.18637/jss.v069.i01
51. Olsson, AI, and Dahlborn, K. Improving housing conditions for laboratory mice: a review of “environmental enrichment”. Lab Anim. (2002) 36:243–70. doi: 10.1258/002367702320162379
52. Meikle, MN, Arévalo, AP, Schlapp, G, Fernández-Graña, G, Menchaca, A, and Crispo, M. Long-term effect of environmental enrichment on reproductive performance of Swiss Webster mice and their female offspring. Animals. (2020) 10:1438. doi: 10.3390/ani10081438
53. Thanos, PK, Hamilton, J, O’rourke, JR, Napoli, A, Febo, M, Volkow, ND, et al. Dopamine D 2 gene expression interacts with environmental enrichment to impact lifespan and behavior. Oncotarget. (2016) 7:19111–23. doi: 10.18632/oncotarget.8088
54. Gaskill, BN, Winnicker, C, Garner, JP, and Pritchett-Corning, KR. The naked truth: breeding performance in nude mice with and without nesting material. Appl Anim Behav Sci. (2013) 143:110–6. doi: 10.1016/j.applanim.2012.10.009
55. Brod, S, Gobbetti, T, Gittens, B, Ono, M, Perretti, M, and D’acquisto, F. The impact of environmental enrichment on the murine inflammatory immune response. JCI Insight. (2017) 2:e90723. doi: 10.1172/jci.insight.90723
57. Scherma, M, Giunti, E, Fratta, W, and Fadda, P. Gene knockout animal models of depression, anxiety and obsessive compulsive disorders. Psychiatr Genet. (2019) 29:191–9. doi: 10.1097/YPG.0000000000000238
58. Li, M, Liu, S, D’Arcy, C, Gao, T, and Meng, X. Interactions of childhood maltreatment and genetic variations in adult depression: a systematic review. J Affect Disord. (2020) 276:119–36. doi: 10.1016/j.jad.2020.06.055
59. Braithwaite, EC, O’Connor, RM, Degli-Esposti, M, Luke, N, and Bowes, L. Modifiable predictors of depression following childhood maltreatment: a systematic review and meta-analysis. Transl Psychiatry. (2017) 7:e1162. doi: 10.1038/tp.2017.140
60. Li, ET, Luyten, P, and Midgley, N. Psychological mediators of the association between childhood emotional abuse and depression: a systematic review. Front Psych. (2020) 11:559213. doi: 10.3389/fpsyt.2020.559213
61. Schmidt, MV, Wang, XD, and Meijer, OC. Early life stress paradigms in rodents: potential animal models of depression? Psychopharmacology. (2011) 214:131–40. doi: 10.1007/s00213-010-2096-0
62. Tractenberg, SG, Levandowski, ML, de Azeredo, LA, Orso, R, Roithmann, LG, Hoffmann, ES, et al. An overview of maternal separation effects on behavioural outcomes in mice: evidence from a four-stage methodological systematic review. Neurosci Biobehav Rev. (2016) 68:489–503. doi: 10.1016/j.neubiorev.2016.06.021
63. Tan, S, Ho, HS, Song, AY, Low, J, and Je, HS. Maternal separation does not produce a significant behavioral change in mice. Exp Neurobiol. (2017) 26:390–8. doi: 10.5607/en.2017.26.6.390
64. Murthy, S, and Gould, E. Early life stress in rodents: animal models of illness or resilience? Front Behav Neurosci. (2018) 12:157. doi: 10.3389/fnbeh.2018.00157
65. Toinon, C, Waiblinger, S, and Rault, JL. Maternal deprivation affects goat kids’ stress coping behaviour. Physiol Behav. (2021) 239:113494. doi: 10.1016/j.physbeh.2021.113494
66. Meagher, RK, Beaver, A, Weary, DM, and von Keyserlingk, MAG. Invited review: a systematic review of the effects of prolonged cow–calf contact on behavior, welfare, and productivity. J Dairy Sci. (2019) 102:5765–83. doi: 10.3168/jds.2018-16021
67. Latham, NR, and Mason, GJ. Maternal deprivation and the development of stereotypic behaviour. Appl Anim Behav Sci. (2008) 110:84–108. doi: 10.1016/j.applanim.2007.03.026
68. Bailoo, JD, Jordan, RL, Garza, XJ, and Tyler, AN. Brief and long periods of maternal separation affect maternal behavior and offspring behavioral development in C57BL/6 mice. Dev Psychobiol. (2014) 56:674–85. doi: 10.1002/dev.21135
69. Harvey, ND, Moesta, A, Kappel, S, Wongsaengchan, C, Harris, H, Craigon, PJ, et al. Could greater time spent displaying waking inactivity in the home environment be a marker for a depression-like state in the domestic dog? Animals. (2019) 9:420. doi: 10.3390/ani9070420
Keywords: depression-like states, waking inactivity, maternal separation, environment, rodents
Citation: Schmitt O, Finnegan E, Trevarthen A, Wongsaengchan C, Paul ES, Mendl M and Fureix C (2024) Exploring the similarities between risk factors triggering depression in humans and elevated in-cage “inactive but awake” behavior in laboratory mice. Front. Vet. Sci. 11:1348928. doi: 10.3389/fvets.2024.1348928
Edited by:
Edward Narayan, The University of Queensland, AustraliaReviewed by:
Ori Pomerantz, University of California, Davis, United StatesJames Edward Brereton, Sparsholt College, United Kingdom
Copyright © 2024 Schmitt, Finnegan, Trevarthen, Wongsaengchan, Paul, Mendl and Fureix. This is an open-access article distributed under the terms of the Creative Commons Attribution License (CC BY). The use, distribution or reproduction in other forums is permitted, provided the original author(s) and the copyright owner(s) are credited and that the original publication in this journal is cited, in accordance with accepted academic practice. No use, distribution or reproduction is permitted which does not comply with these terms.
*Correspondence: Oceane Schmitt, schmitt.oce@gmail.com; Carole Fureix, carole.fureix@bristol.ac.uk
†Present addresses: Oceane Schmitt, Institute of Animal Breeding and Genetics, Justus-Liebig University of Giessen, Giessen, Germany Chanakarn Wongsaengchan, Institute of Behavioral and Neural Sciences, University of St Andrews, St Andrews, Germany