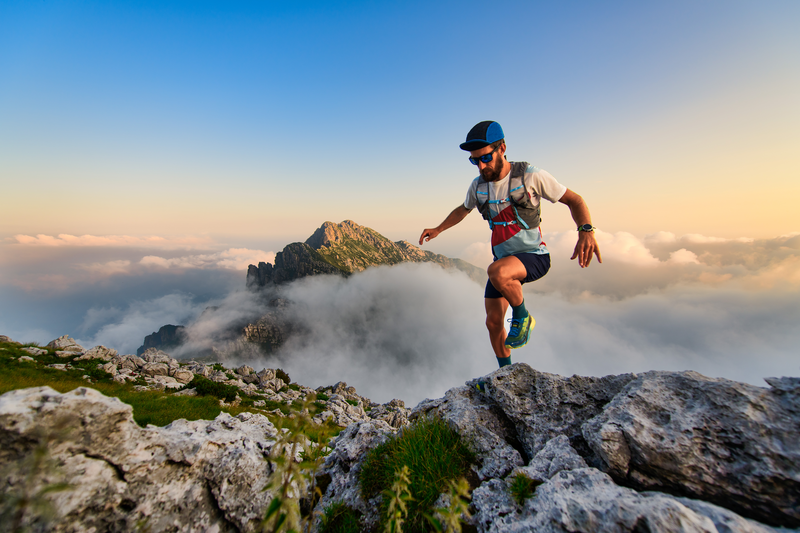
94% of researchers rate our articles as excellent or good
Learn more about the work of our research integrity team to safeguard the quality of each article we publish.
Find out more
ORIGINAL RESEARCH article
Front. Vet. Sci. , 26 March 2024
Sec. Animal Nutrition and Metabolism
Volume 11 - 2024 | https://doi.org/10.3389/fvets.2024.1342310
This article is part of the Research Topic Alternative and Novel Livestock Feed: Reducing Environmental Impact View all 19 articles
The impact of 15% dietary inclusion of Spirulina (Arthrospira platensis) in broiler chickens was explored, focusing on blood cellular components, systemic metabolites and hepatic lipid and mineral composition. From days 14 to 35 of age, 120 broiler chickens were divided and allocated into four dietary treatments: a standard corn and soybean meal-based diet (control), a 15% Spirulina diet, a 15% extruded Spirulina diet, and a 15% Spirulina diet super-dosed with an enzyme blend (0.20% porcine pancreatin plus 0.01% lysozyme). The haematological analysis revealed no significant deviations (p > 0.05) in blood cell counts across treatments, suggesting that high Spirulina inclusion maintains haematological balance. The systemic metabolic assessment indicated an enhanced antioxidant capacity in birds on Spirulina diets (p < 0.001), pointing toward a potential reduction in oxidative stress. However, the study noted a detrimental impact on growth performance metrics, such as final body weight and feed conversion ratio (both p < 0.001), in the Spirulina-fed treatments, with the super-dosed enzyme blend supplementation failing to alleviate these effects but with extrusion mitigating them. Regarding hepatic composition, birds on extruded Spirulina and enzyme-supplemented diets showed a notable increase in n-3 fatty acids (EPA, DPA, DHA) (p < 0.001), leading to an improved n-6/n-3 PUFA ratio (p < 0.001). Despite this positive shift, a reduction in total hepatic lipids (p = 0.003) was observed without a significant change in cholesterol levels. Our findings underscore the need for further exploration into the optimal inclusion levels, processing methods and potential enzymatic enhancements of Spirulina in broiler diets. Ultimately, this research aims to strike a balance between promoting health benefits and maintaining optimal growth performance in poultry nutrition.
The increasing global quest for sustainable and eco-friendly protein sources, especially in poultry farming, has driven the exploration toward unconventional feed components. Among these, microalgae, particularly those from the Spirulina genus, have emerged as noteworthy contenders (1–3). Arthrospira platensis, ubiquitously known as Spirulina, is a cyanobacterium heralded for its superior protein content, carbohydrates, essential fatty acids, vitamins, pigments and diverse mineral profile. However, these contents are subject to modulation based on cultivation conditions and seasonal variations (4, 5).
Apart from its robust nutritional portfolio, Spirulina exhibits a range of functional benefits including antimicrobial, antioxidant, anti-inflammatory and immunomodulatory properties (5–7). However, the alga’s predominantly indigestible cell wall, resembles the Gram-negative bacteria with a composition mostly of peptidoglycan and lipopolysaccharides, presenting digestibility challenges to monogastric animals (8). Moreover, the bioaccessibility of algal proteins can be thwarted due to the protein-pigment complexes, such as phycocyanins interfaced with thylakoid membranes (9).
Although carbohydrases, like lysozyme, have shown promise in partially dismantling the Spirulina cell wall (10), a gelation process appears to ensnare nutrients, impeding their digestion (3, 11). Preliminary attempts to hydrolyse Spirulina proteins through physical pre-treatments coupled with pancreatin or trypsin have been made (12, 13), but the realm of deploying such enzymes to degrade proteins in A. platensis feed for monogastric animals remains largely unexplored. However, emerging studies accentuate the potential efficacy of specific exogenous enzymes, such as carbohydrate-active enzymes, in breaching the Spirulina cell wall and amplifying the bioavailability of its nutritional benefits (14). The incorporation of these exogenous enzymes as feed additives in poultry diets is a well-trodden strategy to mitigate the paucity of endogenous enzymes and neutralize anti-nutritional factors, enhancing the digestibility of dietary constituents (15, 16). Furthermore, these enzymes foster the generation of prebiotic oligosaccharides, thus buoying gut health and growth parameters (17).
Traditionally, microalgae have been utilized more as supplements than core ingredients in monogastric diets (18). Preceding inquiries from this team evaluated the ramifications of integrating microalgae as a feed ingredient on growth performance, health status, and hepatic lipid composition in broilers (19, 20), and extended to pigs (19, 20). This investigation pioneers the examination of the synergistic effects of prolonged feeding with a high level of A. platensis, either alone or supplemented with different enzymes (predominantly peptidases, EC 3.4), on the health and hepatic lipid metabolism in broilers. While the positive impacts of dietary Spirulina supplementation on broiler performance and health have been tentatively probed [e.g., Pestana et al. (3)], a realm unexplored persists concerning the interactive effects of super-dosing multienzymes in conjunction with higher Spirulina inclusion levels. Indeed, previous reports showed the potential of super-dosed (higher than recommended) multi-enzymes to improve growth performance, gut health, and nutrient absorption in poultry (21–23). The application of extruded Spirulina in one of the dietary treatments seems to be a promising combination, as shown in in vitro studies, to enzymatic pre-treatments (e.g., porcine pancreatin), as the high pressure and temperature may cause a disruption in microalga’s cell wall, contributing to improving the bioavailability of relevant proteins, such as phycocyanin (12, 13).
The purpose of this work was to assess the impact of incorporating 15% A. platensis biomass in broiler diets, either exclusively, or in combination with a super-dosing enzyme blend (pancreatin and lysozyme), or when Spirulina is extruded, from day 14 to 35, on broilers’ blood cells, plasma metabolites, and hepatic composition, comprising fatty acids, antioxidant diterpenes, pigments and mineral profile.
The research enlisted a group of one hundred and twenty, one-day-old male Ross 308 broiler chicks acquired from Pinto Valouro (Bombarral, Portugal), each exhibiting an average initial weight of 39.3 ± 2.30 g. From day 1, they were randomly lodged in 40 wire-netted enclosures (56 cm height x 56 cm length x 50 cm width) for 35 days under regulated environmental conditions. Ambient temperature and airflow were persistently supervised as per the previously established protocols (2, 19). Adhering to the 3R’s doctrine (Reduce, Refine and Replace) to curtail the number of animals employed, every enclosure hosted three chicks, with 10 identical enclosures designated for each dietary experiment. The experimental trial was conducted in the School of Agriculture at the University of Lisbon (Lat: 38°42′27.5”N; Lng: 9°10′56.3”W). The Animal Welfare Committee of the School of Agriculture at the University of Lisbon (ORBEA/ISA) approved all the experimental methodologies involving animals, allocating the study a protocol code number 0421/000/000/2022.
In the initial fortnight, chicks were administered a staple diet formulated from corn and soybean meal sourced from Rações Veríssimo S. A. (Leiria, Portugal). Following this phase, from day 14 through day 35, they transitioned to one of four distinct experimental diets furnished ad libitum daily. These diets encompassed: (1) a standard diet predicated on corn and soybean meal (CTR); (2) a diet fortified with 15% Spirulina powder (procured from Allmicroalgae, Pataias, Portugal) (SP); (3) a diet containing 15% extruded Spirulina powder (SPE); and (4) a diet supplemented with 15% Spirulina powder alongside a tailored enzyme blend, constituted by 0.20% porcine pancreatin extract (sourced from Merck, Darmstadt, Germany) and 0.01% lysozyme extracted from chicken egg white (sourced from Sigma-Aldrich, Missouri, United States) (SPM). The microalga extrusion was performed by Sparos company (Olhão, Portugal), following detailed conditions: 340 mL of water addition per minute, at 34 bars and 118°C for the last extrusion barrel. This procedure occurred from 3 to 7 s. Then, the algal pellets were dried for 8 and 10 min at 120°C (12, 13). The encapsulated porcine pancreatin extract comprised 350 FIP-U/g of protease, 6,000 FIP-U/g of lipase, and 7,500 FIP-U/g of amylase. Lysozyme powder contained 70,000 U/mg protein. A detailed composition of the feed constituents and additives for each experimental diet is provided in Table 1. During the trial, a 2.5% mortality was observed (three animals of 120 in total).
Table 1. Ingredient composition of broiler experimental diets from day 14 to day 35 (%, as fed basis).
Weekly assessments were conducted to record the weights of broilers and feeders, with daily feed provisioning to compute the parameters of body weight gain (BWG) (ascertained by the weekly weight differential divided by 7), average daily feed intake (ADFI) (calculated as weekly consumption per cage, divided by 7) and the feed conversion ratio (FCR) (the quotient of weekly consumption divided by 3 and weekly body weight gain).
Table 2 shows the proximal composition of the microalga and the experimental diets offered to broilers from day 14 to 35. The assessment of dry matter, crude protein, crude fat, and ash content was conducted in adherence to the methodologies stipulated by AOAC (24). The quantification of gross energy content within both the microalga and the diets was executed employing an adiabatic bomb calorimetry (Parr 1,261, Parr Instrument Company, Moline, IL).
Table 2. Proximal and nutrient compositions of Arthrospira platensis and experimental diets offered to broilers from day 14 to 35.
The fatty acid composition in A. platensis and the experimental diets was done after a singular extraction and acidic methylation step, aligning with the method delineated by Sukhija and Palmquist (25). The resultant fatty acid methyl esters (FAME) were analyzed utilizing gas chromatography interfaced with a flame ionization detector (HP7890A Hewlett-Packard, Avondale, PA), deploying a Supelcowax® 10 capillary column (30 m × 0.20 mm i.d., 0.20 μm film thickness, Supelco, Bellefonte, PA, United States), by the protocol advanced by Alfaia et al. (26). Identification of FAME within the samples was achieved through juxtaposition with a standard FAME mixture (37 Component FAME mix C4-C24, Supelco, Bellefonte, PA, USA). The heneicosanoic acid (21.0) methyl ester was enlisted as the internal standard for the quantification of individual fatty acids, with the fatty acid content articulated as a percentage of the total fatty acids.
The diterpenes and β-carotene within the experimental diets were assessed by adhering to the methodology delineated by Prates et al. (27). Duplicated samples, each weighing 0.1 g, were fortified with ascorbic acid (vitamin C) and underwent a saponification reaction in a water bath maintained at 80°C for 15 min. Following extraction and centrifugation (2,500 rpm, 10 min), the n-hexane phases were scrutinized utilizing an HPLC system (Agilent 1,100 Series, Agilent Technologies Inc., Palo Alto, CA, United States) equipped with a normal-phase silica column (Zorbax RX-Sil, 5 μm particle size, 250 mm × 4.6 mm i.d.). β-carotene was discerned employing a UV–visible photodiode array detector (λ = 450 nm), while tocopherols and tocotrienols were identified with a fluorescence detector (excitation λ = 295 nm and emission λ = 325 nm). The quantification of β-carotene and analogs of vitamin E was facilitated through the utilization of standard curves correlating peak area to concentration.
The pigment profile of the experimental diets was ascertained with minor alterations to the protocols expounded by Hynstova et al. (28) and Pestana et al. (3). Concisely, 0.1 g of samples were amalgamated with 5 mL of acetone, and homogenized in the dark, followed by centrifugation (2,500 rpm, 10 min) the supernatant was isolated and analysed. Chlorophylls a and b were detected at wavelengths of 662 nm and 645 nm, respectively, and total carotenoids at 470 nm using UV–Vis spectrophotometry (Genesys 150, Thermo Scientific, Waltham, Massachusetts, United States). The pigment content was deduced employing equations provided by Hynstova et al. (28).
The mineral composition of A. platensis and experimental diets was deciphered by allocating 0.3 g of samples into a digestion tube, introducing nitric acid (65%) and hydrochloric acid (37%), and digesting at 95°C within a ventilated chamber for 16 h. Subsequently, hydrogen peroxide (30%) was added as outlined by Ribeiro et al. (29). The resultant solution was filtered and analysed employing Inductively Coupled Plasma - Optical Emission Spectrometry (ICP-OES, iCAP 7,200 duo Thermo Scientific, Waltham, MA, United States), with mineral quantification achieved through standards and calibration curves.
At the end of the trial, in a room with specific conditions for slaughtering, one 35-day-old broiler, medium weight, from each pen was selected, for slaughter, using electrical stunning followed by manual exsanguination. Blood samples were collected (10 mL), on the same day, directly into Sarstedt tubes (Numbrecht, Germany), and were subsequently centrifuged to obtain plasma. Liver samples were collected, minced, vacuum-sealed, and stored at −20°C for future analysis. The remaining birds were euthanized as described above.
As articulated by Madeira et al. (19), red blood cells, white blood cells, and thrombocytes were enumerated employing Sysmex XN-10 analysers (Sysmex Corporation, Kobe, Japan). The red blood cell tally was determined via the impedance variation method, after hydrodynamic focusing. Differential enumeration of white blood cells (%) was executed on blood smears, which were decolourized utilizing the May-Grünwald-Giemsa technique. Haemoglobin concentration was gauged photometrically, with sodium lauryl sulphate serving as the reagent.
Selected plasma metabolites, encompassing glucose, urea, creatinine, total protein and assorted lipid forms (triacylglycerols, TAG; total cholesterol; HDL-cholesterol; and LDL-cholesterol), in addition to enzymatic activities of alanine aminotransferase (ALT, EC 2.6.1.2), aspartate aminotransferase (AST, EC 2.6.1.1), alkaline phosphatase (ALP, EC 3.1.3.1) and γ-glutamyltransferase (GGT, EC 2.3.2.13) were ascertained employing diagnostic kits from Roche Diagnostics’ Modular Hitachi Analytical System (Mannheim, Germany). Formulas delineated by Friedewald et al. (30) and Covaci et al. (31) were applied for the computation of VLDL-cholesterol and total lipids, respectively. C-reactive protein levels were evaluated via immunoturbidimetry (Roche Diagnostics, Meylan, France). Primary electrolytes including Na+, K+ and Cl− were appraised through indirect potentiometry.
Total lipids were isolated from the lyophilized liver specimens, in duplicate, employing a solvent mixture of dichloromethane and methanol (2:1 v/v), and quantified via gravimetric analysis as per the protocol delineated by Folch et al. (32). The fatty acid composition within the liver specimens was deciphered akin to the methodology applied for A. platensis and the experimental diets. Nonetheless, a sequential process of alkaline followed by acidic transesterification was leveraged to convert the fatty acids into FAME, diverging from the sole acidic methylation procedure. The chromatographic conditions were congruent with the protocol formerly articulated by Alfaia et al. (26).
Duplicate liver specimens, each weighing 0.75 g, were employed for the extraction of total cholesterol, diterpenes and β-carotene, adhering to the identical procedure delineated for the experimental diets. This encompassed direct saponification, singular n-hexane extraction, and subsequent HPLC analysis as per the protocol detailed in Prates et al. (27).
For pigment extraction (encompassing chlorophyll-a, chlorophyll-b, and total carotenoids) from liver specimens, a slightly adapted version of the method deployed for the experimental diets was utilized. In brief, 1.5 g of liver tissue was amalgamated with 3 mL of acetone, followed by a 1-min homogenization in a darkened environment. Post-centrifugation (3,000 rpm for 5 min at 4°C), the supernatant was sequestered and examined under the conditions stipulated by Coelho et al. (33). The pigment quantities were ascertained utilizing the equations presented by Hynstova et al. (28).
The hepatic mineral profile was gauged following the established procedure for the microalga and diets, except for bromide, whose determination was conducted as per the method expounded by Delgado et al. (34).
The dataset was subjected to analysis as a completely randomized design employing the Generalized Linear Mixed (GLM) model within the framework of the Statistical Analysis System (SAS) software (SAS Institute Inc., Cary, NC) (35). The experimental diet was the single fixed factor and the primary effect within the statistical model. The experimental unit was either the enclosure or the animal depending on the parameters under evaluation. The enclosure was designated for assessing body weight, body weight gain, average daily feed intake, and feed conversion ratio, while the individual bird was the unit of analysis for the remaining parameters (plasma metabolites, and comprehensive hepatic parameters). The data was tested for the homogeneity of variances using Levene’s test, and for normality using the Shapiro–Wilk test. For multiple comparisons among least squares means, the adjusted Tukey–Kramer approach (PDIFF option) was invoked. All values were articulated as the mean along with the SEM (standard error of the mean). A threshold of p-values less than 0.05 was established for the determination of statistical significance.
To explore the interrelations between blood parameters and hepatic variables, a Principal Component Analysis (PCA) was conducted utilizing the SPSS Statistics for Windows software suite (IBM Corp., 2020 release, version 27.0, Armonk, NY, United States).
The investigation into the growth performance of broiler chickens under different treatments is presented in Figure 1 for contextual purposes. This assessment covered the period from days 14 to 35 of the chickens’ development. The findings showed that diets containing solely Spirulina (SP) and Spirulina combined with the enzyme mix (SPM) significantly reduced the final body weight (BW) of the birds, compared to the control diet. However, no significant differences in final BW were observed between the group fed extruded Spirulina (SPE) and the control group. A similar pattern emerged in the body weight gain (BWG) metrics, with the SPE diet showing results comparable to the control group, distinctly different from the SP and SPM diets. In terms of average daily feed intake (ADFI), an interesting increase was noted in the SPE group compared to the SP diet. Regarding the feed conversion ratio (FCR), all groups incorporating Spirulina led to an increase in FCR relative to the control, signifying a decrease in feed efficiency.
Figure 1. Impact of dietary treatments on broilers´ growth performance: (A) Final body weight (g); (B) Body weight gain (g/day); (C) Average daily feed intake (g); (D) Feed conversion ratio. CTR, corn and soybean meal-based diet; SP, 15% Spirulina; SPE, 15% extruded Spirulina; SPM, 15% Spirulina +0.21% enzyme mixture (0.20% porcine pancreatin +0.01% lysozyme). Values are shown as mean ± SEM (standard error of the mean). a,b,cDifferent superscripts within a row indicate a significant difference (p < 0.05).
Blood cell count and plasma metabolites from broilers fed 15% of A. platensis, either exclusively, or when Spirulina is extruded or combined with an enzyme blend (pancreatin and lysozyme) are displayed in Table 3. The counts for white blood cells (p = 0.702), granulocytes (p = 0.769), lymphocytes (p = 0.741), monocytes (p = 0.473), red blood cells (p = 0.377), thrombocytes (p = 0.708), and also haemoglobin (p = 0.062) were not affected by diets.
Concerning plasma metabolites, glucose levels reached the highest levels in broilers fed SPM, intermediate in SP or SPE, and lowest in broilers fed the control diet (p = 0.021). Urea was reduced in broilers fed SP and SPE relative to the control and SPM (p < 0.001). The renal function was further assessed by creatinine which displayed the highest values in broilers fed SPE and SPM (p < 0.001). Consistently, the SP diet increased lipid parameters which were reduced by the enzyme mixture supplementation for cholesterol (p < 0.001), LDL-cholesterol (p < 0.001), VLDL-cholesterol (p < 0.001), triacylglycerols (p < 0.001), and total lipids (p < 0.001). In terms of liver function, AST was reduced by Spirulina-based diets (p < 0.001) even if ALT was unchanged (p = 0.753) across dietary treatments. ALP (p < 0.001) and GGT (p = 0.002) were increased by the SP diet when compared to the other dietary treatments. Total proteins were kept unchanged across diets (p = 0.358). The SPE diet reduced the acute phase C-reactive protein about the other three dietary treatments (p = 0.002). In assessing the major electrolytes, sodium (p < 0.001) and chloride (p < 0.001) were reduced by the SPM diet relative to the other dietary treatments. Conversely, no significant changes were observed for potassium (p = 0.104).
The hepatic lipid profile and fatty acid composition from broilers fed 15% of A. platensis, either exclusively, or when Spirulina is extruded or combined with an enzyme blend (lysozyme and porcine pancreatin) are displayed in Table 4. A decline was observed in total lipids (p = 0.003) among broilers administered either Spirulina solely or in its extruded state, contrasting with a diminution in cholesterol levels within the control group (p < 0.001). Although the aggregate of saturated fatty acids (SFA) remained invariant across the dietary regimes (p > 0.05), certain specific fatty acids such as lauric acid (12:0) saw an elevation in levels among broilers subjected to the extruded Spirulina diet (p = 0.015), while palmitic acid (16.0) and arachidic acid (20.0) levels were augmented in scenarios where extruded Spirulina or Spirulina coupled with enzymes were administered (p = 0.009 and p = 0.005, respectively).
Table 4. Hepatic total lipids, total cholesterol and fatty acid composition of broilers fed experimental diets.
The monounsaturated fatty acid (MUFA) aggregation peaked in broilers fed Spirulina along with the enzyme concoction, exhibited intermediate values in those fed solely Spirulina or the control diet, and plummeted to the lowest in those administered extruded Spirulina (p = 0.047). This pattern was predominantly driven by fluctuations in the levels of predominant fatty acid oleic acid (18:1c9) (p = 0.031), and minor fatty acids palmitoleic acid (16:1 c9) and vaccenic acid (18:1c11) (p = 0.006). Conversely, an inversely proportional trend was depicted in the total polyunsaturated fatty acids (PUFA) content (p = 0.035), and specifically the n-6 PUFA subcategory (p = 0.018), attributable largely to the proportions of linoleic acid (18:2n-6) (p = 0.006) and eicosadienoic acid (20:2n-6) (p < 0.001).
Furthermore, an elevation of total n-3 PUFA was noted in broilers fed extruded Spirulina, followed by those supplemented with Spirulina and enzymes (p < 0.001), in comparison to the control group. This ascension culminated in a notable reduction in the n-6/n-3 ratio (p < 0.001), particularly pronounced in the SPE diet. All individual n-3 fatty acids recorded an uptick under the Spirulina-based diets (p < 0.001) relative to the control.
Table 5 shows the hepatic diterpene profile and pigment composition from broilers fed 15% of A. platensis, either exclusively, or when Spirulina is extruded or combined with an enzyme blend (lysozyme and porcine pancreatin). In examining the diterpene profile, β-carotene, a precursor of vitamin A, was discerned in the liver of broilers across all experimental diets, albeit with no significant changes among treatments. Conversely, a noteworthy reduction in α-tocopherol levels was observed in birds receiving Spirulina-supplemented diets (p < 0.001). This decrement was pronounced irrespective of whether Spirulina was extruded or complemented with the enzyme blend. Similarly, the aggregate levels of γ-tocopherol and β-tocotrienol exhibited a significant decrease in the Spirulina-fed treatments compared to the control (p < 0.001). α-Tocotrienol levels presented a marginal variance across treatments, nearing the threshold of significance (p = 0.052).
Regarding pigments, the data manifested a marked elevation in chlorophyll-a, total chlorophylls, total carotenoids, and the sum of total chlorophylls plus carotenoids in the liver of broilers under all Spirulina-inclusive diets (p < 0.001). These enhancements were consistently, unaffected by the extrusion of Spirulina or the supplementation of the enzyme blend. Remarkably, chlorophyll-b levels were doubled in birds fed solely on Spirulina in comparison to those on the control diet (p = 0.031).
The mineral profile in the liver of broilers fed 15% of A. platensis, either exclusively, or when Spirulina is extruded or combined with an enzyme blend (pancreatin and lysozyme) is displayed in Table 6. Among the observed minerals, manganese (Mn) emerged as a distinct element displaying a significant uptick in levels in broilers nourished with either extruded Spirulina or Spirulina supplemented with the enzyme concoction, as compared to the control group (p = 0.008).
Contrary to the Mn dynamics, the remainder of the micro and macrominerals, both on an individual basis and cumulatively, maintained a steady state across all experimental factions (p > 0.05). The macro-mineral cadre, encompassing calcium (Ca), magnesium (Mg), phosphorous (P), potassium (K), sodium (Na) and sulphur (S), alongside the micro-mineral consortium of copper (Cu), iron (Fe) and zinc (Zn), showcased remarkable stability.
The Principal Component Analysis (PCA) was performed using variables of broilers’ plasma metabolites and hepatic lipids showed distinct variability patterns across dietary treatments (Figure 2).
Figure 2. Principal component analysis of plasma metabolite profile (A) and hepatic biochemical composition (B) of dietary treatments. CTR, corn and soybean meal-based diet; SP, 15% Spirulina; SPE, 15% extruded Spirulina; SPM, 15% Spirulina +0.21% enzyme mixture (0.20% porcine pancreatin +0.01% lysozyme).
Figure 2A represents the dietary treatments distributed according to the variability of plasma metabolites´ data. The first two factors covered 47.0% of the total variance (31.5% for Factor 1, and 15.5% for Factor 2) leading to a clear separation of treatments. The SPM group was mostly represented in quadrant (a), the control in quadrant (c), and SP and SPE treatments in quadrants (b) and (d). In addition, Figure 2B plots the treatments based on hepatic chemical composition. The first two factors covered 39.4% of the total variance (22.9% for Factor 1, and 16.5% for Factor 2) forming two clusters. These corresponded to the control positioned in quadrants (a) and (c), and Spirulina-containing treatments located in quadrants (b) and (d). The variables of plasma metabolites with a higher discrimination power were total lipids (0.96), LDL-cholesterol (0.92), cholesterol (0.91), VLDL-cholesterol (0.83) and triacylglycerols (0.83), for Factor 1; and HDL-cholesterol (−0.76), AST (−0.59), VLDL-cholesterol (0.45), triacylglycerols (0.45), urea (0.43), K+ (0.38) and C-reactive protein (0.33), for Factor 2 (Table 7). The hepatic variables with the most discriminant power were α-tocopherol (−0.93), 20:5n-3 (0.83), total carotenoids (0.81), γ-tocopherol+ β-tocotrienol (−0.78), β-carotene (0.77), chlorophyll-a (0.77), 22:5n-3 (0.75) and 18:3n-3 (0.74) for Factor 1; and 18:1c9 (−0.94), 20:4n-6 (0.87), 16:1c9 (−0.75), 14:1c9 (−0.74) and 18:0 (0.71) for Factor 2.
Table 7. Loadings of the first two principal components of plasma metabolites and hepatic chemical composition.
The birds’ zootechnical data presented here resonates with the existing literature, attending that the performance of broilers is influenced by multiple factors encompassing the level of microalgae in the diet, the feeding period duration and the broilers’ efficacy in digesting microalgae’s cell walls. Park et al. (2) in a prior study illustrated that a modest dietary inclusion of A. platensis (ranging from 0.25 to 1.0%) over 35 days ameliorated feed efficiency in broiler chickens. Contrarily, our preceding investigation revealed a decline in final BW and BWG, along with an uptick in FCR, in birds fed 15% A. platensis diet, either alone or coupled with exogenous enzymes, over 21 days (3). The current endeavor, employing the identical 15% A. platensis dietary level, with or without commercial enzyme blends enriched with peptidases (VemoZyme P and porcine pancreatin), underscored a detrimental impact on broilers’ growth performance post 4 weeks of feeding, through diminished final BW, BWG and ADFI, coupled with augmented FCR. This trend was consistent throughout the experimental span, highlighting a notable impact of these diets on broiler growth performance. Nevertheless, the group fed with extruded Spirulina changed the impairment of growth performance associated with Spirulina-fed treatments leading to superior values for BW final and BWG (14 to 35d), which were equivalent to those found with the control diet. The extrusion process is not clearly understood but the disruption of microalga’s cell walls may, therefore, enhance bioavailability, with the release of important proteins, like phycocyanins. An improvement in bioavailability could facilitate more efficient hydrolysis by the broilers’ endogenous digestive enzymes, thus enhancing the absorption and consequent utilization of the nutrients, especially proteins, contributing to an improvement in broilers’ growth performance. Becker (36) propounded a cap of 10% microalgae inclusion in poultry diets to avert prospective adverse outcomes like elevated FCR over prolonged durations. The adverse bird performance with higher Spirulina levels could be ascribed to protein gelation, potentially impeding amino acid absorption and augmenting viscosity (11). Moreover, the robust protein content in Spirulina (50 to 70%) might exhibit resistance to the proteolytic action of birds’ endogenous peptidases (37). The attempt to mitigate this decline in animal growth performance via exogenous peptidase supplementation did not yield positive outcomes. A previous study in cockerels (21) also described an absence of effects on animal growth performance of a commercial super-dosed pancreatin (250 to 1,000 mg/kg) incorporated in a standard diet, although an improvement in gut morphology was detected. Conversely, studies in broilers showed a decrease in feed conversion ratio (22) and an increase in nutrient digestibility (23) when feeding similar levels (350 to 1,000 mg/kg) of a super-dosed enzyme blend added to the staple diet. However, none of the studies tested the effect of pancreatin on dietary microalgae. In the present study, the double amount of pancreatin (2000 mg/kg) was used in an attempt to maximize the hydrolysis of Spirulina compounds, but the impact of such enzyme blend on other microalgae suitable for broilers was not assessed, which would be interesting to pursue. Although A. platensis, being oligosaccharide-rich, is touted for its prebiotic potential which could modulate gut fermentation, this effect did not offset the adverse impact on body weight and ADG in this study; hence failing to prevent growth impairment (38). This casts light on the imperative for a more profound comprehension of dietary repercussions and nutrient utilization dynamics in broilers, to better harness the nutritional prowess of A. platensis while averting detrimental growth impacts.
A. platensis at a high level of dietary incorporation promoted no significant changes in blood cell counts, suggesting the maintenance of haematological homeostasis. This result is not aligned with Sugiharto et al. (39) findings which reported a significant reduction in haemoglobin values, erythrocytes and haematocrit in birds fed 1% of A. platensis during 35 days. Several studies reported an immune response balance induced by A. platensis (39–41). In a previous report by this research team (Lopes et al., unpublished data) using 15% of A. platensis in broiler chickens’ diet, we observed that while white blood cell count remained unchanged across diets, distinct variations in granulocyte and lymphocyte counts were depicted. The shift in monocyte count mirrored one of the lymphocytes. White blood cells are a part of the immune system protecting the body from infection. These cells circulate through the bloodstream and tissues to respond to injury or illness by attacking any unknown organisms that enter the body. Anomalies in their count point toward the onset of infectious or inflammatory diseases, leukaemia, lymphoma and bone marrow disorders (42). More recently, much attention has been directed to the immunomodulatory effects of Spirulina when applied as a poultry feed additive highlighting its potential to increase disease resistance and improve growth rates, particularly under stress conditions (40).
The animal’s clinical state and physical condition can be easily perceived by systemic biochemical parameters (43). In this study, we observed that the inclusion of dietary A. platensis at a high-level increased glucose levels in birds fed the enzyme mixture relative to the reference diet. This finding is most probably associated with the carbohydrate-rich composition of A. platensis (44, 45). Interestingly, while creatinine levels were enhanced by Spirulina extruded or combined with enzymes, a significant reduction in urea was observed in birds fed A. platensis, individual or extruded relative to the other treatments. This may suggest that A. platensis did not jeopardize renal function. In addition, reduced urea levels in birds fed the microalga could be a consequence of its lower protein digestibility. Often, common electrolytes such as sodium and potassium are associated with renal failure disorders (46). The kidney plays a pivotal role in maintaining the body’s chloride balance and transport, which is closely associated with sodium transport (47). Even though statistically significant, the variations observed in plasmatic sodium and chloride values, which were consistently reduced by A. platensis in combination with an enzyme mixture, are not relevant from a physiological point of view. In line with these findings, the element potassium did not vary among dietary treatments. Regarding the assessment of the hepatic function except for ALT, which displayed no changes across experimental treatments, the variations observed for AST, ALP and GGT enzymatic activities remained within reference figures for birds (33, 48, 49). This fact allows us to conclude that liver function is unaltered even if small changes were detected for certain aminotransferases. As so, by combining hepatic and renal data, incorporating A. platensis into the diets did not impose any toxicological hazard to birds underlining this microalga potential as a dietary ingredient for broiler livestock production.
The plasma lipid profile was largely influenced by diets. Our study does not support the cholesterol and lipid-lowering properties of A. platensis when provided individually, as previously reported for other microalgae species (50). A consistent pattern of increased total cholesterol, LDL-cholesterol, VLDL-cholesterol, triacylglycerols and total lipids was found when Spirulina was administered as an individual supplement. To the best of our knowledge, this lipemic boost is due to Spirulina addition and may be accountable to enhanced fat absorption in the intestinal tract (50). Notwithstanding, for most of these lipid parameters a counterbalanced hypolipidemic effect was detected for the combination of pancreatin with lysozyme reaching levels near to those observed in control birds. Total proteins in plasma were kept unchanged across experimental diets, even if an increase was expected in broilers fed A. platensis diets. Spirulina is a rich source of protein with protein levels varying from 50 to 70% (37), turning this microalga into a putative alternative for conventional protein sources, such as the traditional soybean meal (51–53). Moreover, the statistically significant differences observed for the acute phase protein, C-reactive protein, are devoid of physiological meaning because the average values found among dietary treatments were similar and followed by a residual standard error. The impacts of applying dietary A. platensis at a level of incorporation above 10%, as the 15% used herein, are scarce. The origin of discrepancies found between our study and the literature are determinant key factors, including dosage and source of the microalga, the experimental trial extension and specific conditions. Moreover, the main constituents of the microalgae biomass are highly dependent on microalga strain, geographical location, harvesting time of the year, and cultivation conditions (5, 28).
The liver, being the primary lipogenic tissue in poultry, is essential in shaping the fatty acid profile of broilers (54). Our experimental data delineates several nuanced interactions between the dietary inclusion of A. platensis and hepatic lipid profiles in broilers. The alteration in total lipids and cholesterol as observed in this study aligns with existing literature in which dietary supplementation with microalgae like Spirulina has been shown to modulate lipid metabolism in broilers, often leading to a reduction in total lipids (40). The cholesterol incremented in treatments fed Spirulina-based diets corroborates findings from other studies (3, 55). The unchanged sum of SFA across dietary treatments, despite the increment in certain individual SFAs, is an intriguing outcome. This finding is somewhat divergent from the literature in which microalgae supplementation has been associated with a decrease in SFAs due to its PUFA-rich profile (56). The elevation of MUFA in treatments fed Spirulina with an enzyme mixture aligns with findings where microalgae supplementation enhanced MUFA composition, particularly oleic acid (18:1 c9) (57). On the other hand, the decline in PUFA and n-6 PUFA in some treatments is contrary to our expectations, given Spirulina’s rich PUFA profile. The increment in n-3 PUFA and the reduction of the n-6/n-3 ratio, particularly in the SPE diet, is a promising finding, as a lower n-6/n-3 ratio is often deemed beneficial for health (58). This enrichment in n-3 PUFA, coupled with a balanced n-6/n-3 ratio, potentially augments the nutritional merit of broiler liver, which may translate to a healthier meat profile conducive to human consumption. These findings echo the complex interplay between dietary constituents and hepatic lipid metabolism, underscoring the need for a meticulous balance to optimize both animal and resultant meat health.
The increase in all individual n-3 fatty acids by Spirulina-based diets is a substantial finding and is supported by literature (56). The differential impacts noted between extruded Spirulina and enzyme-supplemented diets highlight the complexity of interactions between microalgae processing, enzyme supplementation, and nutrient bioavailability. Further exploration in this domain is warranted to elucidate the mechanisms underpinning these observations.
Our examination further encompassed the assessment of the impact of A. platensis diets, with or without enzyme supplementation, on the hepatic tocopherol and pigment profiles. A myriad of bioactive constituents originating from algae, encompassing antioxidants, pigments, vitamins, and polysaccharides, are lauded for their health-promoting attributes in both animals and humans. Spirulina, in particular, stands as an auspicious source of vitamins with potent antioxidant capabilities, known for mitigating inflammation. Vitamin E, a fat-soluble nutrient ubiquitously present in various foods, functions as a cellular antioxidant, fortifying cells against the onslaught of free radicals (59). While the dietary regime did not significantly modulate the levels of vitamin E compounds, α-tocopherol emerged as the predominant vitamin E homolog in all experimental treatments, a finding which is in harmony with the dietary composition. On the contrary, γ-tocopherol along with β-tocotrienol were discerned as minor constituents. In stark contrast, β-carotene, a precursor to vitamin A, alongside chlorophyll-a and total chlorophylls, witnessed a marked enhancement under the A. platensis diets, aligning seamlessly with its innate nutritional composition (2, 60). This unveils the bioavailability of these dietary elements and attests to the nutritional augmentation bestowed by Spirulina inclusion. Chlorophylls and carotenoids, naturally occurring lipophilic pigments, are instrumental in maintaining antioxidant homeostasis (61), thereby playing a pivotal role in both animal and human health (62). The elevations in chlorophyll and carotenoid levels in the liver as observed in this study, reflect the robust antioxidant potential promoted by Spirulina, capable of fostering a conducive antioxidant milieu in broiler chickens. This antioxidant potentiality shields the hepatic tissue from oxidative stress, which is indispensable for maintaining liver health and function. Furthermore, the unchanged tocopherol levels across the treatments underscore the stability and bioavailability of this vital antioxidant, even in the face of dietary modifications. These findings collectively accentuate the nutritional and health-promoting prowess of Spirulina, and by extension, underscore its potential as a viable, nutrient-rich feed ingredient in poultry diets.
Spirulina, like microalgae in general, embodies a rich cache of pivotal minerals (63), rendering them suitable dietary adjuncts for animals (64). Particularly, minerals like copper, iodine, iron, potassium, and zinc, which orchestrate key physiological undertakings such as cellular metabolism (e.g., iodine) and osmotic regulation (e.g., sodium), are abundant in microalgae (65). In light of this, our study ventured into discerning the impact of A. platensis diets on the hepatic mineral terrain of broilers. As showcased in this study, the overall concourse of macrominerals sustained a level of constancy across the Spirulina dietary spectrum, albeit sodium and magnesium manifested divergent trajectories. The hepatic sodium reservoir experienced an uptick in birds nurtured on Spirulina diets, marking a positive stride. Sodium, an indispensable nutrient, plays an important role in cellular homeostasis, fluid and electrolyte equilibrium, and blood pressure modulation. Additionally, it is instrumental in orchestrating muscle and nerve cell excitability alongside facilitating the transit of nutrients and substrates across plasma membranes (66). Conversely, despite a diminution in magnesium levels in birds fed on A. platensis diets, the decrement was marginal. Magnesium, a versatile mineral, serves as a cofactor for an expanse of over 300 enzymes, thereby modulating crucial physiological activities like muscle contraction, neuromuscular conduction, glycaemic control, myocardial contraction, and blood pressure regulation (67). On the flip side, the infusion of A. platensis with enzyme supplementation did not elicit any discernible alterations in micromineral levels.
Microminerals, such as zinc, manganese, and copper, are esteemed as quintessential cofactors for antioxidant enzymes like superoxide dismutase (68) and constitute the vanguard of antioxidant defence. Iron, a life-essential metallic entity, is implicated in engendering deleterious oxygen species via the Fenton reaction, culminating in the genesis of the potent hydroxyl radical. The transport, utilization, and storage of iron are meticulously carried out by specialized proteins like transferrin, ferritin, and haemoproteins (69, 70). The increase in hepatic Mn accentuates the potential role of dietary Spirulina in enhancing the bioavailability or retention of certain trace minerals, thus possibly fortifying the mineral nutritional status of the broilers. Collectively, the micromineral findings echo the maintenance of the redox balance within the birds, potentially underpinning a state of antioxidant homeostasis. This narrative dovetails with the overarching narrative of microalgae, especially Spirulina, being a nourishing source of essential minerals, thereby potentially fortifying the mineral nutritional status of broilers and by extension, showcasing its promise as a robust dietary supplement in poultry nutrition.
The results of the PCA align with prior studies (33, 71, 72), effectively demonstrating the distinct impacts of the experimental diets on plasma metabolites and hepatic variables in broilers. The PCA revealed four distinct clusters corresponding to each treatment group, with notable overlap between the SP and SPE treatments, yet a clear demarcation of the SPM and control treatments. This pattern suggests a significant influence of the enzyme mixture on key discriminant variables such as total lipids, triacylglycerols, and cholesterol. However, these PCA findings do not completely mirror the data presented in Table 3, indicating a complex interaction between diet composition and metabolic outcomes. The differential impact of enzyme supplementation in diets containing microalgae, observed in this study, echoes previous findings where the inclusion of 0.001% of carbohydrases, either a four-enzyme moisture (exo-β-glucosaminidase, alginate lyase, peptidoglycan N-acetylmuramic acid deacetylase and lysozyme) (NzyTech, Lisbon, Portugal) or a PL25 ulvan lyase (NzyTech, Lisbon, Portugal) feed combined with 10% of Chlorella vulgaris (33) and 15% of Ulva lactuca (71), respectively, altered the nutritional dynamics in broiler diets. Additionally, the PCA’s liver variable analysis distinguished control treatments from those fed microalgae, a finding consistent with Costa et al. (72), who reported similar differentiation in diets supplemented with 15% of Laminaria digitata and 0.001% of alginate lyase. The distinct power of Spirulina is attributed to the changes in key discriminant variables like certain n-3 PUFAs (18:3 n-3, 20:5 n-3, and 22:5 n-3), α- and γ-tocopherols, β-carotene, chlorophyll-a, and total carotenoids. These observations corroborate the findings presented in Tables 4, 5, which showed an increase in these fatty acids and pigments and a decrease in vitamin E levels with the inclusion of microalgae. Although Altmann et al. (73) also showed a distinct impact of Spirulina for n-3 PUFA, the results obtained were opposite relative to those presented in this study, which might be attributed to the different nutritional composition of microalga. Collectively, these results underscore the capacity of Spirulina, particularly when combined with enzymatic supplementation, to modulate plasma lipid components. Additionally, the data demonstrate the effect of the microalga alone in enhancing liver lipid metabolism and composition, primarily through the accumulation of health-beneficial n-3 PUFAs and antioxidant pigments. Further dissemination of the present results through social media, evidencing the benefits and constraints of incorporating Spirulina in broiler’s diet to the general public represents a promising prospective for spreading scientific knowledge (74).
This study elucidates the multifaceted impact of 15% dietary inclusion of Spirulina in broiler chickens, highlighting alterations in systemic antioxidant capacity, hepatic fatty acid composition and growth performance. Specifically, the extruded and enzyme-supplemented Spirulina diets significantly elevated hepatic n-3 fatty acid levels, fostering a favorable shift in the n-6/n-3 PUFA ratio, which is indicative of potential health benefits. This inclusion level of Spirulina also led to a reduction in total hepatic lipids and increased antioxidant carotenoids in the broiler’s liver. However, the hepatic cholesterol was increased and α-tocopherol was decreased by the addition of Spirulina, which was not reverted by either the extrusion pre-treatment or the enzymatic supplementation. The incorporation of microalga was also responsible for an increase in total cholesterol, LDL-cholesterol and total lipids in the blood, although the enzymatic mixture counteracted this effect. The microalga had adverse effects on growth performance, which were not mitigated by the enzyme supplementation but partially reverted by microalga extrusion. The extrusion of Spirulina appeared to enhance the bioavailability of some nutrients, signifying a potential processing avenue to maximize the nutritional benefits of Spirulina. These findings underscore a complex interplay between dietary Spirulina inclusion, its processing, enzymatic supplementation and the resultant effects on broiler health and growth performance.
Future studies should attempt to delineate the optimal inclusion levels and processing methods for Spirulina to mitigate the adverse effects on cholesterol and α-tocopherol, and, thus, maximizing health benefits. Additionally, the precise mechanisms underlying the observed effects, particularly the interaction between Spirulina processing, enzyme supplementation and nutrient bioavailability warrant in-depth investigation. The extrusion pre-treatment of microalga shows potential for application up to an industrial scale. Therefore, extending the research to include evaluations of the long-term effects of dietary Spirulina inclusion, and exploring varying levels of enzymatic supplementation combined with pre-extruded alga may provide more nuanced insights into harnessing the potential benefits of Spirulina in poultry diets.
The raw data supporting the conclusions of this article will be made available by the authors, without undue reservation.
The investigation strictly complied with the regulatory framework stipulated by the European Union (Directive 2010/63/EU) and procured requisite endorsements from the Ethics Review Board of CIISA/FMV, the Animal Welfare Committee of the National Veterinary Authority (Direção Geral de Alimentação e Veterinária, Portugal), and ORBEA/ISA (approval code: 0421/000/000/2022).
MS: Writing – review & editing, Investigation, Formal analysis, Methodology. CA: Writing – review & editing, Writing – original draft. MC: Writing – review & editing, Writing – original draft, Validation, Investigation, Formal analysis, Methodology. RP: Writing – review & editing, Investigation, Methodology. PL: Writing – review & editing, Writing – original draft. JPe: Writing – review & editing, Investigation, Methodology. JT: Writing – review & editing, Investigation. AM: Writing – review & editing, Investigation. MM: Writing – review & editing, Investigation, Methodology. BT: Writing – review & editing, Investigation. DC: Writing – review & editing, Investigation, Methodology. CM: Writing – review & editing, Investigation, Methodology. JF: Writing – review & editing, Investigation. ML: Writing – review & editing, Resources. JPr: Writing – review & editing, Writing – original draft, Validation, Supervision, Resources, Project administration, Funding acquisition, Conceptualization.
The author(s) declare that financial support was received for the research, authorship, and/or publication of this article. This research was financially supported by grants awarded by the Foundation for Science and Technology (FCT, Lisbon, Portugal) to CIISA (UIDB/00276/2020), AL4AnimalS (LA/P/0059/2020), and LEAF (UIDB/04129/2020). Additional financial assistance was provided by the FCT Stimulus of Scientific Employment Program to PL (DL57/2016/CP1438/CT0007), a Post-Doctoral fellowship to JP (SFRH/BPD/116816/2016), a PhD grant to MS (UI/BD/153071/2022) and a PhD grant to AM (2022.11690.BD). The project also received funding from the Portugal 2020 project (P2020/17/SI/70114/2019) and an associated researcher contract for MC.
The authors declare that the research was conducted in the absence of any commercial or financial relationships that could be construed as a potential conflict of interest.
All claims expressed in this article are solely those of the authors and do not necessarily represent those of their affiliated organizations, or those of the publisher, the editors and the reviewers. Any product that may be evaluated in this article, or claim that may be made by its manufacturer, is not guaranteed or endorsed by the publisher.
1. Abd El-Hady, A, and El-Ghalid, O. Spirulina platensis algae (Spa): a novel poultry feed additive. Effect of Spa supplementation in broiler chicken diets on productive performance, lipid profile and calcium-phosphorus metabolism. Worlds Poult Sci J. (2018) 7498:1–7.
2. Park, J, Lee, S, and Kim, I-S. Effect of dietary Spirulina (Arthrospira) platensis on the growth performance, antioxidant enzyme activity, nutrient digestibility, Cecal microflora, excreta noxious gas emission, and breast meat quality of broiler chickens. Poult Sci. (2018) 97:2451–9. doi: 10.3382/ps/pey093
3. Pestana, JM, Puerta, B, Santos, H, Madeira, MS, Alfaia, CM, Lopes, PA, et al. Impact of dietary incorporation of Spirulina (Arthrospira platensis) and exogenous enzymes on broiler performance, carcass traits, and meat quality. Poult Sci. (2020) 99:2519–32. doi: 10.1016/j.psj.2019.11.069
4. Bortolini, DG, Maciel, GM, Fernandes, IAA, Pedro, AC, Rubio, FTV, Branco, IG, et al. Functional properties of bioactive compounds from Spirulina Spp.: Current status and future trends. Food Chem. (2022) 5:100134. doi: 10.1016/j.fochms.2022.100134
5. Tounsi, L, Hentati, F, Ben Hlima, H, Barkallah, M, Smaoui, S, Fendri, I, et al. Microalgae as feedstock for bioactive polysaccharides. Int J Biol Macromol. (2022) 221:1238–50. doi: 10.1016/j.ijbiomac.2022.08.206
6. Grover, P, Bhatnagar, A, Kumari, N, Narayan Bhatt, A, Kumar Nishad, D, and Purkayastha, J. C-Phycocyanin-a novel protein from Spirulina platensis- in vivo toxicity, antioxidant and immunomodulatory studies. Saudi J Biol Sci. (2021) 28:1853–9. doi: 10.1016/j.sjbs.2020.12.037
7. Pilotto, MR, Milanez, S, Moreira, RT, Rosa, RD, and Perazzolo, LM. Potential immunomodulatory and protective effects of the Arthrospira-based dietary supplement on shrimp intestinal immune defenses. Fish Shellfish Immunol. (2019) 88:47–52. doi: 10.1016/j.fsi.2019.02.062
8. Cohen, Z . The Chemicals of Spirulina In: A Vonshak , editor. Spirulina platensis Arthrospira: Physiology, Cell-Biology and Biotechnology. London: Taylor&Francis (1997). 175–204.
9. Böcker, L, Hostettler, T, Diener, M, Eder, S, Demuth, T, Adamcik, J, et al. Time-temperature-resolved functional and structural changes of Phycocyanin extracted from Arthrospira platensis/Spirulina. Food Chem. (2020) 316:126374. doi: 10.1016/j.foodchem.2020.126374
10. Coelho, D, Lopes, PA, Cardoso, V, Ponte, P, Brás, J, Madeira, MS, et al. A two-enzyme constituted mixture to improve the degradation of Arthrospira platensis microalga Cell Wall for Monogastric diets. J Anim Physiol Anim Nutr. (2020) 104:310–21. doi: 10.1111/jpn.13239
11. Evans, AM, Smith, DL, and Moritz, JS. Effects of algae incorporation into broiler starter diet formulations on nutrient digestibility and 3 to 21 D bird performance. J Appl Poult Res. (2015) 24:206–14. doi: 10.3382/japr/pfv027
12. Costa, MM, Spínola, MP, and Prates, JAM. Combination of mechanical/physical pretreatments with trypsin or Pancreatin on Arthrospira platensis protein degradation. Agriculture. (2023) 13:198. doi: 10.3390/agriculture13010198
13. Spínola, MP, Costa, MM, and Prates, JA. Studies on the impact of selected pretreatments on protein solubility of Arthrospira platensis microalga. Agriculture. (2023) 13:221. doi: 10.3390/agriculture13010221
14. Shirazi, HM, Karimi-Sabet, J, and Ghotbi, C. Biodiesel production from Spirulina microalgae feedstock using direct transesterification near supercritical methanol condition. Bioresour Technol. (2017) 239:378–86. doi: 10.1016/j.biortech.2017.04.073
15. Alagawany, M, Elnesr, SS, and Farag, MR. The role of exogenous enzymes in promoting growth and improving nutrient digestibility in poultry. Iran J Vet Res. (2018) 19:157–64.
16. Singh, AK, Tiwari, UP, Berrocoso, JD, Dersjant-Li, Y, Awati, A, and Jha, R. Effects of a combination of xylanase, amylase and protease, and probiotics on major nutrients including amino acids and non-starch polysaccharides utilization in broilers fed different level of fibers. Poult Sci. (2019) 98:5571–81. doi: 10.3382/ps/pez310
17. Bedford, MR, and Cowieson, AJ. Exogenous enzymes and their effects on intestinal microbiology. Anim Feed Sci Technol. (2012) 173:76–85. doi: 10.1016/j.anifeedsci.2011.12.018
18. El-Hady, AMA, Elghalid, OA, Elnaggar, AS, and El-khalek, EA. Growth Performance and Physiological Status Evaluation of Spirulina platensis Algae Supplementation in Broiler Chicken Diet. Livest Sci. (2022) 263:105009. doi: 10.1016/j.livsci.2022.105009.19
19. Madeira, MSMS, Lopes, PAAB, Martins, CF, Assunção, JMP, Alfaia, CMRPM, Pinto, RMA, et al. Dietary Arthrospira platensis improves systemic antioxidant potential and changes plasma lipids without affecting related hepatic metabolic pathways in post-weaned piglets. BMC Vet Res. (2021) 17:158. doi: 10.1186/s12917-021-02869-y
20. Martins, CF, Pestana, JM, Alfaia, CM, Costa, M, Ribeiro, DM, Coelho, D, et al. Effects of Chlorella vulgaris as a feed ingredient on the quality and nutritional value of weaned piglets’ meat. Food Secur. (2021) 10:1155. doi: 10.3390/foods10061155
21. Asare, E, Yang, Z, Yang, H, and Wang, Z. Evaluation of dietary Pancreatin as an exogenous enzyme on growth performance, gene expression, immunological responses, serum Immunoglobins, and intestinal morphology in cockerels. J Appl Anim Res. (2022) 50:61–8. doi: 10.1080/09712119.2021.2019044
22. Bromfield, JI, Hoffman, LC, Horyanto, D, and Soumeh, EA. Enhancing growth performance, organ development, meat quality, and bone mineralisation of broiler chickens through multi-enzyme super-dosing in reduced energy diets. Animals. (2021) 11:2791. doi: 10.3390/ani11102791
23. Madigan-Stretton, J, Mikkelsen, D, and Soumeh, EA. Multienzyme super-dosing in broiler chicken diets: The implications for gut morphology, microbial profile, nutrient digestibility, and bone mineralization. Animals. (2020) 11:1. doi: 10.3390/ani11010001
24. AOAC . Official methods of analysis. 17th ed. Washington DC, USA: Association of Official Analytical Chemists (2000).
25. Sukhija, P, and Palmquist, D. Rapid method for determination of total fatty acid composition of feedstuffs and feces. J Agric Food Chem. (1987) 36:1202–6. doi: 10.1021/jf00084a019
26. Alfaia, CM, Pestana, JM, Rodrigues, M, Coelho, D, Aires, MJ, Ribeiro, DM, et al. Influence of Dietary Chlorella vulgaris and Carbohydrate-Active Enzymes on Growth Performance, Meat Quality and Lipid Composition of Broiler Chickens. Poult Sci. (2021) 100:926–37. doi: 10.1016/j.psj.2020.11.034
27. Prates, JA, Quaresma, MA, Bessa, RJ, Fontes, CMG, and Alfaia, CMP. Simultaneous Hplc quantification of Total cholesterol, tocopherols and β-carotene in Barrosã-Pdo veal. Food Chem. (2006) 94:469–77. doi: 10.1016/j.foodchem.2005.01.021
28. Hynstova, V, Sterbova, D, Klejdus, B, Hedbavny, J, Huska, D, and Adam, V. Separation, identification and quantification of carotenoids and chlorophylls in dietary supplements containing Chlorella vulgaris and Spirulina platensis using high performance thin layer chromatography. J Pharm Biomed Anal. (2018) 148:108–18. doi: 10.1016/j.jpba.2017.09.018
29. Ribeiro, DM, Scanlon, T, Kilminster, T, Martins, CF, Greeff, J, Milton, J, et al. Mineral profiling of muscle and hepatic tissues of Australian merino, Damara and Dorper lambs: effect of weight loss. J Anim Physiol Anim Nutr. (2020) 104:823–30. doi: 10.1111/jpn.13339
30. Friedewald, WT, Levy, RI, and Fredrickson, DS. Estimation of the concentration of low-density lipoprotein cholesterol in plasma, without use of the preparative ultracentrifuge. Clin Chem. (1972) 18:499–502. doi: 10.1093/clinchem/18.6.499
31. Covaci, A, Voorspoels, S, Thomsen, C, van Bavel, B, and Neels, H. Evaluation of Total lipids using enzymatic methods for the normalization of persistent organic pollutant levels in serum. Sci Total Environ. (2006) 366:361–6. doi: 10.1016/j.scitotenv.2006.03.006
32. Folch, J, Lees, M, and Sloane Stanley, GH. A simple method for the isolation and purification of Total Lipides from animal tissues. J Biol Chem. (1957) 226:497–509. doi: 10.1016/S0021-9258(18)64849-5
33. Coelho, DFM, Alfaia, CMRPM, Assunção, JMP, Costa, M, Pinto, RMA, de Andrade Fontes, CMG, et al. Impact of dietary Chlorella vulgaris and carbohydrate-active enzymes incorporation on plasma metabolites and liver lipid composition of broilers. BMC Vet Res. (2021) 17:229. doi: 10.1186/s12917-021-02932-8
34. Delgado, I, Ventura, M, Gueifão, S, Coelho, I, Nascimento, AC, Silva, JAL, et al. 12th Ifdc 2017 special issue – iodine, selenium and Iron contents in Portuguese key foods as consumed. J Food Compost Anal. (2019) 79:39–46. doi: 10.1016/j.jfca.2019.03.004
36. Becker, EW . Microalgae for Human and Animal Nutrition. Handbook Microal Cul. (2013):461–503. doi: 10.1002/9781118567166.ch25
37. Soni, RA, Sudhakar, K, and Rana, RS. Spirulina – from growth to nutritional product: a review. Trends Food Sci Technol. (2017) 69:157–71. doi: 10.1016/j.tifs.2017.09.010
38. Cai, B, Yi, X, Han, Q, Pan, J, Chen, H, Sun, H, et al. Structural characterization of oligosaccharide from Spirulina platensis and its effect on the Faecal microbiota in vitro. Food Sci Human Wellness. (2022) 11:109–18. doi: 10.1016/j.fshw.2021.07.012
39. Sugiharto, S, Yudiarti, T, Isroli, I, and Widiastuti, E. Effect of feeding duration of Spirulina platensis on growth performance, Haematological parameters, intestinal microbial population and carcass traits of broiler chicks. S Afr J Anim Sci. (2018) 48:98–107. doi: 10.4314/sajas.v48i1.12
40. El-Shall, NA, Jiang, S, Farag, MR, Azzam, M, Al-Abdullatif, AA, Alhotan, R, et al. Potential of Spirulina platensis as a feed supplement for poultry to enhance growth performance and immune modulation. Front Immunol. (2023) 14:1072787. doi: 10.3389/fimmu.2023.1072787
41. Mirzaie, S, Zirak-Khattab, F, Hosseini, SA, and Donyaei-Darian, H. Effects of dietary Spirulina on antioxidant status, lipid profile, immune response and performance characteristics of broiler chickens reared under high ambient temperature. Asian Australas J Anim Sci. (2018) 31:556–63. doi: 10.5713/ajas.17.0483
42. King, W, Toler, K, and Woodell-May, J. Role of white blood cells in blood- and bone marrow-based autologous therapies. Biomed Res Int. (2018) 2018:6510842–8. doi: 10.1155/2018/6510842
43. Muriel, R, Schmidt, D, Calabuig, CP, Patino-Martinez, J, and Ferrer, M. Factors affecting plasma biochemistry parameters and physical condition of osprey (Pandion Haliaetus) nestlings. J Ornithol. (2013) 154:619–32. doi: 10.1007/s10336-012-0925-0
44. Martins, CF, Ribeiro, DM, Costa, M, Coelho, D, Alfaia, CM, Lordelo, M, et al. Using microalgae as a sustainable feed resource to enhance quality and nutritional value of pork and poultry meat. Food Secur. (2021) 10:2933. doi: 10.3390/foods10122933
45. Wu, H, Li, T, Lv, J, Chen, Z, Wu, J, Wang, N, et al. Growth and biochemical composition characteristics of Arthrospira platensis induced by simultaneous nitrogen deficiency and seawater-supplemented medium in an outdoor raceway pond in winter. Food Secur. (2021) 10:2974. doi: 10.3390/foods10122974
46. Molla, MD, Degef, M, Bekele, A, Geto, Z, Challa, F, Lejisa, T, et al. Assessment of serum electrolytes and kidney function test for screening of chronic kidney disease among Ethiopian Public Health Institute staff members, Addis Ababa, Ethiopia. BMC Nephrol. (2020) 21:494. doi: 10.1186/s12882-020-02166-0
47. Nagami, GT . Hyperchloremia – why and how. Nefrologia. (2016) 36:347–53. doi: 10.1016/j.nefro.2016.04.001
48. Kaneko, JJ, Harvey, JW, and Bruss, M. Clinical biochemistry of domestic animals. 5th ed. Cambridge, MA, USA: Academic Press (1997).
49. Kuttappan, VA, Huff, GR, Huff, WE, Hargis, BM, Apple, JK, Coon, C, et al. Comparison of hematologic and serologic profiles of broiler birds with Normal and severe degrees of white striping in breast fillets. Poult Sci. (2013) 92:339–45. doi: 10.3382/ps.2012-02647
50. Sano, T, Kumamoto, Y, Kamiya, N, Okuda, M, and Tanaka, Y. Effect of lipophilic extract of Chlorella vulgaris on alimentary hyperlipidemia in cholesterol-fed rats. Artery. (1988) 15:217–24.
51. Austic, RE, Mustafa, A, Jung, B, Gatrell, S, and Lei, XG. Potential and limitation of a new defatted diatom microalgal biomass in replacing soybean meal and corn in diets for broiler chickens. J Agric Food Chem. (2013) 61:7341–8. doi: 10.1021/jf401957z
52. Spolaore, P, Joannis-Cassan, C, Duran, E, and Isambert, A. Commercial applications of microalgae. J Biosci Bioeng. (2006) 101:87–96. doi: 10.1263/jbb.101.87
53. Swiatkiewicz, S, Arczewska-Wlosek, A, and Józefiak, D. Application of microalgae biomass in poultry nutrition. Worlds Poult Sci J. (2015) 71:663–72. doi: 10.1017/S0043933915002457
54. Hermier, D . Lipoprotein metabolism and fattening in poultry. J Nutr. (1997) 127:805S–8S. doi: 10.1093/jn/127.5.805S
55. Abdel-Aty, E, El-Sheekh, M, Abdel Daim, M, and Saber, H. Effects of Spirulina platensis and Amphora Coffeaeformis as dietary supplements on blood biochemical parameters, intestinal microbial population, and productive performance in broiler chickens. Environ Sci Pollut Res. (2020) 28:1801–11. doi: 10.1007/s11356-020-10597-3
56. Bonos, E, Kasapidou, E, Kargopoulos, A, Karampampas, A, Nikolakakis, I, Christaki, E, et al. Spirulina as a functional ingredient in broiler chicken diets. S Afr J Anim Sci. (2016) 46:94–102. doi: 10.4314/sajas.v46i1.12
57. Gatrell, SK, Kim, J, Derksen, TJ, O'Neil, EV, and Lei, XG. Creating Ω-3 fatty-acid-enriched chicken using defatted green microalgal biomass. J Agric Food Chem. (2015) 63:9315–22. doi: 10.1021/acs.jafc.5b03137
58. Simopoulos, AP . An increase in the Omega-6/Omega-3 fatty acid ratio increases the risk for obesity. Nutrients. (2016) 8:128. doi: 10.3390/nu8030128
59. Brigelius-Flohé, R, Kelly, FJ, Salonen, JT, Neuzil, J, Zingg, JM, and Azzi, A. The European perspective on vitamin E: current knowledge and future research. Am J Clin Nutr. (2002) 76:703–16. doi: 10.1093/ajcn/76.4.703
60. Holman, BWB, and Malau-Aduli, AEO. Spirulina as a livestock supplement and animal feed. J Anim Physiol Anim Nutr. (2013) 97:615–23. doi: 10.1111/j.1439-0396.2012.01328.x
61. Pérez-Gálvez, A, Viera, I, and Roca, M. Carotenoids and chlorophylls as antioxidants. Antioxidants. (2020) 9:505. doi: 10.3390/antiox9060505
62. Nabi, F, Arain, MA, Rajput, N, Alagawany, M, Soomro, J, Umer, M, et al. Health benefits of carotenoids and potential application in poultry industry: a review. J Anim Physiol Anim Nutr. (2020) 104:1809–18. doi: 10.1111/jpn.13375
63. Christaki, E, Florou-Paneri, P, and Bonos, E. Microalgae: a novel ingredient in nutrition. Int J Food Sci Nutr. (2011) 62:794–9. doi: 10.3109/09637486.2011.582460
64. Morais, T, Inácio, A, Coutinho, T, Ministro, M, Cotas, J, Pereira, L, et al. Seaweed potential in the animal feed: a review. J Mar Sci Eng. (2020) 8:559. doi: 10.3390/jmse8080559
65. Priyadarshani, I, and Rath, B. Commercial and industrial applications of Micro algae–a review. J Algal Biomass Util. (2012) 3:89–100.
67. Al Alawi, AM, Majoni, SW, and Falhammar, H. Magnesium and human health: perspectives and research directions. Int J Endocrinol. (2018) 2018:9041694–17. doi: 10.1155/2018/9041694
68. Fukai, T, Folz, RJ, Landmesser, U, and Harrison, DG. Extracellular Superoxide Dismutase and Cardiovascular Disease. Cardiovasc Res. (2002) 55:239–49. doi: 10.1016/j.yjmcc.2009.08.010
69. Beaumont, C, Laverdant, C, Ardaillou, R, Nordmann, R, David, G, and Sureau, C. Le Métabolisme Intracellulaire Du Fer. Bull Acad Natl Med. (2000) 184:313–24.
70. Comporti, M, Signorini, C, Buonocore, G, and Ciccoli, L. Iron release, oxidative stress and erythrocyte ageing. Free Radic Biol Med. (2002) 32:568–76. doi: 10.1016/s0891-5849(02)00759-1
71. Alfaia, CM, Costa, MM, Pinto, RMA, Pestana, JM, Mourato, M, Carvalho, P, et al. Plasma metabolites and liver composition of broilers in response to dietary Ulva lactuca with Ulvan Lyase or a commercial enzyme mixture. Molecules. (2022) 27:7425. doi: 10.3390/molecules27217425
72. Costa, MM, Lopes, PA, Assunção, JMP, Alfaia, CMRPM, Coelho, DFM, Mourato, MP, et al. Combined effects of dietary Laminaria digitata with alginate Lyase on plasma metabolites and hepatic lipid, pigment and mineral composition of broilers. BMC Vet Res. (2022) 18:153. doi: 10.1186/s12917-022-03250-3
73. Altmann, BA, Wigger, R, Ciulu, M, and Mörlein, D. The effect of insect or microalga alternative protein feeds on broiler meat quality. J Sci Food Agric. (2020) 100:4292–302. doi: 10.1002/jsfa.10473
Keywords: feed enzymes, hepatic compounds, microalga extrusion, plasma metabolites, poultry nutrition
Citation: Spínola MP, Alfaia CM, Costa MM, Pinto RMA, Lopes PA, Pestana JM, Tavares JC, Mendes AR, Mourato MP, Tavares B, Carvalho DFP, Martins CF, Ferreira JI, Lordelo MM and Prates JAM (2024) Impact of high Spirulina diet, extruded or supplemented with enzymes, on blood cells, systemic metabolites, and hepatic lipid and mineral profiles of broiler chickens. Front. Vet. Sci. 11:1342310. doi: 10.3389/fvets.2024.1342310
Received: 21 November 2023; Accepted: 14 March 2024;
Published: 26 March 2024.
Edited by:
Damiano Cavallini, University of Bologna, ItalyReviewed by:
Tumisang Ben Matshogo, North-West University, South AfricaCopyright © 2024 Spínola, Alfaia, Costa, Pinto, Lopes, Pestana, Tavares, Mendes, Mourato, Tavares, Carvalho, Martins, Ferreira, Lordelo and Prates. This is an open-access article distributed under the terms of the Creative Commons Attribution License (CC BY). The use, distribution or reproduction in other forums is permitted, provided the original author(s) and the copyright owner(s) are credited and that the original publication in this journal is cited, in accordance with accepted academic practice. No use, distribution or reproduction is permitted which does not comply with these terms.
*Correspondence: José A. M. Prates, amFwcmF0ZXNAZm12LnVsaXNib2EucHQ=
†These authors have contributed equally to this work and share first authorship
Disclaimer: All claims expressed in this article are solely those of the authors and do not necessarily represent those of their affiliated organizations, or those of the publisher, the editors and the reviewers. Any product that may be evaluated in this article or claim that may be made by its manufacturer is not guaranteed or endorsed by the publisher.
Research integrity at Frontiers
Learn more about the work of our research integrity team to safeguard the quality of each article we publish.