- 1Faculty of Health and Wellbeing, University of Winchester, Winchester, United Kingdom
- 2School of Environment and Science, Griffith University, Nathan, QLD, Australia
Diseases passed to humans from animals (zoonoses) constitute 75% of emerging infectious diseases. Farmed animals are considered a high zoonotic risk, especially poultry and pigs as evidenced by recent outbreaks of avian and swine influenza. This review sought to collate recent knowledge of the disease risks from keeping pigs and chickens intensively and in close proximity to each other. Recent knowledge on influenza viruses compounds the public health concerns; no longer are concerns about “mixing vessel” hosts limited to pigs, but several other animal species too at a high level of probability—most notably chickens and humans. More generally, scientific literature establishing positive associations between intensive animal farming, human population growth, reduced biodiversity, and increased zoonoses risks is abundant. This includes the publication of relevant systematic reviews. The collected scientific evidence on this issue is clear: there is exceptionally strong evidence for a link between low animal welfare levels and high zoonotic risks, exacerbated by animal crowding, low genetic diversity, compromised hygiene, and high animal stress levels which compromise immune systems. Based on this evidence, further industrialized animal farms—especially poultry and pig farms or a mix thereof, and particularly in areas that already have a high concentration of farmed animals—should not generally be permitted to proceed. Instead, efforts should concentrate on supporting arable agriculture (or transitions toward this) and de-intensifying remaining animal farms, in alignment with One Health/One Welfare approaches within which animal health and welfare are integral parts of any farming operation. Among numerous other factors, this would involve reducing stocking densities down to 11 kg/m2 (around five chickens/m2) for meat chickens, and down to one pig/1.5 m2 for pigs (assuming a 100 kg pig).
1 Introduction
In this article we review recent scientific evidence regarding the disease risks posed to public health by intensive pig and poultry farms that are situated in close proximity to each other (hereafter, mixed pig/poultry farms). Recent examples of planning applications for such large-scale mixed swine-poultry farms exist, such as concurrent 2023 planning applications in the Borough Council of King’s Lynn and West Norfolk (UK) for the expansion of existing pig and poultry farms (1, 2). These particular applications come following the most severe avian flu outbreak in the UK since records began (3), and following post-covid increased awareness of viral disease risks for public health, including mutation risks (4). As the aforementioned planning applications concern pigs and chickens reared for meat (hereafter, meat chickens), this review will focus predominantly on pigs and meat chickens. First, evidence for the magnitude of the problem is outlined. Next, key drivers of the problem, and evidence establishing these, are reviewed. Then, methods to mitigate the risks are described. This article closes with a conclusion concerning the disease risks posed by mixed intensive swine and poultry farms, based on the evidence reviewed.
2 Public health risks arising from farmed animal diseases
Infectious diseases in animals can be prevalent within animal populations at varying levels of severity. Diseases may be enzootic (persistent at low levels within a population), epizootic (denoting an outbreak of a higher number of cases within a particular region), or panzootic (denoting an outbreak covering a wide region). These terms are now sometimes used interchangeably with the equivalent terms used for human diseases and populations: endemic, epidemic, and pandemic, respectively [(5), pp. 131–132]. Not only do all diseases in farmed animals compromise animal welfare, but many are also known to transmit from vertebrate animals to humans. Such a disease is termed a zoonosis, or “zoonoses” in plural form (6). The disease-causing agents can be, for instance, bacterial, viral, fungal, parasitic, or protozoic (7). Tomori and Oluwayelu (7) describe key salient examples of recent zoonoses spanning endemics, epidemics, and pandemics. Examples include MERS (Middle East respiratory syndrome) since 2012, Ebola since 1976 in Africa/the Middle East, and SARS (severe acute respiratory syndrome) since 2003 in Asia (see Table 1 for further information and examples).
Zoonotic diseases from farmed animals constitute a public health risk, which may be severe. Zoonoses form the dominant group of diseases among emerging infectious diseases (EIDs)—currently 75% of EIDs (8). Zoonoses are typically spread via contact with feces or saliva of an infected animal, either directly, or through contamination of the environment (6). While approximately three quarters of zoonoses stem from wild animals, the remaining 25% arise from domesticated species (7). Moreover, due to intensive resource requirements (e.g., land to grow feed crops for farmed animals), growing human and farmed animal populations, and growing consumption of meat, milk, and eggs, industrialized intensive animal farming (IIAF) is a leading driver of habitat destruction (9, 10). This brings the interface between humans and wild animals closer, and thereby increases the risk of disease transmission from wild animals [(11), p. 138]. Thus, IIAF confers both direct increased risks of zoonoses from farmed animals (arising from the vast numbers of animals kept, the ways in which they are kept, and close human-farmed animal proximity), and indirect increased zoonoses risks from wild animals (i.e., through intensive resource use by IIAF leading to increased habitat destruction and increased human-wild animal proximity). Antimicrobial use is also high, and this is an exacerbating factor. Seventy-three per cent of antimicrobials consumed globally are used on farmed animals; this is projected to increase by 8% by 2030 (12). These antibiotics are widely used, at low levels, for long periods—all factors known to create antibiotic resistance. Thus, IIAF plays a significant role in the increased risk of antibiotic resistance emergence. For all of these reasons, farmed animals are considered to pose a high zoonotic risk, while other domesticated animals such as cats are deemed a low zoonotic risk [(13), p. 5]. This is further discussed in section 4.
Viruses, and specifically influenza viruses, currently constitute the greatest zoonotic threats. This is because they demonstrate the greatest likelihood of being transmitted between different species, including to humans (7), with the extent to which viral transmission to humans is successful depending on the abundance of all species involved, as well as on the viral richness (the number of viral species found in a host) and the life history traits of host animals [(14), p. 646]. Hence, hereafter the focus will be exclusively on emergent zoonotic influenzas. Nevertheless, it should be noted that there are other viral disease risks aside from influenzas. Coronaviruses (e.g., COVID-19, SARS, MERS) are of the next greatest concern after influenza viruses, and they may have wild or domesticated animal hosts (7).
3 Influenza scrutinized: the connection between human, avian, and swine influenza
In a review of zoonotic influenza, Abdelwhab and Mettenleiter (13) outlined the four types of influenza virus that are known: A, B, C, and D. Each is a distinct viral species, differing in their genome and in the diversity of hosts infected, with type B demonstrating the narrowest host range (humans and pigs), followed by type C (humans, pigs, and seals), type D (cattle, several other ruminant species, pigs, and horses), and type A. Type A is the most widespread as it can infect a wide range of mammals and birds; hence, it is the type of most concern (13). Thus, henceforth, type A will be exclusively focused on.
Type A can be subdivided into a further 18 “H” and 11 “N” subtypes, which are effectively abbreviations of the two proteins found on the virus’s surface—hemagglutinin and neuraminidase—giving viral names such as “H5N1” and “H9N2” (15). The former compound is considered to play a role chiefly in cell entry, and the latter in cell exit for the dispersal of virus progeny [(16), p. 637]. A key reason for type A’s prevalence is its propensity toward both antigenic shift and antigenic drift. Antigenic shift describes the genetic reassortment of strains that can occur when two different strains infect the same host, potentially leading to the creation of a new type A virus strain; while antigenic drift denotes mutations that can occur over a longer period of time to enable a type A virus to adapt to (i.e., overcome) any host immunity that may have developed [(17), p. 2]. Different influenza A viruses (e.g., swine, human, avian) require specific sialic acid receptors on the host animal cell that the viral protein hemagglutinin binds to (18); these will be further delineated in the applicable subsections below.
Influenza viruses infect the respiratory or digestive tracts of their host animals (19). Influenza cases across humans, poultry, and swine can range from a low pathogenicity (asymptomatic or mild respiratory signs) to high pathogenicity, causing pneumonia, severe neurological problems, and high mortality (13, 20). Crucially, low pathogenicity (no or mild symptoms) in one species does not necessarily indicate low pathogenicity of the same strain in humans [(13), p. 7]. It is thought that the viruses replicate primarily in the animals’ intestinal or respiratory tracts and remain active in feces, saliva, or other discharges for weeks. Transmission takes place through direct contact with infected saliva, nasal secretions, or feces, or occurs via airborne particles or contaminated equipment such as vehicles and clothing (19). Transmission via the consumption of properly cooked chicken meat or pork is not currently thought possible (21, 22).
Avian and swine influenzas are of the most concern due to several factors. These include the numerous type A subtypes hosted in these animals, the vast numbers of farmed pigs and poultry, how farmed poultry and pigs are kept, the presence of both domesticated and wild birds and swine, and the close proximity of farmed pigs and poultry to humans (13, 17). Hence, the focus hereafter will be on avian, swine, and human influenzas.
3.1 Avian influenza
Avian influenza viruses (AIVs) include H types 1–16 and N types 1–9 (13); this constitutes all of the subtypes available bar two that are only found in bats. The hemagglutinin viral protein requires so-called α2,3-linked sialic acid cell receptors in the host to achieve cell attachment. Wild birds are the natural host for AIVs, especially mallards and gulls. Many species of natural avian influenza hosts are migratory species, which facilitates the international spread of AIVs—epitomized by the spread into South America for the first time in 2022 (13). This is exacerbated further by the global poultry market, as wild birds transmit influenza to poultry. In poultry, all subtypes are low pathogenic types. However, if poultry catch H5 and H7, these can then morph into high pathogenic types, thus avian influenza is a notifiable disease requiring immediate reporting to authorities where suspicion exists.
The world saw its largest highly pathogenic H5N1 avian influenza outbreak in 2022, causing the culling or death of over 130 million domesticated birds globally (23) and at least 50,000 wild birds in the UK alone (24). It has now reached 81 countries and five continents after spreading into the Antarctica in October 2023 (23, 25). There is currently a high risk of avian influenza cases throughout the year in Europe, whereas until recently, it remained a seasonal epidemic in cooler months [(3, 26), p. 812].
In the UK, the bird species most negatively impacted by avian influenza since 2021 are the barnacle goose and the northern gannet (24, 27). Birds can also transmit influenza to a variety of mammals, including humans and pigs. Table 2 highlights the recorded cases of mammals that AIVs have been transmitted to. The table also highlights that H5N1 is the AIV most commonly transmitted to mammals. As well as being recorded in a range of domesticated and wild birds, between January 2022 and July 2023, H5N1 has been recorded in various non-avians globally including sea lions, minks, foxes, badgers, otters, polecats, racoons, cats, dogs, and humans (28). The potential for poultry to serve as mixing vessels for avian influenza is discussed within section 3.3.
3.2 Human influenza
Human influenza viruses prefer α2,6-linked sialic acid receptors for attachment to host cells, which humans have (18). The primary types are H1N1, H3N2, and H1N2 (i.e., mirroring the swine subtypes outlined in the following subsection), with the H1N2 type being a human/swine influenza mix that evolved in the 2000s (13). Humans are natural hosts for certain influenza A viruses, and these (along with some type B viruses) cause the common, mild seasonal influenzas in cooler months of the year, and there will be partial immunity in the population for pre-existing strains. However, there have been four major agreed-upon influenza pandemics affecting humans thus far (and possibly more), as introduced earlier in Table 1: H1N1 causing the Spanish flu in 1918, H2N2 causing the Asian flu in 1957, H3N2 causing the Hong Kong flu in 1968, and H1N1 from North America in 2009. Collectively, the number of casualties were in the millions. Each of these four pandemics is considered zoonotic in that the first three stemmed from avian influenza, and the last one from swine influenza [(13), p. 7; (17), p. 3].
Other zoonotic cases (not reaching pandemic level—i.e., widespread at national or international level), of AIVs in humans between 1959 and 2023, are reported in Table 3. Taking the two subtypes with the highest number of cases, H5N1 and H7N9, the case mortality rates are very high at around 53 and 39%, respectively. Most cases occurred in slaughterhouse workers via airborne transmission, and there is evidence of some viral replication occurring outside of respiratory organs, even including the brain [(13), p. 6]. Based on their review, Abdelwhab and Mettenleiter (13) estimate <3,000 clinical (laboratory-confirmed) cases of AIV infections in humans, as per Table 3, and < 7,000 subclinical (no symptoms, just antibodies) AIV cases. There is particularly concern regarding the continued pandemic potential of H5N1 due to the recent outbreaks in different species, as discussed in section 3.1, and due to genetic variations within the H5N1 strain that have been detected (28).
In terms of swine-origin influenza in humans, Abdelwhab and Mettenleiter (13) reported approximately a 75% higher number of cases globally within the most recent decade 2010–2021 (almost 700 cases), relative to the previous five decades combined, 1959–2014 (under 400 cases). Moreover, both Kessler et al. (17) and Abdelwhab and Mettenleiter (13) link these case increases to anthropogenic factors – see section 4 for further discussion of this. There are also a few cases of human-to-human transmission of avian/swine influenza, but this is still rare at present. A major public health concern is that the next zoonotic influenza mutation could enable sustained human-to-human transmission and lead to a pandemic.
3.3 “Mixing vessel” host animals and swine influenza
In terms of influenzas, a “mixing vessel” is a host animal able to be infected with two or more types of influenza virus at once; such a host animal can then serve as a “fertile breeding ground” for new mixes and strains of a virus [(17), p. 11]. This potentially allows the transmission of genetic material from one strain to the other, and the emergence of a new influenza strain(s), as per the process of antigenic shift mentioned earlier in section 3 [(13), p. 3]. All four major influenza pandemics have stemmed from avian influenzas that formed new types in humans, pigs, or in another currently undetermined mixing vessel host. Further mixing can also occur via reverse zoonotic infection, whereby influenzas within humans can transmit to other animals, allowing further mixing to occur with any other influenza strains present within those animals [(31), p. 615; (17), p. 10].
Pigs are natural hosts for several influenza viruses, though of fewer types than birds; the primary types of influenza today are H1N1, H1N2, and H3N2, and cases have occurred globally. These stem from genetic mixes of human, avian, and swine influenzas, largely arising from transmissions to pigs during human and avian influenza epidemics (17). Pigs can host an unusually high number of influenza strains, many of which can infect other species (Table 2); hence pigs are particularly well-suited to serving as mixing vessels. This is based on the presence of both the so-called α2,6-linked as well as α2,3-linked sialic acid cell receptors in pigs to which the viral hemagglutinin of both human influenzas and avian influenzas can attach, respectively [(32), p. 161].
Despite pigs’ infamous reputation as a mixing vessel, according to the 2023 review by Abdelwhab and Mettenleiter, there is also a high risk of numerous other animals acting as mixing vessels, as they also have both types of sialic acid cell receptors meaning they can receive both human and avian influenzas. Most worryingly, humans, chickens, and other poultry are included among the list of “high probability” species cited (Figure 1). Thus, concern for mixing vessels within the farming sector should not end with pigs, and in the case of the planning applications outlined in the introduction, there is a triple threat of mixing vessel hosts in close proximity to each other: pigs, chickens, and humans.
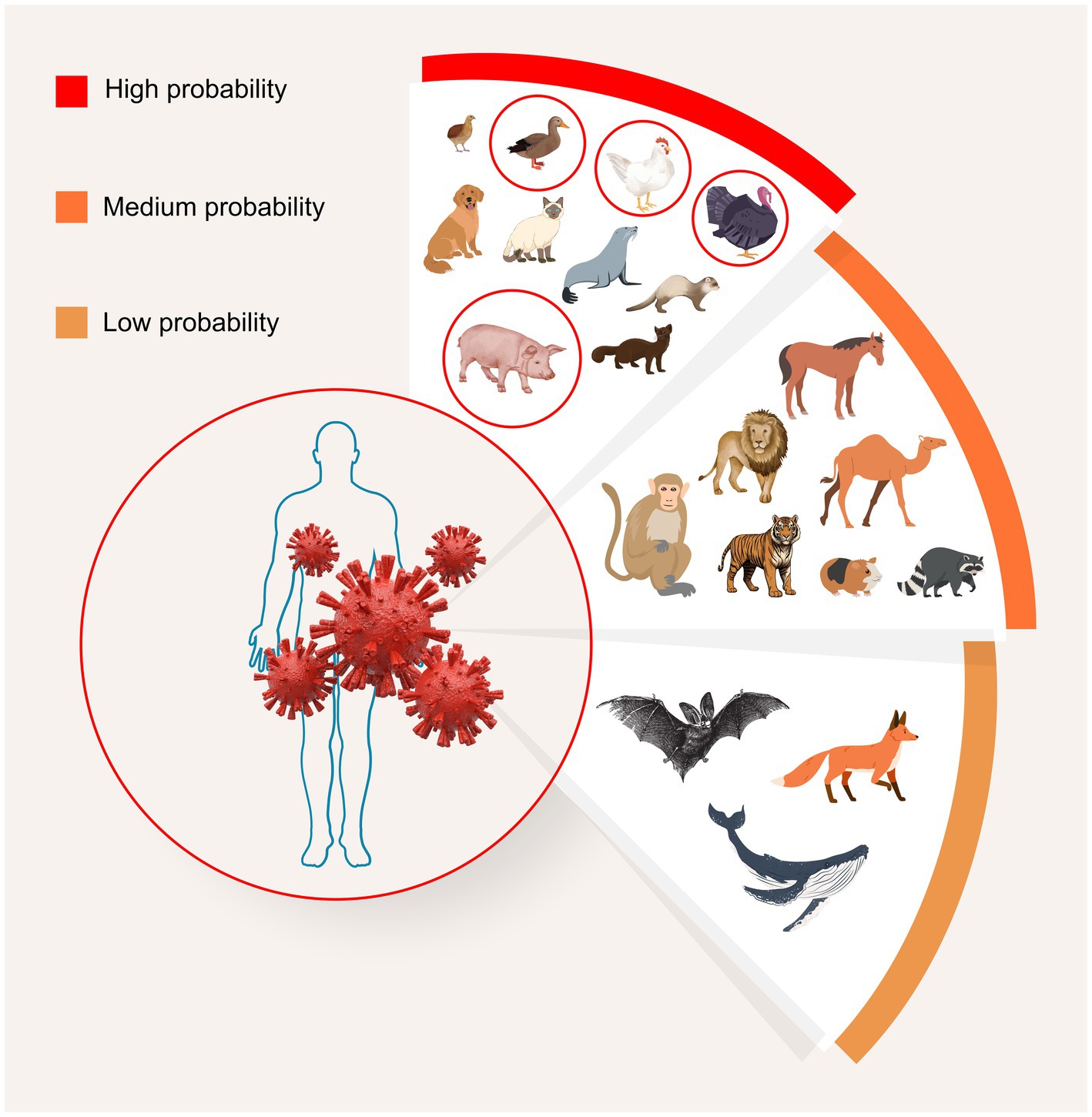
Figure 1. Potential for avian influenza viral mixing, and emergence of new variants, among various animal species. Potential mixing vessel hosts are grouped based on frequency of infection, human proximity, high population numbers, and presence of both avian- and human-type receptors. A diverse range of type A subtypes can be found among different animals in each group. “High probability” mixing vessel hosts include humans, pigs, minks, ferrets, seals, dogs, cats, and birds, particularly turkeys, chickens, quails, and ducks. “Medium probability” hosts are nonhuman primates, raccoons, camels, pikas, zoo animals, including tigers and lions, and horses. “Low probability” hosts include foxes, bats, and whales. After Abdelwhab and Mettenleiter (13). Image: MAD Ideas.
4 Causes of high zoonotic risks in farmed animals
Multiple factors increase viral transmission and infection risks for domesticated species. Through an analysis of published data, Johnson et al. (33) (p. 6) calculated that there is an average of 19.3 viruses in domesticated mammals (although 31 exist in pigs, including hepatitis E) such as Rift Valley fever from the phlebovirus genus; this is in contrast to an average of 0.3 viruses in noncaptive wild mammals, including hantaviruses and henipavirsues (for more examples of viral zoonoses see Table 1). A plethora of studies and reports indicate that the following eight factors account for the higher risk of viral infection among domesticated mammals [(e.g., 8, 11, 17, 34–36)]:
1. High population numbers among farmed pigs, farmed chickens, and humans
2. How the vast majority of farmed pigs and chickens are kept
3. How the vast majority of farmed pigs and chickens are bred
4. Close proximity of farmed pigs and chickens to humans (staff)
5. The globalized and international nature of IIAF
6. The high land/resource requirements of IIAF disrupting ecosystems and increasing proximity to wild animals
7. The locations of IIAF operations
8. Misperceptions of low risks to and posed by farmed animals
Each of the preceding eight points will be addressed in turn, with examples focusing on pigs and chickens, as they are the most consequential farmed species for the purposes of this study. The eight points are also interdependent, as highlighted by Morand (34, 35) within modeling studies. These anthropogenic factors function together to create what is effectively a human-made reservoir, as described by Kessler et al. (17). This human-made reservoir increases the zoonotic risk not just in one way, but in three ways: increased risk of disease emergence, disease amplification (fast replication), and disease transmission [(11), p. 137].
First, the human population surpassed eight billion in November 2022, with a medium projected forecast of 10.4 billion by the 2080s (37). Much of this growth is occurring within developing countries. Driven by the adoption of westernized lifestyles, greater disposable incomes, and industry lobbying, consumption of animal products is also increasing across the globe (8). Jointly, these factors greatly increase the demand for animal produce, and to produce the quantities demanded, while maximizing profits, IIAF has become the norm. This has resulted in enormous farmed animal populations. These greatly surpass the human population—over 80 billion farmed terrestrial vertebrates were slaughtered globally in the most recent available year of 2021 (38), and by 2021 there were well over 25 billion chickens alive at any one time (38). The vast majority (over 70 billion) of the animals slaughtered each year are meat chickens, as they are slaughtered at the age of 6 weeks on average, resulting in a very rapid turnover (38). Additionally, there are close to 1 billion pigs alive at any one time (39). These concurrent massive populations mean less space on Earth for wildlife, more pressures on wildlife, and closer living proximity between humans, chickens, and pigs. This increases the frequency of interaction between humans/pigs/chickens and wild animals, and increases the risks of disease transmission (see the seventh point below for further discussion of this). As an example, Figure 2 highlights the correlation between the number of farmed chickens’ slaughtered over time and the number of human cases of the avian flu subtype generating the most concern (H5N1).
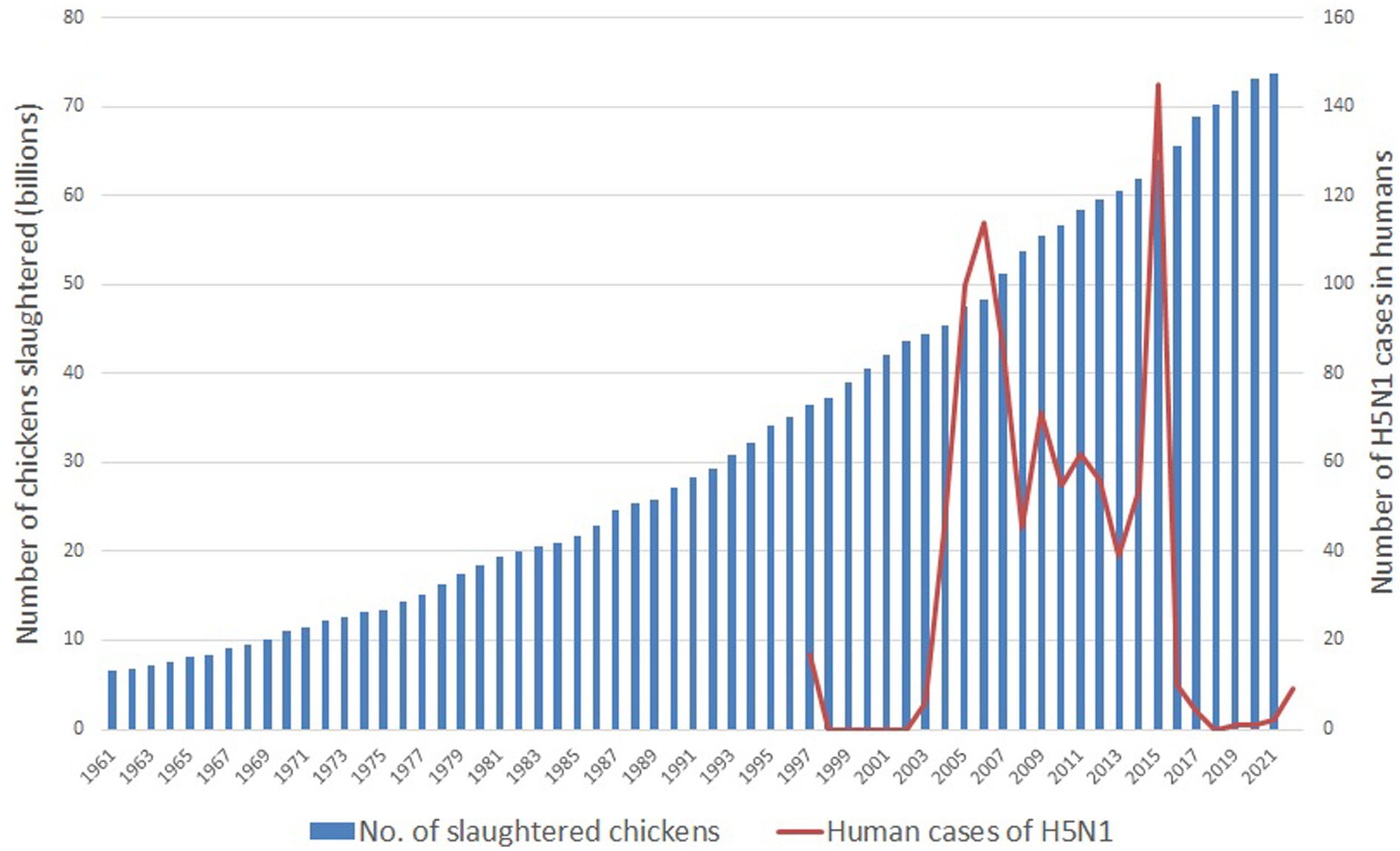
Figure 2. Global number of farmed chickens slaughtered and global cases of H5N1 in humans between 1961 and 2022. An H5 vaccine was introduced in 2017 [(17), p. 5]. H5N1 case data are from the CDC (40) with records beginning in 1997; number of chickens slaughtered are from the UN FAO (38). After Kessler et al. (17).
Second, these high farmed animal populations necessitate certain high-stress living conditions to make the rearing and slaughter of over 80 billion land animals per year feasible. These include high stocking numbers, which facilitate disease transmission (17). The latest UK data demonstrate a 7% increase since 2016 in IIAF operations, with poultry and pig farms comprising the entirety of this number [(41), pp. 59–61], meaning there were over 1,700 such farms by 2023 (i.e., housing at least 40,000 poultry, 2,000 meat pigs, or 750 breeding sows) in the UK. However, there was also a conservative estimate of 1,100 “megafarms,” with the four biggest UK poultry farms stocking 1 million birds each, and the biggest three UK pig farms housing 20,000 pigs each. Lymbery (41) suggests that the increase in these megafarms occurs subtly, often being due to expansions of current farms, rather than the construction of completely new operations—i.e., just as is being proposed in the planning applications cited in the introduction of this review.
Alongside very high stocking numbers of poultry and pigs, are very high stocking densities. The legal maximum stocking density of meat chickens in the UK is 39 kg/m2 or roughly 17 chickens per m2 (42). High stocking densities are known to increase stress in farmed animals, which, if chronic—as is normal under such conditions, can lead to impairment of the immune system (immunocompromisation) and an impaired ability to resist disease (36). The inhibition of many highly motivated chicken and pig behaviors within such crowded conditions also contributes greatly to high stress levels, as does the lack of environmental enrichment/stimulation (36). For instance, pigs in the UK often resort to tail biting in the absence of other exploration-based and foraging enrichment (43), and sows are confined to farrowing crates for around a month surrounding birth. These crates are too small for the sow to even turn around in (44). Poor litter quality also frequently prevents dust-bathing behavior in meat chickens (43).
Moreover, interruptions to social bonds can occur, creating another stressor. Examples include piglets being weaned prematurely and their social group being frequently changed (45). Other significant stressors are physical restraint methods, and mutilations. For instance, despite routine tail docking of piglets being against the law in the UK, in practice, around 80% of piglets have their tails docked, and without anaesthetic (46) even though this is a painful procedure. Feed can also be restricted. Among those most affected by this are so-called “broiler breeders”—the parents of meat chickens—where feed is restricted to slow growth (which would otherwise occur very rapidly in these fast-growing breeds) and to maintain reproductive ability. Such feed restriction is an additional stressor (43). Sanitation is often an additional physiological stressor. For instance, meat chickens’ barns will typically not be cleaned once during their short 6-week life (43). Suboptimal or broken ventilation systems and waste disposal can also lead to pathogen release [(17), p. 10] and further elevated stress levels, if the animals are forced to endure temperature extremes during adverse weather. The links between substantial and chronic stress in farmed animals, resultant immunocompromisation, and consequently increased disease susceptibility, cannot be overstated.
Third, IIAF has used selective breeding over many decades to create the pigs and chickens used in the industry today. The focus within such breeding has been primarily on optimizing the productivity of animals. This may impair the animals’ welfare (i.e., cause stress, as problematized in the previous point above) if aspects of their breeding are likely to cause them harm. For instance, meat chickens have been genetically selected for growth rates that are now over 400% of those less than 40 years ago. This means their physiological and skeletal systems often struggle to cope with the speed at which they put on weight, leading to lameness and thus reduced mobility (36). Pigs have been genetically selected for larger litter sizes. This has led to increased sow size, intensified sow milk production, and aggressive competition for teats among piglets (45, 47). Pigs’ increased growth rates have led to increased lameness and metabolic disease [(48), p. 181]. Moreover, in IIAF, the animals are all bred to be genetically very similar, which reduces the populations’ resistance (“hybrid vigor”) to novel threats such as pathogens [(17), p. 10].
Fourth, IIAF necessitates close proximity of staff (including farm staff, transport staff, and slaughterhouse workers), to an exceedingly high number of animals. These humans are the most at risk of being infected with a zoonosis (13). Fifth, IIAF exists within an international market involving the annual live transportation of over 2 billion farmed animals nationally and internationally with journey durations of hours to weeks. Akin to bird migration, this market has the potential to easily spread new pathogens around the world (36). However, distinct from migratory birds, these farmed animals normally experience more severe physical and emotional stress during their journeys, due to cramped conditions, foreign environments, lack of autonomy, changes to social dynamics, thwarted highly motivated behaviors, exhaustion, hunger, dehydration, fear, discomfort, and physical injuries (36).
Sixth, the considerable impact of IIAF on the climate has become more widely known, albeit scarcely acted upon. This is relevant to this study’s focus as climate change means changes to ecosystems, which often increase the risk of zoonoses (7). However, less frequently discussed are the broader and additional environmental impacts of IIAF. While cattle farming is usually the worst offender from an environmental perspective, other kinds of intensive animal farming, including of chickens and pigs, still demand more resources than arable farming does and contribute to considerable environmental pollution (49, 50). The high use of resources necessitates habitat destruction, which is the leading cause of the sixth mass extinction event since fossil records began, which is currently underway globally [(51), p. 317]. The resultant profound reduction in biodiversity includes a reduction in species that are less likely to harbor pathogens, increases the proximity of wild animals to humans, and disrupts ecosystems—all factors leading to increased risks of zoonoses emerging (11).
Seventh, IIAF operations often congregate in clusters. Norfolk is already one of the IIAF hotspots in the UK, second only to Herefordshire and Shropshire (41), and it is within this very region that the pig and poultry farm expansions have been proposed, as outlined in the introduction. Moreover, IIAF operations are often situated in rural areas close to wildlife habitats, increasing the risk of pathogen “spillover” from wild animals into farmed animals, who then act as a “bridge” for pathogen transmission into humans [(36), p. 330]. Of course, other geographic considerations can also increase the zoonotic risk such as farm positions in close proximity to migratory paths of wild birds, congregation sites of wild birds (e.g., nesting, reproduction), slaughterhouses, and fragile ecosystems [(52), p. 6].
Finally, the EFSA (European Food Safety Authority) is beginning to challenge the perception of some farmed animal types as low risk in terms of zoonosis threat such as meat chickens or so-called breeder chickens [(53), p. 5]. Indeed, there seems abundant recent evidence to support the position that all farmed chickens are at high risk, with numerous cases of avian influenza being found in meat chicken farms and indoor farms, including when under avian influenza restrictions over Winter 2022–2023 when many captive birds were under heightened biosecurity practices such as in the UK [(54, 55); EFSA, personal communication, September 20, 2023].
5 Recommendations for minimizing zoonotic disease risk from farmed animals
Before focusing on recommendations for particular actions, it is important that we recommend against “silver bullet” thinking—the misperception that any one action alone will suffice to reduce zoonotic risks to an adequately low level. An example of such silver bullet thinking would be an overdependence on biosecurity and vaccinations. First, biosecurity practices alone are proving insufficient at lowering the risk of zoonotic disease to a manageable level, as demonstrated by indoor systems with good biosecurity that are still acquiring influenzas (11). To explain this, Kessler et al. (17, p. 10) state, “an exchange of pathogens with the environment can never be fully prevented.” Moreover, they reject the assumption that huge, bio-contained holdings are necessary for controlling influenza viruses. Stevenson (11) (p. 138) highlights how optimized biosecurity may reduce the frequency of zoonoses arising within IIAF, but the nature of IIAF increases their severity when outbreaks do occur. Second, the nature and behavior of viruses and mutations they undergo (see antigenic drift and antigenic shift in section 3) are notoriously difficult to predict. Thus, there will always be gaps in knowledge, and humans will always be on the backfoot playing catch-up in relation to vaccine development. Nevertheless, any trade embargos on vaccinated animals should cease, so as to aid development in this arena (56).
Neither the authors nor the scientific literature suggest that optimizing biosecurity and research into vaccinations is redundant, but given the multifactorial causes of zoonosis outbreaks described in section 4, it is clear that a more holistic approach is required. The very nature of IIAF unavoidably increases zoonosis risks. Accordingly, animal farming needs to (1) de-intensify and (2) adequately safeguard animal welfare. A reduced intensity of animal farming will necessarily mean a reduction in public consumption of animal products. Kessler et al. (17) and Stevenson (11) describe this vision of animal farms as “health-oriented” systems not dependent on routine antibiotic use. Such a vision aligns with the approaches of One Health and One Welfare that recognize the interdependency of human, animal, and planetary health (17, 34, 35) as well as the Sustainable Development Goals (35). Stevenson (11) and CIWF (56) frame such a vision as “radical restructuring” of poultry and pig farming.
Based on recommendations also from the EFSA, as a first key step, Stevenson (11) and CIWF (56) recommend reducing meat chicken stocking densities from an average of 38 kg/m2 in the UK down to 11 kg/m2 (around five chickens/m2). These constitute estimated maximum densities at which highly motivated behaviors can still be fulfilled—which are essential for minimizing stress and safeguarding immune system health, and therefore, essential for minimizing zoonoses risks. Similarly, for pigs, Stevenson (11) calls for a reduction in density from over two pigs/m2 down to one pig/1.5 m2 (assuming a 100 kg pig). Stevenson (11) also calls for reducing clusters of operations to reduce transmission risks, as dust particles can carry viruses and be wind-carried between farms. There are also calls for improved breeding practices—away from a focus on maximizing productivity, and toward lowered susceptibility to infection, and optimal overall health and welfare (57).
Extant research typically also offers guidance for further research. For instance, Tomori and Oluwayelu (7) highlight that little is known about the number of zoonotic viruses that truly exist, and how this can lead to an underestimation of zoonotic effects. Thus, more general research is always required to advance understanding and knowledge of zoonoses, as also recommended by Guégan et al. (58). There is also room for methodological streamlining within future research. For instance, Abdelwhab and Mettenleiter (13) describe how direct comparisons between different studies are often not possible as different studies have used different methods to identify certain viral components. Finally, of note is that influenza viruses can survive for less time outdoors due to sunlight inactivating them [(36), p. 325]; hence, this is a benefit of small outdoor farms to consider and potentially examine further.1
6 Conclusion
The disease risks to humans from industrialized intensive animal farming, and especially mixed swine and poultry farms, are enormous, and must not be understated. Psychological and physiological stress in farmed animals needs to be substantially reduced to maintain their immunocompetence, and thus minimize disease risks. In particular, the latest scientific evidence concerning influenza viruses compounds the concerns about “mixing vessel” hosts; no longer are concerns about mixing vessels limited to pigs, but also several other animal species, and at a level of high probability—most notably chickens and humans. The authors therefore strongly discourage granting any planning applications for new or expanding industrialized intensive animal farms, especially poultry and pig farms or a mix thereof, and especially in areas with high existing concentrations of intensive animal farms. Instead, efforts should concentrate on supporting arable agriculture (or transitions toward this), and on de-intensifying remaining animal farms. This aligns with One Health and One Welfare approaches, which foreground the protection of both animal and human health and welfare as integral to any animal farm. As a recommended first step, stocking densities should be lowered to around five meat chickens/m2 (11 kg/m2) and to one pig/1.5 m2 (assuming a 100 kg pig).
Author contributions
JM: Conceptualization, Data curation, Formal analysis, Investigation, Methodology, Project administration, Resources, Software, Supervision, Validation, Visualization, Writing – original draft, Writing – review & editing. AK: Conceptualization, Funding acquisition, Project administration, Supervision, Validation, Visualization, Writing – review & editing.
Funding
The author(s) declare financial support was received for the research, authorship, and/or publication of this article. This research was funded by Humane Being (UK) (grants August 31, 2023 and September 18, 2023). Open access publication fees were funded by the Karuna Foundation, craigslist Charitable Fund and Greenbaum Foundation. These funders played no role in study design, data collection and analysis, preparation of the resultant manuscript, nor decisions relating to publication.
Conflict of interest
The authors declare that the research was conducted in the absence of any commercial or financial relationships that could be construed as a potential conflict of interest.
Publisher’s note
All claims expressed in this article are solely those of the authors and do not necessarily represent those of their affiliated organizations, or those of the publisher, the editors and the reviewers. Any product that may be evaluated in this article, or claim that may be made by its manufacturer, is not guaranteed or endorsed by the publisher.
Footnotes
1. ^Extensive farming has not been the focus of this review due to the dominance of and increasing global trend toward IIAF (59). Despite the reduced disease risks typically associated with more extensive farming types (e.g., stemming from lower stocking densities, more genetic diversity, higher welfare, less stress, and thus a more robust immune system), the authors acknowledge continued debate about potential higher enzootic disease risks stemming from some extensive farming types [(e.g., 45, 60, 61), p. 65]. Moreover, without significantly lower consumer demand, extensive/higher-welfare farming methods will require even more land than IIAF, further increasing the interface between humans and animals [e.g., (see 62, 63)]. Thus, the authors’ recommendations are for both less intensity in animal farming and lower demand for animal products. More research and support is also required into enhanced means of lowering the general enzootic burden, which includes influenza viruses, especially in developing countries which are most impacted by these (64, 65).
References
1. Borough Council of King’s Lynn and West Norfolk. Planning – application summary, 22/00860/FM (pigs). (2022). Available at: https://online.west-norfolk.gov.uk/online-applications/applicationDetails.do?activeTab=summary&keyVal=RBTQU0IV00I00 (Accessed September 6, 2023).
2. Borough Council of King’s Lynn and West Norfolk. Planning – application summary, 22/00866/FM (poultry). (2022). Available at: https://online.west-norfolk.gov.uk/online-applications/applicationDetails.do?activeTab=summary&keyVal=RBZ32NIV05900. (Accessed September 6, 2023).
3. Hunter, P . Europe’s worst ever bird flu outbreak. EMBO Rep. (2022) 23:e56048. doi: 10.15252/embr.202256048
4. Daniels, RS, and McCauley, JW. The health of influenza surveillance and pandemic preparedness in the wake of the COVID-19 pandemic. J Gen Virol. (2023) 104:001822. doi: 10.1099/jgv.0.001822
5. Maclachlan, NJ, and Dubovi, EJ. Fenner’s veterinary virology. Cambridge, MA: Academic Press (2017).
6. WHO Zoonoses. (2020). Available at: https://www.who.int/news-room/fact-sheets/detail/zoonoses (Accessed September 6, 2023).
7. Tomori, O, and Oluwayelu, DO. Domestic animals as potential reservoirs of zoonotic viral diseases. Annu Rev Anim Biosci. (2023) 11:33–55. doi: 10.1146/annurev-animal-062922-060125
8. UNEP Preventing the next pandemic: zoonotic diseases and how to break the chain of transmission. (2020). Available at: https://unsdg.un.org/sites/default/files/2020-07/UNEP-Preventing-the-next-pandemic.pdf (Accessed September 6, 2023).
9. Bodo, T, Gimah, BG, and Seomoni, KJ. Deforestation and habitat loss: human causes, consequences and possible solutions. J. Geogr. Res. (2021) 4:22–30. doi: 10.30564/jgr.v4i2.3059
10. Scanes, CG . Human activity and habitat loss: destruction, fragmentation, and degradation In: CG Scane and SR Toukhsati, editors. Animals and human society. Cambridge, MA: Academic Press (2017). 451–82.
11. Stevenson, P . Links between industrial livestock production, disease including zoonoses and antimicrobial resistance. Anim Res One Health. (2023) 1:137–44. doi: 10.1002/aro2.19
12. Mulchandani, R, Wang, Y, Gilbert, M, and Van Boeckel, TP. Global trends in antimicrobial use in food-producing animals: 2020 to 2030. PLoS Global Public Health. (2023) 3:e0001305. doi: 10.1371/journal.pgph.0001305
13. Abdelwhab, EM, and Mettenleiter, TC. Zoonotic animal influenza virus and potential mixing vessel hosts. Viruses. (2023) 15:980. doi: 10.3390/v15040980
14. Olival, KJ, Hosseini, PR, Zambrana-Torrelio, C, Ross, N, Bogich, TL, and Daszak, P. Host and viral traits predict zoonotic spillover from mammals. Nature. (2017) 546:646–50. doi: 10.1038/nature22975
15. CDC Types of influenza viruses. (2023). Available at: https://www.cdc.gov/flu/about/viruses/types.htm (Accessed November 21, 2023).
16. Imperia, E, Bazzani, L, Scarpa, F, Borsetti, A, Petrosillo, N, Giovanetti, M, et al. Avian influenza: could the H5N1 virus be a potential next threat? Microbiol Res. (2023) 14:635–45. doi: 10.3390/microbiolres14020045
17. Kessler, S, Harder, TC, Schwemmle, M, and Ciminski, K. Influenza a viruses and zoonotic events—are we creating our own reservoirs? Viruses. (2021) 13:2250. doi: 10.3390/v13112250
18. de Vries, E, Du, W, Guo, H, and Hann, CAM. Influenza a virus hemagglutinin–neuraminidase–receptor balance: preserving virus motility. Trends Microbiol. (2020) 28:57–67. doi: 10.1016/j.tim.2019.08.010
19. WHO Influenza (avian and other zoonotic): key facts. (2018). Available at: https://www.who.int/news-room/fact-sheets/detail/influenza-(avian-and-other-zoonotic) (Accessed September 6, 2023).
20. Kandeil, A, Patton, C, Jones, JC, Jeevan, T, Harrington, WN, Trifkovic, S, et al. Rapid evolution of a(H5N1) influenza viruses after intercontinental spread to North America. Nat Commun. (2023) 14:3082. doi: 10.1038/s41467-023-38415-7
21. ECDC Factsheet on swine influenza in humans and pigs. (2023). Available at: https://www.ecdc.europa.eu/en/swine-influenza/factsheet (Accessed November 23, 2023).
22. Harder, TC, Buda, S, Hengel, H, Beer, M, and Mettenleiter, TC. Poultry food products—a source of avian influenza virus transmission to humans? Clin Microbiol Infect. (2016) 22:141–6. doi: 10.1016/j.cmi.2015.11.015
23. WHO Ongoing avian influenza outbreaks in animals pose risk to humans. (2023). Available at: https://www.who.int/news/item/12-07-2023-ongoing-avian-influenza-outbreaks-in-animals-pose-risk-to-humans (Accessed November 25, 2023).
24. Weston, P., and Kevany, S. More than 50,000 wild birds in UK killed by avian flu – double previous estimates. The Guardian (2023). Available at: https://www.theguardian.com/environment/2023/may/05/50000-wild-birds-uk-killed-h5n1-avian-flu-double-estimates-aoe (Accessed November 25, 2023).
25. BAS [British Antarctic survey] First confirmed cases of Avian Influenza in the Antarctic region. (2023). Available at: https://www.bas.ac.uk/media-post/first-confirmed-cases-of-avian-influenza-in-the-antarctic-region (Accessed November 25, 2023).
26. Xie, R, Edwards, KM, Wille, M, Wei, X, Wong, S-S, Zanin, M, et al. The episodic resurgence of highly pathogenic avian influenza H5 virus. Nature. (2023) 622:810–7. doi: 10.1038/s41586-023-06631-2
28. Charostad, J, Rukerd, MRZ, Mahmoudvand, S, Bashash, D, Hashemi, SMA, Nakhaie, M, et al. A comprehensive review of highly pathogenic avian influenza (HPAI) H5N1: an imminent threat at doorstep. Travel Med Infect Dis. (2023) 55:102638. doi: 10.1016/j.tmaid.2023.102638
29. Roser, M. The Spanish flu: the global impact of the largest influenza pandemic in history. (2020). Available at: https://ourworldindata.org/spanish-flu-largest-influenza-pandemic-in-history (Accessed November 25, 2023).
30. Alexander, DJ, Capua, I, and Koch, G. Highly pathogenic avian influenza outbreaks in Europe, Asia, and Africa since 1959, excluding the Asian H5N1 virus outbreaks In: DE Swayne , editor. Avian influenza, vol. 2008. Ames, IA: Blackwell Publishing (2008). 217–38.
31. Henritzi, D, Petric, PP, Lewis, NS, Graaf, A, Pessia, A, Starick, E, et al. Surveillance of European domestic pig populations identifies an emerging reservoir of potentially zoonotic swine influenza a viruses. Cell Host and Microbe. (2020) 28:614–627.e6. doi: 10.1016/j.chom.2020.07.006
32. Ma, W, Kahn, RE, and Richt, JA. The pig as a mixing vessel for influenza viruses: human and veterinary implications. J. Mol. Genet. Med. (2009) 3:158–66. doi: 10.4172/1747-0862.1000028
33. Johnson, CK, Hitchens, PL, Pandit, PS, Rushmore, J, Evans, TS, Young, CCW, et al. Global shifts in mammalian population trends reveal key predictors of virus spillover risk. Proc R Soc B Biol Sci. (2020) 287. doi: 10.1098/rspb.2019.2736
34. Morand, S . Emerging diseases, livestock expansion and biodiversity loss are positively related at global scale. Biol Conserv. (2020) 248:108707. doi: 10.1016/j.biocon.2020.108707
35. Morand, S . The role of agriculture in human infectious disease outbreaks. CABI Rev. (2022). doi: 10.1079/cabireviews202217060
36. Schuck-Paim, C, Alonso, WJ, and Slywitch, E. Animal welfare and human health In: A Knight, C Phillips, and P Sparks, editors. Routledge handbook of animal welfare. Abingdon: Routledge (2023). 321–35.
37. UN DESA World population prospects 2022: summary of results. (2022). Available at: https://www.un.org/development/desa/pd/sites/www.un.org.development.desa.pd/files/wpp2022_summary_of_results.pdf (Accessed September 6 2023).
38. UN FAO FAOSTAT: crops and livestock products. (2023). Available at: https://www.fao.org/faostat/en/#data/QCL (Accessed September 17, 2023).
39. Our World in Data. Meat and dairy production. (2021). Available at: https://ourworldindata.org/meat-production#number-of-animals-slaughtered (Accessed September 6, 2023).
40. CDC Past reported global human cases with highly pathogenic avian influenza a(H5N1) (HPAI H5N1) by country, 1997–2023. (2023). Available at: (https://www.cdc.gov/flu/avianflu/chart-epi-curve-ah5n1.html).
41. Lymbery, P . Sixty harvests left: how to reach a nature-friendly future. London: Bloomsbury Publishing (2023).
42. Defra. Code of practice for the welfare of meat chickens and meat breeding chickens. (2018). Available at: https://assets.publishing.service.gov.uk/government/uploads/system/uploads/attachment_data/file/694013/meat-chicken-code-march2018.pdf (Accessed September 6, 2023).
43. Widowski, TM, and Rentsch, AK. Farming poultry In: A Knight, C Phillips, and P Sparks, editors. Routledge handbook of animal welfare. Abingdon: Routledge (2023). 47–63.
44. McCulloch, S . The UK must ban farrowing crates. Vet Rec. (2022) 191:262. doi: 10.1002/vetr.2264
45. Edwards, S . Farming pigs In: A Knight, C Phillips, and P Sparks, editors. Routledge handbook of animal welfare. Abingdon: Routledge (2023). 64–75.
46. Morrison, R, and Hemsworth, P. Tail docking of piglets 2: effects of meloxicam on the stress response to tail docking. Animals. (2020) 10:1–9. doi: 10.3390/ani10091701
47. CIWF Selective breeding: How gene editing will perpetuate animal suffering. (2022). Available at: https://www.ciwf.org.uk/media/7452117/selective-breeding-report-september-2022.pdf (Accessed September 6, 2023).
48. Van Marle-Köster, E, and Visser, C. Unintended consequences of selection for increased production on the health and welfare of livestock. Arch Anim Breed. (2021) 64:177–85. doi: 10.5194/aab-64-177-2021
49. Poore, J, and Nemecek, T. Reducing food’s environmental impacts through producers and consumers. Science. (2018) 360:987–92. doi: 10.1126/science.aaq0216
50. Scarborough, P, Clark, M, Cobiac, L, Papier, K, Knuppel, A, Lynch, J, et al. Vegans, vegetarians, fish-eaters and meat-eaters in the UK show discrepant environmental impacts. Nature Food. (2023) 4:565–74. doi: 10.1038/s43016-023-00795-w
51. Fischer, B . Climate change, human-wildlife conflict, and biodiversity loss In: A Knight, C Phillips, and P Sparks, editors. Routledge handbook of animal welfare. Abingdon: Routledge (2023). 311–20.
52. Alarcón, L, Alberto, A, and Mateu, E. Biosecurity in pig farms: a review. Porcine Health Manag. (2021) 7:5. doi: 10.1186/s40813-020-00181-z
53. Adlhoch, C, Fusaro, A, Gonzales, JL, Kuiken, T, Marangon, S, Niqueux, É, et al. Avian influenza overview September – December 2021. EFSA J. (2021) 19:e07108. doi: 10.2903/j.efsa.2021.7108
54. Defra. Notifiable animal disease cases and control zones. (2023). Available at: https://www.gov.uk/animal-disease-cases-england (Accessed September 6, 2023).
55. Gonzales, JL, Hobbelen, PHF, Van Der Spek, AN, Vries, EP, and Elbers, ARW. Highly pathogenic avian influenza a H5 virus outbreaks in broiler farms in the Netherlands – clinical signs, transmission and identification of reporting thresholds. Biorxiv. (2023). doi: 10.1101/2023.01.05.522008
56. CIWF Bird flu: only major farm reforms can end it. (2023). Available at: https://www.ciwf.org.uk/research/animal-welfare/bird-flu-only-major-farm-reforms-can-end-it/ (Accessed September 6, 2023).
57. Ruiz-Hernandez, R, Mwangi, W, Peroval, M, Sadeyen, JR, Ascough, S, Balkissoon, D, et al. Host genetics determine susceptibility to avian influenza infection and transmission dynamics. Sci Rep. (2016) 6:26787. doi: 10.1038/srep26787
58. Guégan, J-F, Roche, B, and Morand, S. Biodiversity and human health: on the necessity of combining ecology and public health In: A Hector, F Isbell, and M Loreau, editors. The ecological and societal consequences of biodiversity loss. Hoboken, NJ: Wiley (2022). 233–59.
59. Manzoor, S, Syed, Z, and Abubabakar, M. Global perspectives of intensive animal farming and its applications In: S Manzoor and M Abubabakar, editors. Intensive animal farming - a cost-effective tactic. London: IntechOpen (2023)
60. Bartlett, H, Holmes, MA, Petrovan, SO, Williams, DR, Wood, JLN, and Balmford, A. Understanding the relative risks of zoonosis emergence under contrasting approaches to meeting livestock product demand. R Soc Open Sci. (2022) 9:211573. doi: 10.1098/rsos.211573
61. McMullin, P . Infectious diseases in free-range compared to conventional poultry production. Avian Pathol. (2022) 51:424–34. doi: 10.1080/03079457.2022.2086448
62. Meemken, E-M, and Qaim, M. Organic agriculture, food security, and the environment. Ann Rev Resour Econ. (2018) 10:39–63. doi: 10.1146/annurev-resource-100517-023252
63. Smith, LG, Kirk, GJD, Jones, PJ, and Williams, AG. The greenhouse gas impacts of converting food production in England and Wales to organic methods. Nat Commun. (2019) 10:4641. doi: 10.1038/s41467-019-12622-7
64. Maudlin, I, Eisler, MC, and Welburn, SC. Neglected and endemic zoonoses. Philos Trans R Soc B. (2009) 364:2777–87. doi: 10.1098/rstb.2009.0067
Keywords: influenza, avian, poultry, swine, pig, porcine
Citation: Mace JL and Knight A (2023) Influenza risks arising from mixed intensive pig and poultry farms, with a spotlight on the United Kingdom. Front. Vet. Sci. 10:1310303. doi: 10.3389/fvets.2023.1310303
Edited by:
Santiago Mirazo, Universidad de la República, UruguayReviewed by:
Florencia Cancela, Universidad de la República, UruguayMarina Winter, National University of Río Negro, Argentina
Copyright © 2023 Mace and Knight. This is an open-access article distributed under the terms of the Creative Commons Attribution License (CC BY). The use, distribution or reproduction in other forums is permitted, provided the original author(s) and the copyright owner(s) are credited and that the original publication in this journal is cited, in accordance with accepted academic practice. No use, distribution or reproduction is permitted which does not comply with these terms.
*Correspondence: Jenny L. Mace, SmVubnkuTWFjZUB3aW5jaGVzdGVyLmFjLnVr