- 1Department of Small Animal Medicine and Surgery, University of Veterinary Medicine Hannover, Foundation, Hannover, Germany
- 2Department of Pharmacology, Toxicology and Pharmacy, University of Veterinary Medicine Hannover, Foundation, Hannover, Germany
- 3Department of Microbiology, University of Veterinary Medicine Hannover, Foundation, Hannover, Germany
- 4Department of Ophthalmology, University of Rostock, Rostock, Germany
Purpose: To investigate the antimicrobial effect of cold atmospheric plasma (CAP) on pathogens associated with canine bacterial keratitis.
Materials and methods: Pseudomonas aeruginosa, Staphylococcus pseudintermedius, and Streptococcus canis strains, which were obtained from dogs with infectious keratitis, were subjected to testing. For each species, four isolates and a reference strain were cultivated on Columbia sheep blood agar and treated with the kiNPen Vet® plasma pen from Neoplas GmbH, Greifswald, Germany. Various continuous treatment durations (0.5, 2, and 5 min) were applied, along with a 0.5-min treatment repeated four times at short intervals. These treatments were conducted at distances of 3 and 18 mm between the agar surface and the pen.
Results: CAP treatment reduced bacterial growth in all three species. The most effective treatment duration was 5 min at 3 mm distance, resulting in inhibition zones ranging from 19 to 22 mm for P. aeruginosa, 26–45 mm for S. pseudintermedius and an overall reduction of bacterial growth for Str. canis. Inhibition zones were smaller with decreasing treatment duration and larger distance. Treatment times of 30 s repeated four times and 2 min showed comparable results. Treatment with argon alone did not lead to visible reduction of bacterial growth.
Conclusion: Argon cold atmospheric plasma demonstrated a potent in vitro antimicrobial effect on P. aeruginosa, S. pseudintermedius and Str. canis strains with the latter showing the highest sensitivity.
Introduction
Cold atmospheric plasma (CAP) is a relatively new tool in medicine that has gained much attention due to its biological effects on micro-organisms and living tissue – capable of stimulating wound healing processes in eucaryotic cells while eradicating prokaryotic cells (1, 2).
CAP is a partially ionized gas that consists of charged and neutral molecules, electromagnetic fields, free reactive molecules and is capable of emitting radiation in visible and ultraviolet range (3). CAP, also termed non-thermal plasma, operates at temperatures tolerated well by mammalian tissue. The most relevant plasma sources in medicine include dielectric barrier discharges and atmospheric pressure plasma jets (1, 4). The principle of the latter device is based on ionization of a working gas in between two electrodes. The components generated during this process are ejected from the pen by the gas flow of the working gas in form of a plasma jet (5). Exposure to this ‘plasma cocktail’ to biological targets generates reactive oxygen and nitrous species (ROS, RNS) which play a dominant role in the impact on the targets (6). ROS and RNS, which are important mediators in signaling processes, will stimulate redox-related processes within cells (7), or can cause cellular damage when reaching concentrations exceeding antioxidative capacities by inducing oxidative stress (8).
Current applications in the medical field mainly comprise therapy of chronic non-healing wounds (9, 10) and treatment of wound infections including multi-drug resistant infections (11). Identified mechanisms of antibacterial effects involve damage to the cell membrane or wall, to intracellular proteins, and DNA (1).
Newer fields of interest include cancer therapy (12, 13), dentistry (14) and ophthalmology (15). Many studies show that CAP can induce apoptosis of cancer cells in vitro (16–18). In dentistry, much attention was gained due to antibacterial efficacy of CAP (19–21). In human ophthalmology, CAP is an area of interest also due to its strong antimicrobial effect, demonstrated against various ocular pathogens, including bacteria (2, 22, 23), fungi (24), protozoa (25) and viruses (26), making it a potential device for the therapy of infectious keratitis. The safety of application of plasma jets was studied and did not cause significant damage to ocular tissue depending on dosage (15, 27). Reitberger et al published the first case series demonstrating the application of argon CAP in the therapy of infectious keratitis in people refractive to standard therapy. Adding argon CAP to the treatment regimen resulted in a substantial improvement of disease after the third or fourth application (15).
Infectious ulcerative keratitis is a common disease in small animals (28). Many corneal pathogens have been identified to be associated with the disease, the majority of isolated bacteria however belong to Pseudomonas aeruginosa, Staphylococcus pseudintermedius and Streptococcus canis species (29–36). The therapy of choice is topical antimicrobial treatment (37, 38). If treatment fails to control infection, for instance either due to incorrect choice of the antimicrobial agent or the involvement of a multidrug resistant bacterial isolate, the disease can rapidly progress with sight and globe threatening consequences (28, 37). Staphylococcus species isolated from dogs with infectious keratitis frequently include methicillin-resistant isolates (39). Rising concerns about the development of antimicrobial resistances among canine corneal pathogens against commonly used antibiotic agents (30, 38, 40) and the restrictive legal use of antibiotics by legislation within the European Union (41), highlight the importance of establishing alternative antimicrobial treatment modalities. We therefore investigated the antibacterial efficacy of argon CAP against corneal pathogens isolated from canine patients with bacterial keratitis.
Materials and methods
Bacterial isolates
Clinical isolates were collected by a laboratory for clinical diagnostics (LABOKLIN GmbH & Ko KG, Bad Kissingen, Germany). Swap samples were obtained from canine patients diagnosed with bacterial ulcerative keratitis for the purpose of species identification and antimicrobial resistance testing. Four field isolates were chosen from each of the following bacteria: Staphylococcus pseudintermedius (SP), Streptococcus canis (SC) and Pseudomonas aeruginosa (PA). For each bacteria a reference strain was included in the study (DSM 20373, DSM 20716, DSM 10880). Inclusion criteria for all isolates included the presence of a stromal ulcer at the time of sampling and available antimicrobial susceptibility profiles, shown in Table 1. We included two methicillin-resistant and two non-methicillin-resistant isolates of SP. In the case of PA included isolates were either resistant or susceptible to difloxacin and orbifloxacin, each group was also represented by two isolates. Antimicrobial susceptibility patterns among included SC isolates were similar.
Plasma source
Experiments were conducted by using a modified kINPen®Vet manufactured by Neoplas ® GmbH, Greifswald, Germany, which consists of an operating device, a gas supply line and the hand-pen. Plasma generation takes place in the hand-pen in-between electrodes with a high-frequency voltage (1.0 MHz, 2–3 kV) when argon gas at a flow rate of 4–6 SLM is applied. This results in plasma expelled in form of a plasma jet, visible as a beam of approximately 1 cm length.
The device was equipped with an additional switch to interrupt plasma emission. Plasma is generated inside the pen during ionization of argon gas with a high-frequency voltage and is then pink spot beam of about 1 cm in length at the tip of the pen. A transparent spacer that keeps a minimum distance of 7 mm between the tip of the pen and the treated surface was placed (Figure 1).
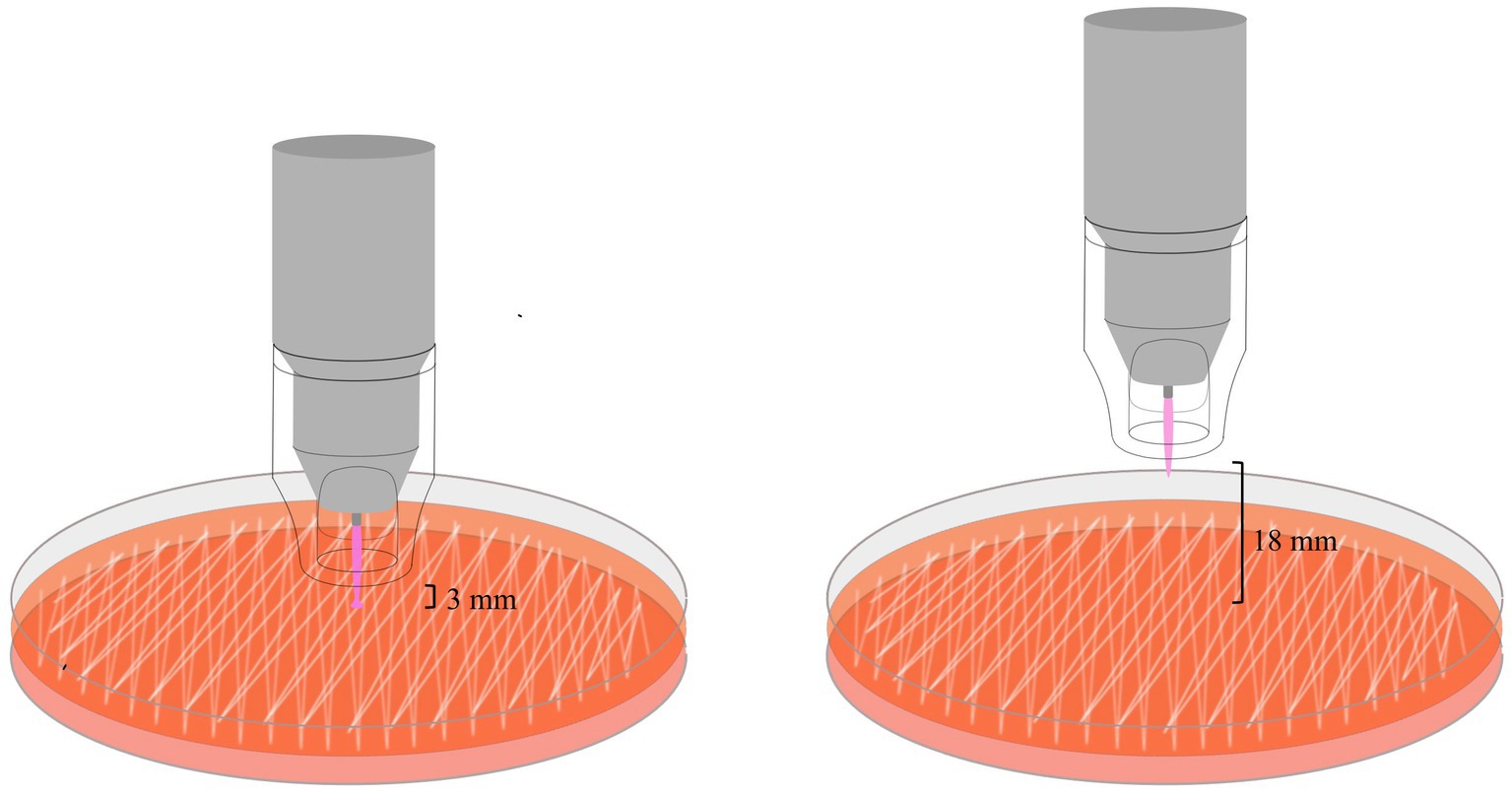
Figure 1. The schematic illustration shows the exposure of bacteria on agar plates with the plasma pen at a distance between the spacer and the agar surface of either 3 or 18 mm. Note the conducting effect of the plasma beam in the left image – the intensity of the light beam increases and the light spreads over a small portion of the treated area. This happens when the plasma directly hits a conductive surface.
The gas flow rate was adjusted to 4.1 SLM at a constant gas pressure of 2.5 bar (recommended pressure by the manufacturer is 2–3 bar). We decided to decrease the gas flow rate as much as the device allowed because the gas flow rate of 5 SLM that was used in human patients (15) resulted in desiccation of the agar surface (observations during preliminary experiments). Modification of the device allowed turning off the production of plasma without modifying the gas flow rate.
Temperature measurement
To evaluate whether to expect thermal effects on bacterial growth, the temperature of the plasma jet was measured at the tip of the plasma jet via a contact k-type element of a digital thermometer (MESTEK 800C®). The contact element was exposed to the tip of the plasma jet for 5 min and measurements were taken every minute.
Experiments
Bacterial isolates were routinely cultured on Columbia blood agar (with 7% Sheep Blood; OXOID) for an incubation period of 20–24 h at 37°C. A bacterial suspension was prepared using an optical densitometer (McFarland = 1.0). A further 10-fold dilution of the suspension was performed to culture isolates for the experiments since this resulted in a uniform growth of bacteria on the agar plates. The same suspension was used for all experiments with the same isolate to eliminate falsifications resulting from variations in bacterial concentration. Two serial dilutions were made for each suspension by spreading 100 μL of suspension onto agar plates in duplicates.
Inoculated plates were treated with either argon gas or plasma for 0.5, 2, 5, and 4 × 0.5 min (with a pause of 0.25 min in between, which is the approximate time needed to apply a lubricant eye drop). Treatment times were selected based on results from the study by Reitberger et al. (15), showing antimicrobial effects following treatment of human corneal pathogens for 2, 5, 7 and 10 min using the same device. We considered a treatment of the corneal surface of small animals for 7 and 10 min as clinically not applicable with the current device and did not include treatments exceeding 5 min. Furthermore, in the previously mentioned study a treatment time of 0.5 min was selected for the application on in vivo experiments. We therefore decided to include 0.5 min in our protocol. An overview of the treatment protocol can be seen in Figure 1.
The plasma pen was stationary and the distance between the spacer and the plate was set to 3 or 18 mm. A distance of 3 mm was the largest distance that still resulted in contact between the plasma beam and the surface, creating a conductive mode. We wanted to investigate the effects of a larger distance that would avoid direct contact of the jet potentially reducing the effects of gas flow on corneal tissue. Preliminary studies (unpublished) did not show consistent results with the previously used distance of 5 cm. A distance of 2 cm resulted in less drying effects (unpublished data) and for technical reasons a distance of 18 mm was used. As a control, all protocols were repeated with argon gas alone. All agar plates were incubated for 19–21 h at 37°C. Serial dilutions were determined by counting colony forming units (CFUs). Treated plates were evaluated for inhibition zones that were measured using a stainless steel vernier caliper (mm). In the case of circular shaped inhibition zones the diameter was measured, in irregular shaped zones two measurements were taken – one at the widest and one at the smallest width of the zone. In the case of ill-defined margins, zones where a reduction of bacterial growth was visible were measured. Overall reduction of bacterial growth was recorded, but not further classified. Experiments were performed in duplicates and results are given as mean values.
Results
Temperature measurement
The results of temperature measurements showed values between 39.8 and 40.1°C throughout the treatment period.
Treatment of isolates
All treatment protocols showed a clear antimicrobial effect visible as an inhibition zone or an overall reduction of bacterial growth on the treated agar plates. No antimicrobial effect was noticed on plates solely treated with argon gas. Inhibition zones varied in shape and definition of margins between bacterial species, treatment duration and distance of the spacer to the treated surface. Treatment of P. aeruginosa, S. pseudintermedius and S. canis isolates resulted in inhibition zones with either a circular shape with distinct or indistinct margins, or an irregular shape or overall reduction of bacterial growth, respectively. Inhibition zones can be seen in Figures 1–3, their measurements can be taken from Table 2.
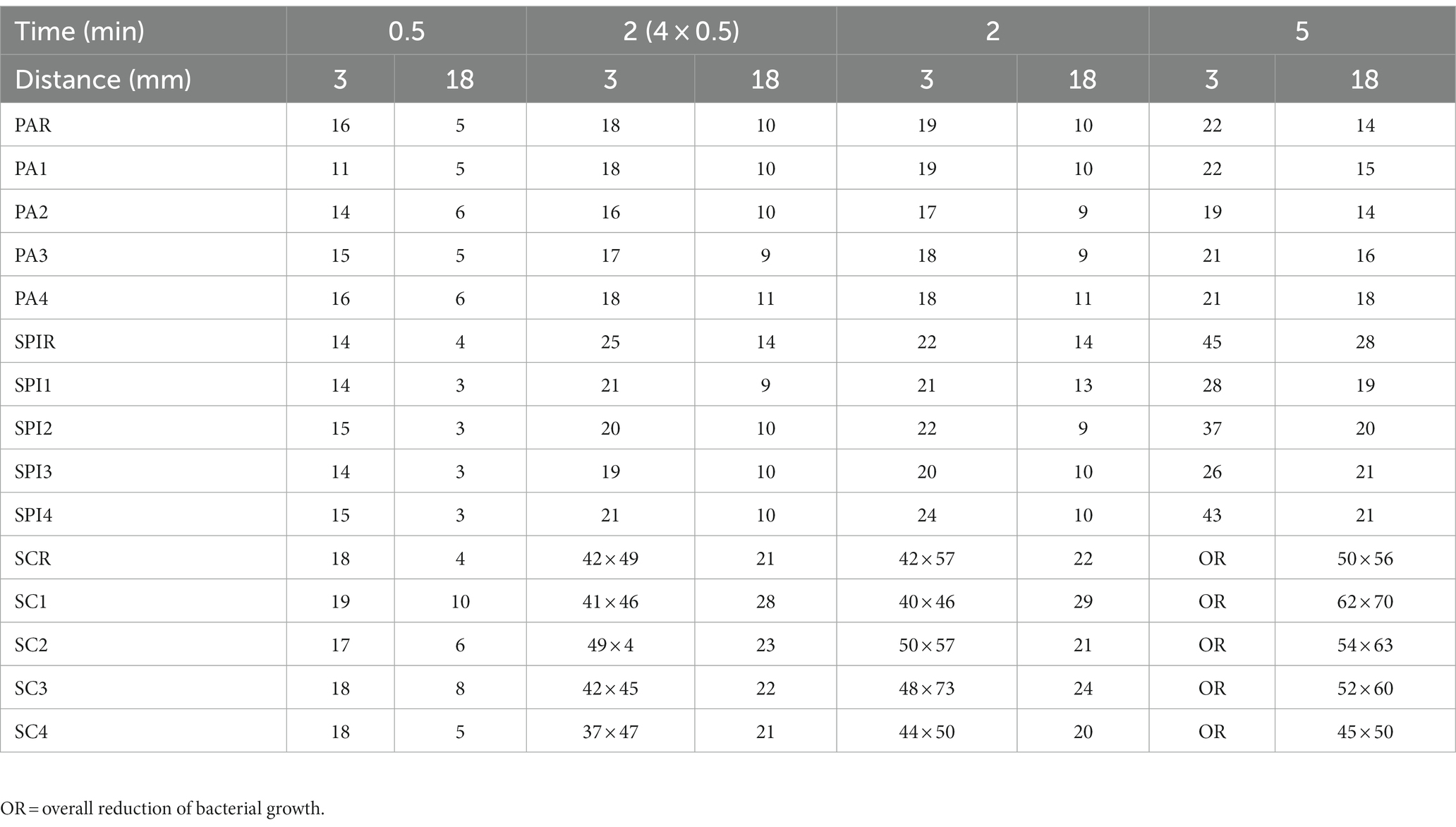
Table 2. Size of inhibition zones of P. aeruginosa isolates (PA1-4), S. canis (SC1-4), S. pseudintermedius (SP1-4) and their reference strains (PAR, SCR, SPR) given in mm.
Pseudomonas aeruginosa
Inhibition zones of all P. aeruginosa isolates and the reference strain were circular with distinct margins as shown in Figure 2. A treatment duration of 5 min at a distance of 3 mm had the strongest antimicrobial effect and resulted in inhibition zones ranging from 19 to 22 mm (reference strain: 22 mm). The least effective treatment duration was 30 s at a distance of 18 mm that resulted in zones ranging from 5 to 6 mm (reference strain: 5 mm). 30-s exposure time at a distance of 3 mm resulted in inhibition zones ranging from 11 to 16 mm (reference strain: 16 mm), 2-min exposure time at the same distance resulted in inhibition zones of 18–19 mm (reference strain: 19 mm). Exposing bacteria to either 2 min or 30 s repeated four times resulted in similar inhibition zones ranging from 17–19 mm and 17–18 mm with 3 mm distance and from 9–11 mm and 9–11 mm with 18 mm distance, respectively.
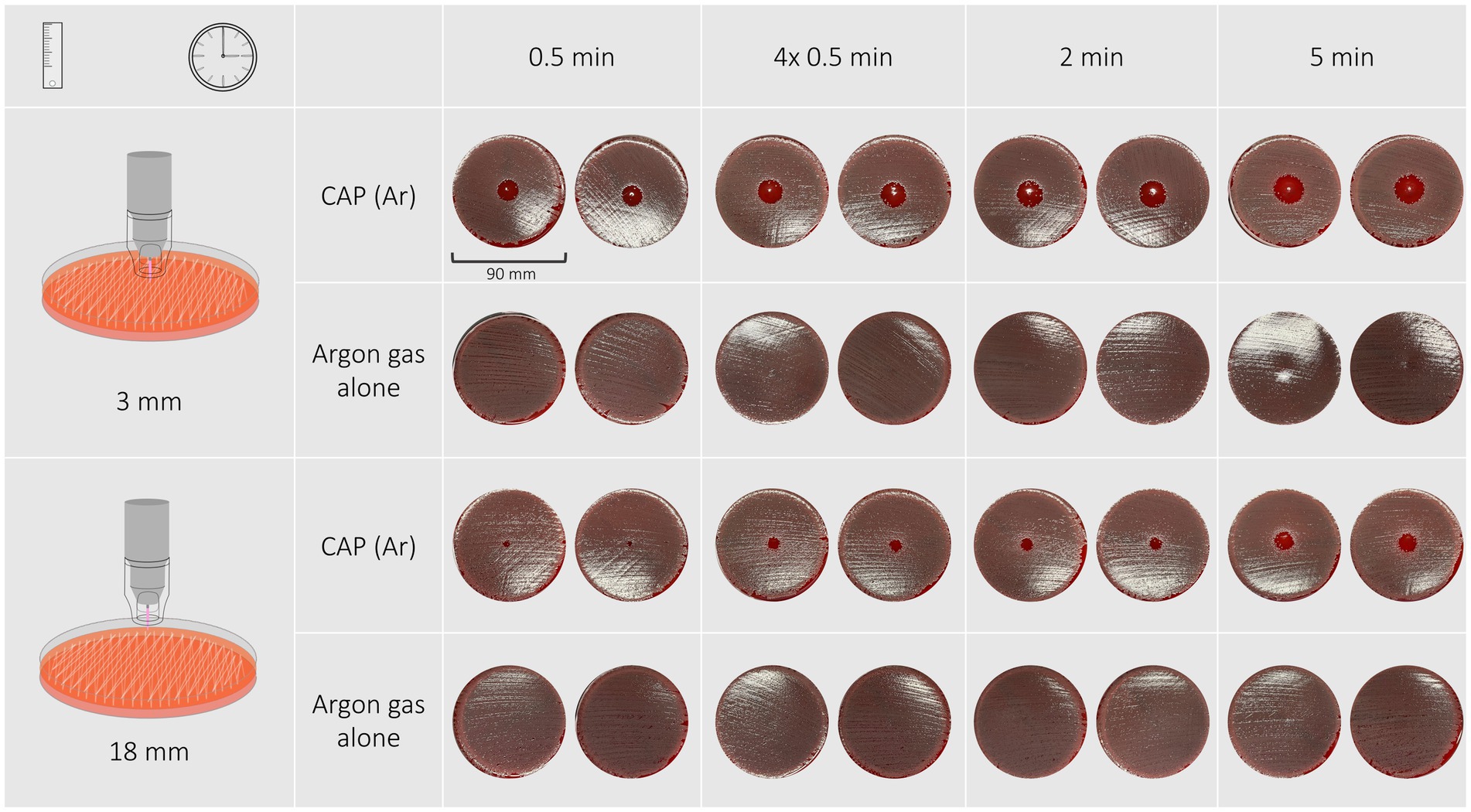
Figure 2. The inhibition zones of a Pseudomonas aeruginosa isolate after exposure to CAP for 30 s, 4 × 30 s, 2 min, 5 min at a distance of 3 mm are circular and have distinct edges. None of the treatments with argon gas alone resulted in the formation of inhibition zones.
Staphylococcus pseudintermedius
Morphology of inhibition zones of S. pseudintermedius isolates and reference strain varied between different exposure times and distances (Figure 3). A treatment duration of 30 s and 2 min resulted regardless of distance in a circular inhibition zone with distinct margins. A treatment duration of 5 min at a distance of 3 mm resulted in either a circular inhibition zone with indistinct margins or an irregular shaped inhibition zone. Treatment duration of 5 min at a distance of 18 mm led to circular shaped zones with indistinct margins.
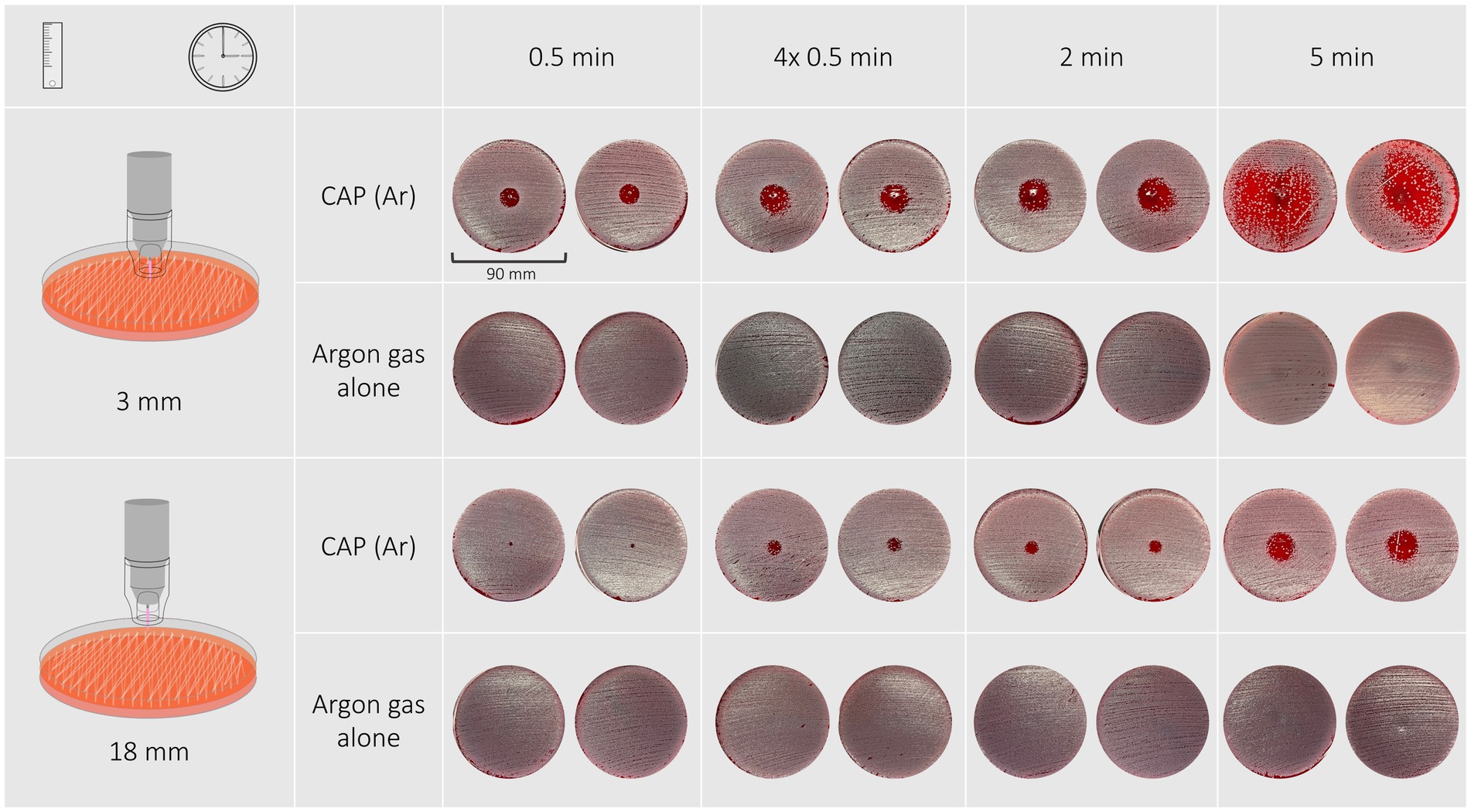
Figure 3. Inhibition zones of a Staphylococcus pseudintermedius isolate after exposure to CAP for 30 s, 4 × 30 s, 2 min, 5 min at a distance of 3 mm are circular and the edges become ill-defined with longer treatment duration.
A treatment duration of 5 min at a distance of 3 mm had the most effective antimicrobial effect and resulted in inhibition zones ranging from 28 to 43 mm (reference strain: 45 mm). The least effective treatment duration was 30 s at a distance of 18 mm that resulted in zones measuring 3 mm (reference strain: 4 mm). 30-s exposure time at a distance of 3 mm resulted in inhibition zones measuring 14 mm (reference strain: 14 mm), 2-min exposure time at the same distance resulted in inhibition zones of 20–24 mm (reference strain: 22 mm). Exposing bacteria to either 2 min or 30 s repeated four times resulted in similar inhibition zones ranging from 20–24 mm and 21–25 mm with 3 mm distance and from 9–14 mm and 9–14 mm with 18 mm distance, respectively.
Streptococcus canis
Response of S. canis isolates and the reference strain to cold plasma treatment was most variable among bacterial species. Inhibition zones were either circular with distinct or indistinct margins, irregular shaped or were not measurable due to an overall reduction in bacterial growth (Figure 4). Treatment for 30 s with both distances resulted in inhibition zones with distinct margins, 2 min and four times 30 s both at 18 mm distance resulted in circular shaped zones with either distinct or indistinct margins. Treatment duration of 2 min and four times 30 s at 3 mm distance resulted in irregular shaped inhibition zones. A 5-min treatment duration resulted in overall reduction of bacterial growth on the plate in the case of 3 mm distance, or in an irregular shaped inhibition zone in the case of 18 mm distance.
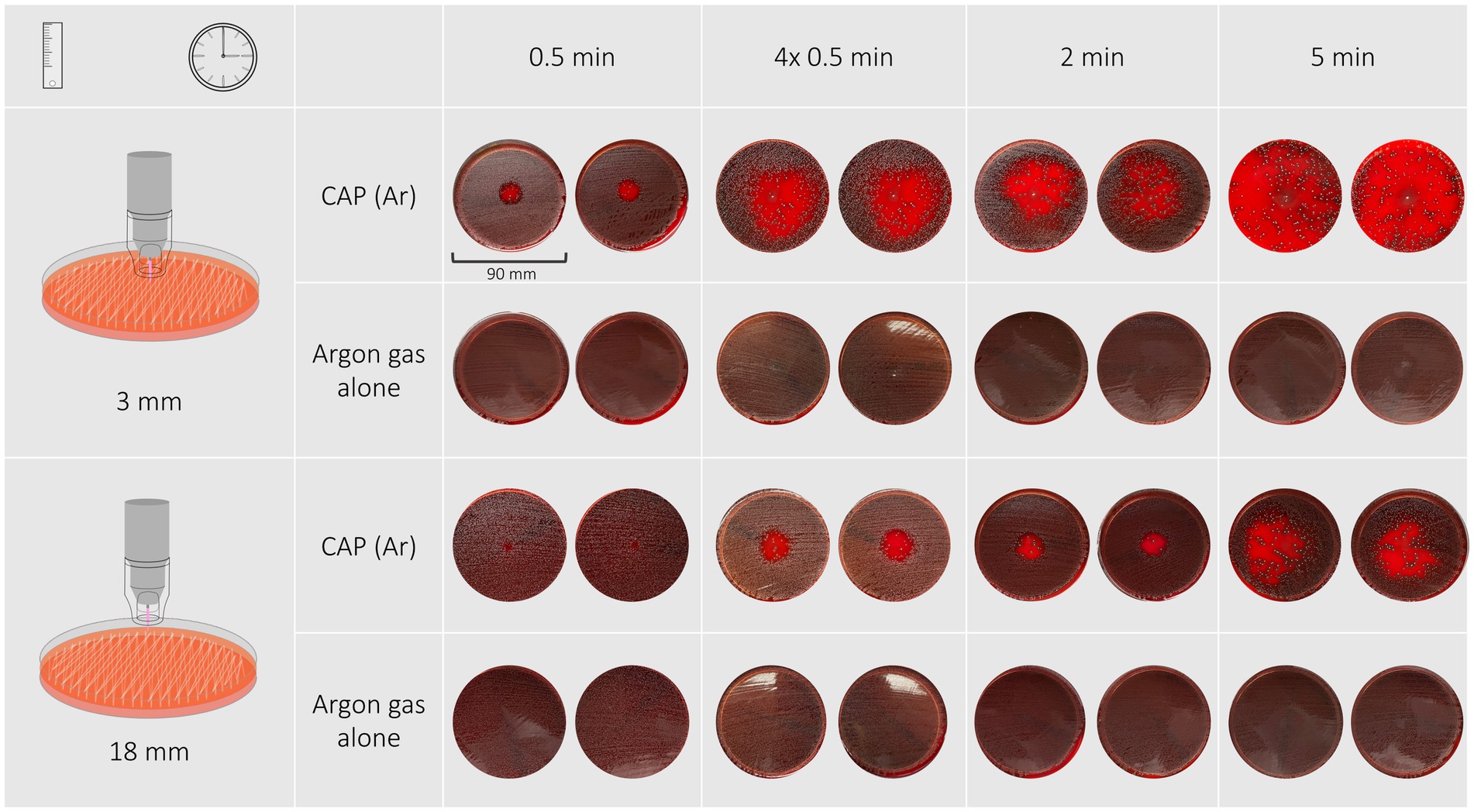
Figure 4. Shown are the inhibition zones of a Streptococcus canis isolate after exposure to CAP for 30 s, 4 × 30 s, 2 min, 5 min at a distance of 3 mm. With short treatment the zones were circular with undefined edges, with longer treatment times the shapes became irregular. A 5-min treatment resulted in a reduction of bacterial growth on the entire agar plate.
The most effective antimicrobial effect was achieved with a treatment duration of 5 min at a distance of 3 mm which resulted in overall reduction of bacterial growth. The least effective treatment duration was 30 s at a distance of 18 mm that resulted in zones ranging from 5 to 10 mm (reference strain: 4 mm). 30-s exposure time at a distance of 3 mm resulted in inhibition zones ranging from 18 to 19 mm (reference strain: 18 mm), 2-min exposure time at the same distance resulted in inhibition zones with a minimal and maximal width ranging from 40 to 50 mm (reference strain: 41 mm) and 46–73 mm (reference strain: 57 mm), respectively. Exposing bacteria to either 2 min or 30 s repeated four times at a distance of 3 mm resulted in variably irregular shaped inhibition zones with a minimal and maximal width ranging from 40–73 mm and 37–54 mm, respectively. Treatment durations of 2 min and 30 s repeated four times at 18 mm distance resulted in inhibition zones ranging from 20–29 mm and 21–28 mm, respectively.
Comparison of bacterial species and treatments
Increasing treatment duration and reducing distance to the spacer resulted in larger inhibition zones in all species, thus the strongest antimicrobial effect was detected after a treatment duration of 5 min at 3 mm distance, the least after a treatment duration of 30 s at 18 mm distance.
A treatment duration of 30 s at a distance of 3 mm resulted in inhibition zones of up to 16, 15 and 19 mm in the case of P. aeruginosa, S. pseudintermedius and S. canis isolates, respectively. These zones were still larger compared to those resulting from a 2-min treatment duration at 18 mm distance in P. aeruginosa and S. pseudintermedius isolates and were even similar to those resulting from a 5-min treatment at 18 mm distance in the case of P. aeruginosa isolates.
Discussion
To the best of our knowledge, this is the first study evaluating the in vitro antibacterial efficacy of argon cold atmospheric plasma against common corneal pathogens involved in canine bacterial keratitis. All clinical isolates of Pseudomonas aeruginosa, Staphylococcus pseudintermedius and Streptococcus canis were susceptible to the exposure to argon CAP.
Sensitivity patterns of different bacteria
The sterilizing effects of CAP on various bacterial species are well reported (2, 11, 15, 19–23, 42–46), there are however differences in sensitivity patterns among species (15, 42, 43, 47–50). Different levels of sensitivity were also seen in our study presenting as variably sized and shaped inhibition zones. Isolates of S. canis showed the largest inhibition zones and bacterial growth was inhibited within areas reaching far over the focally treated spot. Inhibition zones of S. pseudintermedius and S. canis isolates showed ill-defined margins. This could result from a lower concentration of plasma components at the periphery of the plasma jet as they are highly reactive in atmospheric air (3). Inhibition zones of P. aeruginosa, in contrast, had well-defined margins and were circular shaped. We therefore suspect that eradication of P. aeruginosa requires exposure to higher concentrations of plasma, which is supported by a previous study comparing susceptibility of P. aeruginosa to S. pseudintermedius isolates from skin infections in dogs (50). Current in vitro studies show contradictory results when it comes to the difference in susceptibility of Gram positive and Gram negative species (15, 46, 47, 51, 52). Some studies report a higher sensitivity of Gram negative compared to Gram positive bacteria (51, 52). As cellular membrane damage is considered to play a major role in the mechanism of antimicrobial efficacy of CAP it is postulated that cell wall morphology contributes to the difference in sensitivity (51, 52). Results from our study do not support this theory. S. canis isolates were most susceptible in our study which may emphasize that cellular membrane damage is not the only mechanism of antibacterial efficacy (53). This finding correlates with a previous study that showed increased intracellular reactive oxygen radicals in Gram positive compared to Gram negative species following exposure to CAP (48). The exact reason for that remains unknown.
As we postulate that the maximum treatment duration at a time is 30 s – for reasons discussed later – we included an interval treatment of 2 min consisting of 4 intervals with 15 s in between. The results from that protocol showed similar results as those from protocols using 2 min of full exposure in all bacterial species. We therefore do not expect a decrease in antibacterial efficacy of interval treatment in the case of a 2-min treatment duration.
Complex and incompletely understood biochemical and biophysical reactions of the different pathogens to components of CAP cause different sensitivity patterns between bacterial species, S. canis isolates were most susceptible to the exposure to CAP in our study.
Efficacy against multi-drug-resistant isolates
CAP is highly effective in eradicating multi-drug resistant bacteria in vitro and in vivo, such as isolates of methicillin-resistant Staphylococcus aureus (MRSA) (44, 54, 55). This is in concordance with findings in our study showing that the treatment of two methicillin-resistant isolates of S. pseudintermedius achieved very similar results compared to methicillin-sensitive isolates. The development of multidrug resistances against CAP seems unlikely since multiple components are involved in antibacterial efficacy (42, 53, 56, 57). Furthermore, CAP may even increase efficacy of antibiotic agents as its previous exposure increases sensitivity of methicillin-resistant Staphyloccus aureus strains (MRSA) to antibiotics (58). In addition to MRSA, eradication of methicillin-resistant Staphylococcus pseudintermedius isolates may also be a potential goal of the application of CAP.
Factors influencing antimicrobial efficacy
Antimicrobial outcomes depend on a number of variable factors, including the choice of plasma source, power supply, working gas and the dosage of CAP. The type of plasma sources is influencing the efficacy because they may use variable techniques of plasma generation that produce plasma of different composition and quantity (1, 42). The principle of plasma generation of plasma jets is based on ionization of a working gas which flows in between two electrodes. The type of working gas, for instance argon or helium gas, influences antimicrobial efficacy, as concentration of reactive oxygen radicals in plasma differs depending on the type of gas (59, 60). It is reported to be higher in plasma generated with argon gas compared to helium gas (59, 60). The device in our study operates with argon gas which has several advantages. These include a good tissue tolerance (61), its natural occurrence in the atmosphere (62) and simple extraction by air liquefaction (63). Another advantage of argon in research is its ‘inert state’ which enabled us its use in our control experiments which verified that argon gas does not have an antibacterial effect itself. Helium gas, in contrast, is non-renewable and its recycling is far too low to cover the current supply, causing scarcity and rising prices in the long run (64). Another factor influencing antimicrobial efficacy of CAP is the addition of different concentrations of oxygen to the working gas (46). Investigating the effect on pathogens by adding oxygen to argon gas was not within the scope of the current study. The dosage of plasma also has a significant impact on antimicrobial efficacy (15, 42, 48–50). This correlates with results from our study showing that efficacy was stronger with increased exposure time and shorter distance to the treated surface.
The high number of factors potentially influencing in vitro antimicrobial efficacy makes it very difficult to compare antibacterial efficacy from different studies (1). The results seen in our study may therefore not be reproduced using a different plasma source, working gas or experimental design.
Limitations of the study
Limitations of this study were a low number of tested isolates and the inclusion of only three different bacterial species from the ocular surface. Expanding experiments to other corneal pathogens in dogs is necessary to further investigate the antibacterial spectrum of argon cold atmospheric plasma.
Future research
The potential use of CAP generated by the kINPenVET® in the therapy of bacterial keratitis in canine patients requires future research. This should focus on safety for ocular tissue, penetration depth of CAP into corneal tissue and its in vivo efficacy against corneal pathogens.
Examples for potential mechanisms of damage to ocular tissue accompanying the exposure to CAP may include interactions with reactive species, exposure to UV light (65) or desiccation of the ocular surface by high gas flow rates (15).
Current research focusing on the safety of CAP for human corneal tissue is promising, as it shows that CAP does not result in harmful effects on in vitro cultures of different ocular cells if applied for up to 5 min (15, 27). CAP has even been shown to increase viability of in vitro corneal limbal epithelial cells following an exposure to argon CAP for up to 2 min. Exposure for 5 min did not influence viability, while a decrease was seen after 10 min (15). Free radicals were long considered toxic due to their high reactivity (66), recent studies however have shown that intracellular processes rely on the presence of free radicals (1). Their presence most likely plays the dominant role in stimulatory effects on cells resulting in increased cell migration (67) and proliferation (68). Furthermore, in chronic wounds important processes relying on radicals are impaired and can be re-stimulated by increasing intracellular concentrations of reactive species (1). Corneas treated for up to 5 min did not show histopathological changes in studies using different devices (15, 23, 45, 69, 70). The rate of corneal epithelization in rabbits was not affected by the application of CAP for 2 min (69). Ultraviolet light (UV) is one of the components of CAP with well reported toxic effects to corneal tissue (71). The risk of UV-induced damage during exposure to CAP is considered to be low due because emission of UV light during generation of CAP is low (72) and there was no evidence for UV-induced damage to conjunctival fibroblasts and corneal specimens after exposure for up to 5 min in previous studies (2, 27). Desiccation of the ocular surface was observed after application of the kINPen MED plasma pen (Neoplas GmbH®) to the cornea of human patients for 30 s (15). This is the consequence of a high gas flow rate of the working gas which is needed for adequate plasma concentrations and aids in the cooling of the device (personal communication with Neoplas GmbH®). We decided to reduce the gas flow rate to 4.1 SLM due to technical reasons, it can however be reduced up to a flow rate of 2 SLM according to the manufacturer. We postulate that lubrication of the patient’s eye during therapy may be needed every 30 s – interrupting the treatment is less likely to interfere with antimicrobial efficacy as discussed in the previous section.
Research on penetration depth of CAP into corneal tissue is scarce. One study using a corneal stromal tissue model showed that the antimicrobial effect on E. coli was evident in a depth of up to 200 μm (73). CAP in this study was however generated by a different plasma source.
Studies investigating the antimicrobial efficacy of CAP on ocular pathogens under ex or in vivo conditions exist. For instance, CAP was used to successfully treat experimentally infected ex vivo (15, 24, 27, 45) and in vivo corneas (22) of people, rabbits and pigs. Naturally occurring corneal infections in people were treated with CAP using the kINPen MED® (15). In all studies treatment with CAP alone or as an addition to standard therapy was efficient to control infection and resulted in healing of corneal ulceration.
Based on current literature, the application of CAP in patients with corneal infections may be safe and in theory efficient to treat the infection, however, randomized, clinical studies with control groups are needed to confirm its efficacy in the therapy of bacterial keratitis. We encourage research on all these aspects in companion animals as they are currently lacking.
Conclusion
We conclude that argon cold atmospheric plasma demonstrates a potent in vitro antibacterial effect on P. aeruginosa, S. pseudintermedius and Str. canis strains, bacteria most commonly found in canine bacterial keratitis. It is also capable of eradicating multi-drug resistant bacteria, including methicillin-resistant S. pseudintermedius isolates and may increase efficacy of antibiotics if used in combination (58). If future research confirms our results and the safety of its application, cold atmospheric plasma could be a promising alternative or adjuvant to antibiotics on the ocular surface in dogs. It could therefore aid in the reduction of antibiotic consumption which is in line with the one health approach (74).
Data availability statement
The original contributions presented in the study are included in the article/supplementary material, further inquiries can be directed to the corresponding author.
Ethics statement
Ethical approval was not required for the study involving animals in accordance with the local legislation and institutional requirements because the bacterial isolates used in the study were previously obtained from clinical patients for species identification and susceptibility testing (reasons unrelated to the study).
Author contributions
AM: Investigation, Methodology, Visualization, Writing - original draft, Writing - review & editing. HO: Methodology, Resources. JM: Methodology, Resources. JV: Methodology, Resources. TF: Conceptualization. CB: Conceptualization, Methodology, Supervision, Visualization, Writing - review & editing.
Funding
The author(s) declare financial support was received for the research, authorship, and/or publication of this article. This Open Access publication will be funded by the Deutsche Forschungsgemeinschaft (DFG, German Research Foundation) – 491094227 “Open Access Publication Funding” and the University of Veterinary Medicine Hannover, Foundation.
Acknowledgments
We would like to acknowledge Thomas von Woedtke and Veronika Hahn from the Leibniz Institute for Plasma Science and Technology, Greifswald for their support. Furthermore, we acknowledge Paul Lüttjohann and Sven Glitsch from Neoplas GmbH Greifswald for modification of our plasma device and Viktoria Nepke and colleagues from the laboratory of the Department of Pharmacology, Toxicology and Pharmacy for their constant support during our experiments.
Conflict of interest
The authors declare that the research was conducted in the absence of any commercial or financial relationships that could be construed as a potential conflict of interest.
Publisher’s note
All claims expressed in this article are solely those of the authors and do not necessarily represent those of their affiliated organizations, or those of the publisher, the editors and the reviewers. Any product that may be evaluated in this article, or claim that may be made by its manufacturer, is not guaranteed or endorsed by the publisher.
References
1. von Woedtke, T, Laroussi, M, and Gherardi, M. Foundations of plasmas for medical applications. Plasma Sources Sci Technol. (2022) 31:054002. doi: 10.1088/1361-6595/ac604f
2. Martines, E, Zuin, M, Cavazzana, R, Gazza, E, Serianni, G, Spagnolo, S, et al. A novel plasma source for sterilization of living tissues. New J Phys. (2009) 11:115014. doi: 10.1088/1367-2630/11/11/115014
3. Gerling, T, Helmke, A, and Weltmann, K-D. Relevant plasma parameters for certification. In: H-R Metelmann, T Woedtkevon, and K-D Weltmann, Comprehensive clinical plasma medicine: Cold physical plasma for medical application. Cham: Springer International Publishing; (2018). p. 43–70.
4. Weltmann, KD, Kindel, E, Tv, W, Hähnel, M, Stieber, M, and Brandenburg, R. Atmospheric-pressure plasma sources: prospective tools for plasma medicine. Pure Appl Chem. (2010) 82:1223–37. doi: 10.1351/PAC-CON-09-10-35
5. Laroussi, M, and Akan, T. Arc-free atmospheric pressure cold plasma jets: a review. Plasma Process Polym. (2007) 4:777–88. doi: 10.1002/ppap.200700066
6. Graves, DB, and Bauer, G. Key roles of reactive oxygen and nitrogen species. In: H-R Metelmann, T Woedtkevon, and K-D Weltmann, Comprehensive clinical plasma medicine: Cold physical plasma for medical application. Cham: Springer International Publishing; (2018). p. 71–82.
7. Nespolo, M. Free radicals in biology and medicine. 5th. By Barry Halliwell and John M. C. Gutteridge. Oxford Oxford University Press, (2015).
8. Sies, H. On the history of oxidative stress: concept and some aspects of current development. Curr Opin Toxicol. (2018) 7:122–6. doi: 10.1016/j.cotox.2018.01.002
9. Ulrich, C, Kluschke, F, Patzelt, A, Vandersee, S, Czaika, VA, Richter, H, et al. Clinical use of cold atmospheric pressure argon plasma in chronic leg ulcers: a pilot study. J Wound Care. (2015) 24:196:8-200–-3. doi: 10.12968/jowc.2015.24.5.196
10. Heinlin, J, Isbary, G, Stolz, W, Morfill, G, Landthaler, M, Shimizu, T, et al. Plasma applications in medicine with a special focus on dermatology. J Eur Acad Dermatol Venereol. (2011) 25:1–11. doi: 10.1111/j.1468-3083.2010.03702.x
11. Daeschlein, G, Napp, M, Lutze, S, Arnold, A, von Podewils, S, Guembel, D, et al. Skin and wound decontamination of multidrug-resistant bacteria by cold atmospheric plasma coagulation. J Dtsch Dermatol Ges. (2015) 13:143–50. doi: 10.1111/ddg.12559
12. Seebauer, C, Metelmann, H-R, Witzke, K, and Pouvesle, J-M. Palliative treatment of head and neck Cancer. In: H-R Metelmann, T Woedtkevon, and K-D Weltmann, Comprehensive clinical plasma medicine: Cold physical plasma for medical application. Cham: Springer International Publishing; (2018). p. 185–195.
13. Canady, J, Gordon, S, Zhuang, T, Wigh, S, Rowe, W, and Shashurin, A, (, et al.) Cold atmospheric plasma (CAP) combined with chemo-radiation and Cytoreductive surgery: the first clinical experience for stage IV metastatic Colon Cancer. In: H-R Metelmann, T Woedtkevon, and K-D Weltmann, Comprehensive clinical plasma medicine: Cold physical plasma for medical application. Cham: Springer International Publishing; (2018). p. 163–183.
14. Malathi Suresh, SM, Hemalatha, VT, Sundar, N, and Nisha, A. Applications of cold atmospheric pressure plasma in dentistry-a review. J Pharm Res Int. (2022) 34:45–55. doi: 10.9734/jpri/2022/v34i11A35533
15. Reitberger, HH, Czugala, M, Chow, C, Mohr, A, Burkovski, A, Gruenert, AK, et al. Argon cold plasma-a novel tool to treat therapy-resistant corneal infections. Am J Ophthalmol. (2018) 190:150–63. doi: 10.1016/j.ajo.2018.03.025
16. Bekeschus, S, Lin, A, Fridman, A, Wende, K, Weltmann, K-D, and Miller, V. A comparison of floating-electrode DBD and kINPen jet: plasma parameters to achieve similar growth reduction in Colon Cancer cells under standardized conditions. Plasma Chem Plasma Process. (2018) 38:1–12. doi: 10.1007/s11090-017-9845-3
17. Hirst, AM, Frame, FM, Arya, M, Maitland, NJ, and O’Connell, D. Low temperature plasmas as emerging cancer therapeutics: the state of play and thoughts for the future. Tumor Biol. (2016) 37:7021–31. doi: 10.1007/s13277-016-4911-7
18. Dubuc, A, Monsarrat, P, Virard, F, Merbahi, N, Sarrette, JP, Laurencin-Dalicieux, S, et al. Use of cold-atmospheric plasma in oncology: a concise systematic review. Ther Adv Med Oncol. (2018) 10:175883591878647. doi: 10.1177/1758835918786475
19. Jungbauer, G, Favaro, L, Müller, S, Sculean, A, and Eick, S. The in-vitro activity of a cold atmospheric plasma device utilizing ambient air against Bacteria and biofilms associated with periodontal or Peri-implant diseases. Antibiotics. (2022) 11:752. doi: 10.3390/antibiotics11060752
20. Jungbauer, G, Moser, D, Müller, S, Pfister, W, Sculean, A, and Eick, S. The antimicrobial effect of cold atmospheric plasma against dental pathogens-a systematic review of in-vitro studies. Antibiotics (Basel). (2021) 10:211. doi: 10.3390/antibiotics10020211
21. Üreyen Kaya, B, Kececi, AD, Güldaş, HE, Çetin, ES, Öztürk, T, Öksuz, L, et al. Efficacy of endodontic applications of ozone and low-temperature atmospheric pressure plasma on root canals infected with Enterococcus faecalis. Lett Appl Microbiol. (2014) 58:8–15. doi: 10.1111/lam.12148
22. Saleem, W, Benton, AH, Marquart, ME, Wang, S, Saleem, W, Vigil, R, et al. Innovative cold atmospheric plasma (iCAP) decreases mucopurulent corneal ulcer formation and edema and reduces bacterial load in Pseudomonas keratitis. Clin Plasma Med. (2019) 16:100093. doi: 10.1016/j.cpme.2019.100093
23. Martines, E, Brun, P, Brun, P, Cavazzana, R, Deligianni, V, Leonardi, A, et al. Towards a plasma treatment of corneal infections. Clin Plasma Med. (2013) 1:17–24. doi: 10.1016/j.cpme.2013.10.001
24. Nikmaram, H, Rezaei Kanavi, M, Ghoranneviss, M, Balagholi, S, Ahmadieh, H, Roshandel, D, et al. Cold atmospheric pressure plasma jet for the treatment of aspergillus keratitis. Clin Plasma Med. (2018) 9:14–8. doi: 10.1016/j.cpme.2017.12.075
25. Lorenzo-Morales, J, Khan, NA, and Walochnik, J. An update on Acanthamoeba keratitis: diagnosis, pathogenesis and treatment. Parasite. (2015) 22:10. doi: 10.1051/parasite/2015010
26. Alekseev, O, Donovan, K, Limonnik, V, and Azizkhan-Clifford, J. Nonthermal dielectric barrier discharge (DBD) plasma suppresses herpes simplex virus type 1 (HSV-1) replication in corneal epithelium. Transl Vis Sci Technol. (2014) 3:2. doi: 10.1167/tvst.3.2.2
27. Brun, P, Brun, P, Vono, M, Venier, P, Tarricone, E, Deligianni, V, et al. Disinfection of ocular cells and tissues by atmospheric-pressure cold plasma. PLoS One. (2012) 7:e33245. doi: 10.1371/journal.pone.0033245
28. Gelatt, KN, Ben-Shlomo, G, Gilger, BC, Hendrix, DV, Kern, TJ, and Plummer, CE. Veterinary ophthalmology. Hoboken, NJ: John Wiley & Sons (2021).
29. Verdenius, CY, Broens, EM, Slenter, IJM, and Djajadiningrat-Laanen, SC. Corneal stromal ulcerations in a referral population of dogs and cats in the Netherlands (2012–2019): bacterial isolates and antibiotic resistance. Vet Ophthalmol. doi: 10.1111/vop.13080
30. Hewitt, JS, Allbaugh, RA, Kenne, DE, and Sebbag, L. Prevalence and antibiotic susceptibility of bacterial isolates from dogs with ulcerative keratitis in Midwestern United States. Front Vet Sci. (2020) 7:583965. doi: 10.3389/fvets.2020.583965
31. Hindley, KE, Groth, AD, King, M, Graham, K, and Billson, FM. Bacterial isolates, antimicrobial susceptibility, and clinical characteristics of bacterial keratitis in dogs presenting to referral practice in Australia. Vet Ophthalmol. (2016) 19:418–26. doi: 10.1111/vop.12325
32. Prado, MR, Rocha, MF, Brito, EH, Girão, MD, Monteiro, AJ, Teixeira, MF, et al. Survey of bacterial microorganisms in the conjunctival sac of clinically normal dogs and dogs with ulcerative keratitis in Fortaleza, Ceará. Brazil Vet Ophthalmol. (2005) 8:33–7. doi: 10.1111/j.1463-5224.2005.04061.x
33. Lin, CT, and Petersen-Jones, SM. Antibiotic susceptibility of bacterial isolates from corneal ulcers of dogs in Taiwan. J Small Anim Pract. (2007) 48:271–4. doi: 10.1111/j.1748-5827.2007.00348.x
34. Suter, A, Voelter, K, Hartnack, S, Spiess, BM, and Pot, SA. Septic keratitis in dogs, cats, and horses in Switzerland: associated bacteria and antibiotic susceptibility. Vet Ophthalmol. (2018) 21:66–75. doi: 10.1111/vop.12480
35. Tolar, EL, Hendrix, DVH, Rohrbach, BW, Plummer, CE, Brooks, DE, and Gelatt, KN. Evaluation of clinical characteristics and bacterial isolates in dogs with bacterial keratitis: 97 cases (1993–2003). J Am Vet Med Assoc. (2006) 228:80–5. doi: 10.2460/javma.228.1.80
36. Wang, L, Pan, Q, Zhang, L, Xue, Q, Cui, J, and Qi, C. Investigation of bacterial microorganisms in the conjunctival sac of clinically normal dogs and dogs with ulcerative keratitis in Beijing. China Vet Ophthal. (2008) 11:145–9. doi: 10.1111/j.1463-5224.2008.00579.x
37. Ollivier, FJ. Bacterial corneal diseases in dogs and cats. Clin Tech Small Anim Pract. (2003) 18:193–8. doi: 10.1016/S1096-2867(03)90016-8
38. Joksimovic, M, Ford, BA, Lazic, T, Soldatovic, I, Luzetsky, S, and Grozdanic, S. Antibiotic recommendations for treatment of canine stromal corneal ulcers. Vet Sci. (2023) 10:66. doi: 10.3390/vetsci10020066
39. LoPinto, AJ, Mohammed, HO, and Ledbetter, EC. Prevalence and risk factors for isolation of methicillin-resistant Staphylococcus in dogs with keratitis. Vet Ophthalmol. (2015) 18:297–303. doi: 10.1111/vop.12200
40. Kang, M-H, Chae, M-J, Yoon, J-W, Kim, S-G, Lee, S-Y, Yoo, J-H, et al. Antibiotic resistance and molecular characterization of ophthalmic Staphylococcus pseudintermedius isolates from dogs. J Vet Sci. (2014) 15:409–15. doi: 10.4142/jvs.2014.15.3.409
41. The European Parliament and the Council of the European Union. Regulation (EU) 2019/5 of the European Parliament and of the council of 11 December 2018 amending regulation (EC) no 726/2004 laying down community procedures for the authorisation and supervision of medicinal products for human and veterinary use and establishing a European medicines agency, regulation (EC) no 1901/2006 on medicinal products for paediatric use and directive 2001/83/EC on the community code relating to medicinal products for human use. 2018, EU 2019/5. Off J Eur Union EU 2019/5. Washington, DC: The European Parliament and the Council of the European Union (2018).
42. Ehlbeck, J, Schnabel, U, Polak, M, Winter, J, von Woedtke, T, Brandenburg, R, et al. Low temperature atmospheric pressure plasma sources for microbial decontamination. J Phys D Appl Phys. (2011) 44:013002. doi: 10.1088/0022-3727/44/1/013002
43. Daeschlein, G, Scholz, S, Arnold, A, von Podewils, S, Haase, H, Emmert, S, et al. In vitro susceptibility of important skin and wound pathogens against low temperature atmospheric pressure plasma jet (APPJ) and dielectric barrier discharge plasma (DBD). Plasma Process Polym. (2012) 9:380–9. doi: 10.1002/ppap.201100160
44. Flynn, PB, Higginbotham, S, Alshraiedeh, NH, Gorman, SP, Graham, WG, and Gilmore, BF. Bactericidal efficacy of atmospheric pressure non-thermal plasma (APNTP) against the ESKAPE pathogens. Int J Antimicrob Agents. (2015) 46:101–7. doi: 10.1016/j.ijantimicag.2015.02.026
45. Hammann, A, Huebner, NO, Bender, C, Ekkernkamp, A, Hartmann, B, Hinz, P, et al. Antiseptic efficacy and tolerance of tissue-tolerable plasma compared with two wound antiseptics on artificially bacterially contaminated eyes from commercially slaughtered pigs. Skin Pharmacol Physiol. (2010) 23:328–32. doi: 10.1159/000314724
46. Matthes, R, Koban, I, Bender, C, Masur, K, Kindel, E, Weltmann, K-D, et al. Antimicrobial efficacy of an atmospheric pressure plasma jet against biofilms of Pseudomonas aeruginosa and Staphylococcus epidermidis. Plasma Process Polym. (2013) 10:161–6. doi: 10.1002/ppap.201100133
47. Mai-Prochnow, A, Murphy, AB, McLean, KM, Kong, MG, and Ostrikov, KK. Atmospheric pressure plasmas: infection control and bacterial responses. Int J Antimicrob Agents. (2014) 43:508–17. doi: 10.1016/j.ijantimicag.2014.01.025
48. Han, L, Patil, S, Boehm, D, Milosavljević, V, Cullen, PJ, and Bourke, P. Mechanisms of inactivation by high-voltage atmospheric cold plasma differ for Escherichia coli and Staphylococcus aureus. Appl Environ Microbiol. (2016) 82:450–8. doi: 10.1128/AEM.02660-15
49. Isbary, G, Morfill, G, Schmidt, HU, Georgi, M, Ramrath, K, Heinlin, J, et al. A first prospective randomized controlled trial to decrease bacterial load using cold atmospheric argon plasma on chronic wounds in patients. Br J Dermatol. (2010) 163:78–82. doi: 10.1111/j.1365-2133.2010.09744.x
50. Bae, S, Lim, D, Kim, D, Jeon, J, and Oh, T. In vitro antibacterial effects of non-thermal atmospheric plasma irradiation on Staphylococcus pseudintermedius and Pseudomonas aeruginosa. Pol J Vet Sci. (2020) 23:13–9. doi: 10.24425/pjvs.2019.131414
51. Mai-Prochnow, A, Clauson, M, Hong, J, and Murphy, AB. Gram positive and gram negative bacteria differ in their sensitivity to cold plasma. Sci Rep. (2016) 6:38610. doi: 10.1038/srep38610
52. Laroussi, M, Mendis, DA, and Rosenberg, M. Plasma interaction with microbes. New J Phys. (2003) 5:41. doi: 10.1088/1367-2630/5/1/341
53. Alkawareek, MY, Gorman, SP, Graham, WG, and Gilmore, BF. Potential cellular targets and antibacterial efficacy of atmospheric pressure non-thermal plasma. Int J Antimicrob Agents. (2014) 43:154–60. doi: 10.1016/j.ijantimicag.2013.08.022
54. Napp, M, Daeschlein, G, von Podewils, S, Hinz, P, Emmert, S, Haase, H, et al. In vitro susceptibility of methicillin-resistant and methicillin-susceptible strains of Staphylococcus aureus to two different cold atmospheric plasma sources. Infection. (2016) 44:531–7. doi: 10.1007/s15010-016-0888-9
55. Namini, YN, Heidarzadeh, S, Khaledi, A, Abbasi, E, Abbasi, A, and Esmaeili, D. Study on the killing effect of cold atmospheric pressure plasma on MRSA Staphylococcus aureus in vitro and in vivo infection model. Malays J Microbiol. (2019) 15:394–9. doi: 10.21161/MJM.180295
56. Matthes, R, Assadian, O, and Kramer, A. Repeated applications of cold atmospheric pressure plasma does not induce resistance in Staphylococcus aureus embedded in biofilms. GMS Hyg Infect Control. (2014) 9:Doc17. doi: 10.3205/dgkh000237
57. Bourke, P, Ziuzina, D, Han, L, Cullen, PJ, and Gilmore, BF. Microbiological interactions with cold plasma. J Appl Microbiol. (2017) 123:308–24. doi: 10.1111/jam.13429
58. Bayliss, DL, Shama, G, and Kong, MG. Restoration of antibiotic sensitivity in meticillin-resistant Staphylococcus aureus following treatment with a non-thermal atmospheric gas plasma. Int J Antimicrob Agents. (2013) 41:398–9. doi: 10.1016/j.ijantimicag.2012.12.005
59. Aguilera, JA, and Aragón, C. A comparison of the temperatures and electron densities of laser-produced plasmas obtained in air, argon, and helium at atmospheric pressure. Applied Physics A. (1999) 69:S475–8. doi: 10.1007/s003390051443
60. Park, G, Lee, H, Kim, G, and Lee, JK. Global model of he/O2 and Ar/O2 atmospheric pressure glow discharges. Plasma Process Polym. (2008) 5:569–76. doi: 10.1002/ppap.200800019
61. Nespoli, F, Redaelli, S, Ruggeri, L, Fumagalli, F, Olivari, D, and Ristagno, G. A complete review of preclinical and clinical uses of the noble gas argon: evidence of safety and protection. Ann Card Anaesth. (2019) 22:122–35. doi: 10.4103/aca.ACA_111_18
62. Rayleigh, L, and Ramsay, W. Argon, a new constituent of the atmosphere. Philos Trans R Soc Lond A. (1895) 186:187–241. doi: 10.1098/rsta.1895.0006
63. Cao, ZCHG. Unified field theory and topology of nuclei. Int J Theor Phys. (2014) 2:15–22. doi: 10.12691/ijp-2-1-4
64. Olafsdottir, AH, and Sverdrup, HU. Assessing the past and future sustainability of global helium resources, extraction, supply and use, using the integrated assessment model WORLD7. Biophys Econom Sustain. (2020) 5:6. doi: 10.1007/s41247-020-00072-5
65. Wende, K, Schmidt, A, and Bekeschus, S. Safety aspects of non-thermal plasmas. (2018). p. 83–109. Springer, Cham
66. Stohs, SJ. The role of free radicals in toxicity and disease. J Basic Clin Physiol Pharmacol. (1995) 6:205–28. doi: 10.1515/JBCPP.1995.6.3-4.205
67. Arndt, S, Landthaler, M, Zimmermann, JL, Unger, P, Wacker, E, Shimizu, T, et al. Effects of cold atmospheric plasma (CAP) on ß-defensins, inflammatory cytokines, and apoptosis-related molecules in keratinocytes in vitro and in vivo. PLoS One. (2015) 10:e0120041. doi: 10.1371/journal.pone.0120041
68. Kalghatgi, S, Friedman, G, Fridman, A, and Clyne, AM. Endothelial cell proliferation is enhanced by low dose non-thermal plasma through fibroblast growth factor-2 release. Ann Biomed Eng. (2010) 38:748–57. doi: 10.1007/s10439-009-9868-x
69. Alhabshan, R, Belyea, D, Stepp, M, J, B, S, G, and Shashurin, A. Effects of in-vivo application of cold atmospheric plasma on corneal wound healing in New Zealand white rabbits. Int J Ophthalmol Pathol. (2013) 2:1–5. doi: 10.4172/2324-8599.1000118
70. Rosani, U, Tarricone, E, Venier, P, Brun, P, Deligianni, V, Zuin, M, et al. Atmospheric-pressure cold plasma induces transcriptional changes in ex vivo human corneas. PLoS One. (2015) 10:e0133173. doi: 10.1371/journal.pone.0133173
71. Delic, NC, Lyons, JG, Di Girolamo, N, and Halliday, GM. Damaging effects of ultraviolet radiation on the cornea. Photochem Photobiol. (2017) 93:920–9. doi: 10.1111/php.12686
72. Sosnin, EA, Stoffels, E, Erofeev, MV, Kieft, IE, and Kunts, SE. The effects of UV irradiation and gas plasma treatment on living mammalian cells and bacteria: a comparative approach. IEEE Trans Plasma Sci. (2004) 32:1544–50. doi: 10.1109/TPS.2004.833401
73. Lingenfelder, C, Kampmeier, J, Russ, D, Wittig, R, Diener, C, and Werner, JU. Depth effect of cold atmospheric pressure plasma in keratitis therapy: a corneal stroma tissue model. Klin Monatsbl Augenheilkd. (2020) 237:1087–92. doi: 10.1055/a-1142-6442
Keywords: plasma pen, non-thermal plasma, bacterial ulcer, antibacterial efficacy, MRSP
Citation: Marx AH, Oltmanns H, Meißner J, Verspohl J, Fuchsluger T and Busse C (2024) Argon cold atmospheric plasma eradicates pathogens in vitro that are commonly associated with canine bacterial keratitis. Front. Vet. Sci. 10:1320145. doi: 10.3389/fvets.2023.1320145
Edited by:
Amjad Islam Aqib, Cholistan University of Veterinary and Animal Sciences, PakistanReviewed by:
Piera Anna Martino, University of Milan, ItalyCristin Coman, "Cantacuzino", National Institute of Medical-Military Research and Development, Romania
Hafiz Iftikhar Hussain, Cholistan University of Veterinary and Animal Sciences, Pakistan
Copyright © 2024 Marx, Oltmanns, Meißner, Verspohl, Fuchsluger and Busse. This is an open-access article distributed under the terms of the Creative Commons Attribution License (CC BY). The use, distribution or reproduction in other forums is permitted, provided the original author(s) and the copyright owner(s) are credited and that the original publication in this journal is cited, in accordance with accepted academic practice. No use, distribution or reproduction is permitted which does not comply with these terms.
*Correspondence: Claudia Busse, Y2xhdWRpYS5idXNzZUB0aWhvLWhhbm5vdmVyLmRl