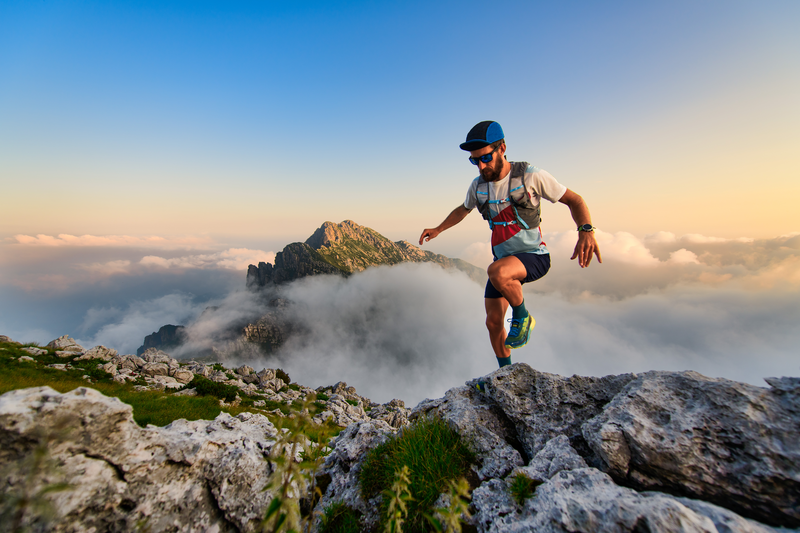
94% of researchers rate our articles as excellent or good
Learn more about the work of our research integrity team to safeguard the quality of each article we publish.
Find out more
SYSTEMATIC REVIEW article
Front. Vet. Sci. , 18 December 2023
Sec. Comparative and Clinical Medicine
Volume 10 - 2023 | https://doi.org/10.3389/fvets.2023.1264668
Introduction: Chemotherapy-induced peripheral neuropathy (CIPN) is a common consequence of cancer treatment and pain is a frequent complaint of the patients. Paclitaxel, a cytostatic drug, generates a well-described peripheral nerve injury and neuroinflammation, which may be experimentally mimicked in animal models. We conducted a systematic review analyzing the experimental design, reporting and mechanisms underlying paclitaxel-induced neuropathy in the included studies to establish the perspectives of translation of the current literature in models of CIPN.
Methods: We elected studies published in Pubmed and Scopus between 1 January 2018 and 3 December 2022.
Results: According to a defined mesh of keywords searched, and after applying exclusion and inclusion criteria, 70 original studies were included and analyzed in detail. Most studies used male Sprague-Dawley rats to induce paclitaxel-induced neuropathy, used low doses of paclitaxel, and the analyzed studies mainly focused at 14-28 days of CIPN. Mechanical nociceptive tests were preferred in the behavioral evaluation. The mechanisms under study were mainly neuroinflammation of peripheral nerves. The overall methodological quality was considered moderate, and the risk of bias was unclear.
Discussion: Despite the ample preclinical research in paclitaxel-induced neuropathy, this systematic review alerts to some flaws in the experimental design along with limitations in reporting, e.g., lack of representation of both sexes in experimental work and the lack of reporting of the ARRIVE guidelines. This may limit the reproducibility of preclinical studies in CIPN. In addition, the clinical features of CIPN should be considered when designing animal experiments, such as sex and age of the CIPN patients. In this way the experimental studies aiming to establish the mechanisms of CIPN may allow the development of new drugs to treat CIPN and translation in the research of CIPN could be improved.
Cancer treatment has a huge impact on patients’ lives. The most common cancer treatment, chemotherapy, typically results in side effects including neuropathy (1). Chemotherapy-induced peripheral neuropathy (CIPN) develops throughout various stages of cancer treatment and may determine a decrease of chemotherapy doses or the interruption of the chemotherapy treatments (2). CIPN may continue even after cancer remission, with a huge impact on the quality of life of cancer survivors (3). The incidence of CIPN may be affected by the chemotherapy protocol, namely cytostatic agent (type and dose) and method of CIPN assessing after cessation of chemotherapy (4). Patients with CIPN commonly express a variety of sensory dysfunctions, including spontaneous pain, hyperalgesia (increased sensitivity to noxious stimuli), and painful reactions to non-noxious stimuli (allodynia). Hypersensitivity to cold and mechanical stimuli are the main symptoms of CIPN (1, 5, 6). Moreover, negative sensory signs along with non-noxious alterations, such as itch, may also occur (7). One of the most used chemotherapy agents, paclitaxel, frequently used to treat breast, ovarian, and lung cancer, was shown to be one of the cytostatic drugs that more commonly induces CIPN (1, 8). Using validated animal models that mimic chemotherapy cycles, paclitaxel-induced neuropathy has been extensively studied in experimental settings (9–11). These rats exhibit several pain-like behaviors namely mechanical and cold hypersensitivity and spontaneous pain (11, 12). Moreover, rodents treated with paclitaxel exhibited anxiety-like and depression-like behaviors (13), as observed in clinical setting (14).
The mechanisms involved in the pathophysiology of paclitaxel-induced neuropathy have been recently studied and reviewed (8). The effects of paclitaxel on neurons at the periphery and spinal cord levels were described however its supraspinal effects remain to be studied (15–20). Our research group developed pioneer studies regarding the neuroplastic changes that occur in pain modulatory brain centers during paclitaxel-induced neuropathy (21, 22), such as the periaqueductal gray and the hypothalamus (23).
The existing preclinical studies of paclitaxel-induced neuropathy mechanisms are yet to produce clinical translation data. This is not exclusive of this neuropathy type since a “crisis of translation” has been discussed in animal pain studies, which was proposed to account for the lack of new analgesic drugs to manage chronic pain (24, 25). The problems derived from the poor degree to which pain neurobiology in rodents may predict pain neurobiology in humans, along with inaccurate experimental design in animal pain studies, should be openly discussed.
In order to assess the quality of preclinical studies using paclitaxel-induced neuropathy in terms of what they can mimic the clinical problems, along with questions of reproducibility and translatability and to identify the mechanisms of paclitaxel-induced neuropathy that are currently under study, we performed a systematic review of the literature that has been published in the last 5 years (2018–2022). The analysis was performed to evaluate the robustness of animal research in paclitaxel-induced neuropathy which may be useful in the identification of mechanisms involved in CIPN seeking be putative translation perspectives.
The literature search was performed on 03 December 2022 using two electronic databases: PubMed Central (via PubMed) and Scopus to identify preclinical studies of paclitaxel-induced neuropathic pain in last 5 years. For each database, the following syntax was used: (((paclitaxel) OR (taxol)) AND ((pain) OR (nociception)) AND ((rat) OR (mice))) from 1 January 2018 to 03 December 2022. The protocol of this review was not registered prior submission.
Relevant peer-reviewed articles, published in English language, were included based on the following criteria: (1) original articles; (2) studies with rats and/or mice; (3) rodent model of paclitaxel- or taxol-induced neuropathy; (4) only one therapeutic approach (pharmacological or non-pharmacological) with the respective mechanisms of action, signaling pathways and/or target receptors discussed. The exclusion criteria included (1) non-original articles (reviews, clinical trials, case reports or conference abstracts); (2) publications written in languages other than English; (3) studies with other CIPN animal models; (4) combination of pharmacological and/or non-pharmacological therapies.
After removing duplicate publications using EndNote, two researchers (CB and JTCP) assessed the eligibility of the articles based on the title and abstract. The second phase of this process, full-text articles were also selected against the following inclusion criteria: (1) previous criteria; (2) full-text access; (3) rodents having the minimum time of experimental condition (paclitaxel- or taxol-induced neuropathy) ≥ 11 days; (4) perform at least 1 nociceptive test (e.g., von Frey, cold and hot plate, Hargreaves or acetone test) to confirm the painful condition underlying the CIPN. A third investigator (IT) acted as mediator of different opinions between the 2 investigators.
The data extraction was carried out by three investigators (CB, JTCP, and IT). The following data were collected from the included articles and compiled, including the following information: reference of the study; characterization of the animal population (species, strain, sex, age, weight, animal supplier, frequency of paclitaxel treatment, dose, route, control group, injection volume, and time of CIPN). For dose of paclitaxel, three categories were created to present the results, studies that used doses of paclitaxel lower than 2 mg/kg, doses greater than or equal to 2 and less than or equal to 8 mg/kg, and studies that used doses greater than 8 mg/kg. Likewise, three categories were created to present the results of duration of CIPN, studies that used animals with a CIPN duration of less than 14 days, greater than or equal to 14 days and less than or equal to 28 days, and a CIPN duration of greater than 28 days.
In addition to these data, it was also extracted information about the main methods (pharmacology, non-pharmacological approaches, cell culture, biochemistry, histopathology, electrophysiology, biochemistry) and, if applicable, the tissues under analysis.
Regarding the behavioral tests, we extracted information about the type of tests and the sensorial modality evaluated, as well as spontaneous pain. Moreover, we also extracted information about types of tests which assess anxiety and depression, and locomotor activity. We grouped the timepoints in weeks (week 1 to week 8). A heatmap were generated where in white are the timepoints not evaluated, in green the timepoints with a positive response and in red the timepoints where a negative response. Furthermore, in yellow are the timepoints where we found more than one different response to the tests in the same week, and in gray are the timepoints where they evaluated the animals but did not report descriptive statistics.
In addition to these data, we also extracted the information to understand which mechanisms underlying paclitaxel-induced neuropathy are being studied as well as the nervous tissues under analysis (peripheral nerves, spinal cord and brain). A summary of the results was reported. The main mechanisms were grouped in classes involving: (1) neuroinflammation, a complex phenomenon involving the activation of the glial cells and release of inflammatory mediators, such as cytokines and chemokines in the nervous system (26); (2) oxidative stress, the imbalance between the production of reactive oxygen species and the ability to remove them, in this specific case, from the neurons (27); (3) cannabinoids and its receptors; (4) opioids and its receptors; (5) monoamines, such as noradrenaline and serotonin, and its receptors; (6) amino acids, which include glutamate and GABA (Gamma-aminobutyric acid), which are, respectively, excitatory and inhibitory neurotransmitters (GABA); and (7) transient receptor potential vanilloid 1 (TRPV1).
After extracting the data as described above, the studies were analyzed regarding their quality, namely the implementation of the ARRIVE (Animal Research: Reporting of In Vivo Experiments) guidelines and the risk of bias, as described below.
The ARRIVE guidelines are a validated checklist used to improve the quality of reporting of animal research (28), which comprise 10 essential (study design, sample size, inclusion and exclusion criteria, randomization, blinding, outcome measures, statistical methods, experimental animals and procedures, and results) and 11 recommended items (abstract, background, objectives, ethical statement, housing and husbandry, animal care and monitoring, interpretation of results, generalization and translation, protocol registration, data access and declaration of interest). Two investigators (JTCP and IT) evaluated the quality of the included studies using the ARRIVE guidelines where each component was classified at three levels of color encoding: red (incomplete) if no sub-item was described, yellow (partly complete) if one or more of the sub-items were described and green (complete) when all sub-items were fulfilled. Adapted from Fonseca-Rodrigues et al. (29), in which each sub-item/color is classified with different scores, each sub-item of the ARRIVE Essential 10 were scored as 1.1, 0.5, or 0 to green, yellow, or red, respectively, and the total score were calculated for each study. If the total score of each study is more than 15, the global rating is considered high, if the score is between 10 and 15, the global rating is considered moderate, and if the score is less than 10, it is considered low. The same scoring method was used for ARRIVE Recommend List, where green was scored 1.25, yellow 0.625 and red zero.
The SYRCLE’s Risk of Bias Tool is an instrument to assess methodological quality of the animal studies (30). This tool covers ten questions which assess the selection bias, performance bias, detection bias, attrition bias, reporting bias and other biases. Each question was scored by color coding, where high risk, low risk and unclear risk of bias were represented by red, green, and yellow, respectively (31). The score was calculated by two investigators (JTCP and IT).
The PRISMA flowchart of the current study is shown in Figure 1. The electronic search identified 506 studies from PubMed (215) and Scopus (291) as eligible for this systematic review using the search strategy detailed in Section 2. After identifying duplicates, 175 studies were removed, and 331 publications were screened based on the title and abstract. As a result, 210 publications were excluded due to non-compliance with the inclusion criteria. In the second phase, after inspection of the full text of 119 publications, 70 studies were included in this review.
Figure 1. PRISMA flowchart of the different stages of the selection process in the current systematic review.
We analyzed the studies to extract data about animal features, namely species, strain, sex, age, weight, animals’ supplier, total number of animals, number of animals per group and the location of studies.
Rats were the dominant specie studied (41 articles; 58.6%) followed by mice (26 articles; 37.1%) and 3 articles used both rats and mice (4.3%). Regarding the predominant strain, in rats it was Sprague–Dawley (81.8%) and in mice C57BL/6 (55.2%). In some articles using mice the authors used more than one strain (5 articles; 17.2%). One article using rats and one using mice, 2.3% and 3.4% respectively, did not mention any strain in their methods sections (Table 1). In rats, all articles reported the sex of animals. Most articles (88.6%) used male animals, 4.5% and 6.8% used female and both sexes, respectively. In studies using mice, 3 articles (10.3%) failed to mention the animals’ sex. Of the studies that reported, 61.5% used male mice, 34.6% used both sexes and only 3.8% used female mice (Table 1).
Table 1. Characterization of the population (species, strain, sex, age, weight, animal supplier, total number of animals, number of animals per group and where were studies performed) in the analyzed studies.
In several studies we noticed the expression “adult” to define the age of the animals and, most important, in 70.5% of the studies using rats, and 37.9% of the studies using mice, did not even report the age. Most articles reported the weight of the animals, 93.2 %and 55.2% for rats and mice, respectively. However, only 22.7% of the publications using rats and 24.1% of the publications using mice reported the weight and age of the animals (Table 1).
Regarding the number of animals used in each study, 34.1% of the publications reported the total number of rats and 24.1% reported the total number of mice. Almost all publications (100% for rats and 96.6% for mice) reported the number of animals in each experimental group (Table 1).
Regarding to the continent where the studies were performed, the majority of studies were carried out in Asia (61.4% for rats and 51.7% for mice), followed by America (29.5% for rats and 51.7% for mice). A reduced number of articles performed the studies in more than one continent (6.8% for rats and 10.3% for mice; Table 1).
In terms of the supplier of rats, in 50% of the articles the animals were bought from commercial suppliers and 50% were bred at the local facilities. Regarding to the supplier of mice, 68% of articles used animals from commercial supplier and 32% of articles the animals were bred at the local facility. However, 14.3% of the publications did not specify the provider that was used to acquire the animals (Table 1).
Nearly all studies (69 out of 70) selected the intraperitoneal (i.p.) route of paclitaxel administration over the intravenous (i.v.) route for the induction of the CIPN model. In rat studies, 36 out of 44 papers (81.8%), the most often employed paclitaxel doses were between 2 and 8 mg/kg. Only 8 publications (18.2%) used a dose lower than 2 mg/kg, and 1 article (2.3%) used a dosage more than 8 mg/kg. Noteworthy, 2 publications (4.5%) used more than one dose. Regarding to the mouse studies, mostly publications (96.6%) employed paclitaxel doses were between 2 and 8 mg/kg. Only 1 publication used a dose higher than 8 mg/kg (Table 2).
Table 2. Summary of the methods used for the induction of CIPN (paclitaxel dose, frequency of administration, vehicle, injection volume and duration of CIPN).
Regarding to the frequency of the administration of paclitaxel, in studies using rats, 84.1% of the papers indicated 4 alternate days. Likewise, in studies using mice, the majority of publications (68.9%) indicated the same induction scheme (Table 2).
Regarding the composition of the vehicle in rat studies, 36.4% used cremophor or its derivates in conjunction with other components, e.g., ethanol and tween 80, 22.7% used saline, 27.3% reported dimethyl sulfoxide (DMSO), and 9.1% stated that other vehicles were used. Two publications (4.5%) did not indicate the vehicle used to dissolve paclitaxel (Table 2). As to the composition of the vehicle in mouse studies, 58.6% used cremophor or its derivates, 17.2% used saline, 6.9% used DMSO and 3.4% used other vehicles. Four publications (13.8%) did not indicate the vehicle (Table 2) herein there was no control group for the vehicle used to prepare the paclitaxel solution.
Most of the articles using rats (86.4%) and mice (86.2%) did not report the injection volume. In rat studies, of those that reported (13.6%), 4 publications indicated a volume that varies according to weight and 1 publication indicated a fixed injection volume per rat. In mouse studies, of those that reported (13.8%), 3 articles indicated a volume that varies according to weight and 1 publication indicated a fixed injection volume per mouse (Table 2).
Regarding the time of CIPN, we categorized the articles into three groups: less than 14 days; between 14 and 28 days; more or equal to 28 days. In publications using rats, 23 articles (52.3%) reported a time of CIPN between 14 and 28 days, whereas 21 articles (47.7%) showed equal or more than 28 days of CIPN. In publications using mice, 16 articles (55.2%) reported a duration of CIN between 14 and 28 days, and 13 publications (44.8%) showed equal or more than 28 days of CIPN. Although we did not include studies with less than 11 days, in our analysis we did not find studies with less than 14 days of CIPN (Table 2).
We analyzed the studies to extract the data regarding the methods of study and the tissues under analysis. The results are shown in Table 3. The techniques most commonly used (90%; 63 studies) were behavioral evaluations both for validation of the induction of CIPN and for testing of various substances (referred as “Pharmacology” in Table 3) or interventions (referred as “Non-pharmacological approaches”). All the behavioral analysis included nociception tests (described in detail Table 3). Immunohistochemistry was a technique frequently used (44.3%; 31 studies), in conjunction with Western-blotting. Multiple biochemical methods were used, such as ELISA and HPLC. Cell cultures and histopathology analysis were also performed (about 17% of the studies). Regarding the tissues collected the peripheral nerves/dorsal root ganglion were frequently collected (64.3%; 45 studies), along with the spinal cord (61.5%; 43 studies), although 6 of those studies analyzed simultaneously the DRG and spinal cord. Only a few studies evaluated supraspinal structures (22.9%; 16 studies). Due to the large preponderance of behavioral tests, we extracted the data regarding the types of tests and, in the case of nociception tests, we analyzed the sensory modality evaluated. The most used behavioral tests (97.1%) were mechanical stimuli with the von Frey test being the most common (84.3%; Supplementary Table 1). Forty-six publications (65.7%) reported thermal nociception tests, 28.5% only used hot stimuli, 17.1% used just cold stimuli and 15.7% employed both thermal modalities. Only 1 article (1.4%) used a spontaneous pain test (Supplementary Table 2). Eleven studies (15.7%) reported other behavioral tests which assess depressive- and anxiety-like behaviors, and locomotor activity (Supplementary Table 3). Fifty-nine publications (84.3%) that were analyzed conducted behavior tests repeatedly during several timepoints. Only 15.7% of the publications tested behavior in a single day. Noteworthy, 2 publications showed behavioral tests at several timepoints, but only reported the statistical analysis of a specific timepoint.
We also analyzed the main mechanisms addressed in the retrieved publications, as well as the tissues and/or areas under study. The summary of the main mechanisms was shown in Table 4. Thirty of the analyzed studies (42.9%) focused on neuroinflammation (32–36, 39, 42–44, 46, 47, 51, 57, 60, 62, 64, 65, 70, 74, 76–78, 81–83, 85, 91, 93, 95, 98). The molecules under study were TNF-α, IL-1β, IL-6. Several of these studies analyzed the role of glial cells in driving neuroinflammation and the role of microglia (36, 39, 60, 62, 65, 91) and astrocytes (32, 36, 44, 57, 60, 65, 74, 81) was frequently evaluated. Oxidative stress (about 15.7%) was also analyzed (34, 41, 44, 58–59, 69, 71, 83, 89, 93). Several oxidative mediators, such as GSH, GSSG, SOD, CAT and MnSOD, were evaluated. Another set of studies focused on neurotransmitters and receptor systems with cannabinoids (8.6%) (37, 62, 70, 73, 79, 94), opioids (2.9%) (51, 75), monoamines (11.4%) (21, 22, 36, 38, 49, 54, 68, 87) and amino acids (5.7%) (46, 55, 84, 97) frequently studied. As to receptors, TRPV-1 studies (10%) stood out (36, 39, 52, 60, 63, 65, 80). It should be noted that several studies under analysis settled to analyze the mechanism underlying the effects of approaches such as “natural extracts” (72, 80, 96). Some studies were not aiming to establish the mechanisms of a drug but rather to apply in CIPN substances or approaches that are already been used in other pathologies, such as metformin (50), hyperbaric oxygen therapy (48), spinal cord stimulation (61), exercise (92), and intracranial self-stimulation (66). There were, however, studies which were not driven by a clear research hypothesis (67). Emerging mechanism in neuroscience research were evaluated namely in what concerns the gut-brain axis (78, 91) or circadian regulation (53).
Table 4. Mechanisms evaluated in each of the analyzed studies, summarizing the results (increases—↑; decreases—↓) in levels or responses in the referred tissues/areas.
As to the tissue sites under study, the peripheral targets stood out, both in what concerns peripheral fibers in the sciatic nerve (34, 44, 69, 83, 89) and neurons at the dorsal root ganglia (33, 42, 46, 47, 51, 60, 63, 65, 71, 77, 82, 87, 93, 95, 98). At the central nervous system the focus was the spinal cord (21, 22, 32, 35, 36, 38, 39, 41, 52, 54, 55, 57–60, 62, 65, 70, 74, 76, 78, 91) and only a few studies approached areas of the descending pain control system, such as the rostroventromedial medulla (22, 37), the somatosensory cortex (81) and the prefrontal cortex (86) along with areas whose main function is not pain control such as the hippocampus (92).
A global rating of strong was attributed to studies with a score higher than 15 (12.9%), moderate for a score between 10 and 15 (78.6%) and weak for those that scored under 10 (8.6%). Generally, the quality of the studies was moderate (Supplementary Table 4).
Five (7.1%) (32, 76, 78, 79, 97) of the 70 analyzed studies referred using the ARRIVE guidelines. The sub-items that the studies score high were the sub-item 1a (description of the experimental groups to be compared; 95.7%), 1b (definition of the experimental unit; 100%) and 7a (description of the statistical methods used; 95.7%). The most incomplete or missing sub-items were 6b (outcome measure that was used to determine the sample size) and item 10b (presentation of the effect size with confidence of interval), which failed 98.6% and 100%, respectively.
Regarding the ARRIVE’s recommended items, the quality of studies was also generally moderate, since 80% of the studies were scored as moderate and 20% was scored as weak (Supplementary Table 5).
The risk of bias was evaluated using the SYRCLE Risk of Bias tool for animal studies and the results are shown in Supplementary Table 6. Only 1 study out of the 70 analyzed, obtained 5 responses with low risk of bias and 5 with high risk or unclear. The remaining included studies presented most of the responses classified as high risk or unclear risk of bias. In detail, almost all studies showed a low risk of bias in components like groups similar at the baseline (80%) and selective reporting bias (93%). In addition, 59% presented low risk of bias in blinded outcome assessment domain. Most of the studies were scored as high risk or unclear risk of bias in several components, namely random group allocation, blinded group allocation, random housing, blinded intervention, random outcome assessment, reporting incomplete data and other sources of bias (Supplementary Table 6).
The present study provides a large and comprehensive summary of the recent research in preclinical CIPN models. We elected CIPN because it is a major problem both for cancer patients and cancer survivors and also due to our research interests in animal models of CIPN (21–23).
Research would benefit if animal models used in preclinical research are clinically relevant. In the case of paclitaxel-induced peripheral neuropathy models, at least one of the cancers treated with paclitaxel (breast cancer) is much more prevalent in females (99) cancer prevalence increases with age (100, 101). However, the characterization of sex and age of the animal population of the 70 analyzed studies shows that most studies were performed in young adult males. Only 4 studies aimed to investigate sex differences during CIPN (49, 66, 86, 88) which indicates that some researchers are concerned with the translational perspectives of the CIPN research. As to age, and besides the lack of studies in old animals, some studies do not even report the age of the animals used or only refer their weight, with need to indirectly infer the age of the animals. The importance of the use of aged animals should be highlighted due to the abovementioned age-related increase in cancer prevalence (100, 101) and since other comorbidities, such as depression, with an impact at CIPN often appear in aging populations (102). Collectively, and regarding sex and age issues, we consider that there are still challenges in translation of the results of the CIPN studies. Similar concerns about sex and age in pain types other than CIPN were previously pinpointed (24, 25). Those challenges in translation may be increased by additional issues of the animal population used in the studies namely lack of heterogeneity of the genetic background along with lack of report of the origin (animal supplier) and genetic background.
As to the features of CIPN induction, most studies aim to reproduce a chemotherapy cycle, and most paclitaxel doses were between 2–8 mg/Kg. A concern emerging from analyzing the studies relates to CIPN induction due to the heterogeneity of paclitaxel solvents, which was not always accompanied by the appropriate controls for the solvent. In several studies, the use of a control group for the solvent used in paclitaxel preparation was not even performed/reported. This is important because the solvents, such as DMSO at high concentrations, commonly used in CIPN studies, have significant neurotoxic effects (103). These neurotoxic effects can introduce important confounding factors. One of the settings of the present systematic review was the analysis of studies with CIPN induction ≥11 days and exclusion of studies with shorter timepoints. This time point was elected since the studies that validated the CIPN animal model evaluate putative clinically relevant pain-like behaviors at those time points the use of long post-induction periods may increase the translational perspectives of the studies (11, 104). Noteworthy, most of the studies under analysis in this systematic review used CIPN times between 14–28 days, which is a period in which mechanisms and neuroplastic changes underlying paclitaxel-induced neuropathy were established (21, 22, 105). However, with the improvement of early diagnosis of cancer and introduction of more effective treatments, besides the problem of CIPN during cancer treatment, another problem is CIPN-associated complaints by cancer survivors (3). These problems need better addressing since the longer time of CIPN study in the studies analyzed was 51 days (43, 50).
Regarding the methods used in the analyzed studies it should be highlighted the diversity of the techniques applied, namely behavioral analysis, histopathology, and biochemistry, which may be considered techniques with translational perspectives. Furthermore, almost all studies used diverse methods directed to enlighten a biological question. Curiously, a single study used brain imaging, which is a technique with a putative translational value (106). As to the behavioral analysis of nociception, most studies tested mechanical hypersensitivity. It should, however, be noted that the main complaints of the patients with CIPN are spontaneous pain and hypersensitivity related the thermal stimuli, including cold allodynia (1, 5, 6). Still regarding the behavioral studies, it should be noted that some studies aim to approach not only the nociceptive responses but also other responses that are interrelated with pain, such as spontaneous pain and emotions, which is important since pain is affected and has an impact in other functions which may be relevant in the translational perspective. Therefore, we conclude that the putative clinical relevance of the animal studies could be increased if the methods to study spontaneous pain will become more frequently used in the future.
As to the mechanisms underlying paclitaxel-induced peripheral neuropathy in the analyzed studies, it is interesting that besides the studies related to drugs frequently used in CIPN treatment, namely antidepressants with their action in serotonin and noradrenaline reuptake, the interest in neuroinflammation is increasing. It could be considered that this is in line with the research trends in other types of neuropathic pain, where inflammation and the role of the balance between pro- and anti-inflammatory cytokines is increasingly studied (107). Regarding the areas of the nervous system under analysis, almost all studies were focused on the peripheral fibers and, in a less extension, at the spinal cord. The supraspinal mechanisms of pain modulation during CIPN remain understudied in spite of the fact that pain is frequently associated with comorbidities, such as anxiety and depression, and in CIPN there are major neuroplastic changes in brain structures.
Regarding reporting and risk of bias, besides the abovementioned constraints in report (e.g., animal age, control groups and types of solvents), only 5 reports state that they were performed in accordance with the ARRIVE guidelines (Animal Research: Reporting of In Vivo Experiments). As to this and related to animal research, the importance of applying adequate experimental design and proper and detailed report, namely following the ARRIVE guidelines (28) needs to be considered to maximize the reproducibility of research, which is also important for the translational issues.
This systematic review includes 70 original papers and presents some limitations. Our first aim was to reflect about animal research on paclitaxel-induced neuropathy but due to the constraints of analyzing in detail large study samples, we excluded studies in which paclitaxel was combined with other therapeutical approaches. This analysis will be performed in a near future since the combination of cytostatic drugs with other approaches such as antibody therapy may decrease the cytostatic drug does or shorten the CIPN protocol. Also, we elected only paclitaxel due to its neurotoxic impact at the peripheral and central nervous system (108). We cannot, herein extrapolate the results of the present systematic review to other CIPN types. Furthermore, due to the exclusion/inclusion criteria and search in 2 databases the number of studies of paclitaxel-induced peripheral neuropathy may be underestimated. Finally, we did not analyze in detail the experimental design of the studies in what concerns the day of each behavioral test or the sequence of experiments because this was also underreported in several of the analyzed studies.
In conclusion, the present systematic review shows that there is a substantial effort in preclinical CIPN research. However, this systematic review also alerts to some potential problems related to underreporting which may mirror the poor experimental design. This could be overcome by a strict report of the ARRIVE guidelines, which is not requested by several journals along with implementation of the guidelines from PREPARE (Planning Research and Experimental Procedures on Animals: Recommendations for Excellence). The latter can substantially improve experimental planning since it takes into account aspects related to the formulation of study (e.g., literature searches, humane endpoints, and experimental design), dialog between scientists and colleagues from the animal facility (e.g., division of labor, education and training, and facility evaluation), and quality control of the components in the study (e.g., housing and husbandry, and necropsy) (109). The need to design studies which are representative of the problems of the CIPN patients, such as sex, age and pain types, needs to be considered. The use of correct terminology in the animal studies, avoiding the terms such as “pain” and “hyperalgesia”, and replacing by the hypersensitivity to noxious events, which has already discussed in other contexts (110), also needs to be considered by the authors and editorial managers of the journals. In conclusion, the detailed analysis of the animal studies of paclitaxel-induced peripheral neuropathy may alert to the importance of ameliorating the experimental design and report of the studies which is important for replication and translation of the results of animal studies into the clinical setting. This is relevant inasmuch that the aim of most of the analyzed studies was the clinical application of the results and since CIPN is a major clinical problem for cancer patients and cancer survivors.
The original contributions presented in the study are included in the article/Supplementary material, further inquiries can be directed to the corresponding author.
CB: Data curation, Formal analysis, Investigation, Writing – review & editing. JC-P: Conceptualization, Data curation, Formal analysis, Investigation, Writing – original draft, Writing – review & editing. IT: Conceptualization, Data curation, Funding acquisition, Investigation, Supervision, Writing – original draft, Writing – review & editing.
The author(s) declare financial support was received for the research, authorship, and/or publication of this article. This research was performed in the grant “Cátedra de Medicina da Dor” ascribed by Fundação Grunenthal to the Faculty of Medicine of the University of Porto.
The authors would like to thank Sandra Marisa Oliveira, from the Commission of Animal Welfare of the Faculty of Medicine of Porto (ORBEA), for the careful reading and suggestions included in the manuscript.
The authors declare that the research was conducted in the absence of any commercial or financial relationships that could be construed as a potential conflict of interest.
The author(s) declared that they were an editorial board member of Frontiers, at the time of submission. This had no impact on the peer review process and the final decision.
All claims expressed in this article are solely those of the authors and do not necessarily represent those of their affiliated organizations, or those of the publisher, the editors and the reviewers. Any product that may be evaluated in this article, or claim that may be made by its manufacturer, is not guaranteed or endorsed by the publisher.
The Supplementary material for this article can be found online at: https://www.frontiersin.org/articles/10.3389/fvets.2023.1264668/full#supplementary-material
1. Cavaletti, G, and Marmiroli, P. Chemotherapy-induced peripheral neurotoxicity. Nat Rev Neurol. (2010) 6:657–66. doi: 10.1038/nrneurol.2010.160
2. Flatters, SJL, Dougherty, PM, and Colvin, LA. Clinical and preclinical perspectives on Chemotherapy-Induced Peripheral Neuropathy (CIPN): a narrative review. Br J Anaesth. (2017) 119:737–49. doi: 10.1093/bja/aex229
3. Li, T, Park, SB, Battaglini, E, King, MT, Kiernan, MC, Goldstein, D, et al. Assessing chemotherapy-induced peripheral neuropathy with patient reported outcome measures: a systematic review of measurement properties and considerations for future use. Qual Life Res. (2022) 31:3091–107. doi: 10.1007/s11136-022-03154-7
4. Cavaletti, G, Frigeni, B, Lanzani, F, Mattavelli, L, Susani, E, Alberti, P, et al. Chemotherapy-Induced Peripheral Neurotoxicity assessment: a critical revision of the currently available tools. Eur J Cancer. (2010) 46:479–94. doi: 10.1016/j.ejca.2009.12.008
5. Kober, KM, Mazor, M, Abrams, G, Olshen, A, Conley, YP, Hammer, M, et al. Phenotypic Characterization of Paclitaxel-Induced Peripheral Neuropathy in Cancer Survivors. J Pain Symptom Manag. (2018) 56:e903:908–919.e3. doi: 10.1016/j.jpainsymman.2018.08.017
6. Scripture, CD, Figg, WD, and Sparreboom, A. Peripheral neuropathy induced by paclitaxel: recent insights and future perspectives. Curr Neuropharmacol. (2006) 4:165–72. doi: 10.2174/157015906776359568
7. Colvin, LA. Chemotherapy-induced peripheral neuropathy: where are we now? Pain. (2019) 160:S1–S10. doi: 10.1097/j.pain.0000000000001540
8. Staff, NP, Fehrenbacher, JC, Caillaud, M, Damaj, MI, Segal, RA, and Rieger, S. Pathogenesis of paclitaxel-induced peripheral neuropathy: A current review of in vitro and in vivo findings using rodent and human model systems. Exp Neurol. (2020) 324:113121. doi: 10.1016/j.expneurol.2019.113121
9. Doyle, T, Chen, Z, Muscoli, C, Bryant, L, Esposito, E, Cuzzocrea, S, et al. Targeting the overproduction of peroxynitrite for the prevention and reversal of paclitaxel-induced neuropathic pain. J Neurosci. (2012) 32:6149–60. doi: 10.1523/JNEUROSCI.6343-11.2012
10. Gadgil, S, Ergun, M, Van Den Heuvel, SA, Van Der Wal, SE, Scheffer, GJ, and Hooijmans, CR. A systematic summary and comparison of animal models for chemotherapy induced (peripheral) neuropathy (CIPN). PLoS One. (2019) 14:e0221787. doi: 10.1371/journal.pone.0221787
11. Polomano, RC, Mannes, AJ, Clark, US, and Bennett, GJ. A painful peripheral neuropathy in the rat produced by the chemotherapeutic drug, paclitaxel. Pain. (2001) 94:293–304. doi: 10.1016/S0304-3959(01)00363-3
12. Griffiths, LA, Duggett, NA, Pitcher, AL, and Flatters, SJL. Evoked and Ongoing Pain-Like Behaviours in a Rat Model of Paclitaxel-Induced Peripheral Neuropathy. Pain Res Manag. (2018) 2018:1–11. doi: 10.1155/2018/8217613
13. Toma, W, Kyte, SL, Bagdas, D, Alkhlaif, Y, Alsharari, SD, Lichtman, AH, et al. Effects of paclitaxel on the development of neuropathy and affective behaviors in the mouse. Neuropharmacology. (2017) 117:305–15. doi: 10.1016/j.neuropharm.2017.02.020
14. Mcneish, BL, Richardson, JK, and Whitney, DG. Chemotherapy-induced peripheral neuropathy onset is associated with early risk of depression and anxiety in breast cancer survivors. Eur J Cancer Care. (2022) 31:e13648. doi: 10.1111/ecc.13648
15. Boehmerle, W, Huehnchen, P, Peruzzaro, S, Balkaya, M, and Endres, M. Electrophysiological, behavioral and histological characterization of paclitaxel, cisplatin, vincristine and bortezomib-induced neuropathy in C57Bl/6 mice. Sci Rep. (2014) 4:6370. doi: 10.1038/srep06370
16. Flatters, SJL, and Bennett, GJ. Studies of peripheral sensory nerves in paclitaxel-induced painful peripheral neuropathy: evidence for mitochondrial dysfunction. Pain. (2006) 122:245–57. doi: 10.1016/j.pain.2006.01.037
17. Li, Y, Adamek, P, Zhang, H, Tatsui, CE, Rhines, LD, Mrozkova, P, et al. The Cancer Chemotherapeutic Paclitaxel Increases Human and Rodent Sensory Neuron Responses to TRPV1 by Activation of TLR4. J Neurosci. (2015) 35:13487–500. doi: 10.1523/JNEUROSCI.1956-15.2015
18. Peters, CM, Jimenez-Andrade, JM, Kuskowski, MA, Ghilardi, JR, and Mantyh, PW. An evolving cellular pathology occurs in dorsal root ganglia, peripheral nerve and spinal cord following intravenous administration of paclitaxel in the rat. Brain Res. (2007) 1168:46–59. doi: 10.1016/j.brainres.2007.06.066
19. Siau, C, Xiao, W, and Bennett, GJ. Paclitaxel- and vincristine-evoked painful peripheral neuropathies: loss of epidermal innervation and activation of Langerhans cells. Exp Neurol. (2006) 201:507–14. doi: 10.1016/j.expneurol.2006.05.007
20. Zhang, H, Yoon, SY, Zhang, H, and Dougherty, PM. Evidence that spinal astrocytes but not microglia contribute to the pathogenesis of Paclitaxel-induced painful neuropathy. J Pain. (2012) 13:293–303. doi: 10.1016/j.jpain.2011.12.002
21. Costa-Pereira, JT, Ribeiro, J, Martins, I, and Tavares, I. Role of Spinal Cord α2-Adrenoreceptors in Noradrenergic Inhibition of Nociceptive Transmission During Chemotherapy-Induced Peripheral Neuropathy. Front Neurosci. (2020a) 13:413. doi: 10.3389/fnins.2019.01413
22. Costa-Pereira, JT, Serrão, P, Martins, I, and Tavares, I. Serotoninergic pain modulation from the rostral ventromedial medulla (RVM) in chemotherapy-induced neuropathy: The role of spinal 5-HT3 receptors. Eur J Neurosci. (2020b) 51:1756–69. doi: 10.1111/ejn.14614
23. Costa-Pereira, JT, Oliveira, R, Guadilla, I, Guillen, MJ, Tavares, I, and Lopez-Larrubia, P. Neuroimaging uncovers neuronal and metabolic changes in pain modulatory brain areas in a rat model of chemotherapy-induced neuropathy—MEMRI and ex vivo spectroscopy studies. Brain Res Bull. (2023) 192:12–20. doi: 10.1016/j.brainresbull.2022.10.018
24. Mogil, JS. The translatability of pain across species. Philos Trans R Soc Lond Ser B Biol Sci. (2019) 374:20190286. doi: 10.1098/rstb.2019.0286
25. Mogil, JS. Qualitative sex differences in pain processing: emerging evidence of a biased literature. Nat Rev Neurosci. (2020) 21:353–65. doi: 10.1038/s41583-020-0310-6
26. Ellis, A, and Bennett, DL. Neuroinflammation and the generation of neuropathic pain. Br J Anaesth. (2013) 111:26–37. doi: 10.1093/bja/aet128
27. Areti, A, Yerra, VG, Naidu, V, and Kumar, A. Oxidative stress and nerve damage: role in chemotherapy induced peripheral neuropathy. Redox Biol. (2014) 2:289–95. doi: 10.1016/j.redox.2014.01.006
28. Percie Du Sert, N, Hurst, V, Ahluwalia, A, Alam, S, Avey, MT, Baker, M, et al. The ARRIVE guidelines 2.0: Updated guidelines for reporting animal research. PLoS Biol. (2020) 18:e3000410. doi: 10.1371/journal.pbio.3000410
29. Fonseca-Rodrigues, D, Almeida, A, and Pinto-Ribeiro, F. A New Gal in Town: A Systematic Review of the Role of Galanin and Its Receptors in Experimental Pain. Cells. (2022) 11:839. doi: 10.3390/cells11050839
30. Hooijmans, CR, Rovers, MM, De Vries, RB, Leenaars, M, Ritskes-Hoitinga, M, and Langendam, MW. SYRCLE's risk of bias tool for animal studies. BMC Med Res Methodol. (2014) 14:43. doi: 10.1186/1471-2288-14-43
31. Matesanz-Garcia, L, Schmid, AB, Caceres-Pajuelo, JE, Cuenca-Martinez, F, Arribas-Romano, A, Gonzalez-Zamorano, Y, et al. Effect of Physiotherapeutic Interventions on Biomarkers of Neuropathic Pain: A Systematic Review of Preclinical Literature. J Pain. (2022) 23:1833–55. doi: 10.1016/j.jpain.2022.06.007
32. Li, DY, Gao, SJ, Sun, J, Zhang, LQ, Wu, JY, Song, FH, et al. Notch signaling activation contributes to paclitaxel-induced neuropathic pain via activation of A1 astrocytes. Eur J Pharmacol. (2022) 928:175130. doi: 10.1016/j.ejphar.2022.175130
33. Ma, D, Wang, X, Liu, X, Li, Z, Liu, J, Cao, J, et al. Macrophage Infiltration Initiates RIP3/MLKL-Dependent Necroptosis in Paclitaxel-Induced Neuropathic Pain. Mediat Inflamm. (2022) 2022:1–10. doi: 10.1155/2022/1567210
34. Nasser, AH, Gendy, AM, El-Yamany, MF, and El-Tanbouly, DM. Upregulation of neuronal progranulin mediates the antinociceptive effect of trimetazidine in paclitaxel-induced peripheral neuropathy: Role of ERK1/2 signaling. Toxicol Appl Pharmacol. (2022) 448:116096. doi: 10.1016/j.taap.2022.116096
35. Sezer, G, Yay, AH, Sarica, ZS, Gonen, Z, Onder, GO, Alan, A, et al. Bone marrow-derived mesenchymal stem cells alleviate paclitaxel-induced mechanical allodynia in rats. J Biochem Mol Toxicol. (2022) 36:e23207. doi: 10.1002/jbt.23207
36. Wang, J, Zhou, F, Zhang, S, Mao, M, Feng, S, and Wang, X. Participation of transient receptor potential vanilloid 1 in the analgesic effect of duloxetine for paclitaxel induced peripheral neuropathic pain. Neurosci Lett. (2022) 773:136512. doi: 10.1016/j.neulet.2022.136512
37. Alkislar, I, Miller, AR, Hohmann, AG, Sadaka, AH, Cai, X, Kulkarni, P, et al. Inhaled Cannabis Suppresses Chemotherapy-Induced Neuropathic Nociception by Decoupling the Raphe Nucleus: A Functional Imaging Study in Rats. Biol Psychiatry Cogn Neurosci Neuroimaging. (2021) 6:479–89. doi: 10.1016/j.bpsc.2020.11.015
38. Chen, N, Ge, MM, Li, DY, Wang, XM, Liu, DQ, Ye, DW, et al. β2-adrenoreceptor agonist ameliorates mechanical allodynia in paclitaxel-induced neuropathic pain via induction of mitochondrial biogenesis. Biomed Pharmacother. (2021) 144:112331. doi: 10.1016/j.biopha.2021.112331
39. Chou, PR, Lu, CY, Kan, JY, Wang, SH, Lo, JJ, Huang, SH, et al. Simultaneous hyperbaric oxygen therapy during systemic chemotherapy reverses chemotherapy-induced peripheral neuropathy by inhibiting TLR4 and TRPV1 activation in the central and peripheral nervous system. Support Care Cancer. (2021) 29:6841–50. doi: 10.1007/s00520-021-06269-8
40. Garrido-Suárez, BB, Garrido, G, Menéndez, AB, Aparicio, G, Valdés, O, Ochoa-Rodríguez, E, et al. Preventive and therapeutic effects of JM-20 on paclitaxel-evoked painful peripheral neuropathy in rats. J Pharmacy Pharmacogn Res. (2021) 9:165–74. doi: 10.56499/jppres20.970_9.2.165
41. Ilari, S, Lauro, F, Giancotti, LA, Malafoglia, V, Dagostino, C, Gliozzi, M, et al. The protective effect of bergamot polyphenolic fraction (Bpf) on chemotherapy-induced neuropathic pain. Pharmaceuticals. (2021) 14:975. doi: 10.3390/ph14100975
42. Ma, D, Zhao, S, Liu, X, Li, Z, Li, H, Liu, J, et al. RIP3/MLKL pathway-regulated necroptosis: A new mechanism of paclitaxel-induced peripheral neuropathy. J Biochem Mol Toxicol. (2021) 35:e22834. doi: 10.1002/jbt.22834
43. Meregalli, C, Monza, L, Chiorazzi, A, Scali, C, Guarnieri, C, Fumagalli, G, et al. Human Intravenous Immunoglobulin Alleviates Neuropathic Symptoms in a Rat Model of Paclitaxel-Induced Peripheral Neurotoxicity. Int J Mol Sci. (2021) 22:1058. doi: 10.3390/ijms22031058
44. Semis, HS, Kandemir, FM, Kaynar, O, Dogan, T, and Arikan, SM. The protective effects of hesperidin against paclitaxel-induced peripheral neuropathy in rats. Life Sci. (2021) 287:120104. doi: 10.1016/j.lfs.2021.120104
45. Wang, G, Zhang, X, Pan, X, and Xiao, Y. FSC231 can alleviate paclitaxel-induced neuralgia by inhibiting PICK1 and affecting related factors. Neurosci Lett. (2021) 741:135471. doi: 10.1016/j.neulet.2020.135471
46. Zhang, X, Wang, J, Ran, R, Peng, Y, and Xiao, Y. FSC231 alleviates paclitaxel-induced neuralgia by inhibiting the interactions between PICK1 and GluA2 and activates GSK-3β and ERK1/2. Brain Behav. (2021) 11:e2380. doi: 10.1002/brb3.2380
47. Zhong, S, Zhou, Z, Lin, X, Liu, F, Liu, C, Liu, Z, et al. Ketogenic diet prevents paclitaxel-induced neuropathic nociception through activation of PPARγ signalling pathway and inhibition of neuroinflammation in rat dorsal root ganglion. Eur J Neurosci. (2021) 54:5341–56. doi: 10.1111/ejn.15397
48. Brewer, AL, Shirachi, DY, Quock, RM, and Craft, RM. Effect of hyperbaric oxygen on chemotherapy-induced neuropathy in male and female rats. Behav Pharmacol. (2020) 31:61–72. doi: 10.1097/FBP.0000000000000497
49. Ferrari, LF, Araldi, D, Green, PG, and Levine, JD. Marked sexual dimorphism in neuroendocrine mechanisms for the exacerbation of paclitaxel-induced painful peripheral neuropathy by stress. Pain. (2020) 161:865–74. doi: 10.1097/j.pain.0000000000001798
50. Hacimuftuoglu, A, Mohammadzadeh, M, Taghizadehghalehjoughi, A, Taspinar, N, Togar, B, Nalcı, KA, et al. The analgesic effect of metformin on paclitaxel-induced neuropathic pain model in rats: By considering pathological results. J Cancer Res Ther. (2020) 16:34–9. doi: 10.4103/jcrt.JCRT_1455_16
51. Huang, J, Chen, D, Yan, F, Wu, S, Kang, S, Xing, W, et al. JTC-801 alleviates mechanical allodynia in paclitaxel-induced neuropathic pain through the PI3K/Akt pathway. Eur J Pharmacol. (2020) 883:173306. doi: 10.1016/j.ejphar.2020.173306
52. Kamata, Y, Kambe, T, Chiba, T, Yamamoto, K, Kawakami, K, Abe, K, et al. Paclitaxel Induces Upregulation of Transient Receptor Potential Vanilloid 1 Expression in the Rat Spinal Cord. Int J Mol Sci. (2020) 21:4341. doi: 10.3390/ijms21124341
53. Kim, HK, Lee, SY, Koike, N, Kim, E, Wirianto, M, Burish, MJ, et al. Circadian regulation of chemotherapy-induced peripheral neuropathic pain and the underlying transcriptomic landscape. Sci Rep. (2020) 10:13844. doi: 10.1038/s41598-020-70757-w
54. Liu, X, Wang, G, Ai, G, Xu, X, Niu, X, and Zhang, M. Selective ablation of descending serotonin from the rostral ventromedial medulla unmasks its pro-nociceptive role in chemotherapy-induced painful neuropathy. J Pain Res. (2020) 13:3081–94. doi: 10.2147/JPR.S275254
55. Wang, XM, Gu, P, Saligan, L, Iadarola, M, Wong, SSC, Ti, LK, et al. Dysregulation of EAAT2 and VGLUT2 Spinal Glutamate Transports via Histone Deacetylase 2 (HDAC2) Contributes to Paclitaxel-induced Painful Neuropathy. Mol Cancer Ther. (2020) 19:2196–209. doi: 10.1158/1535-7163.MCT-20-0006
56. Zhang, R, Gan, Y, Li, J, and Feng, Y. Vagus nerve stimulation transiently mitigates chemotherapy-induced peripheral neuropathy in rats. J Pain Res. (2020) 13:3457–65. doi: 10.2147/JPR.S281190
57. Zhao, YX, Yao, MJ, Liu, Q, Xin, JJ, Gao, JH, and Yu, XC. Electroacupuncture treatment attenuates paclitaxel-induced neuropathic pain in rats via inhibiting spinal glia and the TLR4/NF-κB pathway. J Pain Res. (2020) 13:239–50. doi: 10.2147/JPR.S241101
58. Zhou, YQ, Liu, DQ, Chen, SP, Chen, N, Sun, J, Wang, XM, et al. Nrf2 activation ameliorates mechanical allodynia in paclitaxel-induced neuropathic pain. Acta Pharmacol Sin. (2020a) 41:1041–8. doi: 10.1038/s41401-020-0394-6
59. Zhou, YQ, Liu, DQ, Chen, SP, Chen, N, Sun, J, Wang, XM, et al. PPARγ activation mitigates mechanical allodynia in paclitaxel-induced neuropathic pain via induction of Nrf2/HO-1 signaling pathway. Biomed Pharmacother. (2020b) 129:110356. doi: 10.1016/j.biopha.2020.110356
60. Li, Y, Yin, C, Li, X, Liu, B, Wang, J, Zheng, X, et al. Electroacupuncture Alleviates Paclitaxel-Induced Peripheral Neuropathic Pain in Rats via Suppressing TLR4 Signaling and TRPV1 Upregulation in Sensory Neurons. Int J Mol Sci. (2019) 20:5917. doi: 10.3390/ijms20235917
61. Sivanesan, E, Stephens, KE, Huang, Q, Chen, Z, Ford, NC, Duan, W, et al. Spinal cord stimulation prevents paclitaxel-induced mechanical and cold hypersensitivity and modulates spinal gene expression in rats. Pain Rep. (2019) 4:785. doi: 10.1097/PR9.0000000000000785
62. Wu, J, Hocevar, M, Bie, B, Foss, JF, and Naguib, M. Cannabinoid Type 2 Receptor System Modulates Paclitaxel-Induced Microglial Dysregulation and Central Sensitization in Rats. J Pain. (2019) 20:501–14. doi: 10.1016/j.jpain.2018.10.007
63. Wu, Y, Chen, J, and Wang, R. Puerarin suppresses TRPV1, calcitonin gene-related peptide and substance P to prevent paclitaxel-induced peripheral neuropathic pain in rats. Neuroreport. (2019) 30:288–94. doi: 10.1097/WNR.0000000000001199
64. Al-Mazidi, S, Alotaibi, M, Nedjadi, T, Chaudhary, A, Alzoghaibi, M, and Djouhri, L. Blocking of cytokines signalling attenuates evoked and spontaneous neuropathic pain behaviours in the paclitaxel rat model of chemotherapy-induced neuropathy. Eur J Pain. (2018) 22:810–21. doi: 10.1002/ejp.1169
65. Ba, X, Wang, J, Zhou, S, Luo, X, Peng, Y, Yang, S, et al. Cinobufacini protects against paclitaxel-induced peripheral neuropathic pain and suppresses TRPV1 up-regulation and spinal astrocyte activation in rats. Biomed Pharmacother. (2018) 108:76–84. doi: 10.1016/j.biopha.2018.09.018
66. Legakis, LP, Bigbee, JW, and Negus, SS. Lack of paclitaxel effects on intracranial self-stimulation in male and female rats: comparison to mechanical sensitivity. Behav Pharmacol. (2018) 29:290–8. doi: 10.1097/FBP.0000000000000378
67. Vitet, L, Patte-Mensah, C, Boujedaini, N, Mensah-Nyagan, AG, and Meyer, L. Beneficial effects of Gelsemium-based treatment against paclitaxel-induced painful symptoms. Neurol Sci. (2018) 39:2183–96. doi: 10.1007/s10072-018-3575-z
68. Zhang, Y, Li, A, Xin, J, Ren, K, Berman, BM, Lao, L, et al. Electroacupuncture alleviates chemotherapy-induced pain through inhibiting phosphorylation of spinal CaMKII in rats. Eur J Pain. (2018) 22:679–90. doi: 10.1002/ejp.1132
69. Balkrishna, A, Karumuri, S, Sakat, SS, Haldar, S, and Varshney, A. Anti-oxidant profile of Divya-Peedantak-Vati abates paclitaxel-induced hyperalgesia and allodynia in CD-1 mice model of neuropathic pain. Phytomed Plus. (2022) 2:100229. doi: 10.1016/j.phyplu.2022.100229
70. Cristiano, C, Avagliano, C, Cuozzo, M, Liguori, FM, Calignano, A, and Russo, R. The Beneficial Effects of Ultramicronized Palmitoylethanolamide in the Management of Neuropathic Pain and Associated Mood Disorders Induced by Paclitaxel in Mice. Biomol Ther. (2022) 12:1155. doi: 10.3390/biom12081155
71. Ezaka, M, Marutani, E, Miyazaki, Y, Kanemaru, E, Selig, MK, Boerboom, SL, et al. Oral Administration of Glutathione Trisulfide Increases Reactive Sulfur Levels in Dorsal Root Ganglion and Ameliorates Paclitaxel-Induced Peripheral Neuropathy in Mice. Antioxidants. (2022) 11:2122. doi: 10.3390/antiox11112122
72. Karmakar, V, Mohammad, FS, Baeesa, SS, Alexiou, A, Sivakumar, SR, and Ashraf, GM. Effect of Cliothosa aurivilli on Paclitaxel-induced Peripheral Neuropathy in Experimental Animals. Mol Neurobiol. (2022) 59:2232–45. doi: 10.1007/s12035-021-02685-3
73. Lin, X, Xu, Z, Carey, L, Romero, J, Makriyannis, A, Hillard, CJ, et al. A peripheral CB2 cannabinoid receptor mechanism suppresses chemotherapy-induced peripheral neuropathy: evidence from a CB2 reporter mouse. Pain. (2022) 163:834–51. doi: 10.1097/j.pain.0000000000002502
74. Park, SE, Neupane, C, Noh, C, Sharma, R, Shin, HJ, Pham, TL, et al. Antiallodynic effects of KDS2010, a novel MAO-B inhibitor, via ROS-GABA inhibitory transmission in a paclitaxel-induced tactile hypersensitivity model. Mol Brain. (2022) 15:41. doi: 10.1186/s13041-022-00924-9
75. Paton, KF, Luo, D, La Flamme, AC, Prisinzano, TE, and Kivell, BM. Sex Differences in Kappa Opioid Receptor Agonist Mediated Attenuation of Chemotherapy-Induced Neuropathic Pain in Mice. Front Pharmacol. (2022) 13:3562. doi: 10.3389/fphar.2022.813562
76. Caillaud, M, Patel, NH, Toma, W, White, A, Thompson, D, Mann, J, et al. A fenofibrate diet prevents paclitaxel-induced peripheral neuropathy in mice. Cancer. (2021a) 13:1–21. doi: 10.3390/cancers13010069
77. Caillaud, M, Patel, NH, White, A, Wood, M, Contreras, KM, Toma, W, et al. Targeting Peroxisome Proliferator-Activated Receptor-α (PPAR-α) to reduce paclitaxel-induced peripheral neuropathy. Brain Behav Immun. (2021b) 93:172–85. doi: 10.1016/j.bbi.2021.01.004
78. Cuozzo, M, Castelli, V, Avagliano, C, Cimini, A, D’angelo, M, Cristiano, C, et al. Effects of chronic oral probiotic treatment in paclitaxel-induced neuropathic pain. Biomedicine. (2021) 9:346. doi: 10.3390/biomedicines9040346
79. Foss, JD, Farkas, DJ, Huynh, LM, Kinney, WA, Brenneman, DE, and Ward, SJ. Behavioural and pharmacological effects of cannabidiol (CBD) and the cannabidiol analogue KLS-13019 in mouse models of pain and reinforcement. Br J Pharmacol. (2021) 178:3067–78. doi: 10.1111/bph.15486
80. Son, DB, Choi, W, Kim, M, Go, EJ, Jeong, D, Park, CK, et al. Decursin Alleviates Mechanical Allodynia in a Paclitaxel-Induced Neuropathic Pain Mouse Model. Cells. (2021) 10:547. doi: 10.3390/cells10030547
81. Takanashi, K, Shibata, K, Mizuno, K, Komatsu, R, and Koizumi, S. Goshajinkigan attenuates paclitaxel-induced neuropathic pain via cortical astrocytes. Pharmacol Res Perspect. (2021) 9:e00850. doi: 10.1002/prp2.850
82. Wang, H, Shen, YJ, Li, XJ, Xia, J, Sun, L, Xu, Y, et al. DNMT3b SUMOylation Mediated MMP-2 Upregulation Contribute to Paclitaxel Induced Neuropathic Pain. Neurochem Res. (2021) 46:1214–23. doi: 10.1007/s11064-021-03260-x
83. Balkrishna, A, Sakat, SS, Karumuri, S, Singh, H, Tomer, M, Kumar, A, et al. Herbal Decoction Divya-Peedantak-Kwath Alleviates Allodynia and Hyperalgesia in Mice Model of Chemotherapy-Induced Peripheral Neuropathy via Modulation in Cytokine Response. Front Pharmacol. (2020) 11:6490. doi: 10.3389/fphar.2020.566490
84. Biggerstaff, A, Kivell, B, Smith, JL, Mian, MY, Golani, LK, Rashid, F, et al. The α2,3-selective potentiators of GABA(A) receptors, KRM-II-81 and MP-III-80, produce anxiolytic-like effects and block chemotherapy-induced hyperalgesia in mice without tolerance development. Pharmacol Biochem Behav. (2020) 196:172996. doi: 10.1016/j.pbb.2020.172996
85. Chen, LH, Yeh, YM, Chen, YF, Hsu, YH, Wang, HH, Lin, PC, et al. Targeting interleukin-20 alleviates paclitaxel-induced peripheral neuropathy. Pain. (2020) 161:1237–54. doi: 10.1097/j.pain.0000000000001831
86. Liang, L, Wei, J, Tian, L, Padma Nagendra, BV, Gao, F, Zhang, J, et al. Paclitaxel Induces Sex-biased Behavioral Deficits and Changes in Gene Expression in Mouse Prefrontal Cortex. Neuroscience. (2020) 426:168–78. doi: 10.1016/j.neuroscience.2019.11.031
87. Lu, Y, Zhang, P, Zhang, Q, Yang, C, Qian, Y, Suo, J, et al. Duloxetine Attenuates Paclitaxel-Induced Peripheral Nerve Injury by Inhibiting p53-Related Pathways. J Pharmacol Exp Ther. (2020) 373:453–62. doi: 10.1124/jpet.120.265082
88. Inyang, KE, Mcdougal, TA, Ramirez, ED, Williams, M, Laumet, G, Kavelaars, A, et al. Alleviation of paclitaxel-induced mechanical hypersensitivity and hyperalgesic priming with AMPK activators in male and female mice. Neurobiol Pain. (2019) 6:100037. doi: 10.1016/j.ynpai.2019.100037
89. Kaur, S, and Muthuraman, A. Ameliorative effect of gallic acid in paclitaxel-induced neuropathic pain in mice. Toxicol Rep. (2019) 6:505–13. doi: 10.1016/j.toxrep.2019.06.001
90. Mao, Q, Wu, S, Gu, X, Du, S, Mo, K, Sun, L, et al. DNMT3a-triggered downregulation of K(2p) 1.1 gene in primary sensory neurons contributes to paclitaxel-induced neuropathic pain. Int J Cancer. (2019) 145:2122–34. doi: 10.1002/ijc.32155
91. Ramakrishna, C, Corleto, J, Ruegger, PM, Logan, GD, Peacock, BB, Mendonca, S, et al. Dominant Role of the Gut Microbiota in Chemotherapy Induced Neuropathic Pain. Sci Rep. (2019) 9:20324. doi: 10.1038/s41598-019-56832-x
92. Slivicki, RA, Mali, SS, and Hohmann, AG. Voluntary exercise reduces both chemotherapy-induced neuropathic nociception and deficits in hippocampal cellular proliferation in a mouse model of paclitaxel-induced peripheral neuropathy. Neurobiol Pain. (2019) 6:100035. doi: 10.1016/j.ynpai.2019.100035
93. Tonello, R, Lee, SH, and Berta, T. Monoclonal Antibody Targeting the Matrix Metalloproteinase 9 Prevents and Reverses Paclitaxel-Induced Peripheral Neuropathy in Mice. J Pain. (2019) 20:515–27. doi: 10.1016/j.jpain.2018.11.003
94. Lin, X, Dhopeshwarkar, AS, Huibregtse, M, Mackie, K, and Hohmann, AG. Slowly Signaling G Protein-Biased CB(2) Cannabinoid Receptor Agonist LY2828360 Suppresses Neuropathic Pain with Sustained Efficacy and Attenuates Morphine Tolerance and Dependence. Mol Pharmacol. (2018) 93:49–62. doi: 10.1124/mol.117.109355
95. Kim, HK, Bae, J, Lee, SH, Hwang, SH, Kim, MS, Kim, MJ, et al. Blockers of Wnt3a, Wnt10a, or β-Catenin Prevent Chemotherapy-Induced Neuropathic Pain In Vivo. Neurotherapeutics. (2021) 18:601–14. doi: 10.1007/s13311-020-00956-w
96. Huynh, PN, Giuvelis, D, Christensen, S, Tucker, KL, and Mcintosh, JM. RgIA4 accelerates recovery from paclitaxel-induced neuropathic pain in rats. Mar Drugs. (2020) 18:12. doi: 10.3390/md18010012
97. Chen, Y, Chen, SR, Chen, H, Zhang, J, and Pan, HL. Increased α2δ-1-NMDA receptor coupling potentiates glutamatergic input to spinal dorsal horn neurons in chemotherapy-induced neuropathic pain. J Neurochem. (2019) 148:252–74. doi: 10.1111/jnc.14627
98. Nie, B, Liu, C, Bai, X, Chen, X, Wu, S, Zhang, S, et al. AKAP150 involved in paclitaxel-induced neuropathic pain via inhibiting CN/NFAT2 pathway and downregulating IL-4. Brain Behav Immun. (2018) 68:158–68. doi: 10.1016/j.bbi.2017.10.015
99. Tong, CWS, Wu, M, Cho, WCS, and To, KKW. Recent Advances in the Treatment of Breast Cancer. Front Oncol. (2018) 8:227. doi: 10.3389/fonc.2018.00227
100. Miller, KD, Nogueira, L, Devasia, T, Mariotto, AB, Yabroff, KR, Jemal, A, et al. Cancer treatment and survivorship statistics, 2022. CA Cancer J Clin. (2022) 72:409–36. doi: 10.3322/caac.21731
101. White, MC, Holman, DM, Boehm, JE, Peipins, LA, Grossman, M, and Henley, SJ. Age and cancer risk: a potentially modifiable relationship. Am J Prev Med. (2014) 46:S7–S15. doi: 10.1016/j.amepre.2013.10.029
102. Alexopoulos, GS. Depression in the elderly. Lancet. (2005) 365:1961–70. doi: 10.1016/S0140-6736(05)66665-2
103. Worthley, EG, and Schott, CD. The toxicity of four concentrations of DMSO. Toxicol Appl Pharmacol. (1969) 15:275–81. doi: 10.1016/0041-008X(69)90027-1
104. Segat, GC, Manjavachi, MN, Matias, DO, Passos, GF, Freitas, CS, Costa, R, et al. Antiallodynic effect of beta-caryophyllene on paclitaxel-induced peripheral neuropathy in mice. Neuropharmacology. (2017) 125:207–19. doi: 10.1016/j.neuropharm.2017.07.015
105. Chiba, T, Oka, Y, Kambe, T, Koizumi, N, Abe, K, Kawakami, K, et al. Paclitaxel-induced peripheral neuropathy increases substance P release in rat spinal cord. Eur J Pharmacol. (2016) 770:46–51. doi: 10.1016/j.ejphar.2015.11.055
106. Tracey, I. Neuroimaging mechanisms in pain: from discovery to translation. Pain. (2017) 158:S115–22. doi: 10.1097/j.pain.0000000000000863
107. Sommer, C, Leinders, M, and Uceyler, N. Inflammation in the pathophysiology of neuropathic pain. Pain. (2018) 159:595–602. doi: 10.1097/j.pain.0000000000001122
108. Xu, Y, Jiang, Z, and Chen, X. Mechanisms underlying paclitaxel-induced neuropathic pain: Channels, inflammation and immune regulations. Eur J Pharmacol. (2022) 933:175288. doi: 10.1016/j.ejphar.2022.175288
109. Smith, AJ, Clutton, RE, Lilley, E, Hansen, KEA, and Brattelid, T. PREPARE: guidelines for planning animal research and testing. Lab Anim. (2018) 52:135–41. doi: 10.1177/0023677217724823
Keywords: chemotherapy, neuropathic pain, animal models, reproducibility, clinical translation
Citation: Bacalhau C, Costa-Pereira JT and Tavares I (2023) Preclinical research in paclitaxel-induced neuropathic pain: a systematic review. Front. Vet. Sci. 10:1264668. doi: 10.3389/fvets.2023.1264668
Received: 25 July 2023; Accepted: 21 November 2023;
Published: 18 December 2023.
Edited by:
Ping Yang, Nanjing Agricultural University, ChinaReviewed by:
Hee Kee Kim, University of Texas MD Anderson Cancer Center, United StatesCopyright © 2023 Bacalhau, Costa-Pereira and Tavares. This is an open-access article distributed under the terms of the Creative Commons Attribution License (CC BY). The use, distribution or reproduction in other forums is permitted, provided the original author(s) and the copyright owner(s) are credited and that the original publication in this journal is cited, in accordance with accepted academic practice. No use, distribution or reproduction is permitted which does not comply with these terms.
*Correspondence: Isaura Tavares, aXNhdGF2QG1lZC51cC5wdA==
†These authors share first authorship
Disclaimer: All claims expressed in this article are solely those of the authors and do not necessarily represent those of their affiliated organizations, or those of the publisher, the editors and the reviewers. Any product that may be evaluated in this article or claim that may be made by its manufacturer is not guaranteed or endorsed by the publisher.
Research integrity at Frontiers
Learn more about the work of our research integrity team to safeguard the quality of each article we publish.