- 1Animal Genomics Lab, Animal Biotechnology Centre, ICAR-National Dairy Research Institute, Karnal, India
- 2Lifespan and Population Health, School of Medicine, University of Nottingham, Nottingham, United Kingdom
- 3ICAR-Central Institute for Research on Buffaloes, Hisar, India
Introduction: One of the most evolutionary conserved communication systems, the Wnt signaling pathway is a major gene regulatory pathway that affects the developmental competence of oocytes and regulates most embryonic developmental processes. The present study was undertaken to modulate the canonical Wnt (Wingless/integration) signaling pathway in the poor-quality (colorless cytoplasm after Brilliant Cresyl Blue staining, BCB-) buffalo cumulus-oocyte complexes (COCs) to improve their in vitro maturation (IVM) and embryo production (IVEP) rates.
Methods: The expression of key Wnt pathway genes was initially assessed in the good (blue cytoplasm after Brilliant Cresyl Blue staining, BCB+) and poor quality (BCB-) buffalo COCs to establish a differential activity of the Wnt pathway. The BCB- COCs were supplemented with the Wnt pathway inhibitor, Dickkopf-related protein 1 (DKK1) and later subjected to IVM and IVEP along with the BCB+ and BCB- controls. The cumulus expansion index (CEI), rate of nuclear maturation (mean percentage of oocytes in the MII stage) and embryo production, and the expression of developmentally important genes were evaluated to assess the effect of Wnt pathway inhibition on the development competence of these poor-quality oocytes.
Results: The Wnt pathway genes exhibited a significantly higher expression (p < 0.05) in the poor-quality BCB- oocytes compared to the good-quality BCB+ oocytes during the early maturation stages. The supplementation of BCB- COCs with 100 ng/mL DKK1 effectively inhibited the expression of the key mediators of the Wnt pathway (β-catenin and dishevelled homolog 1, DVL1). DKK1 supplemented BCB- COCs exhibited significantly improved cytoplasmic and nuclear maturation indices, development rates and significantly elevated expression (p < 0.05) of genes implicated in germinal vesicle breakdown (GVBD) and embryonic genome activation (EGA) vis-à-vis BCB- control COCs.
Conclusion: These data indicate that inhibition of the Wnt pathway during the initial course of oocyte maturation can improve the development competence of poor-quality buffalo oocytes.
1 Introduction
An irreplaceable milk producer in tropical countries, Buffalo suffers from several reproductive limitations, e.g., silent estrous expression, low conception rate, seasonal fluctuations in fertility, long calving intervals and a low number of primordial follicles (1–3). Adept implementation and use of assisted reproductive technologies (ARTs) have been suggested as measures to overcome these physiological limitations and for the fast propagation of superior germplasm (4–7). Despite the availability of highly optimized culture conditions and techniques for the stringent quality selection of oocytes, the in vitro embryo production (IVEP) technology in buffalo has not been very successful (8, 9). Such optimized methodologies that are otherwise successful in cattle often result in only a meager percentage of buffalo oocytes reaching the blastocyst stage (10, 11). The development of in vitro fertilized buffalo embryos depends upon numerous extrinsic and instric factors viz. ovarian morphology, season, donor, culture conditions, hormones, and oocyte quality among others (7, 12–14). Besides, the low efficiency of multiple-ovulation and embryo transfer (MOET) often attributed to low fertility and poor response to superovulation further decrease the success rate of buffalo IVEP (4, 9). The identification and selection of good-quality oocytes (high competence) is a crucial step for ensuring their complete maturation and subsequent embryonic development, which is critical for successful IVEP (15–17). The Brilliant Cresyl Blue (BCB) staining framework, which assesses the physiological status of oocytes has been used as a successful methodology for selecting competent oocytes (18–20). This technique relies on the activity of glucose-6-phosphate dehydrogenase (G6PD) in the growing oocytes. A low G6PD activity results in blue coloration of the cytoplasm (BCB+ oocytes) indicating completion of oocyte maturation/growth (21, 22). Contrarily, the BCB- oocytes reduce the BCB stain and appear colorless post-staining. Such oocytes lie far behind the BCB+ oocytes in terms of their developmental potential (23). One of the greatest challenges that still exists in the field of reproduction and developmental biology is to understand what constitutes oocyte developmental competence.
The Wnt pathway is considered one of the major gene regulatory pathways that affect the developmental competence of oocytes and is considered crucial for several early developmental processes, e.g., in-vitro blastocyst development (24–27). One of the most evolutionary conserved communication systems, the Wnt pathway regulates most embryonic developmental processes, e.g., dorsoventral and anteroposterior body axes formation, cell fate determination and organogenesis (28–31). A wide range of effectors (agonists/antagonists) regulates the Wnt pathway and these effectors can act either intracellularly or extracellularly. At the intracellular level, they modify the components of the signaling machinery whereas, at the extracellular level, they modulate the ligand-receptor interactions (32, 33). These antagonists and agonists are of immense importance as they help in the intricate modulation of the Wnt signaling and thus help in the activation or inhibition of Wnt-regulated developmental processes (34). One such antagonist, Dickkopf-Related Protein 1 (DKK-1) is a secreted inhibitor of the Wnt pathway that belongs to the Dickkopf family of evolutionarily conserved glycoproteins (35, 36). DKK1 inhibits the Wnt/β-catenin pathway by binding to the essential co-receptors for Wnt signaling, i.e., low-density lipoprotein receptor-related protein 5 (LRP5) and LRP6 which results in decreased β-catenin protein stability (37). The effect of DKK1 on oocyte maturation and function, embryo development, hatching of blastocysts and their implantation has been studied in detail (38–42). For example, the supplementation of Dickkopf-Related Protein 1 to the maturation medium of in vitro matured oocytes (with increased Wnt gene expression) improved their maturation parameters and the number of nuclei in the resulting blastocyst stage embryos (43). The abovementioned studies revealed that the modulation of the Wnt signaling pathway is critical for IVEP since the dysregulated activity in the Wnt pathway can perturb the embryonic development process. Therefore, we hypothesized that a differential level of Wnt signaling activity exists in the buffalo oocytes of high (BCB+) and low (BCB-) quality and a transient induction/repression of this pathway should improve the development competence of poor-quality oocytes.
Thus, the primary objective of the current study was to investigate whether inhibiting/activating this pathway at a pre-determined course of oocyte maturation can help the buffalo oocytes of poor quality (BCB-) gain enhanced development competence.
2 Methods
All chemicals, media and reagents were procured from Sigma Aldrich Chemical Co. Ltd., (United States) unless stated otherwise and plastic ware was obtained from Nunc (Thermo Fisher Scientific, USA). Fetal bovine serum (FBS) was obtained from Hyclone, Canada.
2.1 COCs (cumulus-oocyte complexes) collection, BCB screening, and in vitro maturation
The collection of COCs and BCB screening were done as described previously for buffalo (44) with minor modifications. Briefly, the buffalo ovaries were collected from a local abattoir (Delhi) and were transported within 4 h to the laboratory in physiological saline (0.9%, w/v NaCl, 38°C) containing Streptomycin–penicillin (50 mg/L). The cumulus-oocyte complexes (COCs) were aspirated from the antral follicles (4–8 mm in diameter) using an 18G needle attached to a 10 mL syringe containing 1 mL Hepes-buffered hamster embryo culture (HH) medium. The quality of COCs was observed under a stereo-zoom microscope (20X magnification) and only the grade A COCs (> 4 layers of cumulus cells and clear, homogenous cytoplasm) were considered for downstream experiments.
The selected grade-A COCs were washed thrice in HH medium, subsequently exposed to 26 μM BCB solution and incubated for 90 min at 38.5°C in a CO₂ incubator. The excess stain was removed by washing once in mDPBS and the COCs were observed under a stereo zoom microscope. COCs were classified based on cytoplasm coloration as either BCB+ (Blue cytoplasm) or BCB- (Colorless cytoplasm). Later, both the BCB+ and BCB- COCs were kept in IVM medium (HEPES buffered TCM-199 supplemented with 10% FBS, 0.14 mg/mL Sodium Pyruvate, 0.1 mg/mL Glutamine, 5 mg/mL Streptomycin, along with 0.5 mg/mL FSH, 0.5 mg/mL LH, 1 μg/mL Estradiol 17-β, 0.1 mg/mL EGF, and 32 mg/mL Cysteamine) for washing. The washed COCs were then placed in 100 μL maturation drops (n = 15 COCs/drop) prepared in 35 mm culture dishes and were then overlaid with 3 mL of mineral oil. The COCs were incubated at 38.5°C in a CO₂ incubator with 98% humidity and 5% CO₂ for 24 h.
2.2 Expression analysis of Wnt pathway genes in BCB+ and BCB- oocytes
For RNA isolation, the denuded BCB+ and BCB- oocytes (n = 10 each) were collected from maturation drops at different hours of maturation, i.e., 0, 8, 16, and 24 h. The oocytes were denuded by brief vortexing followed by repeated washing in PBS (Phosphate Buffer Saline). The 10 oocytes were pooled together for RNA extraction and the experiment was carried out in three biological replicates. The total RNA was isolated using the RNAqueous Micro Kit (Ambion, United States) according to the manufacturer’s instructions and quantified using a NanoDrop ND-1000 UV–Vis spectrophotometer (NanoDrop Technologies Inc., Wilmington, DE, United States). Only the samples with A260/280 and A260/230 close to 2.0 were considered for the gene expression experiments. All the RNA samples were treated with RNase-free DNase I (Ambion, United States) and a fixed amount of RNA (100 μg) was reverse transcribed into cDNA using the RevertAid, H Minus first-strand cDNA synthesis kit (Thermo Scientific, USA) following the manufacturer’s instructions. A reverse transcriptase negative control was prepared to assess the residual genomic DNA contamination in the RNA sample. The cDNA samples were diluted three times (final volume-60 μL) with nuclease-free water and stored at −20°C until further use.
To study the differential activity of the Wnt pathway in BCB+ and BCB- oocytes, the gene expression of the key molecules of the Wnt pathway viz. β-catenin, DVL1, FZD4, WNT3A, and WNT7A were quantified. The intron-spanning primers for the Wnt pathway genes and the reference gene (RPS18) were designed using the Primer 3 software using the sequences obtained from the bovine genome database in GenBank and EMBL (Supplementary Table 1). The self-annealing sites, mismatches and secondary structures in the designed primers were checked using Oligo Calc (45). The specificity of primers was checked using the BLAST alignment tool (46) and in silico PCR (47) was run for each set of primers before commercial synthesis (Sigma-Aldrich, United States). To ensure the quality of RT-qPCR data, MIQE guidelines (48) were followed at every step, wherever possible. The relative quantification of the Wnt pathway genes was done on a Roche LightCycler® 96 platform (Roche Diagnostics, Mannheim, Germany) using the Maxima SYBR Green qPCR master mix (Fermentas, United States) in a 10 μL reaction mix. The reactions were performed in duplicate for each sample with the following thermal profile: denaturation at 95°C for 10 min, then 40 cycles consisting of denaturation at 95°C for 15 s, annealing at optimized temperatures for 15 s and extension at 72°C for 20 s. Upon completion of amplification, a melt curve analysis was performed to ensure a specific, unique product formation and to ascertain minimal primer-dimer formation. A no-template control (NTC) was run in each plate to confirm the absence of nucleic acid contamination. The mean sample Cq (Cycle of quantification) values for different transcripts were calculated for the duplicate samples and the relative expression ratio of the Wnt pathway gene expression was calculated using the ΔΔCt (Cycle threshold) method (49).
2.3 Validation of the differential gene expression between the BCB+ and BCB- oocytes
To validate the differential gene expression between the BCB+ and BCB- oocytes at the protein level, we measured the abundance of β-catenin, a key component of the Wnt pathway (50) at 0, 8, 16, and 24 h of maturation. Briefly, the cumulus cells were separated from the BCB+ and BCB- COCs by vortexing followed by washing in PBS (phosphate-buffered saline). The oocytes were then fixed in freshly prepared 4% (w/v) paraformaldehyde in PBS for 30 min at RT (Room Temperature) and subsequently permeabilized in 0.5% Triton X-100 (in PBS) for 10 min at RT. Thereafter, the oocytes were washed with 0.05% Tween-20 (PBST) and blocked with 1% BSA (Bovine Serum Albumin) for 1 h and subsequently transferred to primary antibody solution (Abcam, ab6302, Rabbit polyclonal to β-catenin, 1:100 in PBST) and incubated at 4°C overnight. The next day, the oocytes were washed 10 times (5 min) in PBST containing 1% BSA and transferred to a FITC conjugated secondary antibody (Abcam, ab97050, 1:500) solution for 30 min and mounted on a glass slide using Prolong Gold Antifade Reagent and observed under a blue filter (Fluorescence filter characteristics: excitation wavelength 470-490 nm, emission wavelength 510 nm) of a fluorescent microscope (Olympus BX-51, fitted with a digital CCD, DP71 camera) using the Olympus MICRO DP71 software ver.03.03. The fluorescent intensity was measured and quantified for a minimum of 50 oocytes in at least 10 fields covering the entire slide (n = five biological replicates) using the Image J software. A “no-primary antibody” control was used to ensure signal from the detection of the antigen (β-catenin) by the primary antibody. The entire periphery of the oocyte was selected as the region of interest (ROI) and was considered for the quantification of the fluorescence signal. The fluorescence intensities were quantified at random from the micrographs that were captured using similar acquisition settings, e.g., the time of exposure, magnification and acquisition formats. The integrated density (IntDen) value, which represents the product of “Area”, and “Mean Gray Value” for the chosen ROIs in a non-calibrated image, was calculated for each cell. Thereafter, the IntDen values were normalized (vis-a-vis the immediate background) and considered the final mean fluorescent intensity (MFI) values.
2.4 Dosage determination of Wnt pathway inhibitor, DKK1
To inhibit the over activity of Wnt signaling in BCB- oocytes, Wnt-inhibitor, DKK-1 (50 ng/L, 100 ng/mL, and 200 ng/mL) was added to the IVM medium for the initial 8 h. After exposure of 8 h the BCB- oocytes supplemented with inhibitors were taken out from the IVM medium, washed thrice in HH media and kept in the IVM medium (without inhibitors). To select the optimum dose of the inhibitor, the cumulus expansion index (CEI) and embryo production rates were assessed.
2.5 Cumulus expansion and in vitro embryo production rates after DKK1 supplementation
After the supplementation of DKK1 for 8 h, the BCB- COCs were evaluated for their level of cumulus expansion after 24 h of IVM. A scale of 0–3 was used to measure the cumulus expansion. The COCs which had the maximum degree of cumulus expansion were assigned scale 3, COCs with no cumulus expansion were given scale 0 and those with intermediate expansion were given scale 1 (only outer layers expand) or 2 (more than 50% of layers expand).
To assess the in vitro embryo production rate upon DKK1 exposure, oocytes from distinct groups viz. BCB+, BCB-(Control), BCB-(+50 ng/mL DKK1), BCB-(+100 ng/mL DKK1), BCB-(+200 ng/mL DKK1) were processed for IVM separately. The in vitro fertilization (IVF) was carried out as per the standard protocol followed in our lab (51) in IVF droplets (100 μL) of Brackett and Oliphant (BO) medium supplemented with 1% BSA (fatty acid-free), 1.9 mg/mL Caffeine Sodium Benzoate, 0.14 mg/mL Sodium Pyruvate and 0.01 mg/mL Heparin. The matured oocytes were transferred into the drops after washing thrice in BO medium. The frozen buffalo semen was thawed simultaneously and processed for in vitro capacitation, as described previously (52). Fifty microliter of IVF media (BO) was replaced with 50 μL of the sperm suspension (1 × 106 spermatozoa/mL) added to each fertilization drop with 15 COCs and then incubated at 38.5°C with 5% CO2 for 12 h. Thereafter, the presumptive zygotes were denuded by brief vortexing and washed five times in a modified Charles and Rosenkrans 2 amino acid (mCR2aa). After washing, the 15 presumptive zygotes were co-cultured with the monolayer of granulosa cells in 100 μL drops of IVC-I medium (mCR2aa supplemented with 8 mg/mL BSA, 180.156 mg/mL Glucose, 29.059 mg/mL Pyruvate, 146.14 Glutamine, 1 × MEM essential amino acid, 1x non-essential amino acid and 50 μg/mL Gentamycin). After 48 h post insemination (hpi) the zygotes were evaluated for evidence of cleavage. At 72 hpi, all the cleaved embryos were transferred to IVC-II medium (IVC-I with 10% FBS) and were maintained for 8 days post-insemination at 38.5°C with 5% CO2. The culture medium was regularly replaced every 48 h.
2.6 Inhibition of the Wnt pathway by DKK1 dosage during the initial stages of maturation
To ascribe the observed improvements in CEI and IVEP in BCB- oocytes upon DKK1 supplementation (100 ng/mL) to the inhibition of the Wnt signaling pathway, the expression of two key mediators of Wnt signaling viz. β catenin and DVL1 was evaluated at five different intervals, i.e., 0 h 2 h, 4 h, 6 h, 8 h of in vitro maturation in n = 10 oocytes/group (3 biological replicates). These time points were selected since the difference in the expression of Wnt genes in the BCB+ and BCB- oocytes was maximum during the initial 8 h of IVM.
2.7 Assessment of nuclear maturation of oocytes upon Wnt pathway inhibition
The nuclear maturation of oocytes at the end of IVM was ascertained by Hoechst staining, a simple and fast technique often used for chromatin evaluation. The cumulus cells were removed from the COCs by vortexing and subsequently washed thrice in PBS-PVP (1 mg/mL). The oocytes were then placed in Hoechst staining solution (10 μg/mL in PBS-PVP) for 15 min at 37°C and again washed (3X). The 12 washed oocytes were placed singly on a clean, grease-free microscopic glass slide having rails of wax along its two sides. The slides were observed under UV fluorescence (Fluorescence filter characteristics: excitation wavelength 355-375 nm, emission 420 nm) on an Olympus BX-51 microscope fitted with a DP-71 camera.
2.8 Effect of Wnt pathway inhibition on blastocyst health
The ICM/TE ratio, which is an indicator of the health of blastocysts, was determined using the differential staining of in vitro-produced blastocysts, as described by Kumar and co-authors (53). Briefly, the blastocysts were removed from the culture and washed with PBS-PVP before being placed in PI solution (100 μg/mL of Propidium iodide in 0.2% Triton X-100) for 20 s. Thereafter, the blastocysts were washed thrice in PBS-PVP (1 mg/mL) and placed in working Hoechst solution (1 μg/mL Hoechst 33342 prepared in 4% formaldehyde (40 mg/mL), in PBS-PVP) for 20 min. The blastocysts were placed on a clean, grease-free microscopic slide in a drop of glycerol and visualized under UV fluorescence and green filter using an Olympus BX-51 fluorescent microscope fitted with a DP-71 camera (Fluorescence filter characteristics: UV- excitation wavelength 355-375 nm, emission 420 nm and Green- excitation wavelength 520-550 nm, emission 580 nm). The two images were overlaid to form a merged image.
2.9 Relative quantification of developmentally important genes after Wnt inhibition
To analyze the effect of Wnt inhibition by DKK1 on oocyte competence, the expression pattern of various genes involved in oocyte maturation and embryonic development was studied. The dynamics of genes related to cumulus expansion (GDF9, HAS2, PTGS2) and GVBD (AKT, Cyclin B and CDC25B) was studied during 0, 2, 4, 6, and 8 h of IVM while the expression of genes related to EGA, i.e., Embryonic Genome Activation (eIF1A, U2AF, and PAP) was evaluated at 2 cell, 4 cell, 8 cell and 16-cell stages (Supplementary Table 1). Briefly, a pool of either 10 oocytes or 10 fertilized oocytes (at 2-cell, 4-cell, 8-cell, and 16-cell stages; 3 biological replicates) from the three sample groups viz. BCB+, BCB- (control), and BCB- supplemented with 100 ng/mL DKK1 were washed with chilled PBS and immediately processed for RNA isolation. The cDNA synthesis and RT-qPCR were carried out as described previously.
2.10 Statistical analysis
Statistical evaluation of the data was done using MS Excel and GraphPad Prism (GraphPad Software, San Diego, CA). The generated data was examined for normality of distribution and transformed if required using arc-sine transformation (Embryo development rate data). Analysis of variance (ANOVA) was used to determine the significance of experimental variables followed by Bonferroni Post-hoc tests to compare the significance of the effect of treatment between groups at 95% confidence intervals (p < 0.05). All the experiments were performed in at least three biological replicates and presented as Mean + SEM (Standard Error of Mean) (standard error of mean).
3 Results
3.1 Wnt pathway genes are differentially expressed in BCB+ and BCB- oocytes
The expression pattern of a panel of five key members of the Wnt signaling pathway viz. β-catenin, Dvl1, FZD4, WNT3A, and WNT7A was examined in the BCB+ and BCB- oocytes at 0, 8, 16, and 24 h of IVM. All the genes considered exhibited a significantly higher expression in BCB- COCs compared to BCB+ COCs at all the time points, particularly during the initial hours of oocyte maturation (Figures 1A–E). The expression of β catenin and FZD4 was highest at 16 h post-IVM in BCB- oocytes whereas the expression of Dvl-1 and WNT3A peaked at 8 and 24 h, respectively. The differences in expression between the BCB+ and BCB- COCs were greatest at 8 h of IVM for all the Wnt pathway genes.
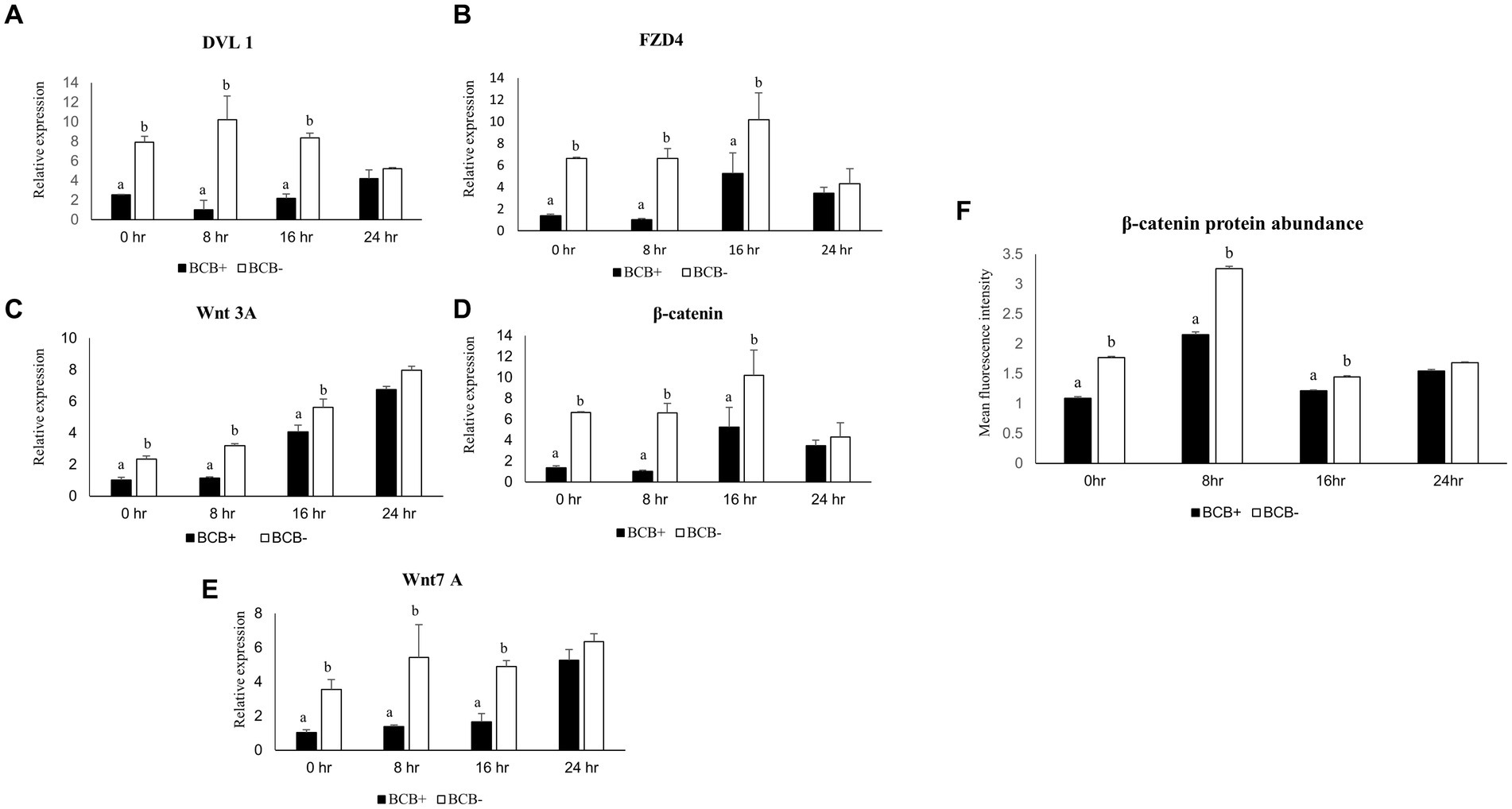
Figure 1. Differential expression of Wnt pathway genes in good and poor-quality buffalo oocytes. Relative expression profiles of Wnt pathway genes in BCB+ and BCB- oocytes during four different time points of maturation (A–E). Mean fluorescence intensities obtained for β-catenin abundance by immunostaining in BCB+ and BCB- oocytes by using Image J (F). Values are presented as Mean ± SEM. Bars with different superscripts indicate a significant difference (p < 0.05).
3.2 A higher abundance of β-catenin protein was observed in BCB- oocytes
β-catenin, a pivotal player in the Wnt pathway was found to be localized below the buffalo oocyte plasma membrane (Supplementary Figure 1), as reported previously in mice (54). The mean fluorescent intensity (MFI) for β-catenin was considerably higher (p < 0.05) in the BCB- oocytes vis-à-vis the BCB+ oocytes during the early course of maturation (Figure 1F). The maximum MFI was observed at 8 h post-IVM in BCB- COCs. Interestingly, the differences in abundance between the BCB+ and BCB- COCs were highest at 8 h post-IVM indicating a correlation between the gene expression and protein abundance. This established that the Wnt pathway is more active in BCB- oocytes than BCB+ oocytes, particularly during the initial 8 h of IVM. Therefore, we considered the first 8 h of maturation for manipulating the Wnt pathway in BCB- oocytes and carried out all further experiments at this time point.
3.3 Supplementation of DKK1 (100 ng/mL) improves CEI and development rates of BCB- oocytes
Cumulus expansion can be considered a morphological indicator of the oocytes’ fertilizing ability and is associated with higher developmental competence (55). To evaluate the effect of Wnt inhibition on cumulus expansion and in vitro embryo development, we considered three doses viz. 50, 100, and 200 ng/mL of Wnt inhibitor, DKK1 based on previous literature (43). The mean percentage of BCB+ oocytes with the maximum expansion, i.e., CEI-3 was significantly higher (p < 0.05) in the BCB+ oocytes compared with BCB- oocytes (Figure 2; Supplementary Figure 2). The supplementation of BCB- oocytes with 100 ng/mL of Wnt inhibitor assisted more oocytes in reaching the highest scale of cumulus expansion in buffalo oocytes.
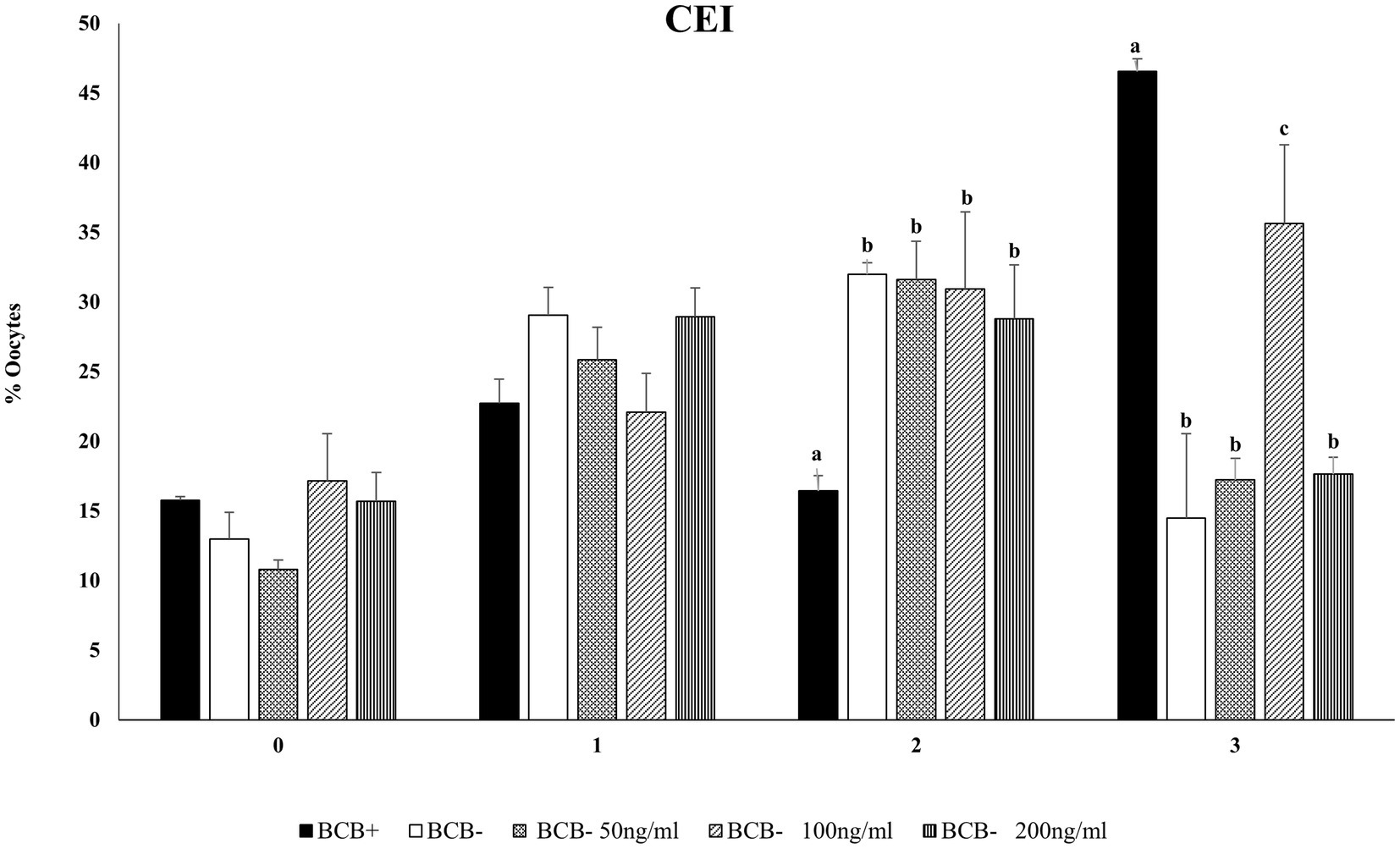
Figure 2. Cumulus expansion index (CEI)Mean percentage of oocytes observed with different cumulus expansion index in BCB+, BCB- (control), and BCB- oocytes supplemented with various concentrations of Wnt inhibitor, DKK1. Values are presented as Mean ± SEM. Bars with different superscripts indicate a significant difference (p < 0.05).
The effect of Wnt inhibition on the development rate of buffalo oocytes was assessed by calculating the percentage of cleaved oocytes, those reaching 8-16cell, morula, and blastocyst stages of the embryo development. The inhibition of the Wnt pathway by DKK1 did not affect the initial stages of embryo development at any of the considered doses (Table 1). However, a significant difference (p < 0.05) in the mean percentage of fertilized oocytes reaching the morula and blastocyst stages was observed between BCB- oocytes supplemented with 100 ng/mL of Wnt inhibitor and the BCB- control.
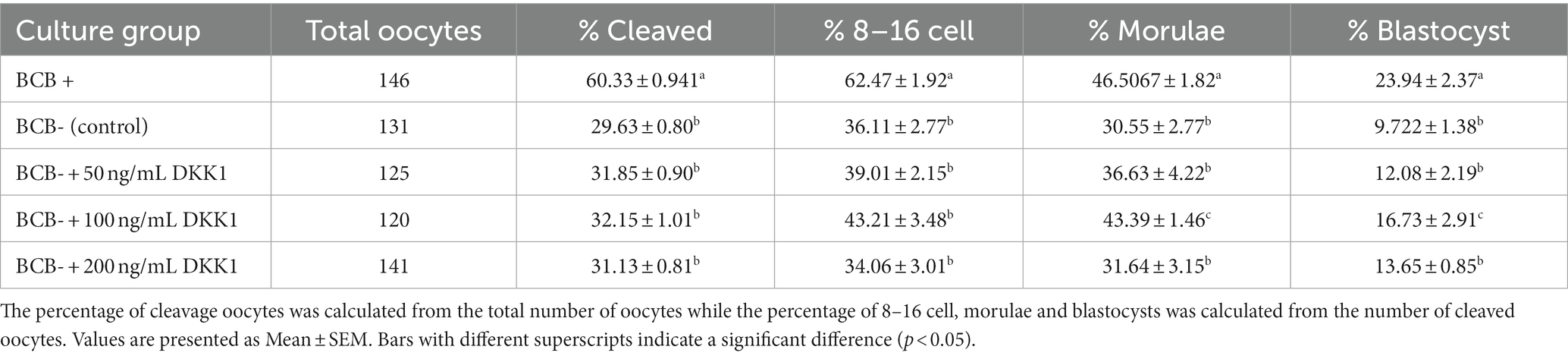
Table 1. Developmental rates of BCB+, BCB-, and BCB- oocytes supplemented with various concentrations of Wnt inhibitor, DKK1.
Based on the developmental data and cumulus expansion index we considered 100 ng/mL dose of DKK1 as the optimum dose for inhibition of Wnt and for carrying out our subsequent experiments.
3.4 DKK1 is an effective antagonist of the Wnt signaling pathway during the initial stages of IVM
The expression of the key mediators of the Wnt signaling pathway viz. β catenin and DVL1 was observed to be significantly reduced (p < 0.05) in the BCB- oocytes supplemented with 100 ng/mL DKK1 compared to the BCB- (control) oocytes at 2, 4, 6, and 8 h of IVM indicating successful inhibition of Wnt pathway by DKK1 (Figure 3).
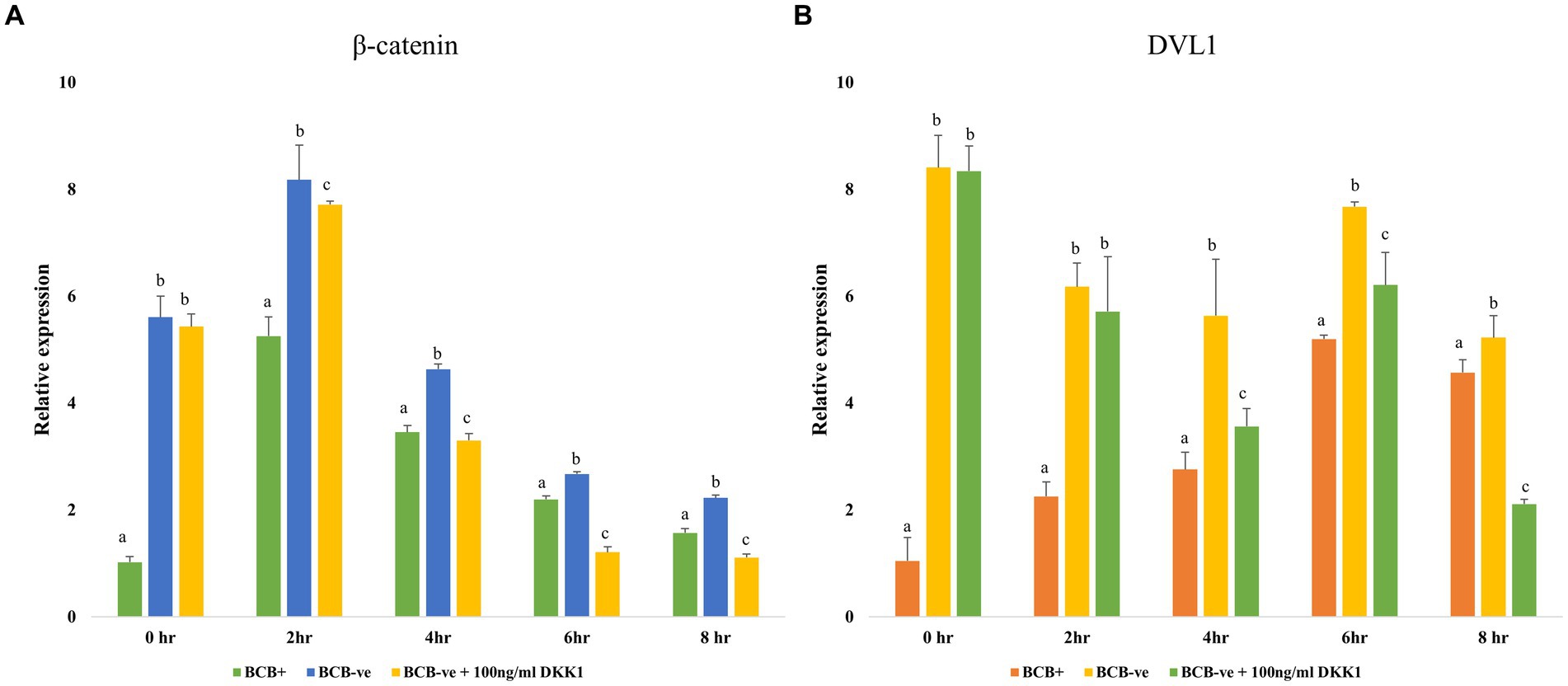
Figure 3. Wnt pathway inhibition Relative expression profiles of key Wnt pathway mediators β catenin (A) and DVL1 (B) in BCB+, BCB- (control), and BCB- oocytes supplemented with 100 ng/mL Wnt inhibitor, DKK1. Values are presented as Mean ± SEM. Bars with different superscripts indicate a significant difference (p < 0.05).
3.5 Inhibition of the Wnt signaling pathway increased the nuclear maturation rate of BCB- oocytes
A total of n = 12 oocytes/group were subject to Hoechst staining to evaluate the nuclear maturation rate of oocytes in the MII stage among the treatment and control groups considered. As expected, the BCB+ oocytes had a significantly higher percentage (p < 0.05) of oocytes in the MII stage than BCB- oocytes (Table 2). The rate of nuclear maturation increased significantly (p < 0.05) in the BCB- oocytes supplemented with 100 ng/mL DKK1 in comparison to the oocytes in the BCB- (control) group.

Table 2. Percentage of oocytes in MII stage in BCB+, BCB- (control) and BCB- oocytes supplemented with 100 ng/mL Wnt inhibitor, DKK1.
3.6 DKK1 Supplementation does not promote cell proliferation in early embryos
Five blastocysts per sample group were subject to differential staining for calculating the ICM/TE ratio. The ICM cells fluoresced blue (Hoechst) whereas the cells which showed reddish-pink fluorescence (Propidium iodide) were considered TE cells (Figure 4). The ICM/TE ratio was significantly higher (p < 0.05) in the BCB+ than in the BCB- oocytes (Table 3). Although the supplementation of BCB- oocytes with 100 ng/mL DKK1 increases the number of TE cells, the difference was deemed statistically non-significant vis-à-vis BCB- control oocytes.
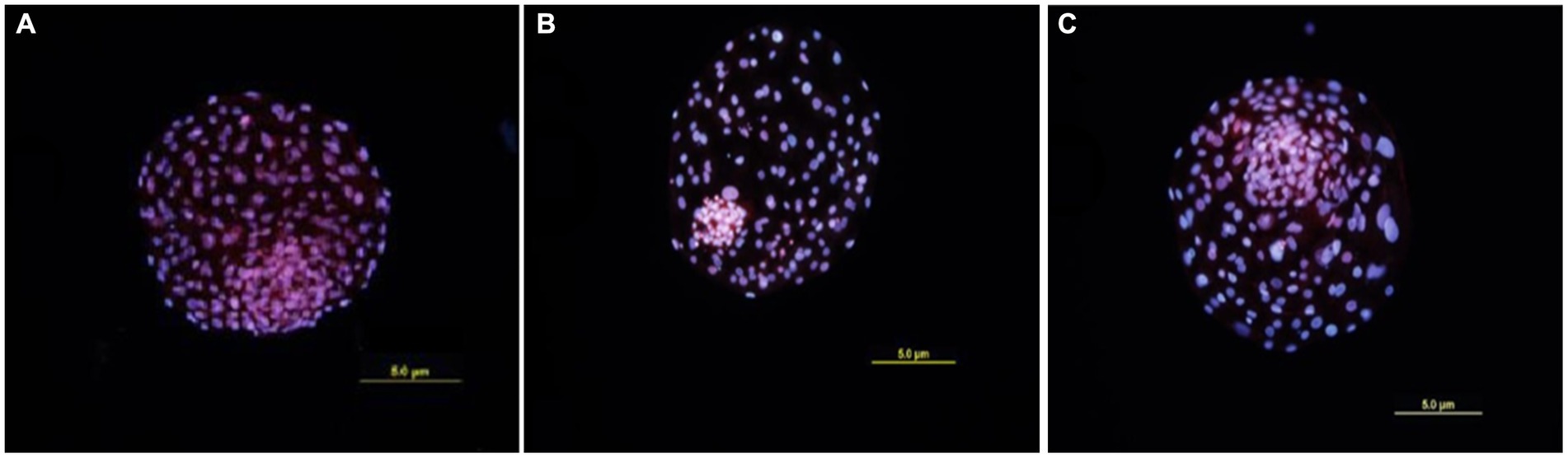
Figure 4. Differential Staining of blastocysts. Cells showing blue fluorescence are ICM cells while red fluorescent cells are TE cells. (A) Blastocyst produced by BCB+ oocytes. (B) Blastocyst produced by BCB- oocytes. (C) Blastocyst produced by BCB- oocytes supplemented with 100 ng/mL DKK1.

Table 3. Total number of ICM and TE cells and ICM/TE ratio (expressed as the percentage) in blastocysts produced from BCB+, BCB- (control), and BCB- oocytes supplemented with 100 ng/mL Wnt inhibitor, DKK1.
3.7 Wnt pathway inhibition increases the expression of developmentally important genes
The developmental ability of oocytes post-DKK1 supplementation was further assessed by monitoring the expression pattern of various genes involved in cumulus expansion, oocyte maturation and embryonic development (Figure 5). The expression of genes related to cumulus expansion elevated significantly (p < 0.05) at 6 h and 8 h of IVM upon DKK1 supplementation of BCB- oocytes (Figures 5A–C) while the expression of genes related to germinal vesicle breakdown in such oocytes increased (p < 0.05) at 8 h of IVM (Figures 5D–F). The expression of genes related to embryonic genome activation increased significantly (p < 0.05) at the 8-cell stage of embryonic development (Figures 5G–I).
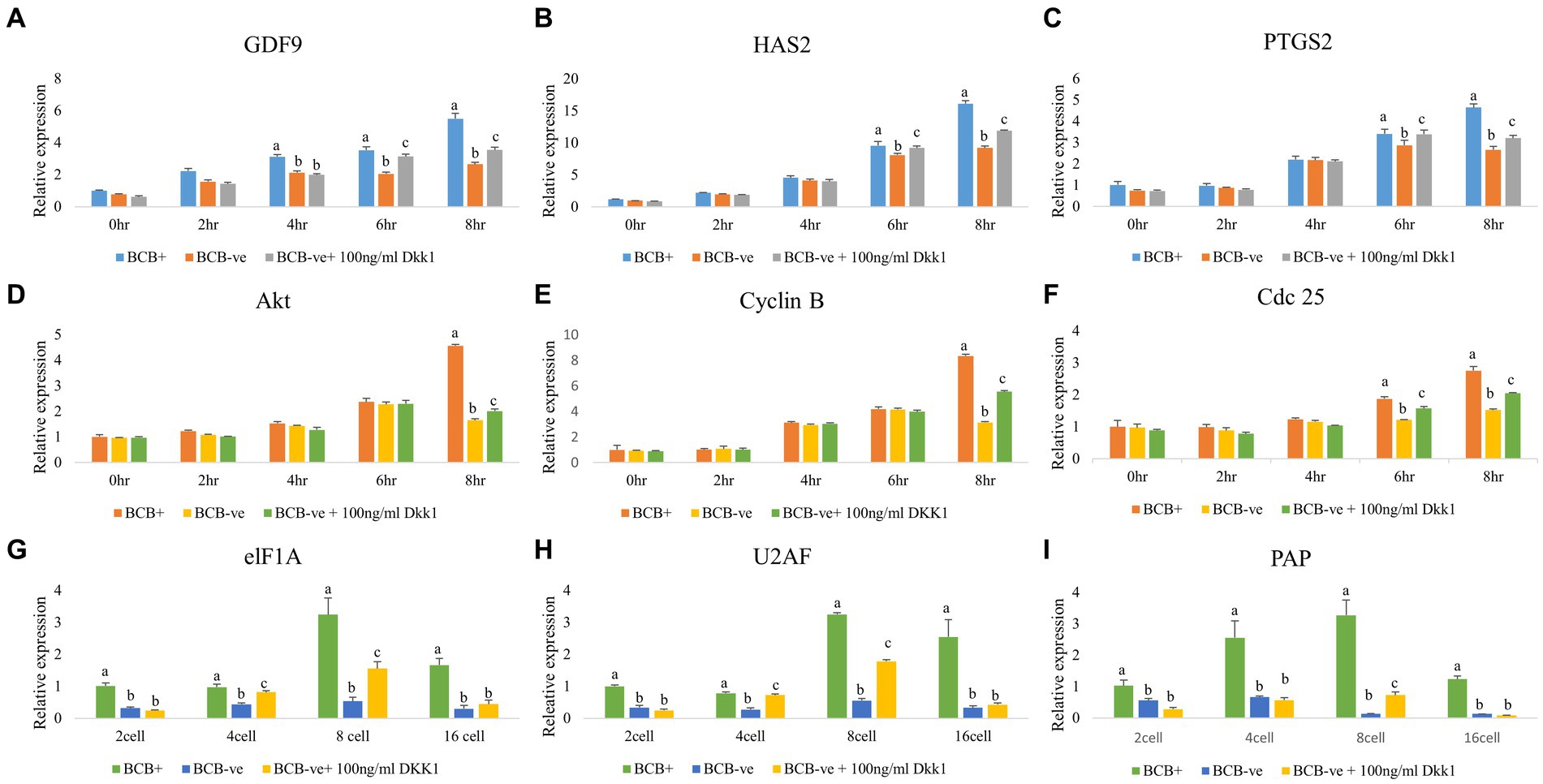
Figure 5. Expression pattern of developmentally important genes in poor-quality oocytes and embryos after Wnt pathway inhibition Real-time expression analysis of cumulus expansion (A–C), GVBD (D–F), and EGA (G–I) genes in BCB+, BCB- (control), and BCB- oocytes supplemented with 100 ng/mL Wnt inhibitor, DKK1 at various stages of IVM (A–F) and embryo development (G–I). Values are presented as Mean ± SEM. Bars with different superscripts indicate a significant difference (p < 0.05).
4 Discussion
The present study was undertaken to investigate whether the poor quality (BCB-) and good quality (BCB+) buffalo oocytes vary vis-à-vis the Wnt signaling pathway and whether the transient inhibition of this pathway at a pre-determined course of oocyte maturation improves the developmental competence of poor quality (BCB-) oocytes. We observed a differential expression of key Wnt genes in the BCB+ and BCB- oocytes, particularly at 8 h of IVM which was further substantiated by assessment of β-catenin protein abundance at this time point. The inhibition of the Wnt pathway in BCB- oocytes using a Wnt antagonist, DKK1 resulted in improved cumulus expansion characteristics, nuclear maturation rate and percentage of oocytes reaching the morula and blastocyst stages (Figure 6). Besides, the expression of developmentally important genes related to cumulus expansion, GVBD and EGA increased in the poor quality (BCB-) oocytes after DKK1 supplementation. The present data underpins the hypothesis that an overactive Wnt signaling pathway perturbs the development potential of growing oocytes and inhibition of this pathway during the initial course of oocyte maturation can improve the developmental ability of poor quality (BCB-) buffalo oocytes.
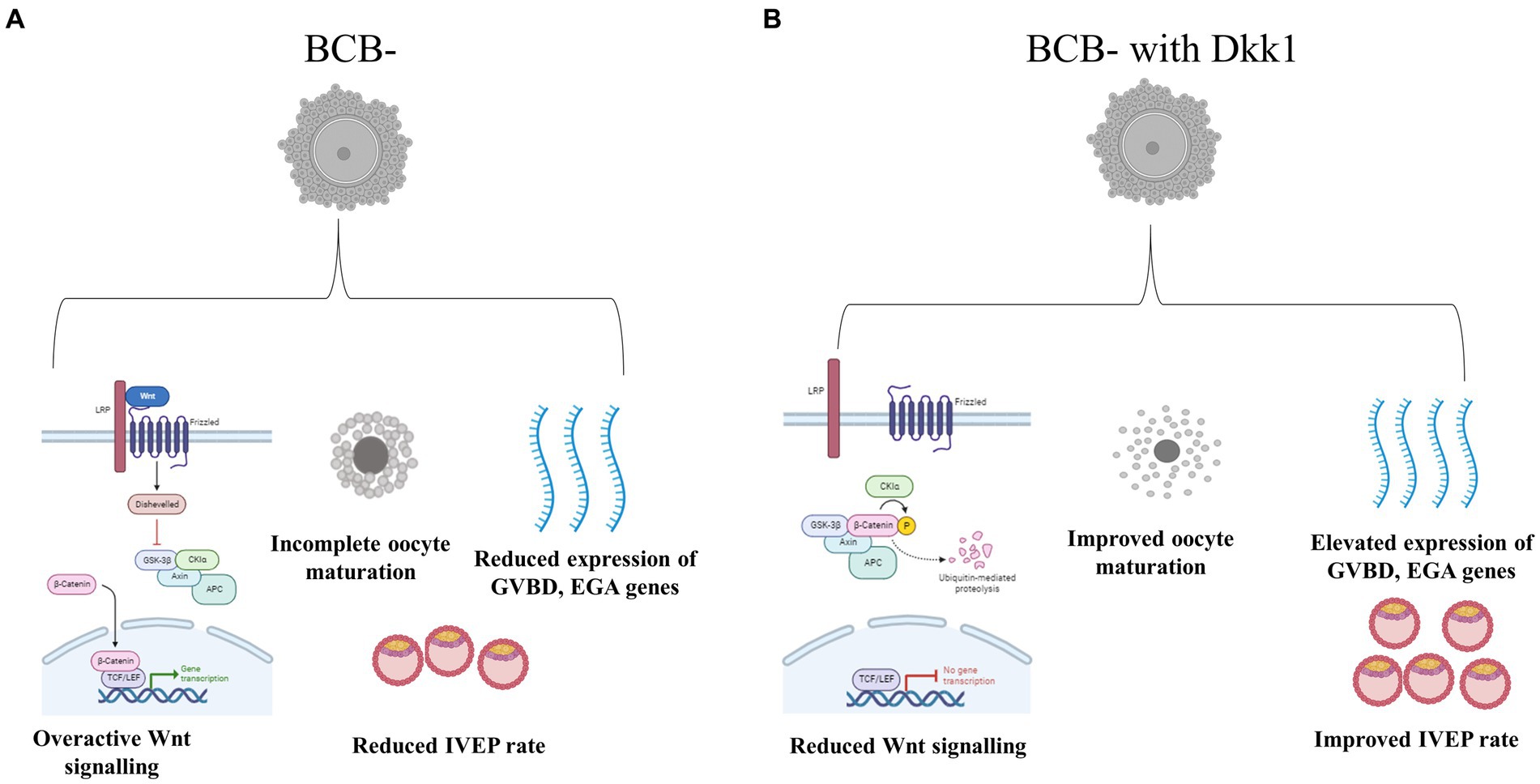
Figure 6. Schematic representation of the effect of Wnt pathway inhibition in BCB- oocytes (A). Supplementation of BCB- oocytes with Wnt pathway antagonist DKK1 resulted in improved development competence of these poor-quality oocytes (B).
Although the BCB screening method provides a solid foundation for differentiating good-quality oocytes from poor-quality oocytes, limited evidence exists to support the variation in their developmental competence. The Wnt signaling pathway is among the most crucial cell–cell communication systems that play indispensable roles in numerous developmental processes, particularly during embryogenesis. It has been reported previously that the over-expression of these pathway genes in the in vitro matured oocytes compromises their quality vis-à-vis the in vivo matured oocytes (43). We observed the maximum difference in the Wnt pathway gene expression between the in vitro matured BCB+ and BCB- oocytes during the initial 8 h (Figures 1A–E). The initial stages of maturation are important since the most important milestone of oocyte development, i.e., GVBD is initiated during this time when the products of different genes required for embryogenesis start accumulating in the oocytes (56). We have previously reported that the GVBD in BCB- oocytes lags by 2–4 h in comparison to the BCB+ oocytes until ~10 h of IVM compromising the developmental ability of poor-quality (BCB-) buffalo oocytes. Nonetheless, the BCB- oocytes were observed to catch up their maturation rates (after 10 h) with that of BCB + oocytes (57). The highest difference in expression and abundance of Wnt pathway elements was observed at 8 h of IVM, therefore we hypothesize a similar synchronization of the Wnt pathway activity between the BCB+ and BCB- oocytes during the initial stages of IVM. It is well-established that each cell has an intrinsic ability to adjust its sensitivity to a signal. The same could be the case with the BCB- oocytes wherein a continuous Wnt signal may cause a swift and substantial increase in the number of endocytic vesicles causing temporary sequestration of receptor protein, e.g., FZD4 in the endosomes (58). However, further studies are warranted to validate this.
It has been reported that the Wnt signaling regulates the blastocyst development and inhibition of this pathway by DKK1 has been demonstrated to improve oocyte maturation (39, 59). Cumulus expansion can be considered as a morphological indicator of oocyte competence and BCB+ oocytes are known to have an optimum CEI which is associated with higher developmental competence (55). Our results agree with previous studies, which have also reported that the BCB+ oocytes have better cumulus expansion than BCB- oocytes (60). Nonetheless, we observed an improvement in the cumulus expansion characteristics of buffalo BCB- oocytes upon supplementation with 100 ng/mL DKK1 (Figure 2), as previously reported in pigs (43, 61). Cumulus expansion significantly influences the maturation of oocytes since the bidirectional crosstalk between the oocytes and the surrounding complex helps in the formation and deposition of an extracellular matrix between cumulus cells which aids in maturation (62). This crosstalk is facilitated by the presence of gap junctions. Cx43 is the major connexin protein that assists in the formation of gap junctions in cumulus cells and is implicated in the modulation of developmental competence (63, 64). A basal level of Wnt signaling, e.g., in BCB+ buffalo oocytes allows the cytoplasmic accumulation of Cx43, whereas an over-activated Wnt signaling will cause the nuclear export of Cx43 and its association with β-catenin destruction complex hence disrupting the gap junctions (65). However, in the BCB- oocytes supplemented with DKK1, the cytoplasmic Cx43 and β-catenin levels are reportedly restored which assists in the formation of gap junctions leading to enhanced cumulus expansion. The supplementation of DKK1 also improved the mean percentage of fertilized oocytes reaching the morula and blastocyst stages in BCB- oocytes (Table 1). Nonetheless, there was no significant difference in the percentage of oocytes that were cleaved or reached the 8–16 cell stage between the BCB-control and BCB- oocytes supplemented with DKK1. It has been reported that DKK1 is less effective in situations where the Wnt activity is essential for cell survival (39, 40). Besides, it has been established that the continuous activation of the Wnt signaling pathway in oocytes does not affect its functions, e.g., folliculogenesis, however, negatively affects blastocyst implantation and embryonic and fetal development (66, 67). Our results are also supported by similar studies which have reported that an activated Wnt signaling led to reduced hatching of blastocysts and a lesser number of nuclei per blastocyst (68). We observed a significant reduction in the level of β-catenin (and Dvl1) gene expression in BCB- oocytes upon DKK1 supplementation. It has been reported that embryos fail to develop beyond the 2-cell stage due to atypical expression of β-catenin (69). In the case of BCB- oocytes, an overactive Wnt signal may cause early translocation of β-catenin inside the nucleus which can cause transcriptional activation of genes like Tap63 which are implicated in determining the fate in oocytes of primordial follicles (70, 71). However, this aspect needs further investigation to establish the effect of DKK1 supplementation on BCB- oocytes.
DKK1 supplementation improved the percentage of BCB- oocytes that matured to the metaphase II stage (Table 2). Our results corroborate the findings in pigs wherein DKK1 supplementation improved the in vitro nuclear maturation rate of oocytes (43). It is highly likely that DKK1 supplementation of BCB- oocytes improves the nuclear maturation of these oocytes by accelerating the downstream fatty acid oxidation in poor-quality (BCB-) COCs (72), nonetheless, further studies are warranted to establish this effect. It has been reported that exposure of bovine embryos to DKK1 results in elevated differentiation of embryonic cells toward the trophectoderm and hypoblast lineages, however, it does not promote cell proliferation in early embryos (40). No effect on blastocyst health (ICM/TE ratio) of BCB- oocytes upon DKK1 supplementation was observed in this study. Our results from differential staining along with the results of IVEP experiments indicate that although DKK1 is required for blastocyst development, it does not regulate ICM proliferation (40, 73).
Based on the CEI score and increased expression of GDF9, HAS2, and PTGS2 upon Wnt inhibition, we inferred that DKK1 helps the poor-quality (BCB-) buffalo oocytes to gain competence. Our data is in line with the previous findings that reported the maximum expression of these genes during the mid-maturation phase (52). However, to understand the reason behind the increased oocyte maturation of BCB- oocytes supplemented with DKK1, we considered the two other important milestones of embryonic development, i.e., GVBD and EGA (Figure 5). The inhibition of the Wnt pathway by DKK1 assists BCB- oocytes to synchronize their timing of GVBD with that of BCB+ oocytes (57, 67). A cell surface receptor for DKK1, CKAP4 (Cytoskeleton associated protein 4), could be implicated in this process. It is now known that upon binding with the DKK1, CKAP4 recruits PI3K to its cytosolic domain which promotes Akt signaling thereby triggering the event of GVBD in BCB- oocytes (74). Besides, the genes elF1A, PAP, and U2AF are considered authentic indicators of EGA and are involved in the DNA and RNA metabolism in oocytes (75). These genes exhibited minimal expression in BCB- (control) oocytes, which increased upon DKK1 supplementation during the 4–8 cell stage which is the time when major embryonic genome activation takes place in buffaloes (75). During EGA, the endogenous molecules responsible for inhibiting canonical Wnt signaling are already present. Additionally, the calcium fluctuations at the time of fertilization also inhibit the canonical Wnt signaling (76). The combined effect of endogenous molecules along with the exogenous supplementation of DKK1 might be responsible for overcoming the negative effects of hyperactive Wnt signaling and hence helping the BCB- oocytes to achieve the milestone of MET earlier than the BCB- control group (57, 67).
The presented results confirm the presence of an overactive Wnt signaling pathway in BCB- oocytes and strengthen the hypothesis that hyperactive Wnt signaling perturbs the development potential of poor-quality oocytes. Transient inhibition of Wnt signaling using DKK1 during the initial course of oocyte maturation can prove to be a feasible strategy for attaining better embryonic development in poor-quality buffalo oocytes in the IVEP regimen.
Data availability statement
The original contributions presented in the study are included in the article/Supplementary material, further inquiries can be directed to the corresponding author.
Ethics statement
Ethical approval was not required for the study involving animals in accordance with the local legislation and institutional requirements because The study was performed on samples procured from an abattoir.
Author contributions
KA: Conceptualization, Data curation, Investigation, Methodology, Validation, Visualization, Writing – original draft, Writing – review & editing. VB: Investigation, Methodology, Visualization, Writing – original draft, Writing – review & editing. RK: Conceptualization, Project administration, Resources, Supervision, Writing – review & editing. TD: Conceptualization, Data curation, Funding acquisition, Investigation, Project administration, Resources, Supervision, Writing – review & editing.
Funding
The author(s) declare financial support was received for the research, authorship, and/or publication of this article. This work was supported by the Bill & Melinda Gates Foundation [grant number OPP1154401].
Conflict of interest
The authors declare that the research was conducted in the absence of any commercial or financial relationships that could be construed as a potential conflict of interest.
Publisher’s note
All claims expressed in this article are solely those of the authors and do not necessarily represent those of their affiliated organizations, or those of the publisher, the editors and the reviewers. Any product that may be evaluated in this article, or claim that may be made by its manufacturer, is not guaranteed or endorsed by the publisher.
Supplementary material
The Supplementary material for this article can be found online at: https://www.frontiersin.org/articles/10.3389/fvets.2023.1324647/full#supplementary-material
References
1. Monteiro, BM, Souza, DC, Vasconcellos, GSFM, Carvalho, NAT, and Baruselli, PS. Effect of season on dairy buffalo reproductive performance when using P4/E2/eCG-based fixed-time artificial insemination management. Theriogenology. (2018) 119:275–81. doi: 10.1016/j.theriogenology.2018.07.004
2. Otava, G, Squicciarini, S, Marc, S, Suici, T, William Onan, G, Hutu, I, et al. Effects of age and season on conception rate of Mediterranean Italian dairy Buffalo (Bubalus bubalis) following oestrus synchronization and fixed-time artificial insemination. Reproduc Domes Anim Zuchthygiene. (2021) 56:1511–8. doi: 10.1111/rda.14013
3. Ciornei, SG, and Roşca, P. Upgrading the fixed-time artificial insemination (FTAI) protocol in Romanian buffaloes. Front Vet Sci. (2023) 10:1265060. doi: 10.3389/fvets.2023.1265060
4. Warriach, HM, McGill, DM, Bush, RD, Wynn, PC, and Chohan, KR. A review of recent developments in buffalo reproduction - a review. Asian Australas J Anim Sci. (2015) 28:451–5. doi: 10.5713/ajas.14.0259
5. Marin, DFD, de Souza, EB, de Brito, VC, Nascimento, CV, Ramos, AS, Filho, STR, et al. In vitro embryo production in buffaloes: from the laboratory to the farm. Anim Reprod. (2019) 16:260–6. doi: 10.21451/1984-3143-AR2018-0135
6. Srirattana, K, Hufana-Duran, D, Atabay, EP, Duran, PG, Atabay, EC, Lu, K, et al. Current status of assisted reproductive technologies in buffaloes. Anim Sci J= Nihon chikusan Gakkaiho. (2022) 93:e13767. doi: 10.1111/asj.13767
7. Kumar, S, da Silva, AFB, Vale, WG, Filho, STR, Ferreira-Silva, JC, Melo, LM, et al. Factors affecting the in vitro embryo production in buffalo (Bubalus bubalis): a review Veterinární medicína. Czech Acad Agric Sci. (2023) 68:45–56. doi: 10.17221/48/2022-VETMED
8. Rubessa, M, Boccia, L, and Di Francesco, S. In vitro embryo production in Buffalo species (Bubalus bubalis). Methods Mol Biol. (2019) s:179–90. doi: 10.1007/978-1-4939-9566-0_13
9. Currin, L, Baldassarre, H, de Macedo, MP, Glanzner, WG, Gutierrez, K, Lazaris, K, et al. Factors affecting the efficiency of in vitro embryo production in Prepubertal Mediterranean water Buffalo. Animals Open Acc J MDPI. (2022) 12:3549. doi: 10.3390/ani12243549
10. Karami Shabankareh, H, Azimi, G, and Torki, M. Developmental competence of bovine oocytes selected based on follicle size and using the brilliant cresyl blue (BCB) test. Iran J Reproduc Med. (2014) 12:771–8.
11. Sadeesh, EM, Sikka, P, Balhara, AK, and Balhara, S. Developmental competence and expression profile of genes in buffalo (Bubalus bubalis) oocytes and embryos collected under different environmental stress. Cytotechnology. (2016) 68:2271–85. doi: 10.1007/s10616-016-0022-y
12. Abdoon, AS, Kandil, OM, Otoi, T, and Suzuki, T. Influence of oocyte quality, culture media and gonadotropins on cleavage rate and development of in vitro fertilized buffalo embryos. Anim Reprod Sci. (2001) 65:215–23. doi: 10.1016/s0378-4320(01)00079-3
13. Amer, HA, Hegab, AR, and Zaabal, SM. Some studies on the morphological aspects of buffalo oocytes in relation to the ovarian morphology and culture condition. In vitro cellular & developmental biology. Animal. (2009). doi: 10.1007/s11626-009-9224-3
14. Baruselli, PS, Soares, JG, Bayeux, BM, Silva, JCB, Mingoti, RD, and Carvalho, NAT. Assisted reproductive technologies (ART) in water buffaloes. Anim Reprod. (2018) 15:971–83. doi: 10.21451/1984-3143-AR2018-0043
15. Egerszegi, I, Alm, H, Rátky, J, Heleil, B, Brüssow, KP, and Torner, H. Meiotic progression, mitochondrial features and fertilisation characteristics of porcine oocytes with different G6PDH activities. Reprod Fertil Dev. (2010) 22:830–8. doi: 10.1071/RD09140
16. Ashry, M, Lee, K, Mondal, M, Datta, TK, Folger, JK, Rajput, SK, et al. Expression of TGFβ superfamily components and other markers of oocyte quality in oocytes selected by brilliant cresyl blue staining: relevance to early embryonic development. Mol Reprod Dev. (2015) 82:251–64. doi: 10.1002/mrd.22468
17. Landeo, L, Zuñiga, M, Gastelu, T, Artica, M, Ruiz, J, Silva, M, et al. Oocyte quality, in vitro fertilization and embryo development of alpaca oocytes collected by ultrasound-guided follicular aspiration or from slaughterhouse ovaries. Animals. (2022) 12:1102. doi: 10.3390/ani12091102
18. Bhojwani, S, Alm, H, Torner, H, Kanitz, W, and Poehland, R. Selection of developmentally competent oocytes through brilliant cresyl blue stain enhances blastocyst development rate after bovine nuclear transfer. Theriogenology. (2007) 67:341–5. doi: 10.1016/j.theriogenology.2006.08.006
19. Su, J, Wang, Y, Li, R, Peng, H, Hua, S, Li, Q, et al. Oocytes selected using BCB staining enhance nuclear reprogramming and the in vivo development of SCNT embryos in cattle. PLoS One. (2012) 7:e36181. doi: 10.1371/journal.pone.0036181
20. Lee, S, Kang, HG, Jeong, PS, Nanjidsuren, T, Song, BS, Jin, YB, et al. Effect of oocyte quality assessed by brilliant Cresyl blue (BCB) staining on cumulus cell expansion and sonic hedgehog signaling in porcine during in vitro maturation. Int J Mol Sci. (2020) 21:4423. doi: 10.3390/ijms21124423
21. Fu, B, Ren, L, Liu, D, Ma, JZ, An, TZ, Yang, XQ, et al. Subcellular characterization of porcine oocytes with different Glucose-6-phosphate dehydrogenase activities. Asian Australas J Anim Sci. (2015) 28:1703–12. doi: 10.5713/ajas.15.0051
22. Bragança, GM, Batista, RITP, Souza-Fabjan, JMG, Alfradique, VAP, Arashiro, EKN, Pinto, PHN, et al. Exogenous progestogens differentially alter gene expression of immature cumulus-oocyte complexes in sheep. Domest Anim Endocrinol. (2021) 74:106518. doi: 10.1016/j.domaniend.2020.106518
23. Mohapatra, SK, Sandhu, A, Neerukattu, VS, Singh, KP, Selokar, NL, Singla, SK, et al. Buffalo embryos produced by handmade cloning from oocytes selected using brilliant cresyl blue staining have better developmental competence and quality and are closer to embryos produced by in vitro fertilization in terms of their epigenetic status and gene expression pattern. Cell Reprogram. (2015) 17:141–50. doi: 10.1089/cell.2014.0077
24. O'Shea, LC, Mehta, J, Lonergan, P, Hensey, C, and Fair, T. Developmental competence in oocytes and cumulus cells: candidate genes and networks. Syst Biol Reprod Med. (2012) 58:88–01. doi: 10.3109/19396368.2012.656217
25. Zhang, Q, and Yan, J. Update of Wnt signaling in implantation and decidualization. Reproduc Med Biol. (2015) 15:95–05. doi: 10.1007/s12522-015-0226-4
26. Liu, J, Xiao, Q, Xiao, J, Niu, C, Li, Y, Zhang, X, et al. Wnt/β-catenin signalling: function, biological mechanisms, and therapeutic opportunities. Signal Transduct Target Ther. (2022) 7:3. doi: 10.1038/s41392-021-00762-6
27. Sidrat, T, Khan, AA, Idrees, M, Joo, MD, Xu, L, Lee, KL, et al. Role of Wnt signaling during in-vitro bovine blastocyst development and maturation in synergism with PPARδ signaling. Cells. (2020) 9:923. doi: 10.3390/cells9040923
28. Yang, Y . Wnt signaling in development and disease. Cell Biosci. (2012) 2:14. doi: 10.1186/2045-3701-2-14
29. Steinhart, Z, and Angers, S. Wnt signaling in development and tissue homeostasis. Development. (2018) 145:dev146589. doi: 10.1242/dev.146589
30. Borday, C, Parain, K, Thi Tran, H, Vleminckx, K, Perron, M, and Monsoro-Burq, AH. An atlas of Wnt activity during embryogenesis in Xenopus tropicalis. PLoS One. (2018) 13:e0193606. doi: 10.1371/journal.pone.0193606
31. Mehta, S, Hingole, S, and Chaudhary, V. The emerging mechanisms of Wnt secretion and signaling in development. Front Cell Dev Biol. (2021) 9:714746. doi: 10.3389/fcell.2021.714746
32. MacDonald, BT, Tamai, K, and He, X. Wnt/beta-catenin signaling: components, mechanisms, and diseases. Dev Cell. (2009) 17:9–26. doi: 10.1016/j.devcel.2009.06.016
33. Cruciat, CM, and Niehrs, C. Secreted and transmembrane wnt inhibitors and activators. Cold Spring Harb Perspect Biol. (2013) 5:a015081. doi: 10.1101/cshperspect.a015081
34. Tepekoy, F, Akkoyunlu, G, and Demir, R. The role of Wnt signaling members in the uterus and embryo during pre-implantation and implantation. J Assist Reprod Genet. (2015) 32:337–46. doi: 10.1007/s10815-014-0409-7
35. Zheng, S, Liu, J, Wu, Y, Huang, TL, and Wang, G. Small-molecule inhibitors of Wnt signaling pathway: towards novel anticancer therapeutics. Future Med Chem. (2015) 7:2485–05. doi: 10.4155/fmc.15.159
36. Reinhold, S, and Blankesteijn, WM. Wnt/β-catenin inhibitor Dickkopf 1. Arterioscler Thromb Vasc Biol. (2019) 39:121–3. doi: 10.1161/ATVBAHA.118.312144
37. Li, Y, Lu, W, King, TD, Liu, CC, Bijur, GN, et al. Dkk1 stabilizes Wnt co-receptor LRP6: implication for Wnt ligand-induced LRP6 down-regulation. PLoS One. (2010) 5:e11014. doi: 10.1371/journal.pone.0011014
38. Lieven, O, Knobloch, J, and Rüther, U. The regulation of DKK1 expression during embryonic development. Dev Biol. (2010) 340:256–68. doi: 10.1016/j.ydbio.2010.01.037
39. Denicol, AC, Dobbs, KB, McLean, KM, Carambula, SF, Loureiro, B, and Hansen, PJ. Canonical WNT signaling regulates development of bovine embryos to the blastocyst stage. Sci Rep. (2013) 3:1266. doi: 10.1038/srep01266
40. Denicol, AC, Block, J, Kelley, DE, Pohler, KG, Dobbs, KB, Mortensen, CJ, et al. The WNT signaling antagonist Dickkopf-1 directs lineage commitment and promotes survival of the preimplantation embryo. FASEB J. (2014) 28:3975–86. doi: 10.1096/fj.14-253112
41. Shyam, S, Goel, P, Kumar, D, Malpotra, S, Singh, MK, Lathwal, SS, et al. Effect of Dickkopf-1 and colony stimulating factor-2 on the developmental competence, quality, gene expression and live birth rate of buffalo (Bubalus bubalis) embryos produced by hand-made cloning. Theriogenology. (2020) 157:254–62. doi: 10.1016/j.theriogenology.2020.07.022
42. Amaral, TF, de Grazia, JGV, Martinhao, LAG, De Col, F, Siqueira, LGB, Viana, JHM, et al. Actions of CSF2 and DKK1 on bovine embryo development and pregnancy outcomes are affected by composition of embryo culture medium. Sci Rep. (2022) 12:7503. doi: 10.1038/s41598-022-11447-7
43. Spate, LD, Brown, AN, Redel, BK, Whitworth, KM, Murphy, CN, and Prather, RS. Dickkopf-related protein 1 inhibits the WNT signaling pathway and improves pig oocyte maturation. PLoS One. (2014) 9:e95114. doi: 10.1371/journal.pone.0095114
44. Chaubey, GK, Kumar, S, Kumar, M, Sarwalia, P, Kumaresan, A, De, S, et al. Induced cumulus expansion of poor quality buffalo cumulus oocyte complexes by interleukin-1beta improves their developmental ability. J Cell Biochem. (2018) 119:5750–60. doi: 10.1002/jcb.26688
45. Kibbe, WA . OligoCalc: an online oligonucleotide properties calculator. Nucleic Acids Res. (2007) 35:W43–6. doi: 10.1093/nar/gkm234
46. Gasteiger, E, Gattiker, A, Hoogland, C, Ivanyi, I, Appel, RD, and Bairoch, A. ExPASy: the proteomics server for in-depth protein knowledge and analysis. Nucleic Acids Res. (2003) 31:3784–8. doi: 10.1093/nar/gkg563
47. Kuhn, RM, Haussler, D, and Kent, WJ. The UCSC genome browser and associated tools. Brief Bioinform. (2013) 14:144–61. doi: 10.1093/bib/bbs038
48. Bustin, SA, Benes, V, Garson, JA, Hellemans, J, Huggett, J, Kubista, M, et al. The MIQE guidelines: minimum information for publication of quantitative real-time PCR experiments. Clin Chem. (2009) 55:611–22. doi: 10.1373/clinchem.2008.112797
49. Livak, KJ, and Schmittgen, TD. Analysis of relative gene expression data using real-time quantitative PCR and the 2(-Delta Delta C(T)). Method Methods. (2001) 25:402–8. doi: 10.1006/meth.2001.1262
50. Willert, K, and Nusse, R. Beta-catenin: a key mediator of Wnt signaling. Curr Opin Genet Dev. (1998) 8:95–02. doi: 10.1016/s0959-437x(98)80068-3
51. Batra, V, Bhushan, V, Ali, SA, Sarwalia, P, Pal, A, Karanwal, S, et al. Buffalo sperm surface proteome profiling reveals an intricate relationship between innate immunity and reproduction. BMC Genomics. (2021) 22:480. doi: 10.1186/s12864-021-07640-z
52. Jain, T, Jain, A, Kumar, P, Goswami, SL, De, S, Singh, D, et al. Kinetics of GDF9 expression in buffalo oocytes during in vitro maturation and their associated development ability. Gen Comp Endocrinol. (2012) 178:477–84. doi: 10.1016/j.ygcen.2012.07.001
53. Kumar, S, Kumar, M, Dholpuria, S, Sarwalia, P, Batra, V, De, S, et al. Transient arrest of germinal vesicle breakdown improved in vitro development potential of Buffalo (Bubalus Bubalis) oocytes. J Cell Biochem. (2018) 119:278–89. doi: 10.1002/jcb.26171
54. Takezawa, Y, Yoshida, K, Miyado, K, Sato, M, Nakamura, A, Kawano, N, et al. β-Catenin is a molecular switch that regulates transition of cell-cell adhesion to fusion. Sci Rep. (2011) 1:68. doi: 10.1038/srep00068
55. Grupen, CG, and Armstrong, DT. Relationship between cumulus cell apoptosis, progesterone production and porcine oocyte developmental competence: temporal effects of follicular fluid during IVM. Reprod Fertil Dev. (2010) 22:1100–9. doi: 10.1071/RD09307
56. Conti, M, and Franciosi, F. Acquisition of oocyte competence to develop as an embryo: integrated nuclear and cytoplasmic events. Hum Reprod Update. (2018) 24:245–6. doi: 10.1093/humupd/dmx040
57. Kumar, M, Faraji, M, Sarwalia, P, Kumar, S, Gohain, M, De, S, et al. Propensity in low-grade oocytes for delayed germinal vesicle breakdown compromises the developmental ability of sub-optimal grade Bubalus bubalis oocytes. Zygote (Cambridge, England). (2018) 26:359–65. doi: 10.1017/S0967199418000321
58. Bandmann, V, Mirsanaye, AS, Schäfer, J, Thiel, G, Holstein, T, and Mikosch-Wersching, M. Membrane capacitance recordings resolve dynamics and complexity of receptor-mediated endocytosis in Wnt signalling. Sci Rep. (2019) 9:12999. doi: 10.1038/s41598-019-49082-4
59. Liu, X, Hao, Y, Li, Z, Zhou, J, Zhu, H, Bu, G, et al. Maternal cytokines CXCL12, VEGFA, and WNT5A promote porcine oocyte maturation via MAPK activation and canonical WNT inhibition. Front Cell Dev Biol. (2020) 8:578. doi: 10.3389/fcell.2020.00578
60. Boruszewska, D, Sinderewicz, E, Kowalczyk-Zieba, I, Grycmacher, K, and Woclawek-Potocka, I. The effect of lysophosphatidic acid during in vitro maturation of bovine cumulus-oocyte complexes: cumulus expansion, glucose metabolism and expression of genes involved in the ovulatory cascade, oocyte and blastocyst competence. Reproduc Biol Endocrinol. (2015) 13:44. doi: 10.1186/s12958-015-0044-x
61. Hwang, SU, Yoon, JD, Kim, M, Cai, L, Choi, H, Oh, D, et al. R-Spondin 2 and WNT/CTNNB1 signaling pathways are required for porcine follicle development and in vitro maturation. Animals. (2021) 11:709. doi: 10.3390/ani11030709
62. Regassa, A, Rings, F, Hoelker, M, Cinar, U, Tholen, E, Looft, C, et al. Transcriptome dynamics and molecular cross-talk between bovine oocyte and its companion cumulus cells. BMC Genomics. (2011) 12:57. doi: 10.1186/1471-2164-12-57
63. Luciano, AM, Franciosi, F, Modina, SC, and Lodde, V. Gap junction-mediated communications regulate chromatin remodeling during bovine oocyte growth and differentiation through cAMP-dependent mechanism(s). Biol Reprod. (2011) 85:1252–9. doi: 10.1095/biolreprod.111.092858
64. Wang, HX, Gillio-Meina, C, Chen, S, Gong, XQ, Li, TY, Bai, D, et al. The canonical WNT2 pathway and FSH interact to regulate gap junction assembly in mouse granulosa cells. Biol Reprod. (2013) 89:39. doi: 10.1095/biolreprod.113.109801
65. Hou, X, Khan, MRA, Turmaine, M, Thrasivoulou, C, Becker, DL, and Ahmed, A. Wnt signaling regulates cytosolic translocation of connexin 43. Am J Physiol Regul Integr Comp Physiol. (2019) 317:R248–61. doi: 10.1152/ajpregu.00268.2018
66. Xie, H, Tranguch, S, Jia, X, Zhang, H, Das, SK, Dey, SK, et al. Inactivation of nuclear Wnt-beta-catenin signaling limits blastocyst competency for implantation. Development. 135:717–27. doi: 10.1242/dev.015339
67. Kumar, M, Camlin, NJ, Holt, JE, Teixeira, JM, McLaughlin, EA, and Tanwar, PS. Germ cell specific overactivation of WNT/βcatenin signalling has no effect on folliculogenesis but causes fertility defects due to abnormal foetal development. Sci Rep. (2016) 6:27273. doi: 10.1038/srep27273
68. Lim, KT, Gupta, MK, Lee, SH, Jung, YH, Han, DW, and Lee, HT. Possible involvement of Wnt/β-catenin signaling pathway in hatching and trophectoderm differentiation of pig blastocysts. Theriogenology. (2013) 79:284–290.e2. doi: 10.1016/j.theriogenology.2012.08.018
69. Yu, J, Guo, X, Chen, X, Qiao, C, Chen, B, Xie, B, et al. Activation of β-catenin causes defects in embryonic development during maternal-to-zygotic transition in mice. Int J Clin Exp Pathol. (2018) 11:2514–21.
70. Wen, J, Yan, H, He, M, Zhang, T, Mu, X, Wang, H, et al. GSK-3β protects fetal oocytes from premature death via modulating TAp63 expression in mice. BMC Biol. (2019) 17:23. doi: 10.1186/s12915-019-0641-9
71. Luan, Y, Yu, SY, Abazarikia, A, Dong, R, and Kim, SY. TAp63 determines the fate of oocytes against DNA damage. Sci Adv. (2022) 8:eade1846. doi: 10.1126/sciadv.ade1846
72. Dunning, KR, Anastasi, MR, Zhang, VJ, Russell, DL, and Robker, RL. Regulation of fatty acid oxidation in mouse cumulus-oocyte complexes during maturation and modulation by PPAR agonists. PLoS One. (2014) 9:e87327. doi: 10.1371/journal.pone.0087327
73. Tribulo, P, Leão, BCDS, Lehloenya, KC, Mingoti, GZ, and Hansen, PJ. Consequences of endogenous and exogenous WNT signaling for development of the preimplantation bovine embryo. Biol Reprod. (2017) 96:1129–41. doi: 10.1093/biolre/iox048
74. Kikuchi, A, Fumoto, K, and Kimura, H. The Dickkopf1-cytoskeleton-associated protein 4 axis creates a novel signalling pathway and may represent a molecular target for cancer therapy. Br J Pharmacol. (2017) 174:4651–65. doi: 10.1111/bph.13863
75. Verma, A, Kumar, P, Rajput, S, Roy, B, De, S, and Datta, TK. Embryonic genome activation events in buffalo (Bubalus bubalis) preimplantation embryos. Mol Reprod Dev. (2012) 79:321–8. doi: 10.1002/mrd.22027
Keywords: Brilliant Cresyl Blue (BCB) screening, oocyte quality and competence, Wnt pathway, in vitro embryo production (IVEP), buffalo
Citation: Ahuja K, Batra V, Kumar R and Datta TK (2024) Transient suppression of Wnt signaling in poor-quality buffalo oocytes improves their developmental competence. Front. Vet. Sci. 10:1324647. doi: 10.3389/fvets.2023.1324647
Edited by:
Mehdi Hajian, Royan Institute, IranReviewed by:
Stefan Gregore Ciornei, Ion Ionescu de la Brad University of Agricultural Sciences and Veterinary Medicine of Iași, Romania Fabian Diaz, Louisiana State University Agricultural Center, United StatesCopyright © 2024 Ahuja, Batra, Kumar and Datta. This is an open-access article distributed under the terms of the Creative Commons Attribution License (CC BY). The use, distribution or reproduction in other forums is permitted, provided the original author(s) and the copyright owner(s) are credited and that the original publication in this journal is cited, in accordance with accepted academic practice. No use, distribution or reproduction is permitted which does not comply with these terms.
*Correspondence: Tirtha Kumar Datta, dGlydGhhLmRhdHRhQGljYXIuZ292Lmlu
†These authors have contributed equally to this work and share first authorship