- 1College of Animal Science and Technology, Zhongkai University of Agriculture and Engineering, Guangzhou, Guangdong, China
- 2Innovative Institute of Animal Healthy Breeding, Zhongkai University of Agriculture and Engineering, Guangzhou, Guangdong, China
- 3College of Life Sciences, Jiaying University, Meizhou, China
- 4State Key Laboratory for Managing Biotic and Chemical Threats to the Quality and Safety of Agro-Products, Institute of Animal Husbandry and Veterinary Science, Zhejiang Academy of Agricultural Sciences, Hangzhou, China
Introduction: The aim of this study was to evaluate the effects of fermented feed on growth performance, antioxidant indexes and intestinal health in lion-head goslings.
Methods: 288 male lion-head goslings (one-day-old) were randomly divided into four groups (6 replicates per group, 12 samples per replicate): control group (basal diet) and fermented feed (FF) groups (basal diet supplemented with 2.5, 5.0 and 7.5% FF, respectively). The experimental period lasted 28 days.
Results: The results showed that 5.0 and 7.5% FF groups decreased feed conversion rate (FCR) when compared with the control group (p < 0.05). The 5.0% FF group reduced the activity of alkaline phosphatase (ALP) and lactate dehydrogenase (LDH) in serum; while the 7.5% FF group decreased the concentration of total cholesterol (TC), ALP and LDH activity (p < 0.05). Furthermore, the 7.5% FF group significantly increased total antioxidant capacity (T-AOC) in serum (p < 0.05); 2.5% and 5.0% FF groups significantly increased glutathione peroxidase (GSH-Px) in serum (p < 0.05); all FF groups increased the activity of superoxide dismutase (T-SOD) in serum (p < 0.05). For intestinal health, the villous height and villi/crypt ratio in jejunum were increased in all FF groups, but crypt depth was decreased (p < 0.05); The 5.0% FF groups enhanced T-AOC activity in jejunum (p < 0.05); The 2.5% and 5.0% FF groups enhanced GSH-Px activity (p < 0.05) in jejunum; All FF groups reduced malondialdehyde (MDA) level in jejunum (p < 0.05). LEfSe analysis showed that the cecum microbiota was significantly dominant in the 2.5% FF group compared to the control group including Firmicutes, Lactobacillales, Lactobacillus, and Prevotella; the flora that were significantly dominant in the 5.0% FF group compared to the control group included Bacteroidaceae, Bacteroides, Megamonas, and Prevotella; and the groups that were significantly dominant in the 7.5% FF group compared to the control group included Bacteroidota, Bacteroides, Bacteroidaceae, and Ruminococcaceae.
Discussion: In summary, dietary FF supplementation improved growth performance, serum biochemical parameters and antioxidant capacity of lion-head goslings, as well as improved jejunal tissue morphology and optimized intestinal flora structure. In particular, the FF addition at a dose of 7.5% was relatively more effective for lion- head goslings.
1. Introduction
With the rapid development of large-scale and intensive livestock farming, livestock diseases have become increasingly complex and diverse and livestock intestinal health problems have become increasingly prominent. The healthy development of animal husbandry has thus necessitated a search for alternative safe, efficient, and reasonable “green feed.” Corn and soybean meal (SBM) are widely used in animal feeds due to their high nutritional value and digestibility of organic matter. However, it has been found that corn and soybean meal contain various antinutritional factors including phytic acid, trypsin inhibitors, and soybean antigenic proteins. These substances have been shown to reduce animal growth performance and health by reducing the efficiency of diets, decreasing the digestibility and absorption rate of the feed, and by disrupting the gut microbiota of animals (1, 2). To this end, fermented feed (FF), an important component of the feed industry, has emerged as an effective strategy to reduce or replace the use of antibiotics in animal feed (3). Indeed, beneficial microorganisms in FF break down or convert macromolecules, antinutritional factors (ANFs), and toxins in the feed into substances that can be easily digested and absorbed by animals (1, 4); thus increasing the nutritional value of the feed and improving its palatability. Gut health is critical to the growth performance and health of poultry. The gut microbiota is the community of microorganisms that colonize the gut of the host organ. Microorganisms colonize the gut through a very complex process of interaction between the microorganisms and the host, which provides a site for microbial survival and evolution (5). Gut microbes are an integral part of the gastrointestinal tract and play important roles in nutrition, physiology and gut morphology. Moreover, the gut microbiota is involved in the host’s immune defence mechanisms against pathogens (6). Beneficial microorganisms in fermented feeds, such as lactic acid bacteria, reduce gut pH by producing organic acids and inhibit colonization of gut pathogens through antagonistic activity, competitive exclusion and bacteriocin production (7). Numerous Studies have shown that the use of FF in poultry production can improve growth performance, antioxidant function, regulate serum biochemical indexes, improve immunity, and maintain intestinal microecological balance (8–10). The use of FF can also reduce the cost of feeding and improve overall economic efficiency. Additionally, due to the wide range of raw materials contained in FF its use has the potential to reduce the waste of resources and environmental pollution associated with traditional feeds (11). Therefore, FF has broad application prospects in livestock and poultry production.
As herbivorous waterfowl, geese have a greater advantage than other poultry species in digesting and utilizing dietary fiber, tolerating roughage and utilizing straw and silage to partially replace concentrates in the goose diet, thus reducing costs in production. The microflora in the gut of the lion- head goose breaks down cellulose, with the final reaction producing volatile fatty acids, ammonia and carbon dioxide (12–14). Furthermore, the lion-head goose, the only large goose species in China, native to Rao Ping County in Chaozhou City, Guangdong Province, is a protected agricultural resource. These geese are characterized by their large size and fast growth rate (15), Lion-head goose meat is of excellent quality, it is rich in essential amino acids needed for human growth and development, and rich in monounsaturated fatty acids, linolenic acid and arachidonic acid, and contains more linolenic and linoleic acid than chicken, Popular with consumers (16). Popular with consumers. However, at present, the entire lion head goose breeding is still in the initial stage, especially in the lion-head goose brood stage, there are many problems, due to the incomplete development of the intestinal tract of the goslings, poor environmental factors and inappropriate feeding and management conditions, such as the temperature, humidity and other stresses, so that the lion-head goslings intestinal health and other problems are becoming increasingly prominent. Serious constraints on the feeding management of lionhead geese in the brood stage, affect the economic benefits of lionhead goose breeding. At present, there have been many studies on the application of fermented feed to chickens, ducks and other poultry. To date, the application of FF in the breeding of lion-head geese has rarely been reported. Therefore, in this study, 1 to 28-day-old lion-head goslings were fed different doses of FF to assess changes to growth performance, serum biochemical parameters, antioxidant capacity and intestinal health compared to a basic diet. The results of this study may provide a theoretical basis for the application of FF in lion-head goose breeding.
2. Materials and methods
2.1. Fermented feed preparation
The FF was developed in-house. The fermentation process was as follows: fermentation substrate (50% bran, 40% ultrapure water, 5% corn flour, 3% molasses) was mixed with liquid fermented Lactobacillus plantarum (1 × 109 cfu/mL, 2%), placed in fermentation bags, and fermented at 37°C for 7 days. The final product has an even yellow-brown color, is weakly acidic (pH = 4.1), and has a soft texture. The nutritional composition of the FF was determined as follows: 45.05% moisture, 7.40% crude protein, 2.07% crude fat, 3.52% crude fiber, 2.48% crude ash, 0.05% calcium, and 0.48% total phosphorus.
2.2. Experimental design and diet
The goslings were provided by “Rao Ping Lion-headed Goose Science and Technology Institute, Chaozhou City, Guangdong Province”. In the experiment, 288 1-day-old healthy male lion-head goslings with similar body weights were selected and randomly divided, under the experimental design (one-way ANOVA), into 4 groups with 6 replicates per group and 12 animals per replicate. FF was added at a concentration of 0 (the control group), 2.5, 5.0, and 7.5% into the basic diet of the 4 groups respectively; the experimental period was set to 28 d. The basic diet used in the experiment was formulated in accordance with the Nutrient Requirements of Poultry (NRC) (1994) and that of the lion-head goose production industry in Guangdong Province. The composition and nutritional levels of the feed are listed in Table 1.
2.3. Feeding procedure
The brood was reared in two phases consisting of: indoors in a net bed on days 1–14, and outdoors on the ground on days 15–28. All goslings were given food and water ad libitum throughout the experiment. During the 28 days, immunization, sanitation, and disinfection procedures were carried out according to actual farm production standards. Welfare monitoring was performed daily to ensure the health status of the geese was maintained.
2.4. Sample collection and index determination
2.4.1. Growth performance
Body weights (BW) of goslings were determined at the beginning and end of the trial. Feed intake was measured for each replicate, and average daily gain (ADG), average daily feed intake (ADFI), and feed conversion rate (FCR) were calculated for each replicate group at the end of the experiment.
2.4.2. Serum biochemical indexes and antioxidant capacity
At the end of 28 days, all goslings were fasted for 12 h. Two goslings of similar body weight were randomly selected from each replicate for blood collection. 5 mL of blood was collected from the sub wing vein and placed at an angle until the serum was precipitated; subsequently, the blood was centrifuged at 3000 r/min for 15 min to separate the serum which was stored at −20°C for the determination of serum biochemical indexes and antioxidant indexes.
The kits were purchased from Maccura Biotechnology Co., Ltd. Total protein (TP) was determined by the bis-urea method (17), albumin (ALB) was determined by the bromocresol green method (18), triglyceride (TG) was determined by the GPO-PAP method (19), total cholesterol (TC) was determined by the CHOD-PAP method (19), alanine aminotransferase was measured using (ALT) by the alanine substrate method (20), and aspartate aminotransferase (AST) by the aspartate substrate method (20), alkaline phosphatase (ALP) was measured by NPP substrate-AMP buffer method (21), blood glucose (GLU) was measured by glucokinase method (22) and lactate dehydrogenase (LDH) enzyme was measured by lactate substrate method (23).
The kits were purchased from Nanjing Jiancheng Institute of Bioengineering. Serum total antioxidant capacity (T-AOC) was determined by ABTS rapid method, serum total superoxide dismutase (T-SOD) was determined by hydroxytoluidine method, serum glutathione peroxidase (GSH-PX) activity was determined by colourimetric method, and serum malondialdehyde (MDA) content was determined by TBA method (24, 25).
2.4.3. Measurement and observation of intestinal morphological indicators
After the goslings were euthanized, a 2 cm section of the mid jejunum was taken; the contents of the intestine were then rinsed with pre-chilled phosphate buffer solution (PBS) and the samples were quickly immersed in 10% formalin and fixed. The effects of FF levels on the morphological and structural development of jejunal villi was assessed using paraffin sections, with photographs taken under by light microscopy. NDP.view2 image viewing software (Hamamatsu Photonics K.K., Japan) was used to measure villous height and crypt depth in the jejunum and to calculate the villi/crypt ratio.
2.4.4. Intestinal antioxidant capacity
After the goslings were euthanized, jejunum tissues were taken and stored at −80°C. The kits were purchased from Nanjing Jiancheng Institute of Bioengineering. The total antioxidant capacity (T-AOC) of jejunum was determined by ABTS rapid method, the total superoxide dismutase (T-SOD) of jejunum was determined by hydroxytoluidine method, the activity of jejunum glutathione peroxidase (GSH-PX) was determined by colourimetric assay, and the content of jejunum malondialdehyde (MDA) was determined by TBA method (24, 25).
2.4.5. Gut microbiota amplicon sequencing analysis
Post-mortem cecum segment contents were collected under sterile conditions, transferred into sterile tubes and snap frozen in liquid nitrogen prior to storage at −80°C. All samples were sent to Harbin Botai Biotechnology Co., Ltd. For DNA extraction and the quality of DNA samples was checked using a UV spectrophotometer. The target fragments of 16S rRNA V3 ~ V4 were amplified by PCR with primers 338F (5′-barcode+ACTCCTACGGGAGGCAGCA-3′) and 806R (5’-GGACTACHVGGGTWTCTAAT-3′). PCR amplification was performed using the NEB Q5 DNA high-fidelity polymerase reaction system. Libraries were built using an Illumina TruSeq Nano DNA LT Library Prep Kit, and 2 × 250 bp double-end sequencing was performed on an Illumina NovaSeq machine using NovaSeq 6,000 SP Reagent Kit (500 cycles) for qualified libraries.
2.4.6. Bioinformatics analysis and data processing
The valid data were clustered into operational taxonomic units (OTUs) at a 97% similarity level using uclust (v1.2.22q) method to obtain the total number of OTUs and out relative abundance. Alpha diversity analysis (Chao1 index, observed species index, Shannon index, and Simpson index) were conducted using QIIME (v1.9.1) and the community composition of each sample was calculated according to the taxonomic level of kingdom, phylum, order, family, genus and species. Principal component analysis (PCA) was performed using R (v3.2.3) to determine the structural similarity of colonies between samples. Linear discriminant analysis effect size (LEfSe) method was used to detect taxonomic units with rich differences between groups (LDA score > 3.0).
2.5. Statistical analysis
One-Way ANOVA was employed for data analysis using SPSS 22.0. All groups were compared with each other using Tukey’s multiple comparison test. Significant differences were determined where p < 0.05 was considered significant and results were expressed as the mean and SEM.
3. Results
3.1. Effects of fermented feed on growth performance of lion-head goslings
As shown in Table 2, the FCR of 5.0 and 7.5% FF groups decreased significantly compared with the control group (p < 0.05), while final weight, ADG and ADFI of the FF groups did not change significantly compared with the control group (p > 0.05).
3.2. Effects of fermented feed on serum biochemical indexes of lion-head goslings
According to Table 3, compared with the control group, 5.0 and 7.5% FF groups significantly decreased the activity of ALP (p < 0.05) in serum; the 7.5% FF group reduced the concentration of TC (p < 0.05) Moreover, 5.0 and 7.5% FF groups decreased LDH activity (p < 0.05). However, FF treatment had no significant on the levels of TP, ALB, GLO, TG, ALT, AST and GLU in serum (p > 0.05).
3.3. Effects of fermented feed on serum antioxidant function of lion-head goslings
According to Figure 1, compared with the control group, serum T-AOC activity were significantly higher in the 7.5% FF group (p < 0.05); while the serum T-SOD activity were significantly increased in all FF groups (p < 0.05). Moreover, the 2.5 and 5.0% FF groups increased the activity of GSH-Px (p < 0.05). However, dietary FF supplementation had no significant on the level of MDA (p > 0.05).
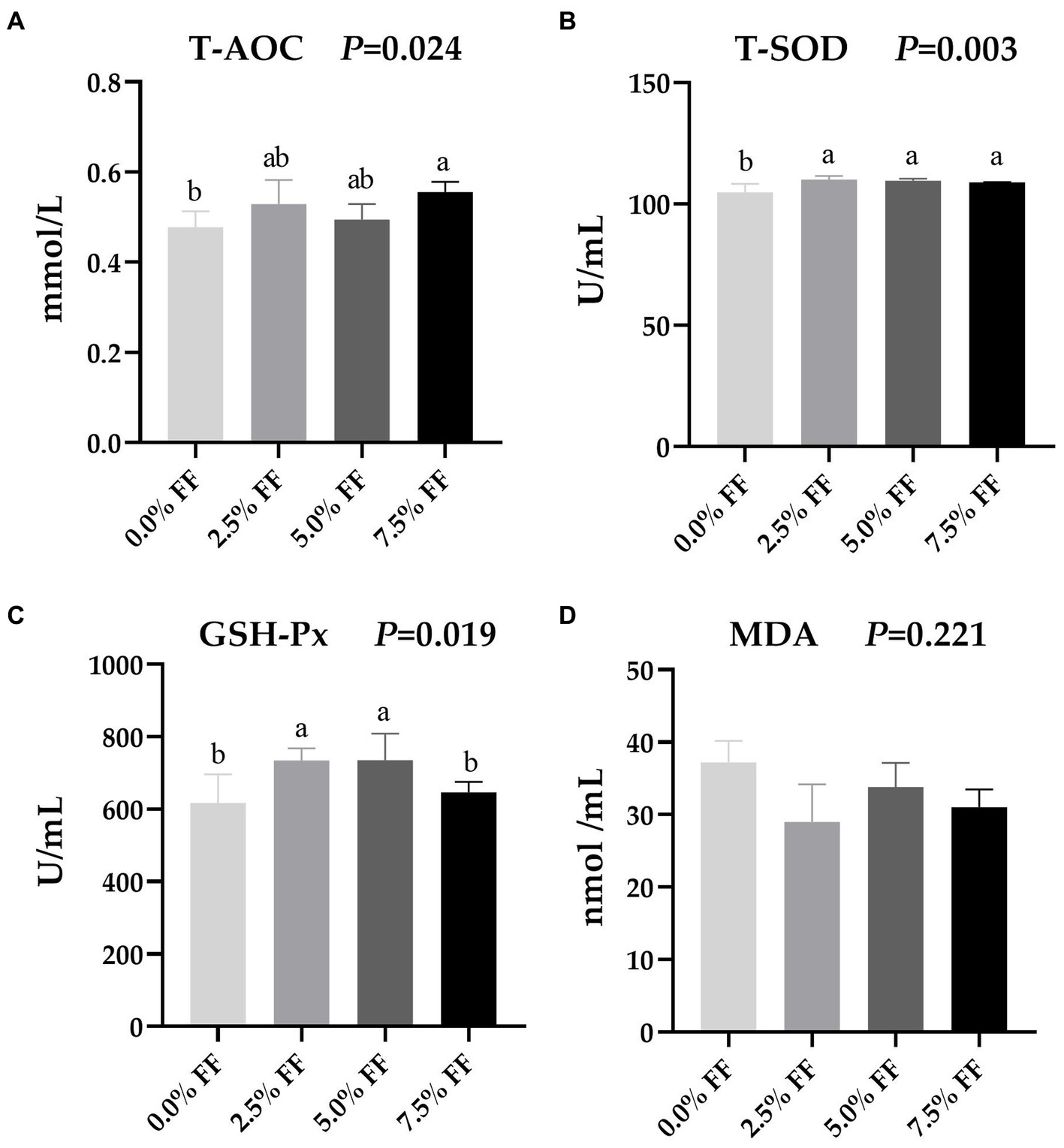
Figure 1. Effects of fermented feed on serum antioxidant function of lion-head goslings. (A–D) Refer to activity of serum T-AOC, activity of serum T-SOD, activity of serum GSH-Px and serum level of MDA, respectively. a,b,c Values with superscripts of different letters in the same row were significantly different (p < 0.05), whereas values with the same or no superscripts showed no differences (p ≥ 0.05).
3.4. Effects of fermented feed on intestine morphology and development of lion-head goslings
As shown in Figure 2, compared with the control group, both the villous height and the villi/crypt ratio in jejunum were increased in all FF groups (p < 0.05), where crypt depth decreased significantly with the increase of the FF ratio (p < 0.05). Herein, there was no significant difference in jejunum villus height between the 2.5 and 5.0% FF groups (p > 0.05). These data reveal that FF has a dose-dependent effect on villi height, crypt depth, and villi/crypt ratio.
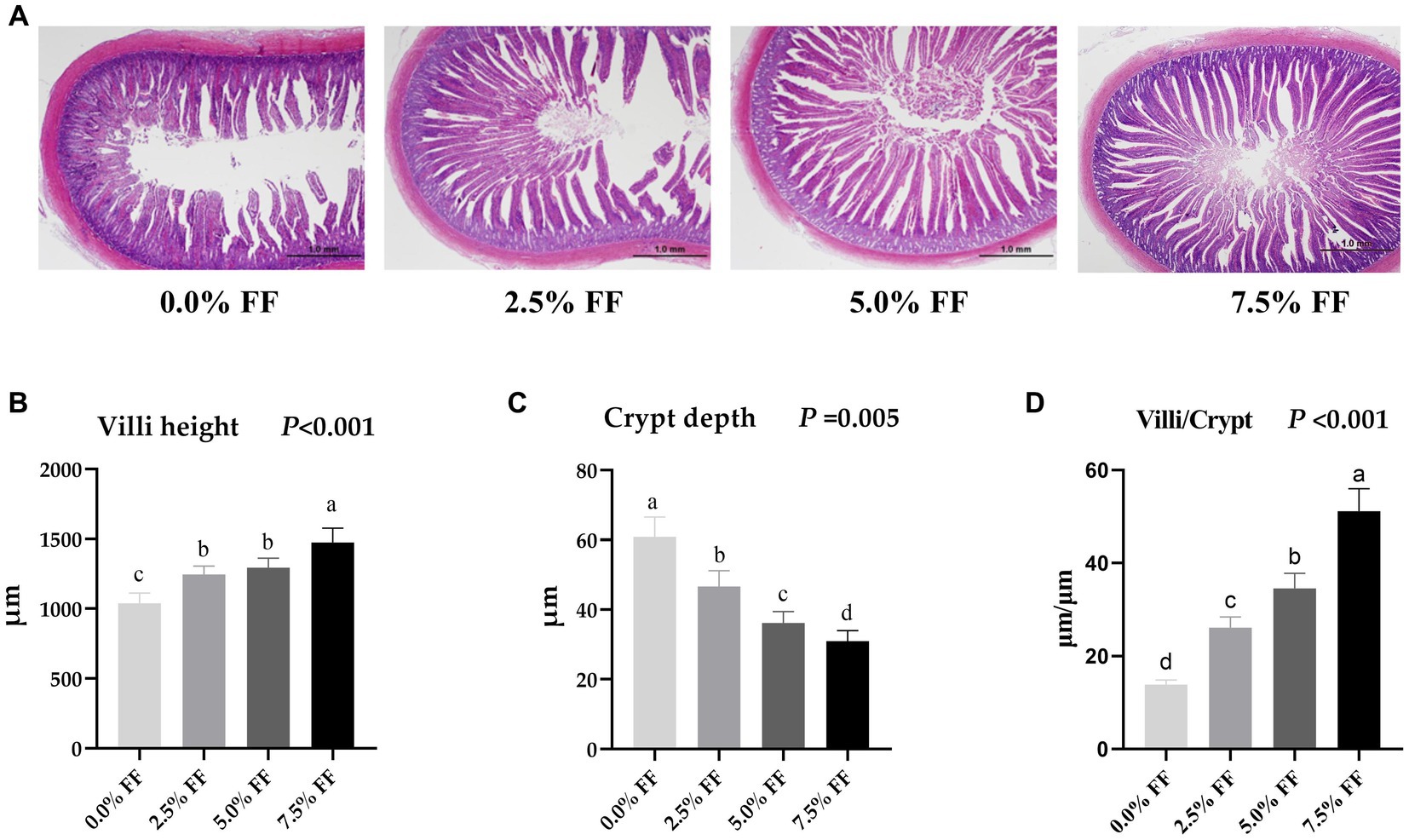
Figure 2. Effects of fermented feed on intestine morphology development of lion-head goslings. (A) Measurement of villous height and crypt depth in jejunum; scale length is 1.0 mm. (B–D) Refer to villous height, crypt depth and villi/crypt in the jejunum, respectively. a,b,c,d Row means with different superscripts are significantly different (p < 0.05).
3.5. Effects of fermented feed on intestinal antioxidant function in lion-head goslings
According to Figure 3, compared with the control group, the 5.0% FF group significantly increased T-AOC activity in the jejunum (p < 0.05). Meanwhile, GSH-Px activity in the 2.5 and 5.0% FF groups was significantly increased (p < 0.05). However, there was no significant difference between the 5.0 and 7.5% FF groups (p > 0.05). Additionally, compared to the control group, MDA levels decreased in all FF groups (p < 0.05). There was no significant effect of the treatment groups on T-SOD activity (p > 0.05).
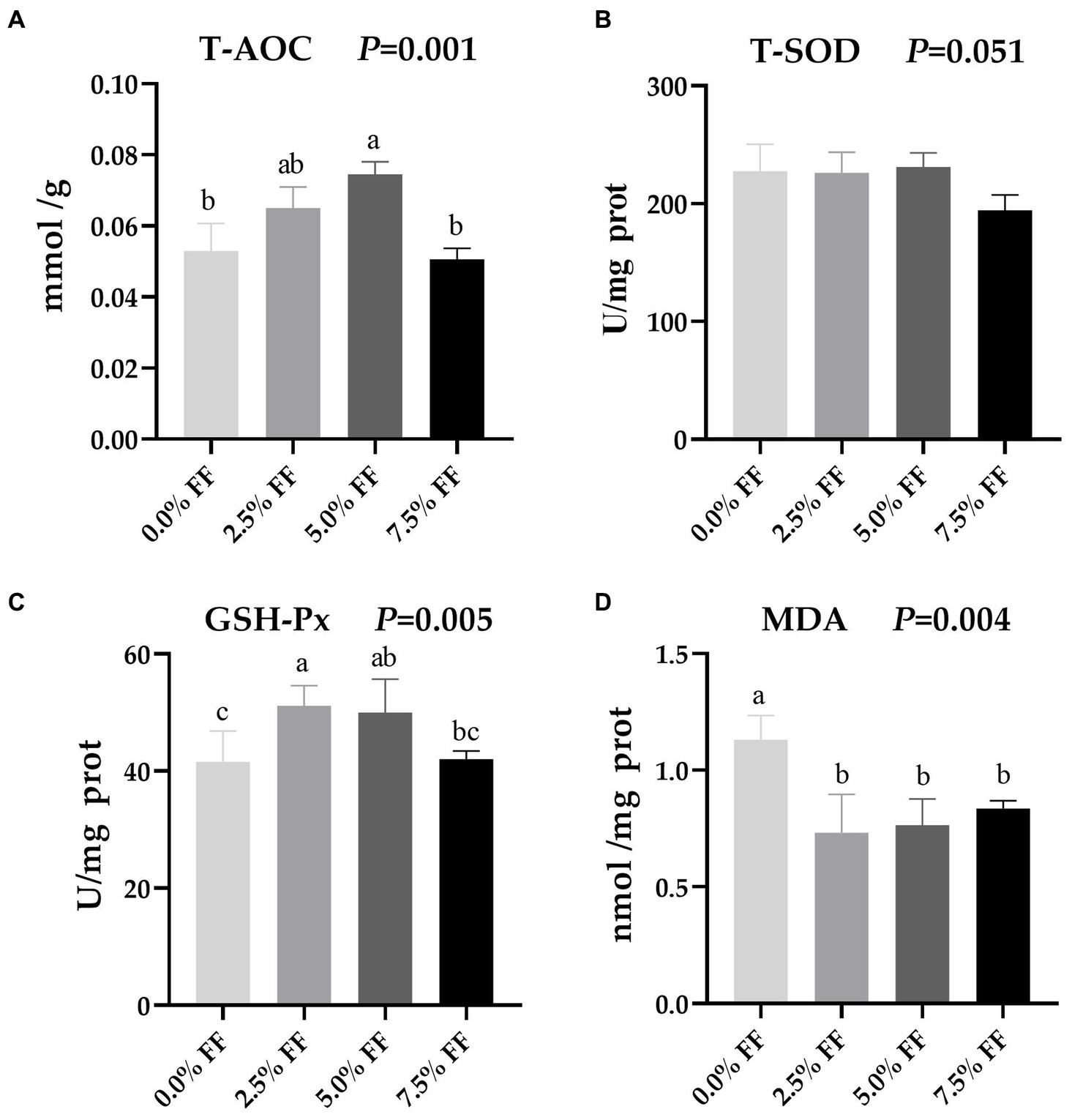
Figure 3. Effects of fermented feed on intestinal antioxidant function of lion-head goslings. (A–D) Refer to activity of T-AOC, activity of T-SOD, activity of GSH-Px and level of MDA in jejunum, respectively. a,b,c Values with superscripts of different letters in the same row were significantly different (p < 0.05), whereas values with the same or no superscripts showed no differences (p ≥ 0.05).
3.6. Effects of fermented feed on gut microbiota composition and diversity in lion-head goslings
Chao1, observed species, Shannon index, and Simpson index were used to characterize the alpha diversity of microorganisms in each group of samples. According to Figure 4A, compared with the control group, the 5.0% FF group significantly decreased (p < 0.05) chao1, observed species, and Shannon index of cecal microbiota, while the Simpson indexes of all groups were not significantly different (p > 0.05). The Venn diagram shows a total of 365 OTUs among the four groups, with 1,135, 1,024, 867 and 1,153 OTUs for each group in order (Figure 4B). The PCA plots showed a significant separation in microbial composition between the treatment groups (Figure 4C).
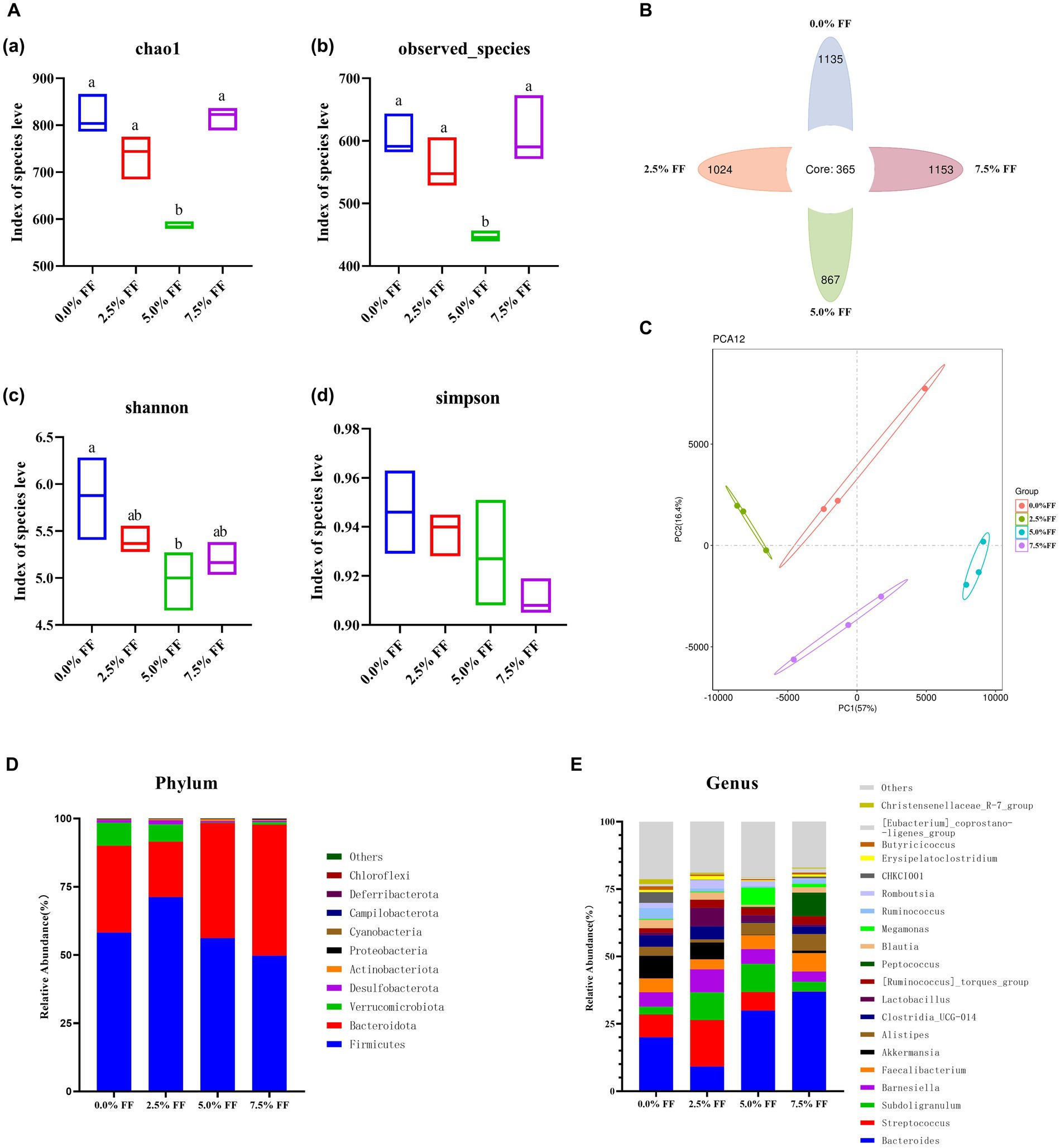
Figure 4. Effects of fermented feed on microbial diversity of lion-head goslings. (A) Alpha diversity analysis. (B) Venn diagram. (C) PCA results. (D) Relative abundance of the top 10 phylum-level groups in each test group. (E) Relative abundance of the top 20 genus-level groups in each test group.
At the phylum level, the dominant phyla in the cecum contents from the control and FF groups were Firmicutes, Bacteroidota, and Verrucomicrobiota; with Firmicutes and Bacteroidota accounting for more than 85% of the total population. The relative abundance of cecum contents microbiota increased, whereas the relative abundance of Verrucomicrobiota decreased in the 5.0 and 7.5% FF groups compared to the control group (Figure 4D). At the genus level, the dominant genera of cecum contents in the control and all FF groups were Bacteroides, Streptococcus and Subdoligranulum. Compared to the control group, the relative abundance of Bacteroides was increased in the 5.0 and 7.5% FF groups, while the relative abundance of Lactobacillus was increased in the 2.5 and 5.0% FF groups. Moreover, the relative abundances of Megamonas and Peptococcus were increased in the 5.0 and 7.5% FF groups, respectively (Figure 4E).
For the results of LEfSe analysis, when compared to the control group, the significant dominant microflora in the cecum contents of the 2.5% FF group at all levels included Firmicutes, Lactobacillales, Lactobacillus, and Prevotella; while the significant dominant microflora in the control group at all levels included Rikenella, Rikenellaceae, and Alistipes (Figure 5A). Compared to the control group, the significantly dominant microflora in cecum contents from 5.0% FF group at all levels included Bacteroidaceae, Bacteroides, Megamonas, and Prevotella. The significantly dominant microflora in the control group at all levels included Rikenella, Rikenellaceae_RC9_gut_group, and Erysipelatoclostridiaceae (Figure 5B). The significantly dominant microflora in cecum contents from the 7.5% FF group at all levels included Bacteroidota, Bacteroides, Bacteroidaceae, and Ruminococcaceae, while the significantly dominant microflora in the control group at all levels included Enterococcus, Erysipelatoclostridiaceae, Desulfovibrio, and Streptococcus (Figure 5C).
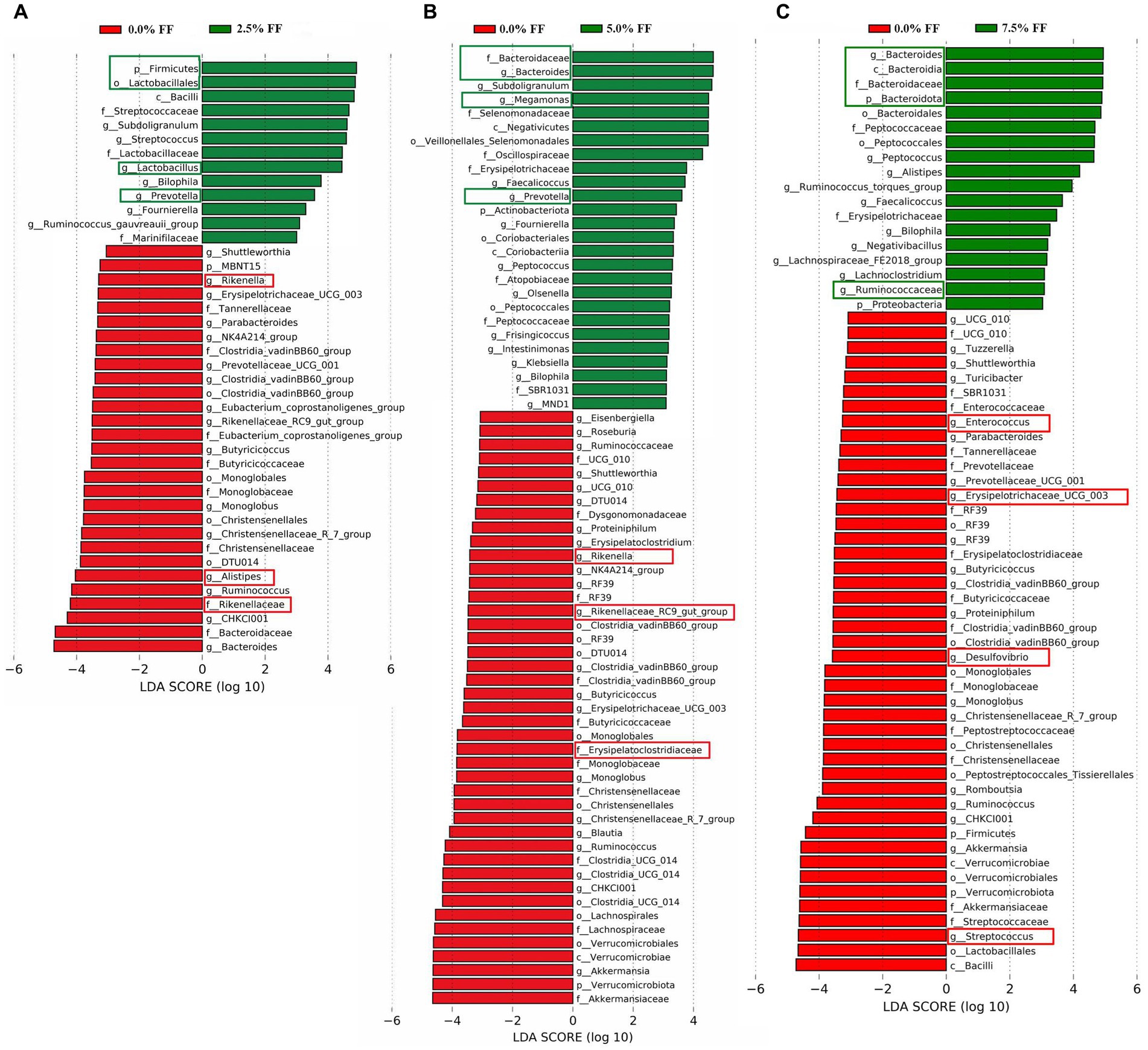
Figure 5. LEfSe histograms, using the default parameter (LDA score > 3.0) identified bacterial taxa with significantly different abundance between all fermented feed groups and the control group (A–C) indicating 2.5, 5.0, and 7.5% fermented feed groups versus the control group, respectively.
4. Discussion
FF is gaining increased attention due to their ability to improve the nutrients in feed (probiotics, vitamins, organic acids, amino acids, peptides, enzymes and growth factors, etc.), thus enhancing the growth performance of animals (26, 27). Indeed, Similarly, Zhu et al. (27) reported that the addition of FF in diets led to significantly increased ADG and significantly decreased FCR of 35-day-old laying hens. In this study, the 5.0 and 7.5% FF groups significantly reduced FCR of 28-day-old goslings. In this study, 28-day-old goslings in the 5.0 and 7.5% FF groups displayed significantly decreased FCR. Zhang et al. (28) reported that the addition of yeast to daily feed led to significantly increased ADG and reduced FCR of Sichuan White Geese. It may be due to the fact that fermented feed reduces pH and pathogenic microbial activity in the gastrointestinal tract of the goslings as well as increases the production of short-chain fatty acids (SCFA), which improves intestinal digestibility of the feed, thus improving the growth performance of the goslings (6). However, Wang et al. (29) found that the addition of cottonseed meal to FF in diets had no significant effect on the growth performance of 1- to 21-day-old broilers. Sun et al. (30) reported that the dietary addition of fermented feed with Bacillus subtilis as the strain could significantly increase the ADG of Hebei meat geese, and geese had high absorption and utilization of substances such as proteins and crude fibers, whereas lignin, cellulose, hemicellulose and other substances that are not easy to be decomposed into small-molecule carbohydrates were substances that are easy to be absorbed and utilized by the intestinal tract, thus improving the growth performance of meat geese. Moreover, the authors also found a positive correlation between growth performance and nutrient digestibility. Chen et al. (31) reported that the addition of Bacillus subtilis and Saccharomyces cerevisiae in diets improved weight of broilers, which can be attributed to the fact that Bacillus subtilis and Saccharomyces cerevisiae FF have increased palatability and result in increased feed intake. Additionally, Niu et al. (32) reported that the addition of fermented Ginkgo biloba to the diet significantly increased ADG and ADFI in broilers at 42 days of age. Wizna et al. (33) reported that dietary supplementation with fermented cassava by-products increased the body weight of 28-day-old chicks, which may be attributed to the positive effect of fermented feeds on the growth performance of chicks due to the increase in the levels and digestibility of threonine, lysine, leucine, and methionine in the diet. However, the addition of fermented cassava by-products to the rations had no effect on the growth performance of 28-day-old chicks, probably because of the increase in glucose content due to the cassava by-products (glucose is produced by the Bacillus amyloliquefaciens cellulase enzyme in the fermented cassava by-products in the gastrointestinal digestive process) and glucose is counted as metabolizable energy. The results of the above studies are not entirely consistent with our research results, and this may be related to the FF process, bacterial strains, the level of antinutritional factors in the diet, feeding management, and the different growth stages of the experimental animal species, thus leading to differential effects of FF on the growth performance of animals (1).
Serum biochemical indexes can be used to indicate the metabolic functioning in animals (34). Protein anabolism and material transport in the body are related to the activity of serum TP and serum ALB levels (35). In this study, FF had no significant effect on the activity of TP and ALB in serum of goslings, indicating that FF had no effect on protein metabolism and substance transport in goslings. Moreover, a study (36) indicated that serum levels of TG and TC may reflect the lipid metabolism of the body. In this study, serum TC levels were significantly lower in the 7.5% FF group compared to the control group, It may be due to the fact that fermented feeds help to inhibit the activity of 3-hydroxy-3-methylglutacyl coenzyme A reductase, which reduces cholesterol biosynthesis. Moreover, fermented feeds may reduce the level of cholesterol in the blood by the mechanism of increasing the synthesis of bile acids, thus improving the lipid metabolism of the gosling organism (37). ALP is a group of isoenzymes located in the outer layer of the cell membrane, which catalyze the hydrolysis of organophosphates. It is present in different tissues of the body and it is present in decreasing concentrations in the placenta, ileal mucosa, kidney, bone, and liver. In the liver, ALP is cytoplasmic and present in the tubular membranes of stem cells, and more than 80% of serum ALP is released from the liver and bone (38). Serum ALP is a marker of liver damage reflecting diseases of the hepatobiliary system and has been widely used as a predictive tool for cholestatic liver disease (39, 40). LDH is widely distributed in eggs, liver, heart, kidneys and muscles, and is a cytoplasmic oxidoreductase enzyme that indicates damage and oxidative stress in the body. In addition, LDH, which can be used to diagnose certain tumors, is also a test indicator for myocardial infarction and liver lesions where the abnormal increase of serum LDH activity may correlate with cardiac or liver injuries (41). Studies have shown that when a patient has cancer, an increase in the number of cancer cells consumes large amounts of glucose, which is used for energy through glycolysis, increasing LDH activity under anaerobic conditions. In addition, growing cancer cells also destroy other tissues of the body and release the intracellular enzyme LDH into the bloodstream through damaged and dead cells, thus increasing LDH activity in the blood (42). Yeh et al. (43) demonstrated that B. subtilis variant N21+, B. coagulans variant L12 in FF led to decreased activity of serum LDH in broilers, which could be caused by the decomposition of the fermentation substrate, or by the reduction of antinutrients due to heat treatment during the pelleting process, thus reducing the activity of serum LDH. Additionally, Wang et al. (40) found that the addition of ginkgo FF to the diet results in a significant decrease in serum ALP activity in meat ducks. In this study, the ALP activity of all FF groups decreased significantly, and the 5.0 and 7.5% FF groups significantly decreased serum LDH activity. These findings suggest that FF protected the liver of goslings to some extent.
Poultry have a protective system that controls the production of free radicals and maintains redox balance; when the balance is disturbed, oxidative stress occurs, resulting in serious effects on the health, growth, and development of poultry (44). T-AOC activity reflect the overall antioxidant capacity of an organism, where an increase represents a higher overall antioxidant capacity of the organism. Additionally, GSH-Px and T-SOD are important antioxidant enzymes in the antioxidant system, which protect the organism from damage by eliminating free radicals in the body. On the other hand, MDA, which is the product of lipid and free radical oxidation reactions in the body, can reflect the degree of lipid peroxidation in the animal body and indirectly reflect the degree of cell damage (45, 46). A study by Hu et al. (36) found that the addition of fermented rapeseed meal in the diet led to significantly increased activity of serum T-SOD in 42-day-old broilers. Similarly, Yin and Huang (47) demonstrated that the addition of fermented alfalfa meal (FAM) in the diet led to significantly increased activity of serum GSH-Px and T-SOD in geese, and significantly decreased serum MDA levels. Moreover, it has been shown that antioxidant function is associated with the nuclear factor-erythroid 2-related factor 2 (Nrf2) signalling pathway, which is a major regulator of oxidative stress damage defence mechanisms and plays an important role in cellular defence against a variety of inflammatory and oxidative stress-induced diseases (48, 49). Niu et al. (34) showed that the addition of fermented Ginkgo biloba to broiler diets increased the mRNA expression of Nrf2, which ultimately increased GSH-Px activity in serum, and T-AOC and sod activity in liver. In this study, the addition of FF in the diet led to significantly increased T-AOC, T-SOD, and activity of GSH-Px of goslings. This may be due to the production of beneficial metabolites such as vitamins and small peptides after the fermentation process which are then present in the FF. These substances help to balance the production of pro-oxidants and antioxidants in the body, thus improving the serum antioxidant capacity of goslings (50).
The small intestine is the main site of nutrient absorption in the animal body, and its surface folds and villi can greatly increase the area for nutrient absorption. Villus height, crypt depth and the ratio of villus to crypt are important indicators to measure the normal function of intestinal mucosa. Specifically, the higher the villous height, the larger the surface area of the intestine and the stronger the absorption capacity of nutrients; whereas the crypt depth reflects the maturation rate of intestinal epithelial cells and the shallower the crypt depth, the increased number of mature cells; finally the villi/crypt ratio reflects the normal morphology and function of the intestine (26, 36, 51). Ding et al. (52) reported that the addition of solid-state fermented sesame meal in diets led to significantly increased villous height and the villi/crypt ratio in jejunum of 21-day-old meat duck. Similarly, Chiang et al. (26) demonstrated that the addition of solid-state fermented rapeseed meal in diets led to significantly increased villous height and villi/crypt ratio in the jejunum and ileum of 21-day-old broilers. Guo et al. (8) reported that the addition of FF in diets led to significantly increased villous height in the duodenum, jejunum, and ileum of laying hens, which could be attributed to the inhibition of Campylobacter and other pathogens by Lactobacillus, thereby reducing damage to the intestinal mucosal epithelium. The results of the present study were in general agreement with Xie et al. (53), in which the villous height and villi/crypt ratio in jejunum increased significantly in all FF groups, while crypt depth decreased significantly. This suggests that FF has a positive effect on intestinal structure. Furthermore, the improvement of intestinal morphology may be attributed to the increase of gastrointestinal probiotics in the FF. After entering the intestine, probiotics colonize and proliferate, supporting a series of metabolic processes The metabolites produced are subsequently absorbed by mucosal cells and promote the growth of intestinal epithelial cells, thus improving the structure of the empty intestinal mucosa, increasing villous height, and reducing crypt depth (53, 54).
Intestinal oxidative stress has a great impact on the health and production of poultry (32). Reactive oxygen species (ROS) are produced by intestinal epithelial cells through oxygen metabolism or intestinal symbiotic bacteria, which affect intestinal health. Increased ROS will lead to the production of free radicals and antioxidant damage, resulting in intestinal oxidative stress. In addition, intestinal oxidative stress can lead to lipid peroxidation, protein modification, and DNA damage as well as membrane damage, which can hinder efficient digestion and absorption of nutrients. Fortunately, supplementation with exogenous vitamins, antioxidants and plant additives with antioxidant characteristics can scavenge ROS, remove intestinal free radicals, help alleviate intestinal oxidative stress, and maintain the integrity of the intestinal mucosa (55, 56). Czech et al. (57) reported that the addition of fermented rapeseed or/and soymeal in diets led to significantly increased activity of SOD in the jejunum of piglets. Furthermore, Niu et al. (32) found that the addition of fermented ginkgo leaf in diets significantly improved the capacity of T-AOC and activity of GSH-Px in the jejunum of broilers and significantly decreased level of MDA. In this study, the activity of T-AOC in the jejunum from 5.0% FF group increased significantly, and 2.5 and 5.0% FF groups’ activity of GSH-Px increased significantly, and all FF groups showed significantly decreased level of MDA in the jejunum, suggesting that FF can enhance antioxidant capacity, to protect intestinal epithelial cells from oxidative stress. FF may also favor the generation of α-amylase, which is responsible for degradation of the cell wall matrix, thereby releasing polyphenols such as flavonoids, which can regulate the production of ROS and antioxidant in cells, thus improving the antioxidant capacity of goose intestine (58–60).
The gastrointestinal tract of poultry contains a complex and dynamic microbial community, where the balance of gastrointestinal microecology is closely related to the health and growth performance in hosts, and changes in gut microbiota can directly affect the digestion and absorption of nutrients in the animal body (61). The diversity of gut microbiota affects the stability of the microflora and the ability of the host to resist pathogenic bacteria (62). FF help to regulate the composition of the gut microbiota of the animal and maintain a healthy gastrointestinal ecosystem by preventing excessive inflammatory responses to pathogens in the intestine (63).
Firmicutes and Bacteroidota are the two major flora in the avian cecum, playing an important role in nutrient digestion and absorption and host metabolism (64). Firmicutes are involved in the digestion of various types of nutrients, promoting the fermentation of polysaccharides in the intestine and improving the health of the host (62). Li et al. (65) reported the addition of FF in diet and found that cecal microbiota of broilers was dominated by Firmicutes and Bacteroidota. Yan et al. (14) reported addition of FF in diet of geese and found that cecal microbiota of the 7.5% FF group was dominated by Firmicutes and Bacteroidota, and the relative abundance of the former is relatively low and the relative abundance of the latter is relatively high, compared with the control group. Our results are in agreement with the previous findings that the high levels of Bacteroidota in FF may help to improve the digestibility of nutrients in the intestinal tract of geese (66). Bacteroidota, are the second largest gut microbiota phylum in poultry and have propionate and acetate as fermentation end products, which are required by the host and gut microbiota. Furthermore, Bacteroidota can degrade macromolecular compounds in the intestinal tract and promote the digestion and absorption of proteins, lipids and polysaccharides in animal body (66, 67). At the genus level, the relative abundance of Lactobacillus in both 2.5 and 5.0% of FF groups was increased, with a significant predominance of Lactobacillus in the 2.5% FF group. Lactobacillus can help prevent the growth and reproduction of pathogenic bacteria in the gastrointestinal tract by lowering the pH of the intestinal environment through the production of lactic acid. Meanwhile, Lactobacillus secretes a variety of metabolites such as organic acids, hydrogen peroxide and carbon dioxide, and hydrogen peroxide activates the catalase-thiocyanate system. When lactate peroxidase is combined with hydrogen peroxide and reacts with thiocyanate to produce oxidative intermediates, it can inhibit the growth of pathogenic bacteria (68, 69). LEfSe analysis showed that except for Bacteroides and Bacteroidaceae in 5.0 and 7.5% FF groups, Megamonas and Prevotella were the dominant bacteria in the 5.0% fermented diet group, while Ruminococcaceae were the dominant bacteria in the 7.5% fermented diet group. In recent studies, Prevotella is generally considered a beneficial genus of bacteria, which plays a key role in the carbohydrate metabolism of the body (70). Ruminococcaceae is the main family in rumen cellulolytic bacteria, which can degrade macromolecules such as cellulose and hemicellulose into nutrients such as short-chain fatty acids (SCFAs) that can be easily digested and absorbed by animals, thus promoting growth (14, 71, 72). Additionally, Desulfovibrio showed a significant advantage in the control group, which included sulfate-metabolizing bacteria, which are known to reduce dietary sulfites as well as sulfated mucopolysaccharides in mucins, leading to the production of the cytotoxic compound hydrogen sulfide, which adversely affects the organism (73). In conclusion, FF can increase the abundance of cecum probiotics, thus regulating the balance of lion-head goslings gut microbiota and improving their overall intestinal health.
5. Conclusion
In conclusion, dietary FF supplementation improved growth performance, serum biochemical indexes, antioxidant capacity and intestinal flora structure of lion-head geese. Notably, dietary 7.5% FF supplementation was optimal for the growth and intestinal health of lion-head geese.
Data availability statement
The datasets generated for this study can be found in the NCBI Repository [accession number: PRJNA1027998].
Ethics statement
The animal study was approved by the Animal Care Committee of Zhongkai University of Agriculture and Engineering (approval number: 20220321). The study was conducted in accordance with the local legislation and institutional requirements.
Author contributions
ZF: Data curation, Writing – original draft. NA: Writing – original draft. XL: Investigation, Supervision, Writing – review & editing. JC: Investigation, Supervision, Writing – review & editing. YW: Investigation, Supervision, Writing – review & editing. QW: Conceptualization, Methodology, Writing – review & editing. JF: Project administration, Conceptualization, Methodology, Writing – review & editing. CL: Writing – review & editing. LL: Writing – review & editing.
Funding
The author(s) declare financial support was received for the research, authorship, and/or publication of this article. This work was supported by the Agricultural Technology Light Cavalry Project of Guangdong Province (XYL2022GZ196C08); China Agriculture Research System of MOF and MARA (No. CARS-42-6); Zhejiang Science and Technology Major Program on Agricultural New Variety Breeding (No. 2021C02068-10); Science and Technology Assistance Project of Guangdong Provincial Department of Science and Technology (KTP20210381 and KTP20210370); the Characteristic Innovation Project of General Universities in the Department of Education of Guangdong Province (2020KTSCX054); Fundamental and Application Research Project of Guangzhou Science and Technology Bureau (202201011863); “RaoPing Lion-head goose Science and Technology Institute” funded by Ministry of Education, Ministry of Agriculture and China Association Science and Technology and “RaoPing Lion-head goose Science and Technology Institute” of Zhongkai University of Agriculture and Engineering; Guangdong Provincial Department of Education General Colleges and Universities Key Areas of Specialization (No. 2023ZDZX4020).
Conflict of interest
The authors declare that the research was conducted in the absence of any commercial or financial relationships that could be construed as a potential conflict of interest.
Publisher’s note
All claims expressed in this article are solely those of the authors and do not necessarily represent those of their affiliated organizations, or those of the publisher, the editors and the reviewers. Any product that may be evaluated in this article, or claim that may be made by its manufacturer, is not guaranteed or endorsed by the publisher.
References
1. Guo, L, Lv, J, Liu, Y, Ma, H, Chen, B, Hao, K, et al. Effects of different fermented feeds on production performance, cecal microorganisms, and intestinal immunity of laying hens. Animals (Basel). (2021) 11:2799. doi: 10.3390/ani11102799
2. Zhang, C, Zhang, C, Du, M, Wang, Y, Zhang, G, and Lee, Y. Effects of dietary supplementation with different fermented feeds on performance, nutrient digestibility, and serum biochemical indexes of fattening lambs. Anim Biosci. (2021) 34:633–41. doi: 10.5713/ajas.20.0445
3. Wang, C, Shi, C, Zhang, Y, Song, D, Lu, Z, and Wang, Y. Microbiota in fermented feed and swine gut. Appl Microbiol Biotechnol. (2018) 102:2941–8. doi: 10.1007/s00253-018-8829-4
4. Herkelman, KL, Cromwell, GL, Stahly, TS, Pfeiffer, TW, and Knabe, DA. Apparent digestibility of amino acids in raw and heated conventional and low-trypsin-inhibitor soybeans for pigs. J Anim Sci. (1992) 70:818–26. doi: 10.2527/1992.703818x
5. Xu, Q, Yuan, X, Gu, T, Li, Y, Dai, W, Shen, X, et al. Comparative characterization of bacterial communities in geese fed all-grass or high-grain diets. PLoS One. (2017) 12:e0185590. doi: 10.1371/journal.pone.0185590
6. Sugiharto, S, and Ranjitkar, S. Recent advances in fermented feeds towards improved broiler chicken performance, gastrointestinal tract microecology and immune responses: a review. Anim Nutr. (2019) 5:1–10. doi: 10.1016/j.aninu.2018.11.001
7. Stanbury, PF, Whitaker, A, and SJ, H. Principles of fermentation technology. 2nd ed. Oxford: Butterworth Heinemann (2003).
8. Guo, W, Xu, LN, Guo, XJ, Wang, W, Hao, QH, Wang, SY, et al. The impacts of fermented feed on laying performance, egg quality, immune function, intestinal morphology and microbiota of laying hens in the late laying cycle. Animal. (2022) 16:100676. doi: 10.1016/j.animal.2022.100676
9. Kim, SK, Kim, TH, Lee, SK, Chang, KH, Cho, SJ, Lee, KW, et al. The use of fermented soybean meals during early phase affects subsequent growth and physiological response in broiler chicks. Asian Australas J Anim Sci. (2016) 29:1287–93. doi: 10.5713/ajas.15.0653
10. Wu, Z, Chen, J, Ahmed Pirzado, S, Haile, TH, Cai, H, and Liu, G. The effect of fermented and raw rapeseed meal on the growth performance, immune status and intestinal morphology of broiler chickens. J Anim Physiol Anim Nutr (Berl). (2022) 106:296–307. doi: 10.1111/jpn.13593
11. Wang, Z. Effect of fermented Broussonetia Papyrifera feed on lactation performance of dairy goats. MD Diss. Univ. NORTHWEST A&F, Xian. (2021). doi: 10.27409/d.cnki.gxbnu.2021.001075
12. Wang, X, Li, G, Liu, Y, Yang, Y, Wang, C, Gong, S, et al. The effects of whole-plant silage maize as replacement commercial feed on the growth performance, carcase yield, relatively organ weight, blood biochemical, and economical traits in Holdobaki goose. Cogent Food Agric. (2023) 9:2236825. doi: 10.1080/23311932.2023.2236825
13. Wei, C, Wang, S, Zhang, Q, Zhao, Y, and Zhang, Y. Effects of dietary metabolizable energy level on production performance of geese: a dose-reponse meta-analysis. Preprints. (2023) 2023090863. doi: 10.20944/preprints202309.0863.v1
14. Yan, J, Zhou, B, Xi, Y, Huan, H, Li, M, Yu, J, et al. Fermented feed regulates growth performance and the cecal microbiota community in Geese. Poult Sci. (2019) 98:4673–84. doi: 10.3382/ps/pez169
15. Zhao, Q, Chen, J, Zhang, X, Xu, Z, Lin, Z, Li, H, et al. Genome-wide association analysis reveals key genes responsible for egg production of lion head goose. Front Genet. (2019) 10:1391. doi: 10.3389/fgene.2019.01391
16. Kozák, J. Goose production and goose products. Worlds Poult Sci J. (2021) 77:403–14. doi: 10.1080/00439339.2021.1885002
17. Lamsal, B, Jung, S, and Johnson, L. Rheological properties of soy protein hydrolysates obtained from limited enzymatic hydrolysis. LWT Food Sci Technol. (2007) 40:1215–23. doi: 10.1016/j.lwt.2006.08.021
18. Duly, EB, Grimason, S, Grimason, P, Barnes, G, and Trinick, TR. Measurement of serum albumin by capillary zone electrophoresis, bromocresol green, bromocresol purple, and immunoassay methods. J. Clin. Pathol. (2003) 56:780–81. doi: 10.1136/jcp.56.10.780
19. Li, P, Chang, X, Fan, X, Fan, C, Tang, T, Wang, R, et al. Dietary calcium status during maternal pregnancy and lactation affects lipid metabolism in mouse offspring. Sci Rep. (2018) 8:16542. doi: 10.1038/s41598-018-34520-6
20. Wang, Z, Maimaitiaili, A, Wang, T, and Song, X. Rifapentine Polylactic acid sustained-release microsphere complex for spinal tuberculosis therapy: preparation, in vitro and in vivo studies. Infect Drug Resist. (2021) 14:1781–94. doi: 10.2147/idr.S304864
21. Zhang, L, Yu, Q, Chen, X, Zhang, M, He, Y, Ji, Z, et al. Mineral and bone disorder biomarkers and inflammation indexes in patients with end stage renal disease. Ann Palliat Med. (2020) 9:3938–46. doi: 10.21037/apm-20-2083
22. Sun, Y, Jiang, N, Li, Z, Li, X, Yang, B, Si, D, et al. A retrospective study of 268 patients with Sars-Cov-2 infection to evaluate the association between blood glucose and severity of Covid-19 pneumonia and patient mortality. Med Sci Monit. (2021) 27:e932156. doi: 10.12659/MSM.932156
23. Zhou, Y, Li, KS, Liu, L, and Li, SL. Microrna-132 promotes oxidative stress-induced pyroptosis by targeting sirtuin 1 in myocardial ischaemia-reperfusion injury. Int J Mol Med. (2020) 45:1942–50. doi: 10.3892/ijmm.2020.4557
24. Yu, Z, Zhao, L, Zhao, J-L, Xu, W, Guo, Z, Zhang, A-Z, et al. Dietary Taraxacum mongolicum polysaccharide ameliorates the growth, immune response, and antioxidant status in association with Nf-Κb, Nrf2 and TOR in Jian carp (Cyprinus Carpio Var. Jian). Aquaculture. (2022) 547:737522. doi: 10.1016/j.aquaculture.2021.737522
25. Du, JH, Xu, MY, Wang, Y, Lei, Z, Yu, Z, and Li, MY. Evaluation of Taraxacum mongolicum flavonoids in diets for Channa Argus based on growth performance, immune responses, apoptosis and antioxidant defense system under lipopolysaccharide stress. Fish Shellfish Immunol. (2022) 131:1224–33. doi: 10.1016/j.fsi.2022.11.034
26. Chiang, G, Lu, W, Piao, X, Hu, J, Gong, L, and Thacker, P. Effects of feeding solid-state fermented rapeseed meal on performance, nutrient digestibility, intestinal ecology and intestinal morphology of broiler chickens. Asian Australas J Anim Sci. (2009) 23:263–71. doi: 10.5713/ajas.2010.90145
27. Zhu, F, Zhang, B, Li, J, and Zhu, L. Effects of fermented feed on growth performance, immune response, and antioxidant capacity in laying hen chicks and the underlying molecular mechanism involving nuclear factor-Κb. Poult Sci. (2020) 99:2573–80. doi: 10.1016/j.psj.2019.12.044
28. Zhang, J, He, H, Yuan, Y, Wan, K, Li, L, and Liu, A. Effects of yeast culture supplementation on growth performance, nutrient digestibility, blood metabolites, and immune response in geese. Animals (Basel). (2022) 12:1270. doi: 10.3390/ani12101270
29. Wang, Y, Deng, Q, Song, D, Wang, W, Zhou, H, Wang, L, et al. Effects of fermented cottonseed meal on growth performance, serum biochemical parameters, immune functions, antioxidative abilities, and cecal microflora in broilers. Food Agric Immunol. (2017) 28:725–38. doi: 10.1080/09540105.2017.1311308
30. Sun, Y, Guo, W, Wei, Y, Guo, X, and Zhu, B. Effects of fermented feed on growth performance and intestinal microorganisms of Hebei meat geese. Pakistan J. Zool (2022) 1–13. doi: 10.17582/journal.pjz/20201130131104
31. Chen, KL, Kho, WL, You, SH, Yeh, RH, Tang, SW, and Hsieh, CW. Effects of Bacillus Subtilis Var. Natto and Saccharomyces Cerevisiae mixed fermented feed on the enhanced growth performance of broilers. Poult Sci. (2009) 88:309–15. doi: 10.3382/ps.2008-00224
32. Niu, Y, Zhang, JF, Wan, XL, Huang, Q, He, JT, Zhang, XH, et al. Effect of fermented Ginkgo Biloba leaves on nutrient utilisation, intestinal digestive function and antioxidant capacity in broilers. Br Poult Sci. (2019) 60:47–55. doi: 10.1080/00071668.2018.1535166
33. Wizna, YR, Abbas, H, Dharma, A, and Kompiang, IP. Influence of dietary fermented tapioca by-products on the performance of broilers and ducklings. Int J Poult Sci. (2009) 8:902–4. doi: 10.3923/ijps.2009.902.904
34. Niu, Y, Wan, X, Zhang, L, Wang, C, He, J, Bai, K, et al. Effect of different doses of fermented Ginkgo Biloba leaves on serum biochemistry, antioxidant capacity hepatic gene expression in broilers. Anim Feed Sci Technol. (2019) 248:132–40. doi: 10.1016/j.anifeedsci.2019.01.003
35. Wei, X, Yang, B, Chen, X, Wen, L, and Kan, J. Zanthoxylum alkylamides ameliorate protein metabolism in type 2 diabetes mellitus rats by regulating multiple signaling pathways. Food Funct. (2021) 12:3740–53. doi: 10.1039/d0fo02695f
36. Hu, Y, Wang, Y, Li, A, Wang, Z, Zhang, X, Yun, T, et al. Effects of fermented rapeseed meal on antioxidant functions, serum biochemical parameters and intestinal morphology in broilers. Food Agric Immunol. (2016) 27:182–93. doi: 10.1080/09540105.2015.1079592
37. Adriani, L, Mayasari, N, and Kartasudjana, R. The effect of feeding fermented Kombucha tea on Hld, Ldl and Total cholesterol levels in the duck bloods. Biotechnol Anim Husb. (2011) 27:1749–55. doi: 10.2298/BAH1104749A
38. Lowe, D, Sanvictores, T, Zubair, M, and John, S. Alkaline phosphatase, vol. 2022. Treasure Island (FL): StatPearls Publishing (2022).
39. Senior, JR. Alanine aminotransferase: a clinical and regulatory tool for detecting liver injury-past, present, and future. Clin Pharmacol Ther. (2012) 92:332–9. doi: 10.1038/clpt.2012.108
40. Wang, J, Ren, S, and Fang, X, editors. Data comparison of the effects of feeding Ginkgo leaf fermentation and chinese Herbal medicine on meat ducks. J. Phys. Conf. Ser. (2019). 1423:012018. doi: 10.1088/1742-6596/1423/1/012018
41. Hsieh, K, and Blumenthal, H. Serum lactic dehydrogenase levels in various disease states. Proc Soc Exp Biol Med. (1956) 91:626–30. doi: 10.3181/00379727-91-22353
42. Chaudhary, A, and Chauhan, V. Lactate dehydrogenase as an Indicator of liver diseases. J Adv Med Dent Sci Res. (2015) 3:S20.
43. Yeh, RH, Hsieh, CW, and Chen, KL. Screening lactic acid bacteria to manufacture two-stage fermented feed and pelleting to investigate the feeding effect on broilers. Poult Sci. (2018) 97:236–46. doi: 10.3382/ps/pex300
44. Surai, PF, Kochish, II, Fisinin, VI, and Kidd, MT. Antioxidant defence systems and oxidative stress in poultry biology: an update. Antioxidants (Basel). (2019) 8:235. doi: 10.3390/antiox8070235
45. Dai, X, Xing, C, Cao, H, Luo, J, Wang, T, Liu, P, et al. Alterations of mitochondrial antioxidant indexes and apoptosis in duck livers caused by molybdenum or/and cadmium. Chemosphere. (2018) 193:574–80. doi: 10.1016/j.chemosphere.2017.11.063
46. Ding, X, Li, H, Wen, Z, Hou, Y, Wang, G, Fan, J, et al. Effects of fermented tea residue on fattening performance, meat quality, digestive performance, serum antioxidant capacity, and intestinal morphology in fatteners. Animals. (2020) 10:185. doi: 10.3390/ani10020185
47. Yin, HC, and Huang, J. Effects of soybean meal replacement with fermented alfalfa meal on the growth performance, serum antioxidant functions, digestive enzyme activities, and cecal microflora of geese. J Integr Agric. (2016) 15:2077–86. doi: 10.1016/S2095-3119(15)61198-4
48. Zhao, L, Zhao, JL, Bai, Z, Du, J, Shi, Y, Wang, Y, et al. Polysaccharide from dandelion enriched nutritional composition, antioxidant capacity, and inhibited bioaccumulation and inflammation in Channa Asiatica under hexavalent chromium exposure. Int J Biol Macromol. (2022) 201:557–68. doi: 10.1016/j.ijbiomac.2021.12.117
49. Qi, G, Mi, Y, Wang, Y, Li, R, Huang, S, Li, X, et al. Neuroprotective action of tea polyphenols on oxidative stress-induced apoptosis through the activation of the Trkb/Creb/Bdnf pathway and Keap1/Nrf2 signaling pathway in Sh-Sy5y cells and mice brain. Food Funct. (2017) 8:4421–32. doi: 10.1039/C7FO00991G
50. Doblado, R, Zielinski, H, Piskula, M, Kozlowska, H, Muñoz, R, Frías, J, et al. Effect of processing on the antioxidant vitamins and antioxidant capacity of Vigna Sinensis Var. Carilla. J Agric Food Chem. (2005) 53:1215–22. doi: 10.1021/jf0492971
51. Li, H, Liu, Y, Wei, L, Lin, Q, and Zhang, Z. Effects of feeding fermented Medicago Sativa (plus soybean and Ddgs) on growth performance, blood profiles, gut health, and carcass characteristics of Lande (meat) geese. Front Physiol. (2022) 13:902802. doi: 10.3389/fphys.2022.902802
52. Ding, X, Li, L, Lv, Y, Xu, F, Kuang, H, and Wang, S. Effects of fermented sesame meal on the performance, serum biochemical parameters and intestinal morphology of ducks. Anim Nutr Feed Technol. (2015) 15:349–59. doi: 10.5958/0974-181X.2015.00036.0
53. Xie, Y, Liu, J, Wang, H, Luo, J, Chen, T, Xi, Q, et al. Effects of fermented feeds and ginseng polysaccharides on the intestinal morphology and microbiota composition of Xuefeng black-bone chicken. PLoS One. (2020) 15:e0237357. doi: 10.1371/journal.pone.0237357
54. Drazbo, A, Ognik, K, Zaworska, A, Ferenc, K, and Jankowski, J. The effect of raw and fermented rapeseed cake on the metabolic parameters, immune status, and intestinal morphology of turkeys. Poult Sci. (2018) 97:3910–20. doi: 10.3382/ps/pey250
55. Wu, QJ, Wang, ZB, Wang, GY, Li, YX, and Qi, YX. Effects of feed supplemented with fermented pine needles (Pinus Ponderosa) on growth performance and antioxidant status in broilers. Poult Sci. (2015) 94:1138–44. doi: 10.3382/ps/pev013
56. Mishra, B, and Jha, R. Oxidative stress in the poultry gut: potential challenges and interventions. Front Vet Sci. (2019) 6:60. doi: 10.3389/fvets.2019.00060
57. Czech, A, Sembratowicz, I, and Kiesz, M. The effects of a fermented rapeseed or/and soybean meal additive on antioxidant parameters in the blood and tissues of piglets. Animals (Basel). (2021) 11:1646. doi: 10.3390/ani11061646
58. Balakrishnan, M, Jeevarathinam, G, Kumar, SKS, Muniraj, I, and Uthandi, S. Optimization and scale-up of α-amylase production by Aspergillus oryzae using solid-state fermentation of edible oil cakes. BMC Biotechnol. (2021) 21:33. doi: 10.1186/s12896-021-00686-7
59. Rafiei, F, and Khajali, F. Flavonoid antioxidants in chicken meat production: potential application and future trends. Worlds Poult Sci J. (2021) 77:347–61. doi: 10.1080/00439339.2021.1891401
60. Cao, FL, Zhang, XH, Yu, WW, Zhao, LG, and Wang, T. Effect of feeding fermented Ginkgo Biloba leaves on growth performance, meat quality, and lipid metabolism in broilers. Poult Sci. (2012) 91:1210–21. doi: 10.3382/ps.2011-01886
61. Yadav, S, and Jha, R. Strategies to modulate the intestinal microbiota and their effects on nutrient utilization, performance, and health of poultry. J Anim Sci Biotechnol. (2019) 10:2. doi: 10.1186/s40104-018-0310-9
62. Guo, JR, Dong, XF, Liu, S, and Tong, JM. High-throughput sequencing reveals the effect of Bacillus Subtilis CGMCC 1.921 on the cecal microbiota and gene expression in ileum mucosa of laying hens. Poult Sci. (2018) 97:2543–56. doi: 10.3382/ps/pey112
63. Missotten, JA, Michiels, J, Dierick, N, Ovyn, A, Akbarian, A, and De Smet, S. Effect of fermented moist feed on performance, gut bacteria and gut histo-morphology in broilers. Br Poult Sci. (2013) 54:627–34. doi: 10.1080/00071668.2013.811718
64. Tilg, H, and Moschen, AR. Microbiota and diabetes: an evolving relationship. Gut. (2014) 63:1513–21. doi: 10.1136/gutjnl-2014-306928
65. Li, J, Tao, L, Zhang, R, and Yang, G. Effects of fermented feed on growth performance, nutrient metabolism and cecal microflora of broilers. Anim Biosci. (2022) 35:596–604. doi: 10.5713/ab.21.0333
66. Tremaroli, V, and Bäckhed, F. Functional interactions between the gut microbiota and host metabolism. Nature. (2012) 489:242–9. doi: 10.1038/nature11552
67. Wrzosek, L, Miquel, S, Noordine, ML, Bouet, S, Joncquel Chevalier-Curt, M, Robert, V, et al. Bacteroides thetaiotaomicron and Faecalibacterium Prausnitzii influence the production of mucus glycans and the development of goblet cells in the colonic epithelium of a gnotobiotic model rodent. BMC Biol. (2013) 11:61. doi: 10.1186/1741-7007-11-61
68. Rybarczyk, A, Bogusławska-Wąs, E, and Łupkowska, A. Effect of Em® probiotic on gut microbiota, growth performance, carcass and meat quality of pigs. Livest Sci. (2020) 241:104206. doi: 10.1016/j.livsci.2020.104206
69. Yang, L, Zeng, X, and Qiao, S. Advances in research on solid-state fermented feed and its utilization: the pioneer of private customization for intestinal microorganisms. Anim Nutr. (2021) 7:905–16. doi: 10.1016/j.aninu.2021.06.002
70. Tett, A, Pasolli, E, Masetti, G, Ercolini, D, and Segata, N. Prevotella diversity, niches and interactions with the human host. Nat Rev Microbiol. (2021) 19:585–99. doi: 10.1038/s41579-021-00559-y
71. Xiaoyu, Z, Yan, Z, Yi, W, Tingting, W, Xiangzhen, L, Daping, L, et al. Production of high-concentration N-caproic acid from lactate through fermentation using a newly isolated Ruminococcaceae bacterium Cpb6. Biotechnol Biofuels. (2017) 10:102. doi: 10.1186/s13068-017-0788-y
72. Yang, Q, Wei, C, Guo, S, Liu, J, and Tao, Y. Cloning and characterization of a L-lactate dehydrogenase gene from Ruminococcaceae bacterium Cpb6. World J Microbiol Biotechnol. (2020) 36:182. doi: 10.1007/s11274-020-02958-4
73. Sawin, EA, De Wolfe, TJ, Aktas, B, Stroup, BM, Murali, SG, Steele, JL, et al. Glycomacropeptide is a prebiotic that reduces Desulfovibrio bacteria, increases cecal short-chain fatty acids, and is anti-inflammatory in mice. Am J Physiol Gastrointest Liver Physiol. (2015) 309:G590–601. doi: 10.1152/ajpgi.00211.2015
Keywords: fermented feed, lion-head goslings, growth performance, antioxidant capacity, intestinal health
Citation: Fu Z, Ao N, Liang X, Chen J, Wang Y, Wang Q, Fu J, Liu C and Lu L (2023) Effects of fermented feed on growth performance, serum biochemical indexes, antioxidant capacity, and intestinal health of lion-head goslings. Front. Vet. Sci. 10:1284523. doi: 10.3389/fvets.2023.1284523
Edited by:
Chunhe Wan, Fujian Academy of Agricultural Sciences, ChinaReviewed by:
Lei Zhao, Heilongjiang Bayi Agricultural University, ChinaXiudong Liao, Chinese Academy of Agricultural Sciences, China
Copyright © 2023 Fu, Ao, Liang, Chen, Wang, Wang, Fu, Liu and Lu. This is an open-access article distributed under the terms of the Creative Commons Attribution License (CC BY). The use, distribution or reproduction in other forums is permitted, provided the original author(s) and the copyright owner(s) are credited and that the original publication in this journal is cited, in accordance with accepted academic practice. No use, distribution or reproduction is permitted which does not comply with these terms.
*Correspondence: Chunpeng Liu, bGl1Y2h1bnBlbmdAMTYzLmNvbQ==; Lizhi Lu, bHVsaXpoaWJveEAxNjMuY29t
†These authors have contributed equally to this work