- 1Department of Genetics, Faculty of Veterinary Sciences, University of Cordoba, Cordoba, Spain
- 2Department of Biosciences, Biotechnologies and Environment, University of Bari ‘Aldo Moro’, Bari, Italy
To date, the biomechanical dynamics in camelids have not been addressed, although it might be a factor that can affect selection and breeding in this species. Therefore, the aim of this article is to conduct curve fitting and discriminant canonical analysis to identify the mathematical function that best captures the dynamics of camel locomotion and to study the impact of kinematic, morphometric, physiological, and phaneroptic variables on gait performance in leisure riding and racing activities in dromedaries, respectively. The cubic function emerged as the most suitable mathematical model to represent the locomotive behavior of camels. Various factors were found to play a pivotal role in the athletic performance of leisure riding and racing dromedary camels. Concretely, angular measurements at the distal fore and rear extremity areas, pelvis inclination, relative volume of the hump, impact forces of the front limbs, post-neutering effects, and the kinematic behavior of the scapula, shoulder, carpus, hip, and foot are the factors that greatly impact gait performance in leisure riding and racing camels. The biomechanical performance at these specific body regions has a profound impact on weight absorption and minimization of mechanic impact during camel locomotion, static/dynamic balance, force distribution, energy of propulsion, movement direction and amplitude, and storage of elastic strain in leisure riding and racing dromedaries. In contrast, other animal- and environment-dependent factors do not exert significant influence on camel gait performance, which can be attributed to species-specific, inherited adaptations developed in response to desert conditions, including the pacing gait, broad foot pads, and energy-efficient movements. The outcomes of our functional data analysis can provide valuable insights for making informed breeding decisions aimed at enhancing animal functional performance in camel riding and racing activities. Furthermore, these findings can open avenues for exploring alternative applications, such as camel-assisted therapy.
1 Introduction
Although dromedary camels (Camelus dromedarius) have been present in the Iberian peninsula since the Roman period (1), the Canarian archipelago is the only Spanish national territory where history, culture, and ecology of these animals have been strongly rooted for more than 600 years (2). The oldest records of the presence of dromedaries in the archipelago coincide with the historical chronicles relating to the process of European colonization of the eastern islands (3). Due to its anatomical-physiological characteristics and its outstanding performance in fatigued draft works in the arid territories of the islands (4, 5), the camel has managed to perpetuate itself in them until the present.
For centuries, the possession of a camel among local farmers was considered a symbol of status and social prosperity as well as a reinforcement of the family livelihood. Unfortunately, the mechanization of agriculture from the last third of the 20th century led to the progressive substitution of camels in rural labor and the migration of entrepreneurs to the tourism sector. Thus, the initiation of the activity of the National Paradors in the late 1950s and the emergence of tourism as an economic activity in the archipelago contributed to the regression of the camel census in rural areas and their functional reorientation. That is, the main productive niche of the camel in the Canary Islands has since become tourist leisure, in which both animal behavior and physical conformation/performance have a notable impact (6).
Considering the census of active breeders, the risk status assigned to the breed was endangered, a condition still in force today (Order AAA/251/2012; Spanish Ministry of Agriculture, Food and Environment). The lack of knowledge about the productive potentials and the underutilization of local animal genetic resources at risk of extinction places them in a scenario of special vulnerability. Although the potential of this camel breed for milk production purposes is preliminary evaluated, camelback leisure riding continues to be the most profitable activity for this camel breed, which has also expanded its geographical distribution to other European countries during the last three decades in an attempt to mitigate the risk of extinction under the potential occurrence of local stochastic phenomena. In this context, the investigation and promotion of the current and future potential of these resources to ensure the sustenance of human beings and the balance of the local environment will help promote existing productive niches and define new ones.
The observable traits expressed by an organism are mainly governed by the inter-locus interaction (epistasis) of alleles for multiple single genes (7). In livestock scenarios, the magnitude and impact of such complex genetic interactions are driven to a great extent both by historical and current uses of animal species and the design and purposes of breeding programs. Indeed, epistatic genes are agreed to be a major feature in the genetic architecture of evolutionarily transcendent phenotypic variation (8). For the particular case of the relationships between physical attributes and gait performance in domestic animal species involved in moderately energy-demanding sports activities, scarce empirical information does exist. As such, selective breeding schemes may fail to reach genetic improvement and interfere with the adaptive natural history of the animals (9).
In the particular case of the Canarian camel, the present study pursues the biomechanical characterization of the breed by means of 2D video captures. Previous related research has performed basic analyses of the biomechanics of camel gaits (10–12) and the elastic extension of tendons (13), identified some environmental factors (e.g., sex and age) affecting the racing performance (14), and studied the morphology of some parts of the distal skeleton (10, 15–17) and the pedal anatomy (18). However, no reference literature reports the exercise- and animal morphometrics and phaneroptic-related factors that are potentially responsible for oscillations in the functional performance of camel locomotion to any degree of accuracy. Thus, the current research primarily aims to perform individual curve estimations through regression analysis to identify the model that best fits camel biomechanics when performing leisure riding or racing activities, for which camel pace is the most prevalent gait (19). Afterward, such curve fitting or functional data analysis approach will solve the need for stakeholders and breeders to identify a model to describe the dynamics of camel locomotion and detect the location and magnitude of differences in gait performance within the evaluated function (20). Following the theoretical body by Saastamoinen and Barrey (21) on the genetics of horse locomotion, this study also hypothesizes that the locomotor performance in dromedary camels is the overall result of the combination of conformational, physiological, and behavioral heritable traits. Indeed, the selection supported by these characters could overcome the generally low heritability of performance traits (22). Hence, after identifying the mathematical model that best describes camel locomotion, this study pursues the identification of the kinematics, animal morphometrics, physiological, and phaneroptic-related variables that significantly impact the gait proficiency of dromedaries in leisure riding and racing activities. The results obtained will aid in refining animal selection strategies for athletic performance in leisure riding and racing activities, as well as in the exploration of different alternatives in which to venture (i.e., assisted therapy) and with which to reinforce the tasks of functional valorization for its sustainable conservation. The methodology proposed is also expected to be translatable in a comparative manner to other camel populations involved in athletic and draft activities, for which these activities constitute the most profitable niches.
2 Materials and methods
2.1 Animal sample and data purge
A total of 130 Canarian dromedaries (72 male and 58 female camels; aged between 18 months and 35 years) were included in this study. These camels were located in three representative breeding locations within Spain: Huelva (Doñana National Park, coordinates 36.972330, −6.427498), Almería (coordinates 36.902180, −2.429520), and Fuerteventura (coordinates 28.186777, −14.158361). Gait evaluation was performed on all the animals, but data collection and extraction were only feasible in those animals that, first, were able to develop any of the three specific gaits performed by dromedaries as described in the literature, namely, walk, pace, and gallop; and second, from which two complete stride cycles could be collected and extracted. Animals that did not fulfill the aforementioned requirements were discarded from the database for further analysis. All animals were deemed healthy after clinical examination by a trained practitioner. No signs of pain or thoracolumbar vertebral alterations were observed.
As a result of this purging process, the number of animals producing data to be considered in statistical evaluations is as follows: 82 animals for walk, 97 for pace, and 10 for gallop. There were some animals (n = 60) that could be evaluated for more than one type of gait. The reduced number of animals that engaged in galloping patterns may be ascribed to the fact that, as described in the literature (18, 23, 24), these animals are inner energetic savers and hence rarely engage in faster patterns of movements except for very concrete situations in which their life may be compromised or threatened, or if trained to do it so as it happens in trained, racing dromedaries. In fact, according to Tharwat et al. (19), although at the beginning of the race most camels gallop, they frequently switch between pacing and galloping throughout the competition. It is intriguing to note that camels can maintain a pace that is nearly as swift as their gallop.
2.2 Biometric variables and force mathematical determination
The development of gaits has been reported to considerably depend not only on the biometry of dromedaries but also on the relative forces that the animals are able to develop. For these reasons, the following variables were considered in the present study.
First, live weight was calculated using the formula by Boujenane (25). Afterward, pull force (maximum and minimum), load force (maximum and minimum), and maximum and minimum power were calculated following the methods described by Delgado et al. (26).
Smoothened movement parameters are adjusted to trigonometric functions based on specific anatomical regions in both the forelimbs and hindlimbs. Given the implication of angles shared between joints, eight angles were considered: angle 1 = cranial angle of the scapula-midway between acromion and head of humerus; angle 2 = midway between acromion and head of humerus-olecranon; angle 3 = olecranon-carpus; angle 4 = carpus-fetlock (metacarpophalangeal joint) on the forelimb; angle 5 = iliac crest-greater trochanter of the femur; angle 6 = greater trochanter of the femur-stifle (knee) joint; angle 7 = knee joint-tarsus; and angle 8 = tarsus-fetlock (metatarsophalangeal joint) on the hindlimb.
The calculation of hump volume (HV = 0.07 L × B × H), hump weight (HW = 0.45 H – 13.8), the ratio HW/HV (fat density), the proportion HV/BW, and the proportion HW/BW was performed using the methods described by Bengoumi et al. (27). Additional indexes reported to condition the quality of movement and kinetics were also calculated, including chest index, cephalic index, pelvic index, dactilothoracic index, weight in cannon index, conformation index, chest height index, and compactness index, upon the methods described by Susana Lopes et al. (28).
The units of measurement for biokinematics variables were set using Dunbar et al. (29) as a reference.
2.3 Experimental setup and video recording
The image collection occurred on a level and solid open ground. Specific lighting conditions were selected to ensure that the animal was not situated in shadowed areas or under lighting that could distort the image capture. Additionally, the color of the animal was taken into account to prevent any potential distortion or misalignment caused by the background color.
To maintain consistency, the camera was positioned at a standardized height of 1 m on a camera stand. This setup was situated 4 m away from the center of balance of the camel. This configuration allowed for capturing the entire animal within the frame during the evaluation. To ensure proper alignment, we followed the guidelines presented by Iglesias et al. (30), which involved marking standard lines on the ground before taking photographs to confirm the animal’s correct positioning.
The image capture process utilized a digital camera (Sony DSC-RX100 SENSOR CMOS Exmor 1.0, 20.1 MP, F1.8–4.9, Zoom 20–100, Optical Zoom 3.6×, 3″ LCD Screen with Image Stabilizer; Sony Electronics, San Diego, CA, United States) in its standard mode. Cameras are strategically positioned to capture the observations without interfering with the animal’s movement along the evaluation track. Dromedaries were recorded from two different views (side view and front view). The images were saved using the Joint Photographic Experts Group (JPEG) compression format.
Before recording, body length and height were measured, and masking tape squares (5 cm × 5 cm) were affixed to the dromedaries’ bodies to serve as joint tracer reference points for data analysis. These markers were placed on the following left-side anatomical landmarks of the dromedaries: cranial angle of the scapula, midway between acromion and head of the humerus (shoulder joint), olecranon (elbow joint), carpus and fetlock (metacarpophalangeal joint) on the forelimb, the iliac crest, greater trochanter of the femur (hip), stifle (knee) joint, point of the hock (tarsus), and fetlock (metatarsophalangeal joint) on the hindlimb, as shown in Figure 1. A trained operator visualized all video sequences and placed video tracers from video analysis software Kinovea software (Kinovea version 0.9.5; Kinovea Org., France). The same operator performed the potential replacement of joint tracers along the video sequences when a disagreement between the real course and the course of the point of the software occurred.
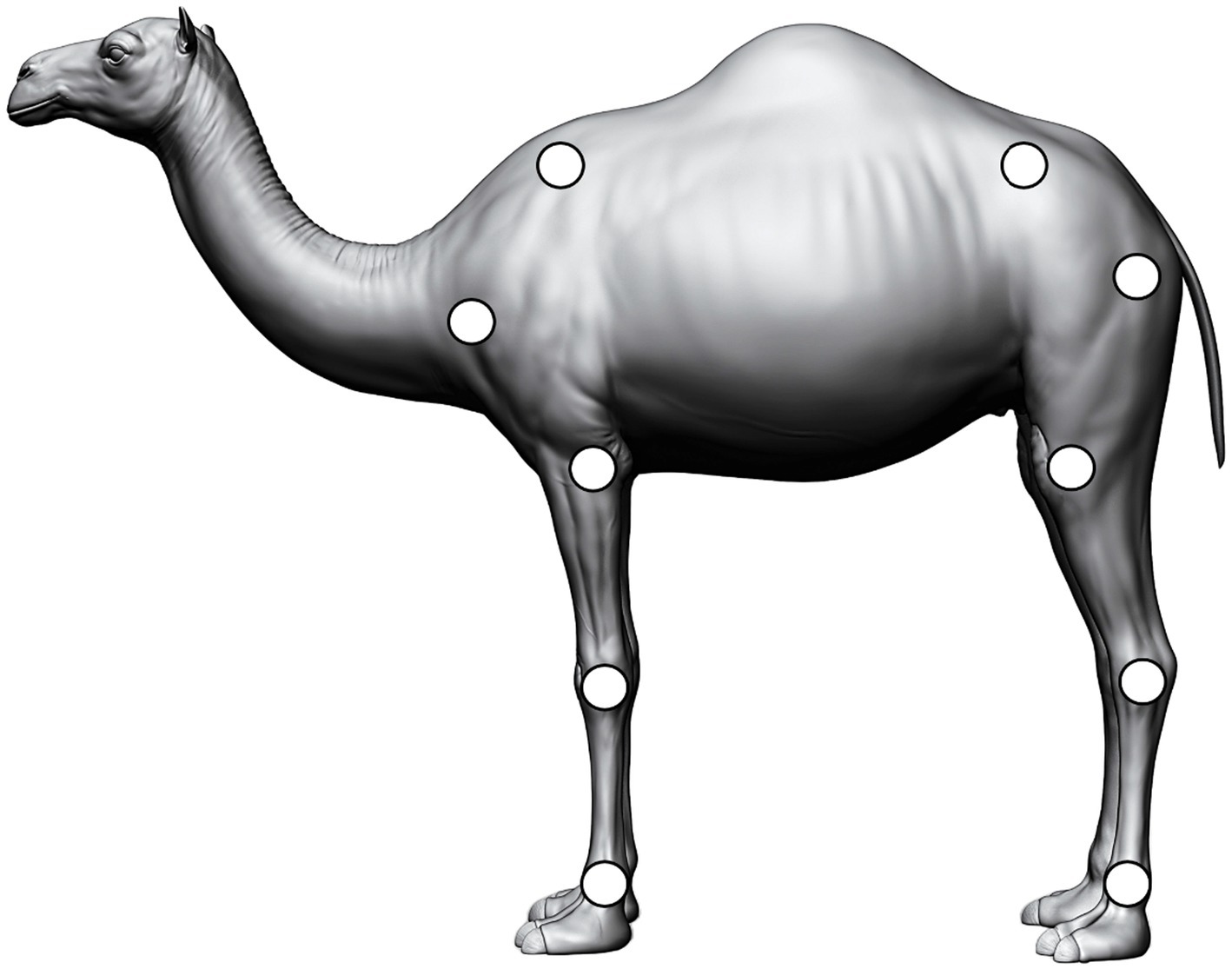
Figure 1. Graphical illustration of the anatomical disposition of masking tape squares (5 cm × 5 cm) at the dromedaries’ bodies to serve as joint tracers reference points for gait analysis.
A wooden pole (100 cm) was positioned parallel to the gait plane to provide a horizontal reference and calibration. The camera, mounted on a tripod, was adjusted to minimize parallax and ensure level alignment. Video footage was collected while the dromedaries were engaged in their regular exercise routines, encompassing walking, pacing, and galloping.
2.4 Qualitative and quantitative stride-based gait analyses
The recording duration corresponds to the time taken by the animal to complete the circuit for each of the three gaits examined (walking, pacing, and galloping), as shown in Figure 2. Two complete stride cycles were considered for each animal. Stride-based gait analysis generally considers the contact of the trailing hindfoot as the starting point of the stride cycle (31). In the relative limb phase, the fraction of a stride in which the left forefoot, right hindfoot, and right forefoot touchdown was calculated following the left hindfoot touchdown. It was calculated by counting the frames of the filmed motion sequences based on the method by Hildebrand (32).
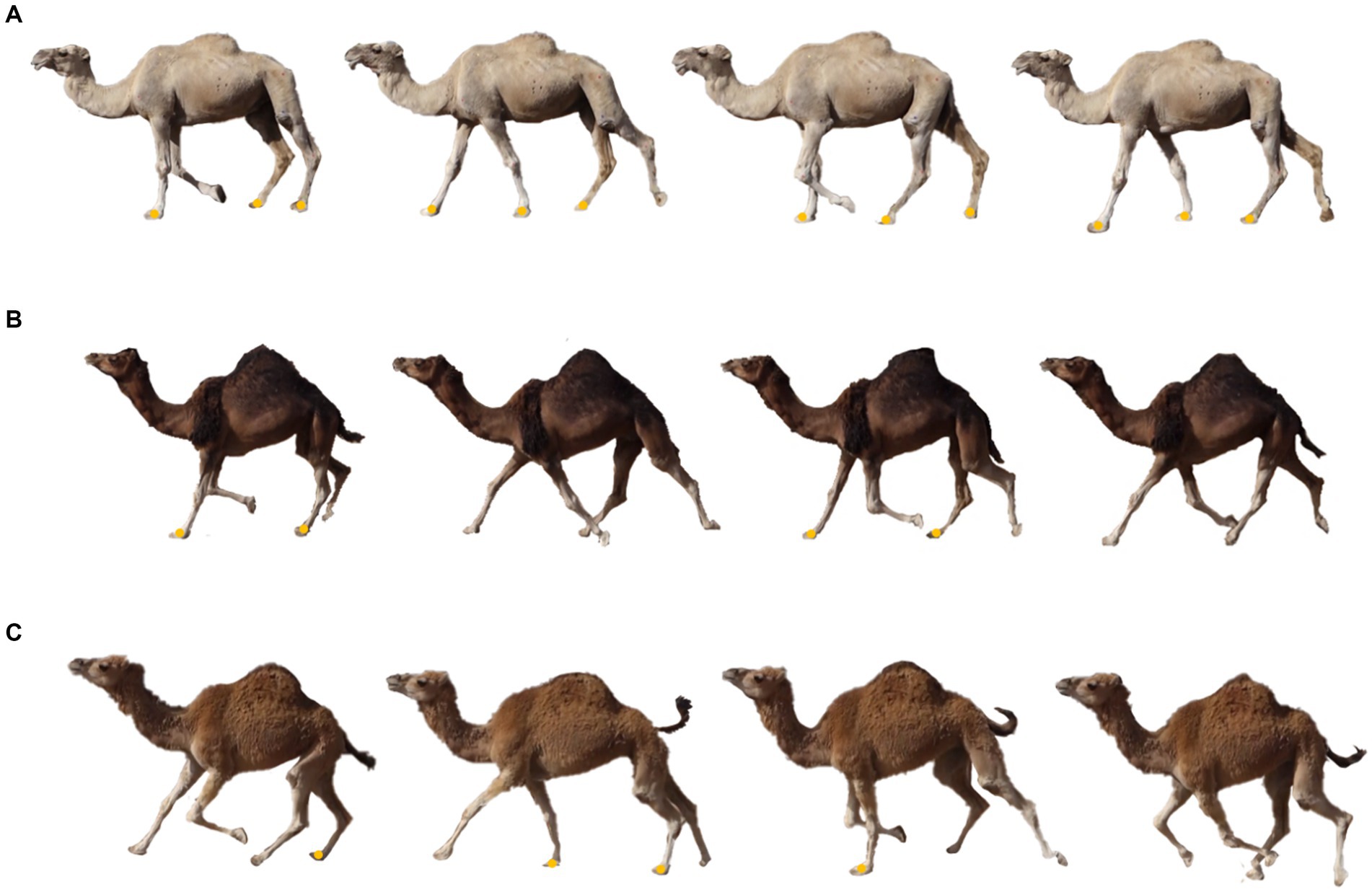
Figure 2. Graphical representation of the gait cycle during the walk (A), pace (B), and gallop (C) in dromedary camels. Yellow dots indicate which limbs are on the ground at each phase of the dromedary gait cycle.
The individual locomotion process is assessed using a qualitative linear scale (1 to 5 points) described by Navas González et al. (33). Gaits characterized by a lack of uniformity, which may be indicative of an absence of balance, cadence, or harmony, and poor limb development were attributed a score of 1. In contrast, animals receiving a score of 5 were observed to exhibit a harmonious, rhythmic, and seamlessly coordinated gait, with their bodily movements demonstrating a high degree of synchronicity.
Kinovea software (Kinovea version 0.9.5; Kinovea Org., France) was used to analyze video sequences and kinematic data extraction. With Kinovea, images are calibrated using coordinate scales from the pictures, and the collected data undergo leveling through three smoothing methods. Eleven kinematic variables were considered to characterize each of the gaits performed by the camels as a default. Biomechanics parameters were as follows: acceleration, horizontal acceleration/position/velocity, total distance, total horizontal/vertical displacement, velocity, and vertical acceleration/position/velocity. An experienced researcher digitized anatomical landmarks to define body segments, including the lower lip, ear, withers (third thoracic vertebra), and tail base (dock). Segments’ axes, such as the head, neck, and trunk, were established using these landmarks. Quantitative analysis was performed on trials where the head, neck, and trunk remained aligned in the sagittal plane. Various linear and angular variables related to the head movement, including displacement and velocity, were calculated using specialized software tools.
2.5 Definition of cubic regression equations and coefficients
Individual curve estimation regression analysis to identify the model that best describes camel biomechanics parameters (acceleration, horizontal acceleration/position/velocity, total distance, total horizontal/vertical displacement, velocity, and vertical acceleration/position/velocity) was performed using the Curve Estimation routine of the Regression package of SPSS Statistics for Windows, Version 25.0, IBM Corp. (2017). The Curve Estimation routine in SPSS software produces curve estimation regression statistics and related plots for 11 different curve estimation regression models, such as linear, quadratic, compound, growth, logarithmic, cubic, S, exponential, inverse, power, and logistic,. A generalized minimum adjusted R2 value of over 0.7 was required for the model to be determined valid to capture data variability. The mathematical model that best fitted (comparatively superior average values of individual R2; >0.7) the dynamics of locomotion of study animals was the cubic model. In these regards, parameters β0, β1, β2, and β3 of the curve for the variables of acceleration, horizontal acceleration/position/velocity, total distance, total horizontal/vertical displacement, velocity, and vertical acceleration/position/velocity, respectively, for each of the limbs were considered as independent variables in discriminant analysis.
In a cubic regression model, the equation takes the form:
Where:
β0: This is the intercept term and represents the predicted value of y when x is 0. However, the interpretation of this term might not be as straightforward in some cases, depending on the range of your data and the context of your problem.
β1: This coefficient represents the change in the predicted y for a one-unit change in the linear term x. It captures the linear relationship between x and y, just like in a simple linear regression model.
β2: This coefficient represents the effect of the squared x on the predicted y. It indicates whether there is a curvature in the relationship between x and y. A positive β2 suggests an upward curvature, while a negative β2 suggests a downward curvature.
β3: This coefficient represents the effect of the cubed x on the predicted y. It introduces another level of curvature to the relationship between x and y. A positive β3 suggests that the curvature continues to be upward, while a negative β3 suggests a downward curvature.
2.6 Statistical analysis
Following the methodology by González Ariza et al. (34), a discriminant canonical analysis was first used to develop a tool that enables the identification of the qualitative individual performance at locomotion (1 to 5 points; clustering criterion), determining whether linear combinations of biomechanics-related and biometrics variables, force and cubic regression coefficients, and categories for the variables “sex”, “coat color”, “coat particularities”, “eye color”, “neutering status”, “owner”, and “training regime” (actively or not actively involved in desensitization protocols) (explanatory variables) describe within- and between-population group clustering patterns. The discriminant canonical analysis is a statistical method that maximizes the discriminatory power of the variables, enhancing the accuracy of the results even with a relatively reduced number of observations (e.g., endangered livestock breeds). This technique has a notable ability to handle multicollinearity and identify the most influential variables that contribute to its accuracy, especially in situations where limited sample sizes may pose challenges for other statistical approaches.
The chi-squared automatic interaction detection (CHAID) decision tree method was used for classification, prediction, interpretation, and manipulation of the observation for the aforementioned independent or explanatory variables discretely categorized into the qualitative evaluation levels of gaits considered as the dependent variables of this analysis.
To assess the reliability of the CHAID decision tree, cross-validation is conducted to measure the predictive performance when applied to new data samples compared to the training sample. This assessment helps determine how well the model generalizes to unseen data. To validate the CHAID decision tree and evaluate whether the selected predictors effectively explain differences across the five qualitative evaluation levels of gaits, 10-fold cross-validation is utilized.
3 Results
3.1 Discriminant canonical analysis model reliability
After 325 rounds of multicollinearity analyses, the variables retained in the discriminant canonical analyses are those presented in Table 1.
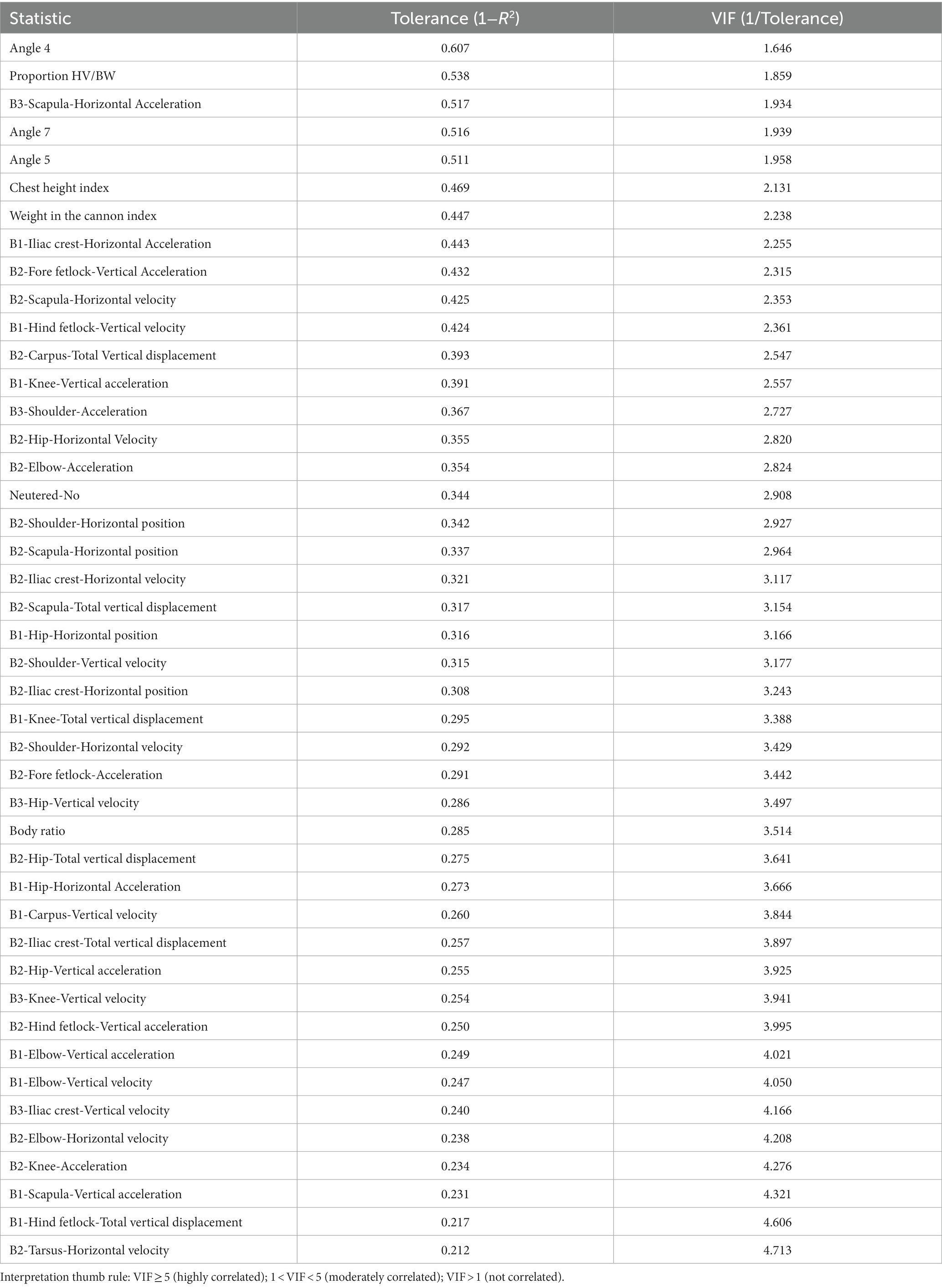
Table 1. Summary of the value of tolerance and VIF after multicollinearity analysis of biomechanical performance traits in Canarian camel breed.
As unbalanced samples and a considerable number of variables were used, the approximation of Rao for Wilks’ Lambda test overcomes the robustness of other tests, such as Pillai’s trace test statistic in the case of heterogeneous variance. Wilks’ Lambda statistic was highly statistically significant when Gait qualitative scale levels (Wilks’ Lambda statistic: 0.001; F (Observed value): 4.202; F (Critical value): 3.258; df1: 736; df2: 7; p = 0.025) were considered clustering criteria. Hence, the validity of the discriminant canonical analysis is ensured.
Five of the thirteen functions revealed after the discriminant analysis were reported to be significant for their discriminant ability (Table 2). The discriminatory power of the F1 function was the highest in comparison to that reported for the rest of the functions (eigenvalue of 6568.067; Figure 3), significantly explaining 89.72% of the variance. This framework makes F1 to F4 significantly explain 100% of the variability.
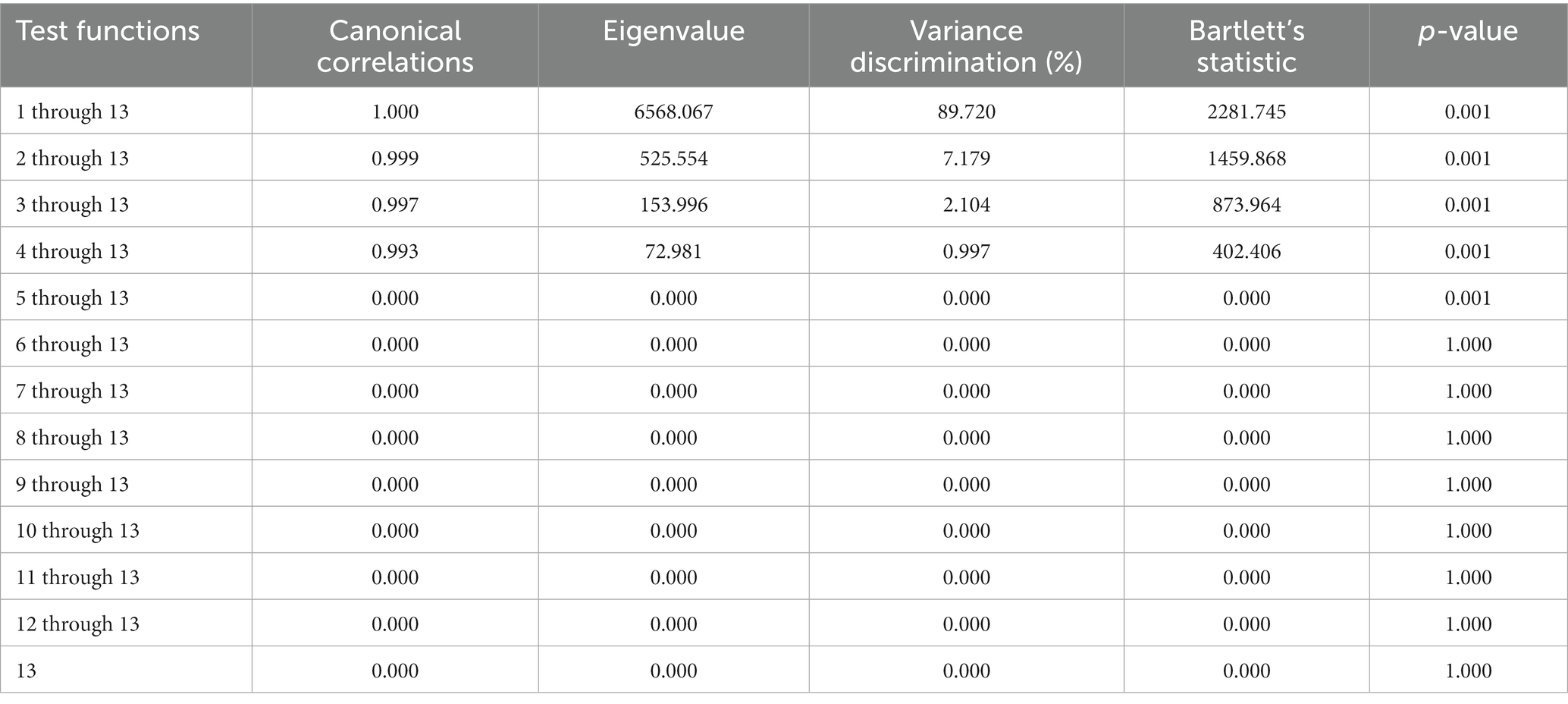
Table 2. Canonical discriminant analysis efficiency parameters to determine the significance of each canonical discriminant function.
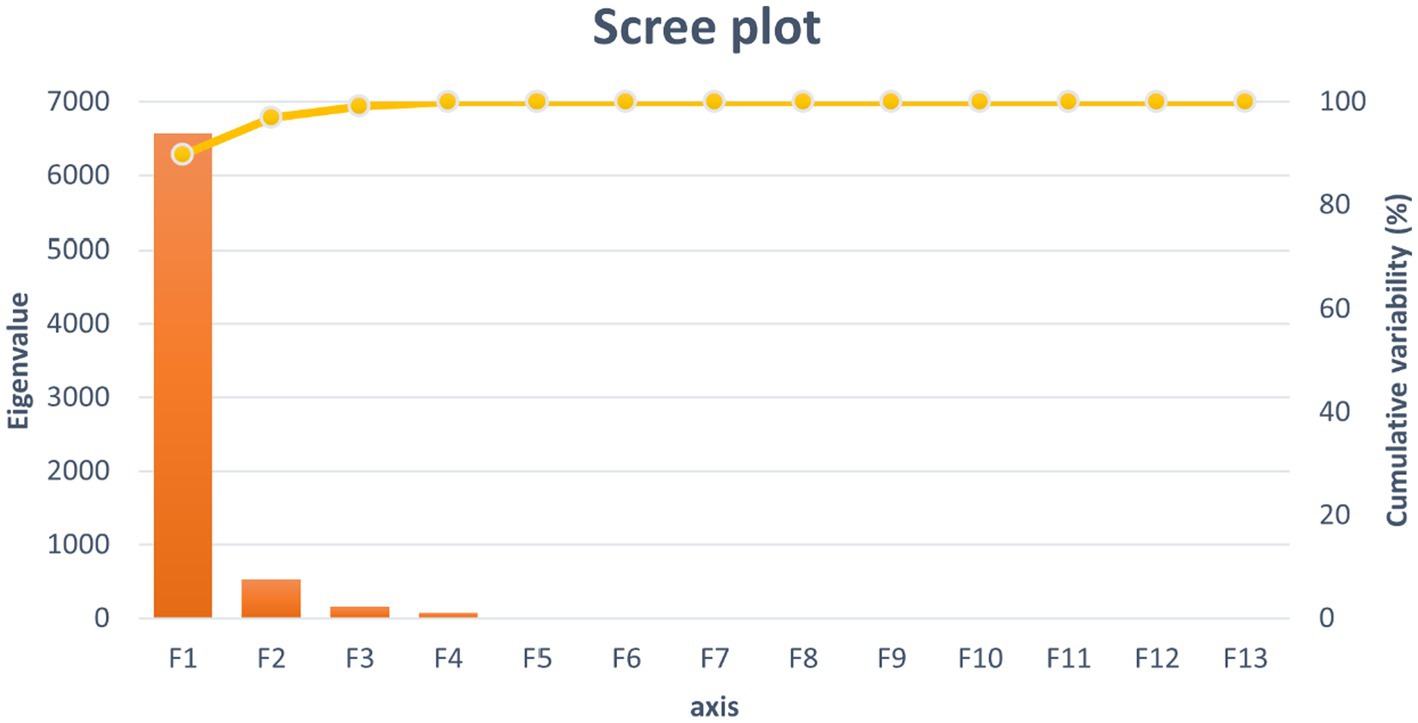
Figure 3. Canonical variable functions and their percentages of self-explained and cumulative variance.
3.2 Canonical coefficients and loading interpretation
The different variables studied in this research were ranked according to their discriminating ability. A test of equality of group means of the independent variables involved in the present discriminant canonical analysis was used, as shown in Table 3. Standardized discriminant coefficients measure the relative weight of each independent variable across the significant discriminant functions (Supplementary Table S1).
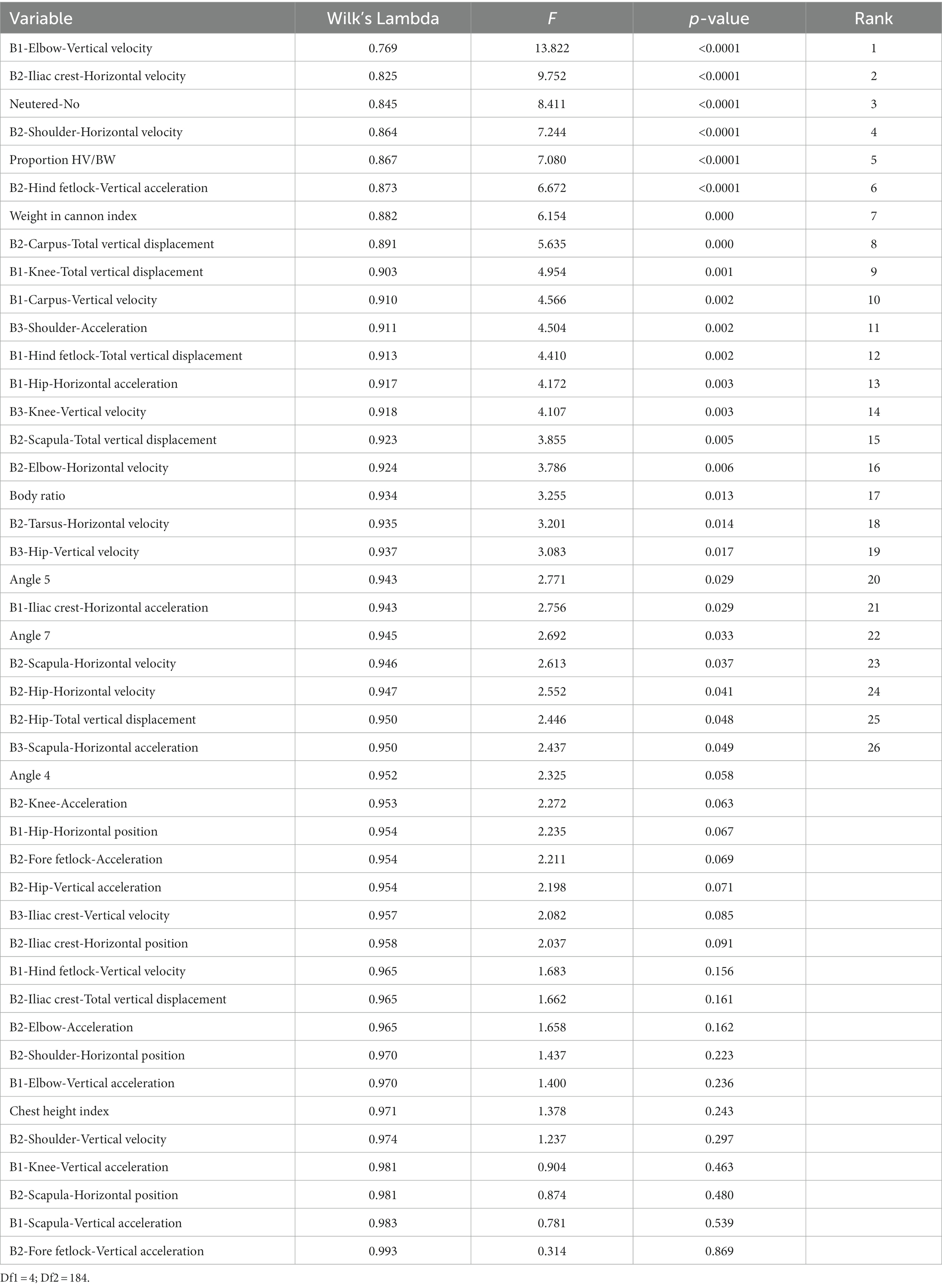
Table 3. Results for the tests of equality of group means to test for difference in the means across sample groups after the removal of redundant variables.
A Press’ Q value of 17.19 (n = 189; n’ = 15; K = 5) was computed for qualitative gait evaluation levels. Thus, predictions can be considered to be better than chance at 95% (35).
Centroids from different levels for qualitative description of gait performance considered in this study are calculated. The relative position of each centroid is determined by substituting the mean value for the observations depicted in each of the five discriminant functions significantly detected (F1 to F5). The results for the functions at the centroids are reported in Table 4.
3.3 Data mining CHAID decision tree
The CHAID decision tree is presented in Supplementary Figure S1.
4 Discussion
Following the premises of Maloiy et al. (24), who stated that locomotory performance is essentially modulated by heritable characters, in lesser proportion by training, for both camels and equines, the present study has investigated which biomechanical and animal morphometric, physiology, and phaneroptic-related variables influence gait performance in dromedary camels leisure riding and racing activities. The mathematical function that had the best fit to a series of data points obtained from the analysis of different gaits (walking, pacing, and galloping) in the studied dromedary camels was identified as a preliminary requirement to effectively study these potential associations. The mathematical model that best fitted (comparatively superior average values of individual R2; ≥0.7) the dynamics of camel locomotion was the cubic function, whose end behavior at the graphs is determined by the sign of their leading coefficients. These results are in accordance with previous research studies aimed at the mathematical and physical modeling of animal-like locomotion through the use of bioinspired robots (36–39), rigid body models (40), and video analysis (41).
The posterior examination of the statistical relationships between the coefficients of the individual curves of movement and other animal attributes and traits with gait performance made possible the identification of those variables that most largely influence this functional character. On the one hand, the kinematic parameters “vertical velocity”, “total vertical displacement”, “horizontal velocity”, “vertical acceleration”, “acceleration”, “horizontal position”, and “horizontal acceleration” appeared to exert a significant effect on the good performance of camel’s gaits when performing leisure riding and racing activities. In addition to the benefits of good speed and acceleration for covering a specific distance in the shortest time possible (42, 43), the “vertical displacement” and the “horizontal position” of certain body regions during locomotion play a fundamental role in the physical performance of these animals. The vertical displacement determines both the shape and magnitude of the impulse created (vertical jump) and weightlifting performance (44, 45). In regards to the horizontal position, this variable could be an indirect reliable measure of joint rotation and skeletal impairments, thus an indicator of stabilization, alignment, and muscular force of the different body areas implicated in locomotion (46, 47). Therefore, overall athletic performance in leisure riding and racing dromedary camels may be the result of the sum of joint powers and other different sports performance characteristics such as velocity, acceleration, jumping, and change of direction.
Such performance characteristics are known to be intrinsically modulated by linear and angular morphometric measurements, as well as animal physiology, in several vertebrate species (48–57). Specifically, based on our results, superior performance in camel gait is likely to be affected by the angle between the carpus and the fetlock on the forelimb (“angle 4”), the angle between the iliac crest and the greater trochanter of the femur (“angle 5”), the angle between the stifle joint and the tarsus (“angle 7”), the proportion hump volume/body weight (“HV/BW”), the weight in cannon index, the chest height index, the body ratio, and the neutering status of the animals.
Similar to other quadrupedal animals that are bred for athletic activities, such as horses and dogs, the skeletal structure and deviations of the vertical line of carpus/tarsus joint, either medially or laterally, cranially or caudally, would significantly predispose the camel to carpus/tarsus lameness and splints, thus negatively affecting the gait score (58–60). Additionally, several authors confirmed the prominent role of the fore and hind fetlock joint angles, stifle and hock joint angle, and pelvis inclination on weight absorption, energy of propulsion, and storage of elastic strain in riding horses (61–64). Closely related to pelvis inclination, camels are known to be the only animal species that bend the thigh when moving (65). In addition, chest development (“chest height index”) and tallness (“body ratio”) are reported to affect the static/dynamic balance and physical resistance in sport horses (66–69) and athletes (70).
Furthermore, if the hump of a camel is mechanically compared to the size and position of a rider on a horse, it could be inferred that the relative weight and dimensions of the hump (relation HV/BW) have a significant effect on camel locomotion given its influence on body balance, force distribution, and location of the center of gravity, analogous to the effects that the rider-horse-saddle aggregation has on equine gait (71). Moreover, the weight in the cannon index is expected to have a prominent effect on camel locomotion, as the study of the evolutionary history of these animal species has recently revealed. Through the analysis of the vertical ground reaction force with body mass in camelid species, Clemente et al. (72) found that camels have large foot contact areas due to broad fat pads. This condition would decrease the loading rate, thus leading to lower peak foot pressures and musculoskeletal stresses on the limbs. It also helps maintain proportional forces as animals grow in body mass or accelerate. These authors also encountered that dromedary camels load their forelimbs more than their hindlimbs, revealing the higher peak forces in forelimbs and, thus, their major functional relevance in this animal species’ locomotion. Hence, this adaptive trait may be correlated with the afore-discussed prominent role of the alignment of the distal part of the forelimbs and the fore fetlock joint angle on the weight absorption and minimization of mechanic impact during camel locomotion.
In conclusion, the correlation between physiology and function was revealed by the significant impact of the neutering status of the camels on gait performance. In fact, different reports have discussed the advantages of castration in dromedary camels. Some authors highlight the larger effect of castration on the body development in this species if carried out after the animals have reached sexual maturity (73, 74). Concretely, gelded camels are larger, more robust, and more enduring (75, 76). In addition, Iglesias Pastrana et al. (77) remarked an increased effect of neutering on camel organic development and behavior if the animals are castrated not only when they have reached their sexual maturity but have also been initiated in the training protocol for their functionality (e.g., leisure riding). Hence, apart from the benefit of body growth and endurance, castration also has effects on camel behavior, which ultimately constitutes a further criterion of notable interest for functional selection in working animals.
However, other morphometric, phaneroptic, and performance-type variables such as the angle between the cranial side of the scapula and the shoulder joint (“angle 1”), the angle between the shoulder joint and the olecranon (“angle 2”), the angle between the olecranon and the carpus (“angle 3”), the angle between the greater trochanter of the femur and the stifle joint (“angle 6”), the angle between the point of the hock (tarsus) and fetlock (metatarsophalangeal joint) on the hindlimb (“angle 8”), body weight, hump volume, hump weight, density of fat, proportion hump weight/body weight, chest index, cephalic index, pelvic index, dactilothoracic index, conformation index, compactness index, pull force, load force, power, sex, age, coat color and particularities, eye color, owner, training regime, and type of gait did not have a significant discriminatory effect on camel gait performance in leisure riding and racing activities.
First, the aforementioned singular function of fore fetlock joint angles, stifle and hock joint angle, and pelvis inclination on weight absorption, energy of propulsion, and storage of elastic strain in riding animals could be explaining the lack of large impact of the remaining angular measurements evaluated for its potential implication in camel locomotion in the present study. Concerning the effects of body weight, hump volume and weight, and the proportional relationship between different body regions in camel gait performance, the absence of significant affectation of this functional trait by the mentioned factors partially resembles the results of de Oliveira Bussiman et al. (78) for Brazilian horses. However, this contrasts with the results reported by Pratt-Phillips and Munjizun (79) and Gómez et al. (80). These authors support the effects of body mass index, body composition profile, and harmony of physical development on the exercise performance of sport horses, probably due to a combined effect between inflammation-type responses and the impacts of excessive weight carriage on limb health. Then, the contrasting results obtained for camels can be ascribed to different species-specific adaptive traits.
Fatty tissue in camels is mostly concentrated on the hump to improve heat removal through the skin, and the hump size is related to the general reserve status (body condition score) of the animals (81). For this reason, the hump volume in relation to the body weight, and neither the volume and weight of the hump nor the body weight considered separately, significantly influences the locomotion of these animals. Such a condition derives from the implication of the proportionality between the hump volume and the body weight for maintaining the static/dynamic balance of the body of the camel. In addition, in the case of hump weight, this variable greatly depends on the specific fatty acid composition (82), so a heavy hump does not necessarily mean a large volume hump. Apart from that, body balance is strongly controlled by the aforementioned functionality of the broad foot pads, which are responsible for solving the differential impact of the animal’s weight and the speed of movement on the locomotor pressures that affect the musculoskeletal system forces. Finally, in the case of the variables that relate different measurements corresponding to the dimensions of the trunk and extremities, their non-significant influence could also be explained by purely evolutionary reasons. Camels are provided with relatively narrow bodies, long legs, no flank fold that makes the movement of hindlimbs difficult, a less rounded abdomen to allow the hindlimbs freer motion, and limbs more closely to the midline to minimize oscillation between strides, as a result of natural selection for energy efficiency of the movement (9, 83). In close relationship with this last statement, from a pure evolutionary physiology background, pull force, load force, and power may have had a non-significant effect on camel gait performance.
Within the same phylogenetic background, sex, age, coat color and particularities, eye color, owner, training regime, and type of gait did not influence in a significant manner the physical performance of camels. When walking, camels move their ipsilateral limbs in unison (“pacing gait”, as referred to in the literature). Tracing back camel evolutive history, pacing gait is agreed to have been developed about 20 million years ago (84). Apart from the fact that the legs of camels cannot collide at this pacing gait, the stride length, speed, and kinematics efficiency are higher when compared to other gaits, such as regular trots. However, pacing gait made the animal more prone to lateral instability. This way, camels have developed a broad foot pad and a digitigrade stance to abate such relative lack of instability (18, 65). Contrary to horses, camels are known to be the only ungulate species that always use this pattern of leg movements independent of speed (18). Moreover, this is a highly conserved ancestral trait (85). Hence, the underlying genetic basis of camel gaits, which is elementally implicated in the sort of neural impulses from the spine to the extremities (86) and presumably free of epistatic interactions from genes that control coat and eye color associated traits, may be extrapolated to the whole range of physical activities that are feasible for camels to perform. This last hypothesis could be confirmed in future approaches aimed at studying which genomic variants are statistically associated with an enhanced or decreased physical performance.
Then, these statements are contrary to the results presented by Al-Shorepy (14) for racing camels, Entin (87) for racehorses and Greyhound dogs, Vicente et al. (88) for classical riding horses, and Navas González et al. (33) for assisted-therapy donkeys, who reported a significant effect of sex and age on animal functional performance but agreed with Senefeld et al. (89), who support the idea that large differences in athletic performance do not exist between sexes in animals. Regarding the association between coat and eye color with different psychological constructs that are transcendental for animal trainability and safe interaction, the molecular basis of these desirable traits has been identified mainly for dogs and horses (90–93), and some inferences are discussed by Iglesias Pastrana et al. (77) for dromedary camels. Furthermore, a non-negligible impact of rider fitness and interaction with the animal (94), training regime (95, 96), and type of gait (97) on exercise performance are also recognized in the literature on sport horses and dogs. Such controversy could definitively be ascribed to methodological issues (e.g., type and number of potential influencing factors considered, and statistical treatment of data), as well as to animal species and breed-mediated effects.
In summary, rather than the morphology of a specific region of the body, which is correlated with the weight of the animal (98), or factors such as sex, age, and phaneroptic-related variables, known to affect athletic performance in some animal species, a reduced number of angular morphometric measurements, definite body proportions that can affect the general balance of the body, the weight supported by structures with notable involvement in the damping of the mechanical forces produced during locomotion, post-neutering effects, and the particular mobility of specific joints are the factors that should be considered when selecting leisure riding and racing dromedary camels for gait proficiency. More specifically, based on the data mining decision tree, the variables that serve to discriminate with greater accuracy between leisure riding and racing camels for gait performance are the neutering status, with the advantage for neutering, and the kinematic behavior of the scapula, shoulder, carpus, hip, and foot, anatomical structures of singular importance for propulsion, movement direction, and limb support in quadrupeds, as cited above.
With special emphasis on kinematics, the horizontal position of the scapula, the total vertical displacement of the carpus, the horizontal velocity of the shoulder, and the horizontal acceleration of the hip in neutered animals, the horizontal and vertical acceleration of the scapula, and the total vertical displacement of foot in non-neutered animals are specific discriminating parameters among good and poor performance camels. It can also be observed that coefficient 2 is more relevant in castrated animals, while coefficients 1 and 3 are more discriminative among non-castrated animals. Based on the graphic behavior of the cubic function, coefficients 1 and 3 for curves of movement could be related to the direction and force of the movement, while coefficient 2 would be with the amplitude of the movement, suspension duration, and speed (99). From the viewpoint of athletic performance, these results may be indicative of better dressage/beauty and endurance riding performance for intact and castrated animals, respectively. In any case, the selection of the animals should be complemented by the use of behavior evaluation tools. Indeed, the common practice of castration in the camel breed studied in the present research consists of the neutering of the animals once they are initiated in the domestication protocol for leisure riding activities and are sexually mature. It is pursued that animals have reached proper general organic maturity by the mediation of plasma androgens (mainly within brain structures) while also avoiding harmful levels of these hormones from an ethological perspective (77, 100).
With regard to the quantitative evaluation of kinematic parameters, better gait performance in leisure riding and racing camels is achieved when the horizontal position of the scapula is greater than 0, the total vertical displacement of the carpus is greater than −3.78, the horizontal velocity of the shoulder is less than or equal to 2.25, and the horizontal acceleration of the hip is greater than 5.74, for neutered animals. For non-neutered animals, optimal gait performance is observed when the acceleration of the scapula is less than or equal to 0 as well as the total vertical displacement of the foot greater than −2.24. Elementally, the total vertical displacement of the carpus, as an anatomical structure significantly involved in forelimb mechanical support and cushioning, would act as a corrector for the horizontal position of the scapula as well as the horizontal velocity of the shoulder, thus achieving greater general stability, harmony, and energy efficiency of the movement (101). In the case of the horizontal acceleration of the hip, the higher its value, the greater the propulsion force and amplitude of the movement. Contrastingly, for intact camels, a lower acceleration of the scapula and a greater vertical displacement of the distal region of the hindlimbs determine a more harmonious and efficient movement. These values, being interpreted on account of the relative significance of coefficients 1 and 3 for this animal subgroup, reflect the relevance of the rear quarters in maintaining the dynamic balance of the body while limiting as far as possible a wide acceleration at the anterior third, in animals presumably more apt for riding/dressage activities. These functionalities make rear quarters broad, deep, and heavy to support load carriage while moving in an efficient way (102).
Concerning the exploration of new potential functional niches, the results of the present study can assist the tasks of camel valorization and selection for their active participation in animal-assisted therapy since those biomechanical and physiological traits of the animals that most influence locomotor performance, regardless of the type of gait, are identified. On the contrary, for equines, since the cadence of movements can vary between gaits, it may be necessary to look at different and varied selection criteria depending on the type of gait involved in each of the assisted activities in which these animals would be involved. Furthermore, camels are less flighty than horses (103), and the pelvic movement of a person when riding a camel is reported to be very similar to natural human pelvic movements during walking (104); thus, therapeutic applications of camelback riding apart from mere leisure are clearly plausible.
Ultimately, some limitations can be identified in this study. Specifically, future applied studies are encouraged to correlate biomechanical traits with physiological indicators, such as activity-dependent hematological and biochemical parameters, to enhance dromedary camel breeding programs for physically demanding activities not only from a genetic improvement perspective but also to ensure the maintenance of optimal welfare status of the animals.
5 Conclusion
Individual curve estimation regression analysis plays a pivotal role in synthesizing complex datasets by constructing validated mathematical descriptions of locomotive behavior in camels. The posterior study of the relationships among morphology, physiology, phaneroptic, and gait performance-related traits made possible the identification of those factors that largely influence gait proficiency in dromedary camels; thus, these criteria should assist breeding decisions for camels dedicated to different athletic activities. Angularity and mechanical forces at distal fore and rear extremity areas, inclination of the pelvis, specific proportionalities affecting the general balance of the body, neutering-mediated effects, and the velocity, acceleration, position, and displacement of particular body regions significantly affect the overall athletic performance in dromedary camels. More specifically, the kinematic behavior of the scapula, shoulder, carpus, hip, and foot, as these anatomical structures primarily control the take-off of the rear limbs and the impact forces of the front limbs, has a prominent impact on the locomotor performance in dromedary camels. Other animal and environment-dependent variables do not influence camel gait performance, probably due to the mixed effect of different species-specific adaptive traits inherited in response to desert environment demands, such as the pacing gait, broad foot pads, and energy efficiency of the movement.
Data availability statement
The original contributions presented in the study are included in the article/Supplementary material, further inquiries can be directed to the corresponding author.
Ethics statement
All farms included in the study followed specific codes of good practices and therefore, the animals received humane care in compliance with the national guide for the care and use of laboratory and farm animals in research. Written consent from the owners was obtained for their participation. The study was conducted in accordance with the Declaration of Helsinki. The Spanish Ministry of Economy and Competitivity through the Royal Decree Law 53/2013 and its credited entity, the Ethics Committee of Animal Experimentation from the University of Córdoba, permitted the application of the protocols present in this study as cited in the 5th section of its 2nd article, as the animals assessed were used for credited zootechnical use. This national Decree follows the European Union Directive 2010/63/UE, from the 22 September of 2010.
Author contributions
CI: Conceptualization, Data curation, Formal analysis, Investigation, Methodology, Software, Validation, Writing – original draft, Writing – review & editing. FN: Conceptualization, Data curation, Formal analysis, Investigation, Methodology, Resources, Software, Supervision, Validation, Visualization, Writing – original draft, Writing – review & editing. EC: Funding acquisition, Investigation, Project administration, Resources, Supervision, Validation, Visualization, Writing – review & editing. CM: Data curation, Formal analysis, Funding acquisition, Resources, Software, Validation, Visualization, Writing – review & editing. JD: Formal analysis, Funding acquisition, Project administration, Resources, Supervision, Validation, Visualization, Writing – review & editing.
Funding
The author(s) declare financial support was received for the research, authorship, and/or publication of this article. The present research was carried out in the financing framework of the international project CA.RA.VA.N – “Toward a Camel Transnational Value Chain” (Reference APCIN-2016-00011-00-00) and during the covering period of a predoctoral contract (FPU Fellowship) funded by the Spanish Ministry of Science and Innovation and a Ramón y Cajal Post-Doctoral Contract with the reference MCIN/AEI/10.13039/501100011033 and the European Union “NextGenerationEU”/PRTR.
Acknowledgments
The authors would also like to thank “Aires Africanos” Eco-tourism Company, Oasis Park Fuerteventura, and “Camelus” Camellos de Almería for their direct technical help and assistance.
Conflict of interest
The authors declare that the research was conducted in the absence of any commercial or financial relationships that could be construed as a potential conflict of interest.
The author(s) declared that they were an editorial board member of Frontiers, at the time of submission. This had no impact on the peer review process and the final decision.
Publisher’s note
All claims expressed in this article are solely those of the authors and do not necessarily represent those of their affiliated organizations, or those of the publisher, the editors and the reviewers. Any product that may be evaluated in this article, or claim that may be made by its manufacturer, is not guaranteed or endorsed by the publisher.
Supplementary material
The Supplementary material for this article can be found online at: https://www.frontiersin.org/articles/10.3389/fvets.2023.1297430/full#supplementary-material
References
1. Moreno-Garcia, M, Pimenta, CM, López Aldana, PM, and Pajuelo Pando, A. The signature of a blacksmith on a dromedary bone from Islamic Seville (Spain). Archaeofauna. (2007) 16:193–202.
2. de Sierra, GF, and Fabelo Marrero, FJ. El camello canario. Lanzarote, Canary Islands, Spain: Asociación de Criadores del Camello Canario (2017).
4. Iglesias, C, Navas, FJ, Ciani, E, Arando, A, González, A, Marín, C, et al. Zoometric characterization and body condition score in Canarian camel breed. Arch zootec. (2020) 69:14–21. doi: 10.21071/az.v69i265.5034
5. Nelson, K, Bwala, D, and Nuhu, E. The dromedary camel; a review on the aspects of history, physical description, adaptations, behavior/lifecycle, diet, reproduction, uses, genetics and diseases. Niger Vet J. (2015) 36:1299–317.
6. Iglesias Pastrana, C, Navas González, FJ, Ciani, E, Nogales Baena, S, and Delgado Bermejo, JV. Camel genetic resources conservation through tourism: a key sociocultural approach of camelback leisure riding. Animals. (2020) 10:1703. doi: 10.3390/ani10091703
7. Mackay, TF. Epistasis and quantitative traits: using model organisms to study gene–gene interactions. Nat Rev Genet. (2014) 15:22–33. doi: 10.1038/nrg3627
8. Caicedo, AL, Stinchcombe, JR, Olsen, KM, Schmitt, J, and Purugganan, MD. Epistatic interaction between Arabidopsis FRI and FLC flowering time genes generates a latitudinal cline in a life history trait. Proc Natl Acad Sci. (2004) 101:15670–5. doi: 10.1073/pnas.0406232101
10. Abdo, M, Haddad, SS, Aziz, EK, Abdeen, A, and Sabek, A. Kinematics biomechanical analysis and three dimensional reconstruction diagnostic technique of carpal joint during gait in one-humped camel (Camelus dromedarius). Alex J Vet Sci. (2019) 60:23. doi: 10.5455/ajvs.30333
11. Dagg, AI. The locomotion of the camel (Camelus dromedarius). J Zool. (1974) 174:67–78. doi: 10.1111/j.1469-7998.1974.tb03144.x
12. Pfau, T, Parsons, K, and Wilson, A. Mechanics of over-ground locomotion in the dromedary camel (Camelus dromedarius). J Biomech. (2006) 39:S359. doi: 10.1016/S0021-9290(06)84436-2
13. Alexander, RM, Maloiy, G, Ker, R, Jayes, A, and Warui, C. The role of tendon elasticity in the locomotion of the camel (Camelus dromedarius). J Zool. (1982) 198:293–313. doi: 10.1111/j.1469-7998.1982.tb02077.x
14. Al-Shorepy, SS. Identification of environmental factors affecting the racingperformance of race camels in the United Arab Emirates. Emir J Food Agric. (2011) 23:424–30.
15. Alsafy, MA, El-Gendy, SA, and Kamal, B. Computed tomographic and radiographic morphology of the pastern and coffin joints of one-humped camel (Camelus dromedarius). Anat Histol Embryol. (2021) 50:108–13. doi: 10.1111/ahe.12607
16. Badawy, AM. Computed tomographic anatomy of the fore foot in one-humped camel (Camelus dromedrus). Glob Vet. (2011) 6:417–23.
17. El-Shafey, A, and Kassab, A. Computed tomography and cross-sectional anatomy of the metatarsus and digits of the one-humped camel (C amelus dromedarius) and Buffalo (B os bubalis). Anat Histol Embryol. (2013) 42:130–7. doi: 10.1111/j.1439-0264.2012.01174.x
18. Janis, CM, Theodor, JM, and Boisvert, B. Locomotor evolution in camels revisited: a quantitative analysis of pedal anatomy and the acquisition of the pacing gait. J Vertebr Paleontol. (2002) 22:110–21. doi: 10.1671/0272-4634(2002)022[0110:LEICRA]2.0.CO;2
19. Tharwat, M, Al-Sobayil, F, and Buczinski, S. Effect of racing on the serum concentrations of cardiac troponin I and creatine kinase myocardial band in racing camels (Camelus dromedarius). Vet Res Commun. (2013) 37:139–44. doi: 10.1007/s11259-013-9556-z
20. Park, J, Seeley, MK, Francom, D, Reese, CS, and Hopkins, JT. Functional vs. traditional analysis in biomechanical gait data: an alternative statistical approach. J Hum Kinet. (2017) 60:39–49. doi: 10.1515/hukin-2017-0114
21. Saastamoinen, MT, and Barrey, E. Genetics of conformation, locomotion and physiological traits In: AT Bowling and A Ruvinsky, editors. The genetics of the horse : CAB International Wallingford UK (2000). 439–72.
22. Sánchez, M, Gómez, M, Molina, A, and Valera, M. Genetic analyses for linear conformation traits in Pura Raza Español horses. Livest Sci. (2013) 157:57–64. doi: 10.1016/j.livsci.2013.07.010
23. Alexander, RM. Energy-saving mechanisms in walking and running. J Exp Biol. (1991) 160:55–69. doi: 10.1242/jeb.160.1.55
24. Maloiy, G, Rugangazi, B, and Rowe, M. Energy expenditure during level locomotion in large desert ungulates: the one-humped camel and the domestic donkey. J Zool. (2009) 277:248–55. doi: 10.1111/j.1469-7998.2008.00535.x
25. Boujenane, I. Comparison of body weight estimation equations for camels (Camelus dromedarius). Trop Anim Health Prod. (2019) 51:1003–7. doi: 10.1007/s11250-018-1771-8
26. Delgado, J, Navas, F, Miranda, J, Miró, M, Arando, A, and Pizarro, M. Preliminary body weight estimation methodology and its applicaction to the Andalusian donkey breed as an energetic producer. Actas Iberoam Conserv Anim. (2014) 4:207–9.
27. Bengoumi, M, Faulconnier, Y, Tabarani, A, Sghiri, A, Faye, B, and Chilliard, Y. Effects of feeding level on body weight, hump size, lipid content and adipocyte volume in the dromedary camel. Anim Res. (2005) 54:383–93. doi: 10.1051/animres:2005029
28. Susana Lopes, M, Azevedo, AR, Mendonça, D, Rojer, H, Cabral, V, Ceraolo, F, et al. Morphological and genetic characterization of the Graciosa donkey breed. J Appl Anim Res. (2023) 51:166–73. doi: 10.1080/09712119.2023.2171421
29. Dunbar, DC, Macpherson, JM, Simmons, RW, and Zarcades, A. Stabilization and mobility of the head, neck and trunk in horses during overground locomotion: comparisons with humans and other primates. J Exp Biol. (2008) 211:3889–907. doi: 10.1242/jeb.020578
30. Iglesias, C, Navas, FJ, Ciani, E, Arando, A, González, A, Marín, C, et al. Biokinematics and applied thermography in the Canarian camel breed. Arch zootec. (2020) 69:102–7. doi: 10.21071/az.v69i265.5045
32. Hildebrand, M. The adaptive significance of tetrapod gait selection. Am Zool. (1980) 20:255–67. doi: 10.1093/icb/20.1.255
33. Navas González, FJ, Jordana Vidal, J, León Jurado, JM, McLean, AK, Pizarro Inostroza, G, and Delgado Bermejo, JV. Genetic parameter estimation and implementation of the genetic evaluation for gaits in a breeding program for assisted-therapy in donkeys. Vet Res Commun. (2018) 42:101–10. doi: 10.1007/s11259-018-9712-6
34. González Ariza, A, Arando Arbulu, A, Navas González, FJ, León Jurado, JM, Delgado Bermejo, JV, and Camacho Vallejo, ME. Data mining-based discriminant analysis as a tool for the study of egg quality in native hen breeds. Sci Rep. (2022) 12:15873. doi: 10.1038/s41598-022-20111-z
36. Bayraktaroglu, ZY, Butel, F, Pasqui, V, and Blazevic, P. Snake-like locomotion: integration of geometry and kineto-statics. Adv Robot. (2000) 14:447–58. doi: 10.1163/156855300741942
37. Ramananarivo, S, Godoy-Diana, R, and Thiria, B. Rather than resonance, flapping wing flyers may play on aerodynamics to improve performance. Proc Natl Acad Sci. (2011) 108:5964–9. doi: 10.1073/pnas.1017910108
38. Tang, C., Ma, S., Li, B., and Wang, Y. (2010). A cubic CPG model for snake-like robot to adapt to environment. Paper presented at the the 2010 IEEE international conference on information and automation.
39. Zheng, L., Bi, S., Cai, Y., and Niu, C. (2010). Design and optimization of a robotic fish mimicking cow-nosed ray. Paper presented at the 2010. Tianjin, China: IEEE International Conference on Robotics and Biomimetics. 1075–1080. doi: 10.1109/ROBIO.2010.5723477
40. Van Den Bogert, A, Schamhardt, H, and Crowe, A. Simulation of quadrupedal locomotion using a rigid body model. J Biomech. (1989) 22:33–41. doi: 10.1016/0021-9290(89)90182-6
41. Kinugasa, R, and Usami, Y. How fast can a human run?− bipedal vs. quadrupedal running. Front Bioeng Biotechnol. (2016) 4:56. doi: 10.3389/fbioe.2016.00056
42. Barrey, E. Biomechanics of locomotion in the athletic horse. Equine Sports Med Surg Basic Clin Sci Equine Athl. (2013):189–211.
43. Clayton, HM, and van Weeren, PR. Performance in equestrian sports. Equine Locomot. (2001):193–226.
44. Suchomel, TJ, Nimphius, S, and Stone, MH. The importance of muscular strength in athletic performance. Sports Med. (2016) 46:1419–49. doi: 10.1007/s40279-016-0486-0
45. Whitmer, TD, Fry, AC, Forsythe, CM, Andre, MJ, Lane, MT, Hudy, A, et al. Accuracy of a vertical jump contact mat for determining jump height and flight time. J Strength Cond Res. (2015) 29:877–81. doi: 10.1519/JSC.0000000000000542
46. Hrysomallis, C. Balance ability and athletic performance. Sports Med. (2011) 41:221–32. doi: 10.2165/11538560-000000000-00000
47. Laudner, KG, Sipes, RC, and Wilson, JT. The acute effects of sleeper stretches on shoulder range of motion. J Athl Train. (2008) 43:359–63. doi: 10.4085/1062-6050-43.4.359
49. Hans, EC, Zwarthoed, B, Seliski, J, Nemke, B, and Muir, P. Variance associated with subject velocity and trial repetition during force platform gait analysis in a heterogeneous population of clinically normal dogs. Vet J. (2014) 202:498–502. doi: 10.1016/j.tvjl.2014.09.022
50. Herrel, A, Gonwouo, L, Fokam, E, Ngundu, W, and Bonneaud, C. Intersexual differences in body shape and locomotor performance in the aquatic frog, X enopus tropicalis. J Zool. (2012) 287:311–6. doi: 10.1111/j.1469-7998.2012.00919.x
51. Ibáñez, B, and Tambussi, CP. Foot-propelled aquatic birds: pelvic morphology and locomotor performance. Ital J Zool. (2012) 79:356–62. doi: 10.1080/11250003.2011.650713
52. Jackson, BE, Segre, P, and Dial, KP. Precocial development of locomotor performance in a ground-dwelling bird (Alectoris chukar): negotiating a three-dimensional terrestrial environment. Proc R Soc B Biol Sci. (2009) 276:3457–66. doi: 10.1098/rspb.2009.0794
53. Kristjansson, T, Bjornsdottir, S, Albertsdóttir, E, Sigurdsson, A, Pourcelot, P, Crevier-Denoix, N, et al. Association of conformation and riding ability in Icelandic horses. Livest Sci. (2016) 189:91–101. doi: 10.1016/j.livsci.2016.05.010
54. Ojanguren, A, and Brana, F. Effects of size and morphology on swimming performance in juvenile brown trout (Salmo trutta L.). Ecol Freshw Fish. (2003) 12:241–6. doi: 10.1046/j.1600-0633.2003.00016.x
55. Stefánsdóttir, G, Jansson, A, Ragnarsson, S, and Gunnarsson, V. Speed of gaits in Icelandic horses and relationships to sex, age, conformation measurements and subjective judges’ scores. Comp Exerc Physiol. (2021) 17:151–60. doi: 10.3920/CEP200039
56. Toro, E, Herrel, A, Vanhooydonck, B, and Irschick, DJ. A biomechanical analysis of intra-and interspecific scaling of jumping and morphology in Caribbean Anolis lizards. J Exp Biol. (2003) 206:2641–52. doi: 10.1242/jeb.00473
57. Voss, K, Wiestner, T, Galeandro, L, Hässig, M, and Montavon, P. Effect of dog breed and body conformation on vertical ground reaction forces, impulses, and stance times. Vet Comp Orthop Traumatol. (2011) 24:106–12. doi: 10.3415/VCOT-10-06-0098
58. Gillette, RL, and Angle, TC. Canine locomotion analysis. Darryl Millis and David Levine. Canine Rehabil Phys Ther. (2014):201–10. doi: 10.1016/B978-1-4377-0309-2.00011-9
59. Mostafa, MB, and Elemmawy, YM. Relationships between morphometric measurements and musculoskeletal disorders in jumping thoroughbred horses. J Equine Sci. (2020) 31:23–7. doi: 10.1294/jes.31.23
60. Senna, NA, Mostafa, MB, Abu-Seida, AM, and Elemmawy, YM. Evaluation of limb conformation in jumping thoroughbred horses. Asian J Anim Sci. (2015) 9:208–16. doi: 10.3923/ajas.2015.208.216
61. Cano, M, Vivo, J, Miró, F, Morales, J, and Galisteo, A. Kinematic characteristics of Andalusian, Arabian and Anglo-Arabian horses: a comparative study. Res Vet Sci. (2001) 71:147–53. doi: 10.1053/rvsc.2001.0504
62. Clayton, H, Hoyt, D, Wickler, S, Cogger, E, and Lanovaz, J. Hindlimb net joint energies during swing phase as a function of trotting velocity. Equine Vet J. (2002) 34:363–7. doi: 10.1111/j.2042-3306.2002.tb05449.x
63. Hobbs, SJ, and Clayton, HM. The Olympic motto through the lens of equestrian sports. Anim Front. (2022) 12:45–53. doi: 10.1093/af/vfac025
64. Holmström, M, Fredricson, I, and Drevemo, S. Biokinematic analysis of the Swedish warmblood riding horse at trot. Equine Vet J. (1994) 26:235–40. doi: 10.1111/j.2042-3306.1994.tb04376.x
65. Villalobos, C. M. (2019). Simbolismo y astrología en los textos antiguos: el caso del camello. Paper presented at the Studia classica et emblematica caro magistro Francisco J. Talauera Esteso dicata.
66. Cervantes, I, Baumung, R, Molina, A, Druml, T, Gutiérrez, J, Sölkner, J, et al. Size and shape analysis of morphofunctional traits in the Spanish Arab horse. Livest Sci. (2009) 125:43–9. doi: 10.1016/j.livsci.2009.03.006
67. do Pantanal, C, Biotecnologia, B-D, Margaret, C, Santos, SA, da Silva, JA, Louvandini, H, et al. Body indices for the Pantaneiro horse. Braz J Vet Res Anim Sci. (2008) 45:362–70.
68. Sánchez, MJ, Gómez, MD, Peña, F, Monterde, JG, Morales, JL, Molina, A, et al. Relationship between conformation traits and gait characteristics in Pura Raza Español horses. Arch Tierz. (2013) 56:137–48. doi: 10.7482/0003-9438-56-013
69. Signer-Hasler, H, Flury, C, Haase, B, Burger, D, Simianer, H, Leeb, T, et al. A genome-wide association study reveals loci influencing height and other conformation traits in horses. PLoS One. (2012) 7:e37282. doi: 10.1371/journal.pone.0037282
70. Granacher, U, Gollhofer, A, Hortobágyi, T, Kressig, RW, and Muehlbauer, T. The importance of trunk muscle strength for balance, functional performance, and fall prevention in seniors: a systematic review. Sports Med. (2013) 43:627–41. doi: 10.1007/s40279-013-0041-1
71. Dyson, S, Ellis, A, Mackechnie-Guire, R, Douglas, J, Bondi, A, and Harris, P. The influence of rider: horse bodyweight ratio and rider-horse-saddle fit on equine gait and behaviour: a pilot study. Equine Vet Educ. (2020) 32:527–39. doi: 10.1111/eve.13085
72. Clemente, CJ, Dick, TJ, Glen, CL, and Panagiotopoulou, O. Biomechanical insights into the role of foot pads during locomotion in camelid species. Sci Rep. (2020) 10:3856. doi: 10.1038/s41598-020-60795-9
74. Payne, WJA, and Wilson, RT. An introduction to animal husbandry in the tropics. Hoboken, New Jersey, United States: Blackwell Science (1999).
75. Pigière, F, and Henrotay, D. Camels in the northern provinces of the Roman empire. J Archaeol Sci. (2012) 39:1531–9. doi: 10.1016/j.jas.2011.11.014
76. Ucko, PJ, and Dimbleby, GW. The domestication and exploitation of plants and animals. Piscataway, New Jersey, United States: Transaction Publishers (2007).
77. Iglesias Pastrana, C, Navas González, FJ, Ciani, E, Arando Arbulu, A, and Delgado Bermejo, JV. The youngest, the heaviest and/or the darkest? Selection potentialities and determinants of leadership in Canarian dromedary camels. Animals. (2021) 11:2886. doi: 10.3390/ani11102886
78. de Oliveira Bussiman, F, da Costa Perez, B, Ventura, RV, Esilva, FF, Peixoto, MGCD, Vizoná, RG, et al. Genetic analysis of morphological and functional traits in Campolina horses using Bayesian multi-trait model. Livest Sci. (2018) 216:119–29. doi: 10.1016/j.livsci.2018.08.002
79. Pratt-Phillips, S, and Munjizun, A. Impacts of adiposity on exercise performance in horses. Animals. (2023) 13:666. doi: 10.3390/ani13040666
80. Gómez, M, Gama, L, León, J, Fernández, J, Attalla, S, and Delgado, J. Genetic parameters for harmony and gaits in Hispano-Arabe horses estimated by Bayesian methods and restricted maximum likelihood. Livest Sci. (2016) 188:159–65. doi: 10.1016/j.livsci.2016.04.016
81. Adah, AS, Ayo, JO, and Adah, DA. Unique physiological and behavioural adaptive features of the one-humped camel (Camelus dromedarius) to arid environments. J Appl Vet Sci. (2023) 8:57–64. doi: 10.21608/JAVS.2022.168375.1184
82. Sbihi, HM, Nehdi, IA, and Al-Resayes, SI. Characterization of Hachi (Camelus dromedarius) fat extracted from the hump. Food Chem. (2013) 139:649–54. doi: 10.1016/j.foodchem.2013.01.118
83. Pfau, T, Hinton, E, Whitehead, C, Wiktorowicz-Conroy, A, and Hutchinson, J. Temporal gait parameters in the alpaca and the evolution of pacing and trotting locomotion in the Camelidae. J Zool. (2011) 283:193–202. doi: 10.1111/j.1469-7998.2010.00763.x
85. Webb, S. Locomotor evolution in camels Forma et functio. An international journal of functional biology. Brunswick, Germany. (1972).
86. Andersson, LS, Larhammar, M, Memic, F, Wootz, H, Schwochow, D, Rubin, C-J, et al. Mutations in DMRT3 affect locomotion in horses and spinal circuit function in mice. Nature. (2012) 488:642–6. doi: 10.1038/nature11399
87. Entin, P. Do racehorses and greyhound dogs exhibit a gender difference in running speed? Equine and Comp Exerc Physiol. (2007) 4:135–40. doi: 10.1017/S1478061507851036
88. Vicente, A, Carolino, N, Ralão-Duarte, J, and Gama, L. Selection for morphology, gaits and functional traits in Lusitano horses: II. Fixed effects, genetic trends and selection in retrospect. Livest Sci. (2014) 164:13–25. doi: 10.1016/j.livsci.2014.03.017
89. Senefeld, JW, Shepherd, JR, Baker, SE, and Joyner, MJ. Sex-based limits to running speed in the human, horse and dog: the role of sexual dimorphisms. FASEB J. (2021) 35:e21562. doi: 10.1096/fj.202100161R
90. Finn, JL, Haase, B, Willet, CE, van Rooy, D, Chew, T, Wade, CM, et al. The relationship between coat colour phenotype and equine behaviour: a pilot study. Appl Anim Behav Sci. (2016) 174:66–9. doi: 10.1016/j.applanim.2015.11.004
91. Jacobs, LN, Staiger, EA, Albright, JD, and Brooks, SA. The MC1R and ASIP coat color loci may impact behavior in the horse. J Hered. (2016) 107:214–9. doi: 10.1093/jhered/esw007
92. Kukekova, AV, Trut, LN, and Acland, GM. Genetics and the behavior of domestic animals: chapter 10 In: Genetics of domesticated behavior in dogs and foxes. Amsterdam, The Netherlands: Elsevier Inc. Chapters (2013)
93. van Rooy, D, and Wade, CM. Association between coat colour and the behaviour of Australian Labrador retrievers. Canine Genet Epidemiol. (2019) 6:1–7. doi: 10.1186/s40575-019-0078-z
94. Williams, J, and Tabor, G. Rider impacts on equitation. Appl Anim Behav Sci. (2017) 190:28–42. doi: 10.1016/j.applanim.2017.02.019
95. Holt, K. M. (2006). Performance of the flat walking Tennessee walking horse yearling before and after a 60-day strength training regime. Mississippi, USA: Theses and Dissertations. Mississippi State University.
96. Inkilä, L, Hyytiäinen, HK, Hielm-Björkman, A, Junnila, J, Bergh, A, and Boström, A. Part I of finnish agility dog survey: training and management of competition-level agility dogs. Animals. (2022) 12:212. doi: 10.3390/ani12020212
97. Ripollés-Lobo, M, Perdomo-González, D, Sánchez-Guerrero, M, Bartolomé, E, and Valera, M. Genetic relationship between free movement and under rider gaits in young Pura Raza Española horses. Livest Sci. (2022) 263:105031. doi: 10.1016/j.livsci.2022.105031
98. Iglesias Pastrana, C, Navas González, FJ, Ciani, E, Camacho Vallejo, ME, and Delgado Bermejo, JV. Bayesian linear regression and natural logarithmic correction for digital image-based extraction of linear and tridimensional zoometrics in dromedary camels. Mathematics. (2022) 10:3453. doi: 10.3390/math10193453
99. Xue, F., Chen, X., Liu, J., and Nardi, D. (2012). Real time biped walking gait pattern generator for a real robot. Paper presented at the RoboCup 2011: Robot Soccer World Cup XV 15.
100. Oliveira, RF. Social behavior in context: hormonal modulation of behavioral plasticity and social competence. Integr Comp Biol. (2009) 49:423–40. doi: 10.1093/icb/icp055
101. Weishaupt, MA, Hogg, H, Auer, J, and Wiestner, T. Velocity-dependent changes of time, force and spatial parameters in warmblood horses walking and trotting on a treadmill. Equine Vet J. (2010) 42:530–7. doi: 10.1111/j.2042-3306.2010.00190.x
102. Lynghaug, F. The official horse breeds standards guide: the complete guide to the standards of all north American equine breed associations. Minnesota, United States: Voyageur Press (2009).
103. Larsson, M, and Brothers, D. Camels in animal-assisted interventions – Survey of practical experiences with children, youth and adults. Bergen, Norway: Paper presented at the International Society of Applied Ethology (2019).
Keywords: gait performance, quantitative genetics, curve estimation regression models, discriminant analysis, breeding criteria, dromedary camel
Citation: Iglesias Pastrana C, Navas González FJ, Ciani E, Marín Navas C and Delgado Bermejo JV (2024) Determination of breeding criteria for gait proficiency in leisure riding and racing dromedary camels: a stepwise multivariate analysis of factors predicting overall biomechanical performance. Front. Vet. Sci. 10:1297430. doi: 10.3389/fvets.2023.1297430
Edited by:
Daniel Mota-Rojas, Metropolitan Autonomous University, MexicoReviewed by:
Sumant Vyas, Central Arid Zone Research Institute (ICAR), IndiaAlejandro Casas Alvarado, Autonomous Metropolitan University, Mexico
Copyright © 2024 Iglesias Pastrana, Navas González, Ciani, Marín Navas and Delgado Bermejo. This is an open-access article distributed under the terms of the Creative Commons Attribution License (CC BY). The use, distribution or reproduction in other forums is permitted, provided the original author(s) and the copyright owner(s) are credited and that the original publication in this journal is cited, in accordance with accepted academic practice. No use, distribution or reproduction is permitted which does not comply with these terms.
*Correspondence: Francisco Javier Navas González, ZmpuYXZhc0B1Y28uZXM=