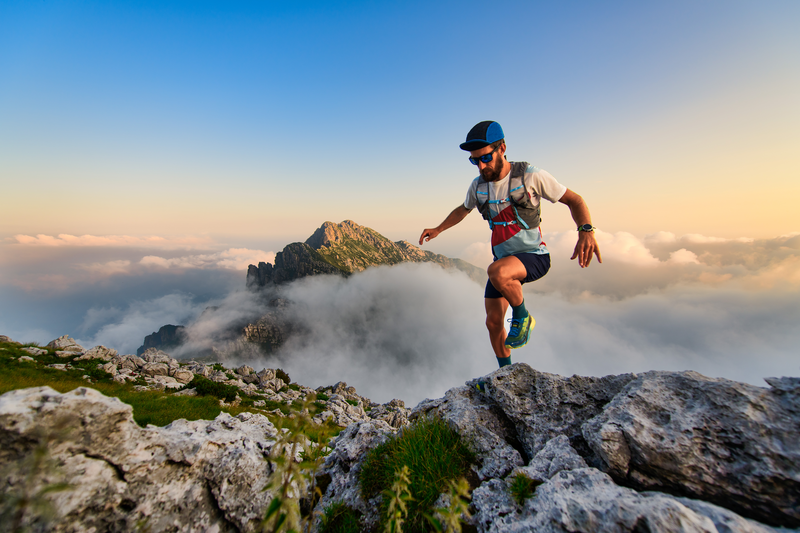
94% of researchers rate our articles as excellent or good
Learn more about the work of our research integrity team to safeguard the quality of each article we publish.
Find out more
ORIGINAL RESEARCH article
Front. Vet. Sci. , 23 April 2024
Sec. Veterinary Infectious Diseases
Volume 11 - 2024 | https://doi.org/10.3389/fvets.2024.1346313
Introduction: Canine bacterial keratitis is a corneal infection that causes various symptoms, including visual impairment, and necessitates eye removal in severe cases. Staphylococcus pseudintermedius is a pathogen that causes significant bacterial keratitis in canine patients. Moreover, multi-drug resistant Staphylococcus pseudintermedius (MDRSP) has been reported in both humans and animals. Regarding treatment failure against multi-drug resistant (MDR) pathogens with classic antibiotics, antimicrobial compounds derived from probiotics have been suggested as an alternative approach.
Methods: Ligilactobacillus animalis SWLA-1 strain and its cell-free supernatant (CFS) have previously demonstrated potent antimicrobial activity against various MDR pathogenic bacteria. Based on this finding, we evaluated the anti-staphylococcal activity of CFS derived from Ligilactobacillus animalis SWLA-1 against MDRSP in a newly established ex vivo canine corneal infection model using fresh canine corneoscleral rims. Additionally, an in vitro cytotoxicity test using human keratocytes was performed.
Results and Discussion: CFS significantly inhibited the growth of MDRSP in the novel ex vivo model and did not exhibit any significant toxicity against keratocytes in vitro. Based on these results, the antimicrobial compounds in CFS show potential as a novel approach for MDR staphylococcal keratitis treatment.
Bacterial keratitis is one of the most common type of infectious keratitis in dogs (1), and the major pathogenic bacteria involved in canine corneal ulcers have been isolated and identified. The most prevalent bacterial genera are Staphylococcus spp., Streptococcus, and Pseudomonas. Staph. pseudintermedius is the predominant species responsible for canine infectious keratitis in numerous geographical regions (2–7). The emergence of multi-drug resistant (MDR) bacteria, attributed to the misuse and abuse of antimicrobial agents, poses a major global threat to humans and animals (8). In the case of canine bacterial pathogens, MDR Staph. pseudintermedius (MDRSP) is a dominant major pathogen with antibiotic resistance (9). As this bacterium is known to be a major pathogen of bacterial keratitis and corneal ulcers in dogs, there have been reported cases of MDRSP infection, including strains belonging to methicillin-resistant Staph. pseudintermedius (MRSP) (10–12). Furthermore, many MDRSP strains exhibit resistance to antimicrobial agents used in veterinary ophthalmology, such as fluoroquinolones and tetracyclines (13, 14).
Action plans to combat MDR bacteria and safeguard important antibiotics have been in operation since the late 20th century within international communities. The World Health Organization, Food and Agriculture Organization, and World Organization for Animal Health (formerly known as Office International des Epizooties) have recommended guidelines for the responsible use of antimicrobial agents and listed critically important antibiotics in human and veterinary medicine (15–17). Furthermore, recognizing the need for alternatives to classic antibiotics against MDR bacteria, several proactive studies have suggested discovering and developing novel alternative approaches that safeguard classic antibiotics (18–20). Among them, antimicrobial molecules produced by probiotics, including bacteriocins and organic acids, have been considered promising alternatives to classical antibiotics and an arsenal to fight against MDR bacteria. Various kinds of these molecules have been discovered and reported for their antimicrobial effect (21–23).
Ligilactobacillus animalis SWLA-1 strain and its cell-free supernatant (CFS), which has versatile antimicrobial activity against both Gram-positive and Gram-negative MDR bacteria, have an inhibitory effect against MDR Staphylococcus spp. in vitro (24). Based on the findings, we aimed to evaluate the antimicrobial activity of CFS derived from L. animalis SWLA-1 against the MDR Staph. pseudintermedius KUVM1701GC strain as a potential alternative to commercial ophthalmic antibiotic agents (Figure 1). To simulate the ocular environment resulting from a staphylococcal infection, a new ex vivo infection model was established using fresh canine corneoscleral rims (CSRs). In addition, an in vitro cytotoxicity assay was performed to determine the cytotoxic effects induced by the CFS derived from L. animalis SWLA-1 on live keratocytes.
Figure 1. Schematic workflow of the ex vivo canine corneal infection model. Each procedure was performed in a sterile environment in a biosafe laminar flow hood. (A) Cross-sectional view of the canine cornea, including four layers: epithelium, stroma, Descemet’s membrane, and endothelium. (B) Rotating corneal brushes were used to stimulate corneal epithelial removal. (C) The corneoscleral rim (CSR) was obtained and placed in a sterile silicone mold with the epithelial side facing down. The agar was then filled with soft agar and left to stand until it solidified. CSRs with solidified agar were placed in a 6-well culture plate with the epithelial side facing up. The culture well was filled with culture medium to the level of the limbus of the CSRs. Culture wells were incubated on a rocking platform. (D) Inoculum of MDRSP was applied to the CSR defect sites. (E) Thereafter, the same amount of topical treatment was applied per experimental design within a biosafe hood. (F) After 48 h of incubation, corneal samples were harvested, and soft agar was removed from each CSR. (G) For microbiological analysis, corneal samples were transferred to individual sterile tubes containing 10 mL polybutylene succinate (PBS). *MDRSP: Multi-drug-resistant Staphylococcus pseudintermedius.
Canine CSRs were cultured as previously described in ex vivo canine models (12). Fresh canine CSRs were obtained from 15 dogs euthanized for reasons unrelated to this study. A total of 30 eyes were extracted from 15 canine subjects. However, six eyes were excluded from the experimental group due to observed corneal pigmentation and degeneration, rendering them unsuitable for the study. Briefly, enucleation of the eye globes was performed by a veterinarian, and no ophthalmic illness was confirmed by macroscopic evaluation. Enucleated globes were immersed in 10% povidone iodine for 30 min at room temperature. Subsequently, the povidone iodine was replaced with sterile phosphate-buffered saline until all visible traces of povidone iodine were eliminated (25). Following this, all globes were transferred to Dulbecco’s modified Eagles’ medium containing 100 μg/mL of streptomycin, 100 units/mL of penicillin, and 0.25 μg/mL of amphotericin. Using slit lamp biomicroscopy, unsuitable samples for this study were excluded from all enucleated eye globes. The corneal culture medium consisted of Dulbecco’s modified Eagle’s medium and Ham’s F-12c nutrient mixture at a ratio of 1:1, supplemented with 10% fetal bovine serum, HEPES buffer, and 0.025% chondroitin sulfate; moreover, the medium was fortified with 10% antimicrobial solution (100 μg/mL of streptomycin, 100 units/mL of penicillin, and 0.25 μg/mL of amphotericin), glutathione, and 1% L-glutamine solution (12).
A frozen pure culture of L. animalis SWLA-1 was thawed and placed on Difco de Man Rogosa Sharp (MRS) agar (BD Biosciences, Sparks, MD, USA). The plate was incubated at 37°C for 24 h. Five single colonies of L. animalis SWLA-1 were selected from the plate, inoculated into the MRS broth, and incubated overnight. This bacterial culture (1.2 × 109 colony-forming unit [CFU]/mL) was collected and centrifuged at 10,000 × g and 4°C for 30 min (Legend X1R; Thermo Fisher Scientific, Waltham, MA, USA) to obtain the crude CFS. As the antimicrobial activity of this CFS was bacteriocin-like, based on a previous study (24), it was concentrated using the trichloroacetic acid (TCA) protein precipitation method. Briefly, 10 mL TCA solution (Sigma-Aldrich, St. Louis, MO, USA) was added to 40 mL crude L. animalis SWLA-1-derived CFS. The samples were incubated for 1 h at 4°C for precipitation. The sample was centrifuged at 14,000 × g and 4°C for 10 min. The supernatant was then removed, leaving the protein pellet intact. This pellet was washed with 2 mL of chilled acetone and centrifuged at 14,000 × g and 4°C for 10 min. The washing step was repeated, and the pellet was dried in a 95°C heat block to evaporate any residual acetone. The pure protein pellet was re-dissolved in 50 μL of dimethyl sulfoxide (Sigma-Aldrich, St. Louis, MO, USA) and diluted with 950 μL of Milli-Q water. The protein concentration of the concentrated CFS was quantified using bicinchoninic acid (BCA) protein assay (Pierce™ BCA Protein Assay Kits, Thermo Fisher, USA) and compared with that of the crude CFS. Albumin protein standards were prepared following manufacturer’s recommendation. These protein standards and concentrated CFS were diluted in the working reagent. 25 μL of each sample was mixed with 200 μL of the working reagent at 96-well microliter plate and incubated in 37°C for 30 min. Every mixture was cooled at room temperature and measured the absorbance at OD562 on plate reader. Finally, the concentration of CFS was determined using the standard curve, which was generated by plotting blank control and each protein standard. The concentrated CFS samples (5×, 10×, 20×, and 40×) were also evaluated to determine the minimum inhibitory concentration against the indicator bacteria.
The MDRSP strain isolated from a clinical specimen provided by the Konkuk University Veterinary Medical Teaching Hospital (KUVMTH) and designated as Staph. pseudintermedius KUVM1701GC was used as the MDR indicator bacterium in this study. This bacterium was collected from the canine patient with chronic endophthalmitis and dermatitis around face. The pure bacterial culture was isolated from eye and skin swab specimen and kept in-80°C. Frozen cultures of Staph. pseudintermedius KUVM1701GC were thawed and plated on sheep blood agar. The agar plates were incubated at 37°C for 24 h. Five single colonies of this bacterium were inoculated into tryptic soy broth (TSB; BD, France) and incubated overnight. These bacterial cultures were used in an ex vivo model of corneal infection. Using the microdilution method, the antimicrobial susceptibility profiles of the indicator bacteria were evaluated using 15 antimicrobial agents, according to the guidelines of the Clinical Laboratory Standard Institute (CLSI VET01, 2019) and the European Committee on Antimicrobial Susceptibility Testing (EUCAST ver.11.0, 2021).
To mimic in vivo bacterial keratitis, corneal anatomical defenses must be compromised. Using a sterile 6-mm punch biopsy (BIOPSY PUNCH, KAI medical, Japan), a same-diameter defect was created at the center of each cornea. Next, an epithelial brush (Occubrush, Occutech, South Korea) and a rotating brush (Amoils epithelial scrubber; Innovative Excimer Solutions, Inc., Toronto, Canada) were used to consistently remove the corneal epithelium. The corneal epithelium was removed for approximately 10s until no remaining attachments were found in the marking margins. The cornea and scleral rim (5 mm from the limbus) were then excised using a sterile disposable scalpel (15 T, Paragon®, England).
Before preparing the CSR to be placed on the agar base and culture medium, structural support was applied to each CSR to maintain the curvature of the cornea intact. Each cornea was placed on a hemispherical dome silicone mold with a concave-shaped molding surface and filled with low-melting agarose gel (UltraPure LMP Agarose, Thermo Fisher Scientific, Waltham, MA, USA) to prevent excessive heat damage to the cornea. The CSRs were filled with a sterile soft agarose gel and incubated at room temperature for 30 min until the agar solidified. Upon solidification of the agarose solution, the prepared CSRs were placed with the epithelial side facing up on Falcon® 6-well cell culture plates (Corning, NY, USA).
Six prepared CSRs were placed on each 6-well cell culture plate and divided into six groups; (1) Group 1: 20 μL of PBS inoculated on the corneal defect; (2) Group 2: 20 μL of Staph. pseudintermedius KUVM1701GC culture inoculated on corneal defect and treated with 20 μL of PBS; (3) Group 3: 20 μL of Staph. pseudintermedius KUVM1701GC culture inoculated on corneal defect and treated with 20 μL of vancomycin (20 μg/mL); (4) Group 4: 20 μL of Staph. pseudintermedius KUVM1701GC culture inoculated on corneal defect and treated with 20 μL of ofloxacin (3 mg/mL); (5) Group 5: 20 μL of Staph. pseudintermedius KUVM1701GC culture inoculated on corneal defect and treated with 20 μL of concentrated CFS (20 ) derived from L. animalis SWLA-1 and (6) Group 6: 20 μL of Staph. pseudintermedius KUVM1701GC culture inoculated on corneal defect and treated with 10 μL of concentrated CFS (20 ) derived from L. animalis SWLA-1 and 10 μL of ofloxacin (3 mg/mL). The CSRs belonged to group 1 and group 2 were not treated with any antibiotics in this experiment. Additionally, the CSRs inoculated with indicator bacteria were pre-incubated for 1 h before being treated with PBS, antibiotics or concentrated CFS. A bacterial culture of Staph. pseudintermedius KUVM1701GC (OD600 = 0.210, 5 × 106 CFU/mL) was used according to the CFU range described by Ubani-Ukoma et al. (25). Since the lowest concentration of concentrated CFS derived from L. animalis SWLA-1 that could inhibit indicator bacteria was 10-fold (118.82 ± 3.27 mg/mL), 20-fold concentrated CFS (232.96 ± 5.23 mg/mL) was used in this experiment, with equal volumes of CFS and inoculum applied to the corneal defect. As the indicator bacteria were susceptible to vancomycin and resistant to ofloxacin, these antibiotics were used as reference antibiotics. Treatment with PBS, antibiotics, or concentrated CFS was administered twice a day at 12-h intervals for 48 h. In Group 6, a combination of concentrated CFS and ofloxacin was used to treat the indicator bacteria to determine any synergistic effects between these compounds.
Culture medium was added to each prepared CSR in the well of culture plates until reaching the limbus. The culture plates were placed on a rocking platform shaker, rotating clockwise in three-dimensional axes (20 rotations/min) in a humidified incubator at 37°C with 5% CO2 for 48 h.
After 48 h, 8 mm of corneal tissue, including the center of the corneal defect, was aseptically collected from each CSR using a sterile 8-mm punch biopsy (BIOPSY PUNCH, KAI Medical, Japan). The collected corneal tissues were suspended in 10 mL of sterile PBS and serially 10-fold diluted for viable colony counting of Staph. pseudintermedius KUVM1701GC. Using the Miles and Misra method, each 20 μL of diluted sample was placed on Staphylococcus No. 110 medium plates (Oxoid, UK). The plates were incubated at 37°C for 24 h. After incubation, the bacterial colonies were counted, and the measured CFU value of each sample was logarithmically transformed (log10 CFU/mL) to compare the mean differences in counts between the experimental groups. Experiments were performed independently using four copies of the culture plates.
The change in corneal opacity after bacterial infection with CSR in the ex vivo corneal infection model was evaluated in each experimental group by three veterinary ophthalmologists. The veterinarians randomly scored pictures of Staphylococcus-negative or Staphylococcus-positive CSR for each experimental group without any information related to the treatments. The scoring of each corneal opacity on macroscopic examination was evaluated using the semiquantitative preclinical ocular toxicology scoring (SPOTS) system (26) and recorded. Briefly, based on this system, the severity of corneal opacity was scored 24 h and 48 h after infection using the SPOTS system (0-normal cornea, 1-minimal loss of corneal transparency, 2-mild loss of corneal transparency, 3-moderate loss of corneal transparency).
Human keratocytes (ScienCell, Catalog #6520) were obtained and cultured in a fibroblast medium (ScienCell, Catalog #2301). Cultures were maintained at 37°C in an atmosphere with 5% CO2.
The cytotoxicity of the concentrated CFS derived from L. animalis SWLA-1 (10 , 118.82 ± 3.27 mg/mL) on human keratocytes was evaluated using Cell Counting Kit-8 assay. Human keratocytes were seeded at a density of 1 × 104 cells per well in a 96-well plate. After 24 h, the cells were treated with serial concentrations of CFS for 72 h. Following the removal of the supernatant, 10% CCK-8 reagent (Sigma-Aldrich, 96,992) in fresh serum-free media was added to the cells. Cells were incubated in a 5% CO2 atmosphere at 37°C. After 3 h, a spectrophotometer (Sunrise microplate reader, Tecan, Austria) was used to measure the absorbance of the viable cells at 450 nm. Cytotoxicity was analyzed by comparing the viability of mock-treated cells, which were considered 100% viable controls. This experiment was performed independently in triplicate.
The quantitative data in this study were evaluated for normality using the Shapiro–Wilk test. As the mean values of corneal opacity scores and CFU data from corneal samples were not normally distributed, the Kruskal–Wallis test was used to non-parametrically compare the multiple means of the experimental data from each group. This test was used to analyze the differences in the observed mean score or CFU data between the groups. Subsequently, the post-hoc Dunn’s test was used to compare the mean scores and CFU data of each group. Since the observed data were non-parametrically distributed, the Scheirer–Ray–Hare test was also used to analyze the relationships between the mean viable counts of the indicator bacteria and the two variables (experimental groups and corneal opacity scores). Post-hoc Dunn’s test was used to compare the experimental data of each group. All tests were performed using the “rstatix” package [version 0.6.0; R Foundation for Statistical Computing, Vienna, Austria; (27)] and “rcompanion” package [version 2.4.3; Rutgers Cooperative Extension, New Brunswick, New Jersey, USA; (28)] in R (version 4.3.1; R Foundation for Statistical Computing, Vienna, Austria; R core team 2023). Significance was set at an α level of 0.05.
The antimicrobial susceptibility profile of Staph. pseudintermedius KUVM1701GC was determined using the microdilution method (Table 1). Among the 15 antimicrobial agents used in this study, the indicator bacteria were resistant to 12 antibiotics (ciprofloxacin, ofloxacin, oxacillin, ampicillin, tetracycline, ceftriaxone, chloramphenicol, gentamicin, azithromycin, trimethoprim/sulfamethoxazole, ceftazidime, and cefotaxime). Only three agents (vancomycin, clindamycin, and amikacin) demonstrated susceptibility to this bacterium. Staph. pseudintermedius KUVM1701GC was identified as a methicillin-resistant and MDRSP.
Table 1. Antimicrobial susceptibility profile of the Staphylococcus pseudintermedius KUVM1701GC strain with the minimum inhibitory concentration.
The susceptibility of concentrated CFS derived from L. L. animalis SWLA-1 against indicator bacteria was also determined using the microdilution method. The minimum inhibitory concentration of CFS was 10-fold concentrated one (118.82 ± 3.27 mg/mL), while the inhibitory effect against Staph. pseudintermedius KUVM1791GC was not observed from the CFS concentrated 5-fold (55.74 ± 6.54 mg/mL).
The changes in corneal opacity after the inoculation of indicator bacteria at each time point (0, 24, and 48 h) are shown in Figure 2. Macroscopic findings showed that corneal opacity increased with time in all experimental groups except for the PBS-only and vancomycin-treated groups. As shown in Figure 3, the mean corneal opacity scores of the groups treated with vancomycin and ofloxacin were significantly lower than those in the Staphylococcus-positive control group (p < 0.01). In contrast, the group treated with concentrated CFS or a combination of concentrated CFS and ofloxacin had significantly higher scores than the Staphylococcus-negative control group or vancomycin-treated group (p < 0.01). Notably, no significant difference was observed between the ofloxacin-treated group and the CFS or combination of CFS and ofloxacin-treated groups (p > 0.05).
Figure 2. Observation of changes in corneal opacity along the time points. The experimental groups are as follows: PBS only, Staphylococcus-negative and inoculated with sterile PBS only; SP + PBS, Staphylococcus-positive and treated with PBS; SP + OFX, Staphylococcus-positive and treated with ofloxacin (3 mg/mL); SP + CCFS, Staphylococcus-positive and treated with concentrated CFS derived from L. animalis SWLA-1 (20×, 232.96 ± 5.23 mg/mL); CCFS+OFX, Staphylococcus-positive and treated with a combination of concentrated CFS (20×, 232.96 ± 5.23 mg/mL) and ofloxacin (3 mg/mL); SP + VA, Staphylococcus-positive and treated with vancomycin (20 μg/mL). PBS: polybutylene succinate.
Figure 3. Differences in the mean corneal opacity score between experimental groups. The values of all groups were analyzed using the Kruskal–Wallis test, followed by Dunn’s test for post-hoc testing. The experimental groups are as follows: Neg, Staphylococcus-negative and inoculated with sterile PBS only; Pos, Staphylococcus-positive and treated with PBS; VA, Staphylococcus-positive and treated with vancomycin (20 μg/mL); OFX, Staphylococcus-positive and treated with ofloxacin (3 mg/mL); CCFS, Staphylococcus-positive and treated with concentrated CFS derived from L. animalis SWLA-1 (20×, 232.96 ± 5.23 mg/mL); CCFS+OFX, Staphylococcus-positive and treated with a combination of concentrated CFS (20×, 232.96 ± 5.23 mg/mL) and ofloxacin (3 mg/mL). The mean of observed data in each experimental group is presented as the horizontal bold line in the figure. Significant differences are denoted by asterisks (***p < 0.001, ****p < 0.0001).
Comparing with the results of the corneal opacity scoring, different results were observed in the microbiological evaluation (Figure 4). The mean CFU values of the group treated with concentrated CFS derived from L. animalis SWLA-1 or a combination of concentrated CFS and ofloxacin were significantly lower than those of the Staphylococcus-positive control group (p < 0.05). Conversely, the mean CFU value of the ofloxacin-only treated group was significantly higher than that of the Staphylococcus-negative control group. Notably, the growth of Staph. pseudintermedius KUVM1701GC was significantly inhibited in the group treated with vancomycin or concentrated CFS compared with that in the other groups, based on the mean CFU differences (p < 0.05). Regarding the result of this experiment, the mean CFU values were the only quantitative result showing significant difference between Staphylococcus-positive group and other groups, because the Scheirer–Ray–Hare test results showed no significant interaction between categorical (experimental groups, corneal score) and quantitative (CFU result) variables (p > 0.05).
Figure 4. Differences in the mean CFU values between experimental groups. The values of all groups were analyzed using the Kruskal–Wallis test, followed by Dunn’s test for post-hoc testing. The experimental groups are as follows: Neg, Staphylococcus-negative and inoculated with sterile PBS only; Pos, Staphylococcus-positive and treated with PBS; VA, Staphylococcus-positive and treated with vancomycin (20 μg/mL); OFX, Staphylococcus-positive and treated with ofloxacin (3 mg/mL); CCFS, Staphylococcus-positive and treated with concentrated CFS derived from L. animalis SWLA-1 (20×, 232.96 ± 5.23 mg/mL); CCFS+OFX, Staphylococcus-positive and treated with combination of concentrated CFS (20×, 232.96 ± 5.23 mg/mL) and ofloxacin (3 mg/mL). The outliers are represented by bullets, and the mean of observed data in each experimental group is represented by the horizontal bold line in the figure. Significant differences are denoted by asterisks (*p < 0.05, **p < 0.01, ****p < 0.0001).
The CCK-8 assay demonstrated a mild cytotoxic effect in human keratocytes when exposed to CFS derived from L. animalis SWLA-1 compared with that in the mock-treated group. However, after a 2-fold dilution, no significant cytotoxic effects on the viability of human keratocytes were observed (Figure 5). This demonstrated that the 2-fold dilution of CFS did not adversely affect cell viability.
Figure 5. Confirmation of cell viabilities of human keratocytes using the CCK-8 assay. Results were obtained 72 h post-treatment with CFS derived from L. animalis SWLA-1, as compared to mock-treated cells at the indicated dilutions. Data are presented as mean ± standard deviation for three independent experiments. Data were analyzed by one-way analysis of variance (n.s: non-significant, ***p<0.001).
Ulcerative keratitis is one of the most common ocular diseases affecting dogs. Owing to various factors that interact with and adversely affect the ocular surface, the corneal epithelium can become disrupted, leading to exposure of the underlying corneal stroma. When the anatomical barrier of the cornea is compromised or disrupted, it can lead to the invasion of pathogenic bacteria through this weakened gap. A secondary bacterial infection in the corneal stroma can destroy the organized corneal structure, potentially causing vision impairment and necessitating the removal of the whole eye globe in severe cases. Cytological examination, microbiological culture of corneal samples, and antibiotic susceptibility tests are recommended for the diagnosis and treatment of bacterial keratitis (1).
Staphylococcus pseudintermedius is the most common opportunistic pathogen responsible for causing infectious keratitis in regions such as America (7, 29, 30), Europe (31), and Asia (10, 32). It has been reported that Staphylococcus pseudintermedius can cause the destruction of corneal tissue by expressing an analogue of protein A, which is produced by Staph. aureus and plays a major role in staphylococcal keratitis (33, 34). The destruction and lysis of corneal structures induced by bacterial infection increase corneal opacity with the presence of opaque or cloudy area on the cornea (35). The change in corneal opacity and the measurement of bacterial growth using CFU was determined in this study.
The emergence of antimicrobial resistance poses a significant challenge in veterinary ophthalmology (36). In a recent study on canine bacterial keratitis, nearly half of the isolates from clinical Staphylococcus spp. infections with corneal stromal ulcers were identified as MRSP. In the same study, one of the isolates from an MRSP-infected patient exhibited multi-drug resistance to antibiotics and presented highly aggressive clinical symptoms (30). Although MDRSP-induced bacterial keratitis is an emerging serious challenge in veterinary medicine, there have been limited studies utilizing corneal infection models of MDRSP. To the best of our knowledge, this is the first study to develop a corneal infection model using MDRSP.
In the present study, a novel ex vivo corneal infection model was established to evaluate the antimicrobial activity of CFS derived from L. animalis SWLA-1. This model can suggest an alternative approach to evaluate the inhibitory effect of antimicrobial substances CFS derived from probiotic bacteria against MDRSP under simulated conditions similar to bacterial keratitis in live animals. Additionally, owing to the absence of a host immune system in this ex vivo model, we successfully evaluated the intrinsic antibacterial properties of CFS derived from L. animalis SWLA-1 against the MDRSP KUVM1701GC strain. Furthermore, ex vivo corneal infection models are easier to establish and allow for the visible progression of lesions within less than 24 h compared to in vivo infection models (37).
We evaluated corneal opacity by scoring corneal haziness 48 h after infection in this study. Clinical features of canine bacterial keratitis include corneal opacity (1, 38). Our results showed no significant correlation between corneal opacity and CFU value among the groups. For instance, the experimental group treated with ofloxacin against indicator bacteria showed significantly lower corneal opacity score compared to the group infected with indicator bacteria and treated with PBS only. However, no significant difference was observed between these two groups based on the result of measuring viable bacterial cell counts. In contrast, the group treated with concentrated CFS showed significantly increased corneal opacity score compared to that of Staphylococcus-negative group. Interestingly, this group was the only one that significantly inhibited the growth of indicator bacteria as well as the group treated with vancomycin, which have no change in corneal opacity and significantly inhibitory effect against indicator bacteria based on viable cell counts. These findings suggest that the clinical assessment of corneal inflammation progression may differ from the actual degree of microbial infection. Further studies should include histopathological analysis of an in vivo model of MDRSP infection.
Obviously, in vivo corneal models offer an excellent platform for investigating host immune defense, inflammation, and corneal healing mechanisms. Nevertheless, these models are unsuitable for examining the initial stages of infection because of the challenge of infecting healthy, intact corneas. Moreover, the process of infection initiation and progression spans several days and lacks certainty (39). Furthermore, the practical use of in vivo studies is hindered by the high cost associated with animal purchase and maintenance costs, as well as ethical concerns regarding the use of dogs as experimental animals.
Recently, there has been considerable interest in utilizing ex vivo corneal models to study keratitis. Ex vivo tissue models that closely mimic both the biochemical and biophysical aspects are more valuable in terms of efficiency and cost-effectiveness. Various techniques have been reported in the literature to induce bacterial infections in ex vivo corneal models, including the use of infected contact lenses (40), corneal scarification (41), and intrastromal injection (37). In this study, we used a rotating epithelial brush to remove the corneal epithelium and establish compromised anatomic defenses (42). This device has been developed for precise corneal epithelium removal during refractive surgery in human medicine, including photorefractive keratectomy and laser-assisted subepithelial keratectomy. Its application ensures the maintenance of consistent size and depth of the corneal defects. Since the ex vivo corneal model can also be used to investigate drug delivery systems related to eye infections (25), it can be used in the evaluation of other antimicrobial compounds in further studies.
Probiotics and their antimicrobial substances have been successfully used to prevent and treat various bacterial infections in both humans and animals (43–46). Recently, effective antimicrobial compounds or metabolites derived from probiotic bacteria have been investigated as alternatives to classical antibiotics for treating MDR bacterial infections. These antimicrobial compounds, also known as postbiotics or pharmaceuticals, can contribute to the preservation of classic antibiotics and the treatment of MDR pathogenic bacteria (47). Additionally, antimicrobial compounds derived from probiotics, such as bacteriocins, have significant advantages over classical antibiotics, including reducing the likelihood of inducing antibiotic resistance in bacteria and the relative ease of modification and bio-engineering owing to their molecular size and structures (21, 48).
Based on the results of the microbiological evaluation, the concentrated CFS derived from L. animalis SWLA-1 significantly inhibited the growth of MDRSP, comparable to the group treated with vancomycin (p < 0.05). As this compound exhibits enhanced antimicrobial activity against pathogens when concentrated using TCA protein precipitation, it appears to function in a concentration-dependent manner, similarly to bacteriocins (49, 50). Further studies should involve the identification and isolation of the active antimicrobial compounds in this CFS through complete genome analysis of L. animalis SWLA-1 or peptides and chemical analysis using mass spectrometry.
According to these results, the antimicrobial activity of concentrated CFS derived from L. animalis SWLA-1 effectively inhibited the growth of MDRSP in an ex vivo corneal infection model. Considering that antimicrobial compounds derived from probiotics have a lower propensity to develop antibiotic resistance in pathogens and are generally recognized as safe for humans and animals, this compound has potential as an alternative to topical antibiotic agents for treating bacterial keratitis.
Although our ex vivo infection model proves useful for assessing efficacy, it has limitations. The primary objective of this study was to establish an MDRSP ex vivo corneal infection model and assess the efficacy of concentrated CFS derived from L. animalis SWLA-1, serving as a preliminary bridge experiment before advancing to an in vivo infection model. In contrast to the in vivo model, the ex vivo model in this study allowed for the evaluation of experimental outcomes with clear macroscopic differences within a short time frame. However, for future experiments utilizing the in vivo model, aspects not addressed in this study, such as the interaction between the complete immune system including tear films and bacterial infection over an extended period, will be studied. This will involve a more in-depth investigation through histopathological studies.
Another limitation of this study is the utilization of the SPOTS system’s corneal opacity scoring method in an ex vivo environment. SPOTS system was developed to scoring lesions in vivo clinically. To better replicate clinical in vivo infection conditions, we customized an “air-liquid” culture environment with a rocking platform that stimulates the blinking of the eye. Also, a serum-containing medium were used to supply growth factors and nutrients that replicate in vivo conditions following methodology used in previous studies (51, 52). Therefore, considering the ex vivo culture setting in this study closely resembles the in vivo environment, and with the additional factor of a relatively short culture time of 48 h, we determined that the SPOTS system is suitable for measuring corneal opacity induced by bacterial infection-induced corneal ulceration, which was the focus of our experiment. In future studies, a thorough analysis using anterior segment optical coherence tomography and histopathological studies will be necessary.
This is the first study to describe the establishment of an ex vivo MDRSP infection corneal culture model using canine corneas. Furthermore, we confirmed the efficacy of the concentrated CFS derived from L. animalis SWLA-1 as a potential alternative antibiotic agent against MDRSP. In future studies, the ex vivo corneal infection model can emerge as a robust platform for the comprehensive evaluation of diverse infectious microorganisms and topical therapeutic interventions applicable to both canine and human ulcerative keratitis.
The original contributions presented in the study are included in the article/Supplementary material, further inquiries can be directed to the corresponding author.
The requirement of ethical approval was waived by Institutinal Animal Care and Use Committee (IACUC) of Konkuk university for the studies involving animals because the tissues were acquired from postmortem conditions; No involvement of animal suffering or manipulation. The studies were conducted in accordance with the local legislation and institutional requirements. Written informed consent was obtained from the owners for the participation of their animals in this study.
J-HJ: Conceptualization, Investigation, Writing – original draft. H-JL: Formal analysis, Investigation, Writing – original draft. D-HK: Investigation, Writing – original draft. S-WL: Project administration, Writing – review & editing. J-YK: Funding acquisition, Project administration, Supervision, Writing – review & editing.
The author(s) declare financial support was received for the research, authorship, and/or publication of this article. This work was supported by Konkuk University in 2023 (Grant number 2023-A019-0041).
The authors declare that the research was conducted in the absence of any commercial or financial relationships that could be construed as a potential conflict of interest.
All claims expressed in this article are solely those of the authors and do not necessarily represent those of their affiliated organizations, or those of the publisher, the editors and the reviewers. Any product that may be evaluated in this article, or claim that may be made by its manufacturer, is not guaranteed or endorsed by the publisher.
The Supplementary material for this article can be found online at: https://www.frontiersin.org/articles/10.3389/fvets.2024.1346313/full#supplementary-material
1. Ledbetter, E, and Gilger, BC. Diseases and surgery of the canine cornea and sclera. Oxford: Wiley-Blackwell (2013).
2. McKeever, JM, Ward, DA, and Hendrix, DV. Comparison of antimicrobial resistance patterns in dogs with bacterial keratitis presented to a veterinary teaching hospital over two multi-year time periods (1993–2003 and 2013–2019) in the southeastern United States. Vet Ophthalmol. (2021) 24:653–8. doi: 10.1111/vop.12897
3. Hindley, KE, Groth, AD, King, M, Graham, K, and Billson, FM. Bacterial isolates, antimicrobial susceptibility, and clinical characteristics of bacterial keratitis in dogs presenting to referral practice in Australia. Vet Ophthalmol. (2016) 19:418–26. doi: 10.1111/vop.12325
4. Wang, L, Pan, Q, Zhang, L, Xue, Q, Cui, J, and Qi, C. Investigation of bacterial microorganisms in the conjunctival sac of clinically normal dogs and dogs with ulcerative keratitis in Beijing, China. Vet Ophthalmol. (2008) 11:145–9. doi: 10.1111/j.1463-5224.2008.00579.x
5. Jinks, MR, Miller, EJ, Diaz-Campos, D, Mollenkopf, DF, Newbold, G, Gemensky-Metzler, A, et al. Using minimum inhibitory concentration values of common topical antibiotics to investigate emerging antibiotic resistance: a retrospective study of 134 dogs and 20 horses with ulcerative keratitis. Vet Ophthalmol. (2020) 23:806–13. doi: 10.1111/vop.12801
6. LoPinto, AJ, Mohammed, HO, and Ledbetter, EC. Prevalence and risk factors for isolation of methicillin-resistant Staphylococcus in dogs with keratitis. Vet Ophthalmol. (2015) 18:297–303. doi: 10.1111/vop.12200
7. Hewitt, JS, Allbaugh, RA, Kenne, DE, and Sebbag, L. Prevalence and antibiotic susceptibility of bacterial isolates from dogs with ulcerative keratitis in Midwestern United States. Front Vet Sci. (2020) 7:583965. doi: 10.3389/fvets.2020.583965
8. Aslam, B, Wang, W, Arshad, MI, Khurshid, M, Muzammil, S, Rasool, MH, et al. Antibiotic resistance: a rundown of a global crisis. Infect Drug Resist. (2018) 11:1645–58. doi: 10.2147/IDR.S173867
9. Lord, J, Millis, N, Jones, RD, Johnson, B, Kania, SA, and Odoi, A. Patterns of antimicrobial, multidrug and methicillin resistance among Staphylococcus spp. isolated from canine specimens submitted to a diagnostic laboratory in Tennessee, USA: a descriptive study. BMC Vet Res. (2022) 18:91. doi: 10.1186/s12917-022-03185-9
10. Ekapopphan, D, Srisutthakarn, A, Moonarmart, W, Buddhirongawatr, R, and Bangphoomi, N. Identification and antimicrobial susceptibility of microorganisms isolated from severe corneal ulcers of dogs in Thailand. J Vet Med Sci. (2018) 80:1259–65. doi: 10.1292/jvms.18-0045
11. Viegas, FM, Santana, JA, Silva, BA, Xavier, RGC, Bonisson, CT, Câmara, JLS, et al. Occurrence and characterization of methicillin-resistant Staphylococcus spp. in diseased dogs in Brazil. PLoS One. (2022) 17:e0269422. doi: 10.1371/journal.pone.0269422
12. Proietto, LR, Whitley, RD, Brooks, DE, Schultz, GE, Gibson, DJ, Berkowski, WM Jr, et al. Development and assessment of a novel canine ex vivo corneal model. Curr Eye Res. (2017) 42:813–21. doi: 10.1080/02713683.2016.1262428
13. Kang, M-H, Chae, M-J, Yoon, J-W, Lee, S-Y, Yoo, J-H, and Park, H-M. Resistance to fluoroquinolones and methicillin in ophthalmic isolates of Staphylococcus pseudintermedius from companion animals. Can Vet J. (2014) 55:678–82.
14. Wang, Z, Guo, L, Li, J, Li, J, Cui, L, Dong, J, et al. Antibiotic resistance, biofilm formation, and virulence factors of isolates of staphylococcus pseudintermedius from healthy dogs and dogs with keratitis. Front Vet Sci. (2022) 9:903633. doi: 10.3389/fvets.2022.903633
15. FAO, UNEP WHO, and WOAH. One health joint plan of action (2022–2026). Working together for the health of humans, animals, plants and the environment. Rome: WHO (2022).
16. World Organization for Animal Health. OIE: OIE list of antimicrobial agents of veterinary importance. (2015). Available at:. (https://www.woah.org/app/uploads/2021/03/oie-list-antimicrobials.pdf)
17. World Health Organization. Critically important antimicrobials for human medicine, 6th revision. (2019). Available at:. (https://www.who.int/publications/i/item/9789241515528)
18. Chen, B, Li, F, Zhu, XK, Xie, W, Hu, X, Zan, MH, et al. Highly biocompatible and recyclable biomimetic nanoparticles for antibiotic-resistant bacteria infection. Biomater Sci. (2021) 9:826–34. doi: 10.1039/D0BM01397H
19. Kortright, KE, Chan, BK, Koff, JL, and Turner, PE. Phage therapy: a renewed approach to combat antibiotic-resistant bacteria. Cell Host Microbe. (2019) 25:219–32. doi: 10.1016/j.chom.2019.01.014
20. Soltani, S, Hammami, R, Cotter, PD, Rebuffat, S, Said, LB, Gaudreau, H, et al. Bacteriocins as a new generation of antimicrobials: toxicity aspects and regulations. FEMS Microbiol Rev. (2021) 45:39. doi: 10.1093/femsre/fuaa039
21. Hols, P, Ledesma-García, L, Gabant, P, and Mignolet, J. Mobilization of microbiota commensals and their bacteriocins for therapeutics. Trends Microbiol. (2019) 27:690–702. doi: 10.1016/j.tim.2019.03.007
22. Perez, RH, Zendo, T, and Sonomoto, K. Novel bacteriocins from lactic acid bacteria (LAB): various structures and applications. Microb Cell Factories. (2014) 13:1–13. doi: 10.1186/1475-2859-13-S1-S3
23. Zhou, Q, Gu, R, Li, P, Lu, Y, Chen, L, and Gu, Q. Anti-Salmonella mode of action of natural L-phenyl lactic acid purified from Lactobacillus plantarum ZJ316. Appl Microbiol Biotechnol. (2020) 104:5283–92. doi: 10.1007/s00253-020-10503-4
24. Lee, H-J, Lee, J-B, Park, S-Y, Choi, I-S, and Lee, S-W. Antimicrobial activity of Ligilactobacillus animalis SWLA-1 and its cell-free supernatant against multidrug-resistant Bacteria and its potential use as an alternative to antimicrobial agents. Microorganisms. (2023) 11:182. doi: 10.3390/microorganisms11010182
25. Ubani-Ukoma, U, Chauhan, A, Schultz, G, and Gibson, DJ. An ex vivo cornea infection model. MethodsX. (2020) 7:100876. doi: 10.1016/j.mex.2020.100876
26. Eaton, JS, Miller, PE, Bentley, E, Thomasy, SM, and Murphy, CJ. The SPOTS system: an ocular scoring system optimized for use in modern preclinical drug development and toxicology. J Ocul Pharmacol Ther. (2017) 33:718–34. doi: 10.1089/jop.2017.0108
27. Kassambara, A. rstatix package, version 0.6.0; R Foundation for Statistical Computing. (2020). Vienna, Austria.
28. Kassambara, A. rcompanion package, version 2.4.3; Rutgers Cooperative Extension. (2023). New Brunswick, New Jersey, USA.
29. Prado, MR, Rocha, MF, Brito, ÉH, Girão, MD, Monteiro, AJ, Teixeira, MF, et al. Survey of bacterial microorganisms in the conjunctival sac of clinically normal dogs and dogs with ulcerative keratitis in Fortaleza, Ceará. Vet Ophthalmol. (2005) 8:33–7. doi: 10.1111/j.1463-5224.2005.04061.x
30. Joksimovic, M, Ford, BA, Lazic, T, Soldatovic, I, Luzetsky, S, and Grozdanic, S. Antibiotic recommendations for treatment of canine stromal corneal ulcers. Vet Sci. (2023) 10:66. doi: 10.3390/vetsci10020066
31. Suter, A, Voelter, K, Hartnack, S, Spiess, BM, and Pot, SA. Septic keratitis in dogs, cats, and horses in Switzerland: associated bacteria and antibiotic susceptibility. Vet Ophthalmol. (2018) 21:66–75. doi: 10.1111/vop.12480
32. Lin, CT, and Petersen-Jones, S. Antibiotic susceptibility of bacterial isolates from corneal ulcers of dogs in Taiwan. J Small Anim Pract. (2007) 48:271–4. doi: 10.1111/j.1748-5827.2007.00348.x
33. Lakhundi, S, Siddiqui, R, and Khan, NA. Pathogenesis of microbial keratitis. Microb Pathog. (2017) 104:97–109. doi: 10.1016/j.micpath.2016.12.013
34. Balachandran, M, Bemis, DA, and Kania, SA. Expression and function of protein a in Staphylococcus pseudintermedius. Virulence. (2018) 9:390–401. doi: 10.1080/21505594.2017.1403710
35. Jadi, PK, Sharma, P, Bhogapurapu, B, and Roy, S. Alternative therapeutic interventions: antimicrobial peptides and small molecules to treat microbial keratitis. Front Chem. (2021) 9:694998. doi: 10.3389/fchem.2021.694998
36. Soimala, T, Lübke-Becker, A, Hanke, D, Eichhorn, I, Feßler, AT, Schwarz, S, et al. Molecular and phenotypic characterization of methicillin-resistant Staphylococcus pseudintermedius from ocular surfaces of dogs and cats suffering from ophthalmological diseases. Vet Microbiol. (2020) 244:108687. doi: 10.1016/j.vetmic.2020.108687
37. Pinnock, A, Shivshetty, N, Roy, S, Rimmer, S, Douglas, I, Mac Neil, S, et al. Ex vivo rabbit and human corneas as models for bacterial and fungal keratitis. Graefes Arch Clin Exp Ophthalmol. (2017) 255:333–42. doi: 10.1007/s00417-016-3546-0
38. Meekins, JM, Rankin, AJ, and Samuelson, DA. Ophthalmic anatomy. Vet Ophthalmol. (2021) 1:41–123.
39. Mun, JJ, Tam, C, Kowbel, D, Hawgood, S, Barnett, MJ, Evans, DJ, et al. Clearance of Pseudomonas aeruginosa from a healthy ocular surface involves surfactant protein D and is compromised by bacterial elastase in a murine null-infection model. Infect Immun. (2009) 77:2392–8. doi: 10.1128/IAI.00173-09
40. Vermeltfoort, PB, van Kooten, TG, Bruinsma, GM, Hooymans, AM, van der Mei, HC, and Busscher, HJ. Bacterial transmission from contact lenses to porcine corneas: an ex vivo study. Invest Ophthalmol Vis Sci. (2005) 46:2042–6. doi: 10.1167/iovs.04-1401
41. Okurowska, K, Roy, S, Thokala, P, Partridge, L, Garg, P, Mac Neil, S, et al. Establishing a porcine ex vivo cornea model for studying drug treatments against bacterial keratitis. J Vis Exp. (2020) 159:e61156. doi: 10.3791/61156-v
42. Chung, HS, Moon, SH, Kang, S-S, Kim, M, Lee, H, Tchah, H, et al. Corneal epithelial removal with a newly designed epithelial brush. J Ophthalmol. (2021) 2021:4668056. doi: 10.1155/2021/4668056
43. Fayol-Messaoudi, D, Berger, CN, Coconnier-Polter, M-H, Lievin-Le Moal, V, and Servin, AL. pH-, lactic acid-, and non-lactic acid-dependent activities of probiotic lactobacilli against Salmonella enterica Serovar typhimurium. Appl Environ Microbiol. (2005) 71:6008–13. doi: 10.1128/AEM.71.10.6008-6013.2005
44. Tejero-Sariñena, S, Barlow, J, Costabile, A, Gibson, GR, and Rowland, I. In vitro evaluation of the antimicrobial activity of a range of probiotics against pathogens: evidence for the effects of organic acids. Anaerobe. (2012) 18:530–8. doi: 10.1016/j.anaerobe.2012.08.004
45. Fernández-Fernández, R, Lozano, C, Eguizábal, P, Ruiz-Ripa, L, Martínez-Álvarez, S, Abdullahi, IN, et al. Bacteriocin-like inhibitory substances in staphylococci of different origins and species with activity against relevant pathogens. Front Microbiol. (2022) 13:870510. doi: 10.3389/fmicb.2022.870510
46. Jiang, Y-H, Xin, W-G, Yang, L-Y, Ying, J-P, Zhao, Z-S, Lin, L-B, et al. A novel bacteriocin against Staphylococcus aureus from Lactobacillus paracasei isolated from Yunnan traditional fermented yogurt: purification, antibacterial characterization, and antibiofilm activity. J Dairy Sci. (2022) 105:2094–107. doi: 10.3168/jds.2021-21126
47. Hill, C. Probiotics and pharmabiotics: alternative medicine or an evidence-based alternative? Bioeng Bugs. (2010) 1:79–84. doi: 10.4161/bbug.1.2.10796
48. Hernández-González, JC, Martínez-Tapia, A, Lazcano-Hernández, G, García-Pérez, BE, and Castrejón-Jiménez, NS. Bacteriocins from lactic acid bacteria. A powerful alternative as antimicrobials, probiotics, and immunomodulators in veterinary medicine. Animals. (2021) 11:979. doi: 10.3390/ani11040979
49. Bruno, ME, and Montville, TJ. Common mechanistic action of bacteriocins from lactic acid bacteria. Appl Environ Microbiol. (1993) 59:3003–10. doi: 10.1128/aem.59.9.3003-3010.1993
50. Wang, Y, Haqmal, MA, Liang, YD, Muhammad, I, Zhao, XO, Elken, EM, et al. Antibacterial activity and cytotoxicity of a novel bacteriocin isolated from Pseudomonas sp. strain 166. Microb Biotechnol. (2022) 15:2337–50. doi: 10.1111/1751-7915.14096
51. Castro-Combs, J, Noguera, G, Cano, M, Yew, M, Gehlbach, PL, Palmer, J, et al. Corneal wound healing is modulated by topical application of amniotic fluid in an ex vivo organ culture model. Exp Eye Res. (2008) 87:56–63. doi: 10.1016/j.exer.2008.04.010
Keywords: bacterial keratitis, multi-drug-resistant staphylococci, ex vivo corneal infection, antimicrobial alternatives, corneal ulceration
Citation: Jang J-H, Lee H-J, Kim D-H, Lee S-W and Kim J-Y (2024) Antimicrobial activity of cell-free supernatant derived from Ligilactobacillus animalis SWLA-1 in a novel ex vivo canine corneal infection model. Front. Vet. Sci. 11:1346313. doi: 10.3389/fvets.2024.1346313
Received: 29 November 2023; Accepted: 04 April 2024;
Published: 23 April 2024.
Edited by:
Piera Anna Martino, University of Milan, ItalyReviewed by:
Cristin Coman, National Institute of Medical-Military Research and Development, RomaniaCopyright © 2024 Jang, Lee, Kim, Lee and Kim. This is an open-access article distributed under the terms of the Creative Commons Attribution License (CC BY). The use, distribution or reproduction in other forums is permitted, provided the original author(s) and the copyright owner(s) are credited and that the original publication in this journal is cited, in accordance with accepted academic practice. No use, distribution or reproduction is permitted which does not comply with these terms.
*Correspondence: Joon-Young Kim, Y2FudmV0QGtvbmt1ay5hYy5rcg==; Y2FudmV0QGhhbmFmb3MuY29t
†These authors have contributed equally to this work
Disclaimer: All claims expressed in this article are solely those of the authors and do not necessarily represent those of their affiliated organizations, or those of the publisher, the editors and the reviewers. Any product that may be evaluated in this article or claim that may be made by its manufacturer is not guaranteed or endorsed by the publisher.
Research integrity at Frontiers
Learn more about the work of our research integrity team to safeguard the quality of each article we publish.