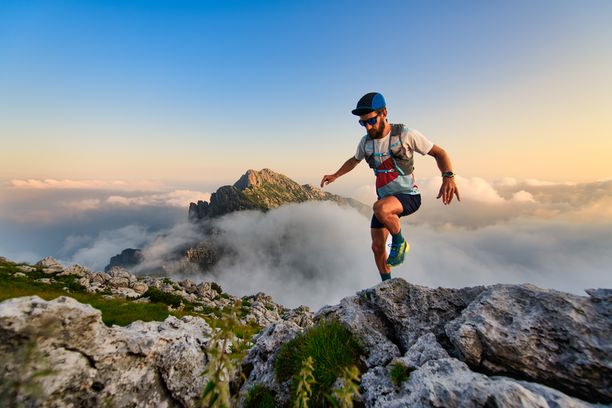
94% of researchers rate our articles as excellent or good
Learn more about the work of our research integrity team to safeguard the quality of each article we publish.
Find out more
ORIGINAL RESEARCH article
Front. Vet. Sci., 08 January 2024
Sec. Anesthesiology and Animal Pain Management
Volume 10 - 2023 | https://doi.org/10.3389/fvets.2023.1330111
This article is part of the Research TopicExploring Anesthetic Risk: Challenges and Solutions in Veterinary MedicineView all 8 articles
Cardiac electrical activity is often altered by administration of anesthetic drugs. While the effects of propofol in this regard have previously been described in dogs, to date, there are no reports of the effect of alfaxalone. This study investigated the impact of both propofol and alfaxalone on the ECG of 60 dogs, after premedication with acepromazine and methadone. Heart rate increased significantly in both groups. The PR and QRS intervals were significantly increased following propofol while with alfaxalone the QRS duration was significantly increased and ST segment depression was observed. The QT and JT interval were significantly shorter following induction with alfaxalone, but, when corrected (c) for heart rate, QTc and JTc in both groups were significantly greater following induction. When comparing the magnitude of change between groups, the change in RR interval was greater in the alfaxalone group. The change in both QT and JT intervals were significantly greater following alfaxalone, but when QTc and JTc intervals were compared, there were no significant differences between the two drugs. The similarly increased QTc produced by both drugs may suggest comparable proarrhythmic effects.
Continuous electrocardiographic (ECG) monitoring is essential in the anesthetized patient for diagnosis of peri-operative arrhythmias. These arrhythmias may be secondary to spontaneous or drug-induced changes in the cardiac electrical activity (1). While the incidence of perioperative arrhythmias in people is extremely high (2, 3), only limited comparable information is available in dogs, with one study reporting a prevalence of 2.5% during general anesthesia (4). In particular, changes in the QT interval are associated with potential arrhythmogenesis. The QT interval is measured from the onset of the QRS to the end of the T wave, and represents the time taken for ventricular depolarization (QRS) and repolarization (JT interval). The JT interval is the time between the J point, where the QRS complex joins the ST segment, and the end of the T wave. Typically, prolongation of the QT interval reflects an increase in the duration and heterogeneity of ventricular repolarization (5). This may lead to the development of potentially malignant arrhythmias through triggered activity, re-entry or both (6). Torsades de pointes are a well-reported consequence of QT prolongation in both dogs and humans (7, 8), and may lead to sudden death. QT prolongation may be congenital or drug-induced. Although congenital QT prolongation has been described in dogs (9), it is less frequently described compared to humans, where the incidence is ~1 in 2,000 (10). A large number of drugs potentially prolong the QT interval (11), and the impact of a new drug on ventricular repolarization still remains a critical step in the overall development process (12). Propofol and alfaxalone are the most popular intravenous anesthetics used in small animal anesthesia, and the former is the most widely used human anesthetic induction agent. Although relatively few studies have examined the effects of propofol on the ECG in dogs, it has been described as potentially arrhythmogenic in this species (13, 14) while, in humans, it seems to display both pro- and antiarrhythmic effects in a concentration-dependent manner (15). In both species, the QT interval is prolonged (14, 16) while no increase in QT dispersion has been found after propofol administration in dogs (14). Alfaxalone in conjunction with alfadolone has been previously approved for use in humans and cats. However, it was removed from the human market, and later the veterinary market due to a high incidence of anaphylactic reactions to its excipient, Cremophor EL (17). Alfaxalone was reformulated in 2-hydroxypropyl-b-cyclodextrin, a synthetic carbohydrate molecule not associated with allergic reactions, and is licensed for use in dogs and cats. The pharmacological and anesthetic properties of alfaxalone in dogs have been reviewed (18), but no studies have evaluated the effects of alfaxalone on the ECG parameters in dogs, and very few studies have assessed the effects on the ECG in humans (19, 20). A reformulated version of alfaxalone also using a cyclodextrin excipient, is currently undergoing human trials for re-introduction as an anesthetic agent in this species, so it would be interesting to assess the effects of alfaxalone on the canine ECG with particular reference to the QT interval as a guide to possible effects in humans. The aim of this study was to compare the effects of propofol and alfaxalone on ECG parameters in healthy dogs undergoing general anesthesia, with emphasis on the QT interval.
This study received ethical approval from the RCVS Ethics Review Panel (Ref: 2021-34), and an Animal Test Certificate was obtained from the Veterinary Medicines Directorate (ATC-S-164). Informed client consent was obtained for all animals recruited to the study.
Sixty healthy adult dogs (American Society of Anesthesiologists status I or II) were included. Aggressive animals, those sedated or anesthetized during the previous 24 h, those with a recent history of vomiting or regurgitation, or dogs with known structural or functional cardiac disease or pre-existing arrhythmias, were excluded. Brachycephalic dogs and dogs which were deemed to be significantly underweight (BCS <4/9) or overweight (BCS >6/9) were also excluded.
Each dog received a complete physical examination and were randomly assigned, using computer-generated random numbers, to group A for anesthetic induction with alfaxalone (10 mg/ml Alfaxan; Jurox, UK), or group P for induction with propofol (10 mg/ml Propoflo Plus; Zoetis, USA). Premedication consisted of 0.03 mg/kg acepromazine (2 mg/ml Acesedate; Jurox, UK) with 0.3 mg/kg methadone (10 mg/ml Comfortan; Dechra, UK) administered intramuscularly in the cervical epaxial musculature. Following this, the dog was moved to a quiet environment for 30 min prior to aseptic intravenous (IV) cannula placement in an appropriate limb. The dog was then gently restrained in right lateral recumbency and allowed a short period of acclimatization.
Non-traumatic electrodes were used to obtain a complete 12 lead ECG. Six limb leads (I, II, III, aVR, aVL and aVF) were used. The precordial lead system (V1, V2, V3, V4, V5, V6) was utilized, with lead V1 positioned at the costochondral junction of the right, first intercostal space (21). The six limb leads were recorded simultaneously with the six chest leads for 30 s at a paper speed of 50 mm/s and voltage of 20 mm/mV.
Following acquisition of the ECG, the dog was preoxygenated with 100% oxygen via face mask for at least 3 min and then induced with either alfaxalone or propofol depending on group assignation, administered IV by hand, to effect. The same anesthetist (VC) performed all inductions with an end-point of loss of consciousness, absent palpebral reflex and loss of jaw tone. A further ECG trace was then taken for 30 s at the same paper speed and gain, with continued administration of 100% oxygen via facemask throughout the recording. Once the ECG recording was completed, the trachea was intubated with an appropriately sized endotracheal tube and anesthesia continued for the planned procedure.
All the ECG measurements were made according to a standardized approach (22, 23). The QT interval was measured from the earliest QRS onset in any lead of the six simultaneously recorded leads to the intersection between the maximal downslope of the terminal portion of the T-wave to the isoelectric baseline (24, 25). All the measurements of PR interval, QRS interval, ST segment deviation from baseline, T-wave amplitude and QT interval were taken from lead II, and recorded in each case as the mean values from three consecutive complexes. The JT interval was calculated as the QT interval minus the QRS interval. Rate-corrected QT and JT intervals (QTc and JTc) were obtained by using the formula QTc = QT − 0.087 (RR – 1, 000) (26) and JTc = QTc − QRS. The RR interval used in this calculation was taken as the mean RR interval across the complexes recorded over 6–10 s (9).
QT dispersion (QTd) was calculated as the difference between the maximum and minimum QT interval observed across all the 12 leads of the ECG (27). All the measurements were made by the same observer (VC).
A power analysis was performed based on a recent study (28) comparing the effects of methadone or hydromorphone on cardiac conductivity, and which used a clinically significant difference of 20 ms and demonstrated a pooled variance of 25 ms on QT interval. Using this data, and based on a power of 80% and alpha of 0.05, 52 dogs were required for our study. Given the potential for some ECG traces to be unsuitable for final analysis (e.g., due to movement artifact), we elected to recruit 60 dogs in total, 30 in each group. Data were analyzed in Excel spreadsheet format (Microsoft Excel for Mac, version 16.16.27) using MedCalc (version 17.1 64-bit, Ostend, Belgium). All data were tested for normality using the Shapiro Wilk test and diagnostic plots. Age and bodyweight between groups were tested using ANOVA. Paired data for ECG variables before and after induction to general anesthesia were tested using Student's t-test. The magnitude of changes was calculated and compared between the two groups using independent t-tests. A p-value of <0.05 was considered statistically significant.
In total, 120 ECG traces were collected with 12 traces excluded due to the presence of artifacts, and 108 ECG were included for statistical analysis (27 dogs in each group). All data were normally distributed. No arrhythmias were detected in the propofol group. One dog in the alfaxalone group demonstrated isolated ventricular escape complexes after premedication, that were not present after induction.
Patient variables, breed distribution and dose of propofol and alfaxalone are described in Table 1. There were no statistically significant differences detected for age and body weight between groups.
Table 1. Animal variables in 54 dogs, premedicated using acepromazine (0.03 mg/kg) and methadone (0.3 mg/kg) intramuscularly and induced to general anesthesia with alfaxalone or propofol.
Measurements from the ECG analysis are reported in Table 2. Heart rate was similar between groups following premedication, but increased significantly in both groups following induction. An increase in the QRS duration was documented in both alfaxalone and propofol groups following induction, although this did not exceed the upper limits of the reported reference interval. A significant increase in PR interval duration was only documented in the propofol group post-induction. A significant ST segment deviation was only observed after alfaxalone administration.
Table 2. Electrocardiogram variables (mean ± SD) in 54 dogs, 30 min after premedication using acepromazine (0.03 mg/kg) and methadone (0.3 mg/kg) intramuscularly and after induction to general anesthesia with alfaxalone or propofol.
In the alfaxalone group, QT interval was significantly shorter following induction, but once corrected for heart rate (QTc), the interval in both groups was significantly greater following induction (Figure 1). Similarly, JT interval decreased in the alfaxalone group, in contrast to JTc which increased in both groups following induction. No significant change to QTd was seen in either group. In one dog in each group, one of the 12 leads was of insufficient quality to determine where the T wave ended, and this lead was excluded when calculating QTd; however, 11 leads were considered sufficient (14).
Figure 1. QT and QT corrected (c) interval (mean ± SD) before and after induction to general anesthesia with alfaxalone or propofol. All values are expressed as milliseconds (ms).
The T wave amplitude increased significantly in the alfaxalone group; however, due to the variation in polarity of the T wave from animal to animal, the T waves were grouped into positive and negative polarities and then re-tested, following which there were no significant differences in T wave amplitude either within or between the groups.
When comparing the magnitude of change between groups (Table 3), the change in RR interval was greater in the alfaxalone group. The change in both QT and JT intervals were significantly more in the alfaxalone group compared with the propofol group. However, when the corrected QT and JT intervals were compared, there were no significant differences.
Table 3. Electrocardiogram variables (mean ± SD) in 54 dogs, 30 min after premedication using acepromazine (0.03 mg/kg) and methadone (0.3 mg/kg) intramuscularly and after induction to general anesthesia with alfaxalone or propofol.
This study expands previous knowledge of the ECG effects of propofol in dogs, and is the first to investigate those of alfaxalone in this species. Given the potential for the latter to receive licensing authorization for use in humans, our results provide a preliminary basis for assessing the potential proarrhythmic effects of alfaxalone in this species.
In this study, a positive chronotropic effect was observed following administration of both propofol and alfaxalone, with higher heart rates observed with alfaxalone. In healthy dogs, clinical doses of alfaxalone have minimal cardiovascular effects: cardiac output (CO) is usually well maintained (29, 30), while arterial blood pressure (ABP) and systemic vascular resistance (SVR) may decrease (29–32), but usually remain in the physiological range. It has been hypothesized that, due to the preservation of the baroreceptor reflex, alfaxalone administration results in a greater increase of heart rate (HR) compared with propofol. This represents a compensatory mechanism to maintain CO and ABP following the decrease in SVR (30, 31). Drugs used for premedication can influence this response, and depending on the protocol used, HR in dogs was reported to increase (32, 33), decrease (34) or remain unchanged (32, 35, 36) following induction with alfaxalone. While propofol is generally reported in humans to cause a reduction in HR (37), other investigators have demonstrated positive chronotropism (38), albeit not as marked as with other drugs such as alfaxalone and thiopentone as shown in some studies in dogs (14, 29). This was observed in our study and is thought to be a result of the differing effects of the drugs on baroreceptor sensitivity, as propofol is reported to cause a complete resetting of the baroreceptor reflex (39). Preservation of the baroreceptor reflex may explain a marked sympathetic response to vasodilation when alfaxalone is administered compared to propofol.
The PR interval reflects the time taken for conduction through the atrioventricular (AV) node, and tends to increase with decreasing HR and shortens as HR increases (40). Prolongation of the PR interval, known as first-degree atrioventricular block (1AVB), is usually well tolerated, but may be exacerbated by drugs or diseases that alter autonomic tone (23). One dog in the propofol group and four dogs in the alfaxalone group demonstrated 1AVB pre-induction, but normalized in two dogs in the alfaxalone group after induction. One further dog in the alfaxalone group developed mild 1AVB post-induction. It is possible that this may have been caused by the drugs used for premedication. There are no published studies assessing the effects of acepromazine on conduction through the AV node. Full mu opioid agonists are known to result in vagally mediated bradycardia (41). In addition, the chemical structure of methadone resembles that of calcium channel blocking agents (42, 43), resulting in functional L-type calcium channel blockade. These characteristics of methadone create the potential for 1AVB, and this has been shown in dogs (28). Following induction with propofol, the PR interval was significantly prolonged compared to pre-induction values. As 5 dogs demonstrated 1AVB pre-induction, we decided to re-analyse the data with these animals excluded to remove any potential influence of premedication on the induction agent effect. However, induction with propofol still resulted in a significantly prolonged PR interval. This is in contrast to the findings of Dennis et al. (14) where the PR interval was unchanged following propofol administration and the reasons for these dissimilar results are unclear. Previous animal studies have examined the effects of propofol on sinus and AV nodal function with conflicting results (44–46). The majority of studies in human medicine did not find any direct effects of propofol on the activity of the AV conduction (47, 48). In contrast, some electrophysiology studies in children, scheduled for radiofrequency ablation, confirm a statistically significant prolongation of the AV node conduction caused by propofol (49, 50). Further studies may be warranted to investigate this effect in dogs.
The QRS complex reflects depolarization of the ventricular myocardium, and in dogs, a wide QRS is defined as >70 ms (22, 23). An increased QRS complex duration pre-induction was observed for four dogs that was still present post-induction, with one dog having a QRS duration of 70.33 ms following alfaxalone administration. Although there was a statistically significant increase in QRS duration in both alfaxalone and propofol groups, all remaining dogs demonstrated values pre- and post-induction that were within the normal range. A number of pathological conditions may cause QRS prolongation, and although we cannot completely exclude the presence of mild subclinical cardiac disease, it is unlikely in this population of dogs. Methadone and acepromazine do not influence QRS complex duration in studies conducted in dogs (51, 52). Therefore, it is unlikely that the drugs used for premedication in our study were responsible for the QRS prolongation observed before induction. Again, data were reanalyzed when these dogs were removed to account for any influence from premedication and this did not change our results. Evidence of a possible interaction between alfaxalone and cardiac ion channels and electrical conductivity is lacking. Although the effects of propofol on inward/outward ion currents have been described (15, 53, 54), no change in QRS duration was observed in a human study that evaluated the effects of propofol on electrical activity in the heart (55). Furthermore, propofol did not affect the QRS interval in healthy dogs premedicated with acepromazine and pethidine (14), which is in contrast with the findings from our study. The reasons for these differences are not immediately apparent but it is important to reiterate that the QRS prolongation found in our study remained within the normal range reported for dogs.
This study showed a significant decrease of QT and JT intervals in dogs induced with alfaxalone. The duration of the QT and JT interval varies with HR: the faster the heart rate, the shorter the interval (56). Therefore, the more marked chronotropic effect of alfaxalone is the reason why these intervals decreased significantly in this group. Due to the effect of HR on the QT and JT intervals, it is more appropriate to calculate corrected intervals. This is a correction based on the R–R interval or average HR, depending on the formula used. Many different formulae have been developed (6), but there is no accepted gold standard in dogs. In this study, Van de Water's linear formula was used (26) as it has been shown to be the most appropriate formula in dogs (57), provides the most consistent correction across a wide range of HR (58), and is simple in comparison with others. Our study demonstrated a significant increase in the QTc interval in both propofol and alfaxalone groups following induction of anesthesia, with no significant difference between the drugs. However, with a reported reference interval between 150 to 240 ms in dogs (23), ~60% of the dogs (33 out of 54) showed an increased QTc interval following premedication but before induction. This finding might suggest either a pre-existing abnormality in these animals, or an effect of the agents used for premedication. Given the high incidence of this phenomenon, it is very unlikely to be related to underlying pathology, and more probably drug-induced. Methadone has previously been shown to increase the QT interval in humans (59), and, more recently, in dogs (28). Methadone blocks cardiac potassium channels and subsequently interrupts the delayed rectifier potassium current, which prolongs the action potential and delays ventricular repolarization, manifesting as a prolonged QT interval on the ECG (60). It is also possible that acepromazine may have played a role. An experimental study in humans reported that acepromazine may have a potentially arrhythmogenic action through the inhibition of human ether-à-go-go-related gene (hERG) potassium channels in cardiac tissues and the prolongation of QT intervals (61). We elected to use methadone as premedication in the dogs in this study as it is the only full opioid agonist currently licensed in this species in the U.K. and Europe, and we wanted to produce data relevant to clinical veterinary practice. The observed prolongation of the QTc interval in both groups can be traced back to the extension of its constituent components: the QRS and JTc intervals, representative of ventricular depolarization and repolarization, respectively. Induction to general anesthesia led to the lengthening of both intervals across both groups. A proposed cellular mechanism for this QT prolongation implicates the inhibition of delayed rectifier potassium currents, causing a diminished potassium efflux from cardiomyocytes and subsequently increasing the ventricular action potential (AP) duration (62). A causative role in QTc prolongation with propofol administration has been demonstrated in vivo and in vitro human studies (63, 64). Propofol has been reported to affect the activity of several ion channels. Some of these interactions are thought to contribute to its anti-arrhythmic and cardio-protective properties, particularly evident in mitigating damage during myocardial ischemia (65–67). Experimental investigations on human (68), rat (69), dog (70) and guinea pig (71–73) cardiomyocytes have demonstrated that propofol reduces calcium Ca2+ influx by inhibiting L-type voltage-dependent Ca2+ channels. However, this reduction results in the shortening of the phase 2 of the cardiac AP, leading to a decrease in the AP duration (71–73). Inhibition of the slowly and rapidly activating delayed rectifier K+ current (IKr, IKs) has also been confirmed in both animal and human experimental studies (73–75). This phenomenon results in the prolongation of the phase 2 and 3 of the cardiac AP, thereby increasing the AP duration. Notably, genetic mutations affecting these ion channels represent the most common cause of Long QT Syndrome (LQTS) in humans (73, 76). Moreover, propofol demonstrates an inhibitory effect on the cardiac Na+ channel Nav1.5 (54), a membrane protein responsible for the INa current triggering cardiac cell depolarization. Mutations in this channel have been identified as causal factors for a variant of LQTS in humans (76). This intricate network of interactions sheds light on the multifaceted impact of propofol on cardiac electrophysiology. Despite the vast body of literature on interaction between propofol and ion channels, to the authors' knowledge, there are no electrophysiology studies in either the human or the veterinary literature describing relationships between alfaxalone and ion channels, or any other mechanisms that could interfere with the ventricular action potentials. Similarly, there are limited clinical data regarding the ECG effects of alfaxalone in human medicine (77). While our study reports for the first time a prolongation of the QTc in dogs following alfaxalone, the precise mechanism for this effect remains unclear, and further studies are also required to establish if a similar situation occurs in humans.
Alfaxalone administration caused a statistically significant ST segment depression; however, this resulted in a value out with the reference (±0.2 mV) in only one dog (−0.27 mV). In veterinary medicine this parameter has been given very little attention because of the low incidence of myocardial infarction and coronary artery disease in domestic species (78). However, during anesthetic procedures, changes in the ST segment can have important clinical implications, possibly indicating impaired myocardial oxygenation (79). Alfaxalone (in combination with alphadolone) has been reported to cause ST segment depression in humans (19), but the underlying mechanism has not been elucidated. It is possible that the significant increase in HR observed with alfaxalone might increase myocardial oxygen consumption, causing ST segment depression, although the clinical relevance is unknown.
The T waves analyzed in this study follow the qualitative and quantitative features of the normal canine population T waves (80). The T wave is one the most variable waves in the electrocardiogram and may change under several pathological conditions (81) or in association with altered autonomic tone, with variable and opposing effects reported (82–84). A reduction in T-wave amplitude has been observed after thiopentone administration in humans (85) and dogs (14). Initial data analysis from this current study showed a significant increase in the T wave amplitude in the alfaxalone group. However, due to the variation in polarity of the T wave from animal to animal, the T waves were subsequently grouped into positive and negative polarities and then re-analyzed, which revealed no significant differences in T wave amplitude either within or between the groups.
Different leads of the standard 12-lead ECG project the repolarization signals of different regions of the myocardial tissue (86). The QTd has been proposed as a measure of the range of this interlead variability (87). Indeed, it is hypothesized that the QTd reflects regional differences of ventricular repolarization (88). Intrinsic differences in the action potential duration of endocardial, mid-myocardial (M cells) and epicardial cells contribute to a physiological phenomenon known as ventricular transmural dispersion of repolarization (TDR). This heterogeneity of action potentials across different layers of the ventricular myocardium is due to a variable expression of ion channels and explains the reason why the repolarization process occurs unevenly in the heart (89). Even in the absence of abnormal anatomical substrates, cardiomyocytes exist in different states of excitability and refractoriness across the ventricular wall, generating different excitable myocardial areas, resulting in an increased TDR. This translates to an exaggerated heterogeneity and predisposes to arrhythmias because of trigger activity (via early afterdepolarization) and functional re-entry (90). Re-entrant circuits allow the generation of abnormal impulse conduction and are believed to be the main mechanisms of ventricular tachyarrhythmias and sudden cardiac death (91, 92). No significant difference in QTd was found either within groups (pre- to post-induction), or between groups, suggesting that both propofol and alfaxalone might have minimal impact on the inhomogeneity of ventricular repolarization and refractoriness. Lack of impact of propofol on QTd has previously been reported in dogs (14), although the values documented in our study are slightly higher compared to those reported by Dennis et al. (14) (17.21 ms pre-induction, 16.99 ms post-induction). However, is difficult to fully interpret these differences as there is no reported reference interval currently available for QTd in dogs.
Our study has some potential limitations. Ideally, when evaluating the effects of an anesthetic induction agent on the ECG, no premedication should be administered, so it is solely the impact of the hypnotic agent, itself, being assessed. Drugs used for premedication probably exerted some influence on the ECG parameters, which might have affected the results obtained. However, the absence of premedication would have resulted in an increased dose requirement for the induction agent, with consequent greater cardiorespiratory depression and is unacceptable in client-owned animals. Given that the premedication protocol was identical for all the dogs, any changes observed between the ECG values obtained pre-induction (post-premedication) and post-induction, should, theoretically, be in response to the hypnotic agent itself, therefore minimizing bias between the groups. Measurement bias is an important limitation of this study as the method used can strongly influence the QT interval (93). Identifying the intersection between the descending branch of the T wave and the isoelectric line can be challenging for trained eyes and also computer algorithms (22, 94). Despite the fact that all measurements were performed by the same operator after a period of training under direct supervision of an EBVS Specialist in Veterinary Cardiology, we cannot completely rule out a degree of intra-individual variability in measurement technique. We also cannot completely exclude the presence of pre-existing structural/functional heart disease or transient arrhythmias in the dogs included in the study, as their absence was based solely on clinical examination and no Holter monitoring or echocardiographic examination was performed. Lastly, the lack of significant difference in QTd in both groups may simply reflect the inability of this parameter to correlate to the electrical and spatial inhomogeneity of the myocardium. More studies are needed to better characterize this parameter in veterinary medicine.
In conclusion, this study demonstrates, for the first time, that alfaxalone, similar to propofol, causes QTc prolongation in dogs, and may, therefore, exhibit arrhythmogenic properties. No significant difference in QTd could be demonstrated with either drug, suggesting that both propofol and alfaxalone might have minimal impact on the inhomogeneity of ventricular repolarization, although more research in this area is required.
The raw data supporting the conclusions of this article will be made available by the authors, without undue reservation.
The animal studies were approved by RCVS Ethics Review Panel (Ref: 2021-34). The studies were conducted in accordance with the local legislation and institutional requirements. Written informed consent was obtained from the owners for the participation of their animals in this study.
VC: Conceptualization, Data curation, Formal analysis, Methodology, Project administration, Visualization, Writing—original draft, Resources, Supervision. VG: Conceptualization, Writing—review & editing, Methodology. AA: Conceptualization, Formal analysis, Funding acquisition, Methodology, Writing—review & editing. SM: Data curation, Resources, Writing—review & editing. DF: Conceptualization, Methodology, Writing—review & editing.
The author(s) declare financial support was received for the research, authorship, and/or publication of this article. The publication of this research has been funded by IVC Evidensia UK.
We want to thank the staff of Southern Counties Veterinary Specialists for their help provided during the data collection phase of the study.
The authors declare that the research was conducted in the absence of any commercial or financial relationships that could be construed as a potential conflict of interest.
All claims expressed in this article are solely those of the authors and do not necessarily represent those of their affiliated organizations, or those of the publisher, the editors and the reviewers. Any product that may be evaluated in this article, or claim that may be made by its manufacturer, is not guaranteed or endorsed by the publisher.
1. Kwon CH, Kim SH. Intraoperative management of critical arrhythmia. Korean J Anesthesiol. (2017) 70:120–6. doi: 10.4097/kjae.2017.70.2.120
2. Forrest JB, Cahalan MK, Rehder K, Goldsmith CH, Levy WJ, Strunin L, et al. Multicenter study of general anesthesia. II. Results. Anesthesiology. (1990) 72:262–8. doi: 10.1097/00000542-199002000-00009
3. Forrest JB, Rehder K, Cahalan MK, Goldsmith CH. Multicenter study of general anesthesia. III. Predictors of severe perioperative adverse outcomes. Anesthesiology. (1992) 76:3–15. doi: 10.1097/00000542-199201000-00002
4. Gaynor JS, Dunlop CI, Wagner AE, Wertz EM, Golden AE, Demme WC. Complications and mortality associated with anesthesia in dogs and cats. J Am Anim Hosp Assoc. (1999) 35:13–7. doi: 10.5326/15473317-35-1-13
5. Lux RL, Fuller MS, MacLeod RS, Ershler PR, Green LS, Taccardi B. QT interval dispersion: dispersion of ventricular repolarization or dispersion of QT interval? J Electrocardiol. (1998) 30:176–80. doi: 10.1016/S0022-0736(98)80071-X
6. Hanton G, Nahas K, Priou C, Rabemampianina Y, Baneux P. The QT interval in the dog ECG: importance in preclinical toxicology and relationship to heart rate. Toxicol Mech Methods. (2001) 11:21–40. doi: 10.1080/105172301300055133
7. Bardy GH, Ungerleider RM, Smith WM, Ideker RE. A mechanism of torsades de pointes in a canine model. Circulation. (1983) 67:52–9. doi: 10.1161/01.CIR.67.1.52
8. Del Rosario ME, Weachter R, Flaker GC. Drug-induced QT prolongation and sudden death. Mo Med. (2010) 107:53−8.
9. Ware WA, Reina-Doreste Y, Stern JA, Meurs KM. Sudden death associated with QT interval prolongation and KCNQ1 gene mutation in a family of English Springer Spaniels. J Vet Intern Med. (2015) 29:561–8. doi: 10.1111/jvim.12550
10. Krahn AD, Laksman Z, Sy RW, Postema PG, Ackerman MJ, Wilde AAM, et al. Congenital long QT syndrome. JACC Clin Electrophysiol. (2022) 8:687–706. doi: 10.1016/j.jacep.2022.02.017
11. Khatib R, Sabir FRN, Omari C, Pepper C, Tayebjee MH. Managing drug-induced QT prolongation in clinical practice. Postgrad Med J. (2021) 97:452–8. doi: 10.1136/postgradmedj-2020-138661
12. Guth BD, Bass AS, Briscoe R, Chivers S, Markert M, Siegl PK, et al. Comparison of electrocardiographic analysis for risk of QT interval prolongation using safety pharmacology and toxicological studies. J Pharmacol Toxicol Methods. (2009) 60:107–16. doi: 10.1016/j.vascn.2009.05.006
13. Scruggs SM, Mama K, Bright JM, Zirofsky D. Accelerated idioventricular rhythm following propofol induction in a dog undergoing ocular surgery. Vet Anaesth Analg. (2010) 37:385–6. doi: 10.1111/j.1467-2995.2010.00539.x
14. Dennis SG, Wotton PR, Boswood A, Flaherty D. Comparison of the effects of thiopentone and propofol on the electrocardiogram of dogs. Vet Rec. (2007) 160:681–6. doi: 10.1136/vr.160.20.681
15. Liu Q, Kong AL, Chen R, Qian C, Liu SW, Sun BG, et al. Propofol and arrhythmias: two sides of the coin. Acta Pharmacol Sin. (2011) 32:817–23. doi: 10.1038/aps.2011.42
16. Saarnivaara L, Hiller A, Oikkonen M. QT interval, heart rate and arterial pressures using propofol, thiopentone or methohexitone for induction of anaesthesia in children. Acta Anaesthesiol Scand. (1993) 37:419–23. doi: 10.1111/j.1399-6576.1993.tb03740.x
17. Moneret-Vautrin DA, Laxenaire MC, Viry-Babel F. Anaphylaxis caused by anti-cremophor EL IgG STS antibodies in a case of reaction to althesin. Br J Anaesth. (1983) 55:469–71. doi: 10.1093/bja/55.5.469
18. Martín Bellido V, Vettorato E. Clinical review of the pharmacological and anaesthetic effects of alfaxalone in dogs. J Small Anim Pract. (2022) 63:341–61. doi: 10.1111/jsap.13454
19. Saarnivaara L. Comparison of thiopentone, althesin and ketamine in anaesthesia for otolaryngological surgery in children. Br J Anaesth. (1977) 49:363–70. doi: 10.1093/bja/49.4.363
20. Saarnivaara L, Kentala E. Comparison of electrocardiographic changes during microlaryngoscopy under balanced anaesthesia induced by althesin or thiopentone. Acta Anaesthesiol Scand. (1980) 24:321–4. doi: 10.1111/j.1399-6576.1980.tb01556.x
21. Santilli RA, Porteiro Vázquez DM, Gerou-Ferriani M, Lombardo SF, Perego M. Development and assessment of a novel precordial lead system for accurate detection of right atrial and ventricular depolarization in dogs with various thoracic conformations. Am J Vet Res. (2019) 80:358–68. doi: 10.2460/ajvr.80.4.358
22. Santilli RA, Moïse NS, Pariaut R, Perego M. Electrocardiography of the Dog and Cat: Diagnosis of Arrhythmia, 2nd ed. New York, NY: Edra (2018). p. 39–58.
23. Willis R, Oliveira P, Mavropoulou A. Guide to Canine and Feline Electrocardiography, 1st ed. Hoboken, NJ John Wiley & Sons Ltd (2018). p. 42–52. doi: 10.1002/9781119254355
24. Charbit B, Samain E, Merckx P, Funck-Brentano C. QT interval measurement: evaluation of automatic QTc measurement and new simple method to calculate and interpret corrected QT interval. Anesthesiology. (2006) 104:255–60. doi: 10.1097/00000542-200602000-00009
25. Schmitt MW, Von Landenberg F, Poth H, Wimmer E, Goddemeier T, Cavero I. Simple-to-use, reference criteria for revealing drug-induced QT interval prolongation in conscious dogs. Eur J Pharmacol. (2007) 554:46–52. doi: 10.1016/j.ejphar.2006.09.056
26. Van de Water A, Verheyen J, Xhonneux R, Reneman RS. An improved method to correct the QT interval of the electrocardiogram for changes in heart rate. J Pharmacol Methods. (1989) 22:207–17. doi: 10.1016/0160-5402(89)90015-6
27. Malik M, Batchvarov VN. Measurement, interpretation and clinical potential of QT dispersion. J Am Coll Cardiol. (2000) 36:1749–66. doi: 10.1016/S0735-1097(00)00962-1
28. Keating S, Fries R, Kling K, Graham L, Clark-Price S, Schaeffer DJ. Effect of methadone or hydromorphone on cardiac conductivity in dogs before and during sevoflurane anesthesia. Front Vet Sci. (2020) 7:573706. doi: 10.3389/fvets.2020.573706
29. Muir W, Lerche P, Wiese A, Nelson L, Pasloske K, Whittem T. Cardiorespiratory and anesthetic effects of clinical and supraclinical doses of alfaxalone in dogs. Vet Anaesth Analg. (2008) 35:451–62. doi: 10.1111/j.1467-2995.2008.00406.x
30. Seo JI, Han SH, Choi R, Han J, Lee L, Hyun C. Cardiopulmonary and anesthetic effects of the combination of butorphanol, midazolam and alfaxalone in Beagle dogs. Vet Anaesth Analg. (2015) 42:304–8. doi: 10.1111/vaa.12223
31. Rodríguez JM, Muñoz-Rascón P, Navarrete-Calvo R, Gómez-Villamandos RJ, Domínguez Pérez JM, Fernández Sarmiento JA, et al. Comparison of the cardiopulmonary parameters after induction of anaesthesia with alphaxalone or etomidate in dogs. Vet Anaesth Analg. (2012) 39:357–65. doi: 10.1111/j.1467-2995.2011.00695.x
32. Okushima S, Vettorato E, Corletto F. Chronotropic effect of propofol or alfaxalone following fentanyl administration in healthy dogs. Vet Anaesth Analg. (2015) 42:88–92. doi: 10.1111/vaa.12166
33. Zapata A, Laredo FG, Escobar M, Agut A, Soler M, Belda E. Effects of midazolam before or after alfaxalone for co-induction of anaesthesia in healthy dogs. Vet Anaesth Analg. (2018) 45:609–17. doi: 10.1016/j.vaa.2018.04.002
34. Muñoz KA, Robertson SA, Wilson DV. Alfaxalone alone or combined with midazolam or ketamine in dogs: intubation dose and select physiologic effects. Vet Anaesth Analg. (2017) 44:766–74. doi: 10.1016/j.vaa.2017.01.004
35. Suarez MA, Dzikiti BT, Stegmann FG, Hartman M. Comparison of alfaxalone and propofol administered as total intravenous anaesthesia for ovariohysterectomy in dogs. Vet Anaesth Analg. (2012) 39:236–44. doi: 10.1111/j.1467-2995.2011.00700.x
36. Miller C, Hughes E, Gurney M. Co-induction of anaesthesia with alfaxalone and midazolam in dogs: a randomized, blinded clinical trial. Vet Anaesth Analg. (2019) 46:613–9. doi: 10.1016/j.vaa.2019.03.009
37. Tramèr MR, Moore RA, McQuay HJ. Propofol and bradycardia: causation, frequency and severity. Br J Anaesth. (1997) 78:642–51. doi: 10.1093/bja/78.6.642
38. Fairfield JE, Dritsas A, Beale RJ. Haemodynamic effects of propofol: induction with 25 mg kg-1. Br J Anaesth. (1991) 67:618–20. doi: 10.1093/bja/67.5.618
39. Cullen PM, Turtle M, Prys-Roberts C, Way WL, Dye J. Effect of propofol anesthesia on baroreflex activity in humans. Anesth Analg. (1987) 66:1115–20. doi: 10.1213/00000539-198711000-00008
40. Hanton G, Rabemampianina Y. The electrocardiogram of the Beagle dog: reference values and effect of sex, genetic strain, body position and heart rate. Lab Anim. (2006) 40:123–36. doi: 10.1258/002367706776319088
41. Stanley TH, Liu WS, Webster LR, Johansen RK. Haemodynamic effects of intravenous methadone anaesthesia in dogs. Can Anaesth Soc J. (1980) 27:52–7. doi: 10.1007/BF03006849
42. Lichtenwalner MR, Mencken T, Tully R, Petosa M. False-positive immunochemical screen for methadone attributable to metabolites of verapamil. Clin Chem. (1998) 44:1039–41. doi: 10.1093/clinchem/44.5.1039
43. Seyler DE, Borowitz JL, Maickel RP. Calcium channel blockade by certain opioids. Fundam Appl Toxicol. (1983) 3:536–42. doi: 10.1093/toxsci/3.6.536
44. Napolitano CA, Raatikainen MJ, Martens JR, Dennis DM. Effects of intravenous anesthetics on atrial wavelength and atrioventricular nodal conduction in guinea pig heart. Potential antidysrhythmic properties and clinical implications. Anesthesiology. (1996) 85:393–402. doi: 10.1097/00000542-199608000-00022
45. Wu MH, Su MJ, Sun SS. Comparative direct electrophysiological effects of propofol on the conduction system and ionic channels of rabbit hearts. Br J Pharmacol. (1997) 121:617–24. doi: 10.1038/sj.bjp.0701155
46. Ikeno S, Akazawa S, Shimizu R, Nakaigawa Y, Ishii R, Inoue S, et al. Propofol does not affect the canine cardiac conduction system under autonomic blockade. Can J Anaesth. (1999) 46:148–53. doi: 10.1007/BF03012549
47. Sharpe MD, Dobkowski WB, Murkin JM, Klein G, Yee R. Propofol has no direct effect on sinoatrial node function or on normal atrioventricular and accessory pathway conduction in Wolff-Parkinson-White syndrome during alfentanil/midazolam anesthesia. Anesthesiology. (1995) 82:888–95. doi: 10.1097/00000542-199504000-00011
48. Warpechowski P, Lima GG, Medeiros CM, Santos AT, Kruse M, Migloransa MH, et al. Randomized study of propofol effect on electrophysiological properties of the atrioventricular node in patients with nodal reentrant tachycardia. Pacing Clin Electrophysiol. (2006) 29:1375–82. doi: 10.1111/j.1540-8159.2006.00550.x
49. Lai LP, Lin JL, Wu MH, Wang MJ, Huang CH, Yeh HM, et al. Usefulness of intravenous propofol anesthesia for radiofrequency catheter ablation in patients with tachyarrhythmias: infeasibility for pediatric patients with ectopic atrial tachycardia. Pacing Clin Electrophysiol. (1999) 22:1358–64. doi: 10.1111/j.1540-8159.1999.tb00629.x
50. Erb TO, Kanter RJ, Hall JM, Gan TJ, Kern FH, Schulman SR. Comparison of electrophysiologic effects of propofol and isoflurane-based anesthetics in children undergoing radiofrequency catheter ablation for supraventricular tachycardia. Anesthesiology. (2002) 96:1386–94. doi: 10.1097/00000542-200206000-00018
51. Kushner LI, Calvert CA, Boyle CR. Effects of acepromazine and buprenorphine on measured indices of the signal-averaged electrocardiogram in healthy dogs. Am J Vet Res. (1996) 57:1511–4.
52. Menegheti TM, Wagatsuma JT, Pacheco AD, Perez B, Pacheco CM, Abimussi CJ, et al. Electrocardiographic evaluation and degree of sedation with three doses of methadone in healthy dogs [corrected]. Vet Anaesth Analg. (2014) 41:97–104. doi: 10.1111/vaa.12086
53. Saint DA. The effects of propofol on macroscopic and single channel sodium currents in rat ventricular myocytes. Br J Pharmacol. (1998) 124:655–62. doi: 10.1038/sj.bjp.0701876
54. Stoetzer C, Reuter S, Doll T, Foadi N, Wegner F, Leffler A. Inhibition of the cardiac Na+ channel α-subunit Nav15 by propofol and dexmedetomidine Naunyn Schmiedebergs. Arch Pharmacol. (2016) 389:315–25. doi: 10.1007/s00210-015-1195-1
55. Wutzler A, De Asmundis C, Matsuda H, Bannehr M, Loehr L, Voelk K, et al. Effects of propofol on ventricular repolarization and incidence of malignant arrhythmias in adults. J Electrocardiol. (2018) 51:170–4. doi: 10.1016/j.jelectrocard.2017.11.003
56. Meyners M, Markert M. Correcting the QT interval for changes in HR in pre-clinical drug development. Methods Inf Med. (2004) 43:445–50. doi: 10.1055/s-0038-1633895
57. Oliveira MS, Muzzi RA, Muzzi LA, Cherem M, Mantovani MM. QT interval in healthy dogs: which method of correcting the QT interval in dogs is appropriate for use in small animal clinics? Pesq Vet Bras. (2014) 34:469–72. doi: 10.1590/S0100-736X2014000500014
58. Patel S, Bhatt L, Patel R, Shah C, Patel V, Patel J, et al. Identification of appropriate QTc formula in beagle dogs for nonclinical safety assessment. Regul Toxicol Pharmacol. (2017) 89:118–24. doi: 10.1016/j.yrtph.2017.07.026
59. Pearson EC, Woosley RL. QT prolongation and torsades de pointes among methadone users: reports to the FDA spontaneous reporting system. Pharmacoepidemiol Drug Saf. (2005) 14:747–53. doi: 10.1002/pds.1112
60. Klein MG, Krantz MJ, Fatima N, Watters A, Colon-Sanchez D, Geiger RM, et al. Methadone blockade of cardiac inward rectifier K+ current augments membrane instability and amplifies U waves on surface ECGs: a translational study. J Am Heart Assoc. (2022) 11:e023482. doi: 10.1161/JAHA.121.023482
61. Joo YS, Lee HJ, Choi JS, Sung KW. Acepromazine inhibits hERG potassium ion channels expressed in human embryonic kidney 293 cells. Korean J Physiol Pharmacol. (2017) 21:75–82. doi: 10.4196/kjpp.2017.21.1.75
62. Al-Akchar M, Siddique MS. Long QT Syndrome. Treasure Island, FL: StatPearls Publishing (2022). Available online at: https://www.ncbi.nlm.nih.gov/books/NBK441860/ (accessed May 15, 2022).
63. Douglas RJ, Cadogan M. Cardiac arrhythmia during propofol sedation. Emerg Med Australas. (2008) 20:437–40. doi: 10.1111/j.1742-6723.2008.01126.x
64. Scalese MJ, Herring HR, Rathbun RC, Skrepnek GH, Ripley TL. Propofol-associated QTc prolongation. Ther Adv Drug Saf. (2016) 7:68–78. doi: 10.1177/2042098616641354
65. Kohro S, Hogan QH, Nakae Y, Yamakage M, Bosnjak ZJ. Anesthetic effects on mitochondrial ATP-sensitive K channel. Anesthesiology. (2001) 95:1435–340. doi: 10.1097/00000542-200112000-00024
66. Kawano T, Oshita S, Tsutsumi Y, Tomiyama Y, Kitahata H, Kuroda Y, et al. Clinically relevant concentrations of propofol have no effect on adenosine triphosphate-sensitive potassium channels in rat ventricular myocytes. Anesthesiology. (2002) 96:1472–7. doi: 10.1097/00000542-200206000-00029
67. Kamada N, Kanaya N, Hirata N, Kimura S, Namiki A. Cardioprotective effects of propofol in isolated ischemia-reperfused guinea pig hearts: role of KATP channels and GSK-3beta. Can J Anaesth. (2008) 55:595–605. doi: 10.1007/BF03021433
68. Fassl J, High KM, Stephenson ER, Yarotskyy V, Elmslie KS. The intravenous anesthetic propofol inhibits human L-type calcium channels by enhancing voltage-dependent inactivation. J Clin Pharmacol. (2011) 51:719–30. doi: 10.1177/0091270010373098
69. Zhou W, Fontenot HJ, Liu S, Kennedy RH. Modulation of cardiac calcium channels by propofol. Anesthesiology. (1997) 86:670–5. doi: 10.1097/00000542-199703000-00020
70. Shibuya N, Higuchi A, Hatakeyama N, Yamazaki M, Ito Y, Momose Y. Effects of propofol on contractility and electrophysiological properties of canine single cardiomyocytes. Masui. (1996) 45:408–14.
71. Puttick RM, Terrar DA. Effects of propofol and enflurane on action potentials, membrane currents and contraction of guinea-pig isolated ventricular myocytes. Br J Pharmacol. (1992) 107:559–65. doi: 10.1111/j.1476-5381.1992.tb12783.x
72. Shigemura T, Hatakeyama N, Shibuya N, Yamazaki M, Masuda A, Chen FS, et al. Effects of propofol on contractile response and electrophysiological properties in single guinea-pig ventricular myocyte. Pharmacol Toxicol. (1999) 85:111–4. doi: 10.1111/j.1600-0773.1999.tb00076.x
73. Hatakeyama N, Sakuraya F, Matsuda N, Kimura J, Kinoshita H, Kemmotsu O, et al. Pharmacological significance of the blocking action of the intravenous general anesthetic propofol on the slow component of cardiac delayed rectifier K+ current. J Pharmacol Sci. (2009) 110:334–43. doi: 10.1254/jphs.09060FP
74. Heath BM, Terrar DA. Block by propofol and thiopentone of the min K current (IsK) expressed in Xenopus oocytes. Naunyn Schmiedebergs Arch Pharmacol. (1997) 356:404–9. doi: 10.1007/PL00005069
75. Han SN, Jing Y, Yang LL, Zhang Z, Zhang LR. Propofol inhibits hERG K+ channels and enhances the inhibition effects on its mutations in HEK293 cells. Eur J Pharmacol. (2016) 791:168–78. doi: 10.1016/j.ejphar.2016.08.028
76. Booker PD, Whyte SD, Ladusans EJ. Long QT syndrome and anaesthesia. Br J Anaesth. (2003) 90:349–66. doi: 10.1093/bja/aeg061
77. Heinonen J, Orko R, Louhija A. Anaesthesia for cardioversion: a comparison of althesin and thiopentone. Br J Anaesth. (1973) 45:49–54. doi: 10.1093/bja/45.1.49
78. Romito G, Castagna P, Pelle NG, Testa F, Sabetti MC, Cipone M. Retrospective evaluation of the ST segment electrocardiographic features in 180 healthy dogs. J Small Anim Pract. (2022) 63:756–62. doi: 10.1111/jsap.13532
79. Goh MH, Yo SL, Ip-Yam PC. The predictive value of intraoperative ST-segment monitoring as a marker of myocardial injury. Ann Acad Med Singap. (2000) 29:173–6.
80. Romito G, Castagna P, Pelle NG, Testa F, Sabetti MC, Cipone M. The canine T wave: a retrospective analysis on qualitative and quantitative T wave variables obtained in 129 healthy dogs and proposed reference intervals. J Vet Cardiol. (2022) 42:52–64. doi: 10.1016/j.jvc.2022.06.003
81. Romito G, Cipone M. Transient deep and giant negative T waves in dogs with myocardial injury. J Vet Cardiol. (2021) 36:131–40. doi: 10.1016/j.jvc.2021.05.009
82. Struthers AD, Reid JL, Whitesmith R, Rodger JC. Effect of intravenous adrenaline on electrocardiogram, blood pressure, and serum potassium. Br Heart J. (1983) 49:90–3. doi: 10.1136/hrt.49.1.90
83. Contrada RJ, Krantz DS, Durel LA, Levy L, LaRiccia PJ, Anderson JR, et al. Effects of beta-adrenergic activity on T-wave amplitude. Psychophysiology. (1989) 26:488–92. doi: 10.1111/j.1469-8986.1989.tb01957.x
84. Contrada RJ, Dimsdale J, Levy L, Weiss T. Effects of isoproterenol on T-wave amplitude and heart rate: a dose-response study. Psychophysiology. (1991) 28:458–62. doi: 10.1111/j.1469-8986.1991.tb00731.x
85. Annila P, Lindgren L, Loula P, Dunkel P, Annila I, Yli-Hankala A, et al. T-wave amplitude changes during thiopentone induction with or without alfentanil. Acta Anaesthesiol Scand. (1995) 39:401–5. doi: 10.1111/j.1399-6576.1995.tb04085.x
86. Zabel M, Woosley RL, Franz MR. Is dispersion of ventricular repolarization rate dependent? Pacing Clin Electrophysiol. (1997) 20:2405–11. doi: 10.1111/j.1540-8159.1997.tb06078.x
87. Day CP, McComb JM, Campbell RW. QT dispersion: an indication of arrhythmia risk in patients with long QT intervals. Br Heart J. (1990) 63:342–4. doi: 10.1136/hrt.63.6.342
88. Kautzner J, Malik M. QT interval dispersion and its clinical utility. Pacing Clin Electrophysiol. (1997) 20:2625–40. doi: 10.1111/j.1540-8159.1997.tb06112.x
89. Kim CJ, Lever N, Cooper JO. Antiarrhythmic drugs and anaesthesia: part 1. Mechanisms of cardiac arrhythmias. BJA Educ. (2023) 23:8–16. doi: 10.1016/j.bjae.2022.11.001
90. Priori SG, Barhanin J, Hauer RN, Haverkamp W, Jongsma HJ, Kleber AG, et al. Genetic and molecular basis of cardiac arrhythmias: impact on clinical management. Study group on molecular basis of arrhythmias of the Working Group on Arrhythmias of the European Society of Cardiology. Eur Heart J. (1999) 20:174–95. doi: 10.1053/euhj.1998.1220
92. Kaplan J, Kanwal A, Ahmed I, Lala V. Reentrant Arrhythmias. Treasure Island, FL: StatPearls Publishing (2023).
93. Kasamaki Y, Ozawa Y, Ohta M, Sezai A, Yamaki T, Kaneko M, et al. Automated versus manual measurement of the QT interval and corrected QT interval. Ann Noninvasive Electrocardiol. (2011) 16:156–64. doi: 10.1111/j.1542-474X.2011.00423.x
Keywords: anesthesia, propofol, alfaxalone, electrocardiogram, arrhythmias, QT interval
Citation: Casoria V, Greet V, Auckburally A, Murphy S and Flaherty D (2024) Comparison of the effects of propofol and alfaxalone on the electrocardiogram of dogs, with particular reference to QT interval. Front. Vet. Sci. 10:1330111. doi: 10.3389/fvets.2023.1330111
Received: 30 October 2023; Accepted: 18 December 2023;
Published: 08 January 2024.
Edited by:
Federico Corletto, University of Nottingham, United KingdomReviewed by:
Veronica Re Bravo, Anicura Glories Veterinary Hospital, SpainCopyright © 2024 Casoria, Greet, Auckburally, Murphy and Flaherty. This is an open-access article distributed under the terms of the Creative Commons Attribution License (CC BY). The use, distribution or reproduction in other forums is permitted, provided the original author(s) and the copyright owner(s) are credited and that the original publication in this journal is cited, in accordance with accepted academic practice. No use, distribution or reproduction is permitted which does not comply with these terms.
*Correspondence: Vincenzo Casoria, dmluY2Vuem8uY2Fzb3JpYUBzY3ZldHNwZWNpYWxpc3RzLmNvLnVr
Disclaimer: All claims expressed in this article are solely those of the authors and do not necessarily represent those of their affiliated organizations, or those of the publisher, the editors and the reviewers. Any product that may be evaluated in this article or claim that may be made by its manufacturer is not guaranteed or endorsed by the publisher.
Research integrity at Frontiers
Learn more about the work of our research integrity team to safeguard the quality of each article we publish.