- 1College of Animal Science and Technology, Gansu Agricultural University, Lanzhou, China
- 2Yizhou District Animal Disease Prevention and Control Center, Hami, China
- 3College of Science, Gansu Agricultural University, Lanzhou, China
Plant extracts are rich in a variety of nutrients and contain a large number of bioactive compounds, and compared with traditional feed additives, they have advantages such as wide sources, natural safety and rich nutrition. This study employed in vitro antioxidant and animal experiments to comprehensively evaluate the use of Toona sinensis extract (TSE) in broiler production. 508 1-day-old Cobb 500 broilers were randomly assigned to the 7 experimental groups with 6 replications and 12 birds/replicate. Two groups received Vitamin C (VC) 300 g/t and Vitamin E 500 g/t, and five dose groups of TSE received 0, 300, 600, 900, and 1,200 g/t of TSE in their feed. The study spanned 42 days, with a starter phase (1–21 days) and a finisher phase (22–42 days). The results showed that compared to ascorbic acid, TSE had the scavenging ability of 2,2-Diphenyl-1-picrylhydrazyl and hydroxyl radical, with IC50 values of 0.6658 mg/mL and 33.1298 mg/mL, respectively. Compared to TSE 0 group, broilers fed with 1,200 g/t TSE showed significant weight gain during the starter phase and increased the feed-to-weight gain ratio during both the starter and finisher phases. Additionally, broilers receiving 1,200 g/t TSE had enhanced dry matter and organic matter utilization. Concerning meat quality, broilers in the 1,200 g/t TSE group demonstrated increased cooked meat yield, and pH value, as well as higher antioxidant capacity (T-AOC), dismutase (SOD), and glutathione peroxidase (GSH-PX) in serum. In addition, there was no significant difference in ileal microflora due to TSE supplementation. In summary, this study confirms the positive impact of a dietary inclusion of 1,200 g/t TSE on broiler growth, meat quality, and serum antioxidants.
Introduction
With the development of the economy and the improvement of living standards, people’s demand for animal products continues to grow, which has promoted the industrial development of animal husbandry. However, backward production capacity will gradually be eliminated by the tightening of national policies. In the context of enhanced environmental protection policies and government regulation, antibiotics play a key role in animal growth and development, improving feed conversion efficiency, and preventing infections (1, 2). However, the largescale use of antibiotics has led to growing concerns about drug resistance and human health risks (3). In recent years, many countries have restricted or even banned the use of antibiotics in animal feed (4). Since July 1, 2020, the production of commercial feed containing growth-promoting drugs and other feed additives has been banned in China. Therefore, reducing or replacing the use of antibiotics has become an urgent issue for the breeding industry. Recent studies have shown that active substances such as flavonoids and polyphenols et al. in plant extracts can enhance the innate immunity of animals, promote growth and development, reduce oxidative stress, maintain intestinal integrity, promote the growth of beneficial bacteria, and reduce intestinal infections (5).
Toona Sinensis (A. Juss.) Roem (TSR), an indigenous Meliaceae family plant and a deciduous woody species, is native to East and Southeast Asia (6). It has been cultivated for over 2,000 years in China, Malaysia, and other parts of Asia as a popular seasonal vegetable (7). In addition, TSR has been utilized as a Chinese patent medicine in Traditional Chinese Medicine (TCM) for various ailments. For instance, TSR bark is used for treatments of dysentery, enteritis, and urinary infections; leaves for dysentery; seeds for chronic gastritis; and the roots are used for heat-eliminating, detoxifying, and astringent properties (8, 9). Previous studies have validated the therapeutic effects of TSR, which are primarily attributed to its flavonoids, phenols, terpenoids, and other bioactive sub-stances that contribute to metabolic regulation and growth and development (10, 11), These bioactive substances not only promote the absorption and metabolism of nutrients but also enhance the quality of livestock products (meat quality, dairy quality, etc.) (12, 13). Furthermore, modern medical research has demonstrated various medicinal properties of TSR. It can regulate lipid metabolism, alleviate hyperglycemia, enhance immunity, delay liver fibrosis, regulate microcirculation, affect blood pressure, combat oxidative stress, induce cancer cell apoptosis, inhibit sarcoma growth, etc. (14–16). Despite its established role in TCM, food, and compounds, there has been limited literature on its application in poultry and animal production, particularly in broiler production. Based on previous findings, we hypothesize that TSR extract (TSE) could serve as a novel feed additive to enhance animal growth performance in specific aspects. The aim of the current study was to evaluate the TSE in vitro antioxidant activity, and its impact of supplementing broiler chickens with varying amounts of these extracts on growth performance, meat quality, serum antioxidants, and intestinal microbial diversity were also investigated.
Materials and methods
Chemicals and reagents
The Toona Sinensis Extract (TSE) used in this experiment was supplied by Personalbio Technology Co., Ltd. as a powder preparation with an effective active substance content of 10%. VC (Vitamin C)-standard products, 2,2-Diphenyl-1-picrylhydrazyl (DPPH) reagents, and salicylic acid were purchased from Jiangsu Enzyme Industry Co., Ltd.
In vitro antioxidant activity assay
The DPPH radical scavenging ability was assessed following the methods of Hu et al. (17). Hydroxyl radical scavenging assays were conducted following the procedure of Li et al. (18). Ascorbic acid (VC) was used as a positive control to evaluate the DPPH and hydroxyl radical scavenging activities of the TSE comparatively.
Broiler feeding management and experimental design
We sourced 504 healthy, 1-day-old Cobb500 male white-feathered broilers (50 ~ 55 g) from Shaanxi Baoji Hualong Animal Husbandry Co., Ltd., China. These birds were randomly allocated into seven distinct groups. Each group consisted of 6 replicates, and each replicate had 12 birds. The dietary interventions for seven groups involved basic feeds supplemented with VC 300 g/t, Vitamin E (VE) 500 g/t, and five dose groups of TSE, including 0, 300, 600, 900, and 1,200 g/t, respectively (The influence factors of animal tests are complex, and double positive controls are needed to confirm the expected effect and exclude accidental factors, so as to improve the credibility and scientific results). In addition, the grower diet contained 0.4% titanium dioxide per kg feed as an indigestible marker to allow for the determination of nutrient digestibility. The precise formulation and nutritional constituents of these feeds are listed in Table 1. The study spanned 42 days with two distinct phases: the “starter” phase (1–21 days) followed by the “finisher” phase (22–42 days). The broilers were randomly allocated, according to similar body weight, to individual wire cages (160 × 70 cm2) with raised-wire floors and automatic nipple drinkers. Throughout the study duration, the broilers resided in a controlled environment with free access to feed and water. For the first 3 days, the temperature was set at 36–38°C, and thereafter, we systematically reduced it by 5°C every week to 24°C. This environment ensured 23 h of consistent lighting, interspersed with a one-hour dark period.
Sample collection and Indicator measurement
Growth performance
Any incidences of animal mortality were noted for all cages daily. On the 21st and 42nd mornings, after ensuring a 12-h fasting period, both the average feed intake (AFI) and the average weight gain (AWG) were systematically assessed. To ensure data accuracy, parameters such as the feed-to-weight gain (F/G) ratio and related metrics were used for adjustments to account for any losses due to mortality.
Apparent total tract digestibility
During the 38 to 41 days, each replicate cage collects excrement and removes impurities from it (5 mL sulfuric acid was added to the collection bowl to prevent nitrogen release before collection), and stored at −20°C. At the end of the collection period, three days of fecal samples were thawed and combined, then dried at 65°C for 72 h for determination dry matter (DM), organic matter (OM), nitrogen (N), and metabolizable energy (ME). The TiO2 assay was conducted as described by Morgan et al. (19). DM, OM, and N levels were estimated using the methods outlined by the AOAC (20). The ME of samples was determined using a calorimeter (C2000, IKAWERKE Co., Ltd., Staufen, Germany).
Meat quality analysis
Meat color (a* redness, b* yellowness, and L* brightness), drip loss, and pH value were determined as described by Jin et al. (21). After 45 min of slaughter, the meat color inside the breast and thigh muscles was measured twice (rotated 90°) with a 50 mm aperture colorimeter (CR410, Minolta, Japan) to calculate the average value. Subsequently, the meat sample was stored at 4°C for 24 h for a repeat measure of the meat color. The pH value of the breast and thigh meat was measured 45 min and 24 h after slaughter using a pH electrode meter (HI99161, Hanna, Italy). Drip loss was determined as described by Jin et al. (21). Briefly, muscle blocks (2 cm thick, 3 cm wide, and 5 cm long) were cut from the same position of the pectoralis and thigh muscles, weighed, put into zipper bags, and hung freely. After storage at 4°C for 24 h, the pieces were reweighed to obtain the final weight. This final weight was used to assess drip loss.
The meat shear force was determined as described by Jin et al. (21). Briefly, meat samples were retrieved from 0–4°C storage and then put in a constant-temperature water bath set at 80°C. The sample was heated until the core temperature reached 70°C, then cooled down to 0–4°C. Using a circular sampler with a diameter of 1.27 cm, meat samples were drilled and cut parallel to the direction of the muscle fibers. The length of the sampling hole should not be less than 2.5 cm, and the sampling position should be at least 5 mm from the edge of the sample. The distance between the edges of two adjacent samples should be no less than 5 mm. Meat shear force was immediately measured with a digital muscle pressure pain tester (C-LM3B, TENOVO, China).
Water loss was determined using the filter paper press method as described by Farouk et al. (22). About 1 g of the meat sample (W1) was weighed. After placing 16 layers of qualitative filter paper above and below the meat sample, a nonabsorbent plastic plate was added on both sides. The sample was then placed on the dilatometer platform and subjected to a continuous pressure of 68.66 KPa for 5 min. After the pressure was removed, the meat sample was weighed again (W2). The water loss rate was calculated as follows:
The meat cooking rate was determined within 12 h of slaughtering. An appropriate amount of chicken breast meat (W1) was put in a steamer (1,500 W) and steamed at 100°C for 45 min. The meat was then taken out and cooled for 30–40 min in a windless room. A filter paper was used to blot the surface moisture, and ultimately the meat was weighed (W2) to determine the cooking rate as follows:
Antioxidant capacity
At 42 d, one bird from each replicate was randomly selected. Blood samples (5–10 mL) were obtained from the inferior wing vein. The serum samples were collected by centrifugation at 3000 × g at 4°C for 10 min and stored at −80°C for further serum antioxidant indices analysis. The kits provided by Jiangsu Enzyme Industry Co., Ltd. were used to measure the levels of superoxide dismutase (SOD), glutathione peroxidase (GSH-PX), malonaldehyde (MDA), and total antioxidant capacity (T-AOC) following the kits’ instructions and a spectrophotometer.
Intestinal microbial diversity
The measurement indicators in this study included effective sequences, optimized sequences, OTUs (operational taxonomic units), species abundance, and community structure. Immediately after the dissection, the ileum was stripped, and the ileal contents were collected in 5 mL cryovials, which were quickly frozen in liquid nitrogen and then stored at −80°C. We performed paired-end (PE) sequencing to determine the intestinal microbial diversity. FLASH software was used for data organization and control, and a small fragment sequence library was constructed for sequencing. Species composition and variability between samples were explored by data filtering and analysis of species annotation curves, abundance distribution curves, alpha diversity, beta diversity, and species distribution. This analysis was aimed at examining the composition and differences of species in a sample. The sequencing of the gut microbiota was performed by Biomicr Biotechnology Co., Ltd., China.
Data statistics and processing
All data were collated in Excel 2007 and then processed in SPSS 22.0 with the One-way ANOVA method. When the difference was significant, Duncan’s method was used for multiple comparison tests. p ≤ 0.05 was set as the cut-off significance level. A trend was identified when 0.05 < p < 0.10. Bioinformatic analysis of microbe-related data was conducted using Qiime, Mothur, and MEGAN4 programs.
Results
Antioxidant activity of TSE and ascorbic acid in vitro
A DPPH free radical assay was performed to assess the antioxidant activity of TSE and ascorbic acid. After their interactions with free radical scavengers, the purple color of the DPPH solution turned lighter; sample absorbance was measured at 517 nm. Figure 1A shows a clear linear relationship (y = 69.94x-3.43, R2 = 0.9997) between TSE con-centration and DPPH radical scavenging rate, i.e., with the increasing TSE concentration, its ability to scavenge DPPH free radicals increased. The IC50 concentration of TSE to achieve 50% clearance of DPPH was 0.6658 mg/mL. We also determined the DPHH-scavenging ability of ascorbic acid as a positive control. As shown in Figure 1B, ascorbic acid also has a linear scavenging rate of DPPH radicals. Based on the regression equation of y = 6.52x-11.78, R2 = 0.9997, the IC50 of ascorbic acid was determined to be 9.47546 μg/mL.
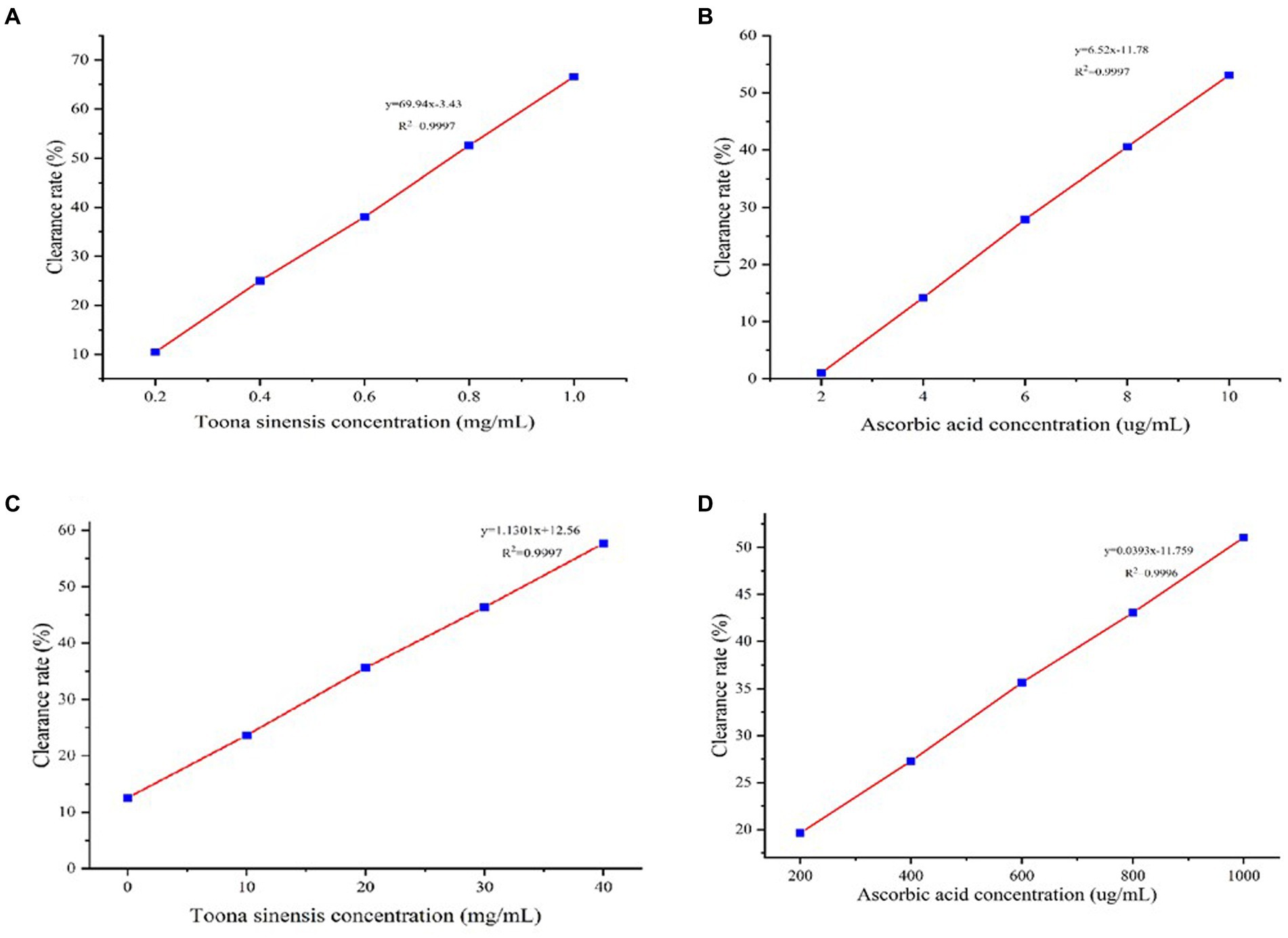
Figure 1. The antioxidant activities of TSE and VC were assessed by measuring their ability to scavenge DPPH and hydroxyl radicals. (A) DPPH radical scavenging rate curve of TSE, (B) DPPH. radical scavenging rate curve of ascorbic acid, (C) Hydroxyl radical scavenging rate curve of TSE, and (D) Hydroxyl radical scavenging rate curve of ascorbic acid.
We next determined the hydroxyl radical scavenging activity of TSE and ascorbic acids. The TSE scavenging rate of hydroxyl radicals is shown in Figure 1C. Based on the regression equation of y = 1.301x + 2.56, R2 = 0.9997, the IC50 concentration of TSE to achieve 50% clearance of hydroxyl radicals was 33.1298 mg/mL. Likewise, we deter-mined the hydroxyl radical scavenging activity of ascorbic acid (VC) (Figure 1D). Based on the regression equation of y = 0.0393x-11.759, R2 = 0.9997, the IC50 concentration of ascorbic acid to achieve 50% clearance of hydroxyl radicals was 973.05 μg/mL.
Effect of TSE on the growth performance of broilers
As shown in Table 2, in the starter stage (1–21 days), there was no significant difference in AFI between treatment groups and control groups (p > 0.05), compared with negative control group (TSE 0) dietary supplementation of VC 300 g/t, VE500 g/t, TSE 300 g/t, 600 g/t, 900 g/t and 1,200 g/t significantly increased AWG (p < 0.05), adding TSE 300 g/t and 1,200 g/t significantly increased the AWG (p < 0.05), and at the same time significantly reduced the F/G (p < 0.05).
In the finisher stage (22–42 days), compared with the negative control group (TSE 0), the AFI and F/G of broilers in each treatment were decreased and increased AWG, but not statistically significant (p > 0.05).
In the total period (1–42 days), dietary supplementation of VC 300 g/t, VE500 g/t, TSE 300 g/t, 600 g/t, 900 g/t and 1,200 g/t significantly decreased the F/G (p < 0.05). Dietary supplementation with 300 g/t VC, TSE 300 g/t, 600 g/t, 900 g/t and 1,200 g/t decreased the AFI. Adding VC 300 g/t, VE500 g/t, TSE 300 g/t, 600 g/t, 900 g/t, 1,200 g/t increased the AWG, but there was no significant difference (p > 0.05).
Nutrient utilization efficiency
As shown in Table 3, compared to other treatments, the broiler chickens fed TSE 900 and TSE 1200 g/t retained the highest amount of DM (p < 0.05). The dietary supplementation of the VC, VE and TSE significantly improved the OM utilization rate of broilers (p < 0.05), and the best effect was exhibited by the TSE 1200 g/t. Except for the TSE 300 g/t, all other groups exhibited a significant increase in nitrogen metabolism rate (p < 0.05), with the TSE 900 g/t demonstrating the highest value (p < 0.05). In addition, dietary supplementation of VC, VE and TSE had no significant effect on ME (p>0.05).
Effect of TSE on meat quality
The results of the meat quality analysis are listed in Table 4. Compared with the TSE 0 g/t, the dietary supplementation of VC and VE increased the drip loss of chicken (p < 0.05), TSE 300, 600, and 900 g/t effectively reduced the drip loss of chicken, but there was no significant difference (p > 0.05). The dietary supplementation with VC, VE, and TSE significantly improved the shear force and cooked yield of breast and thigh muscles (p < 0.05); however, we noticed no significant effect on the meat color or pH45min on breast and thigh muscles (p > 0.05). Notably, pH24h was significantly increased (p < 0.05).
Effect of TSE on serum antioxidants
The results listed in Table 5 show that the dietary supplementation of antioxidants and TSE significantly increased the serum contents of T-AOC, GSH-PX, and SOD in broilers (p < 0.05). The contents of T-AOC, GSH-PX and SOD in serum of broilers were increased with the increase of dietary TSE dose (p < 0.05). In addition, the dietary supplementation of antioxidants and TSE significantly reduced the MDA content in broiler serum (p < 0.05), and with the increase of TSE addition, MDA content showed a continuous decrease (p < 0.05).
Effect of TSE on the intestinal microflora of broilers
Gut microbial raw data processing and sample sequence number statistics
According to the statistical results of production performance of broiler chickens during feeding, AWG and F/G of TSE1200g/t group are more advantageous than those of other treatment groups. In addition, the chickens in this test are commercial generation. Therefore, under the premise of economic benefits, the ileum microbiota of TSE 0 g/t (group C) and TSE 1200 g/t (group T) broilers was further studied.
In this experiment, we processed the PE sequencing data obtained by Hiseq sequencing by splicing (merging) them into sequence tags based on the overlap between PE reads. We also conducted quality control and filtering for the read quality and merge effect. FLASH v1.2.7 software was used to splice samples and obtain the original data, followed by data filtering using the Trimmomatic v0.33 software. The optimized number of sequences was then subjected to chimeric sequence removal by UCHIME v4.2 software. Data in Table 6 show that the effective sequence count for broiler ileum in the group C was 12,787, with 12,683 sequences for subsequent analysis. In the group T, with effective sequence count of the ileum was 12,848, with 12,357 sequences for subsequent analysis.
Ileal microbial diversity
The QLLME 1.8.0 software was utilized for clustering with a similarity threshold set at 97%, resulting in the detection of a total of 470 OTU. Specifically, there were 463 OTU observed for TSE 0 g/t (group C) and 462 OTU for TSE 1200 g/t (group T) (Figure 2A). The dilution curves, which illustrate the species’ diversity and richness in each sample, exhibit a plateau at 8,000 reads, indicating that the sequencing coverage has reached saturation (Figure 2B).
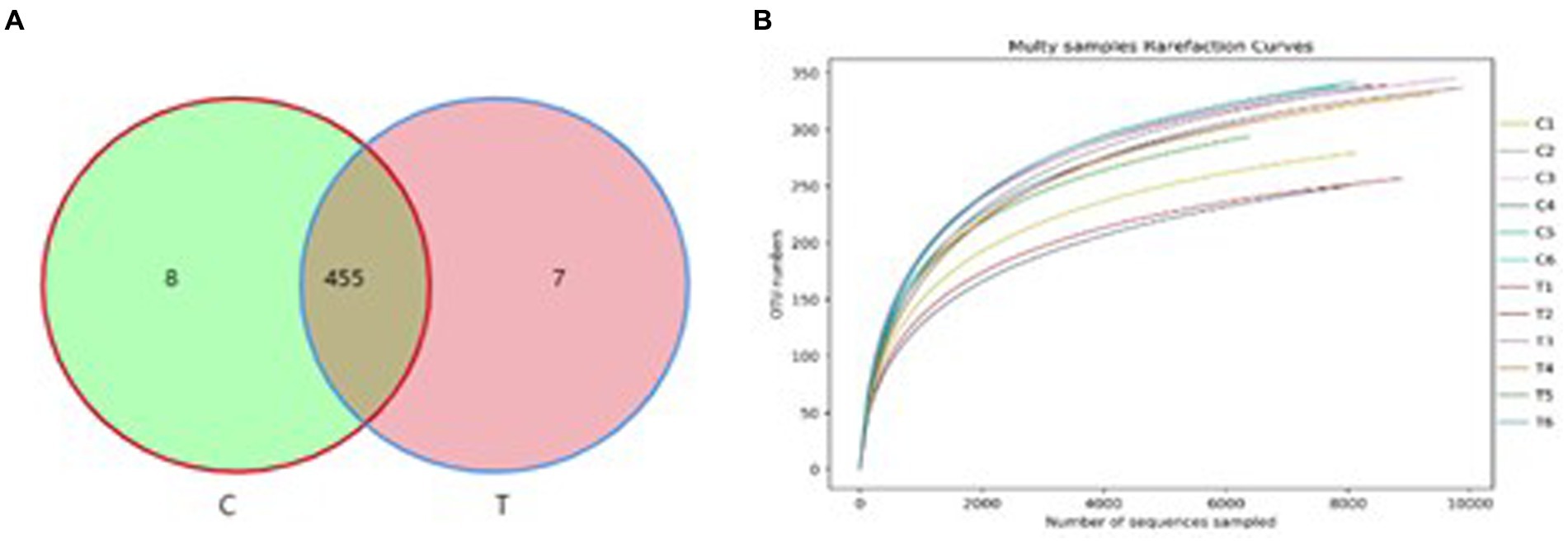
Figure 2. OTU statistical analysis. (A) Analysis results of OTU-Venn and (B) Dilution curve analysis.
Diversity analysis
The results of the alpha-diversity analysis showed that the dietary TSE supple-mentation had no significant effect on the abundance and diversity of ileal microorganisms in broilers (Figure 3). Principal coordinates analysis (PCoA) and non-metric multidimensional scaling (NMDS) are dimensionality reduction techniques that employ a two-dimensional plane to visually represent the dissimilarity between samples. The x and y axes of the PCoA plot corresponded to 19.70% and 17.84% contributions, respectively (Figure 4A). This indicated that the data effectively captured the variation among samples. The stress value of 0.132 indicated that sample points within the same group exhibited heterogeneity (Figure 4B).
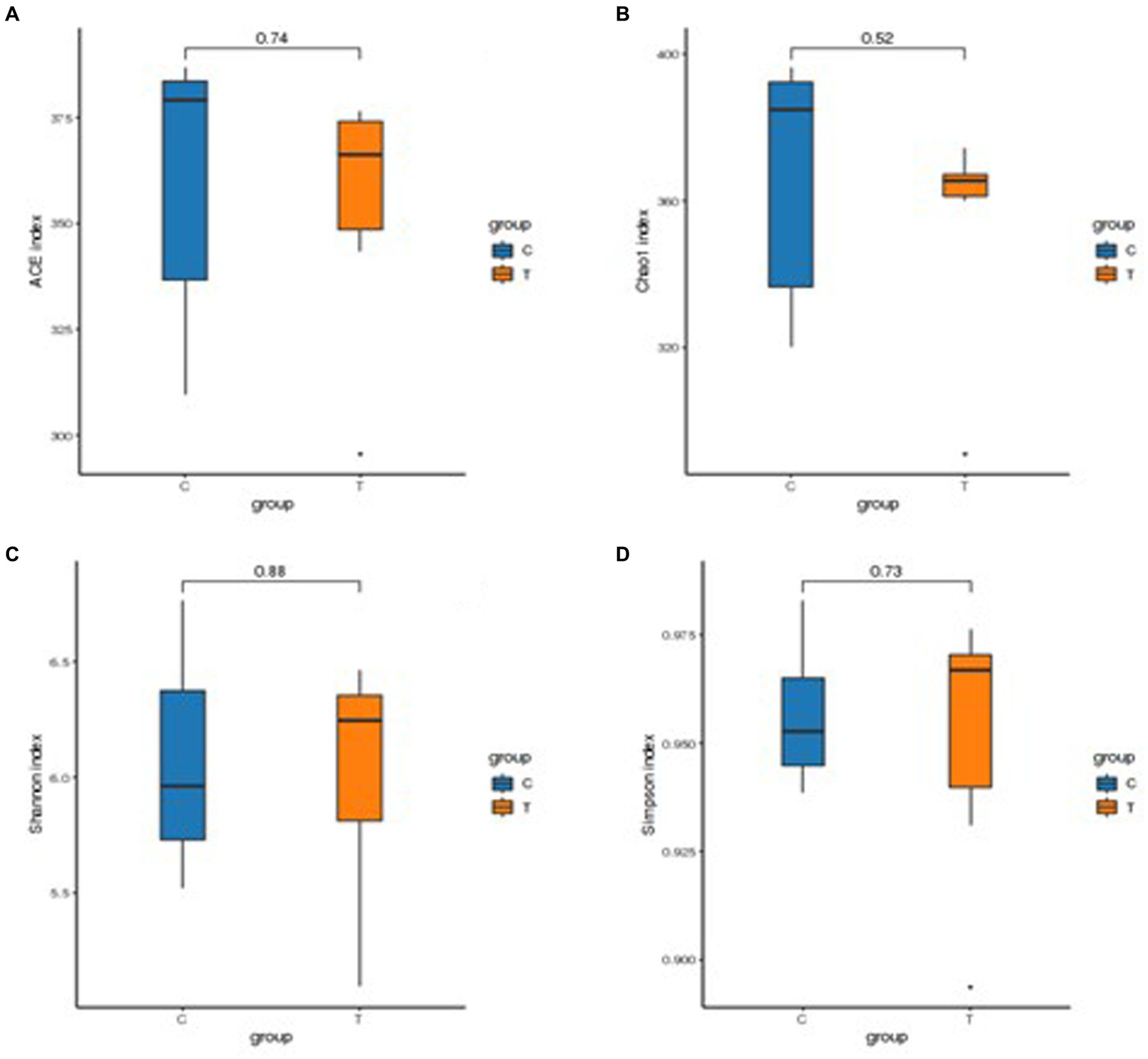
Figure 3. Statistical results of alpha diversity analysis of intestinal flora. (A) ACE index, (B) Chao 1 index, (C) Shannon index, and (D) Simpson index.
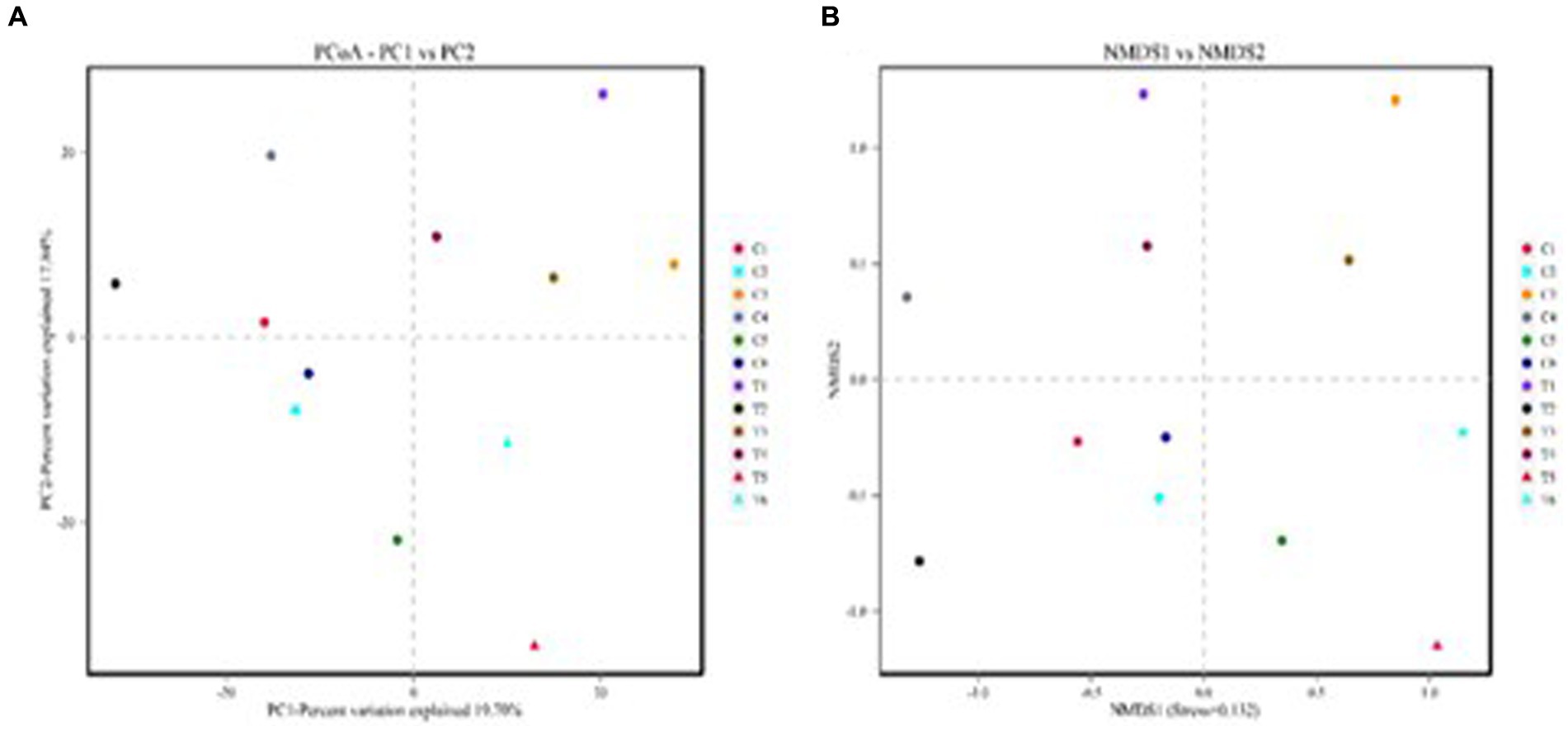
Figure 4. Statistical results of beta diversity analysis of intestinal flora. (A) PCoA and (B) NMDS.
Microbial composition analysis
According to the species dilution results, we selected the top eight microorganisms with the highest abundance at the phylum level for relative histogram analysis (Figure 5A). Based on the statistical analysis of the species abundance of broiler intestinal microorganisms at the phylum level, we identified Firmicutes, Bacteroidetes, Actinobacteria, Cyanobacteria, Verrucomicrobiota, Desulfobacterota Proteobacteria, and un-known bacteria. Among them, Firmicutes accounted for more than 60% of the intestinal bacteria of broilers. The levels of Cyanobacteria in T-group broilers were higher than those in the C-group. The proportion of Bacteroidetes was about the same in both groups.
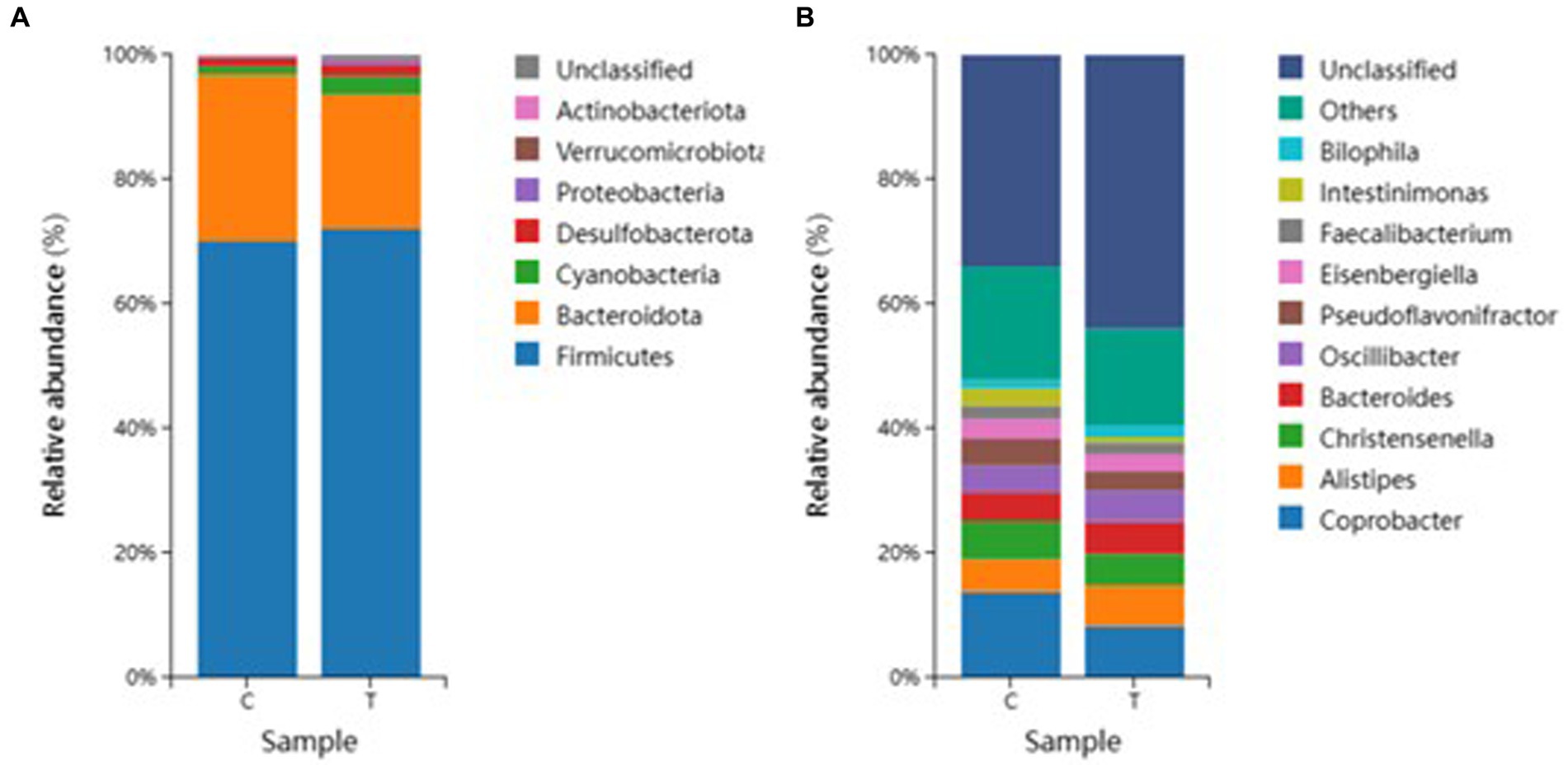
Figure 5. Species composition analysis. (A) Phylum level species composition and (B) Genus level species composition.
In a further analysis focused on the genus level, we selected the top twelve microorganisms with the highest abundance at the genus level in each test sample to make a relative histogram (Figure 5B). The analysis and statistics of the species abundance of broiler intestinal microorganisms at the genus level found Coprobacter, Alistipes, Christensenella, Bacteroides, Oscillibacter, Pseudoflavonifractor, Eisenbergiella, Faccalibacterium, Intestinimonas, Bilophila, and other unknown genera. The relative abundance of Coprobacter in the C group was higher than that in the T group. The distribution of Intestinimonas in group C was greater than that in group T, and the unknown bacteria and other bacteria in the C and T groups accounted for more than 60% of the total bacterial content.
Discussion
The human health benefits of antioxidants, including their anticancer, antiviral, cardioprotective, and neuroprotective properties, are widely recognized (23–26). Cellular enzymes involved in oxidation and reduction play a vital role in counteracting oxidative damage caused by excessive production of reactive oxygen species (ROS). However, this cellular defence mechanism can be compromised due to disease or aging (27). Toona leaves contain natural antioxidants like flavonoids and polyphenols, which are important phenolic derivatives (28). Researchers, nutritionists, and food manufacturers are keen on understanding the health impact of dietary polyphenols. Polyphenolic compounds have a wide range of biological effects, including combating bacteria, reducing inflammation, protecting liver function, preventing blood clotting, and promoting vasodilation. These diverse functions are often linked to their scavenging of free radicals and antioxidant properties (29, 30). Antioxidants achieve their benefits through various mechanisms, such as reducing the concentration of molecular oxygen or oxidative metal ions, trapping and neutralizing ROS like O2− and hydrogen peroxide, scavenging free radicals that initiate chain reactions (e.g., •OH, RO• or ROO•), disrupting these chains, or quenching singlet oxygen (31). Free radicals are chemically reactive species with unpaired electrons in their outer orbitals, making them highly reactive and initiators of chain reactions (24, 32). Most free radicals in the body are ROS or reactive nitrogen species (RNS). To comprehensively understand the free radical scavenging properties of a substance, it’s recommended to use multiple assays. Accordingly, we selected one ROS (OH) and one RNS (DPPH) species to evaluate the free radical scavenging ability of TSE. Based on the in vitro antioxidant results of this study, although TSE has a lower free radical scavenging rate than VC, it also has certain free radical scavenging ability.
In the modern commercial breeding system, the elevated temperature environment has led to numerous adverse effects on the growth and development of poultry. Under high-temperature conditions, poultry’s feed intake decreases, growth rate slows down, intestinal health is compromised, oxidative stress intensifies, and the immune response is impeded (33). When the environment changes, animals adjust and restore their homeostatic conditions. In this process, certain stress responses are triggered (34). Abnormal temperature and rainfall patterns resulting from global warming pose various stressors on poultry farming, including technical, environmental, and nutritional challenges. These stressful conditions eventually result in oxidative stress (35). Research has demonstrated that oxidative stress can cause apoptosis in follicle cells, reducing the number of follicles, and consequently leading to diminished egg production (36). Moreover, stress can reduce egg quality characteristics, yolk lipid, and cholesterol con-tent. In the case of laying hens, sexual maturity and the onset of egg-laying are of great importance, and oxidative stress can adversely affect their performance (34). In the case of chicks, oxidative stress during growth and development may result in nutrient deficiencies that affect liver and bone metabolism (37). To mitigate the impact of oxidative stress in poultry farming, managers typically enhance poultry adaptability by improving feed nutrition and poultry housing, thus enhancing growth performance. The TSE selected in this study is a novel type of plant-based feed additive, which is less commonly used in livestock and poultry production. However, through the analysis of the chemical composition of Toona sinensis, it was discovered that its flavonoids are widely utilized in livestock and poultry farming, playing a significant role in enhancing animals’ growth performance (38–40). In this study, we show that TSE has a positive impact on the growth performance of broilers. TSE increased AWG and reduced the F/G ratio in chickens in the starter phase. There was also a decrease in the F/G ratio in chickens in the total period. Moreover, 1,200 g/t TSE was found significantly superior to VC and VE supplements. The TSE-mediated enhancement of broilers’ growth performance can be attributed to its flavonoids. They may help alleviate oxidative stress and regulate beneficial intestinal microflora. Good intestinal health is pivotal for animals’ digestion, ab-sorption, and immune functions (41–43). Furthermore, flavonoids can enhance animals’ blood circulation, increasing oxygen and nutrient supply (44, 45). Proper blood circulation is indispensable for enhancing an animal’s physical activity, growth rate, and overall growth performance. As for the reason that TSE is only effective in the starter phase and not effective in the finisher phase, we speculate that it may be because in the early stage of broiler growth (1–21 days), plant extracts may promote growth and improve feed utilization, thus significantly improving the production performance of broilers. However, as broilers grow to the later stage (22–42 days), there may be saturation of feed intake and slowing down of growth rate, resulting in the effect of plant extracts is no longer obvious, but the specific influencing factors still need to be further explored.
Enhancing poultry product quality and nutritional value is a key goal in the farming industry, responding to the growing demand for healthy food. Natural plant extracts are frequently added to poultry feed to optimize nutrient utilization. Toona sinensis have rich in nutrients and biologically active compounds (46). Our study revealed that dietary TSE significantly enhances the utilization of DM and OM in broilers, with the 1,200 g/t addition group showing the best results. Furthermore, TSE boosts broilers’ nitrogen utilization, reducing nitrogen emissions. However, TSE’s regulatory mechanisms on broiler nutrient efficiency remain underexplored. Analysis of TSE reveals that poly-phenolic compounds and cellulose stimulate intestinal peristalsis, digestive juice secretion, and nutrient absorption (47, 48). Flavonoids and phenolic acids maintain intestinal microbe balance, promoting beneficial bacteria growth while reducing harmful bacteria, thus enhancing intestinal health. This supports better nutrient absorption and utilization (49–51). Flavonoids and polyphenols also inhibit RNS production in urine while improving nitrogen absorption and utilization, ultimately reducing nitrogen excretion (52). In summary, TSE may reduce fecal nitrogen content and improve growth performance of broilers by inhibiting nitrogen excretion and promoting nitrogen utilization, but the specific regulatory mechanisms and details may require further research and discussion. Broiler farming has transitioned to large-scale production, driven by increased productivity and technological advancements. Current priorities involve optimizing broiler meat characteristics that influence consumer purchasing decisions, such as appearance, texture, juiciness, wateriness, firmness, tenderness, smell, and flavor. Additionally, quantifiable properties like water holding capacity, shear force, drip loss, cooked meat ratio, and pH are essential considerations (53). In the context of the global antibiotic ban, poultry nutrition plays a crucial role in poultry meat quality and safety. Finding feed additives that prevent poultry diseases and improve meat quality is a pressing issue in the poultry breeding industry. Our experiment reveals that incorporating TSE into broiler diets reduces chicken drip loss, enhances cooked meat ratios and pH, and extends shelf-life. However, TSE and antioxidants did not improve tenderness; instead, they increased meat shear force. Currently, there is limited research on TSE’s effect on broiler meat quality. The results showed that polyphenol extracts from eucalyptus leaves, flavonoids from scutellaria and oyster mushrooms had positive effects on animal meat quality (54–57). We find that antioxidant compounds in the TSE reduce lipid and protein oxidation in chicken meat, delaying oxidative aging and enhancing color, taste, and storage stability. This suggests that TSE’s antioxidants, such as polyphenols and flavonoids, inhibit lipid oxidation and radical reactions, extending chicken freshness and improving water retention and texture. However, shear force varies for different meats, making it an insufficient criterion for meat quality.
Antioxidant indices T-AOC, SOD, GSH-PX, and MDA in serum have different meanings for animals. These indexes can reflect the antioxidant capacity and oxidative stress state in animals. A higher level of T-AOC, an indicator of overall system capacity, indicates a stronger antioxidant capacity in the animal, which helps fight against free radical damage (58). SOD is an important antioxidant enzyme, and its activity level can reflect the animal’s ability to scavenge superoxide anions (59). GSH-PX can protect cells from oxidative damage by reducing hydrogen peroxide, and its activity level reflects the animal’s ability to cope with oxidative stress (60). MDA is a representative of lipid peroxidation products in cells, produced by lipid peroxidation reactions caused by oxidative stress, and the level of MDA reflects the degree of oxidative damage in animals (61). We showed that the addition of antioxidants and TSE in the diet improved the antioxidant capacity and significantly reduced the oxidative damage of broilers. Additionally, the antioxidant effect of the test group with TSE added at 1200 g/t was similar to that of the test group with VC and VE. The specific mechanism by which TSE enhances anti-oxidant capacity in animals was not considered in this study and needs further examination. However, polyphenols and flavonoids in plant extracts have been shown to have antioxidant activity and may positively affect the antioxidant capacity of animals (62, 63). Polyphenolic compounds are excellent hydrogen or electron donors with stable free radical intermediates, inhibiting the free radical chain reactions, and thereby reducing the occurrence of oxidation reactions (64). Flavonoids can donate hydrogen to lipid free radicals and transform themselves into stable phenolic free radicals, reducing the transmission rate of auto-oxidative chain reactions (55, 65). Furthermore, polyphenols and flavonoids can enhance the antioxidant capacity of cells by promoting the expression of antioxidant genes through the regulation of intracellular signaling pathways, such as the Nrf2/ARE signaling pathway (66, 67).
A healthy intestinal environment ensures the well-being of the animal. The number and types of microorganisms in different intestinal tracts of broilers vary significantly. The dynamic balance of intestinal microorganisms in broilers ensures normal growth performance, nutrient utilization metabolism, physiological and biochemical roles, immune system, and antioxidant processes. Intestinal microbes can be classified into beneficial and harmful bacteria (68). Under normal circumstances, intestinal microorganisms coordinate with host animals to ensure the dynamic balance of intestinal microorganisms. However, sudden changes in the external environment and alterations in animal feed can affect the animal’s body to some extent, leading to the proliferation of harmful bacteria and an excessive bacterial population, ultimately affecting the normal growth performance of animals (69). Therefore, maintaining the balance of intestinal flora plays a crucial role in the host’s health (70), making it an essential prerequisite for ensuring the normal physiological well-being of animals. Research has shown that Chinese herbal medicine monomers or plant extracts, and compound preparations can significantly enhance the structure of intestinal flora, increase the abundance of beneficial intestinal bacteria, and reduce the number of harmful bacteria, thus preventing and treating diseases (71). Currently, the research on plant’s impact on poultry intestinal microbes mainly focuses on extracts. It was reported that dietary supplementation of astragalus polysaccharides and glycyrrhizin polysaccharides increased the richness and diversity of microflora in the cecum of broilers (72). Additionally, studies have found that adding eleuthero and achyranthes knuckle extracts to the diet can increase the number of lactic acid bacteria in the intestinal tract of poultry and reduce the number of Escherichia coli and Salmonella (73, 74). However, there are few reports on the application of TSE in livestock and poultry production. Studies have shown that the microbiota of mammals is passed down vertically to the next generation, while poultry is the egg-laying animals, the microbial composition of poultry seldom influences the microbial characteristics of their offspring, resulting in a significant decrease in the diversity and abundance of intestinal flora in poultry (75). Upon hatching, changes occur in the poultry’s intestinal flora due to various internal and external factors that mainly affect digestion and absorption processes taking place in the small intestine (76). The ileum, situated after the jejunum and connected to the cecum, constitutes the final section of this organ system. Therefore, this study sought to investigate the impact of TSE on the microflora present in broilers’ ileums. The result showed that the inclusion of TSE had no impact on the gut microbial diversity of broilers. By analyzing and comparing the intestinal microbial communities, it was observed that the addition of TSE did not alter the composition or disrupt the homeostasis of the intestinal microbiota. Combining the growth performance and nutrient digestion and utilization data of this experiment, we have observed a positive impact on broiler growth performance and nutrient utilization with the addition of TSE. However, no significant changes were noted in the ileal microflora, this is consistent with the results of Shang et al. (77). This suggests that while gut flora is one factor influencing individual productivity, it cannot be solely relied upon for improving growth performance. Other relevant factors such as feed composition, feeding environment, and feeding management need to be considered.
Conclusion
In vitro antioxidant results showed that TSE has better scavenging rates against DPPH and hydroxyl free radicals. During the stater phase, dietary supplementation 1,200 g/t TSE significantly decreased the AFI, F/G, and F/G was also decreased during the whole feeding phase. In addition, adding 1,200 g/t TSE to the diet increased the nutrient utilization, meat quality, and serum antioxidant function of broilers.
Data availability statement
The original contributions presented in the study are included in the article/supplementary material, further inquiries can be directed to the corresponding author.
Ethics statement
The animal studies were approved by Faculty Animal Policy and Welfare Committee of Gansu Agricultural University (No. GSAU-Eth-AST-2021-001). The studies were conducted in accordance with the local legislation and institutional requirements. Written informed consent was obtained from the owners for the participation of their animals in this study.
Author contributions
XZ: Conceptualization, Data curation, Formal analysis, Software, Visualization, Writing – original draft, Writing – review & editing, Investigation. BD: Data curation, Supervision, Writing – review & editing. MW: Data curation, Writing – review & editing. JL: Data curation, Writing – review & editing. SQ: Data curation, Writing – review & editing. FN: Data curation, Writing – review & editing. DT: Conceptualization, Data curation, Funding acquisition, Project administration, Resources, Validation, Writing – review & editing.
Funding
The author(s) declare financial support was received for the research, authorship, and/or publication of this article. This research was funded by the Gansu Province University Industry Support Plan under Grant (no. 2023CYZC-47) and Key R&D Plan of Gansu Province under Grant (no. 22YF7NA113) and Discipline Team Project of Gansu Agricultural University under Grant (no. GAU-XKTD-2022-24) and Fuxi Young Talent Cultivation project of Gansu Agricultural University under Grant (no. GAUFX-02Y07).
Acknowledgments
The authors acknowledge the students at the Animal Nutrition Laboratory, Gansu Agricultural University, China, for all their help and support. The authors also acknowledge all the reviewers who participated in the review, as well as MJ Editor (www.mjeditor.com) for providing English editing services during the preparation of this manuscript.
Conflict of interest
The authors declare that the research was conducted in the absence of any commercial or financial relationships that could be construed as a potential conflict of interest.
Publisher’s note
All claims expressed in this article are solely those of the authors and do not necessarily represent those of their affiliated organizations, or those of the publisher, the editors and the reviewers. Any product that may be evaluated in this article, or claim that may be made by its manufacturer, is not guaranteed or endorsed by the publisher.
References
1. Castanon, JI . History of the use of antibiotic as growth promoters in european poultry feeds. Poult Sci. (2007) 86:2466–71. doi: 10.3382/ps.2007-00249
2. Suresh, G, Das, RK, Kaur, BS, Rouissi, T, Avalos, RA, Chorfi, Y, et al. Alternatives to antibiotics in poultry feed: molecular perspectives. Crit Rev Microbiol. (2018) 44:318–35. doi: 10.1080/1040841X.2017.1373062
3. Van Boeckel, TP, Brower, C, Gilbert, M, Grenfell, BT, Levin, SA, Robinson, TP, et al. Global trends in antimicrobial use in food animals. Proc Natl Acad Sci U S A. (2015) 112:5649–54. doi: 10.1073/pnas.1503141112
4. Brussow, H . Adjuncts and alternatives in the time of antibiotic resistance and in-feed antibiotic bans. Microb Biotechnol. (2017) 10:674–7. doi: 10.1111/1751-7915.12730
5. Lillehoj, H, Liu, Y, Calsamiglia, S, Fernandez-Miyakawa, ME, Chi, F, Cravens, RL, et al. Phytochemicals as antibiotic alternatives to promote growth and enhance host health. Vet Res. (2018) 49:76. doi: 10.1186/s13567-018-0562-6
6. Liao, JW, Yeh, JY, Lin, YC, Wei, MM, and Chung, YC. Mutagenicity and safety evaluation of water extract of fermented toona sinensis roemor leaves. J Food Sci. (2009) 74:T7–T13. doi: 10.1111/j.1750-3841.2008.01007.x
7. Liao, JW, Chung, YC, Yeh, JY, Lin, YC, Lin, YG, Wu, SM, et al. Safety evaluation of water extracts of toona sinensis roemor leaf. Food Chem Toxicol. (2007) 45:1393–9. doi: 10.1016/j.fct.2007.01.020
8. Chen, YC, Chen, HJ, Huang, BM, Chen, YC, and Chang, CF. Polyphenol-rich extracts from toona sinensis bark and fruit ameliorate free fatty acid-induced lipogenesis through ampk and lc3 pathways. J Clin Med. (2019) 8:1664. doi: 10.3390/jcm8101664
9. Dong, X, Zhu, Y, Bao, G, Hu, F, and Qin, G. New limonoids and a dihydrobenzofuran norlignan from the roots of toona sinensis. Molecules. (2013) 18:2840–50. doi: 10.3390/molecules18032840
10. Feng, W, Wang, M, Cao, J, Sun, J, and Jiang, W. Regeneration of denatured polyphenol oxidase in toona sinensis (a.juss.) roam. Process Biochem. (2007) 42:1155–9. doi: 10.1016/j.procbio.2007.05.010
11. Hsieh, TJ, Wang, JC, Hu, CY, Li, CT, Kuo, CM, and Hsieh, SL. Effects of rutin from toona sinensis on the immune and physiological responses of white shrimp (litopenaeus vannamei) under vibrio alginolyticus challenge. Fish Shellfish Immunol. (2008) 25:581–8. doi: 10.1016/j.fsi.2008.07.014
12. Mazur-Kuśnirek, M, Antoszkiewicz, Z, Lipiński, K, Kaliniewicz, J, Kotlarczyk, S, and Żukowski, P. The effect of polyphenols and vitamin e on the antioxidant status and meat quality of broiler chickens exposed to high temperature. Arch Anim Nutr. (2019) 73:111–26. doi: 10.1080/1745039X.2019.1572342
13. Orzuna-Orzuna, JF, Dorantes-Iturbide, G, Lara-Bueno, A, Chay-Canul, AJ, Miranda-Romero, LA, and Mendoza-Martínez, GD. Meta-analysis of flavonoids use into beef and dairy cattle diet: performance, antioxidant status, ruminal fermentation, meat quality, and milk composition. Front Vet Sci. (2023) 10:1134925. doi: 10.3389/fvets.2023.1134925
14. Chen, Y, Chien, L, Huang, B, Chia, Y, and Chiu, H. Aqueous extracts of toona sinensis leaves inhibit renal carcinoma cell growth and migration through jak2/stat3, akt, mek/erk, and mtor/hif-2[alpha] pathways. Nutr Cancer. (2016) 68:654–66. doi: 10.1080/01635581.2016.1158292
15. Short, FJ, Gorton, P, Wiseman, J, and Boorman, KN, Nottingham Univ. L. L. U. K. Determination of titanium dioxide added as an inert marker in chicken digestibility studies. Anim Feed Sci Tech. (1996) 59:215–21. doi: 10.1016/0377-8401(95)00916-7
16. Wu, CC, Liu, CH, Chang, YP, and Hsieh, SL. Effects of hot-water extract of toona sinensis on immune response and resistance to aeromonas hydrophila in oreochromis mossambicus. Fish Shellfish Immunol. (2010) 29:258–63. doi: 10.1016/j.fsi.2010.04.021
17. Hu, Y, Zhang, J, Yu, C, Li, Q, Dong, F, Wang, G, et al. Synthesis, characterization, and antioxidant properties of novel inulin derivatives with amino-pyridine group. Int J Biol Macromol. (2014) 70:44–9. doi: 10.1016/j.ijbiomac.2014.06.024
18. Li, GM, Liu, LP, Yin, B, Liu, YY, Dong, WW, Gong, S, et al. Heat stress decreases egg production of laying hens by inducing apoptosis of follicular cells via activating the fasl/fas and tnf-alpha systems. Poult Sci. (2020) 99:6084–93. doi: 10.1016/j.psj.2020.07.024
19. Morgan, NK, Scholey, DV, and Burton, EJ. A comparison of two methods for determining titanium dioxide marker content in broiler digestibility studies. Animal (Cambridge, England). (2014) 8:529–33. doi: 10.1017/S1751731114000068
21. Jin, C, Wang, Q, Zhang, Z, Xu, Y, Yan, H, Li, H, et al. Dietary supplementation with pioglitazone hydrochloride and chromium methionine improves growth performance, meat quality, and antioxidant ability in finishing pigs. J Agr Food Chem. (2018) 66:4345–51. doi: 10.1021/acs.jafc.8b01176
22. Farouk, MM, Wieliczko, KJ, and Merts, I. Ultra-fast freezing and low storage temperatures are not necessary to maintain the functional properties of manufacturing beef. Meat Sci. (2004) 66:171–9. doi: 10.1016/S0309-1740(03)00081-0
23. Danta, CC, and Piplani, P. The discovery and development of new potential antioxidant agents for the treatment of neurodegenerative diseases. Expert Opin Drug Discov. (2014) 9:1205–22. doi: 10.1517/17460441.2014.942218
24. Galano, A, Mazzone, G, Alvarez-Diduk, R, Marino, T, Alvarez-Idaboy, JR, and Russo, N. Food antioxidants: chemical insights at the molecular level. Annu Rev Food Sci Technol. (2016) 7:335–52. doi: 10.1146/annurev-food-041715-033206
25. Parhiz, H, Roohbakhsh, A, Soltani, F, Rezaee, R, and Iranshahi, M. Antioxidant and anti-inflammatory properties of the citrus flavonoids hesperidin and hesperetin: an updated review of their molecular mechanisms and experimental models. Phytother Res. (2015) 29:323–31. doi: 10.1002/ptr.5256
26. Roleira, FM, Tavares-da-Silva, EJ, Varela, CL, Costa, SC, Silva, T, Garrido, J, et al. Plant derived and dietary phenolic antioxidants: anticancer properties. Food Chem. (2015) 183:235–58. doi: 10.1016/j.foodchem.2015.03.039
27. Liu, Y, Wu, Y, Zhang, R, Lam, J, Ng, JC, Xu, ZP, et al. Investigating the use of layered double hydroxide nanoparticles as carriers of metal oxides for theranostics of ros-related diseases. ACS Appl Bio Mater. (2019) 2:5930–40. doi: 10.1021/acsabm.9b00852
28. Maulana, TI, Falah, S, and Andrianto, D. Total phenolic content, total flavonoid content, and antioxidant activity of water and ethanol extract from surian (toona sinensis) leaves. IOP Conf Series Earth Environ Sci. (2019) 299:012021. doi: 10.1088/1755-1315/299/1/012021
29. Middleton, EJ, Kandaswami, C, and Theoharides, TC. The effects of plant flavonoids on mammalian cells: implications for inflammation, heart disease, and cancer. Pharmacol Rev. (2000) 52:673–751.
30. Soobrattee, MA, Neergheen, VS, Luximon-Ramma, A, Aruoma, OI, and Bahorun, T. Phenolics as potential antioxidant therapeutic agents: mechanism and actions. Mutat Res. (2005) 579:200–13. doi: 10.1016/j.mrfmmm.2005.03.023
31. Pisoschi, AM, and Pop, A. The role of antioxidants in the chemistry of oxidative stress: a review. Eur J Med Chem. (2015) 97:55–74. doi: 10.1016/j.ejmech.2015.04.040
32. Poljsak, B, Suput, D, and Milisav, I. Achieving the balance between ros and antioxidants: when to use the synthetic antioxidants. Oxidative Med Cell Longev. (2013) 2013:956792. doi: 10.1155/2013/956792
33. Goel, A, Ncho, CM, Gupta, V, and Choi, YH. Embryonic modulation through thermal manipulation and in ovo feeding to develop heat tolerance in chickens. Anim Nutr. (2023) 13:150–9. doi: 10.1016/j.aninu.2023.01.005
34. Scanes, CG . Biology of stress in poultry with emphasis on glucocorticoids and the heterophil to lymphocyte ratio. Poult Sci. (2016) 95:2208–15. doi: 10.3382/ps/pew137
35. Surai, PF, Kochish, II, Fisinin, VI, and Kidd, MT. Antioxidant defence systems and oxidative stress in poultry biology: an update. Antioxidants (Basel). (2019) 8:235. doi: 10.3390/antiox8070235
36. Li, W, Zhang, X, He, Z, Chen, Y, Li, Z, Meng, T, et al. In vitro and in vivo antioxidant activity of eucalyptus leaf polyphenols extract and its effect on chicken meat quality and cecum microbiota. Food Res Int. (2020) 136:109302. doi: 10.1016/j.foodres.2020.109302
37. Woelders, H, Zuidberg, CA, and Hiemstra, SJ. Animal genetic resources conservation in the Netherlands and europe: poultry perspective. Poult Sci. (2006) 85:216–22. doi: 10.1093/ps/85.2.216
38. Ouyang, K, Xu, M, Jiang, Y, Wang, W, and Plaizier, J. Effects of alfalfa flavonoids on broiler performance, meat quality, and gene expression. Can J Anim Sci. (2016) 96:332–41. doi: 10.1139/cjas-2015-0132
39. Xue, F, Wan, G, Xiao, Y, Chen, C, Qu, M, and Xu, L. Growth performances, gastrointestinal epithelium and bacteria responses of yellow-feathered chickens to kudzu-leaf flavonoids supplement. AMB Express. (2021) 11:125. doi: 10.1186/s13568-021-01288-4
40. You, T, Tang, J, Yin, S, Jia, G, Liu, G, Tian, G, et al. Effect of dietary licorice flavonoids powder on performance, intestinal immunity and health of weaned piglets. J Anim Physiol Anim Nutr (Berl). (2023) 107:147–56. doi: 10.1111/jpn.13694
41. Balakrishnan, G, and Schneider, RG. Quinoa flavonoids and their bioaccessibility during in vitro gastrointestinal digestion. J Cereal Sci. (2020) 95:103070. doi: 10.1016/j.jcs.2020.103070
42. Han, S, Luo, Y, Hu, Z, Qin, D, and Luo, F. Targeting gut microbiota in type 2 diabetes mellitus: potential roles of dietary flavonoids. Food Biosci. (2022) 45:101500. doi: 10.1016/j.fbio.2021.101500
43. Zhao, K, Yao, M, Zhang, X, Xu, F, Shao, X, Wei, Y, et al. Flavonoids and intestinal microbes interact to alleviate depression. J Sci Food Agric. (2022) 102:1311–8. doi: 10.1002/jsfa.11578
44. Sun, HF, Song, MF, Zhang, Y, and Zhang, ZL. Transcriptome profiling reveals candidate flavonoid-related genes during formation of dragon's blood from dracaena cochinchinensis (lour.) S.C.chen under conditions of wounding stress. J Ethnopharmacol. (2021) 273:113987. doi: 10.1016/j.jep.2021.113987
45. Wu, Y, Wang, T, Xin, Y, Wang, G, and Xu, LA. Overexpression of gbf3'5'h1 provides a potential to improve the content of epicatechin and gallocatechin. Molecules. (2020) 25:4836. doi: 10.3390/molecules25204836
46. Peng, W, Liu, Y, Hu, M, Zhang, M, Yang, J, Liang, F, et al. Toona sinensis: a comprehensive review on its traditional usages, phytochemisty, pharmacology and toxicology. Rev Bras. (2019) 29:111–24. doi: 10.1016/j.bjp.2018.07.009
47. Dong, Y, Ruan, J, Ding, Z, Zhao, W, Hao, M, Zhang, Y, et al. Phytochemistry and comprehensive chemical profiling study of flavonoids and phenolic acids in the aerial parts of allium mongolicum regel and their intestinal motility evaluation. Molecules. (2020) 25:577. doi: 10.3390/molecules25030577
48. Xie, T, Jin, F, Jia, X, Mao, H, Xu, Y, and Zhang, S. High cellulose diet promotes intestinal motility through regulating intestinal immune homeostasis and serotonin biosynthesis. Biol Chem. (2022) 403:279–92. doi: 10.1515/hsz-2021-0216
49. Frolinger, T, Sims, S, Smith, C, Wang, J, Cheng, H, Faith, J, et al. The gut microbiota composition affects dietary polyphenols-mediated cognitive resilience in mice by modulating the bioavailability of phenolic acids. Sci Rep-UK. (2019) 9:3546. doi: 10.1038/s41598-019-39994-6
50. Pei, R, Liu, X, and Bolling, B. Flavonoids and gut health. Curr Opin Biotechnol. (2020) 61:153–9. doi: 10.1016/j.copbio.2019.12.018
51. Russell, W, and Duthie, G. Plant secondary metabolites and gut health: the case for phenolic acids. Proc Nutr Soc. (2011) 70:389–96. doi: 10.1017/S0029665111000152
52. Saenjum, C, Pattananandecha, T, and Nakagawa, K. Detection of antioxidant phytochemicals isolated from camellia japonica seeds using hplc and epr imaging. Antioxidants. (2020) 9:493. doi: 10.3390/antiox9060493
53. Mir, NA, Rafiq, A, Kumar, F, Singh, V, and Shukla, V. Determinants of broiler chicken meat quality and factors affecting them: a review. J Food Sci Technol. (2017) 54:2997–3009. doi: 10.1007/s13197-017-2789-z
54. Li, Q, Mi, Y, Tan, W, and Guo, Z. Highly efficient free radical-scavenging property of phenolic-functionalized chitosan derivatives: chemical modification and activity assessment. Int J Biol Macromol. (2020) 164:4279–88. doi: 10.1016/j.ijbiomac.2020.08.250
55. Liao, X, Wen, Q, Zhang, L, Lu, L, Zhang, L, and Luo, X. Effect of dietary supplementation with flavonoid from scutellaria baicalensis georgi on growth performance, meat quality and antioxidative ability of broilers. J Integr Agr. (2018) 17:1165–70. doi: 10.1016/S2095-3119(17)61803-3
56. Song, Z, Wei, J, Cao, Y, Yu, Q, and Han, L. Development and characterization of tapioca starch/pectin composite films incorporated with broccoli leaf polyphenols and the improvement of quality during the chilled mutton storage. Food Chem. (2023) 418:135958. doi: 10.1016/j.foodchem.2023.135958
57. Vargas-Sánchez, RD, Torrescano-Urrutia, GR, Ibarra-Arias, FJ, Portillo-Loera, JJ, Ríos-Rincón, FG, and Sánchez-Escalante, A. Effect of dietary supplementation with pleurotus ostreatus on growth performance and meat quality of japanese quail. Livest Sci. (2018) 207:117–25. doi: 10.1016/j.livsci.2017.11.015
58. Wang, Y, Heng, C, Zhou, X, Cao, G, Jiang, L, Wang, J, et al. Supplemental bacillus subtilis dsm 29784 and enzymes, alone or in combination, as alternatives for antibiotics to improve growth performance, digestive enzyme activity, anti-oxidative status, immune response and the intestinal barrier of broiler chickens. Brit J Nutr. (2021) 125:494–507. doi: 10.1017/S0007114520002755
59. Gupta, N, Al-Saikhan, FI, Patel, B, Rashid, J, and Ahsan, F. Fasudil and sod packaged in peptide-studded-liposomes: properties, pharmacokinetics and ex-vivo targeting to isolated perfused rat lungs. Int J Pharm. (2015) 488:33–43. doi: 10.1016/j.ijpharm.2015.04.031
60. Upton, JR, Edens, FW, and Ferket, PR. The effects of dietary oxidized fat and selenium source on performance, glutathione peroxidase, and glutathione reductase activity in broiler chickens. J Appl Poult Res. (2009) 18:193–202. doi: 10.3382/japr.2008-00019
61. Lin, Q, Peng, S, Li, Y, Jiang, G, Liao, Z, Fan, Z, et al. Magnolol additive improves carcass and meat quality of linwu ducks by modulating antioxidative status. Anim Sci J. (2020) 91:e13301. doi: 10.1111/asj.13301
62. Kuppusamy, S, Thavamani, P, Megharaj, M, Nirola, R, Lee, YB, and Naidu, R. Assessment of antioxidant activity, minerals, phenols and flavonoid contents of common plant/tree waste extracts. Ind Crop Prod. (2016) 83:630–4. doi: 10.1016/j.indcrop.2015.12.060
63. Sowa, P, Marcinčáková, D, Miłek, M, Sidor, E, Legáth, J, and Dżugan, M. Analysis of cytotoxicity of selected asteraceae plant extracts in real time, their antioxidant properties and polyphenolic profile. Molecules (Basel, Switzerland). (2020) 25:5517. doi: 10.3390/molecules25235517
64. Ortega-Moo, C, Durán, R, Herrera, B, Gutiérrez-Oliva, S, Toro-Labbé, A, and Vargas, R. Study of antiradical mechanisms with dihydroxybenzenes using reaction force and reaction electronic flux. Phys Chem Chemical Phys. (2017) 19:14512–9. doi: 10.1039/c7cp01304c
65. Zheng, Y, Deng, G, and Zhang, Y. Multiple free radical scavenging reactions of flavonoids. Dyes Pigments. (2022) 198:109877. doi: 10.1016/j.dyepig.2021.109877
66. Suraweera, L, HPV, R, Dellaire, G, and Xu, Z. Regulation of nrf2/are pathway by dietary flavonoids: a friend or foe for cancer management? Antioxidants. (2020) 9:973. doi: 10.3390/antiox9100973
67. Zhao, F, Yan, S, and Tian, M. Blueberry polyphenol extracts enhance the intestinal antioxidant capacity in weaned rats by modulating the nrf2-keap1 signal pathway. Front Physiol. (2021) 12:640737. doi: 10.3389/fphys.2021.640737
68. Hooper, LV, and Gordon, JI. Commensal host-bacterial relationships in the gut. Science. (2001) 292:1115–8. doi: 10.1126/science.1058709
69. Sekelja, M, Rud, I, Knutsen, SH, Denstadli, V, Westereng, B, Naes, T, et al. Abrupt temporal fluctuations in the chicken fecal microbiota are explained by its gastrointestinal origin. Appl Environ Microb. (2012) 78:2941–8. doi: 10.1128/AEM.05391-11
70. Tremaroli, V, and Bäckhed, F. Functional interactions between the gut microbiota and host metabolism. Nature (London). (2012) 489:242–9. doi: 10.1038/nature11552
71. Zhou, SS, Xu, J, Zhu, H, Wu, J, Xu, JD, Yan, R, et al. Gut microbiota-involved mechanisms in enhancing systemic exposure of ginsenosides by coexisting polysaccharides in ginseng decoction. Sci Rep. (2016) 6:22474. doi: 10.1038/srep22474
72. Qiao, Y, Liu, C, Guo, Y, Zhang, W, Guo, W, Oleksandr, K, et al. Polysaccharides derived from astragalus membranaceus and glycyrrhiza uralensis improve growth performance of broilers by enhancing intestinal health and modulating gut microbiota. Poultry Sci. (2022) 101:101905. doi: 10.1016/j.psj.2022.101905
73. Ao, X, and Kim, IH. Effects of achyranthes bidentata polysaccharides on performance, immunity, antioxidant capacity, and meat quality in Pekin ducks. Poultry Sci. (2020) 99:4884–91. doi: 10.1016/j.psj.2020.06.026
74. Long, LN, Zhang, HH, Wang, F, Yin, YX, Yang, LY, and Chen, JS. Research note: effects of polysaccharide-enriched acanthopanax senticosus extract on growth performance, immune function, antioxidation, and ileal microbial populations in broiler chickens. Poultry Sci. (2021) 100:101028. doi: 10.1016/j.psj.2021.101028
75. Moeller, AH, Suzuki, TA, Phifer-Rixey, M, and Nachman, MW. Transmission modes of the mammalian gut microbiota. Science. (2018) 362:453–7. doi: 10.1126/science.aat7164
76. Ramakrishna, BS . Role of the gut microbiota in human nutrition and metabolism. J Gastroen Hepatol. (2013) 28:9–17. doi: 10.1111/jgh.12294
Keywords: broilers, Toona sinensis extract, growth performance, meat quality, serum antioxidant, intestinal microbes
Citation: Zhao X, Du B, Wan M, Li J, Qin S, Nian F and Tang D (2024) Analysis of the antioxidant activity of toons sinensis extract and their biological effects on broilers. Front. Vet. Sci. 10:1337291. doi: 10.3389/fvets.2023.1337291
Edited by:
Caven Mguvane Mnisi, North-West University, South AfricaReviewed by:
Felix Njeri, University of Guelph, CanadaAhmed N. F. Neamat-Allah, Zagazig University, Egypt
Chidozie Freedom Egbu, Alvan Ikoku Federal College of Education, Nigeria
Copyright © 2024 Zhao, Du, Wan, Li, Qin, Nian and Tang. This is an open-access article distributed under the terms of the Creative Commons Attribution License (CC BY). The use, distribution or reproduction in other forums is permitted, provided the original author(s) and the copyright owner(s) are credited and that the original publication in this journal is cited, in accordance with accepted academic practice. No use, distribution or reproduction is permitted which does not comply with these terms.
*Correspondence: Defu Tang, dGFuZ2RmQGdzYXUuZWR1LmNu