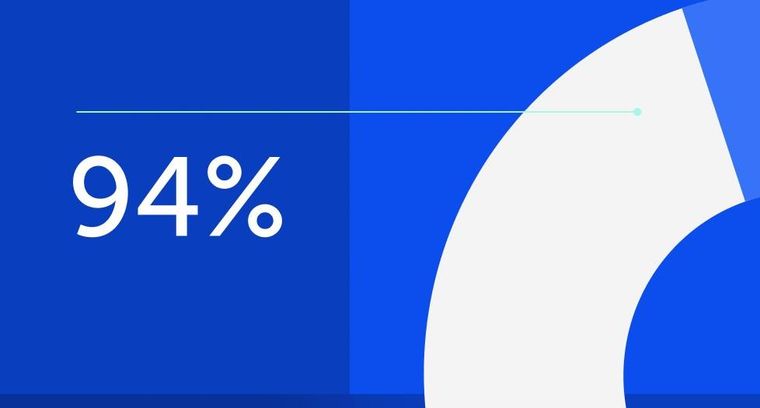
94% of researchers rate our articles as excellent or good
Learn more about the work of our research integrity team to safeguard the quality of each article we publish.
Find out more
REVIEW article
Front. Vet. Sci., 25 October 2023
Sec. Animal Nutrition and Metabolism
Volume 10 - 2023 | https://doi.org/10.3389/fvets.2023.1279506
This article is part of the Research TopicNutricines and Derivatives of Nutrients in Animal Health and Disease PreventionView all 15 articles
Yeast Saccharomyces and its derivatives have been largely used in livestock and poultry nutrition for their potential positive impact on growth, performance, and general health. Originally included in animal diets as a source of protein, yeasts can also offer a wide range of by-products with interesting bioactive compounds that would confer uses beyond nutrition. Although its supplementation in livestock, poultry and even in humans is well documented, the available body of literature on the use of yeast and its derivatives in companion animals’ food, mainly dogs and cats’ diets, is still developing. Despite this, gut microbiota modulation, immune system enhancement or decreasing of potentially pathogenic microorganisms have been reported in pets when using these products, highlighting their possible role as probiotics, prebiotics, and postbiotics. This review attempts to provide the reader with a comprehensive on the effects of Saccharomyces and its derivatives in pets and the possible mechanisms that confer their functional properties.
As the pet food industry keeps steadily growing annually, so does the demand for functional ingredients in dogs and cats’ diets. Owners look for ingredients that can provide additional health benefits beyond basic nutrition, echoing the functional foods trend already established in humans. Examples of these ingredients are omega-3 fatty acids, probiotics/prebiotics/postbiotics, or plants and vegetables’ extracts. Yeasts may also represent a good candidate. There are about 500 different yeasts species and some of them have been used both in humans and animals, especially livestock, for many years, Saccharomyces cerevisiae (S. cerevisiae or SC) being the predominant one. In this review, we will specifically focus on the use of S. cerevisiae, referring to it also as yeast.
Although the use of yeast in livestock and poultry feed has been widely reviewed, there is still a lack of information about the effects on dogs and cats, admittedly the number of studies is increasing. Moreover, despite the many beneficial health effects reported, the supplementation of yeast-based products has not always resulted in consistent effects, and the precise mode of action of these compounds has been difficult to determine. Thus, the aim of this review is to provide an overview on the use of Saccharomyces and/or their derivatives, as a functional ingredient in pet food, discussing its general possible modes of action with a specific focus on in vivo studies in companion animals.
Yeasts cells intracellular composition includes amino acids, peptides, carbohydrates, salts, vitamins, monosodium glutamate, nucleic acids, enzymes, and cofactors. On the other hand, yeast cell wall (YCW) is mostly formed by Beta-glucans (β-glucans), glycoproteins, and mannan oligosaccharides (MOS), and to a lesser extent by chitin. There are different forms of yeasts being studied, ranging from viable yeast cells to purified components of the cell wall, as shown in Table 1. From primary yeast cultures that undergo propagation and fermentation steps, yeasts by-products are obtained via downstream processing (1, 2). Live yeast cells can be inoculated in specific culture media to ferment it and subsequently be dried, forming a yeast culture containing a wide variety of metabolic products. Yeast cells can also undergo a process of hydrolysis or autolysis to obtain yeast lysates that contain both intracellular and cell wall fractions. However, intracellular components and carbohydrates of the cell wall can also be found separately as yeast extract and cell wall products, respectively.
Table 1. Most common Saccharomyces cerevisiae products added in animal feed, with its production process and the major composition (1).
Most of the yeast-based products available in the European market are registered under the European Food Safety Authority (EFSA) category of zootechnical additives, and subcategories of gut microbiota stabilizers or digestibility enhancers, depending on the product and target species. Whereas dried yeast is used for its nutritional value as a specialty amino acid, vitamin and mineral supplement, the rest of the products could fall under the definitions of probiotics, prebiotics, and postbiotics established by the International Scientific Association for Probiotics and Prebiotics (ISAPP). Active dry yeast is formed by live yeast cells and acts as a probiotic, defined by ISAPP as “live microorganisms that when administered in adequate amounts, confer a health benefit on the host” (3). The probiotic role of Saccharomyces cerevisiae var. boulardii (S. boulardii), a strain of the same genus as SC, nearly identical at a molecular level but physiologically different (4), is well established. However the probiotic role of S. cerevisiae needs further investigation, although some recent studies in dogs reported positive and promising results on modulation of fermentative metabolites and microbiota composition and function (5). On the other hand, yeast lysates, yeast extracts, and YCW components, mostly MOS and β-glucans, could be considered prebiotics and their use is more extended, with more studies reporting benefits on both human and animal health (1, 6). Prebiotics are defined by the ISAPP as “a substrate that is selectively utilized by host microorganisms conferring a health benefit” (7). Lastly, yeast culture, also known as Saccharomyces cerevisiae fermentation product (SCFP), would pertain to the still recent term “postbiotics” defined by the ISAPP as “preparation of inanimate microorganisms and/or their components that confers a health benefit on the host” (8).
A balanced gut microbiome is of vital importance for the health of the host and yeasts have been proven to cause shifts in the bacteria population of the gastrointestinal tract in several species (9, 10). In vitro studies reported that the association of some strains of Saccharomyces and Lactobacillus rhamnosus exert a protective effect on human microbiota following antibiotic administration (11) or against pathogenic bacteria (12). The use of yeast β-glucan also promoted the growth of Bifidobacterium longum and other health-promoting gut microbiota in a study simulating human saliva, gastric and intestinal digestion (13). Similarly, mannan and MOS derived from yeast were able to grow specific lactic acid bacteria strains in vitro and beneficial Bacteroides in humans (14, 15). Thus, yeast and yeast-based products may potentially promote host’s gut health.
The supplementation of yeast products can modulate the immune system through changes in the innate and adaptative response. It is thought that the main responsible of these properties are the β-glucan and MOS present in yeast. The specific changes are different depending mainly on the type of product and the general health status of the animals. As reviewed by many authors, yeast products can affect concentrations of platelets and WBC populations, phagocytic capacity of cells, secretion of pro- and anti-inflammatory cytokines, acute phase proteins concentrations, and antibodies production by binding with complement receptor type three (CR3), dectin-1 or TLRs on different cells (16–18). Yeasts may also have an indirect effect on the immune system through microbiota; as previously mentioned, β-glucans and MOS could increase Bifidobacterium population which, in return, has been shown to decrease the expression of toll-like receptors (TLRs), NFkB, and cytokines, in vitro and in vivo with mice (19–21). Moreover, emerging studies also point out the potential of yeast of altering the metabolism, including availability of glucose and fatty acids, as another factor contributing to their capacity of modulate the immune system (16). In conclusion, the immune response is a complex process that goes beyond the response of a single immune parameter; thus, to truly understand the mechanisms behind the possible beneficial effects of the use of yeast as an immunomodulator, integration of the different factors is needed.
Glucans present in yeasts could improve nutrient digestibility through the modification of passage rate in the small intestine. This effect would be mediated by the formation of a semisolid gel promoted by yeast’s alkali-soluble glucans that could slow the passage and increase the time that the chyme is in contact with enzymes, resulting in a higher digestibility of nutrients (22). As a result of this, bacterial fermentation in the large intestine would be reduced as less fermentable substrate would be available. However, one should be cautious with this hypothesis as in vivo studies with yeast β-glucans have reported increases in apparent total tract digestibility (ATTD) in pre-ruminant calves (23) or no changes at all in dogs (24). Other possible mechanism of yeast to modulate nutrient digestibility is the production of enzymes, such as amylases, lipases, and proteases. Some studies with fish and hens have reported increases in digestive enzymes with yeast supplementation, resulting in improved nutrients digestibility (25, 26).
Despite this, other studies have reported decreases in crude protein (CP), nitrogen-free extracts and dry matter (DM) apparent digestibility in dogs supplemented with MOS (27). These reductions in digestibility were accompanied by increased total fecal water and decreased unbound water which could mean different physical properties of intestinal chyme. Thus, the higher water binding could have had an impact on the solubility of nutrients and affected digestibility.
Saccharomyces cerevisiae and other genetically close yeasts can exert a protective effect against toxins and pathogens through different mechanisms that involve the binding of bacteria and toxins, enhancement of immunoglobulins production by the host, and releasing of proteases that destroy certain toxins, among others (28, 29).
One of the most frequent protection mechanisms of yeasts that can be found in the literature is the capacity of binding pathogenic bacteria, avoiding its union with the host’s receptors, being MOS the main responsibles for this binding activity. Tiago et al. (30) showed both in vivo with mice and in vitro that selected strains of S. cerevisiae were able to capture some enteropathogenic bacteria on the surface, regardless of the yeast viability. However, this capturing ability was limited to specific Gram-negative enteropathogens such as Salmonella and Escherichia coli (E. coli). Mannan oligosaccharides form part of Saccharomyces cell walls, and their concentration is especially high in yeast derivatives such as YCW. The mechanism would be that mannose can occupy binding sites on fimbrial adhesins on the surface of bacteria. This way, mannose residues in live yeast or derivatives could prevent certain bacteria that possess fimbria type-1 from adhering to the intestinal mucosa, thus avoiding colonization and multiplication to disease-causing levels (31–33).
Saccharomyces cerevisiae var. boulardii has been widely used in treating Clostridium difficile diarrhea and colitis. The mechanisms of this effect include increasing the activity of intestinal disaccharidases (34) and stimulation of the intestinal secretion of s-IgA and of the secretory component of immunoglobulins (35, 36). Other authors characterized a serine protease released by the yeast that hydrolyzes toxins A and B, which are C. difficile’s mediators of diarrhea and colitis, thus inhibiting the binding of the toxins to its intestinal receptor (37–39). More recent studies have found that S. boulardii exerts an effect on the structural component of the microbe’s biofilm matrix in vitro, possibly affecting intestinal colonization (40). Reports about the use of S. cerevisiae against this pathogen are scarce but some demonstrate a protective effect of certain strains of the yeast in rats and mice (41, 42). Inhibition of other toxins such as cholera toxin or aflatoxin B1 has also been observed in in vitro and in vivo trials using rats, with S. cerevisiae and S. boulardii, hypothesizing that in this case the mode of action included binding of the toxin through a receptor located in its surface (43, 44).
Saccharomyces cerevisiae was also able to reduce heat-labile enterotoxins production from Enterotoxigenic Escherichia coli (ETEC), associated with the removal of the toxin by the yeast and the reduction in the expression of its corresponding gene eltB (45). In the same series of assays, the yeast also interacted directly with ETEC through agglutination in a mannose-related manner.
Lastly, S. cerevisiae-based probiotic has shown benefits in the treatment of bacterial vaginosis produced by Gardnerella vaginalis in mice (46). In this case, the suggested mechanisms of action include direct interference with adherence to vaginal tissues, inhibition of sialidase activity and reduction of vaginal epithelial exfoliation.
The activity of S. cerevisiae and derivatives has been assessed to explore their potential as natural antioxidants, protecting cells against oxidative stress. Although it is probably a result of the combined action of different factors, cell wall β-glucans and mannans are thought to be the main actors in the antioxidant capacity of yeasts. As widely review in the literature, these compounds can boost the antioxidant status of the body by improving the activity of antioxidant enzymes such as glutathione peroxidase (GPx), catalase or superoxide dismutase (SOD) (17). Experiments with porcine jejunum epithelial cell lines damaged by deoxynivalenol, a strong inducer of oxidative stress, reported promising results. The association of mannan and β-glucan from yeast cell walls was able to down-regulate the production of malondialdehyde (MDA) and reactive oxygen species (ROS), while up-regulating the production of glutathione (47). In vivo studies with weaned piglets, supplemented with S. cerevisiae cell wall, also resulted in decreased serum MDA and increased activity of the enzymes GPx, catalase and SOD (48). The addition of yeast β-glucans in broilers exposed to mycotoxins improved serum levels of antioxidant enzymes and lower lipid peroxidation as well (49). Moreover, the naturally good antioxidant capacities of selenium seem to be improved by the enrichment with yeast, due to a synergic effect, as shown in in vitro and in vivo assays with mice (50).
Saccharomyces cerevisiae extract, known occasionally as “skin respiratory factor,” has largely been used by humans for the treatment of burns, wounds and hemorrhoids (51). Studies with human and animal models indicate that it accelerates healing stages: inflammation, angiogenesis, granulation tissue and epithelial migration (52–55). The active fraction of the extract has been identified as a mixture of peptides from 6 to 17 kD formed by: copper zinc superoxide-dismutase ubiquitin, glucose-lipid regulated protein and acyl-CoA binding protein (51, 52). Gruenstein et al. (56) used a human monocyte-derived cell line, THP-1, to study the mechanisms involved in the extract and wound healing. These cells are widely used to study monocyte functions and mechanisms, being monocytes one of the first immune cells to reach the wound site. Apparently, the yeast derivative activates phospholipase-C which results in an increase of cytoplasmic Ca2+. This in turn initiates the transcription of early genes for tissue repair, such as c-fos. β-glucans are other components of the yeast cell wall that can have an effect on wound healing, facilitating the transportation of macrophages to the wound site, stimulating the tissue granulation and re-epithelization, and improving collagen deposition (57).
Topical application of S. cerevisiae has been shown to improve the healing of burn wounds and to enhance the action of a collagen hydrogel-scaffold, increasing epithelialization and biomechanical performance of wound area and reducing the scar size, in rats (58). Studies with seabreams and trouts showed that feed supplementation with S. cerevisiae improved skin health and skin recovery from wounds (59, 60).
The evidence of S. cerevisiae wound healing effect and its antioxidant properties have targeted the yeast as a potential biotechnological raw material in dermo-cosmetic formulations. Dermatological formulations that include S. cerevisiae extract or derivatives showed positive effects in skin moisture and roughness in animal and human models (15, 57, 61); protection against UV irradiation both in vitro and in humans (62, 63) and itch relief in chronic pruritus in humans (64, 65).
Saccharomyces cerevisiae has also been used as a skin cell model in experimental biology. Lastly, this yeast has been linked to different skin diseases such as psoriasis or atopic dermatitis playing a pro- or anti-inflammatory effect, but the exact mechanism has yet to be determined (66).
Probiotic ingestion has been linked to reduced anxiety-like behaviors via the hypothalamic–pituitary–adrenal axis and the gut-brain axis (67). Supplementing a mixture of Bifidobacterium lactis, Lactobacillus acidophilus, and Lactobacillus fermentum to rats exposed to noise stress decreased behaviors related to anxiety and also serum corticosterone (68). Saccharomyces sp. has also been proven to attenuate anxiety-like behaviors in rats exposed to LPS, possibly through modulation of the gut microbiota and hypothalamic–pituitary–adrenal axis (69). In this previous study, yeast supplementation led to significantly lower serum levels of cortisol and corticosterone. Supplementation prevented the reduction in the serum levels of serotonin and brain-derived neurotrophic factor (downregulated during stress) in rats challenged with LPS, compared with those not supplemented and challenged; however, the difference was not significant. Healthy medical students presented a decreased salivary serotonin concentration when facing a stressful event after 30-day supplementation with Saccharomyces sp., although an association with anxiety, depression or eating attitudes was not found (70). Hemiplegic spastic cerebral palsy rats that exhibited depression behavior improved their mental state when a Saccharomyces sp. treatment was applied. This yeast increased gut microbiota diversity and decreased the level of inflammation and the hypothalamic–pituitary–adrenal axis activity (71). In a study with healthy human volunteers, subjects consuming the S. cerevisiae yeast hydrolysate showed better marks in questionnaires that assessed stress and anxiety, suggesting that this derivative could be effective in modulating behavior (72).
There are limited publications examining the impact of yeast or yeast by-products in the dog and cat, although the number is increasing. This contrasts with livestock, where there are a wide number of publications on the use of yeast or yeast by-products. Nevertheless, there is an ever-increasing demand for functional ingredients that improve companion animal health and yeast may prove to provide such benefits.
Pets could benefit from the inclusion of yeast products in their diet, as some promising results have been described in the scientific literature including the modulation of the intestinal microbiota (with potential increases in Bifidobacterium or Lactobacillus), enhancing of the immune function, reduction of potentially pathogenic microorganisms such as E. coli, improvement of blood parameters related with obesity and possible improvement in the antioxidant status, among others. In the following sections we review some of the most recent evidence on the potential benefits of using yeast or derivatives as functional ingredients in petfood. The main outputs of studies with live yeast S. cerevisiae and its by-products in dogs and cats are shown in Tables 2, 3, respectively.
Table 2. Main outputs of different studies on the use of live yeast Saccharomyces cerevisiae and by-products in dogs.
Table 3. Main outputs of different studies on the use of live yeast Saccharomyces cerevisiae and by-products in cats.
The addition of yeast by-products in dogs’ diet produces changes in gut’s bacterial population. Supplementation with SCFP has resulted in increases in the relative abundance of the phyla Firmicutes and/or Actinobacteria, and decreases of Fusobacteria (79, 81). Accordingly, use of dried yeast as primary protein source in a dog diet resulted in higher relative abundances of several genera belonging to Firmicutes phylum (105). An in vivo study simulating the canine intestinal microbial ecosystem also reported a decrease in Fusobacteria and an increase in Bacteroidetes with the addition of YCW (85).
At the genus level, Bifidobacterium increased with live yeast, SCFP, yeast hydrolysate, or MOS addition (5, 81, 82, 88, 94). Prevotella relative abundance increased while Fusobacterium decreased with higher doses of SCFP supplementation (81). Santos et al. (79) also observed reduced Fusobacterium and Allobaculum, as well as increased Clostridium with the inclusion of SCFP; in this same study, a level of 0.6% SCFP of the diet resulted in a decrease of Prevotella and an increase of genus Collinsella. Increases in genera considered beneficial and related with the production of SCFA, such as Bacteroides, Faecalibacterium, Coprococcus, and Phascolarctobacterium, were reported with the use of yeast products (75). Fecal Lactobacillus tended to be higher and total aerobes tended to be lower in dogs supplemented with MOS whereas supplementation with both FOS and MOS resulted in a tendency of lower total anaerobe concentration compared with control (95), although no impact on Bifidobacterium was noted in this case.
Increases in Lactobacillus and Bifidobacterium would likely yield increased lactic acid and short-chain fatty acids (SCFA), which could be the reason behind the lower fecal pH observed by some authors after the inclusion of yeast derivatives in dogs’ diets along with decreased fecal ammonia (27, 84). Bastos et al. (5) also observed lower fecal ammonia levels after an abrupt dietary change in dogs supplemented with live yeast compared to control ones. However, these finding have not been consistent as other authors have reported higher fecal pH (82, 90, 95) or no change (24, 74, 81, 86).
Short chain fatty acids are rapidly absorbed by colonocytes, thus determining their levels in vivo can be challenging, perhaps contributing to the varying results reported. Unaltered fecal concentrations of SCFA have been reported when yeast or yeast by-products were used (24, 74, 81, 95, 97). However, decreases in isovalerate and/or increases in propionate, butyrate, acetate and total SCFA when using yeast products have been reported by different authors (5, 75, 81, 95). Van den Abbeele et al. (85) also observed increases in acetate, butyrate and propionate when adding YCW in their in vitro experiment simulating the canine gut. Moreover, concentration of fecal phenol and/or total phenol + indole were lower in dogs supplemented with yeast products (5, 75, 81, 95). Total fecal biogenic amines decreased significantly in dogs receiving live yeast (5) (5). These results may indicate the possible beneficial effects of yeast and yeast by-products on the canine intestinal functionality.
Yeast cell wall supplementation in cats also led to increased fecal Bifidobacterium and Lactobacillus, and reductions of Clostridium perfringens (C. perfringens), E. coli, Hafnia alvei, and Shigella spp. (112). The inclusion of SCFP in cats’ diet reduced C. perfringens abundance, however no change for Bifidobacterium, Lactobacillus, or E. coli was observed (107). Supplementation with a microencapsulation of probiotic strains which include S. cerevisiae for 20 days resulted in fecal Lactobacillus count increase, but the effect of yeast cannot be isolated in this study (113). Aquino et al. (110) did not find differences in lactic acid bacteria counts nor clostridium-reductor when substituting up to 0.6% of the wet diet with yeast extract in cats.
There were no differences in cats’ fecal pH when SCFP or yeast extract was supplemented (107, 109). Matheus et al. (107) compared a control diet without SCFP with two different inclusion levels (0.3 and 0.6% of the diet), and failed to find differences in fecal pH, ammonia, or SCFA between treatments (except for isovaleric acid, which was lower in 0.3% SCFP inclusion group), However they did report higher fecal lactic acid concentration in cats supplemented with SCFP compared with control ones. On the other hand, lactic acid was not changed and neither propionate, isovalerate, acetate, isobutyrate, total SCFA, and total BCFA when YCW was included in the feed at 0.2, 0.4, or 0.6% of the diet (112). These last authors also found that the fecal concentrations of cadaverine, histamine, putrescine, and total biogenic amines increased linearly with the inclusion of YCW. Collectively, these studies present a lack of consistent responses to yeast or yeast derivatives, which may suggest strain specific impact, but that too will require additional assessments.
Immunity is certainly an area of interest for functional ingredients and may be positively influenced by yeast intake, at least in dogs. Supplementation of dogs with YCW, alone or in combination with FOS, resulted in increased ileal and fecal IgA (86, 90, 95), indicating enhanced mucosal immunity. Some of these authors also found serum IgA increases of around 20% compared with control when including YCW at 0.45–0.5% of the diet. Lin et al. (81), on the other hand, did not find differences in serum IgA, IgE, IgG, and IgM when supplementing SCFP compared to control, but higher SCFP dosages led to higher IgE concentrations. However, these latter authors found that dogs supplemented with SCFP had increased population of antigen-presenting cells, including B-cells and monocytes expressing surface major histocompatibility complex class II. One hypothesis would be that fewer immune cells are needed when immune capacity is enhanced. Moreover, in the same study cells obtained from dogs supplemented with SCFP had a lower TNF-α secretion when stimulated with toll-like receptor (TLR) agonists compared to control dogs, suggesting an anti-inflammatory effect of SCFP. Accordingly, lower pro-inflammatory interleukin 6 (IL-6) and TNF-α, as well as higher blood monocyte phagocytic index, were shown by dogs receiving YCW in the study by Theodoro et al. (87). This increased phagocytic index was also observed with the inclusion of SCFP (79), althought others authors did not report any changes with yeast hydrolysate supplementation (82). The ingestion of yeast-derived β-glucan also produced lower levels of TNF-α in obese dogs (98), as well as lower serum concentrations of IL-6, increased anti-inflammatory IL-10, reduced haptoglobin (marker of inflammatory status), and tended to reduce gut permeability (97, 101).
Pawar et al. (92) found an enhanced cell-mediated immune response, CD4+ lymphocyte population and CD4+:CD8+ ratio in dogs supplemented with MOS. In contrast, Kroll et al. (91), did not observe changes in those cell communities when supplementing adult or senior dogs a second-generation MOS, rich in mannoproteins.
Studies with dogs involving stress challenges suggest some benefits related to the modulation of the immune system as well. Dogs supplemented with SCFP and challenged with exercise and transport stress had increased serum TNF-α and decreased IgE and IgG, although the vitality of these animals was higher (80). In another study involving transport stress challenge, SCFP addition caused stable or decreased cyclooxygenase-2 and myeloperoxidase mRNA expression, two key inflammatory mediators. These findings suggest a positive impact through suppression of innate immune cell activation against stress.
The supplementation of β-glucans derived from S. cerevisiae also influenced the humoral immune response to vaccination, affecting IgA and IgM responses (104), whereas Traughber et al. (99) did not observe any effect.
The modulatory effect of yeasts in the immune system is especially relevant in the treatment of some inflammatory diseases; in fact, β-glucan was superior to levamisole in the treatment of dogs affected by Inflammatory Bowel Disease (IBD) (101) and had promising results treating dogs with signs of canine atopy and osteoarthritis (102, 103).
Hematological changes due to yeast supplementation, similar to other parameters, vary greatly among the studies. Some studies have found an increase within the reference ranges in mean corpuscular volume and mean corpuscular hemoglobin concentration and no effect on white blood cells (WBC) counts in dogs fed live yeast (74). Others noted a decrease in WBC, monocyte and eosinophils counts in dogs given SCFP or YCW components (81, 90). Although these last authors did not find differences in lymphocyte counts, others found an increase (95) and a decrease (78, 94) when supplementing yeast derivatives to dogs.
Although the number of studies evaluating immune modulation in dogs is limited, even less is known regarding to cat. Matheus et al. (107) did not report any difference in cats supplemented with SCFP when comparing CD4+ or CD8+ lymphocytes, CD4+/CD8+ ratio, or phagocytosis index, among other parameters involved in immune response which also did not change. Hematological changes were neither observed when yeast extract was supplemented up to 10% in the diet for cats (108).
As previously discussed, supplementation of yeast and its derivatives can modulate gut microbiota, providing possible benefits. This subsection will go through the changes in potentially pathogenic microorganisms that also inhabit the gastrointestinal tract, and the role that yeast can have in the management of some gastrointestinal disorders.
Although E. coli can sometimes have a positive effect in the gut, such as stimulation of the immune system or vitamin K production, its proliferation is more often associated with a negative impact. In this regard, the supplementation of live yeast, autolyzed YCW and MOS from S. cerevisiae has resulted in lower counts of E. coli (5, 74, 90, 94), although some other authors have not found differences with addition of MOS, FOS, or the combination of both (95). Even in an in vitro experiment simulating the canine gut, adding YCW decreased the presence of the Enterobacteriaceae family, which includes E. coli (85). Another potentially harmful bacteria that could be modulated by yeast is C. perfringens. Whereas some authors did not find differences with MOS supplementation (95), other studies have reported higher C. perfringens counts with yeast extract supplementation but neither was accompanied by diarrhea (86, 90). However, Oba et al. (76) observed that C. perfringens abundance increased in control dogs and was stable for dogs fed SCFP for 21 days. Similarly, decreases in other species of Clostridium such as Clostridium celerecrescens and Clostridium saccharolyticum were observed in dogs fed YCW (88, 89). Bastos et al. (5) reported that control dogs, compared with dogs receiving a live yeast supplementation, showed upregulation in genes related to virulence factors, antibiotic resistance, and osmotic stress.
The use of S. boulardii in dogs with gastrointestinal disorders has also been studied, with promising results. One publication focused on the use of this yeast as a probiotic in dogs with diarrhea induced by lincomycin (114). Twenty-four dogs were given lincomycin intramuscularly (150 mg/kg BW/d) and three groups were formed (n = 8): (I) no S. boulardii, (II) S. boulardii (1,000 mg/d orally for 10 days) after lincomycin induced diarrhea onset, and (III) S. boulardii (1,000 mg/d orally for 10 days) together with lincomycin. The results showed that groups I and II had diarrhea and lower fecal SCFA concentrations, but not group III, and the duration of this gastrointestinal alteration was shorter in group II compared to control. Another publication used S. boulardii in dogs with chronic enteropathy (CE) (115). Four healthy dogs and 20 dogs with CE were used and S. boulardii was administered at a dose of 1 × 109 CFU/kg BW orally in capsules every 12 h. In healthy dogs, the administration of S. boulardii for 10 days reached a steady state in 5 days and was eliminated completely 4 days after administration, without side effects. Dogs with CE received placebo or S. boulardii for 60 days: the yeast significantly improved clinical activity index, stool frequency, and consistency and body condition score over control dogs.
Lastly, yeast cell wall could also have a positive impact in recovering gut health. Dogs fed MOS and experimentally infected with different strains of enteropathogenic E. coli recovered faster than control ones, indicating an improvement in the defense system of the animals (93). On the other hand, yeast-derived β-glucan were superior against levamisole in the treatment of dogs affected by IBD, achieving a fast recovery with no recurrence, at least in 6 months (101).
Components of yeasts such as β-glucan could play a role in obesity prevention and treatment, as obese dogs fed yeast-derived β-glucan had lower plasma basal glycemic values, serum cholesterol and triglyceride levels (98). Reduced serum total- and LDL-cholesterol concentration was also reported in dogs supplemented with MOS (92) and β-glucans (97, 98) from SC.
However, the potential effect of yeast on weight is not clear. Several studies reported no effect on feed intake or body weight (BW) in dogs (24, 73, 81, 86, 90, 97, 105). However, Stercova et al. (74) observed that dogs fed capsulated live yeast SC showed a higher increase of BW compared with the control ones, but the difference was very limited. Increases in intake ratio for diets containing yeast products when compared to diets without yeast have also been reported, although they were not accompanied by differences in dry matter intake or body weight increase (83, 84).
Lastly, a study involving dogs with hyperthyroidism showed that the cessation of yeast as a vitamin B1 source in hypothyroid dogs resulted in loss of appetite and anorexia (106).
Regarding the potential effect of yeast on digestive function and digestibility of nutrients, results in the literature are variable and sometimes contradictory, although the most common findings are decreases in CP ATTD and occasionally increases in fiber ATTD.
Live yeast supplementation had no significant effect on DM, ash, crude fiber (CF), CP and fat ATTD (5); only non-digestible fiber ATTD was higher in supplemented dogs (74). Santos et al. (79) also found increased ATTD of crude fiber when SCFP was added, but decreased ATTD of CP, nitrogen-free extract and metabolizable energy.
On the other hand, Middelbos et al. (90) tried different doses of YCW inclusion on the diet (from 0 to 0.65% of the diet, mostly MOS) and reported increases in DM, organic matter (OM), CP, and gross energy (GE) ileal digestibility compared to control animals but decreases in DM and CP ATTD. Similarly, YCW included as 1.4% of the diet resulted in reduced ATTD of DM, OM, CP, fat, and GE (89) and MOS as 5% of the diet reduced ATTD of DM, CP and nitrogen-free extracts (27). Addition of yeast extract or dried yeast also lowered ATTD of different macronutrients (84, 105). Protein excretion may result from increased bacterial growth and excretion due to increased fermentation, underestimating CP digestibility and, in turn, lowering OM and DM digestibility. However, other studies with various kinds of yeast products (MOS, β-glucans, SCFP and SC from Brewer’s yeast) did not find significant differences neither in ileal nor in apparent total tract nutrient digestibilities when compared to control diet (24, 75, 81, 83, 92, 94–96).
Fecal score was higher (wetter feces) in some studies supplementing SCFP, YCW or MOS to dogs when compared to control groups, although the values remained within the ideal score range (81, 89). Martins et al. (83) observed that dietary yeast inclusion of 30% of the diet resulted in diarrhea and soft feces but an inclusion of up to 15% was suitable, without changes in fecal consistency. However, the inclusion of a live SC yeast as in 7 × 1010 CFU/kg feed resulted in significant higher fecal dry matter, although the magnitude of the changes was of questionable biological relevance (73). Nevertheless, many of the other studies mentioned in this section did not find any differences in fecal score with the dietary inclusion of yeast products generally up to 5% of the diet, with the exception of the 12% inclusion of yeast extract in Kaelle et al. (84).
As for cats, regarding digestibility, there were no differences in nutrient and energy utilization when yeast extract was included in the feed up to 10% in the diet (108) or when yeast extract was added without an acidifying agent (109). Matheus et al. (107) also found relatively similar results, except for CF and ash digestibility, which were higher with SCFP inclusion, although the authors recommend taking these results with cautions as they warn that there may be calculation errors due to low fiber concentration of the diets. Yeast cell wall inclusion also resulted in improved DM and ash digestibility in cats (112).
Dogs kidney and liver function assessed through serum urea, creatinine, alkaline phosphatase (ALP), and alanine transaminase (ALT) seems to be unaltered in some studies where dogs were supplemented with live yeast or yeast by-products (74, 81). However, the activity of ALT and aspartate aminotransferase (ASP) was increased in dogs supplemented with yeast hydrolysate during and after 28 days of supplementation (82). This same study showed increased serum urea levels in dogs receiving the hydrolysate in the post-treatment period whereas total protein, albumin, glucose, and ALP did not change significantly.
Very few studies have assessed the effect of S. cerevisiae on the oxidative status of dogs, although some positive results have been obtained. Supplementation with SCFP at a dose of 500 mg/day decreased thioboarbituric acid reactive substance and increased total antioxidant capacity in serum of dogs exposed to exercise and transport stress, which could indicate an enhanced protection against oxidative damage (80). Wilson et al. (78) also measured markers and expression of genes related to oxidative stress in dogs fed SCFP compared to control ones and observed that superoxide dismutase enzyme increased from baseline and mRNA expression of catalase was lower. However, more investigation is necessary as another study of the same authors showed that SCFP-fed dogs increased malondialdehyde and 8-isoprostane levels due to transport stress (77). Lastly, the supplementation of MOS or SCFP (125, 250, and 500 mg/day) did not exert any effect on erythrocytic antioxidant indices when compared to the non-supplemented group (81, 92).
To the knowledge of the authors, only two studies have investigated the effects of S. cerevisiae supplementation on skin and hair health of dogs. A tendency for higher change from baseline in sebum concentrations was observed in dogs fed SCFP that did not result in greasy hair coat (78). In this study, higher Transepidermal Water Loss (TEWL) in the ear region was also measured in supplemented dogs, possibly indicating enhanced skin integrity. However, TEWL in the back region was higher in control dogs but the authors of the study warn that this may be due to alterations during sampling. In fact, Beloshapka et al. (88) found no difference in the skin condition score in the tail region or any other skin and coat scores when dogs were supplemented with YCW.
Palatability tests comparing standard diets with diets including yeast products have resulted in a preference for the latter, probably due to the presence of glutamic acid and nucleotides, which can intensify flavors of the food (75, 81, 84). These results indicate that yeast or yeast by-products are well accepted by dogs, so their inclusion in commercial diets would not cause refusal and thereby negatively affect the food intake and body condition.
Regarding S. boulardii, there is one publication about the production of meat pet snacks containing this yeast that studied the production process, viability and acceptability of the snacks, showing that the addition of yeast had a good acceptance (116).
Contrary to dogs, in which the use of live yeast or derivatives seemed to increase palatability, results in cats are not uniform and tend to show worse palatability with yeast supplementation. No difference in feed intake compared to control group was found when evaluating the use of live yeast, SCFP or YCW (73, 107, 112).
On the other hand, Lima et al. (108) evaluated the use of yeast extract as a palatability agent observing that cats preferred the control diet over 2% inclusion yeast diet. Similar to Lima, Ogoshi et al. (109) found that the inclusion of yeast extract at 1.5% of the diet reduced feed consumption. Aquino et al. (111) reported lower palatability with wet diets that contained a 0.4% of YCW. Lower preference for yeast extract seems to be countered with the addition of sodium pyrophosphate; this blend was more preferred than control (117). Excess of umami taste and monosodium glutamate could reduce palatability and cats could have a lower threshold of acceptance. Moreover, processing may affect the flavor profile so thermal processes can decrease the flavor-enhancing effect and increase the acceptance of the ingredient. It is also hypothesized that the rejection of yeast extract is due to its high content in leucine as cats reject amino acids “bitter” for humans such as this one.
Pet humanization has led to an increase in the demand for functional ingredients in the pet food industry. Owners are more concerned about pet health and wellness and are willing to invest in diets with superior properties even if it means that they are more expensive, as long as their dogs and cats are healthier. The wide range of yeast-based products with potential to be used as prebiotic, probiotic, or postbiotic is of great interest at present, as they are becoming more related to the successful treatment of diseases. Based on the fact that the main scientific knowledge is gathered from human and livestock studies, further investigations are still required in pet species to better understand the mode of action, doses, safety, and guidelines for use of these products.
While the gut will probably remain the heartland of research, new clinical applications will continue to expand in other research areas including immune system, skin, nervous system, oral cavity, kidney, respiratory system, and weight management field. Thanks to significant and accelerating advances in biotechnologies and bioinformatics methodologies, new research will better characterize mechanistic insights into the mode of action of yeast and their derivatives, leading to a more targeted-oriented development of S. cerevisiae-based products.
MM: Methodology, Writing – review & editing. LC: Methodology, Writing – review & editing, Supervision. SM-O: Methodology, Supervision, Writing – review & editing. AM: Writing – review & editing. OC: Writing – review & editing. AF: Writing – review & editing. AA: Writing – review & editing, Funding acquisition, Methodology, Supervision.
The author(s) declare that no financial support was received for the research, authorship, and/or publication of this article.
The authors declare that the research was conducted in the absence of any commercial or financial relationships that could be construed as a potential conflict of interest.
All claims expressed in this article are solely those of the authors and do not necessarily represent those of their affiliated organizations, or those of the publisher, the editors and the reviewers. Any product that may be evaluated in this article, or claim that may be made by its manufacturer, is not guaranteed or endorsed by the publisher.
1. Shurson, GC. Yeast and yeast derivatives in feed additives and ingredients: sources, characteristics, animal responses, and quantification methods. Anim Feed Sci Technol. (2018) 235:60–76. doi: 10.1016/j.anifeedsci.2017.11.010
2. Patterson, R, Rogiewicz, A, Kiarie, EG, and Slominski, BA. Yeast derivatives as a source of bioactive components in animal nutrition: a brief review. Front Vet Sci. (2022) 9:1067383. doi: 10.3389/fvets.2022.1067383
3. Hill, C, Guarner, F, Reid, G, Gibson, GR, Merenstein, DJ, Pot, B, et al. The international scientific association for probiotics and prebiotics consensus statement on the scope and appropriate use of the term probiotic. Nat Rev Gastroenterol Hepatol. (2014) 11:506–14. doi: 10.1038/nrgastro.2014.66
4. Fietto, JLR, Araújo, RS, Valadão, FN, Fietto, LG, Brandão, RL, Neves, MJ, et al. Molecular and physiological comparisons between Saccharomyces cerevisiae and Saccharomyces boulardii. Can J Microbiol. (2004) 50:615–21. doi: 10.1139/w04-050
5. Bastos, TS, Souza, CMM, Legendre, H, Richard, N, Pilla, R, Suchodolski, JS, et al. Effect of yeast Saccharomyces cerevisiae as a probiotic on diet digestibility, fermentative metabolites, and composition and functional potential of the fecal microbiota of dogs submitted to an abrupt dietary change. Microorganisms. (2023) 11:506. doi: 10.3390/microorganisms11020506
6. Moyad, MA, Robinson, LE, Kittelsrud, JM, Reeves, SG, Weaver, SE, Guzman, AI, et al. Immunogenic yeast-based fermentation product reduces allergic rhinitis-induced nasal congestion: a randomized, double-blind, placebo-controlled trial. Adv Ther. (2009) 26:795–804. doi: 10.1007/s12325-009-0057-y
7. Gibson, GR, Hutkins, R, Sanders, ME, Prescott, SL, Reimer, RA, Salminen, SJ, et al. Expert consensus document: the international scientific association for probiotics and prebiotics (ISAPP) consensus statement on the definition and scope of prebiotics. Nat Rev Gastroenterol Hepatol. (2017) 14:491–502. doi: 10.1038/nrgastro.2017.75
8. Salminen, S, Collado, MC, Endo, A, Hill, C, Lebeer, S, Quigley, EMM, et al. The international scientific association of probiotics and prebiotics (ISAPP) consensus statement on the definition and scope of postbiotics. Nat Rev Gastroenterol Hepatol. (2021) 18:649–67. doi: 10.1038/s41575-021-00440-6
9. Elghandour, MMY, Tan, ZL, Abu Hafsa, SH, Adegbeye, MJ, Greiner, R, Ugbogu, EA, et al. Saccharomyces cerevisiae as a probiotic feed additive to non and pseudo-ruminant feeding: a review. J Appl Microbiol. (2020) 128:658–74. doi: 10.1111/jam.14416
10. Perricone, V, Sandrini, S, Irshad, N, Savoini, G, Comi, M, and Agazzi, A. Yeast-derived products: the role of hydrolyzed yeast and yeast culture in poultry nutrition. A review. Animals. (2022) 12:1426. doi: 10.3390/ani12111426
11. Duysburgh, C, Van den Abbeele, P, Morera, M, and Marzorati, M. Lacticaseibacillus rhamnosus GG and Saccharomyces cerevisiae boulardii supplementation exert protective effects on human gut microbiome following antibiotic administration in vitro. Benefic Microbes. (2021) 12:365–79. doi: 10.3920/BM2020.0180
12. Moens, F, Duysburgh, C, van den Abbeele, P, Morera, M, and Marzorati, M. Lactobacillus rhamnosus GG and Saccharomyces cerevisiae boulardii exert synergistic antipathogenic activity in vitro against enterotoxigenic Escherichia coli. Benefic Microbes. (2019) 10:923–35. doi: 10.3920/BM2019.0064
13. Wang, H, Chen, G, Li, X, Zheng, F, and Zeng, X. Yeast β-glucan, a potential prebiotic, showed a similar probiotic activity to inulin. Food Funct. (2020) 11:10386–96. doi: 10.1039/D0FO02224A
14. Hernández-Macias, S, Comas-Basté, O, Jofré, A, Bover-Cid, S, Latorre-Moratalla, ML, and Vidal-Carou, MC. Growth-promoting effect of cava lees on lactic acid Bacteria strains: a potential revalorization strategy of a winery by-product. Foods. (2021) 10:1636. doi: 10.3390/foods10071636
15. Tanihiro, R, Sakano, K, Oba, S, Nakamura, C, Ohki, K, Hirota, T, et al. Effects of yeast mannan which promotes beneficial bacteroides on the intestinal environment and skin condition: a randomized, double-blind, placebo-controlled study. Nutrients. (2020) 12:1–16. doi: 10.3390/nu12123673
16. Sanchez, NCB, Broadway, PR, and Carroll, JA. Influence of yeast products on modulating metabolism and immunity in cattle and swine. Animals. (2021) 11:1–14. doi: 10.3390/ani11020371
17. Liu, Y, Wu, Q, Wu, X, Algharib, SA, Gong, F, Hu, J, et al. Structure, preparation, modification, and bioactivities of β-glucan and mannan from yeast cell wall: a review. Int J Biol Macromol. (2021) 173:445–56. doi: 10.1016/j.ijbiomac.2021.01.125
18. Thomas, S, Rezoagli, E, Abidin, IZ, Major, I, Murray, P, and Murphy, EJ. Beta-glucans from yeast. Immunomodulators from novel waste resources. Appl Sci. (2022) 12:5208. doi: 10.3390/app12105208
19. Heuvelin, E, Lebreton, C, Grangette, C, Pot, B, Cerf-Bensussan, N, and Heyman, M. Mechanisms involved in alleviation of intestinal inflammation by Bifidobacterium Breve soluble factors. PLoS One. (2009) 4:5184. doi: 10.1371/journal.pone.0005184
20. Okada, Y, Tsuzuki, Y, Hokari, R, Komoto, S, Kurihara, C, Kawaguchi, A, et al. Anti-inflammatory effects of the genus Bifidobacterium on macrophages by modification of phospho-IκB and SOCS gene expression. Int J Exp Pathol. (2009) 90:131–40. doi: 10.1111/j.1365-2613.2008.00632.x
21. Riedel, CU, Foata, F, Philippe, D, Adolfsson, O, Eikmanns, BJ, and Blum, S. Anti-inflammatory effects of bifidobacteria by inhibition of LPS-induced NF-kappaB activation. World J Gastroenterol. (2006) 12:3729–35. doi: 10.3748/wjg.v12.i23.3729
22. Nguyen, TH, Fleet, GH, and Rogers, PL. Composition of the cell walls of several yeast species. Appl Microbiol Biotechnol. (1998) 50:206–12. doi: 10.1007/s002530051278
23. Ma, T, Tu, Y, Zhang, NF, Guo, JP, Deng, KD, Zhou, Y, et al. Effects of dietary yeast β-glucan on nutrient digestibility and serum profiles in pre-ruminant Holstein calves. J Integr Agric. (2015) 14:749–57. doi: 10.1016/S2095-3119(14)60843-1
24. Kilburn-Kappeler, LR, and Aldrich, CG. Evaluation of a yeast β-glucan blend in a pet food application to determine its impact on stool quality, apparent nutrient digestibility, and intestinal health when fed to dogs. Front Anim Sci. (2023) 4:1125061. doi: 10.3389/fanim.2023.1125061
25. El-Bab, AFF, Saghir, SAM, El-Naser, IAA, El-Kheir, SMMA, Abdel-Kader, MF, Alruhaimi, RS, et al. The effect of dietary Saccharomyces cerevisiae on growth performance, oxidative status, and immune response of sea bream (Sparus aurata). Life. (2022) 12:1013. doi: 10.3390/life12071013
26. Matur, E, Ergul, E, Akyazi, I, Eraslan, E, and Cirakli, ZT. The effects of Saccharomyces cerevisiae extract on the weight of some organs, liver, and pancreatic digestive enzyme activity in breeder hens fed diets contaminated with aflatoxins. Poult Sci. (2010) 89:2213–20. doi: 10.3382/ps.2010-00821
27. Zentek, J, Marquart, B, and Pietrzak, T. Intestinal effects of mannanoligosaccharides, transgalactooligosaccharides, lactose and lactulose in dogs. J Nutr. (2002) 132:1682S–4S. doi: 10.1093/jn/132.6.1682S
28. Kaźmierczak-Siedlecka, K, Ruszkowski, J, Fic, M, Folwarski, M, and Makarewicz, W. Saccharomyces boulardii CNCM I-745: a non-bacterial microorganism used as probiotic agent in supporting treatment of selected diseases. Curr Microbiol. (2020) 77:1987–96. doi: 10.1007/s00284-020-02053-9
29. Czerucka, D, and Rampal, P. Diversity of Saccharomyces boulardii CNCM I-745 mechanisms of action against intestinal infections. World J Gastroenterol. (2019) 25:2188–203. doi: 10.3748/wjg.v25.i18.2188
30. Tiago, FCP, Martins, FS, Souza, ELS, Pimenta, PFP, Araujo, HRC, Castro, IM, et al. Adhesion to the yeast cell surface as a mechanism for trapping pathogenic bacteria by Saccharomyces probiotics. J Med Microbiol. (2012) 61:1194–207. doi: 10.1099/jmm.0.042283-0
31. Firon, N, Ashkenazi, S, Mirelman, D, Ofek, I, and Sharon, N. Aromatic alpha-glycosides of mannose are powerful inhibitors of the adherence of type 1 fimbriated Escherichia coli to yeast and intestinal epithelial cells. Infect Immun. (1987) 55:472–6. doi: 10.1128/iai.55.2.472-476.1987
32. Neeser, JR, Koellreutter, B, and Wuersch, P. Oligomannoside-type glycopeptides inhibiting adhesion of Escherichia coli strains mediated by type 1 pili: preparation of potent inhibitors from plant glycoproteins. Infect Immun. (1986) 52:428–36. doi: 10.1128/iai.52.2.428-436.1986
33. Spring, P, Wenk, C, Connolly, A, and Kiers, A. A review of 733 published trials on bio-Mos®, a mannan oligosaccharide, and Actigen®, a second generation mannose rich fraction, on farm and companion animals. J Appl Anim Nutr. (2015) 3:1–11. doi: 10.1017/jan.2015.6
34. Buts, JP, Bernasconi, P, Van Craynest, MP, Maldague, P, and Meyer, R. Response of human and rat small intestinal mucosa to oral administration of Saccharomyces boulardii. Pediatr Res. (1986) 20:192–6. doi: 10.1203/00006450-198602000-00020
35. Buts, JP, Bernasconi, P, Vaerman, JP, and Dive, C. Stimulation of secretory IgA and secretory component of immunoglobulins in small intestine of rats treated with Saccharomyces boulardii. Dig Dis Sci. (1990) 35:251–6. doi: 10.1007/BF01536771
36. Stier, H, and Bischoff, SC. Influence of Saccharomyces boulardii CNCM I-745 on the gut-associated immune system. Clin Exp Gastroenterol. (2016) 9:269–79. doi: 10.2147/CEG.S111003
37. Castagliuolo, I, Thomas Lamont, J, Nikulasson, ST, and Pothoulakis, C. Saccharomyces boulardii protease inhibits Clostridium difficile toxin a effects in the rat ileum. Infect Immun. (1996) 64:5225–32. doi: 10.1128/iai.64.12.5225-5232.1996
38. Castagliuolo, I, Riegler, MF, Valenick, L, LaMont, JT, and Pothoulakis, C. Saccharomyces boulardii protease inhibits the effects of Clostridium difficile toxins A and B in human colonic mucosa. Infect Immun. (1999) 67:302–7. doi: 10.1128/IAI.67.1.302-307.1999
39. Qamar, A, Aboudola, S, Warny, M, Michetti, P, Pothoulakis, C, LaMont, JT, et al. Saccharomyces boulardii stimulates intestinal immunoglobulin A immune response to Clostridium difficile toxin A in mice. Infect Immun. (2001) 69:2762–5. doi: 10.1128/IAI.69.4.2762-2765.2001
40. Lacotte, PA, Simons, A, Bouttier, S, Malet-Villemagne, J, Nicolas, V, and Janoir, C. Inhibition of in vitro Clostridioides difficile biofilm formation by the probiotic yeast Saccharomyces boulardii CNCM I-745 through modification of the extracellular matrix composition. Microorganisms. (2022) 10:1082. doi: 10.3390/microorganisms10061082
41. Izadnia, F, Wong, CT, and Kocoshis, SA. Brewer’s yeast and Saccharomyces boulardii both attenuate Clostridium difficile-induced colonic secretion in the rat. Dig Dis Sci. (1998) 43:2055–60. doi: 10.1023/A:1018811331596
42. Martins, F, Nardi, R, Arantes, R, Rosa, C, Neves, M, and Nicoli, J. Screening of yeasts as probiotic based on capacities to colonize the gastrointestinal tract and to protect against enteropathogen challenge in mice. J Gen Appl Microbiol. (2005) 51:83–92. doi: 10.2323/jgam.51.83
43. Armando, M, Dogi, C, Pizzolitto, R, Escobar, F, Peirano, M, Salvano, M, et al. Saccharomyces cerevisiae strains from animal environment with in vitro aflatoxin B1 binding ability and anti-pathogenic bacterial influence. World Mycotoxin J. (2010) 4:59–68. doi: 10.3920/WMJ2010.1208
44. Brandão, RL, Castro, IM, Bambirra, EA, Amaral, SC, Fietto, LG, Tropia, MJM, et al. Intracellular signal triggered by cholera toxin in Saccharomyces boulardii and Saccharomyces cerevisiae. Appl Environ Microbiol. (1998) 64:564–8. doi: 10.1128/AEM.64.2.564-568.1998
45. Roussel, C, Sivignon, A, de Vallée, A, Garrait, G, Denis, S, Tsilia, V, et al. Anti-infectious properties of the probiotic Saccharomyces cerevisiae CNCM I-3856 on enterotoxigenic E. coli (ETEC) strain H10407. Appl Microbiol Biotechnol. (2018) 102:6175–89. doi: 10.1007/s00253-018-9053-y
46. Sabbatini, S, Monari, C, Ballet, N, Mosci, P, Decherf, AC, Pélerin, F, et al. Saccharomyces cerevisiae–based probiotic as novel anti-microbial agent for therapy of bacterial vaginosis. Virulence. (2018) 9:954–66. doi: 10.1080/21505594.2018.1464362
47. Guo, W, Gu, X, Tong, Y, Wang, X, Wu, J, and Chang, C. Protective effects of mannan/β-glucans from yeast cell wall on the deoxyniyalenol-induced oxidative stress and autophagy in IPEC-J2 cells. Int J Biol Macromol. (2019) 135:619–29. doi: 10.1016/j.ijbiomac.2019.05.180
48. Liu, G, Yu, L, Martínez, Y, Ren, W, Ni, H, Abdullah Al-Dhabi, N, et al. Dietary Saccharomyces cerevisiae Cell Wall extract supplementation alleviates oxidative stress and modulates serum amino acids profiles in weaned piglets. Oxidative Med Cell Longev. (2017) 2017:1–7. doi: 10.1155/2017/3967439
49. Zhang, J, Fang, Y, Fu, Y, Jalukar, S, Ma, J, Liu, Y, et al. Yeast polysaccharide mitigated oxidative injury in broilers induced by mixed mycotoxins via regulating intestinal mucosal oxidative stress and hepatic metabolic enzymes. Poult Sci. (2023) 102:102862. doi: 10.1016/j.psj.2023.102862
50. Guo, H, Guo, S, and Liu, H. Antioxidant activity and inhibition of ultraviolet radiation-induced skin damage of selenium-rich peptide fraction from selenium-rich yeast protein hydrolysate. Bioorg Chem. (2020) 105:104431. doi: 10.1016/j.bioorg.2020.104431
51. Schlemm, DJ, Crowe, MJ, McNeill, RB, Stanley, AE, and Keller, SJ. Medicinal yeast extracts. Cell Stress Chaperones. (1999) 4:171–6. doi: 10.1379/1466-1268(1999)004<0171:MYE>2.3.CO;2
52. Bentley, JP, Hunt, TK, Weiss, JB, Taylor, CM, Hanson, AN, Davies, GH, et al. Peptides from live yeast cell derivative stimulate wound healing. Arch Surg. (1990) 125:641–6. doi: 10.1001/archsurg.1990.01410170089019
53. Crowe, MJ, McNeill, RB, Schlemm, DJ, Greenhalgh, DG, and Keller, SJ. Topical application of yeast extract accelerates the wound healing of diabetic mice. J Burn Care Rehabil. (1999) 20:155–62. doi: 10.1097/00004630-199903000-00032
54. Goodson, W, Hohn, D, Hunt, TK, and Leung, DYK. Augmentation of some aspects of wound healing by a “skin respiratory factor”. J Surg Res. (1976) 21:125–9. doi: 10.1016/0022-4804(76)90072-X
55. Kaplan, JZ. Acceleration of wound healing by a live yeast cell derivative. Arch Surg. (1984) 119:1005–8. doi: 10.1001/archsurg.1984.01390210009003
56. Gruenstein, EI, Schlemm, DJ, Bethi, M, and Keller, SJ. The early signaling pathway of live yeast cell derivative in THP-1 monocytes. Cell Calcium. (2018) 73:112–20. doi: 10.1016/j.ceca.2018.04.008
57. Du, B, Bian, Z, and Xu, B. Skin health promotion effects of natural beta-glucan derived from cereals and microorganisms: a review. Phyther Res. (2014) 28:159–66. doi: 10.1002/ptr.4963
58. Oryan, A, Jalili, M, Kamali, A, and Nikahval, B. The concurrent use of probiotic microorganism and collagen hydrogel/scaffold enhances burn wound healing: an in vivo evaluation. Burns. (2018) 44:1775–86. doi: 10.1016/j.burns.2018.05.016
59. Espinosa, C, and Esteban, MA. Effect of dietary supplementation with yeast Saccharomyces cerevisiae on skin, serum and liver of gilthead seabream (Sparus aurata L). J Fish Biol. (2020) 97:869–81. doi: 10.1111/jfb.14449
60. Sheikhzadeh, N, Heidarieh, M, Karimi Pashaki, A, Nofouzi, K, Ahrab Farshbafi, M, and Akbari, M. Hilyses®, fermented Saccharomyces cerevisiae, enhances the growth performance and skin non-specific immune parameters in rainbow trout (Oncorhynchus mykiss). Fish Shellfish Immunol. (2012) 32:1083–7. doi: 10.1016/j.fsi.2012.03.003
61. Gaspar, LR, Camargo, FB, Gianeti, MD, and Maia Campos, PMBG. Evaluation of dermatological effects of cosmetic formulations containing Saccharomyces cerevisiae extract and vitamins. Food Chem Toxicol. (2008) 46:3493–500. doi: 10.1016/j.fct.2008.08.028
62. Lu, Y, Liu, M, Cao, Y, Yin, J, Zhou, H, Yu, W, et al. Hydrogel sunscreen based on yeast /gelatin demonstrates excellent UV-shielding and skin protection performance. Colloids Surf B: Biointerfaces. (2021) 205:111885. doi: 10.1016/j.colsurfb.2021.111885
63. Zülli, F, Suter, F, Biltz, H, and Nissen, HP. Improving skin function with CM-glucan, a biological response modifier from yeast. Int J Cosmet Sci. (1998) 20:79–86. doi: 10.1046/j.1467-2494.1998.171740.x
64. Dhurat, R, Sharma, A, Surve, R, McCoy, J, Kovacevic, M, Goren, A, et al. Novel yeast extract is superior to colloidal oatmeal in providing rapid itch relief. J Cosmet Dermatol. (2021) 20:207–9. doi: 10.1111/jocd.13436
65. Zhang, Y, Tan, Y, Zou, Y, Bulat, V, Lugovic Mihic, L, Kovacevic, M, et al. Yeast extract demonstrates rapid itch relief in chronic pruritus. J Cosmet Dermatol. (2020) 19:2131–4. doi: 10.1111/jocd.13265
66. Hassan, S, Poulos, C, Bhatti, J, Rangwani, S, Khan, Z, Mohammed, T, et al. Saccharomyces cerevisiae as a skin physiology, pathology, and treatment model. Dermatol Online J. (2020) 26:1–4. doi: 10.5070/D32611048118
67. Doney, E, Cadoret, A, Dion-Albert, L, Lebel, M, and Menard, C. Inflammation-driven brain and gut barrier dysfunction in stress and mood disorders. Eur J Neurosci. (2022) 55:2851–94. doi: 10.1111/ejn.15239
68. Hadizadeh, M, Hamidi, GA, and Salami, M. Probiotic supplementation improves the cognitive function and the anxiety-like behaviors in the stressed rats. Iran J Basic Med Sci. (2019) 22:506–14. doi: 10.22038/ijbms.2019.33956.8078
69. Babaei, F, Mirzababaei, M, Mohammadi, G, Dargahi, L, and Nassiri-Asl, M. Saccharomyces boulardii attenuates lipopolysaccharide-induced anxiety-like behaviors in rats. Neurosci Lett. (2022) 778:136600. doi: 10.1016/j.neulet.2022.136600
70. Karbownik, MS, Kręczyńska, J, Wiktorowska-Owczarek, A, Kwarta, P, Cybula, M, Stilinović, N, et al. Decrease in salivary serotonin in response to probiotic supplementation with Saccharomyces boulardii in healthy volunteers under psychological stress: secondary analysis of a randomized, double-blind, placebo-controlled trial. Front Endocrinol. (2022) 12:1836. doi: 10.3389/fendo.2021.800023
71. Tao, D, Zhong, T, Pang, W, and Li, X. Saccharomyces boulardii improves the behaviour and emotions of spastic cerebral palsy rats through the gut-brain axis pathway. BMC Neurosci. (2021) 22:76–16. doi: 10.1186/s12868-021-00679-4
72. Lee, H, Jung, Y, and Chae, J. The effects of Saccharomyces Cerevisiae yeast extract SCP-20 on stress response, anxiety and depression: a double-blind placebo-controlled trial. Anxiety Mood. (2009) 5:8–13.
73. Bampidis, V, Azimonti, G, Bastos, ML, Christensen, H, Dusemund, B, Fašmon, M, et al. Safety and efficacy of a feed additive consisting of Saccharomyces cerevisiae MUCL 39885 (Biosprint®) for cats and dogs (Prosol S.P.A.). EFSA J. (2021) 19:e06699. doi: 10.2903/j.efsa.2021.6699
74. Stercova, E, Kumprechtova, D, Auclair, E, and Novakova, J. Effects of live yeast dietary supplementation on nutrient digestibility and fecal microflora in beagle dogs. J Anim Sci. (2016) 94:2909–18. doi: 10.2527/jas.2016-0584
75. Bastos, TS, Souza, CMM, Kaelle, GCB, do Nascimento, MQ, de Oliveira, SG, and Félix, AP. Diet supplemented with Saccharomyces cerevisiae from different fermentation media modulates the faecal microbiota and the intestinal fermentative products in dogs. J Anim Physiol Anim Nutr. (2023) 107:30–40. doi: 10.1111/jpn.13824
76. Oba, PM, Carroll, MQ, Sieja, KM, de Souza Nogueira, JP, Yang, X, Epp, TY, et al. Effects of a Saccharomyces cerevisiae fermentation product on fecal characteristics, metabolite concentrations, and microbiota populations of dogs subjected to exercise challenge. J Anim Sci. (2023) 101, 101:1–13. doi: 10.1093/jas/skac424
77. Wilson, SM, Oba, PM, Applegate, CC, Koziol, SA, Panasevich, MR, Norton, SA, et al. Effects of a Saccharomyces cerevisiae fermentation product-supplemented diet on fecal characteristics, oxidative stress, and blood gene expression of adult dogs undergoing transport stress. J Anim Sci. (2023) 101:378. doi: 10.1093/jas/skac378
78. Wilson, SM, Oba, PM, Koziol, SA, Applegate, CC, Soto-Diaz, K, Steelman, AJ, et al. Effects of a Saccharomyces cerevisiae fermentation product-supplemented diet on circulating immune cells and oxidative stress markers of dogs. J Anim Sci. (2022) 100:1–11. doi: 10.1093/jas/skac245
79. Santos, KM, Risolia, LW, Rentas, MF, Amaral, AR, Rodrigues, RBA, Urrego, MIG, et al. Saccharomyces cerevisiae dehydrated culture modulates fecal microbiota and improves innate immunity of adult dogs. Fermentation. (2021) 8:1–19. doi: 10.3390/fermentation8010002
80. Varney, JL, Coon, CN, and Norton, SA. PSV-B-20 effects of Saccharomyces Cerevisiae fermentation product (SCFP) postbiotic in labrador retrievers during exercise and transport stress. J Anim Sci. (2021) 99:332–3. doi: 10.1093/jas/skab235.611
81. Lin, CY, Alexander, C, Steelman, AJ, Warzecha, CM, De Godoy, MRC, and Swanson, KS. Effects of a Saccharomyces cerevisiae fermentation product on fecal characteristics, nutrient digestibility, fecal fermentative end-products, fecal microbial populations, immune function, and diet palatability in adult dogs. J Anim Sci. (2019) 97:1586–99. doi: 10.1093/jas/skz064
82. Strompfová, V, Kubašová, I, Mudroňová, D, Štempelová, L, Takáčová, M, Gąsowski, B, et al. Effect of hydrolyzed yeast administration on faecal microbiota, haematology, serum biochemistry and cellular immunity in healthy dogs. Probiot Antimicrob Prot. (2021) 13:1267–76. doi: 10.1007/s12602-021-09765-9
83. Martins, MS, Sakomura, NK, Souza, DF, Filho, FOR, Gomes, MOS, Vasconcellos, RS, et al. Brewer’s yeast and sugarcane yeast as protein sources for dogs. J Anim Physiol Anim Nutr. (2014) 98:948–57. doi: 10.1111/jpn.12145
84. Kaelle, GCB, Souza, CMM, Bastos, TS, Vasconcellos, RS, Oliveira, SG, and Félix, AP. Diet digestibility and palatability and intestinal fermentative products in dogs fed yeast extract. Ital J Anim Sci. (2022) 21:802–10. doi: 10.1080/1828051X.2022.2054733
85. Van den Abbeele, P, Duysburgh, C, Rakebrandt, M, and Marzorati, M. Dried yeast cell walls high in beta-glucan and mannan-oligosaccharides positively affect microbial composition and activity in the canine gastrointestinal tract in vitro. J Anim Sci. (2020) 98:1–10. doi: 10.1093/jas/skaa173
86. Lin, CY, Carroll, MQ, Miller, MJ, Rabot, R, and Swanson, KS. Supplementation of yeast cell wall fraction tends to improve intestinal health in adult dogs undergoing an abrupt diet transition. Front Vet Sci. (2020) 7:905. doi: 10.3389/fvets.2020.597939
87. Theodoro, S, Putarov, TC, Tiemi, C, Volpe, LM, de Oliveira, CAF, Mb, G, et al. Effects of the solubility of yeast cell wall preparations on their potential prebiotic properties in dogs. PLoS One. (2019) 14:e0225659. doi: 10.1371/journal.pone.0225659
88. Beloshapka, AN, Dowd, SE, Suchodolski, JS, Steiner, JM, Duclos, L, and Swanson, KS. Fecal microbial communities of healthy adult dogs fed raw meat-based diets with or without inulin or yeast cell wall extracts as assessed by 454 pyrosequencing. FEMS Microbiol Ecol. (2013) 84:532–41. doi: 10.1111/1574-6941.12081
89. Beloshapka, AN, Duclos, LM, Vester, BM, and Swanson, KS. Effects of inulin or yeast cell-wall extract on nutrient digestibility, fecal fermentative end-product concentrations, and blood metabolite concentrations in adult dogs fed raw meat–based diets. Am J Vet Res. (2012) 73:1016–23. doi: 10.2460/ajvr.73.7.1016
90. Middelbos, IS, Godoy, MR, Fastinger, ND, and Fahey, GC. A dose-response evaluation of spray-dried yeast cell wall supplementation of diets fed to adult dogs: effects on nutrient digestibility, immune indices, and fecal microbial populations. J Anim Sci. (2007) 85:3022–32. doi: 10.2527/jas.2007-0079
91. Kroll, FSA, Putarov, TC, Zaine, L, Venturini, KS, Aoki, CG, Santos, JPF, et al. Active fractions of mannoproteins derived from yeast cell wall stimulate innate and acquired immunity of adult and elderly dogs. Anim Feed Sci Technol. (2020) 261:114392. doi: 10.1016/j.anifeedsci.2020.114392
92. Pawar, MM, Pattanaik, AK, Sinha, DK, Goswami, TK, and Sharma, K. Effect of dietary mannanoligosaccharide supplementation on nutrient digestibility, hindgut fermentation, immune response and antioxidant indices in dogs. J Anim Sci Technol. (2017) 59:11. doi: 10.1186/s40781-017-0136-6
93. Gouveia, EMMF, Silva, IS, Nakazato, G, Onselem, VJV, Corrêa, RAC, Araujo, FR, et al. Action of phosphorylated mannanoligosaccharides on immune and hematological responses and fecal consistency of dogs experimentally infected with enteropathogenic Escherichia coli strains. Braz J Microbiol. (2013) 44:499–504. doi: 10.1590/S1517-83822013000200027
94. Grieshop, CM, Flickinger, EA, Bruce, KJ, Patil, AR, Czarnecki-Maulden, GL, and Fahey, GC. Gastrointestinal and immunological responses of senior dogs to chicory and mannan-oligosaccharides. Arch Anim Nutr. (2004) 58:483–94. doi: 10.1080/00039420400019977
95. Swanson, K, Grieshop, C, Flickinger, EA, Bauer, LL, Healy, HP, Dawson, KA, et al. Supplemental Fructooligosaccharides and mannanoligosaccharides influence immune function, ileal and total tract nutrient digestibilities, microbial populations and concentrations of protein catabolites in the large bowel of dogs. J Nutr. (2002) 132:980–9. doi: 10.1093/jn/132.5.980
96. Strickling, JA, Harmon, DL, Dawson, KA, and Gross, KL. Evaluation of oligosaccharide addition to dog diets: influences on nutrient digestion and microbial populations. Anim Feed Sci Technol. (2000) 86:205–19. doi: 10.1016/S0377-8401(00)00175-9
97. Rummell, LM, Steele, MA, Templeman, JR, Yohe, TT, Akhtar, N, Lambie, JG, et al. A proof of principle study investigating the effects of supplemental concentrated brewer’s yeast on markers of gut permeability, inflammation, and fecal metabolites in healthy non-challenged adult sled dogs. J Anim Sci. (2022) 100:1–11. doi: 10.1093/jas/skac281
98. Ferreira, CS, Vendramini, THA, Amaral, AR, Rentas, MF, Ernandes, MC, da Silva, FL, et al. Metabolic variables of obese dogs with insulin resistance supplemented with yeast beta-glucan. BMC Vet Res. (2022) 18:14–15. doi: 10.1186/s12917-021-03106-2
99. Traughber, Z, He, F, de Godoy, MR, Varney, JL, Fowler, JW, and Coon, CN. PSVI-24 immunological effects of a purified 1,3/1,6 β-glucan supplementation in retorted canine diets. J Anim Sci. (2020) 98:313–3. doi: 10.1093/jas/skaa278.558
100. Vetvicka, V, and Oliveira, C. β(1-3)(1-6)-D-glucans modulate immune status and blood glucose levels in dogs. J Pharm Res Int. (2014) 4:981–91. doi: 10.9734/BJPR/2014/7862
101. Rychlik, A, Nieradka, R, Kander, M, Nowicki, M, Wdowiak, M, and Kołodziejska-Sawerska, A. The effectiveness of natural and synthetic immunomodulators in the treatment of inflammatory bowel disease in dogs. Acta Vet Hung. (2013) 61:297–308. doi: 10.1556/avet.2013.015
102. Beynen, AC, Saris, DHJ, Paap, PM, van Altena, F, Visser, EA, Middelkoop, J, et al. Dietary beta-1,3/1,6-glucans reduce clinical signs of canine atopy. Am J Anim Vet Sci. (2011) 6:146–52. doi: 10.3844/ajavsp.2011.146.152
103. Beynen, AC, and Legerstee, E. Influence of dietary beta-1,3/1,6-glucans on clinical signs of canine osteoarthritis in a double-blind, placebo-controlled trial. Am J Anim Vet Sci. (2010) 5:97–101. doi: 10.3844/ajavsp.2010.97.101
104. Stuyven, E, Verdonck, F, Van Hoek, I, Daminet, S, Duchateau, L, Remon, JP, et al. Oral administration of β-1,3/1,6-glucan to dogs temporally changes total and antigen-specific IgA and IgM. Clin Vaccine Immunol. (2010) 17:281–5. doi: 10.1128/CVI.00344-09
105. Reilly, LM, He, F, Rodriguez-Zas, SL, Southey, BR, Hoke, JM, Davenport, GM, et al. Use of legumes and yeast as novel dietary protein sources in extruded canine diets. Front Vet Sci. (2021) 8:667642. doi: 10.3389/fvets.2021.667642
106. Drill, VA. The calorie intake and weight balance of hyperthyroid dogs in relation to vitamin B1 and yeast. Am J Physiol Content. (1941) 132:629–35. doi: 10.1152/ajplegacy.1941.132.3.629
107. Matheus, LFO, Risolia, LW, Ernandes, MC, de Souza, JM, Oba, PM, Vendramini, THA, et al. Effects of Saccharomyces cerevisiae cell wall addition on feed digestibility, fecal fermentation and microbiota and immunological parameters in adult cats. BMC Vet Res. (2021) 17:351–10. doi: 10.1186/s12917-021-03049-8
108. Lima, LM, Silva, JW, Ogoshi, RCS, dos Reis, JS, Franca, J, Zangeronimo, MG, et al. Evaluation of raw yeast extract (Saccharomyces cerevisiae) as an ingredient, additive or palatability agent in wet diet for cats. Int J Biol. (2015) 8:1. doi: 10.5539/ijb.v8n1p1
109. Ogoshi, RCS, Zangeronimo, MG, Dos Reis, JS, França, J, Santos, JPF, Pires, CP, et al. Acidifying and yeast extract in diets for adults cats. Anim Sci J. (2014) 85:555–61. doi: 10.1111/asj.12166
110. Aquino, AA, Saad, FMOB, Santos, JPF, Leite, CAL, Sampaio, GR, and Feliciano, MAR. Efeitos da parede de levedura em dieta úmida na microbiota fecal, na produção de gás e na morfologia intestinal de gatos adultos. Arq Bras Med Veterinária Zootec. (2013) 65:1673–80. doi: 10.1590/S0102-09352013000600013
111. Aquino, AA, Saad, FMOB, Santos, JPF, Alves, MP, Ferrazza, RA, and Miranda, MCMG. Efeitos do extrato da parede de levedura na digestibilidade, no escore fecal e na palatabilidade de dietas para gatos. Arq Bras Med Veterinária Zootec. (2010) 62:622–30. doi: 10.1590/S0102-09352010000300018
112. Santos, J, Aquino, A, Glória, M, Avila-Campos, M, Oba, P, Santos, K, et al. Effects of dietary yeast cell wall on faecal bacteria and fermentation products in adult cats. J Anim Physiol Anim Nutr. (2018) 102:1091–101. doi: 10.1111/jpn.12918
113. Rodrigues, BM, Olivo, PM, Osmari, MP, Vasconcellos, RS, Ribeiro, LB, Bankuti, FI, et al. Microencapsulation of probiotic strains by lyophilization is efficient in maintaining the viability of microorganisms and modulation of fecal microbiota in cats. Int J Microbiol. (2020) 2020:1–10. doi: 10.1155/2020/1293481
114. Aktas, MS, Borku, MK, and Ozkanlar, Y. Efficacy of Saccharomyces boulardii as a probiotic in dogs with lincomycin induced diarrhoea. Bull Vet Inst Pulawy. (2007) 51:365–9.
115. D’Angelo, S, Fracassi, F, Bresciani, F, Galuppi, R, Diana, A, Linta, N, et al. Effect of Saccharomyces boulardii in dogs with chronic enteropathies: double-blinded, placebo-controlled study. Vet Rec. (2018) 182:258–8. doi: 10.1136/vr.104241
116. Baroncello, S, Candiago, NT, Gelinski, JMLN, Caliari, V, and Baratto, CM. Meat pet snacks by containing encapsulated Saccharomyces boulardii. Eur J Agric Food Sci. (2020) 2:1–9. doi: 10.24018/ejfood.2020.2.4.69
Keywords: companion animals, prebiotics, probiotics, postbiotics, yeast
Citation: Maturana M, Castillejos L, Martin-Orue SM, Minel A, Chetty O, Felix AP and Adib Lesaux A (2023) Potential benefits of yeast Saccharomyces and their derivatives in dogs and cats: a review. Front. Vet. Sci. 10:1279506. doi: 10.3389/fvets.2023.1279506
Received: 18 August 2023; Accepted: 09 October 2023;
Published: 25 October 2023.
Edited by:
Tao Wang, Jilin Agricultural University, ChinaReviewed by:
Chunyin Geng, Yanbian University, ChinaCopyright © 2023 Maturana, Castillejos, Martin-Orue, Minel, Chetty, Felix and Adib Lesaux. This is an open-access article distributed under the terms of the Creative Commons Attribution License (CC BY). The use, distribution or reproduction in other forums is permitted, provided the original author(s) and the copyright owner(s) are credited and that the original publication in this journal is cited, in accordance with accepted academic practice. No use, distribution or reproduction is permitted which does not comply with these terms.
*Correspondence: Achraf Adib Lesaux, YS5hZGlibGVzYXV4QHBoaWxlby5sZXNhZmZyZS5jb20=
Disclaimer: All claims expressed in this article are solely those of the authors and do not necessarily represent those of their affiliated organizations, or those of the publisher, the editors and the reviewers. Any product that may be evaluated in this article or claim that may be made by its manufacturer is not guaranteed or endorsed by the publisher.
Research integrity at Frontiers
Learn more about the work of our research integrity team to safeguard the quality of each article we publish.