- 1Laboratory of Veterinary Internal Medicine, Tottori University, Tottori, Japan
- 2Joint Graduate School of Veterinary Sciences, Tottori University, Tottori, Japan
- 3Department of Veterinary Clinical Pathobiology, Graduate School of Agricultural and Life Sciences, The University of Tokyo, Bunkyo, Japan
- 4Technical Department, Tottori University, Tottori, Japan
Introduction: The spread of extended-spectrum β-lactamase-producing Enterobacteriaceae (ESBL-E) is a serious concern in companion animal medicine owing to their ability to develop multidrug resistance. Cefmetazole (CMZ) is a candidate drug for treating ESBL-E infections; however, its regimen in dogs has not been established. In this study, we investigated the pharmacokinetic (PK) indices of CMZ in dogs and performed PK–pharmacodynamic (PD) analyses using Monte Carlo Simulation (MCS).
Methods: In total, six healthy dogs received an intravenous bolus dose of CMZ (40 mg/kg body weight). Serum CMZ concentrations were evaluated using liquid chromatography–mass spectrometry, and PK indices were determined based on non-compartmental analysis. The PK–PD cut-off (COPD) values were calculated as the highest minimum inhibitory concentration (MIC) that achieved ≥90% probability of target attainment for a target value of unbounded drug concentration exceeding 40% of the dosing interval. The cumulative fraction of response (CFR) was calculated based on the MIC distribution of wild-type ESBL-E from companion animals.
Results: The area under the concentration–time curve and elimination half-time were 103.36 ± 7.49 mg·h/L and 0.84 ± 0.07 h, respectively. MCS analysis revealed that COPD values for regimens of 40 mg/kg q12, q8h, and q6h were ≤ 0.5, ≤2, and ≤ 4 μg/mL, respectively. A regimen of 40 mg/kg q6h was estimated to achieve a CFR of 80–90% for Escherichia coli and Klebsiella pneumoniae. By contrast, all regimens exhibited a CFR of ≤70% for Proteus mirabilis and Enterobacter cloacae.
Discussion: We conclude that CMZ at 40 mg/kg q6h could be a viable treatment regimen for dogs infected with ESBL-producing Escherichia coli and Klebsiella pneumoniae.
1. Introduction
The spread of extended-spectrum β-lactamase (ESBL)-producing Enterobacteriaceae (ESBL-E) has emerged as a serious concern in companion-animal and human medicine (1–3). Plasmids encoding ESBLs often carry resistance genes for different classes of antimicrobials; therefore, ESBL-E have developed multidrug resistance with high resistance against β-lactams and other classes of antimicrobials, thus limiting the number of effective antimicrobial agents available for treatment (1). Carbapenems are recommended as the first-line drugs for treating ESBL-E infections in humans but not in animals owing to the risk of developing carbapenemase-producing Enterobacteriaceae (CPE), which is a more serious threat than ESBL-E (4, 5). Therefore, the use of carbapenems is discouraged in veterinary medicine due to the potential risk of zoonotic transmission from companion animals to humans through close contact (6). Thus, exploring alternatives to carbapenems is crucial in veterinary medicine.
Cefmetazole (CMZ) belongs to the group of cephamycins and exhibits high stability against β-lactamases due to the presence of the 7α methoxy constituent (7, 8). A previous study in humans has reported that compared with carbapenems, CMZ exhibits non-inferior clinical efficacy for treating ESBL-E infections (9). Furthermore, we previously investigated the pharmacodynamics (PD) of CMZ and found that the drug exhibits high in vitro efficacy against ESBL-E isolates from companion animals (10, 11), as well as some essential oils (12). These findings suggest that CMZ can be used as a carbapenem-sparing drug in companion-animal medicine. However, CMZ regimens for treating ESBL-E infections in dogs have not been established because the pharmacokinetics (PK) of CMZ in dogs is not known.
Recently, PK–PD analysis using Monte Carlo Simulation (MCS) has been used to study appropriate dosing regimens of antimicrobial drugs (13, 14). MCS can establish large virtual populations via the randomization of PK and PD indices and thereby estimate the probability of achieving antimicrobial efficacy (probability of target attainment, PTA) by dosage regimen (14). In this study, we calculated the PK indices of CMZ in dogs after intravenous administration study. To the best of our knowledge, this is the first study to perform PK–PD analysis using MCS to determine the PK–PD cut-off value (COPD) and propose dosing regimens of CMZ which can be clinically effective for ESBL-E infections in dogs.
2. Materials and methods
2.1. Animals
In total, six healthy beagles (4 males, 2 females, aged 5.3 ± 2.0 years and weighing 12.5 ± 1.8 kg, SHIMIZU Laboratory Supplies Co., Ltd., Kyoto, Japan) were used in this study. The dogs were clinically healthy based on their condition, physical examination, complete blood counts, and blood biochemical tests and did not receive any drugs in the 6 months before the study. They were fed the same commercial food (Aiken Genki, Unicharm Corporation, Tokyo, Japan) and were individually housed in separate cages in the same room at the experiment animal facility. This study was conducted under an ethics committee-approved protocol in accordance with the Tottori University Animal Use Committee (approval number No. 19-T-17).
2.2. Drug administration and serum sampling
The day before drug administration, a central venous catheter (Covidien Japan, Inc., Tokyo, Japan) was placed in the jugular vein of the dog under general anesthesia. Anesthesia was induced by intravenously administering propofol (4 mg/kg body weight, Propoflo, DS Pharma Animal Health Co., Ltd., Osaka, Japan), and subsequently intubated with a cuffed endotracheal tube. The vaporizer was adjusted to deliver 2% isoflurane (ISOFLURANE Inhalation Solution, Mylan EPD G.K., Tokyo, Japan) at an oxygen flow rate of 2 L/min. CMZ sodium (Nichi-Iko Pharmaceutical Co., Ltd., Tokyo, Japan) was dissolved in water for a bolus injection (Nissin Pharmaceutical Co., Ltd., Yamagata) at a final concentration of 100 mg/mL, which was injected in the radial cutaneous vein at 40 mg/kg body weight. For CMZ quantification, venous blood samples (2 mL each) were collected from each participant via a central venous catheter at predetermined time points (0, 5, 10, 15, 30, 45, 60, 90, 120, 150, 180, 240, 360, 480, 600, and 720 min). Serum samples were obtained after coagulation and then frozen at −80°C until quantification.
2.3. Sample preparation
CMZ was extracted from each serum sample using solid-phase extraction (SPE) according to a previously described protocol (15). Ethylparaben (Sigma-Aldrich Japan, Tokyo, Japan) was used as an internal standard (IS), which was dissolved in methanol to 10 μg/mL, because the compound is stable and shows a retention time (6.36 min) close to that of CMZ (3.6 min). The prepared sample was stored frozen at −80°C until analysis.
2.4. Determination of antimicrobial concentrations
The serum concentration of CMZ was determined according to a previously described protocol with slight modifications (15). High-performance liquid chromatography (HPLC) separations were performed with a 10A HPLC system (Shimadzu, Kyoto, Japan) under 50:50 isocratic conditions using the following two solutions: 0.1% (v/v) formic acid in 10 mM ammonium formate water as mobile phase A and 0.1% (v/v) formic acid-acetonitrile as mobile phase B at a flow rate of 0.2 mL/min. A 20 μL sample of serum was injected and target molecules were separated using TSKgel Reversed Phase Chromatography (TSKgel ODS-100Z 3 μm, 2.0 mm i.d. x 150 mm, TOSOH, Tokyo, Japan), with the temperature controlled at 40°C. Mass spectra (MS) were measured using an Exactive mass spectrometer (Thermo Fisher Scientific, Waltham, MA, United States) under ESI with a tube lens voltage of 80 V and a skimmer voltage of 30 V. CMZ was detected by [M + H]+ precursor ion m/z = 472.1, and IS was detected by [M-H]− precursor ion m/z = 165.1. The area under the peak was determined using commercial analytical software (Xcalibur QualBrowser, Thermo Fisher Scientific Inc., MA, United States). The concentration of CMZ in each sample was calculated based on the calibration curve (0.1, 1, 5, 10, 50, 100, and 200 μg/mL) constructed by mixing canine serum with the known concentrations of the drug. The reliability of the analytical method was based on the guidelines of the Ministry of Health, Labor, and Welfare (MHLW) (16).
2.5. Monte Carlo Simulation
MCS was performed to calculate PTA based on the PK–PD parameters of CMZ when administered at 40 mg/kg body weight at q12h, q8h, and q6h, using commercial software (Oracle Crystal Ball version 11.1.2.4.850, Kozo Keikaku Engineering Inc., Tokyo, Japan). The PK parameters in the non-compartment model (17) were calculated based on the serum CMZ concentrations in the six dogs by computing 10,000 bootstrap replicates using the PK package (ver. 4.0.3) of R software (ver. 4.2.1) (18). Assuming that PK parameters were distributed lognormally, 10,000 virtual patients were generated for each dosage regimen to build the drug serum concentration-time profile. The percentage of time for which the unbound drug concentrations remained above the minimum inhibitory concentration (MIC) (fTAM) was estimated as the PK–PD index to determine the optimal dosage regimen (19, 20). In this study, a serum protein-binding rate of 26% was applied according to a previous study (21). The PTA for each dosing regimen was calculated at each MIC to determine the percentage of subjects achieving the PK–PD index that could achieve bacteriostatic activity (i.e., fTAM ≥40%) (19, 20). COPD was calculated as the highest MIC that achieved the target of PTA ≥ 90% (20). The cumulative fraction of response (CFR) was calculated as the proportion of %PTA of each MIC based on the wild-type MIC distribution (22), of which CMZ in ESBL-E (Escherichia coli, Klebsiella pneumoniae, Proteus mirabilis, and Enterobacter cloacae) isolates from companion animals were determined in the previous studies (10, 11).
3. Results
None of the dogs exhibited clinical signs of adverse reactions or abnormal blood test results throughout the experiment.
The coefficient of variation (CV) of CMZ, IS, and CMS peak area value corrected by IS (CMZ/IS) was 4.01, 12.1, and 9.50%, respectively, all of which fulfilled the criteria (< 15%) according to the MHLW guideline (16). The blood concentration-time curves and PK parameters in dogs after intravenous bolus administration of CMZ at 40 mg/kg are presented in Figure 1 and Table 1, respectively. Serum CMZ concentration at 5 min was 154.32 ± 14.01 μg/mL, which decreased gradually.
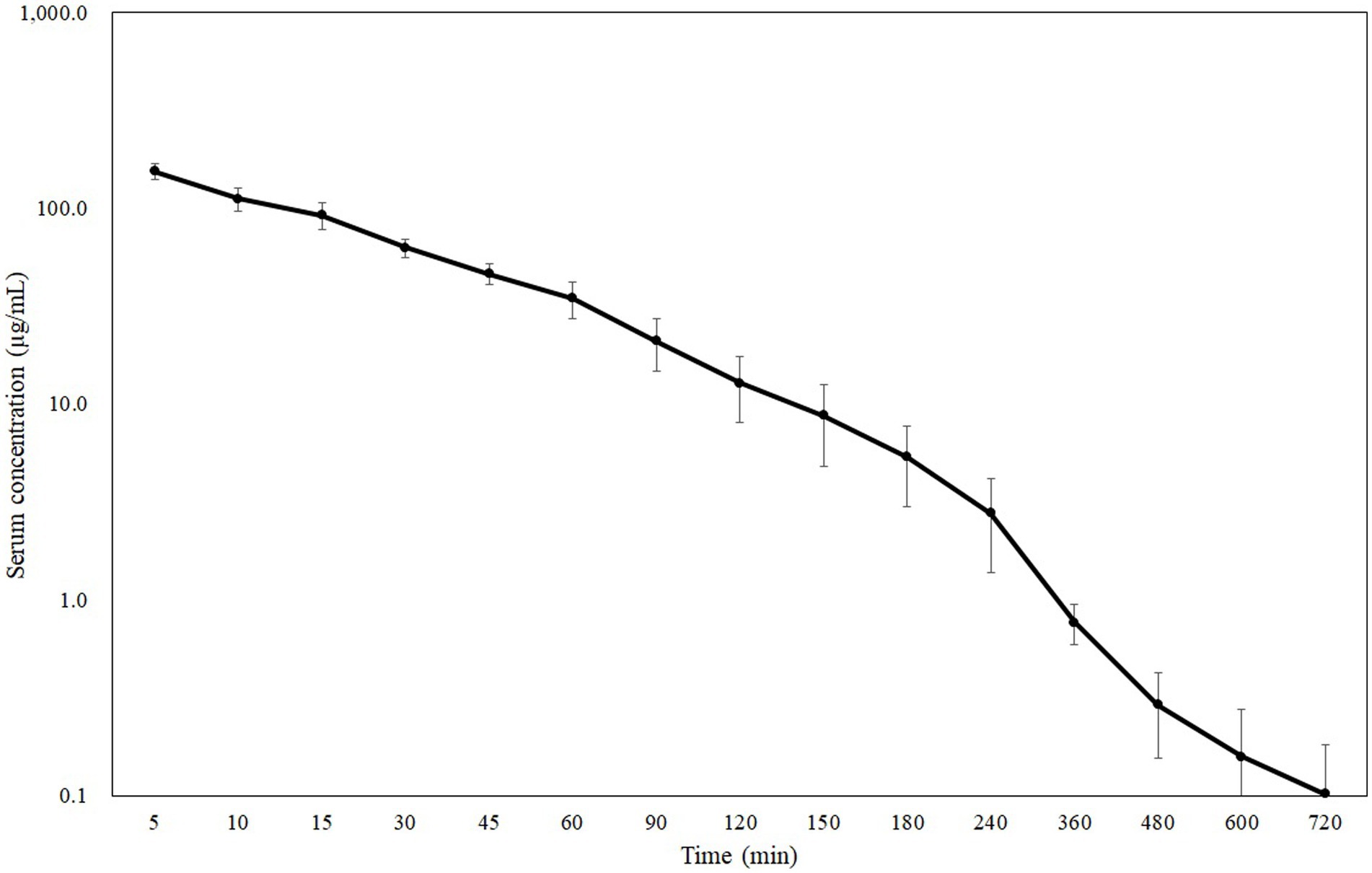
Figure 1. Semilogarithmic plot of serum CMZ concentration in dogs administered a dose of 40 mg/kg body weight (Mean ± SD, n = 6).
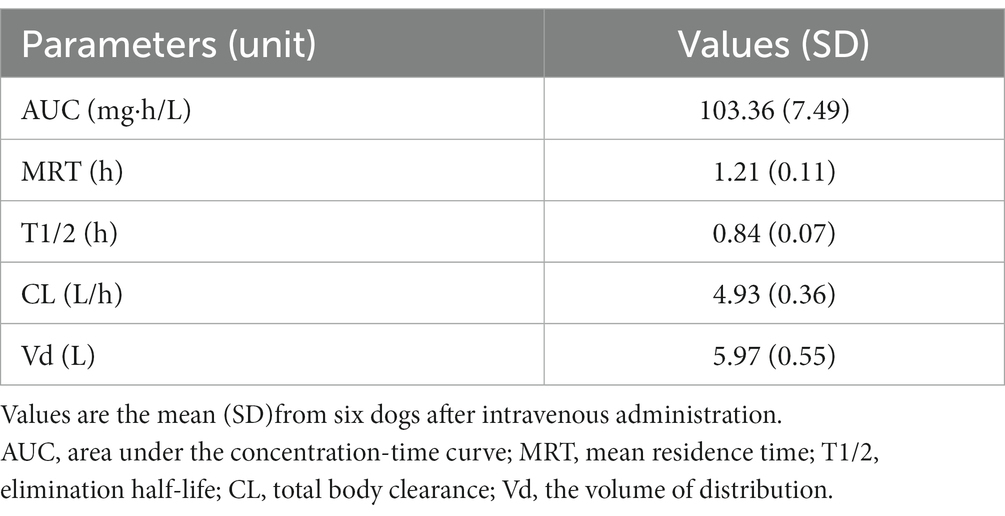
Table 1. Pharmacokinetic parameters determined after intravenous administration of CMZ at the dose of 40 mg/kg body weight in dogs.
We previously determined MICs of CMZ in a total of 308 ESBL-producing isolates of Escherichia coli (n = 90), Klebsiella pneumoniae (n = 120), Proteus mirabilis (n = 29), and Enterobacter cloacae (n = 69), using the agar dilution method according to the Clinical and Laboratory Standards Institute guideline (10, 11). These investigations showed that the MIC50 and MIC90 of CMZ were 1 and 8 μg/mL for Escherichia coli, 2 and 32 μg/mL for Klebsiella pneumoniae, 4 and 32 μg/mL for Proteus mirabilis, and 256 and > 256 μg/mL for Enterobacter cloacae. In this study, we observed the mean blood concentrations decreased below the MIC90 for Klebsiella pneumoniae and Proteus mirabilis at 1 h and below that for Escherichia coli at 3 h. Furthermore, the serum concentration decreased below the MIC50 for Proteus mirabilis at 4 h and below that for Escherichia coli and Klebsiella pneumoniae at 6 h. In contrast, the mean blood concentration at 5 min did not exceed the MIC50 and MIC90 for Enterobacter cloacae.
The PTA results at each MIC with regimens of 40 mg/kg q12h, q8h, and q6h are shown in Figure 2. All regimens achieved a PTA of ≥90%, with a MIC of ≤0.5 μg/mL but not with a MIC of ≥8 μg/mL. Based on the calculated PTA, the COPD values for q12, q8h, and q6h were ≤ 0.5, ≤2, and ≤ 4 μg/mL, respectively.
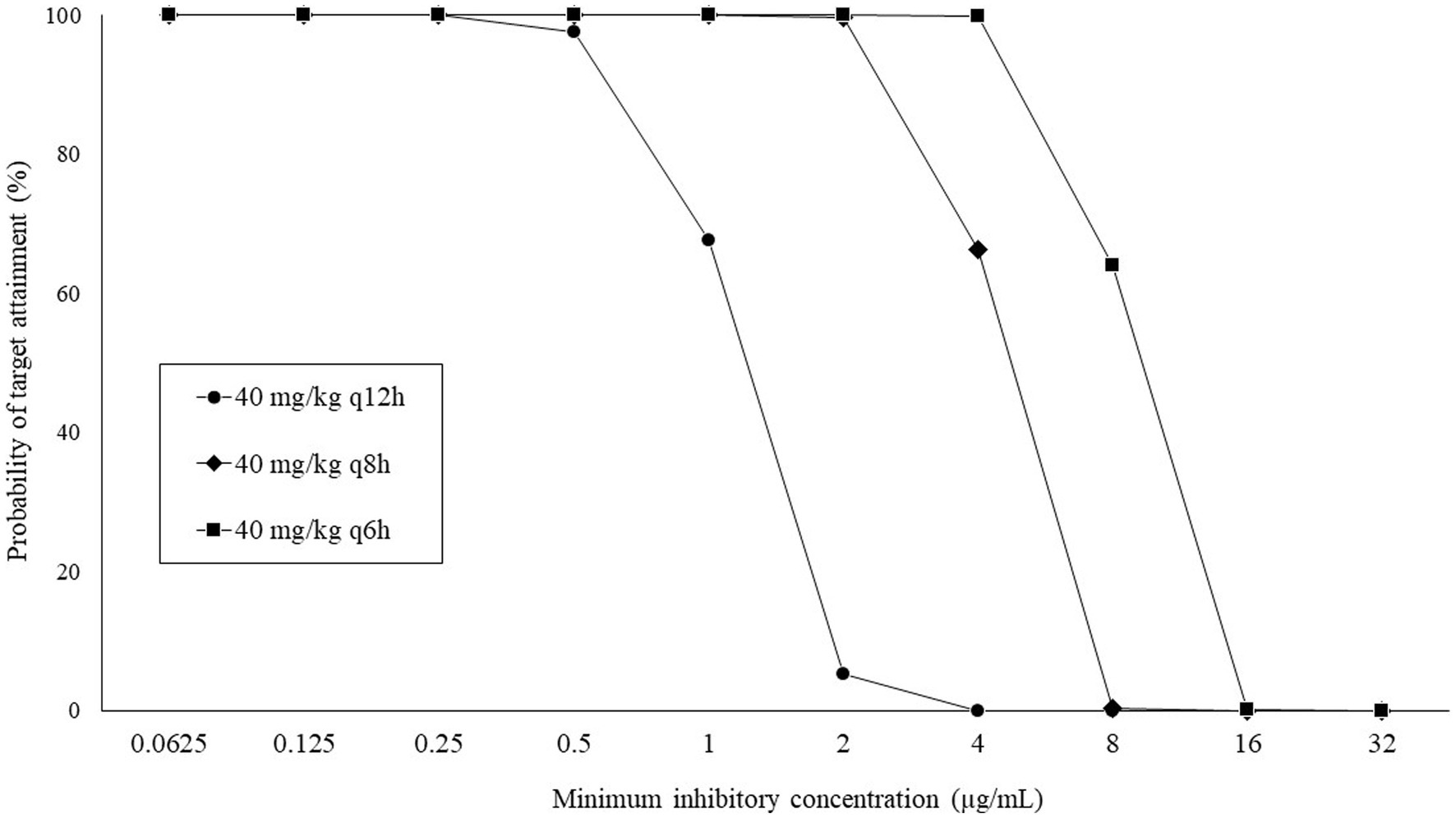
Figure 2. Probability of target attainment (%) at each minimum inhibitory concentration following intravenous administration of CMZ.
Table 2 shows the CFR results that were calculated based on the wild-type MIC distribution of ESBL-E. Overall, higher CFRs were observed for Escherichia coli and Klebsiella pneumoniae than for Proteus mirabilis, and extremely low CFRs (<10%) were confirmed for Enterobacter cloacae, regardless of the regimen. When using fTAM ≥40% as the target of the PK–PD index, none of the regimens achieved a CFR ≥90% in canines infected with all bacterial species. Regimens of 40 mg/kg q6h and q8h were estimated to achieve CFR of 80–90% and 70–80%, respectively, for patients infected with Escherichia coli and Klebsiella pneumoniae. In contrast, all regimens were estimated to have a CFR of ≤70% for patients infected with Proteus mirabilis and Enterobacter cloacae.

Table 2. Cumulative fraction of response following regimens of 40 m/kg CMZ in dogs against wild-type MIC distribution of ESBL-producing Enterobacteriaceae.
4. Discussion
There is a growing demand for alternative carbapenem-based antibiotics to appropriately treat ESBL-E infections without spreading CPE (5). Unfortunately, these antibiotics have not been thoroughly investigated in veterinary medicine. CMZ is one of the candidate drugs to spare carbapenems because the drug is highly stable against ESBLs (5). However, PK-PD properties of the drug, which are essential to consider optimal dosing regimens (23, 24), are still unknown. To resolve this issue, we investigated the PK indices of CMZ in dogs and performed PK–PD analyzes using MCS. Furthermore, we estimated the COPD values of CMZ when administered with expected regimens using MCS and calculated the CFR based on the MIC distribution of wild-type ESBL-E.
We investigated the PK parameters of CMZ in dogs based on the results of blood concentration transition after bolus administration. Borin et al. (25) determined the corresponding PK data in young adult humans, ranging in age from 21 to 40 years, when intravenously administered, as follows: the mean residence time (MRT) was 1.78 h, the elimination half-life (T1/2) was 1.34 h, total body clearance (CL) was 0.10 L/h/kg, and the volume of distribution (Vd) was 0.17 L/kg. Our study indicates the shorter MRT and T1/2, higher CL, and larger Vd in dogs administered with CMZ, compared with the PK data in humans. These results may be explained by the fact that the protein-binding rate of CMZ is significantly lower in dogs than in humans (66%) (21), which can result in wider tissue distribution and faster renal excretion. These PK traits of CMZ in dogs may negatively affect its clinical efficacy because its bactericidal activity is time-dependent (26).
Breakpoints of antimicrobial susceptibility are essential for appropriate antimicrobial treatment. The Clinical Laboratory Standards Institute (27) provided the human-specific breakpoint of the CMZ but not the canine-specific breakpoint. Therefore, we studied the canine-specific COPD values of CMZ for practical application in dogs with bacterial infections. Our finding revealed that COPD values can be increased by shortening dose interval; however, COPD values of CMZ based on all dosage regimens were lower than the CLSI susceptibility breakpoint for Enterobacteriaceae (≤16 μg/mL), probably due to the higher clearance of the drug in dogs. Therefore, the application of the CLSI breakpoint may overestimate CMZ susceptibility in canine pathogens, resulting in treatment failure.
The present COPD values with all regimens were also lower than the previously reported MIC90 values of ESBL-producing Escherichia coli (8 μg/mL), Klebsiella pneumoniae (32 μg/mL), Proteus mirabilis (32 μg/mL), and Enterobacter cloacae (>256 μg/mL) (10, 11). Such lower COPD values were supported by other findings in this study that CMZ exhibited a relatively short time during which the blood concentration remained above the MIC90 of these bacteria. When administered with the regimens of q6h and q8h, the COPD induced by CMZ exceeded the MIC50 values of ESBL-producing Escherichia coli (1 μg/mL) and Klebsiella pneumoniae (2 μg/mL), but not Proteus mirabilis (4 μg/mL) and Enterobacter cloacae (256 μg/mL). Furthermore, we calculated the CFR based on the wild-type MIC distribution of ESBL-E and thereby clarified that the q6h regimens exhibited more than 80% CFRs for ESBL-producing Escherichia coli and Klebsiella pneumoniae. Generally, regimens with ≥90% CFR are optimal, whereas those with 80–90% CFR are associated with moderate probabilities of success (28). Therefore, at least the regimens of CMZ 40 mg/kg q6h may have moderate clinical efficacy in canine patients infected with ESBL-producing Escherichia coli and Klebsiella pneumoniae. However, all regimens in this study exhibited lower CFRs against ESBL-producing Proteus mirabilis and Enterobacter cloacae and thus may have less efficacy for these bacteria. Such differences in the putative clinical efficacy of CMZ between bacteria should be considered when administering CMZ in dogs.
Unfortunately, the optimal dose of CMZ in dogs has not yet been determined. In previous reports (29–31), CMZ was administered to canine patients at 20–25 mg/kg body weight per dose. However, these usages were intended for the perioperative prevention of infection but not for the treatment of bacterial infection. Our preliminary study showed that COPD and CFR at a dose of 20 mg/kg were extremely low (data not shown); thus, this dosage is unlikely to be suitable for treating dogs with ESBL-E infections. Therefore, we used 40 mg/kg per dose in this study, referencing the human dosage (i.e., a maximum of 37.5 mg/kg four times per day). This dose is considered acceptable from a safety perspective because CMZ has a wide margin of safety (32) and extremely high level of no observed effect in dogs (i.e., 1,200 mg/kg) (33).
We applied a fTAM of ≥40% as the PK–PD target values (19, 20), but McKinnon et al. (34) reported that a fTAM of 100% is recommended as the target value of cefepime and ceftazidime for serious infection. Furthermore, in recent years, Takemura et al. (35) demonstrated that a fTAM of >57.6% was preferable as the target value of CMZ for static effects on ESBL-producing Es. coli infections in a murine infection model. These studies emphasize the need to consider higher PK–PD target values for CMZ in dogs. More than half of dog-origin ESBL-E strains have been isolated from urinary tract infections (10, 11). In addition, the peak drug concentration is approximately 20 times higher in the urine than in the blood of dogs after administration of CMZ (36), implying that CMZ may have high efficacy against urinary tract infection in dogs with ESBL-E, even if the blood concentration during repeated administration cannot achieve the target value. Further clinical studies are required to confirm this hypothesis.
Our study has several limitations. We only used a small number of dogs to calculate the PK–PD parameters due to animal welfare concerns. However, we increased the reliability of these parameters by using bootstrap replicates. Furthermore, PK parameters were determined in healthy beagle dogs, which may differ from those in patients with renal dysfunction, as previously reported in humans (37). Monaghan et al. (38) demonstrated that similar to that of CMZ, the blood concentration of ampicillin, an antibiotic that is mainly excreted renally, can increase in dogs with renal diseases. Such increased antibiotic concentrations may result in increased efficacy of the drug. Lastly, MIC-based PK-PD analysis was performed in this study; therefore, we did not consider other PD parameters, such as multiple PD parameters and kill rate (39).
To conclude, we estimated the COPD of CMZ when administered with regimens of 40 mg/kg q6h, q8h, and q12h using MCS and calculated the CFR based on the MIC distribution of wild-type ESBL-E. Our results indicated that a CMZ breakpoint lower than the CLSI for humans is preferable for dogs. Furthermore, a CMZ regimen of 40 mg/kg q6h could be a treatment option for dogs infected with ESBL-producing Escherichia coli and Klebsiella pneumoniae. We believe that these data provide a basis for the use of CMZ in dogs with ESBL-E infections.
Data availability statement
The raw data supporting the conclusions of this article will be made available by the authors, without undue reservation.
Ethics statement
The animal study was approved by Tottori University Animal Use Committee. The study was conducted in accordance with the local legislation and institutional requirements.
Author contributions
MK: Data curation, Investigation, Methodology, Writing – original draft. TM: Conceptualization, Formal analysis, Software, Visualization, Writing – review & editing. HU: Data curation, Investigation, Methodology, Writing – review & editing. MY: Data curation, Investigation, Methodology, Writing – review & editing. KH: Conceptualization, Data curation, Formal analysis, Funding acquisition, Investigation, Methodology, Software, Visualization, Writing – original draft, Writing – review & editing.
Funding
The author(s) declare financial support was received for the research, authorship, and/or publication of this article. This work was supported by JPSP KAKENHI (grant #21K05917).
Conflict of interest
The authors declare that the research was conducted in the absence of any commercial or financial relationships that could be construed as a potential conflict of interest.
The author(s) declared that they were an editorial board member of Frontiers, at the time of submission. This had no impact on the peer review process and the final decision.
Publisher’s note
All claims expressed in this article are solely those of the authors and do not necessarily represent those of their affiliated organizations, or those of the publisher, the editors and the reviewers. Any product that may be evaluated in this article, or claim that may be made by its manufacturer, is not guaranteed or endorsed by the publisher.
References
1. Pitout, JD, and Laupland, KB. Extended-spectrum beta-lactamase-producing Enterobacteriaceae: an emerging public-health concern. Lancet Infect Dis. (2008) 8:159–66. doi: 10.1016/S1473-3099(08)70041-0
2. Castanheira, M, Simner, PJ, and Bradford, PA. Extended-spectrum β-lactamases: an update on their characteristics, epidemiology and detection. JAC Antimicrob Resist. (2021) 3:dlab092. doi: 10.1093/jacamr/dlab092
3. Salgado-Caxito, M, Benavides, JA, Adell, AD, Paes, AC, and Moreno-Switt, AI. Global prevalence and molecular characterization of extended-spectrum β-lactamase producing Escherichia coli in dogs and cats - a scoping review and meta-analysis. One Health. (2021) 12:100236. doi: 10.1016/j.onehlt.2021.100236
4. Huang, C, Shi, Q, Zheng, B, Ji, J, Ying, C, Yu, X, et al. Simulating moxalactam dosage for extended-spectrum β-lactamase-producing Enterobacteriaceae using blood antimicrobial surveillance network data. Infect Drug Resist. (2019) 12:1199–208. doi: 10.2147/IDR.S193712
5. Karaiskos, I, and Giamarellou, H. Carbapenem-sparing strategies for ESBL producers: when and how. Antibiotics (Basel). (2020) 9:61. doi: 10.3390/antibiotics9020061
6. Roscetto, E, Varriale, C, Galdiero, U, Esposito, C, and Catania, MR. Extended-spectrum β-lactamase-producing and carbapenem-resistant Enterobacterales in companion animals and animal-assisted intervention dogs. Int J Environ Res Public Health. (2021) 18:12952. doi: 10.3390/ijerph182412952
7. Neu, HC. β-Lactam antibiotics: structural relationships affecting in vitro activity and pharmacologic properties. Rev Infect Dis. (1986) 8:S237–59. doi: 10.1093/clinids/8.supplement_3.s237
8. Jones, RN. Cefmetazole (CS-1170), a "new" cephamycin with a decade of clinical experience. Diagn Microbiol Infect Dis. (1989) 12:367–79. doi: 10.1016/0732-8893(89)90106-5
9. Hamada, Y, Matsumura, Y, Nagashima, M, Akazawa, T, Doi, Y, and Hayakawa, K. Retrospective evaluation of appropriate dosing of cefmetazole for invasive urinary tract infection due to extended-spectrum β-lactamase-producing Escherichia coli. J Infect Chemother. (2021) 27:1602–6. doi: 10.1016/j.jiac.2021.07.009
10. Shimizu, T, Harada, K, Tsuyuki, Y, Kimura, Y, Miyamoto, T, Hatoya, S, et al. In vitro efficacy of 16 antimicrobial drugs against a large collection of β-lactamase-producing isolates of extraintestinal pathogenic Escherichia coli from dogs and cats. J Med Microbiol. (2017) 66:1085–91. doi: 10.1099/jmm.0.000535
11. Kusumoto, M, Kanao, Y, Narita, H, Jitsuiki, M, Iyori, K, Tsunoi, M, et al. In vitro efficacy of cephamycins against multiple extended-spectrum β-lactamase-producing Klebsiella pneumoniae, Proteus mirabilis, and Enterobacter cloacae isolates from dogs and cats. J Vet Med Sci. (2023) 85:653–6. doi: 10.1292/jvms.23-0052
12. Sipahi, N, Kekeç, AI, and Halaç, B. In vitro effect of some essential oils against multiple antibiotic-resistant bacteria from cats and dogs. Pak Vet J. (2022) 42:561–5. doi: 10.29261/pakvetj/2022.055
13. Bradley, JS, Garonzik, SM, Forrest, A, and Bhavnani, SM. Pharmacokinetics, pharmacodynamics, and Monte Carlo simulation: selecting the best antimicrobial dose to treat an infection. Pediatr Infect Dis J. (2010) 29:1043–6. doi: 10.1097/INF.0b013e3181f42a53
14. Trang, M, Dudley, MN, and Bhavnani, SM. Use of Monte Carlo simulation and considerations for PK-PD targets to support antibacterial dose selection. Curr Opin Pharmacol. (2017) 36:107–13. doi: 10.1016/j.coph.2017.09.009
15. Ohmori, T, Suzuki, A, Niwa, T, Ushikoshi, H, Shirai, K, Yoshida, S, et al. Simultaneous determination of eight β-lactam antibiotics in human serum by liquid chromatography-tandem mass spectrometry. J Chromatogr B Analyt Technol Biomed Life Sci. (2011) 879:1038–42. doi: 10.1016/j.jchromb.2011.03.001
16. Ministry of Health, Labour and Welfare. Guideline on bioanalytical method validation in pharmaceutical development. (2013). Available at: https://www.nihs.go.jp/drug/BMV/250913_BMV-GL_E.pdf
17. Ikawa, K, and Tanaka, J. Introduction to pharmacokinetic analysis -focus on phase I study. Jpn J Biomet. (2015) 36:S3–S18. doi: 10.5691/jjb.36.S3
18. Jaki, T, and Wolfsegger, MJ. Estimation of pharmacokinetic parameters with the R package PK. Pharmaceut Statist. (2010) 10:284–8. doi: 10.1002/pst.449
19. Craig, WA. Pharmacokinetic/pharmacodynamic parameters: rationale for antibacterial dosing of mice and men. Clin Infect Dis. (1998) 26:1–10. doi: 10.1086/516284
20. Papich, MG. Pharmacokinetic-pharmacodynamic (PK-PD) modeling and the rational selection of dosage regimens for the prudent use of antimicrobial drugs. Vet Microbiol. (2014) 171:480–6. doi: 10.1016/j.vetmic.2013.12.021
21. Murakawa, T, Sakamoto, H, Fukada, S, Nakamoto, S, Hirose, T, Itoh, N, et al. Pharmacokinetics of ceftizoxime in animals after parenteral dosing. Antimicrob Agents Chemother. (1980) 17:157–64. doi: 10.1128/AAC.17.2.157
22. Jitaree, K, Sathirakul, K, Houngsaitong, J, Asuphon, O, Saelim, W, Thamlikitkul, V, et al. Pharmacokinetic/pharmacodynamic (PK/PD) simulation for dosage optimization of colistin against carbapenem-resistant Klebsiella pneumoniae and carbapenem-resistant Escherichia coli. Antibiotics (Basel). (2019) 8:125. doi: 10.3390/antibiotics8030125
23. Cagnardi, P, Cesare, FD, Toutain, PL, Bousquet-Mélou, A, Ravasio, G, and Villa, R. Population pharmacokinetic study of cefazolin used prophylactically in canine surgery for susceptibility testing breakpoint determination. Front Pharmacol. (2018) 9:1137. doi: 10.3389/fphar.2018.01137
24. Cómitre, MDV, Cortellini, S, Cherlet, M, Devreese, M, Roques, BB, Bousquet-Melou, A, et al. Population pharmacokinetics of intravenous amoxicillin combined with clavulanic acid in healthy and critically ill dogs. Front Vet Sci. (2021) 8:770202. doi: 10.3389/fvets.2021.770202
25. Borin, MT, Peters, GR, and Smith, TC. Pharmacokinetics and dose proportionality of cefmetazole in healthy young and elderly volunteers. Antimicrob Agents Chemother. (1990) 34:1944–8. doi: 10.1128/AAC.34.10.1944
26. Shah, S, Barton, G, and Fischer, A. Pharmacokinetic considerations and dosing strategies of antibiotics in the critically ill patient. J Intensive Care Soc. (2015) 16:147–53. doi: 10.1177/1751143714564816
27. Clinical Laboratory and Performance Standards Institute. Performance standards for antimicrobial susceptibility testing. 33rd ed. Wayne, PA: CLSI Supplement M100 (2023).
28. Wang, G, Yu, W, Cui, Y, Shi, Q, Huang, C, and Xiao, Y. Optimal empiric treatment for KPC-2-producing Klebsiella pneumoniae infections in critically ill patients with normal or decreased renal function using Monte Carlo simulation. BMC Infect Dis. (2021) 21:307. doi: 10.1186/s12879-021-06000-2
29. Katayama, M, Seki, T, Takei, Y, and Takahira, A. Preputial reconstruction and urethrostomy after subtotal penile amputation in a dog. J Hell Vet Med Soc. (2017) 68:669–74. doi: 10.12681/jhvms.16072
30. Kanno, N, Hayakawa, N, Suzuki, S, Harada, Y, Yogo, T, and Hara, Y. Changes in canine C-reactive protein levels following orthopaedic surgery: a prospective study. Acta Vet Scand. (2019) 61:33. doi: 10.1186/s13028-019-0468-y
31. Mochizuki, Y, Mikawa, S, Kurata, K, Sugimoto, K, Sakoya, H, Ohnisi, A, et al. Local hemodynamic changes immediately after correction of an aberrant right subclavian artery in a dog: a contrast computed tomographic study. Vet Sci. (2021) 8:104. doi: 10.3390/vetsci8060104
32. Moe, JB, Piper, RC, Tanase, H, Sotani, K, and Manabe, J. Preclinical safety studies of cefmetazole. J Antimicrob Chemother. (1989) 23 (Suppl.D):125-9) 23:125–9. doi: 10.1093/jac/23.suppl_d.125
33. Masuda, H, Kimura, K, Okada, T, Matsunuma, N, Tanase, H, Maita, K, et al. Toxicological studies of CS-1170. I. Acute, subacute and chronic toxicities of CS-1170 in experimental animals. Ann Rep Sankyo Res Lab. (1978) 30:112–47.
34. McKinnon, PS, Paladino, JA, and Schentag, JJ. Evaluation of area under the inhibitory curve (AUIC) and time above the minimum inhibitory concentration (T>MIC) as predictors of outcome for cefepime and ceftazidime in serious bacterial infections. Int J Antimicrob Agents. (2008) 31:345–51. doi: 10.1016/j.ijantimicag.2007.12.009
35. Takemura, W, Tashiro, S, Hayashi, M, Igarashi, Y, Liu, X, Mizukami, Y, et al. Cefmetazole as an alternative as an alternative to carbapenems against extended-spectrum beta-lactamase-producing Escherichia coli infections based on in vitro and in vivo pharmacokinetics/pharmacodynamics experiments. Pharm Res. (2021) 38:1839–46. doi: 10.1007/s11095-021-03140-7
36. Komiya, M, Kikuchi, Y, Tachibana, A, and Yano, K. Absorption, distribution, metabolism and excretion of cefotetan (YM09330), a new broad spectrum cephamycin, in experimental animals. Chemotherapy. (1982) 30:106–18.
37. Ohkawa, M, Orito, M, Sugata, T, Shimamura, M, Sawaki, M, Nakashita, E, et al. Pharmacokinetics of cefmetazole in normal subjects and in patients with impaired renal function. Antimicrob Agents Chemother. (1980) 18:386–9. doi: 10.1128/AAC.18.3.386
38. Monaghan, KN, Labato, MA, and Papich, MG. Ampicillin pharmacokinetics in azotemic and healthy dogs. J Vet Intern Med. (2021) 35:987–92. doi: 10.1111/jvim.16026
Keywords: cefmetazole, extended-spectrum β-lactamase, Enterobacteriaceae, Monte Carlo Simulation, dog, pharmacokinetics, pharmacodynamics
Citation: Kusumoto M, Motegi T, Uno H, Yokono M and Harada K (2023) Pharmacokinetic–pharmacodynamic analysis of cefmetazole against extended-spectrum β-lactamase-producing Enterobacteriaceae in dogs using Monte Carlo Simulation. Front. Vet. Sci. 10:1270137. doi: 10.3389/fvets.2023.1270137
Edited by:
Guadalupe Virginia Nevárez-Moorillón, Autonomous University of Chihuahua, MexicoReviewed by:
Mashkoor Mohsin, University of Agriculture, Faisalabad, PakistanAneliya Milanova, Trakia University, Bulgaria
Copyright © 2023 Kusumoto, Motegi, Uno, Yokono and Harada. This is an open-access article distributed under the terms of the Creative Commons Attribution License (CC BY). The use, distribution or reproduction in other forums is permitted, provided the original author(s) and the copyright owner(s) are credited and that the original publication in this journal is cited, in accordance with accepted academic practice. No use, distribution or reproduction is permitted which does not comply with these terms.
*Correspondence: Kazuki Harada, k-harada@tottori-u.ac.jp
†These authors have contributed equally to this work