- 1Yunnan Provincial Key Laboratory of Animal Nutrition and Feed Science, Faculty of Animal Science and Technology, Yunnan Agricultural University, Kunming, China
- 2College of Biotechnology and Engineering, West Yunnan University, Lincang, China
- 3College of Veterinary Medicine, Yunnan Agricultural University, Kunming, China
Pu-erh tea pomace (PTP), a solid substance after extracting functional substances or steeping tea, is rich in crude protein, and crude fiber, and could be used as considerable bioactive substances in animal production. However, its application as poultry feed and its role in regulating the characteristics of gut microorganisms is unclear. The present study investigated the effects of PTP on growth performance and gut microbes of chicken. A total of 144 Chahua chickens No. 2 were individually housed and divided into three groups which were fed diets containing 0% (CK), 1% PTP (T1), and 2% PTP (T2), respectively. The serum and cecum contents were collected after slaughter for analysis. The results indicated that growth performance and carcass traits were not affected by the PTP content. Serum total triglyceride (TG), total cholesterol (TC), and low-density lipoprotein cholesterol (LDL-C) levels in the T1 and T2 groups were significantly lower than in the CK group (p < 0.05). The gut microbiota α-diversity in the T2 group was significantly lower than in the CK group (p < 0.05). Based on partial least squares-discriminant analysis (PLS-DA), we observed significant segregation in gut bacterial communities among the groups. At the phylum level, Bacteroidetes and Firmicutes were dominant in the cecum, occupying about 85% of the cecum flora. The relative abundance of Bacteroidetes tended to increase. At the genus level, the relative abundance of Bacteroides is the highest in the CK、T1 and T2 groups. The relative abundances of Bacteroides and Prevotellaceae_UCG-001 microorganisms in the T2 group were significantly higher than in the CK group (p < 0.05). However, the relative abundance of CHKCI001 microorganisms in the T2 group was significantly lower compared to the CK group (p < 0.05). TG content was significantly positively correlated with CHKCI001 relative abundance, and significantly negatively correlated with Prevotellaceae_UCG-001 relative abundance (p < 0.05). Moreover, the LDL-C content was significantly positively correlated with CHKCI001 relative abundance (p < 0.05). In conclusion, PTP could decrease the cholesterol levels in the blood by improving the composition of gut microbiota, which provides a reference for the application of PTP in the poultry industry.
1 Introduction
Global population growth is putting tremendous pressure on the food industry for meat, egg, and milk products, which are the best way for humans to obtain protein and energy (1, 2). Animal feed production accounts for approximately 70% of total production costs (3, 4), which restricts the development of animal husbandry (5). Therefore, developing non-conventional feed sources is one of the main tasks of those working in the livestock industry (6).
Pu-erh tea is one of the most consumed teas in China (7), It is an over-fermented tea formed under the complex joint action of many microorganisms, resulting in a unique flavor and rich composition (8), which storage has been 718,900 tons in 2019 (9). Tea pomace (TP), a solid substance after extracting functional substances or steeping tea, is rich in crude protein, crude fiber, and considerable bioactive substances (4, 10, 11). Tea, its by-products and extract can enhance production performance, improve egg and meat quality, reduce fat deposition, regulate the diversity and abundance of gut microbiota, and increase antioxidative properties in poultry (8, 12–17). However, TPT is rarely used as an unconventional feed source in the poultry industry.
The intestine is not only an important digestive organ of broilers but also contains abundant microorganisms (18, 19). There is a complex and close association between gut microbes and their hosts (20, 21), and healthy individuals are accompanied by a continuously and steadily changing microbial community (22, 23). In mature chickens (broilers and egg layers), advantageous microbes in the cecum are Firmicutes and Bacteroidetes (24). Numerous studies have shown that chicken production, fat deposition, and body metabolism are associated with the gut microbiota (24–28).
Chahua chickens No.2, a line of Chahua chickens obtained after multi-generation inbreeding in Yunnan Province, is a synthetic breed. This study aimed to investigate the feasibility of Pu-erh tea pomace (PTP) as a non-conventional feed resource, and its role in regulating chicken growth performance, serum biochemistry, and cecum flora diversity, which provides theoretical data and reference for the application in poultry production.
2 Materials and methods
2.1 Pu-erh tea pomace components
PTP grounds were obtained from a company specializing in Pu-erh tea (TAETEA Group; Kunming), which was dried at 65°C for 4 h, ground, and passed through a 40 mesh sieve. Moisture, crude ash, crude protein, crude fiber, and crude fat were determined separately according to the methods in GB 6435–2014, GB/T 6438–2007, GB/T 6432–2018, GB/T 6434–2022, and GB/T 6433–2006. Amino acids were determined using a Sykam fully automated analyzer module (S433D; Sykam, Germany), according to the method described in GB 5009.124–2016.
Tea polyphenol content was determined by a Multifunction microplate reader (Multiskan GO ELISA, Thermo, USA). Ethanol (1 mL) was added to TPT (0.1 g) in a 2 mL tube. The polyphenol in the sample was released by ultrasonication for 30 min at 60°C, and centrifuged at 12000 rpm for 10 min. The supernatant (0.25 mL) was taken into the assay tea polyphenol at 760 nm.
2.2 Experimental design and treatments
All animal procedures were performed according to the Guide for Animal Care and Use of Laboratory Animals in the Institutional Animal Care and Use Committee of Yunnan Agricultural University. The Department Animal Ethics Committee approved the experimental protocol of the Yunnan Agricultural University. All Chahua chickens are obtained from the farm of the Yunnan Agricultural University. All chickens were housed individually in 35 × 35 × 45 cm cages. Healthy Chahua chickens No.2 (meat-based laying hens; n = 144; 1730 g ± 50 g; 45 weeks old) were assigned to the control group (CK), trial 1 group (T1), and trial 2 groups (T2), which groups CK, T1, and T2 were fed a basic diet, 1% PTP, and 2% PTP, respectively. The nutritional requirements of the chickens were met by corn, soybean meal, and soybean oil, according to the feeding standard for yellow-finned meat-based laying hens mentioned in NY/T 33–2004 (Table 1). The chickens were fed the corresponding meal for 28 days (test phase). During the experiment, the chicken house was provided 16 h of light and 8 h of dark each day, the temperature was kept at (21 ± 2°C), and the humidity was maintained at (50–55%).
2.3 Performance measurement and sample collection
The feed intake, health status, and mortality of the chickens were recorded throughout the trial period. At 28 d, one chicken from each replicate was randomly selected for slaughter by carotid artery bloodletting. The blood (5 mL) was collected from the left inferior wing vein of the chicken through a negative pressure collection vessel. After 45 min, serum was collected by centrifugation at 3500 rpm/min for 10 min in a high-speed centrifuge. The serum was collected in tubes and stored in a refrigerator at −80°C. Carcass traits were determined, and the contents of the chicken cecum contents were stored in a − 80°C refrigerator for 16S rRNA sequencing.
2.4 Blood biochemistry indexs determination
The content of blood biochemistry indexs including glucose (GLU), total triglyceride (TG), total cholesterol (TC), low-density lipoprotein cholesterol (LDL-C), and high-density lipoprotein cholesterol (HDL-C) was measured by Mindray automatic analyzer (BS-190, Shenzhen Mindray Bio-Medical Electronics Co., Ltd., Shenzhen, Guangdong, China) according to the commercial kits (Shenzhen Mindray Bio-Medical Electronics Co., Ltd., Shenzhen, China) as described by Cheng (29).
2.5 16S rRNA sequencing
Cecal microbiota traits were determined according to a previously described method (4). The OmicSmart platform was used for composition, diversity, correlation, and functional prediction. 16S rDNA was extracted from the cecal contents of Chahua chicken No. 2 using the HiPure fecal DNA extraction kit, and the quality of extraction was detected by NanoDrop 2000 microspectrophotometer and agarose gel electrophoresis. The V3/V4 region of 16S rDNA was amplified by F:5 ‘-CCTACGGGNGGCGG-3’ /R:5 ‘-GGACTACHVGGGTATC TAAT-3 ‘primer sequence. The 16S rDNA sequence was amplified once and twice using the Q5 enzyme amplification system and KOD enzyme amplification, respectively. The PCR procedure was as follows: predenaturation at 95 ° C for 5 min; There were 30 cycles of denaturation at 95 ° C for 1 min, annealing at 60 ° C for 1 min, and stretching at 72 ° C for 1 min, followed by stretching at 72 ° C for 7 min. PCR instrument (ETC811, Eastwin Scientific Equipment, China). PCR products were purified using AMPure XP Beads. Secondary PCR amplification products were quantified using the ABI StepOnePlus Real-Time PCR system and sequenced by pooling in PE250 mode on a Novaseq 6,000. After sequencing, FASTP software was used to screen high-quality reads from raw reads, FLSAH and QIIME software were used for splicing and tag filtering, and UCHIME software was used to remove chimeras to obtain OTUs. Subsequently, the OTU with the lowest abundance was filtered (flattened) by the Omicsmart platform at a filtering level of 0.005% to reduce OTU misassembly results caused by low-abundance read clustering. Cluster analysis of the optimized OTUs was performed using UPARSE process software. According to the existing microbial annotation information, the OTU after quality control was aligned with the existing microbial information database, and the “OTU- microorganisms “annotation was completed by RDP classifier software. The composition and abundance of microbes in each group were analyzed based on the Omicsmart platform. The 16S rDNA results were annotated using Tax4FUN software and PICRUSt software to predict the functional and phenotypic differences in the microbiota between the different groups.
2.6 Correlation analysis of variance indicators
Spearman’s correlation coefficients for serum indicators (TG, TC, and LDL-C) and microorganisms were calculated using the Corr function in SAS 15.0 software. Correlation heat maps were generated using GraphPad PRISM 9 software.
2.7 Data analysis
Growth performance, carcass traits, and serum biochemical data were initially collated using excel, and all data were analyzed by the GLM method using SAS 15.0 software. 16S rRNA sequencing data were analyzed using the Omicsmart platform, and significance was tested using Welch’s t-test between two groups and the KW rank sum test between multiple groups. Data were expressed by sample mean and one total standard error of the mean (SEM), with p < 0.05 indicating a significant level of difference and p < 0.01 indicating a highly significant level of difference.
3 Results
3.1 Pu-erh tea pomace (PTP) contents
16 amino acids were detected in PTP. The highest content in PTP was glutamic acid (about 47.23 mg/g) and the lowest content in PTP was cysteine (about 0.4 mg/g). In addition, the crude protein, crude fiber, crude fat, crude ash, moisture, and total Polyphenols in PTP were about 27.27%, 21.5, 3.8%, 3.7%, 4.8%, and 2.61 mg/g, respectively (Table 2).
3.2 Growth performance and carcass traits
There were no significant differences in growth performance and carcass traits among the CK, T1, and T2 groups including initial body weight, final body weight, average daily feed intake, slaughter weight, half-evisceration weight, evisceration weight and belly fat weight (p > 0.05) (Table 3).
3.3 Blood biochemistry
Serum total triglyceride (TG), total cholesterol (TC), and low-density lipoprotein cholesterol (LDL-C) levels in the T1 and T2 groups were significantly lower than in the CK group (p < 0.05). Compared with the CK group, the TC content in the T1 and T2 groups was decreased by 25.75 and 28.14% (p < 0.05), respectively. The TG content in the T1 and T2 groups was decreased by 51.88 and 52.48% (p < 0.05), respectively. The LDL-C content in the T1 and T2 groups was decreased by 30 and 46.36% (p < 0.05), respectively (Table 4).
3.4 Microbiological diversity
The gut microbiota α-diversity in the T2 group was significantly lower than the CK group (p < 0.05), while there was no significant change in the T1 group (Figure 1). The gut microbiota α-diversity in the T2 group was significantly lower than in the T1 group (p < 0.05). Based on partial least squares- discriminant analysis (PLS-DA), we observed significant segregation in gut bacterial communities among the groups (Figure 2).
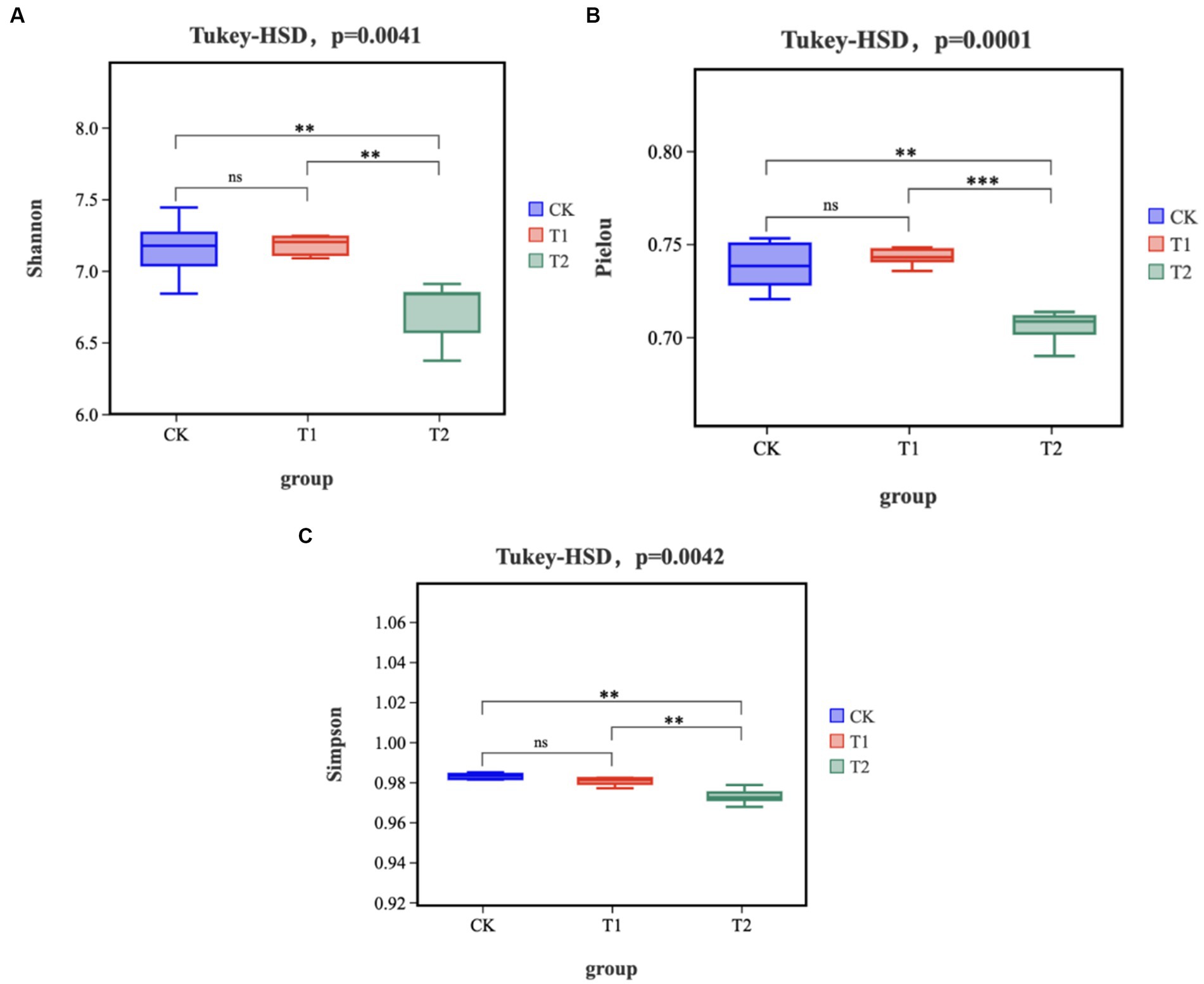
Figure 1. Alpha Diversity (A) Shannon index (B) Pielou index (C) Simpson index. ‘ns’ represents p > 0.05, ‘**’ represents p < 0.01, and ‘***’ represents p < 0.001. CK, 0% Pu-erh tea pomace diet; T1, 1.0% Pu-erh tea pomace diet; T2, 2.0% Pu-erh tea pomace diet. CK, 0% Pu-erh tea pomace diet; T1, 1.0% Pu-erh tea pomace diet; T2, 2.0% Pu-erh tea pomace diet.
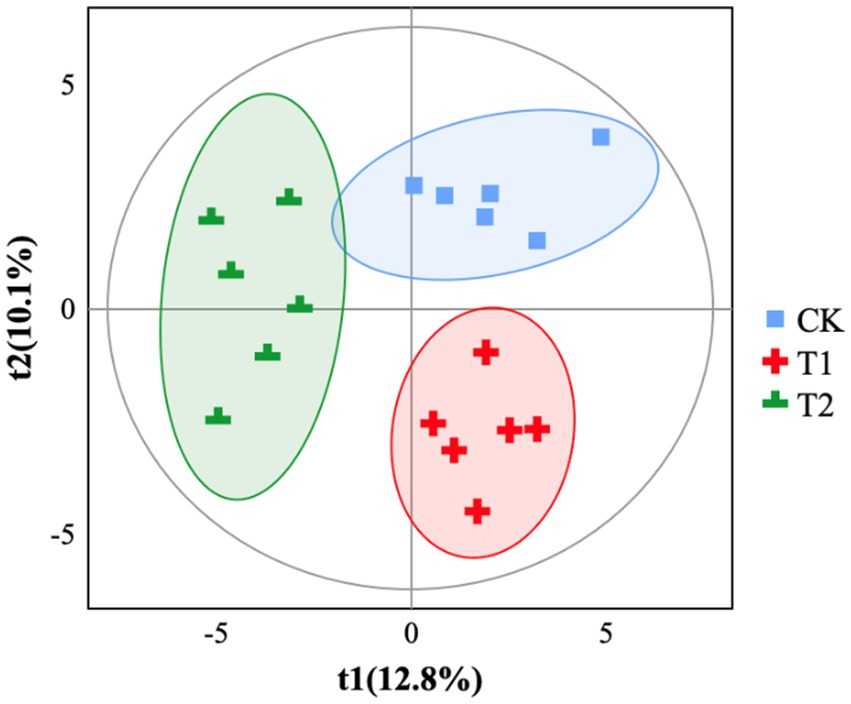
Figure 2. PLS-DA analysis. CK, 0% Pu-erh tea pomace diet; T1, 1.0% Pu-erh tea pomace diet; T2, 2.0% Pu-erh tea pomace diet. t1 (%): principal component 1, the position of the sample in the horizontal coordinate, the percentage indicates the interpretability of the sample by principal component 1; t2 (%): principal component 2, the position of the sample in the vertical coordinate, the percentage indicates the interpretability of the sample by principal component 2. Different groupings of samples are indicated using different colors. The more similar the sample composition, the closer the samples are on the graph.
3.5 OTU cluster and microbiological composition
A total of 914, 880, and 873 OTUs were observed in groups CK, T1, and T2, respectively. There are 725 OTUs in the intersection of 3 groups; 784 OTUs in the intersection of CK and T1; 789 OTUs in the intersection of CK and T2; and 766 OTUs in the intersection of T1 and T2. The number of OTUs crossed in the CK, T1, and T2 groups was 725, and the number of OTUs intersecting each other was 59, 41, and 64, respectively, While the number of OTUs exclusive to CK, T1, and T2 groups ware 66, 55 and 43, respectively (Figure 3).
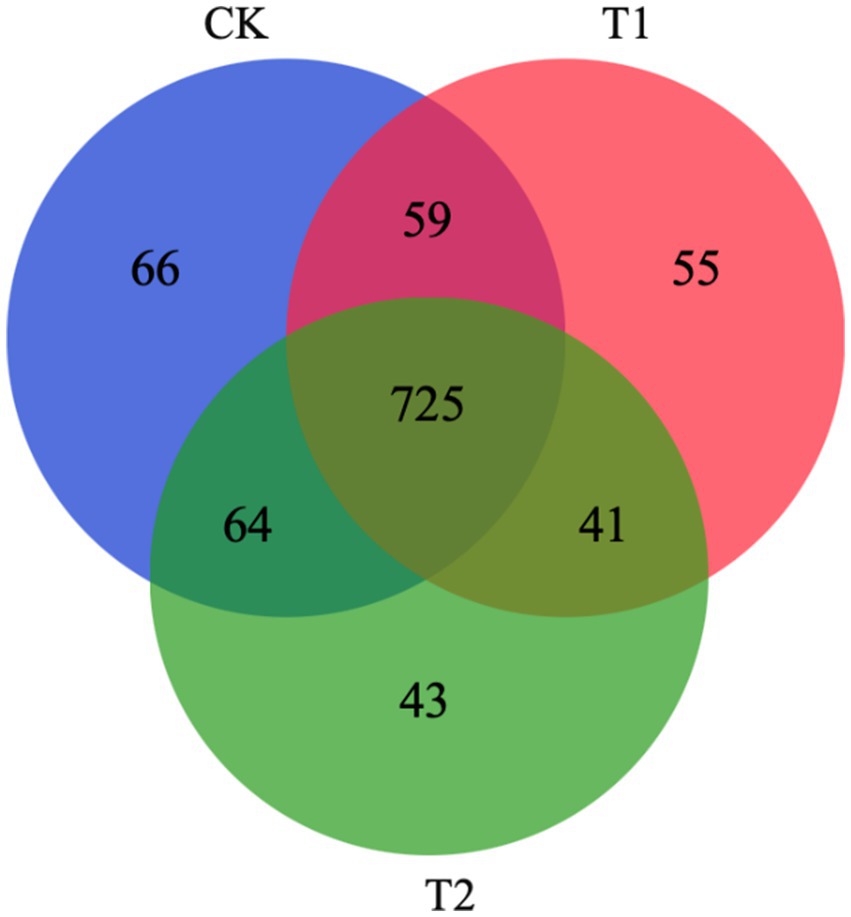
Figure 3. Shared OTU analysis of the different groups. CK, 0% Pu-erh tea pomace diet; T1, 1.0% Pu-erh tea pomace diet; T2, 2.0% Pu-erh tea pomace diet.
At the phylum level, Bacteroidetes, Firmicutes, Proteobacteria, and Spirochaetes were dominant in the cecum, occupying more than 90% of the cecum flora. The relative abundance of Bacteroidetes tended to increase. The ratio of Firmicutes/Bacteroidetes (F/B) in the T1 and T2 groups decreased by 14.12 and 34.00%, respectively. The ratio of F/B tended to decrease in contrast to the CK (Figure 4). At the genus level, Bacteroides, Rikenllaceae_RC9_gut_group, Phascolarctobaterium, Lactobacillus, Ruminococcus_torques_group, Prevotellaceae_UCG-001, and CHKCI001 were dominant. These microbes accounted for more than 45% of the horizontal microorganisms in the cecum, whereas unidentified species accounted for 36.58%. The relative abundance of Bacteroides is the highest in the CK、T1 and T2 groups (Figure 5).
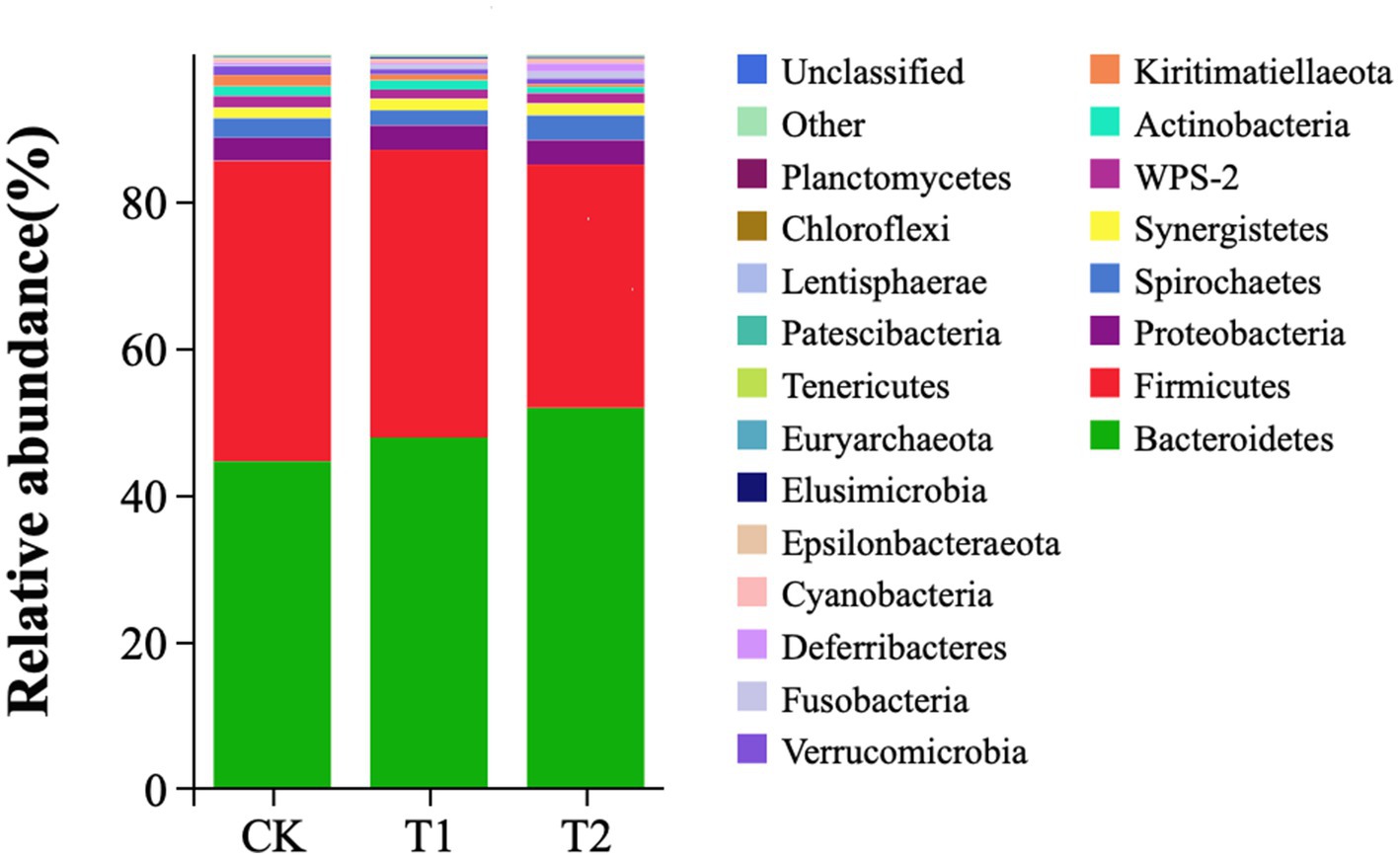
Figure 4. Microbial composition analysis (phylum level). CK, 0% Pu-erh tea pomace diet; T1, 1.0% Pu-erh tea pomace diet; T2, 2.0% Pu-erh tea pomace diet.
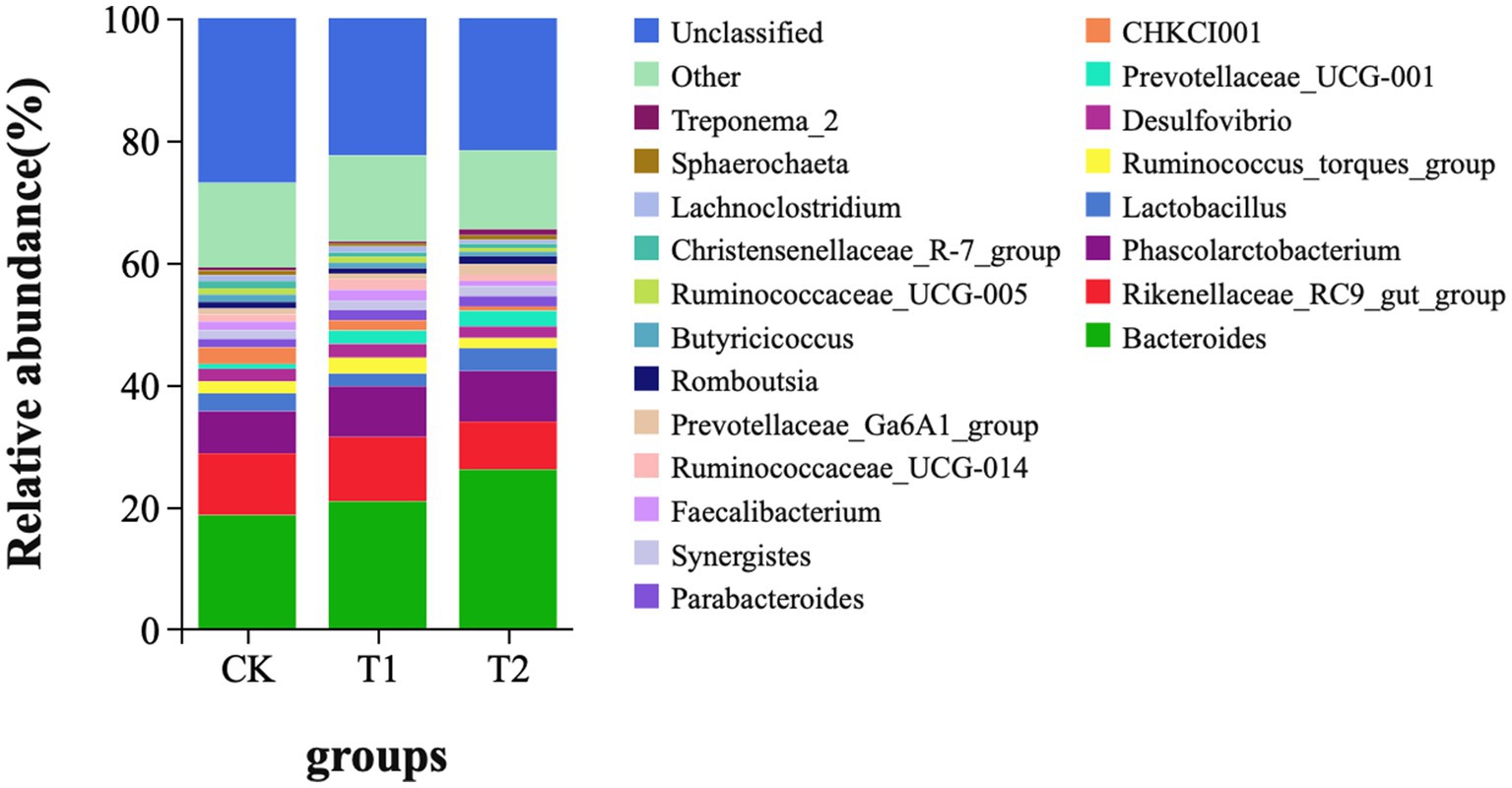
Figure 5. Microbial composition (genus level). CK, 0% Pu-erh tea pomace diet; T1, 1.0% Pu-erh tea pomace diet; T2, 2.0% Pu-erh tea pomace diet.
Eleven different genera were screened among the three groups: Bacteroides, Prevotellaceae_UCG-001, CHKCI001, Oscillibacter, Akkermansia, Eubacteri-um_coprostanoligenes_group, GCA-900066575, Ruminococcaceae_UCG-004, and Flavonifractor (Figure 6). The genera with connectivity ≥5 included Bacteroides, CHKCI001, Oscillibacter, and Akkermansia, among which the relative abundance and connectivity of Bacteroides were the largest, indicating that Bacteroides plays a role in regulating other indicator species, whereas the latter three genera may be the “central bridge” for Bacteroides to function.
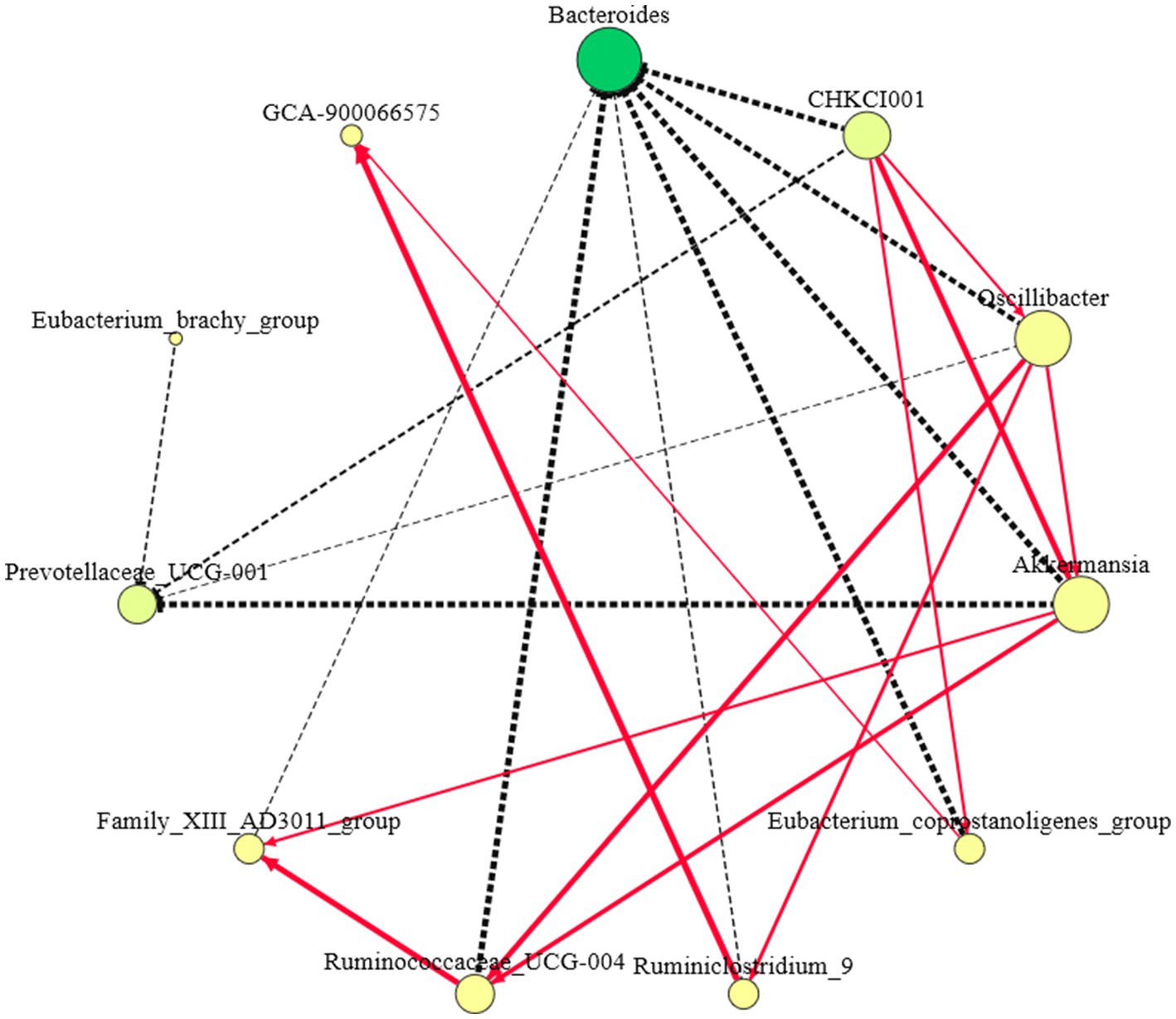
Figure 6. Indicator species correlation network diagram. (1) The red solid line represents the positive correlation of connected species, the black dashed line represents the negative correlation of connected species, and the thicker the line segment represents the stronger correlation; (2) The larger the node indicates stronger connectivity, and the darker the color indicates higher abundance.
Bacteroides negatively regulated CHKCI001, Oscillibacter, Akkermansia, Eubacterium_coprostanoligenes_group, Ruminiclostridium_9, Ruminococcaceae_UCG-004, and Family_XIII_AD3011_group. CHKCI001, Oscillibacter, Akkermansia, Ruminococcaceae_UCG-004, and Family_XIII_AD3011_group may be an intermediate group that transmits the positive regulatory effect of Bacteroides on Prevotellaceae_UCG-001, whereas Bacteroides negatively regulates GCA-900066575 through CHKCI001, Oscillibacter, Eubacterium_coprostanoligenes_group, and Ruminiclostridium_9.
3.6 Differential microbes
A Tukey-TSD test was performed on the top 10 genera of relative abundance in the cecum of chickens (Figure 7). The relative abundances of Bacteroides and Prevotellaceae_UCG-001 microorganisms in the T2 group were significantly higher than in the CK group (p < 0.05). However, the relative abundance of CHKCI001 microorganisms in the T2 group was significantly lower compared the CK group (p < 0.05). The relative abundances of Bacteroides and Prevotellaceae_UCG-001and CHKCI001 in group T1 was no significant difference in the cecum, in which the relative abundance of Prevotellaceae_UCG-001 tended to increase (p = 0.07).
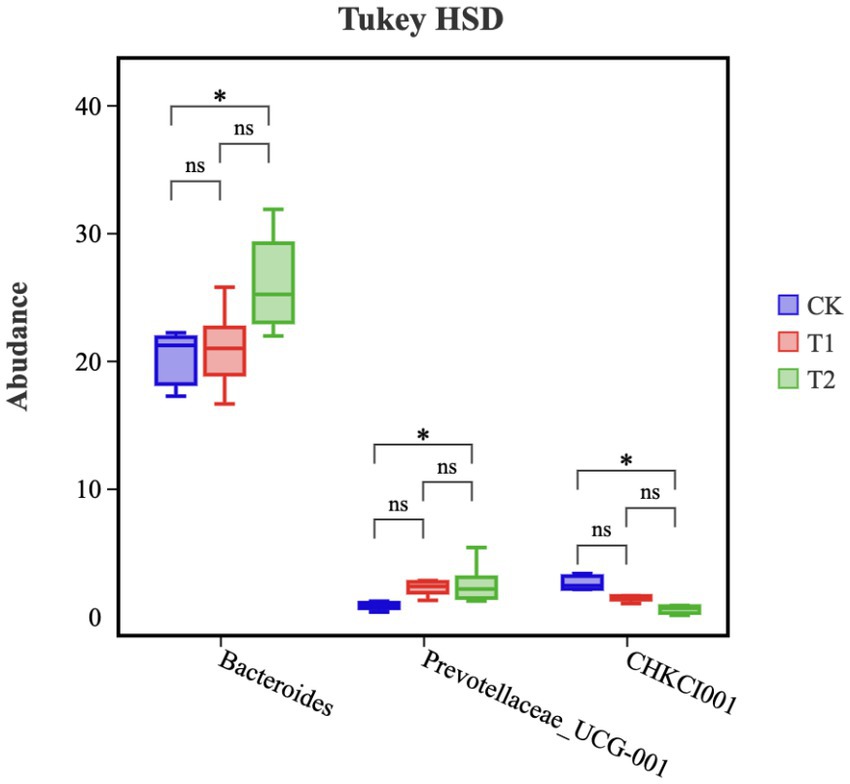
Figure 7. Microbial composition of cecum (genus). ‘*’ represents a significant difference, and ‘ns’ represents no significant difference. CK, 0% Pu-erh tea pomace diet; T1, 1.0% Pu-erh tea pomace diet; T2, 2.0% Pu-erh tea pomace diet.
The results of LEfSe analysis in the CK, T1 and T2 groups showed that Ruminococcaceae, Akkermansiaceae, Oscillibacter, Lachnospiraceae, Flavonifractor, Eubacterium_brachy_group, Family_XIII, Ruminococcaceae_UCG_004, Peptococcaceae, Ruminiclostridium_9, Clostridiales, Lactobacillus_agilis Angelakisella, Family_XIII_AD3011_group, Akkermansia, Clostridia, CHKCI001, Verrucomicrobiales, and Anaerotruncus clustered mainly to the CK group (p < 0.05). Aeriscardovia, Aeriscardovia_aeriphila, Eubacterium_coprostanoligenes_group, GCA_900,066,575, Hydrogenoanaerobacterium, Eubacterium, and Eubacterium_nodatum_group were mainly clustered to group T1 (p < 0.05), while Bacteroides, Propionibacteriales, Prevotellaceae_UCG_001, Intestinimonas, Bacteroidaceae, Mediterranea_massiliensis, Pirellulales, Sphingomonadaceae, Sphingomonadales, Nocardioidaceae, Nocardioides, Pirellulaceae, and Bacteroides_gallinaceum were mainly clustered to the T2 group (p < 0.05) (Figure 8).
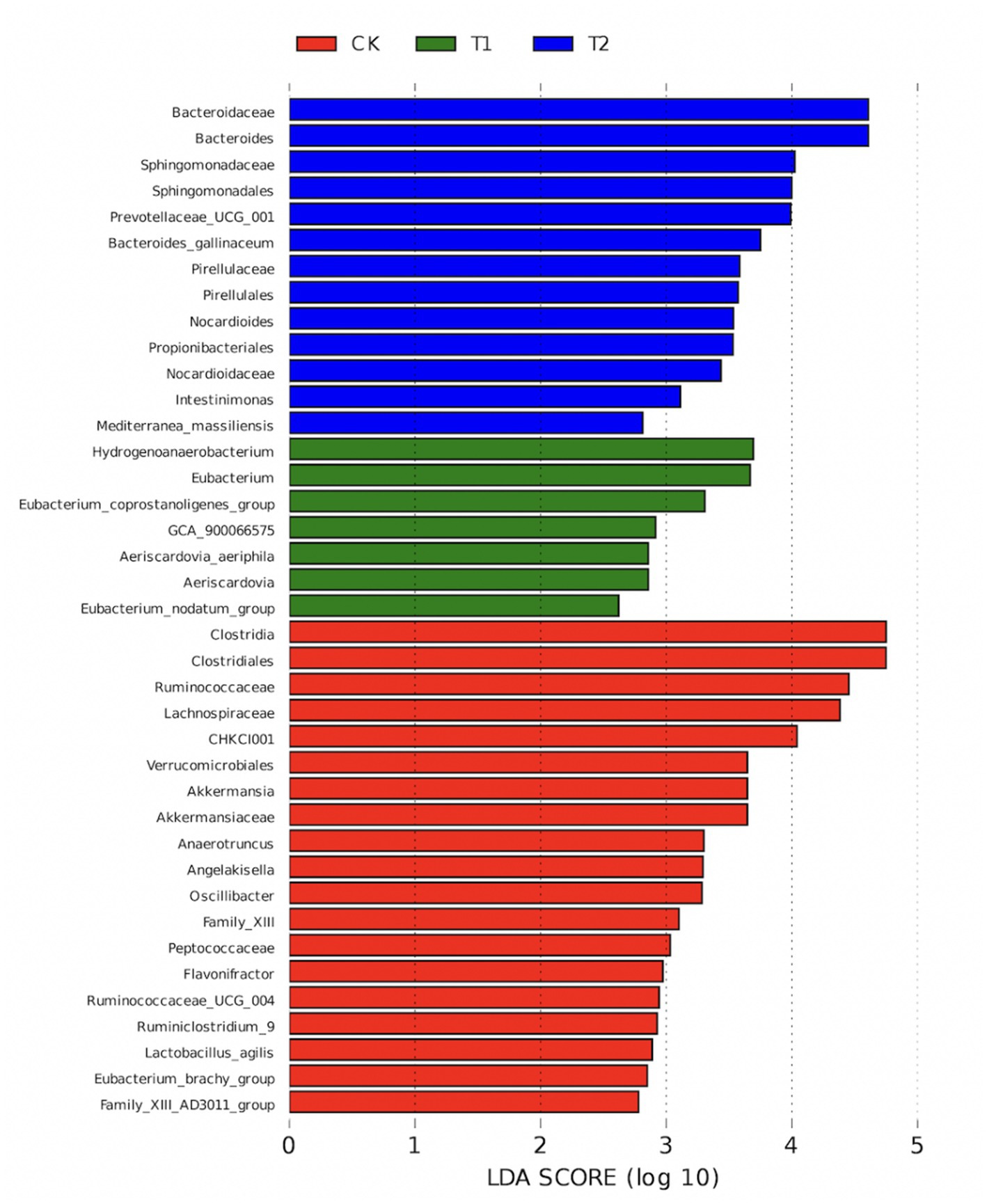
Figure 8. CK, T1, and T2 LEfSe analysis chart. CK, 0% Pu-erh tea pomace diet; T1, 1.0% Pu-erh tea pomace diet; T2, 2.0% Pu-erh tea pomace diet.
3.7 Functional forecast
At Level 3, the relative abundances of other glycan degradation, secondary bile acid biosynthesis, beta-alanine metabolism, primary bile acid biosynthesis, and apoptotic metabolism in the T2 group were significantly higher than those in the CK group (Figure 9). The relative abundance of metabolic pathways was significantly higher in the CK group, and the metabolic pathways of secondary bile acid biosynthesis, beta-alanine metabolism, and primary bile acid biosynthesis were higher than those in the T1 group, whereas the relative abundance of metabolic pathways in the CK and T1 groups was not significantly higher (Figure 9). The relative abundance of metabolic pathways was not significantly different between the CK and T1 groups (Figure 9).
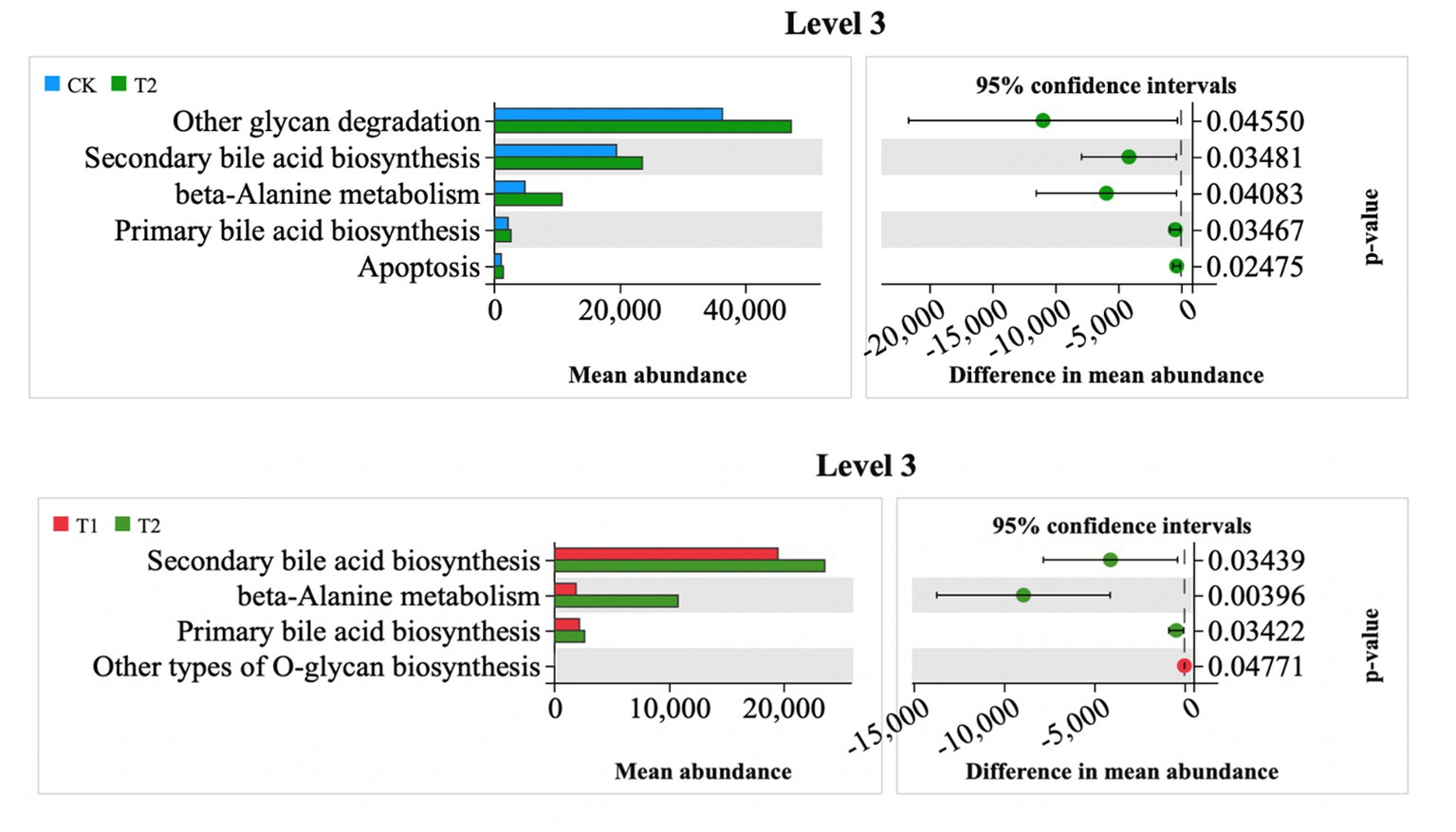
Figure 9. Differential metabolic pathway prediction map. CK, 0% Pu-erh tea pomace diet; T1, 1.0% Pu-erh tea pomace diet; T2, 2.0% Pu-erh tea pomace diet.
3.8 Correlation analysis
Serum TG levels were significantly positively correlated with CHKCI001 (p < 0.01) and negatively correlated with Prevotellaceae_UCG-001 (p < 0.05), whereas serum LDL-C levels were significantly positively correlated with CHKCI001 (p < 0.05), whereas serum TC levels were not correlated with Bacteroides, CHKCI001 or Prevotellaceae_UCG-001 (Figure 10).
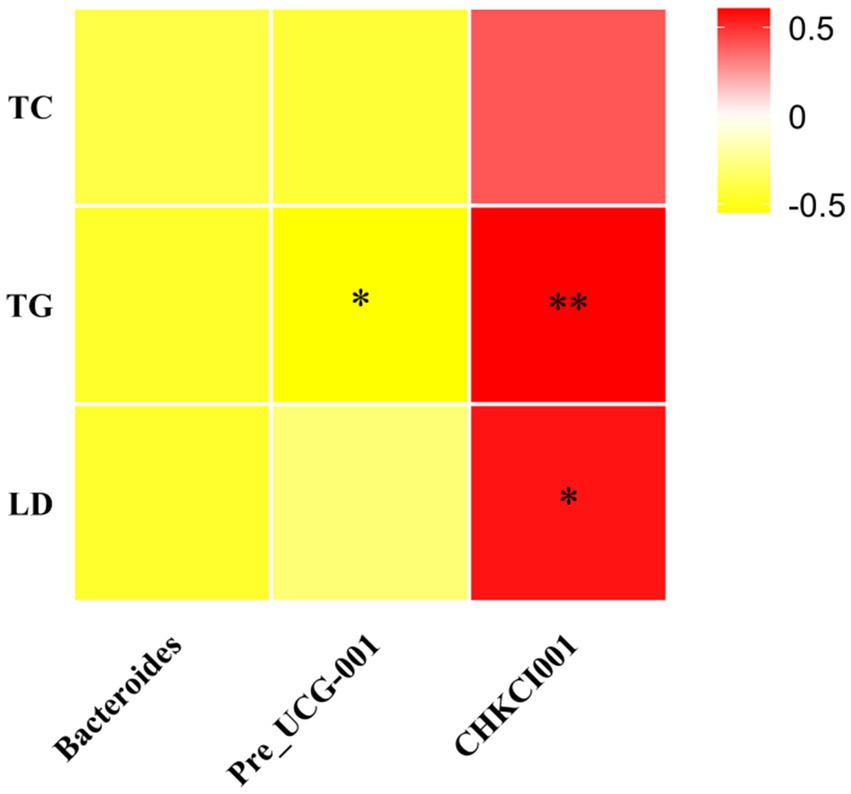
Figure 10. Heat map of microbial correlation between differential serum biochemical indicators and genus level. CK, 0% Pu-erh tea pomace diet; T1, 1.0% Pu-erh tea pomace diet; T2, 2.0% Pu-erh tea pomace diet.
4 Discussion
Pu-erh tea pomace (TPT) is the residue left after the extraction of active substances from Pu′er tea, most of which is treated and disposed of as waste. Some studies have shown that tea powder can improve the growth performance and intestinal microbial diversity of chickens (17, 18, 30). However, there are few reports on the nutritional composition of PTP and its impact on poultry production performance and intestinal microbiota. This study shows that the nutritional composition, amino acids, and tea polyphenols in PTP were measured. 16 amino acids were detected in PTP. The highest content in PTP was glutamic acid (about 47.23 mg/g) and the lowest content in PTP was cysteine (about 0.4 mg/g). In addition, the crude protein, crude fiber, crude fat, crude ash, moisture, and total Polyphenols in PTP were about 27.27%, 21.5, 3.8%, 3.7%, 4.8%, and 2.61 mg/g, respectively. The data showed that PTP contains abundant amino acids, crude protein, crude fiber, and tea polyphenols, which can be used as a non-conventional feed source.
We added 1 and 2% PTP to explore their effects on the chicken characteristics. The results showed that growth performance and carcass traits were not affected by the PTP content. Contrary to previous study results showing that green tea pomace could reduce the chicken abdominal fat rate (31), no significant changes in chicken abdominal fat occurred in this study. The timing of the experiment may not have been sufficient for the observation of adipose tissue, which is the final destination of fatty acids.
Pu-erh tea (PT) can reduce fat deposition, TG, TC, HDL-C, LDL-C, and GLU levels are important indicators of glucolipid metabolism. TC is the main form of energy storage in the body, and serum TG concentration shows the capacity of fat deposition in animals. TC is the total cholesterol contained in lipoproteins in the blood that is typically considered the primary biomarker of fatty acid metabolism (32). Previous reports have shown that pu-erh tea can reduce lipid deposition by regulating fat metabolism (33, 34). However, PTP addition did not reduce the weight and fat weight of chickens in the present study. This study indicated that PTP could decrease TG, TC, and LDL-C levels, which is consistent with the results of a previous study (30). Therefore, PTP may be responsible for regulating lipid metabolism.
In the digestive tract of chickens, complex gut microecosystems play a potential role in digesting and inhabiting pathogens and upholding the structural integrity of the digestive tract to promote a healthy body (35). The chicken cecum contains the largest number and diversity of microorganisms important for the growth, development, and health of chickens (24). Firmicutes and Bacteroidetes were the top two bacterial phyla in this study, which is consistent with a previous summary of chicken gut microbial data (36). Firmicutes are the main microbes and their relative abundance decreases with age in chicks, whereas the Bacteroidete’s relative abundance increases in mature chickens (35). This trend may be explained by the fact that mature chickens are equipped to feed more diverse and structurally complex diets for microorganisms that can degrade structured floating substances (37). In the present study, the relative abundance of Bacteroidetes increased, whereas that of Firmicutes decreased with the addition of 2% PTP. Studies have shown that the ratio of Firmicutes/Bacteroidetes was associated with lipid metabolism (38). In the present study, the ratio of Firmicutes/Bacteroidetes in the T1 and T2 groups tended to decrease in contrast to the CK group. Result, PPT affects the composition of gut microbes.
Pu-erh tea can reverse the low abundance and diversity of gut microbes associated with obesity (8, 15). This study showed that the cecal microbial alpha diversity index was reduced in the T2 group, and similar results were obtained in the Venn diagram. A previous study showed that alpha diversity was reduced in the group fed Pu-erh tea extract compared to that in the intestine of mice fed conventional chow (15). It is reasonable that PTP can reduce the alpha diversity of the flora in the cecum, as evidenced by the reduction in the abundance of CHK001 in this study. CHK001 belongs to the Lachnospiraceae family, indicating that most taxonomic species belong to an inferior group of bacteria (35). Based on partial least squares-discriminant analysis (PLS-DA), we observed significant segregation in gut bacterial communities among the groups, which directly indicated that a diet with 2% PTP shaped the structure of the cecal flora. Overall, feeding a diet with 2% PTP effectively altered the anatomical structure of the flora.
We performed a correlation analysis of the marker microorganisms in the three groups because the effect of microorganisms on the host is not caused by a single group (35). The results showed that Bacteroides increased the abundance of Prevotellaceae_UCG-001 by decreasing the abundance of CHKCI001, which in turn regulated the abundance of Eubacterium_coprostanoligenes_group that other related microorganisms may act as hubs. Another correlation result showed that blood TG levels were negatively correlated with Prevotellaceae_UCG-001 and TG, and LDL-C was positively correlated with CHKCI001, suggesting that altered microbial abundance may be the main reason for the decrease in blood lipid metabolism indicators (15). TG, TC, and LDL-C data showed that PTP affected lipid metabolism in chickens and was associated with Bacteroidetes. PTP could decrease the cholesterol levels in the blood by improving the composition of gut microbiota.
In conclusion, PTP could decrease the cholesterol levels in the blood by improving the composition of gut microbiota, which provides a reference for the application of PTP in the poultry industry. We would focus on further studies on the molecular mechanism by which PTP regulates lipid metabolism through microbial influence.
Data availability statement
The data presented in the study are deposited in the GenBank repository, accession numbers OR657057-OR658421.
Ethics statement
The animal study was approved by All animal experiments were approved by the Animal Ethics Committee of Yunnan Agricultural University. The study was conducted in accordance with the local legislation and institutional requirements.
Author contributions
YiH: Writing – original draft. YoH: Writing – original draft. ZP: Writing – review & editing. HH: Writing – review & editing. MY: Writing – review & editing. HP: Writing – review & editing. SZ: Writing – review & editing. YL: Writing – review & editing.
Funding
The author(s) declare financial support was received for the research, authorship, and/or publication of this article. This study was financially supported by the Open Fund of Yunnan Provincial Key Laboratory of Animal Nutrition and Feed Science (2021YNPKLANF005), the National Key Research and Development Program of China (2022YFD1601905), China Agriculture Research System (CARS-40-S25), and Scientific Research Fund project of Yunnan Education Department (2022J0291).
Conflict of interest
The authors declare that the research was conducted in the absence of any commercial or financial relationships that could be construed as a potential conflict of interest.
Publisher’s note
All claims expressed in this article are solely those of the authors and do not necessarily represent those of their affiliated organizations, or those of the publisher, the editors and the reviewers. Any product that may be evaluated in this article, or claim that may be made by its manufacturer, is not guaranteed or endorsed by the publisher.
References
1. Kim, SW, Less, JF, Wang, L, Yan, T, Kiron, V, Kaushik, SJ, et al. Meeting global feed protein demand: challenge, opportunity, and strategy. Annu Rev Anim Biosci. (2019) 7:221–43. doi: 10.1146/annurev-animal-030117-014838
2. Ricke, SC. Prebiotics and alternative poultry production. Poult Sci. (2021) 100:101174. doi: 10.1016/j.psj.2021.101174
3. Wen, C, Yan, W, Mai, C, Duan, Z, Zheng, J, Sun, C, et al. Joint contributions of the gut microbiota and host genetics to feed efficiency in chickens. Microbiome. (2021) 9:126. doi: 10.1186/s40168-021-01040-x
4. Yi, L, Zhang, Z, Li, Z, Li, Q, Yang, M, Huang, Y, et al. Effects of citrus pulp on the composition and diversity of broiler cecal microbes. Poult Sci. (2023) 102:102454. doi: 10.1016/j.psj.2022.102454
5. Kumar, P, Abubakar, AA, Verma, AK, Umaraw, P, Adewale Ahmed, M, and Mehta, N. New insights in improving sustainability in meat production: opportunities and challenges. Crit Rev Food Sci Nutr. (2022) 12:1–29. doi: 10.1080/10408398.2022.2096562
6. Gernat, AA, Santos, FBO, and Grimes, JL. Alternative approaches to antimicrobial use in the Turkey industry: challenges and perspectives. Ger J Vet Res. (2021) 1:37–47. doi: 10.51585/gjvr.2021.3.0018
7. Xu, J, Wei, Y, Li, F, Weng, X, and Wei, X. Regulation of fungal community and the quality formation and safety control of Pu-erh tea. Compr Rev Food Sci Food Saf. (2022) 21:4546–72. doi: 10.1111/1541-4337.13051
8. Liu, J, He, D, Xing, Y, Zeng, W, Ren, K, Zhang, C, et al. Effects of bioactive components of Pu-erh tea on gut microbiomes and health: a review. Food Chem. (2021) 353:129439. doi: 10.1016/j.foodchem.2021.129439
9. Dou, Z, Ji, M, Wang, M, and Shao, Y. Price prediction of Pu'er tea based on ARIMA and BP models. Neural Comput & Applic. (2022) 34:3495–511. doi: 10.1007/s00521-021-05827-9
10. Miao, S, Wei, Y, Chen, J, and Wei, X. Extraction methods, physiological activities and high-value applications of tea residue and its active components: a review. Crit Rev Food Sci Nutr. (2022) 14:1–19. doi: 10.1080/10408398.2022.2099343
11. Oanh, NC, Thu, CTT, Hong, NT, Giang, NTP, Hornick, JL, and Dang, PK. Growth performance, meat quality, and blood characteristics of finisher crossbred pigs fed diets supplemented with different levels of green tea (Camellia sinensis) by-products. Vet World. (2023) 16:27–34. doi: 10.14202/vetworld.2023.27-34
12. Biswas, AH, and Wakita, M. Effect of dietary Japanese green tea powder supplementation on feed utilization and carcass profiles in broilers. J Poult Sci. (2001) 38:50–7. doi: 10.2141/jpsa.38.50
13. Chen, X, Zhou, X, Li, S, Zhang, H, and Liu, Z. Effects of tea residues-fermented feed on production performance, egg quality, antioxidant capacity, caecal microbiota, and ammonia emissions of laying hens. Front Vet Sci. (2023) 10:1195074. doi: 10.3389/fvets.2023.1195074
14. Khan, SH. The use of green tea (Camellia sinensis) as a phytogenic substance in poultry diets. Onderstepoort J Vet Res. (2014) 81:1–8. doi: 10.4102/ojvr.v81i2.706
15. Lu, X, Liu, J, Zhang, N, Fu, Y, Zhang, Z, Li, Y, et al. Ripened Pu-erh tea extract protects mice from obesity by modulating gut microbiota composition. J Agric Food Chem. (2019) 67:6978–94. doi: 10.1021/acs.jafc.8b04909
16. Seidavi, A, Belali, M, Elgandour, MMY, Adegbeye, MJ, and Salem, AZM. Potential impacts of dietary inclusion of green tea (Camellia sinensis L.) in poultry feeding: a review. Agrofor Syst. (2020) 94:1161–70. doi: 10.1007/s10457-019-00444-x
17. Ye, J, Zhao, Y, Chen, X, Zhou, H, Yang, Y, Zhang, X, et al. Pu-erh tea ameliorates obesity and modulates gut microbiota in high-fat diet-fed mice. Food Res Int. (2021) 144:110360. doi: 10.1016/j.foodres.2021.110360
18. Rahman, MM, Hossain, MS, Abid, MH, Nabi, MR, and Hamid, MA. Effect of green tea powder as an alternative of antibiotic on growth performance, meat quality and blood lipid profile of broiler. Bangladesh J Vet Med. (2018) 16:23–9. doi: 10.3329/bjvm.v16i1.37369
19. Shehata, AA, Attia, Y, Khafaga, AF, Farooq, MZ, El-Seedi, HR, and Eisenreich, W. Restoring healthy gut microbiome in poultry using alternative feed additives with particular attention to phytogenic substances: challenges and prospects. Ger J Vet Res. (2022) 2:32–42. doi: 10.51585/gjvr.2022.3.0047
20. Hou, J, Xiang, J, Li, D, Liu, X, and Pan, W. Gut microbial response to host metabolic phenotypes. Front Nutr. (2022) 9:1019430. doi: 10.3389/fnut.2022.1019430
21. Wang, C, and Jin, L. Microbial persisters and host: recent advances and future perspectives. Crit Rev Microbiol. (2022) 49:658–70. doi: 10.1080/1040841X.2022.2125286
22. Pan, S, Yan, J, Xu, X, Chen, Y, Chen, X, Li, F, et al. Current development and future application prospects of plants-derived polyphenol bioactive substance curcumin as a novel feed additive in livestock and poultry. Int J Mol Sci. (2022) 23:11905. doi: 10.3390/ijms231911905
23. Zheng, Y, Zhang, Z, Tang, P, Wu, Y, Zhang, A, Li, D, et al. Probiotics fortify intestinal barrier function: a systematic review and meta-analysis of randomized trials. Front Immunol. (2023) 14:1143548. doi: 10.3389/fimmu.2023.1143548
24. Kers, JG, Velkers, F, Fischer, EAJ, Hermes, GDA, Stegeman, JA, and Smidt, H. Host and environmental factors affecting the intestinal microbiota in chickens. Front Microbiol. (2018) 9:235. doi: 10.3389/fmicb.2018.00235
25. Bortoluzzi, C, Pedroso, AA, Mallo, JJ, Puyalto, M, Kim, WK, and Applegate, TJ. Sodium butyrate improved performance while modulating the cecal microbiota and regulating the expression of intestinal immune-related genes in broiler chickens. Poult Sci. (2017) 96:3981–93. doi: 10.3382/ps/pex218
26. Sonnenburg, JL, and Backhed, F. Diet-microbiota interactions as moderators of human metabolism. Nature. (2016) 535:56–64. doi: 10.1038/nature18846
27. Wen, C, Yan, W, Sun, C, Ji, C, Zhou, Q, Zhang, D, et al. The gut microbiota is largely independent of host genetics in regulating fat deposition in chickens. ISME J. (2019) 13:1422–36. doi: 10.1038/s41396-019-0367-2
28. Zhao, S, Zhang, K, Ding, X, Celi, P, Yan, L, Bai, S, et al. The impact of dietary supplementation of different feed additives on performances of broiler breeders characterized by different egg-laying rate. Poult Sci. (2019) 98:6091–9. doi: 10.3382/ps/pez316
29. Cheng, H, Chen, J, Tang, S, Guo, S, He, C, and Qu, X. Effects of essential oil/palygorskite composite on performance, egg quality, plasma biochemistry, oxidation status, immune response, and intestinal morphology of laying hens. Poult Sci. (2022) 101:101632. doi: 10.1016/j.psj.2021.101632
30. Li, J, Chang, X, Chen, X, Ma, R, Qi, R, Liu, W, et al. Effects of green tea powder on production performance, egg quality, and blood biochemical parameters in laying hens. Poult Sci. (2023) 102:102924. doi: 10.1016/j.psj.2023.102924
31. Hrnčár, C, and Bujko, J. Effect of different levels of green tea (Camellia sinensis) on productive performance, carcass characteristics, and organs of broiler chickens. Acta Fytotech Zootech. (2017) 11:623–8. doi: 10.15414/afz.2021.24.04.334-339
32. Qin, L, Huang, T, Jing, R, Wen, J, and Cao, M. Mulberry leaf extract reduces abdominal fat deposition via adenosine-activated protein kinase/sterol regulatory element binding protein-1c/acetyl-CoA carboxylase signaling pathway in female arbor acre broilers. Poult Sci. (2023) 102:102638. doi: 10.1016/j.psj.2023.102638
33. Hayat, K, Iqbal, H, Malik, U, Bilal, U, and Mushtaq, S. Tea and its consumption: benefits and risks. Crit Rev Food Sci Nutr. (2015) 55:939–54. doi: 10.1080/10408398.2012.678949
34. Huang, F, Wang, S, Zhao, A, Zheng, X, Zhang, Y, Lei, S, et al. Pu-erh tea regulates fatty acid metabolism in mice under high-fat diet. Front Pharmacol. (2019) 10:63. doi: 10.3389/fphar.2019.00063
35. Pan, D, and Yu, Z. Intestinal microbiome of poultry and its interaction with host and diet. Gut Microbes. (2014) 5:108–19. doi: 10.4161/gmic.26945
36. Oakley, BB, Lillehoj, HS, Kogut, MH, Kim, WK, Maurer, JJ, Pedroso, A, et al. The chicken gastrointestinal microbiome. FEMS Microbiol Lett. (2014) 360:100–12. doi: 10.1111/1574-6968.12608
37. Flint, HJ, Scott, KP, Louis, P, and Duncan, SH. The role of the gut microbiota in nutrition and health. Nat Rev Gastroenterol Hepatol. (2012) 9:577–89. doi: 10.1038/nrgastro.2012.156
Keywords: Pu-erh tea pomace, Chahua chickens No. 2, growth performance, blood biochemistry indexes, cecum microflora
Citation: Huang Y, He Y, Peng Z, Hu H, Yang M, Pan H, Zhao S and Li Y (2023) Effect of Pu-erh tea pomace on the composition and diversity of cecum microflora in Chahua chicken No. 2. Front. Vet. Sci. 10:1289546. doi: 10.3389/fvets.2023.1289546
Edited by:
Massimo Todaro, University of Palermo, ItalyReviewed by:
Petru Alexandru Vlaicu, National Research Development Institute for Animal Biology and Nutrition, RomaniaAwad A. Shehata, NAT Technische Universität München, Germany
Copyright © 2023 Huang, He, Peng, Hu, Yang, Pan, Zhao and Li. This is an open-access article distributed under the terms of the Creative Commons Attribution License (CC BY). The use, distribution or reproduction in other forums is permitted, provided the original author(s) and the copyright owner(s) are credited and that the original publication in this journal is cited, in accordance with accepted academic practice. No use, distribution or reproduction is permitted which does not comply with these terms.
*Correspondence: Sumei Zhao, emhhb3NtMjAwOUAxMjYuY29t; Yongneng Li, eW5hdWx5bjc3QHNpbmEuY29t
†These authors have contributed equally to this work