- 1Unidad de Anatomía, Histología y Patología Veterinaria, Escuela de Medicina Veterinaria, Facultad de Ciencias Naturales, Universidad San Sebastián, Campus Puerto Montt, Puerto Montt, Chile
- 2Departamento de Anatomía y Anatomía Patológica Comparadas y Toxicología, UIC Zoonosis y Enfermedades Emergentes ENZOEM, Universidad de Córdoba, Córdoba, Spain
- 3Departamento de Sanidad Animal (Área de Parasitología), UIC Zoonosis y Enfermedades Emergentes ENZOEM, Universidad de Córdoba, Córdoba, Spain
Fasciola hepatica is distributed worldwide, causing substantial economic losses in the animal husbandry industry. Human fasciolosis is an emerging zoonosis in Andean America, Asia, and Africa. The control of the disease, both in humans and animals, is based on using anthelmintic drugs, which has resulted in increased resistance to the most effective anthelmintics, such as triclabendazole, in many countries. This, together with the concerns about drug residues in food and the environment, has increased the interest in preventive measures such as a vaccine to help control the disease in endemic areas. Despite important efforts over the past two decades and the work carried out with numerous vaccine candidates, none of them has demonstrated consistent and reproducible protection in target species. This is at least in part due to the high immunomodulation capacity of the parasite, making ineffective the host response in susceptible species such as ruminants. It is widely accepted that a deeper knowledge of the host-parasite interactions is needed for a more rational design of vaccine candidates. In recent years, the use of emerging technologies has notably increased the amount of data about these interactions. In the present study, current knowledge of host-parasite interactions and their implication in Fasciola hepatica vaccine development is reviewed.
1 Introduction
Fasciolosis is a parasitic disease with worldwide distribution, excluding Antarctica. In livestock, it has major economic implications with estimated worldwide economic losses amounting to USD 3,200 million, including anthelmintic treatments, control of intermediate hosts (molluscicides), research, and the implication of economic losses in dairy and meat livestock production (1, 2).
Human fasciolosis has persisted since prehistoric times (3), and currently, it has a significant global health impact in specific geographic locations. The World Health Organization (WHO) has classified fasciolosis as a neglected tropical disease (4), and it is the most geographically distributed parasitic zoonosis (5, 6). F. hepatica human infections range between 2.4 and 17 million people (7), with 91 to 180 million people at risk of infection annually (8, 9).
Currently, the control of fasciolosis in ruminants continues to be based on management measures such as pasture rotation and the use of anthelmintics (10). The continued use of anthelmintics has resulted in an increase in parasite-resistant strains for the most effective and widely used flukicides, such as triclabendazole and albendazole (11, 12). Over the past three decades, there has been a rising interest in obtaining vaccines that help prevent and control fasciolosis in ruminants (13). However, the development of vaccines against fasciolosis has been slow, partly due to the great immunomodulatory capacity of the parasite. Hence, a better understanding of the parasite-host interactions is necessary for a more rational design of new vaccine candidates (14, 15).
2 Etiology and biological cycle of the parasite
Fasciolosis is caused by flukes of the genus Fasciola, known as liver flukes. The two species most implicated as the etiologic agents of fasciolosis are F. hepatica, which is distributed mainly in temperate climate regions, and F. gigantica, which is located in tropical regions. Further, hybrid forms have been described in regions where the two species coexist (16, 17). Real-time PCR (qPCR) targeting ITS1 rDNA, ITS2 rDNA, and 28S rDNA have been used to differentiate the two distinct genetic signatures representing each species (18–20). The epidemiological potential of hybridization and introgression between F. hepatica and F. gigantica remains unknown; therefore, it is important to use the correct terminology consistently and not use the two terms interchangeably (21).
The life cycle of Fasciola spp. is quite complex, involving several variations. In general, it involves one or more intermediate hosts, which are the mollusks. At least 20 species of the Lymnaeidae family have been reported as intermediate hosts (22, 23). The asexual larvae undergo several multiplications (24–26) before finally infecting a definitive host in which sexual reproduction occurs.
3 Pathogenesis
The penetration, migration, and localization of the parasites in the bile ducts exert a traumatic action that causes a series of lesions in the liver parenchyma and in the bile ducts (27). The newly excysted juveniles (NEJs) of Fasciola spp. penetrate the intestinal mucosa and can be found in the abdominal cavity 72 h after metacercaria ingestion. NEJs migrate through the peritoneum to the liver surface and present no clinical sinology in animals (28). The destination of the majority of NEJs is the left hepatic lobe, probably due to its anatomical proximity to the duodenum and the fact that they reach less of the other hepatic lobes. Sometimes, due to massive infestations, these juveniles can have an aberrant migration to other organs, such as the diaphragm and the lung, causing pneumonia and fibrinous pleurisy (29).
Fasciolosis pathogenesis occurs in two phases—the parenchymal and biliary phases. The parenchymal phase begins when the NEJs cross the liver capsule (Glisson's capsule), continuing with the migration of the juvenile stages through the liver parenchyma. This migration causes mechanical damage through abrasion by the tegument that presents spines that help maintain the parasite's position within the liver tissues and probably by-products secreted by migrating larvae. Several pathological processes occur simultaneously within the liver parenchyma, including the migration of juvenile stages that cause necrotic and hemorrhagic lesions, which, in turn, cause inflammatory reactions activating the immune system (30). This response can be found throughout the tortuous migrating trajectory of the parasites, suggesting that the excretion and secretion of these products remain in the tissue, attracting more infiltration of inflammatory cells of an immune nature (31). The biliary phase begins when the parasites enter the bile ducts, where they exert a combined mechanical and chemical action. Through the oral sucker, adult parasites cause mechanical damage while feeding on blood and the liver parenchyma adjacent to the duct. Macerated hepatocytes have been observed inside the sucker and pharynx (27), leading to erosion of the epithelium, trauma, focal rupture of the duct, and puncture of small blood vessels. The enlargement of the bile duct can be chemically induced (32), and it has been suggested that the amino acid proline, which is essential for the synthesis of collagen by fibroblasts, is also released in large quantities by the parasite (33, 34). These two actions exerted by the adult parasite cause a severe eosinophilic and granulomatous inflammatory response, particularly when eggs reach hepatic parenchyma (35), and marked hyperplasia of the bile ducts in which the parasites lodge (36).
The effect of these two phases causes a series of lesions in the liver parenchyma, which is widely correlated with the infective dose; a high dose causes more severe lesions that are more acute and even fatal. However, different studies carried out in sheep (35) and goats (37) have also shown that small repetitive doses (trickle infections) caused more severe hepatic damage than a single dose using the same total number of metacercariae. These findings suggest that the mechanical and enzymatic activities of the parasite may be the initial cause of liver damage. Therefore, the immune response or healing, as well as simultaneous infection at different stages and the immune response to the first infection, play an important role in the pathogenesis of fasciolosis (31).
4 Host immune response
4.1 Innate immune response
The initial recognition of NEJs takes place within the epithelial mucosa of the intestinal tract with extensive activation. The response to NEJs can occur through the recognition of glycosylated protein and carbohydrate residues that behave as tegumental antigens and induce T-cell proliferation through dendritic cell activation (38, 39). Excretory secretory products containing antigens released by F. hepatica (FhESP) can also induce a response of bovine macrophages, which is partially TLR4-dependent (40, 41).
The function of mast cells is not really defined, nor is there evidence that it is protective (42). These cells are residents of tissues that respond to activation of both the innate and acquired immune systems by producing and releasing different inflammatory mediators present in their cytoplasmic granules, prostaglandins, leukotrienes, and certain cytokines such as tumor necrosis factor-alpha (TNF-α) or interleukin-4 (IL-4) (43). In addition, they can release certain active substances against parasites by binding the parasite antigen-IgE complexes with their high-affinity IgE receptors (44, 45). It is estimated that its role is more decisive in the initial stages (peritoneum) of the infection (42, 46, 47). However, it has been described in cattle that after getting infected by F. hepatica, there is little evidence of an increase in the percentage of basophils and mast cells (48, 49) and in peritoneal fluid in sheep (50). In contrast, F. gigantica infection in buffaloes induces increases in the number of mast cells in the hepatic inflammatory infiltrate (51). In numerous parasitic processes, we can find a population of resident intraepithelial mast cells responsible for rapid parasite rejection phenomena at the epithelial level (52–54). However, these cells have neither been described in the intestine after the migration of F. hepatica (30, 36) nor in bile cells such as macrophages and neutrophils, whose function is phagocytic and can release substances such as reagents derived from nitric oxide or active oxygen species that act directly against the parasite (55, 56). On the other hand, infection by F. hepatica provokes a Th2-type immune response with IgE production (57) and infiltration of eosinophils and mast cells in the liver (48).
Human neutrophils from patients with acute fasciolosis showed a greater phagocytic function compared to those in the chronic stage of infection (58). Similarly, neutrophils from chronically infected goats showed a poor phagocytic response compared to those from uninfected goats. This poor phagocyte response was correlated with fluke burdens (59). The role of neutrophils in protective responses has not been reported yet in fluke infections.
In cattle, sheep, and goats, F. hepatica induces liver and blood eosinophilia, and F. gigantica infection in sheep gives the same profile (60–62). However, vaccination of calves and goats showing protection had reduced eosinophil counts (30, 63), which may be due to the lower fluke burdens and hepatic lesions in partially protected animals. In acute stages of F. hepatica infection, a dramatic increase of eosinophils has been described in the peritoneal cavity (50, 64) as well as in hepatic lesions, both during the migratory stage (30, 36, 65, 66) and during the chronic stage (35). Eosinophils have been shown to mediate antibody-dependent cell cytotoxicity (ADCC) against F. hepatica in rats (42). In Indonesian thin-tailed (ITT) sheep which display resistance to F. gigantica but not F. hepatica, it has been observed that ADCC by eosinophils plays a role (ex vivo) in killing F. gigantica but not F. hepatica newly excysted juveniles (NEJs) (56). However, peripheral eosinophilia was not related to resistance to F. gigantica, suggesting that this cell type is effective only within the gut or peritoneal cavity but not the liver, at least in ITT sheep (67).
Peritoneal macrophages from ITT sheep have also been shown to kill F. gigantica but not F. hepatica by ADCC (56, 68). This mechanism occurs by attaching effector cells with NEJs in the presence of serum from infected sheep. Macrophages participating in the effective ADCC mechanism against F. gigantica showed increased levels of superoxide radicals than those participating in ineffective ADCC against F. hepatica, suggesting oxygen radicals play a role in killing F. gigantica NEJs (56). It has been reported that in calves protected by experimental vaccination, ADCC mediated by macrophages is nitric oxide-mediated and induces a Th1 cytokine response relying on IgG2a (69). In vitro studies have revealed that bovine macrophages were able to kill NEJs in the presence of serum from infected animals. However, NEJs were able to produce molecules such as a family of TGF-like molecules (FhTLM) that significantly reduces ADCC. These macrophages showed features of alternative activation with the expression of high levels of IL-10 (70). In non-protected animals, it has been observed that NEJs induce alternative (M2) activation of macrophages and secrete the regulatory cytokines IL-10 and transforming growth factor-beta (TGF-β) during the peritoneal migration (71–73). M2-activated macrophages have an important role in tissue repair, but they have a reduced capacity to kill NEJs (41, 70).
4.2 Adaptive immune response
B-cells have shown importance in Fasciola spp.-infected animals as well as in those that have been previously vaccinated (74), highlighting the increase in CD19+ B-cells at the level of hepatic lymph nodes, increasing the recruitment of these cells (66). In cattle, sheep, and goats, IgG1 is the dominant antibody, raising at 4–5 weeks post-infection (wpi) and reaching peaks at 12–15 wpi (37, 75, 76). An increase in specific IgG2 has been shown to correspond to vaccine-induced protection, and an increase in IgG1 has been associated with a non-protective Th2 response (76–78). IgA specific for fluke antigens has not been detected in serum (75), but it has been found in the bile and liver of infected cattle (51), where this immunoglobulin may participate in activating eosinophils to kill NEJs by ADCC (49). Despite this interesting suggestion, few studies have investigated the presence of IgA in bile and liver in both experimental and natural infections.
The immune response exerted during the early stages of fasciolosis is generally regarded as a mixed Th1/Th2 response displaying an increase of certain cytokines such as IFN-γ, IL-4, IL-10, and TGF-β. As the infection progresses, a Th2 response is amplified in conjunction with suppression of Th1 inflammation, thus allowing a prolonged infection that may be dependent on IL-4 (79). In the early stages of sheep and cattle F. hepatica infection, both IFN-γ and IL-10 are increased, confirming the initial mixed immune response (75, 80, 81). When the infection progresses, a Th2 response is amplified in conjunction with suppression of Th1 response with reduced IFN-γ and increased IL-4 levels (79). In the early stages of bovine F. hepatica infection, both IFN-γ and IL-10 are increased, corroborating the idea that the initial immune response is mixed (75). Buffaloes with both primary and secondary infection of F. gigantica also showed a mixed Th1/Th2 response in serum with elevated IFN-γ, IL-4, IL-5, and TGF-β during the early stages of infection. In contrast, when the infection progressed, the Th2 response was dominant (82). The Th1/Th2 response was not the same in different compartments—in sheep liver, IFN-γ increased during the early stages of infection (80, 81), and it remained high during chronic states of infections (81). At the same time, in the hepatic lymph nodes, IFN-γ was reduced both in infected and reinfected animals in acute and chronic stages of infections (81). The high levels of IFN-γ reported in the liver during acute and chronic stages of F. hepatica infections contrast with the downregulation of this cytokine in PBMC (83) and hepatic lymph nodes (80, 81) and could be due to a response to hepatic necrosis caused by migrating or adult flukes and granulomata formation.
5 Immunomodulation strategies
The inflammatory reaction in fasciolosis is one of the points to be treated primarily to understand the immune response and its evasion. Since metacercariae are excysted in the gut lumen, NEJs are exposed to the host immune response to kill the parasite. However, Fasciola spp. has developed a variety of strategies to evade the host response in the different compartments where they stay during the early and late stages of infection, which allows the parasite to live for years within the host. Some of these strategies may be considered passive, as the protection conferred by the tegument, which consists of a syncytial layer covering the entire body of the parasite, formed by a plasma membrane that serves as a support for the outer glycocalyx and a basement membrane that is connected through channels. These structures allow the passage of the components needed for the replacement of the tegument. The rapid replacement of the glycocalyx that covers the tegument—which takes place every 2 to 3 h—may also be an obstacle for products released by inflammatory cells to reach the parasite tegument (84), which is composed of at least 369 proteins. Additionally, the presence of abundant N-glycosylated proteins and glycolipids has made it difficult to characterize its physiological and immune regulatory functions (85).
The majority of strategies used by the parasite to evade the host response may be considered active since they imply the release of a large amount of parasite molecules into the parasite vicinity. These molecules can be released free or within extracellular vesicles (EVs) that are covered by a membrane, and they can be internalized by the host cells, causing their modulation (84, 85). EVs are produced by all developmental stages of F. hepatica, and they are considered efficient transporters of parasite molecules to different host compartments, preventing the action of antibodies due to the membrane surrounding the parasite molecules contained in EVs (86). In EVs from F. hepatica, up to 618 proteins have been identified, which gives us an idea of how important EVs are for the parasite to interact with the host (87).
Fasciola spp. not only use proteins to modulate the host immune response, but EVs also contain microRNAs (miRNAs), molecules with modulating gene expression capacity. miRNAs are abundant in both metacercariae, juvenile and adult F. hepatica worms and may play a main role in regulating the developmental and metabolic processes of the parasite, as well as in host-parasite interactions (88–90). The miRNA content in the EVs is different when they are produced by adult or juvenile parasites, leading to different influences in the host cells. These data support the hypothesis that miRNAs are the mediators of the previously demonstrated immune modulatory function of the EVs. However, current data do not allow a fundamental understanding of their regulatory mechanisms in different processes of host-parasite interaction (88–91).
Another mechanism used by liver fluke to survive, migrate, obtain nutrients, and evade the immune response of the host, is the release of excretory secretory products (ESP) (92). FhESP from adult F. hepatica contains up to 160 different proteins, including proteases such as cathepsins B and L (FhCB and FhCL), leucine aminopeptidase and carboxypeptidase, fatty acid-binding protein (FABP), and the F. hepatica saposin-like protein (FhSAP), all of them necessary for its metabolism (93) (Table 1). FhESP also contains numerous antioxidant enzymes to protect the parasite from reactive oxygen species released by eosinophils and macrophages, such as superoxide dismutase (SOD), glutathione-S-transferase (GST), thioredoxin peroxidase (TPx), and peroxiredoxin (Px) (Table 1). These enzymes not only participate in inactivating reactive oxygen species but also in several important metabolic processes important for parasite survival, such as the excyst of the metacercariae, tissue migration, feeding, and immune evasion (92, 105, 106). Some strategies that Fasciola spp. use to evade the host response are discussed below.
5.1 Parasite movement
During the hepatic migration, it has been reported that some larvae show a heavy inflammatory infiltrate, mainly composed of eosinophils attached to the parasite cuticula and in the vicinity of the parasite. However, in other larvae, no inflammatory reaction was found in their vicinity, but necrotic tract and inflammation were observed 2–3 mm behind them (30, 36). It has been suggested that when the parasites are disturbed by the inflammatory reaction, they move ahead, leaving the inflammatory cells behind them (66).
5.2 Apoptosis of effector and immune cells
There is an intimate connection between the inflammatory response and the immune response when suffering from fasciolosis. The innate immune response determines the cell populations involved in the inflammatory response by attracting and activating inflammatory cells (107). Eosinophils play a key role in the host response to Fasciola spp infection, as suggested by the rapid increase of this cell type in blood, peritoneum, and liver during the early migration of juveniles in sheep (35, 60), cattle (48), and rodents (108). In vitro studies have reported that FhESP antigens from F. hepatica induce apoptosis of rat eosinophils and macrophages (109, 110). In vivo studies have described apoptosis in eosinophils in the liver inflammatory infiltrate during the acute and chronic phases of infection in sheep (65) and the migratory stage in a relevant percentage of peritoneal macrophages, eosinophils, and lymphocytes (50). Increased expression of the pro-apoptotic gene in peripheral blood mononuclear cells of infected sheep and cattle has also been reported (111, 112). More recently, the role of a variety of F. hepatica molecules in the induction of apoptosis has been investigated; some of them have been identified as glutathione S-transferase Omega type (GSTO1), which down-regulated the ratio of Bcl-2/Bax and induced increased expression of caspase-3 and apoptosis of macrophages in vitro (94). Recombinant cystatin from F. hepatica (rFhCystatin) has been shown to induce apoptosis of murine macrophages (104), and fatty acid binding protein (Fh12) induced apoptosis of murine dendritic cells in in vitro studies (99).
5.3 Modulation of Th1/Th2 and Th17 responses
The immune response mounted during the early stages of fasciolosis is generally a mixed Th1/Th2 response with elevated levels of cytokines such as IFN-γ, IL-4, IL-10, and TGF-β. As the infection progresses, a Th2 response is amplified in conjunction with the suppression of Th1 cytokine production, particularly IFN-γ, which facilitates parasite survival in mice, cattle, and sheep infected with F. hepatica (41, 79–81, 113). A similar Th1/Th2 dynamic has been reported in buffaloes infected with F. gigantica (82). It has been reported that a variety of parasitic molecules are able to produce modulation of the Th1/Th2 host response; thus, rFhCystatin induced reduced production of IL-6 and TNF-α and increased production of IL-10 and TGF-β in murine macrophages (104). F. hepatica Kunitz-type molecule induced suppression of the Th1 and Th17 responses in murine and human dendritic cells (DC) in in vitro studies (97).
5.4 Modulation of macrophage and antigen-presenting cell functions
In the early stages of F. hepatica infection, the recruitment of macrophages and alternative (M2) activation in the peritoneal cavity has been reported in rats at 24 h post-infection (hpi) (71) and at 48 hpi in mice (114). Moreover, FhESP induced M2 activation of peritoneal macrophages in mice (114). In sheep, marked M2 activation has been described by gene expression in PBMC at 7 dpi (83), although peritoneal sheep macrophages showed M2 activation at 24 hpi (73). In cattle, F. hepatica also induced M2-activation of macrophages (115, 116). M2-activated macrophages participate in tissue repair, but they show limited ability to control helminth infections (117). F. hepatica possesses FhTLM, which is highly expressed in NEJs and unembryonated eggs. It has been reported that FhTLM induces the differentiation of the monocyte-derived macrophages to M2 activation with increased production of IL-10, arginase-1, mannose receptor, and PD-L1 (70).
It has been reported that different antigenic preparations of this parasite, such as total extract, F. hepatica tegumental antigen (FhTeg), and Fasciola hepatica ESP, decrease the activation state of dendritic cells (DCs) in mice (118–121), and F. gigantica ESP induces the modulation of buffalo DCs (122). More specifically, it has been reported that FhTeg induces DC modulation, provoking the absence of T-cell Th1 cytokine response and proliferative activity (38). Glycan products produced by F. hepatica have also been reported to induce modulation of DC maturation, resulting in increased production of IL-10 and IL-4 during infection, inducing a Th2/regulatory-polarized immune response (40, 79, 113, 123, 124). In addition, F. hepatica cathepsin L1 (FhCL1), glutathione S-transferase (FhGST), and Kunitz-type molecule participate in the modulation of DCs, leading to the suppression of the adaptive immune responses, Th1, and/or Th17 (40, 97). F. hepatica-infected sheep showed increased numbers of DCs in the hepatic lymph nodes but reduced expression of MHC class II and CD83, suggesting suppression of the antigen-presentation process in lymphocytes both in the early and late stages of infection (125).
5.5 Expansion of T regulatory cells
F. hepatica-infected sheep and goats showed expansion of T regulatory cells (Treg) Foxp3+ during early and late stages of infection in the liver and hepatic lymph nodes (50, 81, 126). Moreover, the increase of Foxp3+ cells was more severe in the vicinity of hyperplastic bile ducts during chronic states of infections (50). This expansion of Foxp3+ Treg has been related to IL-10 and parasite survival (127, 128).
6 Vaccine development
Over the past two decades, there have been considerable advances in identifying potential vaccine molecules for the control of fasciolosis in livestock. However, despite some promising results with some vaccine candidates in ruminants, a consistent efficacy required for commercialization has not yet been reached (13). A major obstacle to developing vaccines for fasciolosis is the immune suppression/modulation induced by Fasciola spp. that prevents the induction of a protective immune response (Figure 1), evidenced by the lack of immunity observed in naturally and experimentally infected sheep (31, 70, 129). In cattle, natural or experimental infections have been shown to induce certain protection against reinfection, which is maintained long-term (up to 26 weeks post-infection). It has been attributed to the severe fibrosis induced by the primary infection that makes the hepatic migration difficult during the secondary infection (130) or by an increase of intestinal eosinophil and mucosal mast cells (47). Some studies have also reported evidence that protection against F. hepatica is inducible in rats, sheep, or cattle by passive transfer of immune sera and cells (131). However, other studies have reported no resistance to reinfection measured by fluke burdens (75). Moreover, no differences in fluke burdens, fecal egg counts, humoral response (specific IgG1 and IgG2), and cell-mediated immune response (IFN-γ production) were reported in calves challenged with F. hepatica after single or trickle infection (48, 57, 75) suggesting that reinfections do not induce protection. Experimental studies reported no protection against reinfection in sheep (35, 81, 132) and goats (37), although the host response was different; thus, primo-infected sheep showed a mixed Th1/Th2/Th17 response while reinfected ones presented a more Th2 polarized response (81) and a lower humoral response (132).
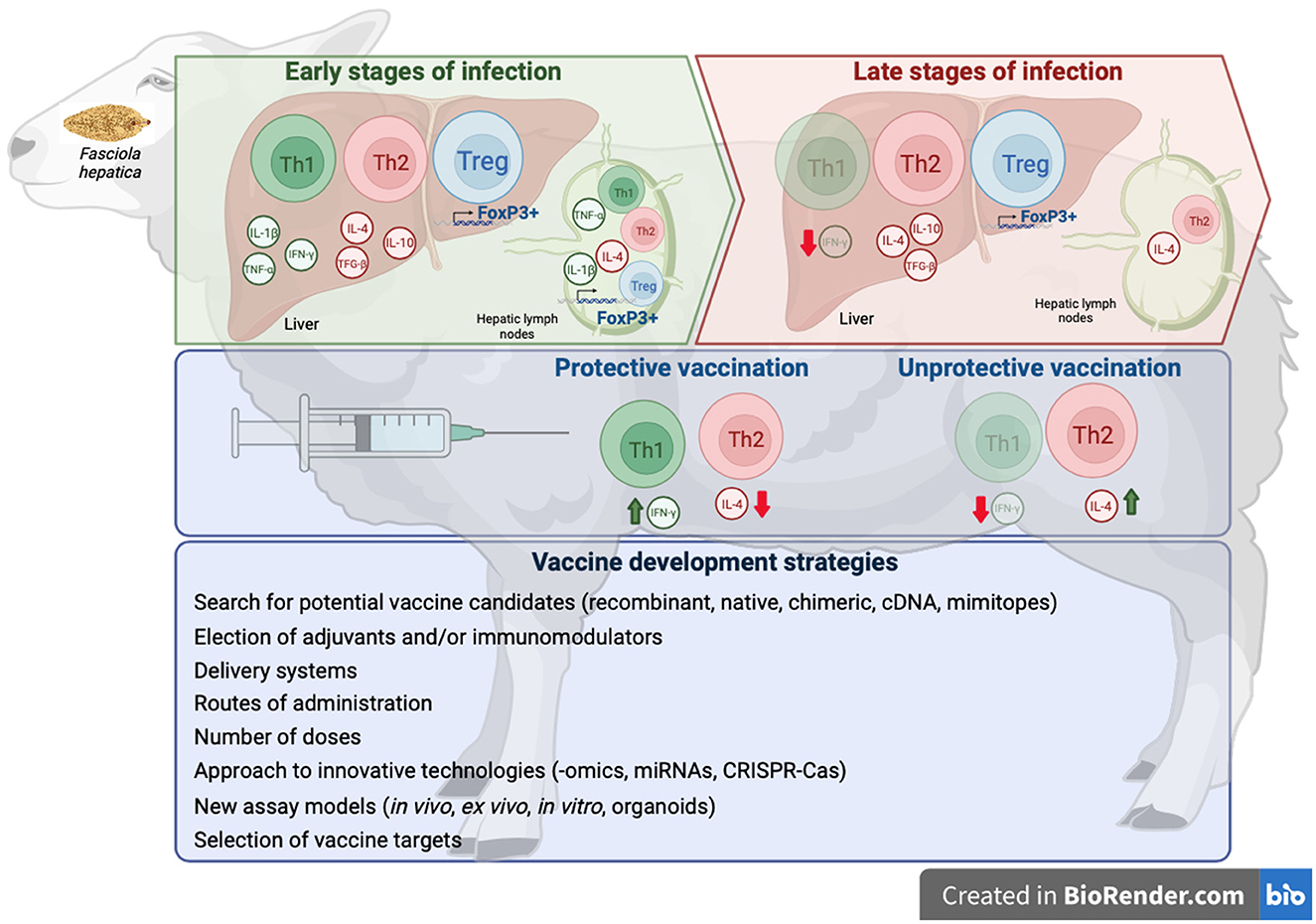
Figure 1. Scheme of immune responses exerted at early and late stages of F. hepatica infections, immune responses induced by protective and unproductive vaccines against F. hepatica, and strategies to develop effective vaccines. Created with BioRender.com.
It has been reported that in protective vaccines in sheep (133) and goats (134), a mixed Th1/Th2 response was found with higher levels of IFN-γ and lower levels of IL-4 in vaccinated groups than in the infected control group (133). In sheep immunized with a non-protective vaccine, the host immune response showed a predominantly Th2 profile during chronic stages of the infection, similar to that found in non-vaccinated and infected animals (80). The challenge is to identify the specific antigens that are the targets of this protective immunity and incorporate these in vaccine formulations that induce a mixed Th1/Th2 response to enhance vaccine efficacy (135). It has been estimated that a vaccine with an efficacy of 50–60% in fluke reduction would likely be beneficial in numerous countries to significantly reduce economic losses, and it also would have a positive impact on epidemiology by reducing eggs in pasture (13).
Several strategies have been used to design vaccine candidates for fasciolosis in livestock. The first vaccine trials used native proteins isolated using conventional biochemical methods from the excreted/secreted (ES) proteins of adult parasites (136, 137). Despite good protection being found in sheep and cattle in these trials using native FhCL1 and FhGST, the use of native proteins in a commercial vaccine for fasciolosis in livestock is not feasible, which is why the majority of subsequent vaccine trials have been carried out using recombinant proteins of different stages of the parasite (13). Some vaccine trials using recombinant proteins reported high protection of up to 89% in fluke reduction (Tables 2, 3); however, this high protection has not been reproducible in different labs and conditions. A combination of recombinant vaccines (cocktail vaccines) has also been used recently with variable efficacy (Table 3). The majority of vaccine trials have used the subcutaneous or intramuscular administration route. However, a few trials have used mucosal vaccine delivery with promising results. For instance, Norbury et al. (154) administered a cocktail vaccine containing FhCL5 and FhCB2 by an intranasal method in sheep, obtaining a 40.5% fluke reduction and a 92% egg viability reduction, while the same vaccine administered intramuscularly did not induce protection. The oral route has also been used to administer freeze-dried transgenic lettuce expressing the cysteine proteinase of F. hepatica (CPFhW) in sheep and cattle, inducing significant protection in cattle (56.2%) and 35.5% fluke reduction (not significant) in sheep (142).
Most vaccine trials in ruminants have used proteases, antioxidant enzymes, or fatty acid-binding proteins as antigens (Tables 2, 3). However, these proteins are quite abundant in Fasciola spp, and blocking one or several of them by a vaccine probably does not cause serious problems to the worm since it has other proteins with similar functions. This might be a reason for the limited efficacy obtained in the numerous vaccine trials conducted with these antigens in ruminants.
7 Conclusion and remarks
The slow progress to date in developing a protective vaccine to be used in the control of fasciolosis in livestock suggests that new approaches should be investigated, such as the use of new antigens, evaluation of immunity induced by recombinant proteins, use of different adjuvants, formulations, and delivery systems. Despite important advances in the knowledge of host-parasite interactions in fasciolosis, a more rational vaccine candidate design requires a deeper knowledge of the mechanisms and molecules involved in host-parasite cross-talk in relevant target host species (sheep, cattle, goats, buffalo). The progress of the -omics technologies and the immunoinformatic/immunoproteomic approaches should provide useful data in the next few years. An example is the new proteomic technologies applied to NEJs after crossing the gut (158) or during the early stages of hepatic migration, which may be useful to select new vaccine candidates directed against NEJs, a stage of the parasite that it is more exposed to the host immune system than adult ones located within the bile ducts.
Author contributions
LF-V: Writing—original draft. MR-C: Writing—original draft. GH-T: Writing—review & editing. ÁM-M: Writing—review & editing. FM-M: Writing—review & editing. RZ: Writing—review & editing. LB: Writing—review & editing. PR-M: Writing—review & editing. VM-H: Conceptualization, Writing—original draft, Writing—review & editing. JP: Conceptualization, Writing—original draft, Writing—review & editing.
Funding
The author(s) declare that financial support was received for the research, authorship, and/or publication of this article. The work has been supported by National Grant PID2019-108782RB-C21. VM-H was supported by the financial support of the Regional Government of Andalusia (Junta de Andalucía, Consejería de Conocimiento, Investigación y Universidad)-FEDER (project P18-RTJ-1956).
Conflict of interest
The authors declare that the research was conducted in the absence of any commercial or financial relationships that could be construed as a potential conflict of interest.
Publisher's note
All claims expressed in this article are solely those of the authors and do not necessarily represent those of their affiliated organizations, or those of the publisher, the editors and the reviewers. Any product that may be evaluated in this article, or claim that may be made by its manufacturer, is not guaranteed or endorsed by the publisher.
References
1. Mehmood K, Zhang H, Sabir AJ, Abbas RZ, Ijaz M, Durrani AZ, et al. A review on epidemiology, global prevalence and economical losses of fasciolosis in ruminants. Microb Pathog. (2017) 109:253–62. doi: 10.1016/j.micpath.2017.06.006
2. Hayward AD, Skuce PJ, McNeilly TN. The influence of liver fluke infection on production in sheep and cattle: a meta-analysis. Int J Parasitol. (2021) 51:913–24. doi: 10.1016/j.ijpara.2021.02.006
3. Dittmar K, Teegen WR. The presence of Fasciola hepatica (liver-fluke) in humans and cattle from a 4,500 year old archaeological site in the Saale-Unstrut valley, Germany. Mem Inst Oswaldo Cruz. (2003) 98(Suppl 1):141–3. doi: 10.1590/S0074-02762003000900021
4. WHO. Neglected Tropical Diseases. Geneva: World Health Organization (2017). Available online at: http://www.who.int/neglected_diseases/diseases/en/ (accessed May 30, 2020).
5. Cabada MM, White AC Jr. New developments in epidemiology, diagnosis, and treatment of fascioliasis. Curr Opin Infect Dis. (2012) 25:518–22. doi: 10.1097/QCO.0b013e3283567b7e
6. Nyindo M, Lukambagire AH. Fascioliasis: an ongoing zoonotic trematode infection. Biomed Res Int. (2015) 2015:786195. doi: 10.1155/2015/786195
7. Mas-Coma S, Valero MA, Bargues MD. Fascioliasis. Adv Exp Med Biol. (2019) 1154:71–103. doi: 10.1007/978-3-030-18616-6_4
8. Mas-Coma S. Epidemiology of fascioliasis in human endemic areas. J Helminthol. (2005) 79:207–16. doi: 10.1079/JOH2005296
9. Keiser J, Utzinger J. Food-borne trematodiases. Clin Microbiol Rev. (2009) 22:466–83. doi: 10.1128/CMR.00012-09
10. Carson A, Jones B, Grove-White D. Managing liver fluke on hill farms. Vet Rec. (2022) 191:115–7. doi: 10.1002/vetr.2105
11. Fairweather I. Reducing the future threat from (liver) fluke: realistic prospect or quixotic fantasy? Vet Parasitol. (2011) 180:133–43. doi: 10.1016/j.vetpar.2011.05.034
12. Carmona C, Tort JF. Fasciolosis in South America: epidemiology and control challenges. J Helminthol. (2017) 91:99–109. doi: 10.1017/S0022149X16000560
13. Spithill TW, Toet H, Rathinasamy V, Zerna G, Swan J, Cameron T, et al. Vaccines for fasciola: new thinking for an old problem. In:Dalton, JP, , editor. Fasciolosis 2nd edn. Cambridge: CABI Publishing (2022). p. 379–422. doi: 10.1079/9781789246162.0012
14. Beesley NJ, Caminade C, Charlier J, Flynn RJ, Hodgkinson JE, Martinez-Moreno A, et al. Fasciola and fasciolosis in ruminants in Europe: identifying research needs. Transbound Emerg Dis. (2018) 65(Suppl 1):199–216. doi: 10.1111/tbed.12682
15. Cwiklinski K, Drysdale O, López Corrales J, Corripio-Miyar Y, De Marco Verissimo C, Jewhurst H, et al. Targeting secreted protease/anti-protease balance as a vaccine strategy against the helminth Fasciola hepatica. Vaccines. (2022) 10:155. doi: 10.3390/vaccines10020155
16. Agatsuma T, Arakawa Y, Iwagami M, Honzako Y, Cahyaningsih U, Kang SY, et al. Molecular evidence of natural hybridization between Fasciola hepatica and F. gigantica. Parasitol Int. (2000) 49:231–8. doi: 10.1016/S1383-5769(00)00051-9
17. Lotfy WM, Brant SV, DeJong RJ, Le TH, Demiaszkiewicz A, Rajapakse RP, et al. Evolutionary origins, diversification, and biogeography of liver flukes (Digenea, Fasciolidae). Am J Trop Med Hyg. (2008) 79:248–55. doi: 10.4269/ajtmh.2008.79.248
18. Marcilla A, Bargues MD, Mas-Coma S. A PCR-RFLP assay for the distinction between Fasciola hepatica and Fasciola gigantica. Mol Cell Probes. (2002) 16:327–33. doi: 10.1006/mcpr.2002.0429
19. Alasaad S, Soriguer RC, Abu-Madi M, El Behairy A, Jowers MJ, Baños PD, et al. A TaqMan real-time PCR-based assay for the identification of Fasciola spp. Vet Parasitol. (2011) 179:266–71. doi: 10.1016/j.vetpar.2011.01.059
20. Calvani NED, Ichikawa-Seki M, Bush RD, Khounsy S, Šlapeta J. Which species is in the faeces at a time of global livestock movements: single nucleotide polymorphism genotyping assays for the differentiation of Fasciola spp. Int J Parasitol. (2020) 50:91–101. doi: 10.1016/j.ijpara.2019.12.002
21. Calvani NED, Šlapeta J. Fasciola gigantica and Fasciola Hybrids in Southeast Asia. In:Dalton, JP, , editor. Fasciolosis 2nd edn. Cambridge: CABI Publishing (2022). p. 423–60. doi: 10.1079/9781789246162.0013
22. Torgerson P, Claxton J. Epidemiology and control. In:Dalton JP, , editor. Fasciolosis. Wallingford: CAB International (1999). p. 113–49.
23. Correa AC, Escobar JS, Durand P, Renaud F, David P, Jarne P, et al. Bridging gaps in the molecular phylogeny of the Lymnaeidae (Gastropoda: Pulmonata), vectors of Fascioliasis. BMC Evol Biol. (2010) 10:381. doi: 10.1186/1471-2148-10-381
24. Wilson RA, Pullin R, Denison J. An investigation of the mechanism of infection by digenetic trematodes: the penetration of the miracidium of Fasciola hepatica into its snail host Lymnaea truncatula. Parasitology. (1971) 63:491–506. doi: 10.1017/S003118200008001X
25. Thomas AP. The natural history of the liver-fluke and the prevention of rot. J R Agric Soc. (1883) 19:276–305.
26. Thomas AP. The life history of the liver-fluke (Fasciola hepatica). Q J Microsc Sci. (1883) 23:99–133. doi: 10.1242/jcs.s2-23.89.99
27. Dawes B, Hughes DL. Fascioliasis: the invasive stages of Fasciola hepatica in mammalian hosts. Adv Parasitol. (1964) 2:97–168. doi: 10.1016/S0065-308X(08)60587-4
28. Dow C, Ross JG, Todd JR. The histopathology of Fasciola hepatica infections in sheep. Parasitology. (1968) 58:129–35. doi: 10.1017/S0031182000073480
29. Boray JC. Experimental fascioliasis in Australia. Adv Parasitol. (1969) 7:95–210. doi: 10.1016/S0065-308X(08)60435-2
30. Zafra R, Pérez-Écija RA, Buffoni L, Moreno P, Bautista MJ, Martínez-Moreno A, et al. Early and late peritoneal and hepatic changes in goats immunized with recombinant cathepsin L1 and infected with Fasciola hepatica. J Comp Pathol. (2013) 148:373–84. doi: 10.1016/j.jcpa.2012.08.007
31. Molina-Hernández V, Mulcahy G, Pérez J, Martínez-Moreno Á, Donnelly S, O'Neill SM, et al. Fasciola hepatica vaccine: we may not be there yet but we're on the right road. Vet Parasitol. (2015) 208:101–11. doi: 10.1016/j.vetpar.2015.01.004
32. Lopez P, Tuñon MJ, Gonzalez P, Diez N, Bravo AM, Gonzalez-Gallego J. Ductular proliferation and hepatic secretory function in experimental fascioliasis. Exp Parasitol. (1993) 77:36–42. doi: 10.1006/expr.1993.1058
33. Isseroff H, Sawma JT, Reino D. Fascioliasis: role of proline in bile duct hyperplasia. Science. (1977) 198:1157–9. doi: 10.1126/science.929191
34. Modavi S, Isseroff H. Fasciola hepatica: collagen deposition and other histopathology in the rat host's bile duct caused by the parasite and by proline infusion. Exp Parasitol. (1984) 58:239–44. doi: 10.1016/0014-4894(84)90040-7
35. Pérez J, Ortega J, Moreno T, Morrondo P, López-Sández C, Martínez-Moreno A. Pathological and immunohistochemical study of the liver and hepatic lymph nodes of sheep chronically reinfected with Fasciola hepatica, with or without triclabendazole treatment. J Comp Pathol. (2002) 127:30–6. doi: 10.1053/jcpa.2002.0561
36. Zafra R, Pérez-Écija RA, Buffoni L, Pacheco IL, Martínez-Moreno A, LaCourse EJ, et al. Early hepatic and peritoneal changes and immune response in goats vaccinated with a recombinant glutathione transferase sigma class and challenged with Fasciola hepatica. Res Vet Sci. (2013) 94:602–9. doi: 10.1016/j.rvsc.2012.10.026
37. Martínez-Moreno A, Jiménez-Luque V, Moreno T, Redondo ES, de las Mulas JM, Pérez J. Liver pathology and immune response in experimental Fasciola hepatica infections of goats. Vet Parasitol. (1999) 82:19–33. doi: 10.1016/S0304-4017(98)00262-3
38. Aldridge A, O'Neill SM. Fasciola hepatica tegumental antigens induce anergic-like T cells via dendritic cells in a mannose receptor-dependent manner. Eur J Immunol. (2016) 46:1180–92. doi: 10.1002/eji.201545905
39. Garcia-Campos A, Ravidà A, Nguyen DL, Cwiklinski K, Dalton JP, Hokke CH, et al. Tegument glycoproteins and cathepsins of newly excysted juvenile Fasciola hepatica Carry mannosidic and paucimannosidic N-glycans. PLoS Negl Trop Dis. (2016) 10:e0004688. doi: 10.1371/journal.pntd.0004688
40. Dowling DJ, Hamilton CM, Donnelly S, La Course J, Brophy PM, Dalton J, et al. Major secretory antigens of the helminth Fasciola hepatica activate a suppressive dendritic cell phenotype that attenuates Th17 cells but fails to activate Th2 immune responses. Infect Immun. (2010) 78:793–801. doi: 10.1128/IAI.00573-09
41. Flynn RJ, Mulcahy G. Possible role for Toll-like receptors in interaction of Fasciola hepatica excretory/secretory products with bovine macrophages. Infect Immun. (2008) 76:678–84. doi: 10.1128/IAI.00732-07
42. van Milligen FJ, Cornelissen JB, Gaasenbeek CP, Bokhout BA. A novel ex vivo rat infection model to study protective immunity against Fasciola hepatica at the gut level. J Immunol Methods. (1998) 213:183–90. doi: 10.1016/S0022-1759(98)00026-X
43. Prussin C, Metcalfe DD. 4. IgE, mast cells, basophils, and eosinophils. J Allergy Clin Immunol. (2003) 111:S486–94. doi: 10.1067/mai.2003.120
44. Gurish MF, Bryce PJ, Tao H, Kisselgof AB, Thornton EM, Miller HR, et al. IgE enhances parasite clearance and regulates mast cell responses in mice infected with trichinella spiralis. J Immunol. (2004) 172:1139–45. doi: 10.4049/jimmunol.172.2.1139
45. Yoshimoto T, Nakanishi K. Roles of IL-18 in basophils and mast cells. Allergol Int. (2006) 55:105–13. doi: 10.2332/allergolint.55.105
46. Doy TG, Hughes DL, Harness E. Hypersensitivity in rats infected with Fasciola hepatica: possible role in protection against a challenge infection. Res Vet Sci. (1981) 30:360–3. doi: 10.1016/S0034-5288(18)32558-X
47. Wicki P, Schwalbach B, Charbon JL, Steiner A, Lang M, Loup F, et al. Intestinal cellular reaction of cattle after infection by Fasciola hepatica. Schweiz Arch Tierheilkd. (1991) 133:429–37.
48. Bossaert K, Jacquinet E, Saunders J, Farnir F, Losson B. Cell-mediated immune response in calves to single-dose, trickle, and challenge infections with Fasciola hepatica. Vet Parasitol. (2000) 88:17–34. doi: 10.1016/S0304-4017(99)00200-9
49. McCole DF, Doherty ML, Baird AW, Davis WC, McGill K, Torgerson PR. Concanavalin a-stimulated proliferation of T cell subset-depleted lymphocyte populations isolated from Fasciola hepatica-infected cattle. Vet Immunol Immunopathol. (1998) 66:289–300. doi: 10.1016/S0165-2427(98)00207-4
50. Escamilla A, Pérez-Caballero R, Zafra R, Bautista MJ, Pacheco IL, Ruiz MT, et al. Apoptosis of peritoneal leucocytes during early stages of Fasciola hepatica infections in sheep. Vet Parasitol. (2017) 238:49–53. doi: 10.1016/j.vetpar.2017.03.015
51. Molina EC, Skerratt LF. Cellular and humoral responses in liver of cattle and buffaloes infected with a single dose of Fasciola gigantica. Vet Parasitol. (2005) 131:157–63. doi: 10.1016/j.vetpar.2005.04.028
52. Huntley JF, Newlands G, Miller HR. The isolation and characterization of globule leucocytes: their derivation from mucosal mast cells in parasitized sheep. Parasite Immunol. (1984) 6:371–90. doi: 10.1111/j.1365-3024.1984.tb00809.x
53. Stankiewicz M, Jonas WE, Douch PC, Rabel B, Bisset S, Cabaj W. Globule leukocytes in the lumen of the small intestine and the resistance status of sheep infected with parasitic nematodes. J Parasitol. (1993) 79:940–5. doi: 10.2307/3283734
54. Balic A, Bowles VM, Meeusen EN. The immunobiology of gastrointestinal nematode infections in ruminants. Adv Parasitol. (2000) 45:181–241. doi: 10.1016/S0065-308X(00)45005-0
55. Delves PJ, Martin SJ, Burton DR, Roitt IM. Roitt's Essential Immunology 13th edn. Wiley-Blackwell (2014).
56. Piedrafita D, Estuningsih E, Pleasance J, Prowse R, Raadsma HW, Meeusen EN, et al. Peritoneal lavage cells of Indonesian thin-tail sheep mediate antibody-dependent superoxide radical cytotoxicity in vitro against newly excysted juvenile Fasciola gigantica but not juvenile Fasciola hepatica. Infect Immun. (2007) 75:1954–63. doi: 10.1128/IAI.01034-06
57. Bossaert K, Farnir F, Leclipteux T, Protz M, Lonneux JF, Losson B. Humoral immune response in calves to single-dose, trickle and challenge infections with Fasciola hepatica. Vet Parasitol. (2000) 87:103–23. doi: 10.1016/S0304-4017(99)00177-6
58. Osman MM, Rashwan E, Farag HF. Phagocytic activity of neutrophils in human fasciolosis before and after treatment. J Egypt Soc Parasitol. (1995) 25:321–7.
59. Martínez-Moreno A, Jiménez-Luque V, Cámara S, Martínez-Moreno FJ, Acosta I, Hernández S. Oxidative responses during bacterial phagocytosis of polymorphonuclear leucocytes in primarily and secondarily Fasciola hepatica infected goats. Int J Parasitol. (2000) 30:1013–7. doi: 10.1016/S0020-7519(00)00082-5
60. Chauvin A, Moreau E, Boulard C. Responses of Fasciola hepatica infected sheep to various infection levels. Vet Res. (2001) 32:87–92. doi: 10.1051/vetres:2001113
61. Zhang WY, Moreau E, Hope JC, Howard CJ, Huang WY, Chauvin A. Fasciola hepatica and Fasciola gigantica: comparison of cellular response to experimental infection in sheep. Exp Parasitol. (2005) 111:154–9. doi: 10.1016/j.exppara.2005.06.005
62. Zafra R, Buffoni L, Martínez-Moreno A, Pérez-Ecija A, Martinez-Moreno FJ, Pérez J, et al. study of the liver of goats immunized with a synthetic peptide of the Sm14 antigen and challenged with Fasciola hepatica. J Comp Pathol. (2008) 139:169–76. doi: 10.1016/j.jcpa.2008.06.004
63. Wedrychowicz H, Kesik M, Kaliniak M, Kozak-Cieszczyk M, Jedlina-Panasiuk L, Jaros S, et al. Vaccine potential of inclusion bodies containing cysteine proteinase of Fasciola hepatica in calves and lambs experimentally challenged with metacercariae of the fluke. Vet Parasitol. (2007) 147:77–88. doi: 10.1016/j.vetpar.2007.03.023
64. Ruiz-Campillo MT, Molina Hernandez V, Escamilla A, Stevenson M, Perez J, Martinez-Moreno A, et al. Immune signatures of pathogenesis in the peritoneal compartment during early infection of sheep with Fasciola hepatica. Sci Rep. (2017) 7:2782. doi: 10.1038/s41598-017-03094-0
65. Escamilla A, Bautista MJ, Zafra R, Pacheco IL, Ruiz MT, Martínez-Cruz S, et al. Fasciola hepatica induces eosinophil apoptosis in the migratory and biliary stages of infection in sheep. Vet Parasitol. (2016) 216:84–8. doi: 10.1016/j.vetpar.2015.12.013
66. Meeusen E, Lee CS, Rickard MD, Brandon MR. Cellular responses during liver fluke infection in sheep and its evasion by the parasite. Parasite Immunol. (1995) 17:37–45. doi: 10.1111/j.1365-3024.1995.tb00964.x
67. Pleasance J, Raadsma HW, Estuningsih SE, Widjajanti S, Meeusen E, Piedrafita D. Innate and adaptive resistance of Indonesian thin tail sheep to liver fluke: a comparative analysis of Fasciola gigantica and Fasciola hepatica infection. Vet Parasitol. (2011) 178:264–72. doi: 10.1016/j.vetpar.2011.01.037
68. Piedrafita D, Parsons JC, Sandeman RM, Wood PR, Estuningsih SE, Partoutomo S, et al. Antibody-dependent cell-mediated cytotoxicity to newly excysted juvenile Fasciola hepatica in vitro is mediated by reactive nitrogen intermediates. Parasite Immunol. (2001) 23:473–82. doi: 10.1046/j.1365-3024.2001.00404.x
69. Golden O, Flynn RJ, Read C, Sekiya M, Donnelly SM, Stack C, et al. Protection of cattle against a natural infection of Fasciola hepatica by vaccination with recombinant cathepsin L1 (rFhCL1). Vaccine. (2010) 28:5551–7. doi: 10.1016/j.vaccine.2010.06.039
70. Sulaiman AA, Zolnierczyk K, Japa O, Owen JP, Maddison BC, Emes RD, et al. A trematode parasite derived growth factor binds and exerts influences on host immune functions via host cytokine receptor complexes. PLoS Pathog. (2016) 12:e1005991. doi: 10.1371/journal.ppat.1005991
71. Donnelly S, O'Neill SM, Sekiya M, Mulcahy G, Dalton JP. Thioredoxin peroxidase secreted by Fasciola hepatica induces the alternative activation of macrophages. Infect Immun. (2005) 73:166–73. doi: 10.1128/IAI.73.1.166-173.2005
72. Donnelly S, Stack CM, O'Neill SM, Sayed AA, Williams DL, Dalton JP. Helminth 2-Cys peroxiredoxin drives Th2 responses through a mechanism involving alternatively activated macrophages. FASEB J. (2008) 22:4022–32. doi: 10.1096/fj.08-106278
73. Ruiz-Campillo MT, Molina-Hernández V, Pérez J, Pacheco IL, Pérez R, Escamilla A, et al. Study of peritoneal macrophage immunophenotype in sheep experimentally infected with Fasciola hepatica. Vet Parasitol. (2018) 257:34–9. doi: 10.1016/j.vetpar.2018.05.019
74. Chung JY, Bae YA, Yun DH, Yang HJ, Kong Y. Experimental murine fascioliasis derives early immune suppression with increased levels of TGF-β and IL-4. Korean J Parasitol. (2012) 50:301–8. doi: 10.3347/kjp.2012.50.4.301
75. Clery D, Torgerson P, Mulcahy G. Immune responses of chronically infected adult cattle to Fasciola hepatica. Vet Parasitol. (1996) 62:71–82. doi: 10.1016/0304-4017(95)00858-6
76. Phiri IK, Phiri AM, Harrison LJ. Serum antibody isotype responses of Fasciola-infected sheep and cattle to excretory and secretory products of Fasciola species. Vet Parasitol. (2006) 141:234–42. doi: 10.1016/j.vetpar.2006.05.019
77. Mulcahy G, O'Connor F, McGonigle S, Dowd A, Clery DG, Andrews SJ, et al. Correlation of specific antibody titre and avidity with protection in cattle immunized against Fasciola hepatica. Vaccine. (1998) 16:932–9. doi: 10.1016/S0264-410X(97)00289-2
78. Mulcahy G, Dalton JP. Cathepsin L proteinases as vaccines against infection with Fasciola hepatica (liver fluke) in ruminants. Res Vet Sci. (2001) 70:83–6. doi: 10.1053/rvsc.2000.0425
79. O'Neill SM, Brady MT, Callanan JJ, Mulcahy G, Joyce P, Mills KH, et al. Fasciola hepatica infection downregulates Th1 responses in mice. Parasite Immunol. (2000) 22:147–55. doi: 10.1046/j.1365-3024.2000.00290.x
80. Pacheco IL, Abril N, Morales-Prieto N, Bautista MJ, Zafra R, Escamilla A, et al. Th1/Th2 balance in the liver and hepatic lymph nodes of vaccinated and unvaccinated sheep during acute stages of infection with Fasciola hepatica. Vet Parasitol. (2017) 238:61–5. doi: 10.1016/j.vetpar.2017.03.022
81. Ruiz-Campillo MT, Barrero-Torres DM, Abril N, Pérez J, Zafra R, Buffoni L, et al. Fasciola hepatica primoinfections and reinfections in sheep drive distinct Th1/Th2/Treg immune responses in liver and hepatic lymph node at early and late stages. Vet Res. (2023) 54:2. doi: 10.1186/s13567-022-01129-7
82. Meng Z, Zhai L, Guo Y, Zheng M, Li L, Wen C, et al. Secondary infection of Fasciola gigantica in buffaloes shows a similar pattern of serum cytokine secretion as in primary infection. Front Vet Sci. (2023) 10:1109947. doi: 10.3389/fvets.2023.1109947
83. Fu Y, Chryssafidis AL, Browne JA, O'Sullivan J, McGettigan PA, Mulcahy G. Transcriptomic study on ovine immune responses to Fasciola hepatica infection. PLoS Negl Trop Dis. (2016) 10:e0005015. doi: 10.1371/journal.pntd.0005015
84. Haçariz O, Sayers G, Baykal AT. A proteomic approach to investigate the distribution and abundance of surface and internal Fasciola hepatica proteins during the chronic stage of natural liver fluke infection in cattle. J Proteome Res. (2012) 11:3592–604. doi: 10.1021/pr300015p
85. Ravidà A, Aldridge AM, Driessen NN, Heus FA, Hokke CH, O'Neill SM. Fasciola hepatica surface coat glycoproteins contain mannosylated and phosphorylated N-glycans and exhibit immune modulatory properties independent of the mannose receptor. PLoS Negl Trop Dis. (2016) 10:e0004601. doi: 10.1371/journal.pntd.0004601
86. Sánchez-López CM, Trelis M, Jara L, Cantalapiedra F, Marcilla A, Bernal D. Diversity of extracellular vesicles from different developmental stages of Fasciola hepatica. Int J Parasitol. (2020) 50:663–9. doi: 10.1016/j.ijpara.2020.03.011
87. Murphy A, Cwiklinski K, Lalor R, O'Connell B, Robinson MW, Gerlach J, et al. Fasciola hepatica extracellular vesicles isolated from excretory-secretory products using a gravity flow method modulate dendritic cell phenotype and activity. PLoS Negl Trop Dis. (2020) 14:e0008626. doi: 10.1371/journal.pntd.0008626
88. Fontenla S, Langleib M, de la Torre-Escudero E, Domínguez MF, Robinson MW, Tort J. Role of Fasciola hepatica Small RNAs in the interaction with the mammalian host. Front Cell Infect Microbiol. (2022) 11:812141. doi: 10.3389/fcimb.2021.812141
89. Herron CM, O'Connor A, Robb E, McCammick E, Hill C, Marks NJ, et al. Developmental regulation and functional prediction of microRNAs in an expanded Fasciola hepatica miRNome. Front Cell Infect Microbiol. (2022) 12:811123. doi: 10.3389/fcimb.2022.811123
90. Ricafrente A, Cwiklinski K, Nguyen H, Dalton JP, Tran N, Donnelly S. Stage-specific miRNAs regulate gene expression associated with growth, development and parasite-host interaction during the intra-mammalian migration of the zoonotic helminth parasite Fasciola hepatica. BMC Genomics. (2022) 23:419. doi: 10.1186/s12864-022-08644-z
91. Fromm B, Ovchinnikov V, Høye E, Bernal D, Hackenberg M, Marcilla A. On the presence and immunoregulatory functions of extracellular microRNAs in the trematode Fasciola hepatica. Parasite Immunol. (2017) 39. doi: 10.1111/pim.12399
92. Cancela M, Acosta D, Rinaldi G, Silva E, Durán R, Roche L, et al. A distinctive repertoire of cathepsins is expressed by juvenile invasive Fasciola hepatica. Biochimie. (2008) 90:1461–75. doi: 10.1016/j.biochi.2008.04.020
93. Robinson MW, Dalton JP, Donnelly S. Helminth pathogen cathepsin proteases: it's a family affair. Trends Biochem Sci. (2008) 33:601–8. doi: 10.1016/j.tibs.2008.09.001
94. Xifeng W, Jiahua Z, Ningxing L, Guowu Z, Yunxia S, Xuepeng C, et al. The regulatory roles of Fasciola hepatica GSTO1 protein in inflammatory cytokine expression and apoptosis in murine macrophages. Acta Trop. (2023) 245:106977. doi: 10.1016/j.actatropica.2023.106977
95. Carmona C, Dowd AJ, Smith AM, Dalton JP. Cathepsin L proteinase secreted by Fasciola hepatica in vitro prevents antibody-mediated eosinophil attachment to newly excysted juveniles. Mol Biochem Parasitol. (1993) 62:9–17. doi: 10.1016/0166-6851(93)90172-T
96. Mebius MM, Op Heij JMJ, Tielens AGM, de Groot PG, Urbanus RT, van Hellemond JJ. Fibrinogen and fibrin are novel substrates for Fasciola hepatica cathepsin L peptidases. Mol Biochem Parasitol. (2018) 221:10–3. doi: 10.1016/j.molbiopara.2018.02.001
97. Falcón CR, Masih D, Gatti G, Sanchez MC, Motrán CC, Cervi L. Fasciola hepatica Kunitz type molecule decreases dendritic cell activation and their ability to induce inflammatory responses. PLoS ONE. (2014) 9:e114505. doi: 10.1371/journal.pone.0114505
98. Ramos-Benítez MJ, Ruiz-Jiménez C, Aguayo V, Espino AM. Recombinant Fasciola hepatica fatty acid binding protein suppresses toll-like receptor stimulation in response to multiple bacterial ligands. Sci Rep. (2017) 7:5455. doi: 10.1038/s41598-017-05735-w
99. Ruiz-Jiménez C, Celias D, Valdés B, Ramos-Pérez WD, Cervi L, Espino AM. Fasciola hepatica fatty acid binding protein (Fh12) induces apoptosis and tolerogenic properties in murine bone marrow derived dendritic cells. Exp Parasitol. (2021) 231:108174. doi: 10.1016/j.exppara.2021.108174
100. Alvarado R, O'Brien B, Tanaka A, Dalton JP, Donnelly S. A parasitic helminth-derived peptide that targets the macrophage lysosome is a novel therapeutic option for autoimmune disease. Immunobiology. (2015) 220:262–9. doi: 10.1016/j.imbio.2014.11.008
101. Cancela M, Santos GB, Carmona C, Ferreira HB, Tort JF, Zaha A. Fasciola hepatica mucin-encoding gene: expression, variability and its potential relevance in host-parasite relationship. Parasitology. (2015) 142:1673–81. doi: 10.1017/S0031182015001134
102. Noya V, Brossard N, Rodríguez E, Dergan-Dylon LS, Carmona C, Rabinovich GA, et al. mucin-like peptide from Fasciola hepatica instructs dendritic cells with parasite specific Th1-polarizing activity. Sci Rep. (2017) 7:40615. doi: 10.1038/srep40615
103. De Marco Verissimo C, Jewhurst HL, Dobó J, Gál P, Dalton JP, Cwiklinski K. Fasciola hepatica is refractory to complement killing by preventing attachment of mannose binding lectin (MBL) and inhibiting MBL-associated serine proteases (MASPs) with serpins. PLoS Pathog. (2022) 18:e1010226. doi: 10.1371/journal.ppat.1010226
104. Zhang K, Liu Y, Zhang G, Wang X, Li Z, Shang Y, et al. Molecular characteristics and potent immunomodulatory activity of Fasciola hepatica cystatin. Korean J Parasitol. (2022) 60:117–26. doi: 10.3347/kjp.2022.60.2.117
105. Zawistowska-Deniziak A, Wasyl K, Norbury LJ, Wesołowska A, Bień J, Grodzik M, et al. Characterization and differential expression of cathepsin L3 alleles from Fasciola hepatica. Mol Biochem Parasitol. (2013) 190:27–37. doi: 10.1016/j.molbiopara.2013.06.001
106. McNulty SN, Tort JF, Rinaldi G, Fischer K, Rosa BA, Smircich P, et al. Genomes of Fasciola hepatica from the Americas reveal colonization with neorickettsia endobacteria related to the agents of potomac horse and human sennetsu fevers. PLoS Genet. (2017) 13:e1006537. doi: 10.1371/journal.pgen.1006537
107. Flynn RJ, Mulcahy G, Elsheikha HM. Coordinating innate and adaptive immunity in Fasciola hepatica infection: implications for control. Vet Parasitol. (2010) 169:235–40. doi: 10.1016/j.vetpar.2010.02.015
108. Tliba O, Sibille P, Boulard C, Chauvin A. Local hepatic immune response in rats during primary infection with Fasciola hepatica. Parasite. (2000) 7:9–18. doi: 10.1051/parasite/2000071009
109. Serradell MC, Guasconi L, Cervi L, Chiapello LS, Masih DT. Excretory-secretory products from Fasciola hepatica induce eosinophil apoptosis by a caspase-dependent mechanism. Vet Immunol Immunopathol. (2007) 117:197–208. doi: 10.1016/j.vetimm.2007.03.007
110. Guasconi L, Serradell MC, Masih DT. Fasciola hepatica products induce apoptosis of peritoneal macrophages. Vet Immunol Immunopathol. (2012) 148:359–63. doi: 10.1016/j.vetimm.2012.06.022
111. Fu Y, Browne JA, Killick K, Mulcahy G. Network analysis of the systemic response to Fasciola hepatica infection in sheep reveals changes in fibrosis, apoptosis, toll-like receptors 3/4, and B cell function. Front Immunol. (2017) 8:485. doi: 10.3389/fimmu.2017.00485
112. Garcia-Campos A, Correia CN, Naranjo-Lucena A, Garza-Cuartero L, Farries G, Browne JA, et al. Fasciola hepatica infection in cattle: analyzing responses of Peripheral Blood Mononuclear Cells (PBMC) using a transcriptomics approach. Front Immunol. (2019) 10:2081. doi: 10.3389/fimmu.2019.02081
113. Flynn RJ, Mulcahy G. The roles of IL-10 and TGF-beta in controlling IL-4 and IFN-gamma production during experimental Fasciola hepatica infection. Int J Parasitol. (2008) 38:1673–80. doi: 10.1016/j.ijpara.2008.05.008
114. Guasconi L, Serradell MC, Garro AP, Iacobelli L, Masih DT. C-type lectins on macrophages participate in the immunomodulatory response to Fasciola hepatica products. Immunology. (2011) 133:386–96. doi: 10.1111/j.1365-2567.2011.03449.x
115. Flynn RJ, Irwin JA, Olivier M, Sekiya M, Dalton JP, Mulcahy G. Alternative activation of ruminant macrophages by Fasciola hepatica. Vet Immunol Immunopathol. (2007) 120:31–40. doi: 10.1016/j.vetimm.2007.07.003
116. Garza-Cuartero L, O'Sullivan J, Blanco A, McNair J, Welsh M, Flynn RJ, et al. Fasciola hepatica infection reduces mycobacterium bovis burden and mycobacterial uptake and suppresses the pro-inflammatory response. Parasite Immunol. (2016) 38:387–402. doi: 10.1111/pim.12326
117. Kreider T, Anthony RM, Urban JF Jr, Gause WC. Alternatively activated macrophages in helminth infections. Curr Opin Immunol. (2007) 19:448–53. doi: 10.1016/j.coi.2007.07.002
118. Hamilton CM, Dowling DJ, Loscher CE, Morphew RM, Brophy PM, O'Neill SM. The Fasciola hepatica tegumental antigen suppresses dendritic cell maturation and function. Infect Immun. (2009) 77:2488–98. doi: 10.1128/IAI.00919-08
119. Falcón C, Carranza F, Martínez FF, Knubel CP, Masih DT, Motrán CC, et al. Excretory-secretory products (ESP) from Fasciola hepatica induce tolerogenic properties in myeloid dendritic cells. Vet Immunol Immunopathol. (2010) 137:36–46. doi: 10.1016/j.vetimm.2010.04.007
120. Falcón CR, Carranza FA, Aoki P, Motrán CC, Cervi L. Adoptive transfer of dendritic cells pulsed with Fasciola hepatica antigens and lipopolysaccharides confers protection against fasciolosis in mice. J Infect Dis. (2012) 205:506–14. doi: 10.1093/infdis/jir606
121. Vukman KV, Adams PN, O'Neill SM. Fasciola hepatica tegumental coat antigen suppresses MAPK signalling in dendritic cells and up-regulates the expression of SOCS3. Parasite Immunol. (2013) 35:234–8. doi: 10.1111/pim.12033
122. Mei XF, Shi W, Zhang YY, Zhu B, Wang YR, Hou LJ, et al. DNA methylation and hydroxymethylation profiles reveal possible role of highly methylated TLR signaling on Fasciola gigantica excretory/secretory products (FgESPs) modulation of buffalo dendritic cells. Parasit Vectors. (2019) 12:358. doi: 10.1186/s13071-019-3615-4
123. Walsh KP, Brady MT, Finlay CM, Boon L, Mills KH. Infection with a helminth parasite attenuates autoimmunity through TGF-beta-mediated suppression of Th17 and Th1 responses. J Immunol. (2009) 183:1577–86. doi: 10.4049/jimmunol.0803803
124. Rodríguez E, Kalay H, Noya V, Brossard N, Giacomini C, van Kooyk Y, et al. Fasciola hepatica glycoconjugates immuneregulate dendritic cells through the dendritic cell-specific intercellular adhesion molecule-3-grabbing non-integrin inducing T cell anergy. Sci Rep. (2017) 7:46748. doi: 10.1038/srep46748
125. Ruiz-Campillo MT, Molina-Hernández V, Bautista MJ, Pacheco IL, Zafra R, Buffoni L, et al. Characterization of dendritic cells and follicular dendritic cells in the hepatic lymph nodes and liver of sheep experimentally infected with Fasciola hepatica. Vet Res. (2020) 51:33. doi: 10.1186/s13567-020-00757-1
126. Pacheco IL, Abril N, Zafra R, Molina-Hernández V, Morales-Prieto N, Bautista MJ, et al. Fasciola hepatica induces Foxp3 T cell, proinflammatory and regulatory cytokine overexpression in liver from infected sheep during early stages of infection. Vet Res. (2018) 49:56. doi: 10.1186/s13567-018-0550-x
127. Taylor MD, Van Der Werf N, Maizels RM. T cells in helminth infection: the regulators and the regulated. Trends Immunol. (2012) 33:181–9. doi: 10.1016/j.it.2012.01.001
128. McNeilly TN, Rocchi M, Bartley Y, Brown JK, Frew D, Longhi C, et al. Suppression of ovine lymphocyte activation by teladorsagia circumcincta larval excretory–secretory products. Vet Res. (2013) 44:70. doi: 10.1186/1297-9716-44-70
129. Dalton JP, Robinson MW, Mulcahy G, O'Neill SM, Donnelly S. Immunomodulatory molecules of Fasciola hepatica: candidates for both vaccine and immunotherapeutic development. Vet Parasitol. (2013) 195:272–85. doi: 10.1016/j.vetpar.2013.04.008
130. Doy TG, Hughes DL. Fasciola hepatica: site of resistance to reinfection in cattle. Exp Parasitol. (1984) 57:274–8. doi: 10.1016/0014-4894(84)90101-2
131. Piedrafita D, Raadsma HW, Prowse R, Spithill TW. Immunology of the host-parasite relationship in fasciolosis (Fasciola hepatica and Fasciola gigantica). Can J Zool. (2004) 82:233–50. doi: 10.1139/z03-216
132. Chauvin A, Bouvet G, Boulard C. Humoral and cellular immune responses to Fasciola hepatica experimental primary and secondary infection in sheep. Int J Parasitol. (1995) 10:1227–41. doi: 10.1016/0020-7519(95)00039-5
133. Villa-Mancera A, Olivares-Pérez J, Olmedo-Juárez A, Reynoso-Palomar A. Phage display-based vaccine with cathepsin L and excretory-secretory products mimotopes of Fasciola hepatica induces protective cellular and humoral immune responses in sheep. Vet Parasitol. (2021) 289:109340. doi: 10.1016/j.vetpar.2020.109340
134. Villa-Mancera A, Reynoso-Palomar A, Utrera-Quintana F, Carreón-Luna L. Cathepsin L1 mimotopes with adjuvant Quil A induces a Th1/Th2 immune response and confers significant protection against Fasciola hepatica infection in goats. Parasitol Res. (2014) 113:243–50. doi: 10.1007/s00436-013-3650-6
135. Toet H, Piedrafita DM, Spithill TW. Liver fluke vaccines in ruminants: strategies, progress and future opportunities. Int J Parasitol. (2014) 44:915–27. doi: 10.1016/j.ijpara.2014.07.011
136. Dalton JP, McGonigle S, Rolph TP, Andrews SJ. Induction of protective immunity in cattle against infection with Fasciola hepatica by vaccination with cathepsin L proteinases and with hemoglobin. Infect Immun. (1996) 64:5066–74. doi: 10.1128/iai.64.12.5066-5074.1996
137. Morrison CA, Colin T, Sexton JL, Bowen F, Wicker J, Friedel T, et al. Protection of cattle against Fasciola hepatica infection by vaccination with glutathione S-transferase. Vaccine. (1996) 14:1603–12. doi: 10.1016/S0264-410X(96)00147-8
138. Orbegozo-Medina RA, Martínez-Sernández V, González-Warleta M, Castro-Hermida JA, Mezo M, Ubeira FM. Vaccination of sheep with Quil-A® adjuvant expands the antibody repertoire to the Fasciola MF6p/FhHDM-1 antigen and administered together impair the growth and antigen release of flukes. Vaccine. (2018) 36:1949–57. doi: 10.1016/j.vaccine.2018.02.115
139. Villa-Mancera A, Méndez-Mendoza M. Protection and antibody isotype responses against Fasciola hepatica with specific antibody to pIII-displayed peptide mimotopes of cathepsin L1 in sheep. Vet J. (2012) 194:108–12. doi: 10.1016/j.tvjl.2012.03.003
140. Villa-Mancera A, Alcalá-Canto Y, Reynoso-Palomar A, Olmedo-Juárez A, Olivares-Pérez J. Vaccination with cathepsin L phage-exposed mimotopes, single or in combination, reduce size, fluke burden, egg production and viability in sheep experimentally infected with Fasciola hepatica. Parasitol Int. (2021) 83:102355. doi: 10.1016/j.parint.2021.102355
141. Villa-Mancera A, Alcalá-Canto Y, Olivares-Pérez J, Molina-Mendoza P, Hernández-Guzmán K, Utrera-Quintana F, et al. Vaccination with cathepsin L mimotopes of Fasciola hepatica in goats reduces worm burden, morphometric measurements, and reproductive structures. Microb Pathog. (2021) 155:104859. doi: 10.1016/j.micpath.2021.104859
142. Wesołowska A, Kozak Ljunggren M, Jedlina L, Basałaj K, Legocki A, Wedrychowicz H, et al. A preliminary study of a lettuce-based edible vaccine expressing the cysteine proteinase of Fasciola hepatica for fasciolosis control in livestock. Front Immunol. (2018) 9:2592. doi: 10.3389/fimmu.2018.02592
143. Maggioli G, Acosta D, Silveira F, Rossi S, Giacaman S, Basika T, et al. The recombinant gut-associated M17 leucine aminopeptidase in combination with different adjuvants confers a high level of protection against Fasciola hepatica infection in sheep. Vaccine. (2011) 29:9057–63. doi: 10.1016/j.vaccine.2011.09.020
144. Raina OK, Nagar G, Varghese A, Prajitha G, Alex A, Maharana BR, et al. Lack of protective efficacy in buffaloes vaccinated with Fasciola gigantica leucine aminopeptidase and peroxiredoxin recombinant proteins. Acta Trop. (2011) 118:217–22. doi: 10.1016/j.actatropica.2011.02.008
145. López-Abán J, Casanueva P, Nogal J, Arias M, Morrondo P, Diez-Baños P, et al. Progress in the development of Fasciola hepatica vaccine using recombinant fatty acid binding protein with the adjuvant adaptation system ADAD. Vet Parasitol. (2007) 145:287–96. doi: 10.1016/j.vetpar.2006.12.017
146. Nambi PA, Yadav SC, Raina OK, Sriveny D, Saini M. Vaccination of buffaloes with Fasciola gigantica recombinant fatty acid binding protein. Parasitol Res. (2005) 97:129–35. doi: 10.1007/s00436-005-1397-4
147. Kumar N, Anju V, Gaurav N, Chandra D, Samanta S, Gupta SC, et al. Vaccination of buffaloes with Fasciola gigantica recombinant glutathione S-transferase and fatty acid binding protein. Parasitol Res. (2012) 110:419–26. doi: 10.1007/s00436-011-2507-0
148. Buffoni L, Martínez-Moreno FJ, Zafra R, Mendes RE, Pérez-Écija A, Sekiya M, et al. Humoral immune response in goats immunised with cathepsin L1, peroxiredoxin and Sm14 antigen and experimentally challenged with Fasciola hepatica. Vet Parasitol. (2012) 185:315–21. doi: 10.1016/j.vetpar.2011.09.027
149. Maggioli G, Bottini G, Basika T, Alonzo P, Salinas G, Carmona C. Immunization with Fasciola hepatica thioredoxin glutathione reductase failed to confer protection against fasciolosis in cattle. Vet Parasitol. (2016) 224:13–9. doi: 10.1016/j.vetpar.2016.05.007
150. Wesołowska A, Basałaj K, Zawistowska-Deniziak A, Januszkiewicz K, Kozak Ljunggren M, Jedlina L, et al. The failure of a DNA prime/protein boost regime and CTLA-4 mediated targeting to improve the potency of a DNA vaccine encoding Fasciola hepatica phosphoglycerate kinase in sheep. Vet Immunol Immunopathol. (2019) 217:109941. doi: 10.1016/j.vetimm.2019.109941
151. Pérez-Caballero R, Siles-Lucas M, González-Miguel J, Martínez-Moreno FJ, Escamilla A, Pérez J, et al. Pathological, immunological and parasitological study of sheep vaccinated with the recombinant protein 14-3-3z and experimentally infected with Fasciola hepatica. Vet Immunol Immunopathol. (2018) 202:115–21. doi: 10.1016/j.vetimm.2018.07.006
152. Zerna G, Rathinasamy VA, Toet H, Anderson G, Dempster R, Spithill TW, et al. Evaluation of immunogenicity and efficacy of Fasciola hepatica tetraspanin 2 (TSP2) Fused to E. coli heat-labile enterotoxin B subunit LTB adjuvant following intranasal vaccination of cattle. Vaccines. (2021) 9:1213. doi: 10.3390/vaccines9111213
153. Garza-Cuartero L, Geurden T, Mahan SM, Hardham JM, Dalton JP, Mulcahy G. Antibody recognition of cathepsin L1-derived peptides in Fasciola hepatica-infected and/or vaccinated cattle and identification of protective linear B-cell epitopes. Vaccine. (2018) 36:958–68. doi: 10.1016/j.vaccine.2018.01.020
154. Norbury LJ, Basałaj K, Zawistowska-Deniziak A, Sielicka A, Wilkowski P, Wesołowska A, et al. Intranasal delivery of a formulation containing stage-specific recombinant proteins of Fasciola hepatica cathepsin L5 and cathepsin B2 triggers an anti-fecundity effect and an adjuvant-mediated reduction in fluke burden in sheep. Vet Parasitol. (2018) 258:14–23. doi: 10.1016/j.vetpar.2018.05.008
155. Ortega-Vargas S, Espitia C, Sahagún-Ruiz A, Parada C, Balderas-Loaeza A, Villa-Mancera A, et al. Moderate protection is induced by a chimeric protein composed of leucine aminopeptidase and cathepsin L1 against Fasciola hepatica challenge in sheep. Vaccine. (2019) 37:3234–40. doi: 10.1016/j.vaccine.2019.04.067
156. McCusker P, Toet H, Rathinasamy V, Young N, Beddoe T, Anderson G, et al. Molecular characterisation and vaccine efficacy of two novel developmentally regulated surface tegument proteins of Fasciola hepatica. Vet Parasitol. (2020) 286:109244. doi: 10.1016/j.vetpar.2020.109244
157. Zafra R, Buffoni L, Pérez-Caballero R, Molina-Hernández V, Ruiz-Campillo MT, Pérez J, et al. Efficacy of a multivalent vaccine against Fasciola hepatica infection in sheep. Vet Res. (2021) 52:13. doi: 10.1186/s13567-021-00895-0
158. Becerro-Recio D, Serrat J, López-García M, Molina-Hernández V, Pérez-Arévalo J, Martínez-Moreno Á, et al. Study of the migration of Fasciola hepatica juveniles across the intestinal barrier of the host by quantitative proteomics in an ex vivo model. PLoS Negl Trop Dis. (2022) 16:e0010766. doi: 10.1371/journal.pntd.0010766
Keywords: Fasciola hepatica, pathogenesis, host-pathogen interaction, immunomodulation, vaccine, livestock, onehealth, zoonosis
Citation: Flores-Velázquez LM, Ruiz-Campillo MT, Herrera-Torres G, Martínez-Moreno Á, Martínez-Moreno FJ, Zafra R, Buffoni L, Rufino-Moya PJ, Molina-Hernández V and Pérez J (2023) Fasciolosis: pathogenesis, host-parasite interactions, and implication in vaccine development. Front. Vet. Sci. 10:1270064. doi: 10.3389/fvets.2023.1270064
Received: 31 July 2023; Accepted: 30 October 2023;
Published: 11 December 2023.
Edited by:
Francisco Javier Salguero, UK Health Security Agency (UKHSA), United KingdomReviewed by:
Mihaela Niculae, University of Agricultural Sciences and Veterinary Medicine of Cluj-Napoca, RomaniaPeter Geldhof, Ghent University, Belgium
Copyright © 2023 Flores-Velázquez, Ruiz-Campillo, Herrera-Torres, Martínez-Moreno, Martínez-Moreno, Zafra, Buffoni, Rufino-Moya, Molina-Hernández and Pérez. This is an open-access article distributed under the terms of the Creative Commons Attribution License (CC BY). The use, distribution or reproduction in other forums is permitted, provided the original author(s) and the copyright owner(s) are credited and that the original publication in this journal is cited, in accordance with accepted academic practice. No use, distribution or reproduction is permitted which does not comply with these terms.
*Correspondence: Verónica Molina-Hernández, dm1vbGluYSYjeDAwMDQwO3Vjby5lcw==
†These authors have contributed equally to this work