- 1Key Laboratory of Efficient Utilization of Non-grain Feed Resources (Co-construction by Ministry and Province), Ministry of Agriculture and Rural Affairs, Shandong Provincial Key Laboratory of Animal Biotechnology and Disease Control and Prevention, Department of Animal Science and Veterinary Medicine, Shandong Agricultural University, Tai’an, China
- 2Shandong Taishan Shengliyuan Group Co., Ltd., Tai’an, China
- 3Chambroad Holding Group, Binzhou, China
- 4College of Biology and Brewing Engineering, Mount Taishan College, Tai’an, China
- 5Shandong New Hope Liuhe Group Co., Ltd., Qingdao, China
The present study aimed to evaluate the application of different wheat bran fermentation sources in growing pigs. A total of 320 pigs (43 ± 0.21 kg), were randomly allocated to 5 groups in a 21-d trial. The control group was fed a basal diet (CON) containing raw wheat bran, and the other four treatments were fed the diets in which the raw wheat bran in the basal diet was substituted with Aspergillus niger (WBA), Bacillus licheniformis (WBB), Candida utilis (WBC), and Lactobacillus plantarum (WBL) fermented wheat bran, respectively. The results showed that compared to the CON group, the crude fiber and pH values were decreased (p < 0.05), while the gross energy (GE), crude protein (CP), and lactic acid values were increased (p < 0.05) in all the wheat bran fermented by different strains. Compared with other treatments, feeding B. licheniformis fermented wheat bran had higher final weight, average daily gain, as well as lower feed-to-gain ratio. Compared with CON group, pigs fed with fermented wheat bran diets had higher dry matter, CP, and GE availability, serum total protein, albumin and superoxide dismutase levels, and fecal Lactobacillus counts, as well as lower malondialdehyde level and fecal Escherichia coli count. Collectively, our findings suggested that feeding fermented wheat bran, especially B. licheniformis fermented wheat bran, showed beneficial effects on the growth performance, nutrient digestibility, serum antioxidant capacity, and the gut microbiota structure of growing pigs.
Introduction
With the steady growth of animal production, feed price is increased (1, 2). Correspondingly, fermentation is recognized as a very effective approach to improve the nutrition and digestion of unconventional feed sources (3–5). Numerous studies have illustrated that the fermented feed improved growth performance, antioxidant capacity, and intestine health of pigs (6–8).
Wheat bran, a byproduct derived from flour processing, is a readily accessible material with an annual global yield of 100–150 million tons (9). Due to its high contents of energy value, crude protein (CP), and trace elements, wheat bran is extensively utilized as animal feed raw materials (10). In feedstuff production, wheat bran processing primarily involves mechanical and chemical methods (11–13). Kraler et al. (14) reported that wheat bran fermented by Lactobacillus paracasei and Lactobacillus plantarum significantly increased the apparent digestibilities of dry matter (DM), organic matter (OM), ether extract (EE), and gross energy (GE) in growing pigs. Additionally, fermentation could effectively reduce the content of insoluble dietary fiber in rice bran and corn bran (15, 16), and the enzymes produced during fermentation can enhance the absorption of minerals (17). The Aspergillus niger, Bacillus licheniformis, Candida utilis and L. plantarum are the four strains used most widely in feed fermentation. Previous studies have demonstrated that the anti-nutritional factors presenting in soybean and sorghum, specifically glycinin, β-conglycinin and tannins, can be effectively degraded by fermentation with A. niger or L. plantarum (18, 19). Ahmed et al. (20) found that fermented feed by B. licheniformis significantly increased growth performance of broilers. The C. utilis fermented rapeseed meal improved the intestinal morphology of broilers (21). However, there is little literature available on the comparative study of wheat bran fermented by A. niger, B. licheniformis, C. utilis, and L. plantarum on fermentation parameters and their application on growing pigs.
Therefore, the experiment was conducted to investigate the effects of wheat bran fermented by different strains (A. niger, B. licheniformis, C. utilis, and L. plantarum) on growth performance, nutrient digestibility, serum antioxidant capacity, and fecal microbiota of growing pigs, and select the optimal strain for wheat bran fermentation, helping to select the most effective fermentative source to improve the feed utilization.
Materials and methods
Preparation of fermented wheat bran
The starter culture of A. niger (CICC 2041), B. licheniformis (CICC 21886), C. utilis (CICC 31430), and L. plantarum (CICC 6076) used in the test were obtained from Shandong Taishan Shengliyuan Group Co., Ltd. (Tai’an, China), which obtained according to optimized cultivation methods (Supplementary Table S1). Before fermentation, the moisture content of wheat bran was adjusted to 45%. Then the WBA, WBB, WBC, and WBL were fermented according to the conditions shown in Supplementary Table S2. The WBA, WBB, WBC, and WBL products were all obtained by drying at 45°C for approximately 48 to 72 h. Samples of fermented wheat bran were collected to determine their GE, CP, EE, and crude fiber (CF) contents according to the methods described by the Association of Official Analytical Chemists (AOAC) (22). To determine pH, a sample of 5 g fermented wheat bran was dissolved in 50 mL distilled water. The pH value of the supernatant was measured with pH meter (Shanghai Russell Technology Co., Ltd., Shanghai, China) after centrifugation at 4000 × g for 5 min. The lactic acid content was determined with a high-performance liquid chromatography autoanalyzer (Waters PICO TAG amino acid autoanalyzer; Millipore, MA, USA) according to standard procedures.
Experimental design and management
Three hundred and twenty (Duroc × Landrace × Yorkshire) pigs (43 ± 0.21 kg) were randomly divided into 5 treatments with 8 replicates per group and 8 pigs per replicate. Control group was fed a basal diet (CON), and other 4 treatments were fed the diets in which wheat bran of the basal diet were replaced with 10% A. niger (WBA), B. licheniformis (WBB), C. utilis (WBC), and L. plantarum (WBL) fermented wheat bran (air-dry basis), respectively. The basal diet (Table 1) was formulated with reference to National Research Council (NRC, 2012). Pigs were housed in a temperature-and humidity-controlled room (26–28°C, 55–60% RH), and were fed three times daily at 0800, 1400, and 1800 h. During the period of the experiment, all pigs had free access to feed and water. Daily feed intake per replicate was recorded, and the pigs were weighted individually on the morning of day 21 of the experiment before breakfast.
Apparent nutrient digestibility
Apparent nutrient digestibility was conducted using acid insoluble ash (AIA) indicator method. Fresh feces excreted by each replicate were collected daily for four consecutive days starting on day 17 of the experiment. Daily collected feces were weighed and mixed, and 10 mL of 10% sulfuric acid per 100 g of the feces samples were added to avoid evaporation of nitrogen in the form of ammonia. Samples were stored temporarily at −20°C. All fecal samples per replicate collected consecutively during the 4 days were mixed evenly, and the nutrient contents of fecal and feed samples including AIA, GE, DM, OM, EE, and CP were analyzed according to AOAC (2012) (22), respectively. The apparent digestibilities of all parameters were calculated as indicated below:
where AIA-1 was the AIA content of the diets, AIA-2 was the AIA content of the fecal, F-1 was the nutrient content of the diets, and F-2 was the nutrient content of the fecal.
Sampling procedure
Blood samples were taken from the jugular vein of 40 pigs (one pig per replicate) after being fasted for 12 h in the morning of d 21. About 5 mL of blood samples for hematological tests were collected into the routine blood tubes containing the anticoagulant (EDTA), and the other 10 mL were collected into another vacuum blood collection tube without anticoagulant for biochemical analyses. Serum samples were obtained after centrifugation at 3000 × g for 15 min and stored in 1.5 mL Eppendorf tubes at −20°C until analysis. Meanwhile, fresh fecal samples were collected from the 40 pigs by rectal stimulation, and then stored at −20°C until Escherichia coli and Lactobacillus analysis.
Serum biochemical parameters analysis
Serum biochemical parameters including alkaline phosphatase (ALP), aspartate amino transferase (AST), alanine aminotransferase (ALT), total protein (TP), albumin (ALB), triglyceride (TG), and total cholesterol (TCHO) were determined with commercial kits (Nanjing Jiancheng Bioengineering Institute, Nanjing, China) using an automatic clinical chemistry analyzer (Roche, Cobus-MiraPlus, Roche Diagnostic System Inc., United States).
Haemato-immunological blood parameters analysis
The white blood cell count (WBC), lymphocyte ratio (LYN), red blood cell count (RBC), hemoglobin (HGB), mean corpuscular volume (MCV), mean corpuscular hemoglobin (MCH), and mean corpuscular hemoglobin concentration (MCHC) were detected using an Sysmex KX-21 Automated Hematological Analyzer (Abacus Junior Vet, Diatron, Vienna, Austria) with specific software.
Determination of antioxidant index
The superoxide dismutase (SOD) activity and malondialdehyde (MDA) level of serum were determined by assay kits (Nanjing Jiancheng Biotechnology Institute, China) according to the manufacturer’s instructions.
Microbiota determination
The frozen fecal samples were incubated at 4°C for 10 h before enumeration. The E. coli and Lactobacillus were isolated by plating serial tenfold dilution (in sterile physiological saline) onto Luria Broth (LB) agar plates and MacConkey agar plates, respectively. All agar plates were incubated at 37°C for 36 h. The LB agar plates were aerobic incubation, while the MacConkey agar plates was incubated under the anaerobic conditions. Then the E. coli and Lactobacillus were counted according to the methods of Ganzle et al. (23) and Liu et al. (24).
Statistical analysis
The data were statistically analyzed using one-way ANOVA of SAS 9.2 (Inst. Inc., Cary, NC). Variations among the 5 treatments were compared with each other using Duncan’s multiple comparisons. The mean and total standard error of the means (SEM) were used to present the results, and the differences between treatments were considered significant when p < 0.05.
Results
Fermentation parameters and nutrient content of wheat bran
The fermentation parameters and nutrient content of wheat bran fermented by different strains are shown in Figure 1 and Table 2, respectively. Compared to CON treatment, all fermented WB treatments had lower pH values and CF content, and higher lactic acid and CP values (p < 0.05). Meanwhile, WBA, WBB, and WBC treatments had higher GE value compared to CON group (p < 0.05).
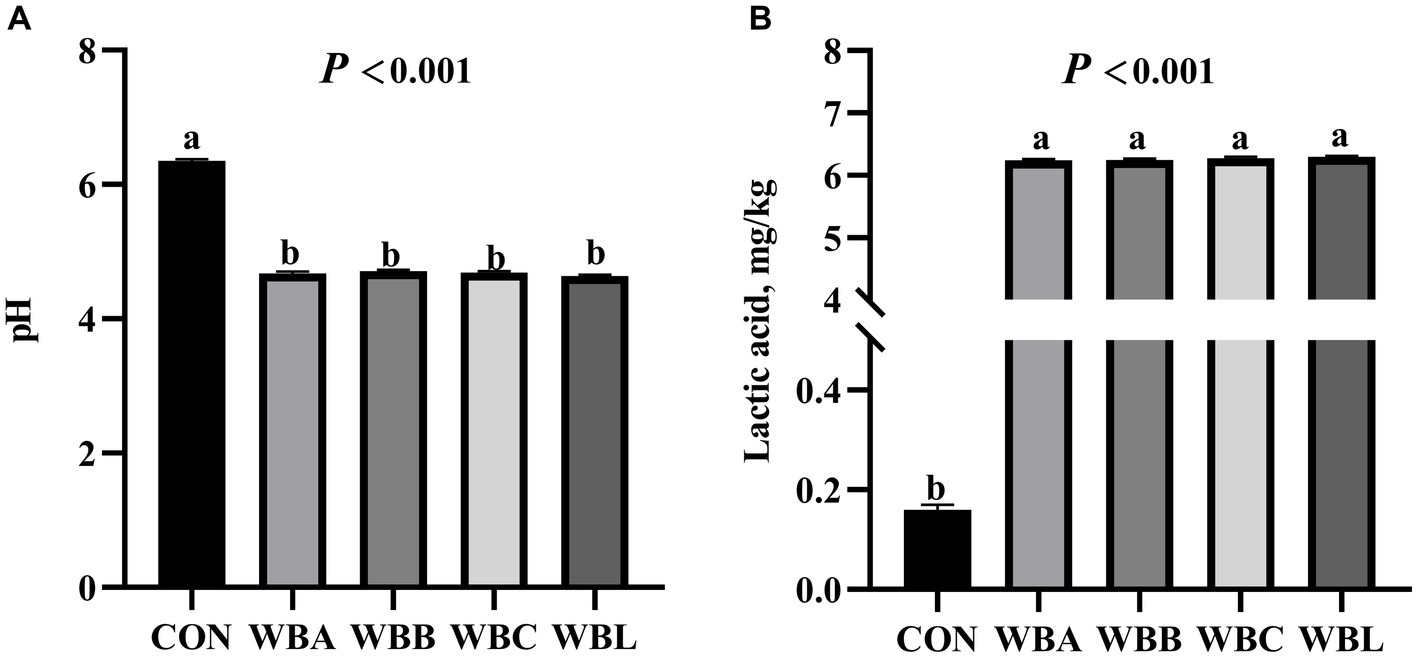
Figure 1. The fermentation parameters of wheat bran fermented by different strains (n = 8). CON is raw wheat bran, WBA, WBB, WBC, and WBL were A. niger, B. licheniformis, C. utilis, and L. plantarum fermented wheat bran, respectively. (A) The pH value; (B) The contents of lactic acid. Data are means for 8 replicates. a,bMeans among the experimental groups with different letters are significantly different (p < 0.05).
Growth performance
The effects of wheat bran fermented by different strains on the growth performance of growing pigs are shown in Table 3. Compared to the CON, WBA, WBB, and WBC treatments had higher final weight, ADG, and lower F/G (p < 0.05). Meanwhile, the WBB treatments had lower F/G value compared to the WBL group (p < 0.05).
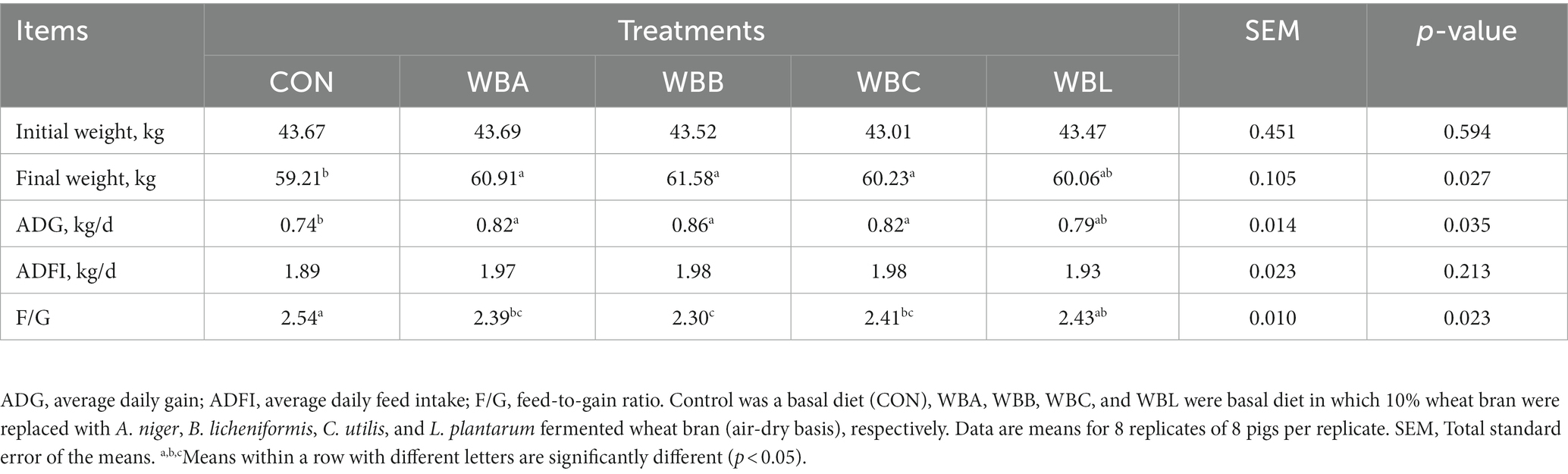
Table 3. Effects of wheat bran fermented by different strains on the growth performance of growing pigs.
Apparent nutrient digestibility
The effects of wheat bran fermented by different strains on the apparent nutrient digestibility of growing pigs are shown in Table 4. Compared to CON group, WBB, WBC, and WBL treatments had higher apparent digestibility of DM, while WBA and WBB treatments had higher apparent digestibility of CP (p < 0.05). All pigs fed fermented wheat bran diets exhibited higher apparent digestibility of GE (p < 0.05). There were no significant differences in the apparent digestibilities of OM and EE among all the experimental groups (p > 0.05).
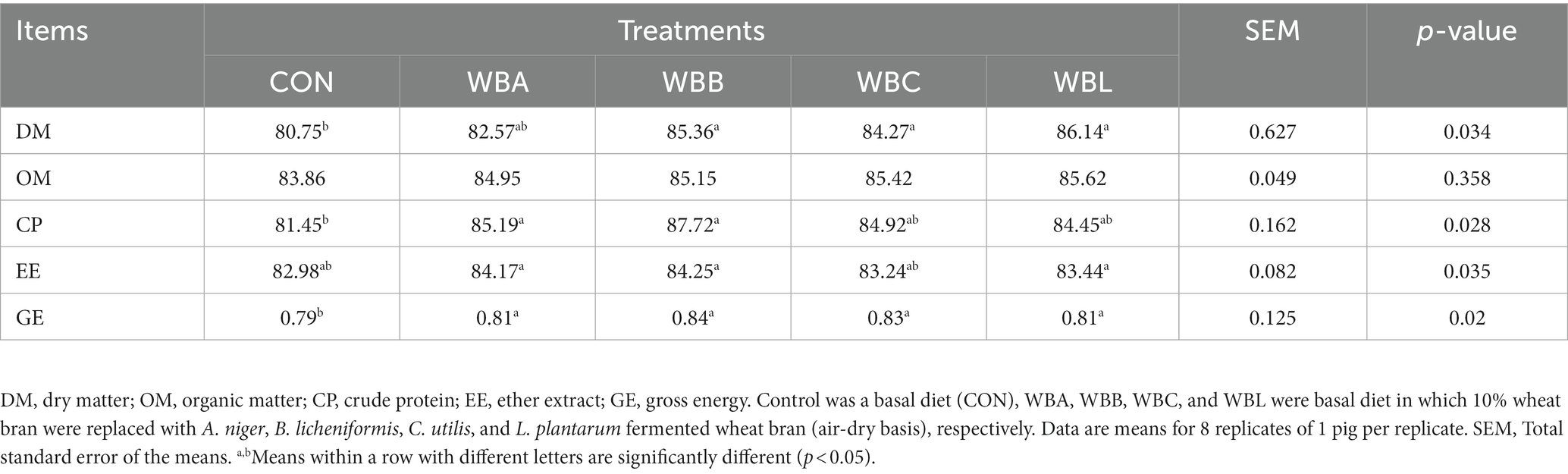
Table 4. Effects of wheat bran fermented by different strains on the apparent nutrient digestibility of growing pigs %.
Serum biochemical parameters
The effects of wheat bran fermented by different strains on the serum biochemical parameters of growing pigs are shown in Table 5. Compared to CON group, WBB treatment had higher serum ALP activity (p < 0.05). Meanwhile, all pigs fed fermented wheat bran diets had higher values of serum ALB and TP than CON group (p < 0.05). There were no significant differences in serum ALT, AST, TG, and TCHO concentrations among all the experimental groups (p > 0.05).
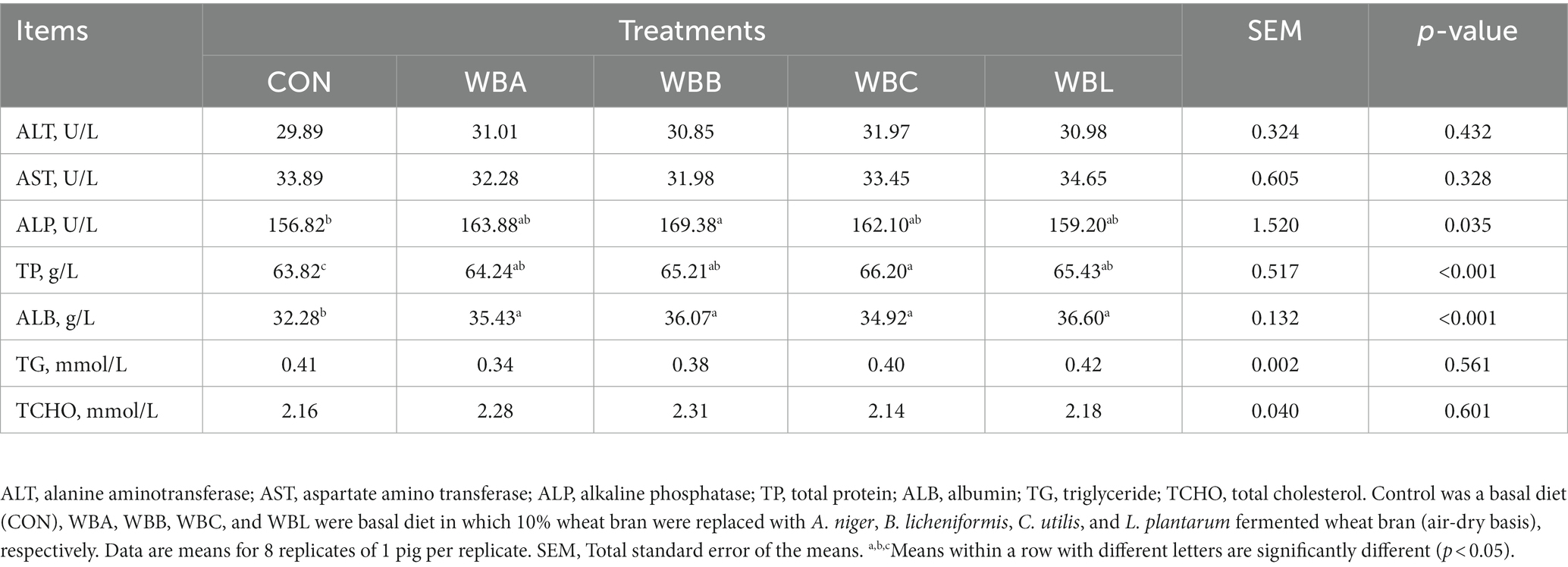
Table 5. Effects of wheat bran fermented by different strains on the serum biochemical parameters of growing pigs.
Haemato-immunological blood parameters
The effects of wheat bran fermented by different strains on the haemato-immunological blood parameters of growing pigs are shown in Table 6. Compared to the CON and WBA groups, WBB and WBC treatments had higher HGB value (p < 0.05). The higher MCH value were observed in WBB, WBC, WBL, and WBA treatments in respective order compared to the CON group (p < 0.05). There were no significant differences in WBC, LYN, RBC, MCV, and MCHC among all the experimental groups (p > 0.05).
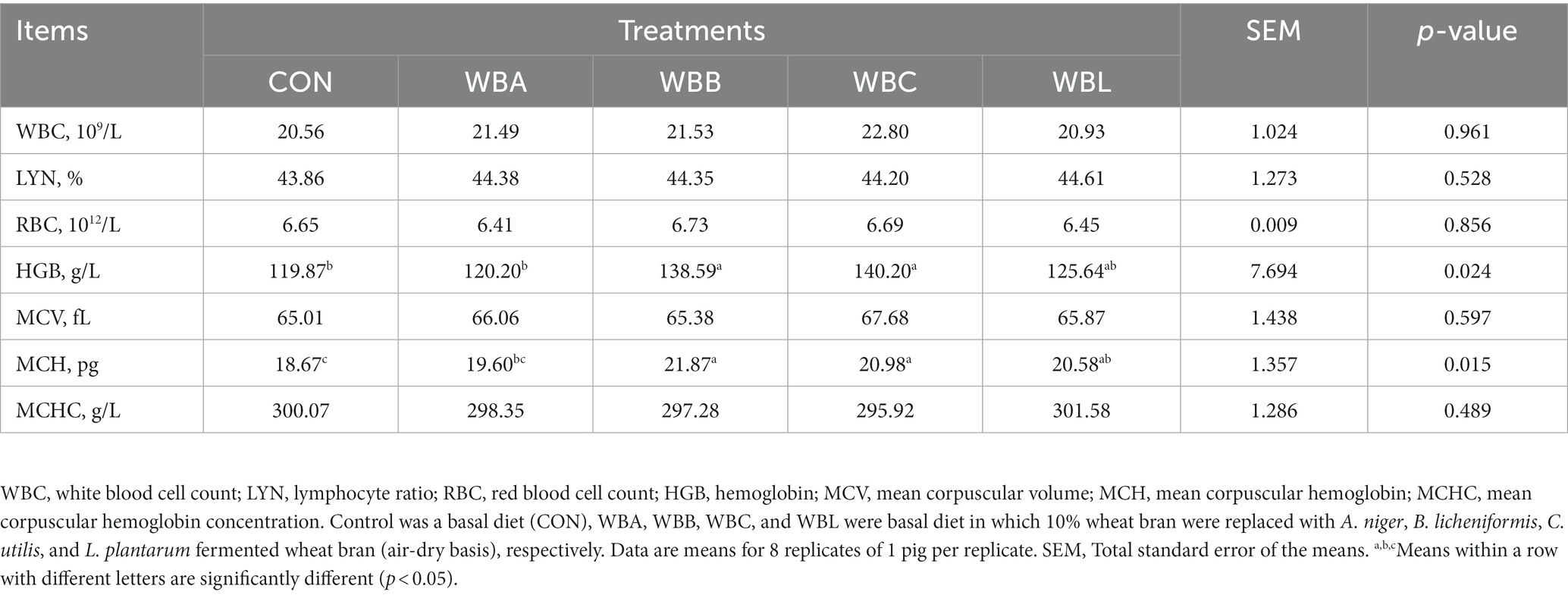
Table 6. Effects of wheat bran fermented by different strains on the haemato-immunological parameters of growing pigs.
Antioxidant index
The effects of wheat bran fermented by different strains on antioxidant capacity of growing pigs are shown in Table 7. Compared to CON, all pigs fed fermented wheat bran diets had higher serum SOD activity and lower MDA concentration (p < 0.05).
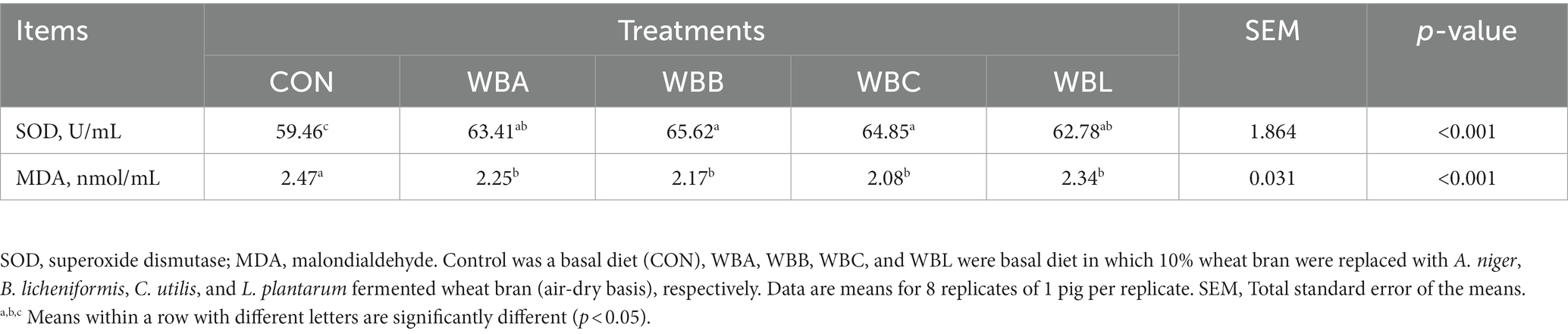
Table 7. Effects of wheat bran fermented by different strains on antioxidant capacity in serum of growing pigs.
Fecal microbiota
The effects of wheat bran fermented by different strains on fecal microbiota of growing pigs are shown in Table 8. Compared to the CON group, pigs fed B. licheniformis, C. utilis, and L. plantarum fermented wheat bran diets had lower fecal Escherichia coli count (p < 0.05); pigs fed fermented wheat bran diets exhibited higher fecal Lactobacillus counts (p < 0.05).
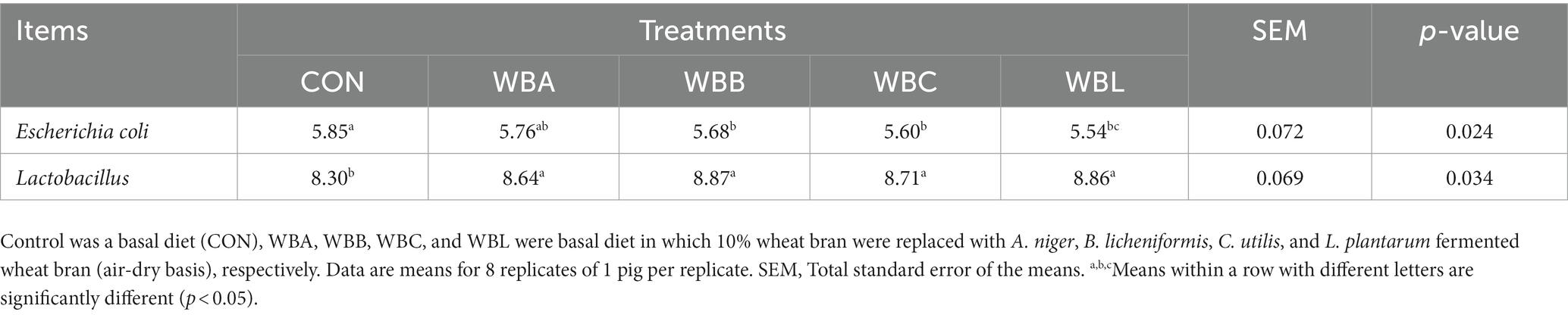
Table 8. Effects of wheat bran fermented by different strains on fecal microbiota of growing pigs (log10CFU/g).
Discussion
Microbial fermentation is considered a highly efficient and cost-effective processing technique for improving the nutritional quality of feed (25). The pH is a crucial reference used to estimate the quality of fermented feed, and a lower pH is advantageous for the nutrient digestion and suppression of harmful bacteria growth (26). Our results showed significant lower pH values of fermented wheat brans, which might be attributed to the increase in lactic acid (27). During the fermentation, the synthesis of microbial protein and the synergistic effect of enzymes secreted by strains may lead to an increase in CP content and a decrease in CF content (28). Consistent with previous research, our data also indicated that the CP values of wheat brans after fermentation were increased, while the CF values were decreased. Additionally, the GE values of wheat bran fermented by A. niger, B. licheniformis, and C. utilis were significantly higher than that of unfermented wheat bran, which was similar to Liu et al. (7).
Under the action of microorganisms, complex macromolecular compounds in feed are degraded into small molecular substances, which are effectively absorbed and utilized by animals (29). Vast majority of studies have reported the positive effects of fermented feed on growth performance. For instance, Dei et al. (20) found that feed fermented by A. niger increased feed intake and decreased F/G of broilers. Zhao et al. (30) also proved that fermented Ginkgo-leaves by C. utilis and A. niger improved the feed conversation ratio of laying hens. Similarly, our data showed that pigs fed with WBA, WBB, and WBC diets exhibited significant increases in the final weight and ADG values, and decreases in the F/G ratio. The mechanism by which fermented feed enhanced animal growth performance of above studies might be related to its promotion of intestinal development (31). However, pigs fed with wheat bran fermented by L. plantarum did not exhibit significant improvement in growth performance of pigs. Consistently, Le et al. (32) also did not observe significant difference of weaned pigs fed with wheat fermented by Lactobacillus.
The nutrient digestibility is an important indicator associated with growth performance. In the present study, the fermented wheat bran using different strains improved the digestibility of nutrient (CP, DM, and GE) to varying degrees, which was consistent with results in previous studies related to fermented soybean meal (33, 34). It was reported that the complex macromolecular compounds of wheat bran could be degraded into small molecular nutrients after fermentation (35), and fermented wheat bran supplementation could increase the digestive enzymatic activity in the intestine. Feng et al. (36) indicated that wheat bran fermented by Bacillus cereus increased the activity of amylase in the duodenum of broilers. Similar results were obtained from Deng et al. (37), who demonstrated that the Bacillus subtilis increased the activities of amylase and lipase in the ileum of piglets. It is worth emphasizing that Cruz et al. (38) demonstrated that dietary supplementation with C. utilis promoted the gene expression related to nutrient transporters in jejunum of broilers. Nevertheless, intestinal morphology analysis is necessary in the further study to determine the crucial mechanism of fermented wheat bran on nutrient digestibility.
The haemato-parameters are of great significance in evaluating physical health status and predicting disease occurrence (39). Our data showed the significant increase in HGB of the WBB and WBC diets, as well as MCH of the WBB, WBC, and WBL diets. These results suggested an improvement in the body’s iron absorption, which might be associated with a reduction in anti-nutritional factors (40). Dietary fiber, due to its high phytate content, has been reported to reduce the non-heme iron absorption through chelation (41). Therefore, the improvement of HGB and MCH in pigs fed fermented wheat bran might be attributed to the degradation of CF in wheat bran after fermentation.
Serum biochemical parameters reflect the nutritional metabolism and comprehensive functions of body organs, indirectly predicting the occurrence of diseases in pigs (42, 43). The ALP not only benefits the formation of hard tissue, especially bones, but also has anti-inflammatory functions (44). We found that the wheat bran fermented by B. licheniformis significantly increased serum ALP activity of pigs, which was consistent with previous studies in piglets (45) and tilapia fish (46). This might be due to the presences of abundant enzyme activities (phytase and enzymes that hydrolyze non starch polysaccharide components) in fermented feed, leading to an increase in mineral intake during the ossification process (47). Serum TP and ALB levels are related to body protein metabolism (48). Higher TP levels benefit to promote tissue development, while ALB is responsible for scavenging free radicals and transporting nutrients (49). In the present study, pigs fed the fermented wheat bran sources had higher serum TP and ALB concentration, indicating an improvement in body nutritional metabolism. The changes in serum TP and ALB concentration might be linked to the alteration of quantity and quality of proteins intake after wheat bran fermentation (50). Zhao et al. (35) reported that the high molecular weight proteins were degraded into low molecular weight peptides in wheat bran fermented by yeast.
MDA is an advanced oxidation product and a recognized biomarker of oxidative stress, whose presence have a negative impact on growth performance of pigs (51, 52). Serum SOD is a well-known antioxidant enzyme responsible for scavenging superoxide anion radicals and converting them into hydrogen peroxide and molecular oxygen (53, 54). In the present study, pigs fed with fermented wheat bran diets showed decreased MDA level and increased SOD activity in the serum, indicating an improvement in antioxidant capacity of the body. Zhao et al. (35) reported that yeast and Lactobacillus bulgaricus fermentation increased the total phenols contents in wheat bran, which contributed to the improvement of antioxidant capacity of the body. Additionally, Kumari et al. (55) found that B. licheniformis fermentation increased antioxidant activity of soybean meal hydrolysates. In summary, our findings suggested the fermented wheat bran had potential to alter the blood profiles of growing pigs, and the mechanism behind these associations needs further research.
The fecal microbiota plays a pivotal role in safeguarding the hosts against disease resulting from bacterial colonization, promoting nutrient absorption, and maintaining intestinal morphology (56, 57). The E. coli is a harmful pathogen known to cause poor growth performance, diarrhea and mortality in pigs (58). On the other hand, it has been reported that Lactobacillus casei has the ability to regulate intestinal immune function, maintain microbial balance and reduce inflammatory reactions (59). Multiple studies have demonstrated that fermented feed has the potential to decrease the abundance of Enterobacteriaceae and increase the abundance of Lactobacilli in the gastrointestinal tract of animals (60, 61). Consistent with previous studies, our data showed a significant decrease in fecal E. coli counts in pigs fed the WBB, WBC, and WBL diets, while a significant increase in fecal Lactobacillus counts in pigs fed the fermented wheat bran diets, which might associate with the increased lactic acid content in fermented wheat bran in our study. The presences of numerous organic acids in fermented feed not only establish an optimal environment for beneficial bacteria to adhere (4), but also compete against pathogenic bacteria by freely traversing membrane, resulting in enzyme breakdown and proton movement (62, 63). In summary, our findings indicated the beneficial effects of fermented wheat bran on fecal microbial composition of growing pigs.
Conclusion
In conclusion, our findings suggested that replacing 10% wheat bran with B. licheniformis fermented wheat bran could improve the growth performance, nutrient digestibility, serum antioxidant capacity, and gut microbiota composition in growing pigs. This study provides new ideas for the application of wheat bran in pig production.
Data availability statement
The original contributions presented in the study are included in the article/Supplementary material, further inquiries can be directed to the corresponding authors.
Ethics statement
The animal study was approved by Animal Care and Use Committee of Shandong Agricultural University (Approval Number: SDAUA-2017-0318). The study was conducted in accordance with the local legislation and institutional requirements.
Author contributions
HL: Data curation, Formal analysis, Methodology, Project administration, Resources, Software, Writing – original draft. XR: Methodology, Writing – review & editing, Data curation, Formal analysis, Project administration, Resources. YL: Methodology, Validation, Writing – review & editing. QC: Writing – review & editing. LiY: Formal analysis, Methodology, Validation, Writing – review & editing. SJ: Conceptualization, Investigation, Methodology, Software, Validation, Writing – review & editing. JF: Data curation, Project administration, Resources, Writing – review & editing. JG: Conceptualization, Funding acquisition, Investigation, Supervision, Writing – review & editing. LeY: Writing – review & editing. JL: Conceptualization, Investigation, Visualization, Writing – review & editing. WY: Conceptualization, Funding acquisition, Investigation, Supervision, Visualization, Writing – review & editing.
Funding
The author(s) declare financial support was received for the research, authorship, and/or publication of this article. This research was supported by the University-Industry Collaborative Education Program (KGRCYJY2021004), Natural Science Foundation of Shandong Province (ZR2022QC084), Shandong Science and Technology-Based Small and Medium-Sized Enterprises Innovation Capacity Improvement Project (grant number: 2022TSGC1275), the Shandong Province Pig Industry Technology System (SDAIT-08-04), and Major Innovative Projects in Shandong Province of research and application of environmentally friendly feed and the critical technologies for pigs and poultry without antibiotic (2019JZZY020609).
Conflict of interest
XR and JL were employed by Shandong Taishan Shengliyuan Group Co., Ltd., QC was employed by Chambroad Holding Group, and LeY was employed by Shandong New Hope Liuhe Group Co., Ltd.
The remaining authors declare that the research was conducted in the absence of any commercial or financial relationships that could be construed as a potential conflict of interest.
Publisher’s note
All claims expressed in this article are solely those of the authors and do not necessarily represent those of their affiliated organizations, or those of the publisher, the editors and the reviewers. Any product that may be evaluated in this article, or claim that may be made by its manufacturer, is not guaranteed or endorsed by the publisher.
Supplementary material
The Supplementary material for this article can be found online at: https://www.frontiersin.org/articles/10.3389/fvets.2023.1289010/full#supplementary-material
References
1. Kim, TI, Mayakrishnan, V, Lim, DH, Yeon, JH, and Baek, KS. Effect of fermented total mixed rations on the growth performance, carcass and meat quality characteristics of Hanwoo steers. Anim Sci J. (2018) 89:606–15. doi: 10.1111/asj.12958
2. Martin, NP, Russelle, MP, Powell, JM, Sniffen, CJ, Smith, SI, Tricarico, JM, et al. Invited review: sustainable forage and grain crop production for the US dairy industry. J Dairy Sci. (2017) 100:9479–94. doi: 10.3168/jds.2017-13080
3. Dai, Z, Cui, L, Li, J, Wang, B, Guo, L, Wu, Z, et al. Fermentation techniques in feed production. Anim Agric. (2020) 2020:407–29. doi: 10.1016/B978-0-12-817052-6.00024-0
4. Sugiharto, S, and Ranjitkar, S. Recent advances in fermented feeds towards improved broiler chicken performance, gastrointestinal tract microecology and immune responses: a review. Anim Nutr. (2019) 5:1–10. doi: 10.1016/j.aninu.2018.11.001
5. Yeh, RH, Hsieh, CW, and Chen, KL. Screening lactic acid bacteria to manufacture two-stage fermented feed and pelleting to investigate the feeding effect on broilers. Poult Sci. (2018) 97:236–46. doi: 10.3382/ps/pex300
6. Hao, L, Su, W, Zhang, Y, Wang, C, Xu, B, Jiang, Z, et al. Effects of supplementing with fermented mixed feed on the performance and meat quality in finishing pigs. Anim Feed Sci Technol. (2020) 266:114501. doi: 10.1016/j.anifeedsci.2020.114501
7. Liu, S, Xiao, H, Xiong, Y, Chen, J, Wu, Q, Wen, X, et al. Effects of fermented feed on the growth performance, intestinal function, and microbiota of piglets weaned at different age. Front Vet Sci. (2022) 9:841762. doi: 10.3389/fvets.2022.841762
8. van Winsen, RL, Urlings, BAP, Lipman, LJA, Snijders, JMA, Keuzenkamp, D, Verheijden Jos, HM, et al. Effect of fermented feed on the microbial population of the gastrointestinal tracts of pigs. Am Soc Microbiol. (2001) 67:3071–6. doi: 10.1128/aem.67.7.3071-3076.2001
9. Apprich, S, Tirpanalan, Ö, Hell, J, Reisinger, M, Böhmdorfer, S, Siebenhandl-Ehn, S, et al. Wheat bran-based biorefinery 2: valorization of products. LWT Food Sci Technol. (2014) 56:222–31. doi: 10.1016/j.lwt.2013.12.003
10. Mateo, AN, Hemery, YM, Bast, A, and Haenen, GR. Optimizing the bioactive potential of wheat bran by processing. Food Funct. (2012) 3:362–75. doi: 10.1039/c2fo10241b
11. Coda, R, Rizzello, CG, Curiel, JA, Poutanen, K, and Katina, K. Effect of bioprocessing and particle size on the nutritional properties of wheat bran fractions. Innovative Food Sci Emerg Technol. (2014) 25:19–27. doi: 10.1016/j.ifset.2013.11.012
12. Du, B, Meenu, M, and Xu, B. Insights into improvement of physiochemical and biological properties of dietary fibers from different sources via Micron Technology. Food Rev Int. (2019) 36:367–83. doi: 10.1080/87559129.2019.1649690
13. Zhang, M, Liao, A, Thakur, K, Huang, J, Zhang, J, and Wei, Z. Modification of wheat bran insoluble dietary fiber with carboxymethylation, complex enzymatic hydrolysis and ultrafine comminution. Food Chem. (2019) 297:124983. doi: 10.1016/j.foodchem.2019.124983
14. Kraler, M, Schedle, K, Domig, KJ, Heine, D, Michlmayr, H, and Kneifel, W. Effects of fermented and extruded wheat bran on total tract apparent digestibility of nutrients, minerals and energy in growing pigs. Anim Feed Sci Technol. (2014) 197:121–9. doi: 10.1016/j.anifeedsci.2014.07.010
15. Chu, J, Zhao, H, Lu, Z, Lu, F, Bie, X, and Zhang, C. Improved physicochemical and functional properties of dietary fiber from millet bran fermented by Bacillus natto. Food Chem. (2019) 294:79–86. doi: 10.1016/j.foodchem.2019.05.035
16. Liu, P, Zhao, J, Guo, P, Lu, W, Geng, Z, Levesque, CL, et al. Dietary corn bran fermented by Bacillus subtilis MA139 decreased gut cellulolytic bacteria and microbiota diversity in finishing pigs. Front Cell Infect Microbiol. (2017) 7:526. doi: 10.3389/fcimb.2017.00526
17. Haefner, S, Knietsch, A, Scholten, E, Braun, J, Lohscheidt, M, and Zelder, O. Biotechnological production and applications of phytases. Appl Microbiol Biotechnol. (2005) 68:588–97. doi: 10.1007/s00253-005-0005-y
18. Chen, L, Zhao, Z, Yu, W, Zheng, L, Li, L, Gu, W, et al. Nutritional quality improvement of soybean meal by Bacillus velezensis and Lactobacillus plantarum during two-stage solid-state fermentation. AMB Express. (2021) 11:23. doi: 10.1186/s13568-021-01184-x
19. Espitia-Hernández, P, Ruelas-Chacón, X, Chávez-González, ML, Ascacio-Valdés, JA, Flores-Naveda, A, and Sepúlveda-Torre, L. Solid-state fermentation of Sorghum by Aspergillus oryzae and Aspergillus niger: effects on tannin content, phenolic profile, and antioxidant activity. Foods. (2022) 11:3121. doi: 10.3390/foods11193121
20. Saleh, AA, Shukry, M, Farrag, F, Soliman, MM, and Abdel-Moneim, AME. Effect of feeding wet feed or wet feed fermented by Bacillus licheniformis on growth performance, histopathology and growth and lipid metabolism marker genes in broiler chickens. Animals. (2021) 11:83. doi: 10.3390/ani11010083
21. Hu, Y, Wang, Y, Li, A, Wang, Z, Zhang, X, Yun, T, et al. Effects of fermented rapeseed meal on antioxidant functions, serum biochemical parameters and intestinal morphology in broilers. Food Agric Immunol. (2016) 27:182–93. doi: 10.1080/09540105.2015.1079592
22. Association of Official Analytical Chemists (AOAC) . Official methods of analysis, association of official analytical chemist. 19th ed. (Washington D.C: Association of Official Analytical Chemists (AOAC)) (2012).
23. Gänzle, MG, Hertel, C, van der Vossen, JM, and Hammes, WP. Effect of bacteriocin-producing lactobacilli on the survival of Escherichia coli and Listeria in a dynamic model of the stomach and the small intestine. Int J Food Microbiol. (1999) 48:21–35. doi: 10.1016/s0168-1605(99)00025-2
24. Liu, P, Piao, X, Kim, SW, Wang, L, Shen, Y, Lee, HS, et al. Effects of chito-oligosaccharide supplementation on the growth performance, nutrient digestibility, intestinal morphology, and fecal shedding of Escherichia coli and Lactobacillus in weaning pigs. J Anim Sci. (2008) 86:2609–18. doi: 10.2527/jas.2007-0668
25. Yang, L, Zeng, X, and Qiao, S. Advances in research on solid-state fermented feed and its utilization: the pioneer of private customization for intestinal microorganisms. Anim Nutr. (2021) 7:905–16. doi: 10.1016/j.aninu.2021.06.002
26. Varsha, KK, Priya, S, Devendra, L, and Nampoothiri, KM. Control of spoilage fungi by protective lactic acid bacteria displaying probiotic properties. Appl Biochem Biotechnol. (2014) 172:3402–13. doi: 10.1007/s12010-014-0779-4
27. Shi, C, Zhang, Y, Lu, Z, and Wang, Y. Solid-state fermentation of corn-soybean meal mixed feed with Bacillus subtilis and Enterococcus faecium for degrading antinutritional factors and enhancing nutritional value. J Anim Sci Biotechnol. (2017) 8:50. doi: 10.1186/s40104-017-0184-2
28. Liu, Y, Feng, J, Wang, Y, Lv, J, Li, J, Guo, L, et al. Fermented corn–soybean meal mixed feed modulates intestinal morphology, barrier functions and cecal microbiota in laying hens. Animals. (2021) 11:3059. doi: 10.3390/ani11113059
29. He, W, Gao, Y, Guo, Z, Yang, Z, Wang, X, Liu, H, et al. Effects of fermented wheat bran and yeast culture on growth performance, immunity, and intestinal microflora in growing-finishing pigs. J Anim Sci. (2021) 99:skab308. doi: 10.1093/jas/skab308
30. Zhao, L, Zhang, X, Cao, F, Sun, D, Wang, T, and Wang, G. Effect of dietary supplementation with fermented Ginkgo-leaves on performance, egg quality, lipid metabolism and egg-yolk fatty acids composition in laying hens. Livest Sci. (2013) 155:77–85. doi: 10.1016/j.livsci.2013.03.024
31. He, W, Yang, Z, Ding, H, and Shi, B. Dietary supplementation with fermented wheat bran improves the inflammatory state and intestinal health in pigs. Livest Sci. (2023) 274:105286. doi: 10.1016/j.livsci.2023.105286
32. Le, MHA, Galle, S, Yang, Y, Landero, JL, Beltranena, E, Gänzle, MG, et al. Effects of feeding fermented wheat with Lactobacillus reuteri on gut morphology, intestinal fermentation, nutrient digestibility, and growth performance in weaned pigs. J Anim Sci. (2016) 94:4677–87. doi: 10.2527/jas.2016-0693
33. Torres-Pitarch, A, Gardiner, GE, Cormican, P, Mary, R, Fiona, C, Doherty, JV, et al. Effect of cereal fermentation and carbohydrase supplementation on growth, nutrient digestibility and intestinal microbiota in liquid-fed grow-finishing pigs. Sci Rep. (2020) 10:13716. doi: 10.1038/s41598-020-70443-x
34. Yuan, L, Chang, J, Yin, Q, Lu, M, Di, Y, Wang, P, et al. Fermented soybean meal improves the growth performance, nutrient digestibility, and microbial flora in piglets. Anim Nutr. (2017) 3:19–24. doi: 10.1016/j.aninu.2016.11.003
35. Zhao, H, Guo, X, and Zhu, K. Impact of solid state fermentation on nutritional, physical and flavor properties of wheat bran. Food Chem. (2017) 217:28–36. doi: 10.1016/j.foodchem.2016.08.062
36. Feng, Y, Wang, L, Khan, A, Zhao, R, Wei, S, and Jing, X. Fermented wheat bran by xylanase-producing Bacillus cereus boosts the intestinal microflora of broiler chickens. Poult Sci. (2020) 99:263–71. doi: 10.3382/ps/pez482
37. Deng, B, Wu, J, Li, X, Zhang, C, Men, X, and Xu, Z. Effects of Bacillus subtilis on growth performance, serum parameters, digestive enzyme, intestinal morphology, and colonic microbiota in piglets. AMB Express. (2020) 10:212–09. doi: 10.1186/s13568-020-01150-z
38. Cruz, A, Håkenåsen, IM, Skugor, A, Mydland, LT, Åkesson, CP, Hellestveit, SS, et al. Candida utilis yeast as a protein source for weaned piglets: effects on growth performance and digestive function. Livest Sci. (2019) 226:31–9. doi: 10.1016/j.livsci.2019.06.003
39. Huyut, MT, and Ilkbahar, F. The effectiveness of blood routine parameters and some biomarkers as a potential diagnostic tool in the diagnosis and prognosis of COVID-19 disease. Int Immunopharmacol. (2021) 98:107838. doi: 10.1016/j.intimp.2021.107838
40. Soltan, MA . Potential of using raw and processed canola seed meal as an alternative fish meal protein source in diets for Nile tilapia, (Oreochromis niloticus). Nutr Feeds. (2005) 8:1111–28.
41. Baye, K, Guyot, JP, and Mouquet-Rivier, C. The unresolved role of dietary fibers on mineral absorption. Crit Rev Food Sci Nutr. (2017) 57:949–57. doi: 10.1080/10408398.2014.953030
42. He, L, Li, H, Huang, N, Zhou, X, Tian, J, Li, T, et al. Alpha-ketoglutarate suppresses the NF-κB-mediated inflammatory pathway and enhances the PXR-regulated detoxification pathway. Oncotarget. (2017) 8:102974:–102988. doi: 10.18632/oncotarget.16875
43. Xu, X, Li, L, Li, B, Guo, W, Ding, X, and Xu, F. Effect of fermented biogas residue on growth performance, serum biochemical parameters, and meat quality in pigs. Asian Australas J Anim Sci. (2017) 30:1464–70. doi: 10.5713/ajas.16.0777
44. Fernandez, NJ, and Kidney, BA. Alkaline phosphatase: beyond the liver. Vet Clin Pathol. (2007) 36:223–33. doi: 10.1111/j.1939-165x.2007.tb00216.x
45. Wlazło, Ł, Nowakowicz-Dębek, B, Ossowski, M, Łukaszewicz, M, and Czech, A. Effect of fermented rapeseed meal in diets for piglets on blood biochemical parameters and the microbial composition of the feed and faeces. Animals. (2022) 12:2972. doi: 10.3390/ani12212972
46. Gobi, N, Vaseeharan, B, Chen, J, Rekha, R, Vijayakumar, S, Anjugam, M, et al. Dietary supplementation of probiotic Bacillus licheniformis Dahb 1 improves growth performance, mucus and serum immune parameters, antioxidant enzyme activity as well as resistance against Aeromonas hydrophila in tilapia Oreochromis mossambicus. Fish Shellfish Immunol. (2018) 74:501–8. doi: 10.1016/j.fsi.2017.12.066
47. Bosco, R, Leeuwenburgh, SCG, Jansen, JA, and van den Beucken, JJJP. Configurational effects of collagen/ALP coatings on enzyme immobilization and surface mineralization. Appl Surf Sci. (2014) 311:292–9. doi: 10.1016/j.apsusc.2014.05.057
48. Yang, Z, Wang, Z, Yang, H, Xu, L, and Gong, D. Effects of dietary methionine and betaine on slaughter performance, biochemical and enzymatic parameters in goose liver and hepatic composition. Anim Feed Sci Technol. (2017) 228:48–58. doi: 10.1016/j.anifeedsci.2017.04.003
49. Su, Y, Chen, G, Chen, L, Li, J, Wang, G, He, J, et al. Effects of antimicrobial peptides on serum biochemical parameters, antioxidant activity and non-specific immune responses in Epinephelus coioides. Fish Shellfish Immunol. (2019) 86:1081–7. doi: 10.1016/j.fsi.2018.12.056
50. Walkowiak, J, Mądry, E, Lisowska, A, Szaflarska-Popławska, A, Grzymisławski, M, Stankowiak-Kulpa, H, et al. Adaptive changes of pancreatic protease secretion to a short-term vegan diet: influence of reduced intake and modification of protein. Br J Nutr. (2012) 107:272–6. doi: 10.1017/s0007114511002923
51. Chang, PL, Boyd, RD, Zier-Rush, C, Rosero, DS, and van Heugten, E. Lipid peroxidation impairs growth and viability of nursery pigs reared under commercial conditions. J Anim Sci. (2019) 97:3379–89. doi: 10.1093/jas/skz183
52. Yonny, ME, García, EM, López, A, Arroquy, JI, and Nazareno, MA. Measurement of malondialdehyde as oxidative stress biomarker in goat plasma by HPLC-DAD. Microchem J. (2016) 129:281–5. doi: 10.1016/j.microc.2016.07.010
53. Savas, HA, Gergerlioglu, HS, Armutcu, F, Herken, H, Yilmaz, HR, Kocoglu, E, et al. Elevated serum nitric oxide and superoxide dismutase in euthymic bipolar patients: impact of past episodes. World J Biol Psych. (2006) 7:51–5. doi: 10.1080/15622970510029993
54. Xu, J, Su, X, Burley, SK, and Zheng, XFS. Nuclear SOD1 in growth control, oxidative stress response, amyotrophic lateral sclerosis, and cancer. Antioxidants. (2022) 11:427. doi: 10.3390/antiox11020427
55. Kumari, R, Sharma, N, Sharma, S, Samurailatpam, S, Padhi, S, Singh, SP, et al. Production and characterization of bioactive peptides in fermented soybean meal produced using proteolytic Bacillus species isolated from kinema. Food Chem. (2023) 421:136130. doi: 10.1016/j.foodchem.2023.136130
56. Patterson, JA, and Burkholder, KM. Application of prebiotics and probiotics in poultry production. Poult Sci. (2003) 82:627–31. doi: 10.1093/ps/82.4.627
57. von Martels, JZH, Sadaghian, SM, Bourgonje, AR, Blokzijl, T, Dijkstra, G, Faber, KN, et al. The role of gut microbiota in health and disease: in vitro modeling of host-microbe interactions at the aerobe-anaerobe interphase of the human gut. Anaerobe. (2017) 44:3–12. doi: 10.1016/j.anaerobe.2017.01.001
58. Wang, J, Yoo, JS, Kim, HJ, Lee, JH, and Kim, IH. Nutrient digestibility, blood profiles and fecal microbiota are influenced by chitooligosaccharide supplementation of growing pigs. Livest Sci. (2009) 125:298–303. doi: 10.1016/j.livsci.2009.05.011
59. Eun, CS, Kim, YS, Han, D, Choi, JH, Lee, AR, and Park, YK. Lactobacillus casei prevents impaired barrier function in intestinal epithelial cells. J Apmis. (2011) 119:49–56. doi: 10.1111/j.1600-0463.2010.02691.x
60. Jin, W, Ye, H, Zhao, J, Zhou, Z, and Huan, F. Consuming fermented distillers' dried grains with solubles (DDGS) feed reveals a shift in the faecal microbiota of growing and fattening pigs using 454 pyrosequencing. J Integr Agric. (2017) 16:900–10. doi: 10.1016/s2095-3119(16)61523-x
61. Vadopalas, L, Ruzauskas, M, Lele, V, Starkute, V, Zavistanaviciute, P, Zokaityte, E, et al. Pigs’ feed fermentation model with antimicrobial lactic acid bacteria strains combination by changing extruded soya to biomodified local feed stock. Animals. (2020) 10:783. doi: 10.3390/ani10050783
62. Engberg, RM, Hammersh, JM, Johansen, NF, Abousekken, MS, Steenfeldt, S, and Jensen, BB. Fermented feed for laying hens: effects on egg production, egg quality, plumage condition and composition and activity of the intestinal microflora. Br Poult Sci. (2009) 50:228–39. doi: 10.1080/00071660902736722
Keywords: fermented wheat bran, growing pig, growth performance, antioxidant capacity, fecal microflora
Citation: Liu H, Ren X, Li Y, Cao Q, Yang L, Jiang S, Fu J, Gao J, Yan L, Li J and Yang W (2023) Effects of different wheat bran fermentation sources on growth performance, nutrient digestibility, serum antioxidant capacity and fecal microbiota in growing pigs. Front. Vet. Sci. 10:1289010. doi: 10.3389/fvets.2023.1289010
Edited by:
Tao Wang, Jilin Agricultural University, ChinaReviewed by:
Hongbin Pan, Yunnan Agricultural University, ChinaMohammed Hamdy Farouk, Al-Azhar University, Egypt
Copyright © 2023 Liu, Ren, Li, Cao, Yang, Jiang, Fu, Gao, Yan, Li and Yang. This is an open-access article distributed under the terms of the Creative Commons Attribution License (CC BY). The use, distribution or reproduction in other forums is permitted, provided the original author(s) and the copyright owner(s) are credited and that the original publication in this journal is cited, in accordance with accepted academic practice. No use, distribution or reproduction is permitted which does not comply with these terms.
*Correspondence: Weiren Yang, d3J5YW5nQHNkYXUuZWR1LmNu; Junxun Li, ODkyNjg2OTIzQHFxLmNvbQ==