- 1School of Life Sciences and Food Engineering, Hebei University of Engineering, Handan, China
- 2Chenguang Biotechnology Group Handan Co., Ltd., Handan, China
- 3Hebei Plant Extraction Innovation Center Co., Ltd., Handan, China
- 4Hebei Province Plant Source Animal Health Products Technology Innovation Center, Handan, China
Ursolic acid (UA) is a plant-derived pentacyclic triterpenoid with 30 carbon atoms. UA has anti-inflammatory, antioxidative, antimicrobial, hepato-protective, anticancer, and other biological activities. Most studies on the biological functions of UA have been performed in mammalian cell (in vitro) and rodent (in vivo) models. UA is used in animal husbandry as an anti-inflammatory and antiviral agent, as well as for enhancing the integrity of the intestinal barrier. Although UA has been shown to have significant in vitro bacteriostatic effects, it is rarely used in animal nutrition. The use of UA as a substitute for oral antibiotics or as a novel feed additive in animal husbandry should be considered. This review summarizes the available data on the biological functions of UA and its applications in animal husbandry.
1. Introduction
Natural products derived from plants, including polyphenols, flavonoids, terpenoids, essential oils, and alkaloids, have many biological and pharmacological properties, including antibacterial, antiviral, anticancer, anti-inflammatory, anti-diabetic, and hepato-protective activities (1). Ursolic acid (UA) is a natural plant product with the chemical formula of C30H48O3 (Figure 1). It is found in the stem bark, leaves, and peel of Chinese herbs and fruits and has been shown to have a wide range of pharmaceutical properties (Table 1). UA contents vary in different plants, parts of plants, and the sources of plants (15), ranging from 0.091 to 1.58% in five different species of the Lamiaceae family (Rosmarinus officinalis L., Salvia officinalis L., Satureja montana L., Salvia sclarea L., and Salvia glutinosa L.) (16), reaching 49.7% in apple pomace and 22.7% in rosemary leaves (17). UA contents tend to be highest in flowers and leaves, and lower in stems and rhizomes (18). Details on UA contents and extraction methods from different plants are summarized in Table 2.
In terms of biopharmaceutical classification, UA is a Class IV compound (“low” solubility and “low” permeability) (26). The clinical application of UA is limited because of its poor bioavailability, together with low intestinal permeability and solubility. UA can be used as a component in the vesicle-like nanocarrier system because of its small size. Moreover, UA can act not only as an anticancer additive in the nanocarrier system, but it also shows synergy with other drugs, indicating further advantages in medical treatments (27). Previous research has suggested several strategies, including complexation with hydrophilic cyclodextrins (28), structure modification (29), nanotechnology (30), and creating a supramolecular coamorphous system of UA with piperine (31), to overcome these limitations.
UA has been proposed as a candidate drug for treating various cancers (32–35), inflammatory diseases (36), diabetes (37), Parkinson’s disease (38), Alzheimer’s disease (39), and liver-related diseases in humans. However, studies have only been performed in mouse models, and there are few studies on the potential applications of UA in farm animals. This review aims to discuss the biological activities of UA and investigate the feasibility of using UA in animal husbandry.
2. Beneficial effects of UA
2.1. Anti-inflammatory effects
The close association between inflammation and many diseases, such as Parkinson’s disease, osteoarthritis, cardiovascular events, diabetic nephropathy, cancer, and influenza infection, is well known (40). Previous in vivo and in vitro studies have shown that UA acts against both endogenous and exogenous inflammatory stimuli, with favorable anti-inflammatory effects. UA (25 mg/kg body weight, oral) can prevent the degeneration of dopaminergic neurons in 1-methyl-4-phenyl-1,2,3,6-tetrahydropyridine-induced Parkinsonian mice (41). UA has been shown to significantly suppress xylene-induced ear edema in vivo as well as protect against lipopolysaccharide (LPS)-induced acute kidney injury by blocking the Toll-like receptor/myeloid differentiation primary response 88 pathway in vitro (42). In addition, UA can alleviate osteoarthritis by inhibiting the nuclear factor kappa B/NOD-like receptor protein-3 (NF-κB/NLRP3) inflammasome pathway (43). Furthermore, UA, at concentrations of 160 and 320 μg/mL, could inhibit inflammatory responses via the phosphatidylinositol 3-kinase/protein kinase B (PI3K/Akt) and NF-κB signaling pathways, reducing the viability of breast cancer cells (44). Treatment with 50 mg/kg UA in combination with caprylic acid (60 mg/kg) was found to decrease the levels of inflammatory cytokines such as tumor necrosis factor-alpha (TNF-α), interleukin-1-beta (IL-1β), to reverse pentylenetetrazole-induced seizure-like symptoms in a zebrafish model (45).
When used at a concentration of 20 μg/mL, UA, in an influenza A virus (IAV)-treated A549 cell model, resolved both cell injury and the inflammatory response (46). Zhou and Wink (47) also revealed that UA can play an anti-inflammatory role by inhibiting NF-κB nuclear translocation, thereby reducing the expression of inflammation-related factors, such as TNF-α, cyclooxygenase-2, and inducible nitric oxide synthase (iNOS). UA derivatives have also been found to be effective in treating inflammation. In a two-stage skin carcinogenesis mouse model, two weeks of treatment with UA (2 μmol) or its synthetic derivatives significantly inhibited the expression of pro-inflammatory genes, such as interleukin-1-alpha (IL-1α), IL-1β, IL-6, and IL-23. Moreover, a few UA derivatives, such as corosolic acid and 3-epi-corosolic acid, were found to have stronger anti-inflammatory activities than UA (48). Similarly, another UA derivative, β-D-glucopyranosyl ester, was also found to have anti-inflammatory activity (49). Overall, previous studies have established that UA and its derivatives regulate the development of inflammation, and hence may be useful for treating inflammation-related diseases.
2.2. Antioxidant activity
Oxidative stress results from excessive production of reactive oxygen species (ROS) in cells and tissues, which further leads to various inflammation-associated diseases (50). The in vitro antioxidant activity of UA was evaluated by inhibiting 2,2-diphenyl-1-picryl-hydroxyl at an inhibitory concentration (IC50) of 59.7 ± 1.0 μg/mL (51). Administration of UA (25 and 50 mg/kg of body weight/day, intragastrically) for 6 weeks was found to reduce CCl4-induced nephrotoxicity, demonstrating the antioxidant activity of UA and its ability to inhibit the phosphorylation of transcription 3 (STAT3)-NF-κB pathway (52). In human lymphocytes and the hamster V79 lung fibroblast cell line, UA was demonstrated to be a natural antioxidant that prevents DNA damage caused by hydrogen peroxide, causing a 50% decrease in cell viability at 224.85 mM (53). Furthermore, UA has been suggested as a potential candidate for the treatment and prevention of oxidative stress-mediated diseases (54), including neurodegenerative diseases in mouse models (55), obesity/diabetes and cardiovascular diseases in mice, skin carcinogenesis in mouse epidermal cells (56), liver disease in mice with carbon tetrachloride (CCl4)-induced liver fibrosis (57), and osteoporosis in MG-63 cells (58).
UA plays a vital role in the injury caused by cerebral ischemia in mice by activating the nuclear factor-erythroid 2-related factor 2 (Nrf2) pathway (59). Nrf2, encoded by the gene NFE2L2, is a regulatory factor of phase II antioxidant enzymes, protecting the body from oxidative stress and inflammation. Nrf2(−/−) mice showed obvious symptoms of neurodegeneration and oxidative stress, which were significantly reduced by intraperitoneal injection of UA (100 mg/kg), demonstrating the neuroprotective effects of UA (55). UA (50 mg/kg/day, 6 weeks, oral gavage) was also shown to attenuate CCl4-induced hepatic oxidative damage and inflammation by increasing the expression levels of NAD(P)H:quinone-oxidoreductase-1, glutathione S-transferases, heme oxygenase-1, B-cell lymphoma-2 (Bcl-2), and nuclear Nrf2 (57). In a Caenorhabditis elegans model used for the evaluation of neurological drugs, UA (100 μM) showed antioxidant activity by upregulating the expression of peroxiredoxin-2 and skn-1 transcription factor (which corresponds to human Nrf2); this also led to an anti-depressant effect (60). Dietary administration of 0.1% UA (for 8 weeks) attenuated the tumor growth of transplanted VCaP (human prostatic cancer cell) xenografts in immunodeficient mice, together with epigenetic CpG methylation reprogramming, suggesting potential applications for the treatment/prevention of human prostate cancer (61). Treatment of JB6 P+ mouse epidermal cells with 2.5 μM UA increased Nrf2 expression by altering the methylation status of the Nrf2 promoter, thus inhibiting the development of skin cancer (56). In cisplatin-resistant HepG2/DDP cells, UA, as a natural adjuvant, increased the sensitivity of hepatocellular carcinoma cells to cisplatin through the Nrf2/antioxidant reaction element (ARE) signaling pathway, thus exhibiting anticancer effects (62). In conclusion, the results of various studies indicate that Nrf2 may be the target of the biological activity of UA.
2.3. Anticancer activity
In terms of its anticancer activity, UA is mainly associated with apoptosis and death of cancer cells. Mitochondria are essential for cell respiration and oxidative phosphorylation, and mitochondrial damage leads to apoptosis (63). UA exerts its anticancer effect by activating mitochondrial-dependent signaling pathways (Figure 2).
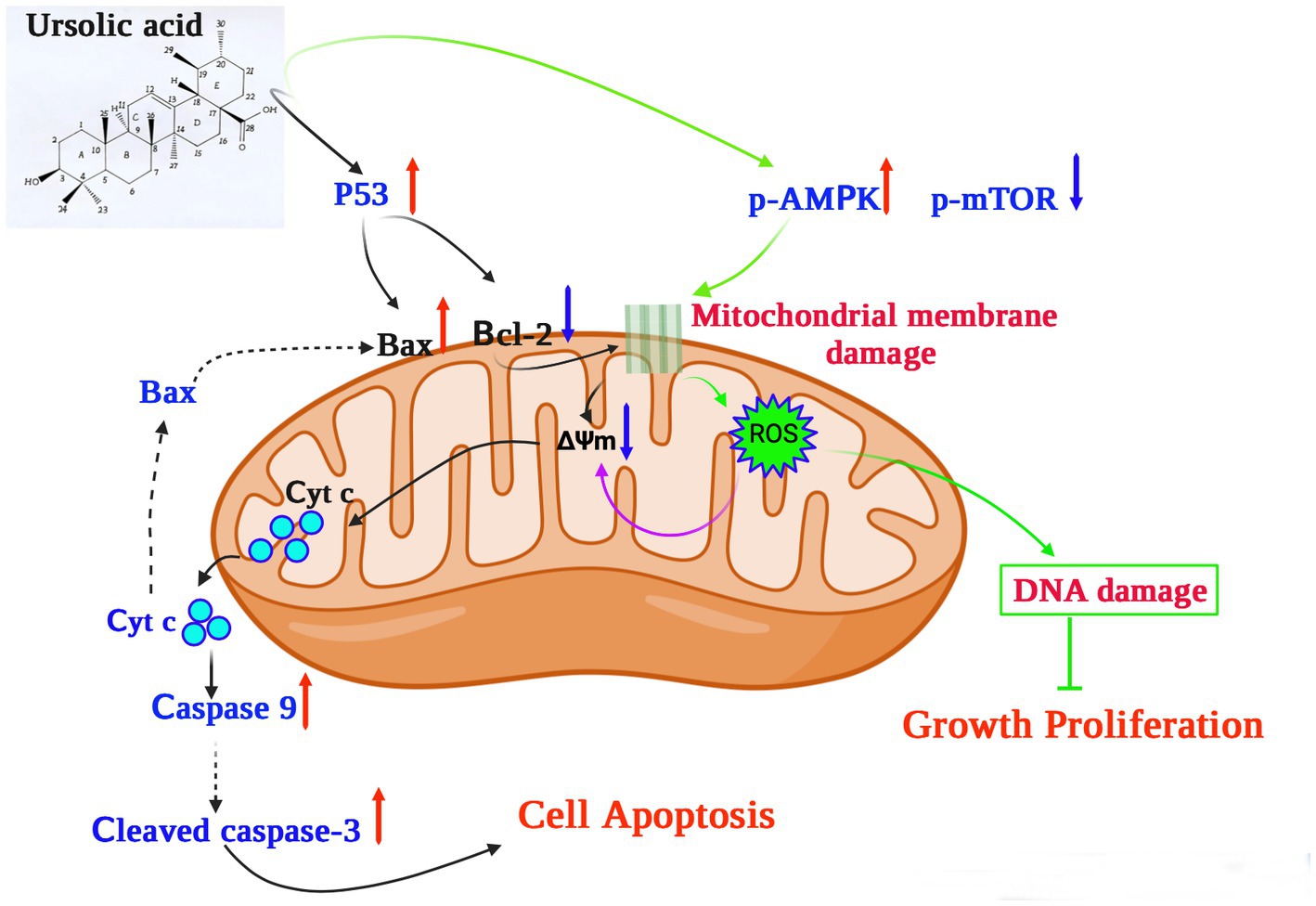
Figure 2. The potential mechanism underlying the anticancer effects of ursolic acid (64–68). UA upregulates and activates the expression of the cell tumor antigen p53 and the AMP-activated protein kinase (AMPK) signaling pathway, respectively, together with suppression of the mammalian target of rapamycin (mTOR) pathways and downregulation of the apoptosis regulator Bcl-2. UA damages the mitochondrial membrane, decreasing the mitochondrial membrane potential ΔΨm, which induces cytochrome c release from the mitochondria to the cytosol, activating the caspase proteins and leading to apoptosis. In addition, UA treatment significantly increases the production of mitochondrial ROS, reducing both DNA synthesis and cell proliferation. UA, ursolic acid; p-AMPK, phosphorylated AMP-activated protein kinase; mTOR, mammalian target of rapamycin; Bcl-2, B-cell lymphoma 2; ROS, reactive oxygen species; Cyt C, Cytochrome C; ∆Ψm, mitochondrial membrane potential.
For example, UA upregulates and activates the expression of the cell tumor antigen p53 and the AMP-activated protein kinase (AMPK) signaling pathway, respectively, and downregulates the expression of the apoptosis regulator Bcl-2 (64). In a previous study, UA was shown to induce apoptosis of human breast adenocarcinoma (MCF7), osteosarcoma (U2OS), cervical adenocarcinoma (HeLa), and colorectal cancer (HCT116) cells after 24 h of treatment, with lethal dose 50 (LD50) values of 12.8 μM, 7.7 μM, 16.8 μM, and 19 μM, respectively (69). Furthermore, UA (20 μM) could inhibit the expression of AKT mRNA, thereby activating the AMPK signaling pathway to promote autophagy and apoptosis (65). Furthermore, in the presence of 30 μM UA for 48 h, the proliferative activity of human lung cancer cells (A549 and H460) decreased significantly, whereas the expression of caspases 3 and 9 increased, further increasing the levels of the apoptosis-related protein Bax and decreasing those of the anti-apoptotic protein Bcl-2 (66). These results suggest that UA can induce apoptosis through caspase-related signaling pathways. Through the action of UA, AMPK is activated and the mammalian target of the rapamycin (mTOR) signaling pathway is suppressed, thus controlling protein synthesis and cell growth (66). In addition, structurally modified UA may also activate non-apoptotic cell death pathways associated with autophagosome and lysosome accumulation (67). UA also showed therapeutic effects on drug-resistant cancer cell lines. In vitro experiments confirmed that 48 h of UA treatment (16 μM) could reduce the adhesion and infiltration of the human breast cancer adriamycin-resistant cell line MCF-7/ADR to human umbilical vein endothelial cells (HUVECs) and could also reduce migration in MCF-7/ADR cells (68). UA induced cellular DNA damage and initiated G0/G1 phase arrest in embryonic cancer cells, and could be a candidate for inhibiting the recurrence of cancer (70).
2.4. Hepato-protective activity
When C57BL/6 mice were fed a high-fat diet for 15 weeks, accompanied by oral administration of UA, 80 mg/kg of UA could significantly reduce the total cholesterol (TC) and triglyceride (TG) levels in the liver and plasma, effectively relieve liver steatosis and reduce the number of epididymal fat cells. Meanwhile, in vitro experiments also confirmed that UA (20 μM) significantly reduced TC (37.2%) and TG (50.4%) contents in HepG2 cells and upregulated P-AMPK protein expression (71). Therefore, UA may reduce the lipid content of cells and inhibit lipid synthesis by activating the AMPK signaling pathway. UA improves the richness of beneficial intestinal flora through inhibition of the NOX4/NLRP3 inflammasome pathway, thus alleviating liver fibrosis caused by CCl4 (72). UA can also reduce the activation of mitogen-activated protein kinases (JNK, p38 MAPK, ERK) and inactivate the immunoregulatory transcription factor NF-κB in the liver after treatment with CCl4, thereby relieving CCl4-induced inflammation (73).
In vitro experiments have also shown that UA can reverse the progression of liver fibrosis by inhibiting the activation of the NADPH oxidase (NOX)/ROS signaling pathway in hepatic stellate cells (74). UA protects the intestinal barrier by inhibiting the inflammatory factor TNF-α and increasing the expression of tight junction proteins and antimicrobial peptides. A 16S rRNA gene sequencing study also confirmed that UA treatment increased the abundance of beneficial bacteria, such as Firmicutes, Lactobacillus, and Bifidobacterium, thus alleviating the liver fibrosis process (75). Speculatively, the protective effect of UA on the liver may be related to reducing inflammation and oxidative stress levels or regulating intestinal flora. The possible mechanism underlying the role of UA in hepatoprotection against liver fibrosis, inhibiting the proliferation of cancer cells, and reversing nonalcoholic fatty liver disease, thus promoting liver regeneration by different pathways, is shown in Figure 3.
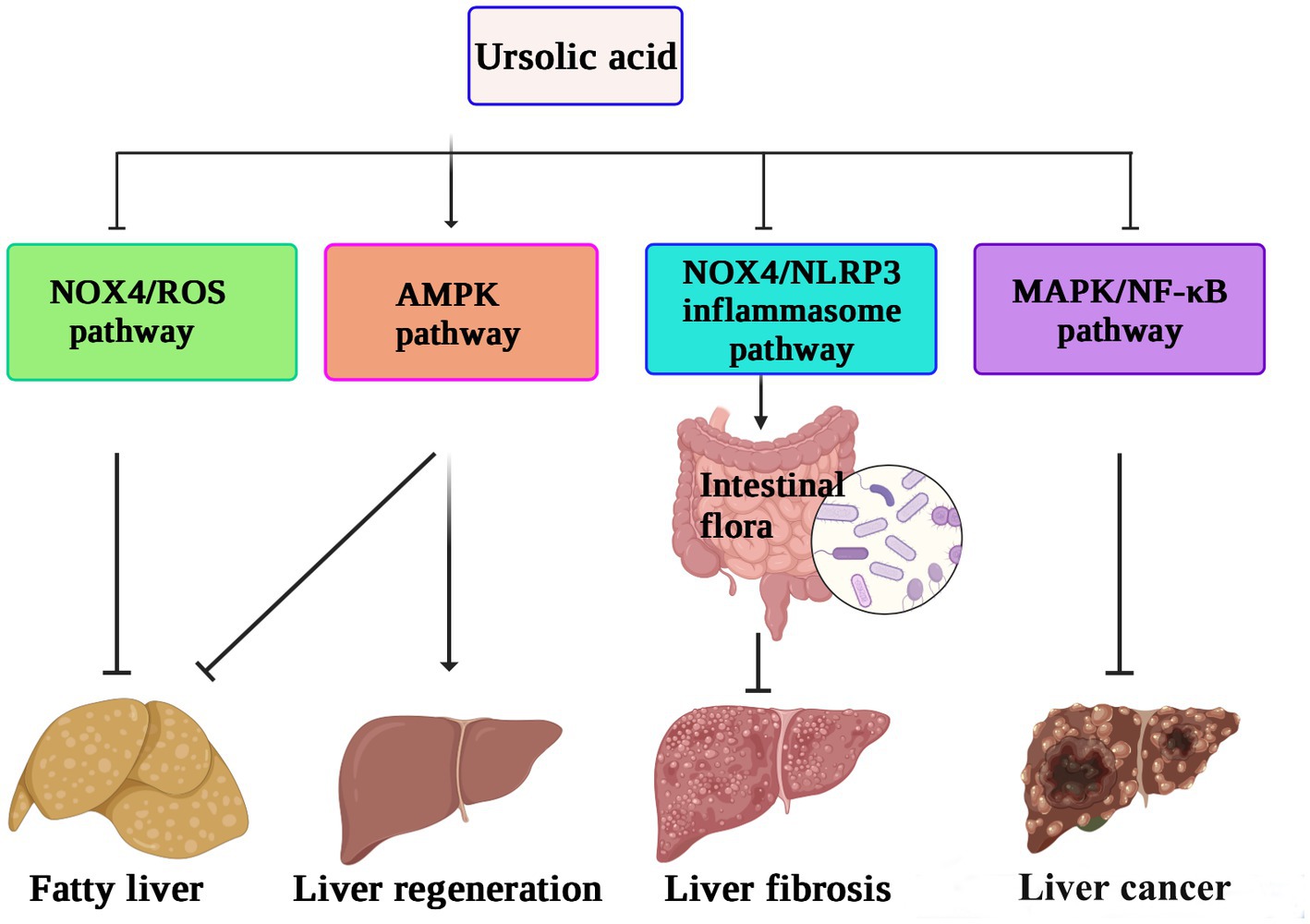
Figure 3. The hepatoprotective mechanisms of ursolic acid (71–75). UA suppresses the NOX4/ROS pathway to relieve nonalcoholic fatty liver disease, activates the AMPK pathway to enhance liver regeneration, and inhibits the MAPK/NF-κB pathway to decrease the proliferation of cancer cells. In addition, the protective effect of UA against liver fibrosis may be related to reducing inflammation and oxidative stress levels or regulating intestinal flora by downregulation of the NOX4/NLRP3 pathway. UA, ursolic acid; AMPK, AMP-activated protein kinase; NOX4, NADPH oxidase 4; MAPK, mitogen-activated protein kinase. NLRP3, NOD-like receptor thermal protein domain associated protein 3; ROS, reactive oxygen species; NF-κB, nuclear factor kappa-B.
2.5. Antibacterial activity
Antibiotic resistance and the emergence of superbugs have become serious public health problems in both human and veterinary medicine. To treat infections caused by multi-drug resistant pathogens, many different strategies have been used, including phages (76), antimicrobial peptides (77), metal nanoparticles (78), and plant-derived compounds--polyphenols (79), flavonoids (80), terpenoids (81), plant essential oils (82), and alkaloids (83). Traditional Chinese Medicine and Ayurveda have also been used in health care and disease prevention in ancient China and Egypt (84). UA, as a representative triterpenoid, showed significant antibacterial activity against the production of biofilm by Escherichia coli CFT073 (ATCC 700928), Enterobacter cloacae ATCC-BAA 2468, and Pseudomonas aeruginosa ATCC 25000 (81).
Although the antibacterial effects of plant extracts may not be as obvious as those of antibiotics, plant extracts can have synergistic effects when combined with antibiotics. UA showed a strong synergistic effect when combined with β-lactam antibiotics (ampicillin and benzacillin), increasing the sensitivity of Staphylococcus aureus and Staphylococcus epidermidis to antibiotics (85). UA (32 μg/mL) also displayed synergy with colistin against clinical isolates of Klebsiella pneumoniae BC936 and E. coli U3790 (86). Table 3 shows the MIC values of UA against different Gram-positive and Gram-negative pathogens. The MIC value of UA or its oleanolic acid isomer was 31.25 μg/mL against both S. aureus ATCC 6538 and methicillin-resistant S. aureus, whereas the MIC against Listeria monocytogenes ATCC 19115 was 250 μg/mL (91). UA is also known to show antifungal activity against Cryptococcus neoformans H99 with a MIC value of 250 μg/mL (92).
UA exposure led to a decrease in intracellular pH and ATP, downregulated the expression of four biofilm-related genes (pgaA, luxS, wbbM, and wzm), and inhibited biofilm formation of carbapenem-resistant Klebsiella pneumoniae (90). The antimicrobial activity of UA is stronger than that of tetracycline, with reductions of 50.5 and 12.7%, respectively, in staphylococcal membrane integrity (87). The MIC value of UA against E. coli (ATCC 25922), K. pneumoniae (ATCC10031), and Shigella flexella (ATCC12022) was 64 μg/mL. However, the MIC of UA against these bacteria was significantly reduced by modification of C-3 (hydroxyl) in UA to form UA ester analogs. Hence, the modified UA derivatives showed markedly increased antibacterial effects (51). Gram-negative bacteria contain an outer membrane, which is rich in LPS and phospholipids and prevents entry of UA into the bacterial cells, and hence the MICs of UA against Gram-negative bacteria are higher than that against Gram-positive bacteria (81).
UA kills bacteria by altering the structure of the bacterial cell, particularly by interfering with the cell membrane and adhesion proteins (93). UA also affects cell morphology and controls the expression of genes related to virulence factors, such as pili and fritillary (94). UA inhibits bacterial growth by reducing the ability of bacteria to adhere to host cells, as well as disrupting biofilm formation (95). In the presence of 10 mg/L UA from Diospyros dendo leaves/flowers, decreases of 72, 87, and 57% were observed in the biofilm biomass of Escherichia coli (ATCC 25404), Pseudomonas aeruginosa, and Vibrio havii, respectively, after culture for 24 h. Furthermore, 10 and 30 mg/L of UA induced the expression of genes related to chemotaxis (cheA, motAB, tap, and tsr). Overexpression of the motAB genes has been shown to cause increased bacterial activity, decreasing their stability in the biofilm environment and resulting in reduced biofilm formation (96). Experiments, such as measurement of bacterial glucosyltransferase activity, computer simulation, site-directed mutagenesis, and capillary electrophoresis, have revealed that UA competes for occupancy of the active site of glycosyltransferase, a key enzyme required for the synthesis of extracellular polymeric substances (EPS), secreted by S. mutans, thus inhibiting EPS formation and reducing the viability of S. mutans and the structural integrity of its biofilm (97). Therefore, in vitro results support the use of UA for the treatment of bacterial diseases. However, UA is rarely used to prevent or treat bacterial diseases in animal husbandry.
3. The side effects of UA
It has been reported that UA (5 mg/kg body weight, intraperitoneally) could inhibit the spermatogenesis in Wistar strain male albino rats (3 months old) (98). 50 μM UA purified from loquat (Eriobotrya japonica) also exerts the cytotoxicities against A549 (lung cancer cell line) and NTUB1 cells (human bladder cancer cell line) (99). In the acute and toxicity test, UA (21.5 g/kg body weight, oral) extracted from Ledum pulastre L. can destroy the nervous and digestive systems of mice, and the LD50 of the UA was 9.26 g/ kg (100).
4. Application of UA in livestock, poultry, and aquatic animals
At present, the application of UA in animal husbandry is limited. UA is mainly used to improve antioxidant capacity in broilers (101) and as a cryopreservative for porcine semen (102). UA is also used to treat bacterial pneumonia in calves (103) and improve intestinal immunity or treat viral diseases in fish, indicating potential applications in aquaculture (104).
Although the protective effect of UA against asthenozoospermia in rats by increasing sperm density and motility has already been established (105), its use as a cryopreservative for porcine semen has also been documented. As UA is a natural antioxidant, its addition (at a concentration of 1.6 mg/mL) to the frozen diluent of porcine sperm resulted in a significant improvement in the integrity of the mitochondrial membranes, plasma membranes, and acrosomes of the sperm (102). Supplementation of UA (400 mg/kg, purified from pine bark, and Rosmarinus officinalis L., Aurantii fructus immaturus, and Eucommla ulmoides leaves) for 42 days resulted in a significant reduction in elimination and mortality rates of the sperm, while the malondialdehyde content was also decreased due to an increase in the activity of superoxide dismutase (101). UA or UA derivatives have also been used as anti-microbial and anti-influenza drugs (106–109), and feed additives for the improvement of intestinal health and immunity in livestock and poultry (110–112).
In addition, using in vitro studies, UA has been demonstrated as a promising candidate for the treatment of bovine endometritis (113). Furthermore, it was found that when UA was used at a concentration of 20 μM, it provided protection in an LPS-induced model of endometrial cell inflammation. Notably, reductions in the expression of several pro-inflammatory cytokines, such as IL-6, TNF-α, and IL-1β, and, particularly, the NF-κB signaling pathway, were observed (113). Treatment with UA can result in the suppression of IL-17A expression in the lungs of newborn calves, and UA has also been demonstrated to have some therapeutic effect on bovine bacterial pneumonia caused by Mannheimia haemolytica (103). UA treatment (at a concentration of 1 ppm) was also found to be significantly useful in relieving renal malformations (atrophic glomeruli and curvature defects of the anterior renal ducts) caused by aristolochia acid in zebrafish embryos (114). A previous study demonstrated the useful therapeutic effects of sage and lemon verbena, which are also rich in triterpenoids. These molecules can increase the number of intestinal goblet cells, change the glycosylation properties of mucin agglutinin, and enhance intestinal immunity in juvenile gilthead seabream (Sparus aurata) (115).
Although the water insolubility of UA limits its clinical application, structurally modified UA shows antiviral activity. UA derivatives (esterification of the C-17 carboxyl or conversion of the carboxyl into amide) also exhibited a strong anti-H5N1 activity (116). Furthermore, 3-o-β-UA as well as UA’s ester equivalents showed antiviral activity against porcine reproductive and respiratory syndrome viruses in vitro (117). UA is one of the main components of Prunella vulgaris L., and hence showed antiviral activity against hematopoietic necrosis virus (IHNV) in rainbow trout (Oncorhynchus mykiss). When UA was injected intraperitoneally, a reduction in the expression levels of IHNV glycoprotein mRNA (in the spleen) was observed on day 1 after viral infection (118). In addition, 10% UA obtained from Lippia citriodora and Salvia officinalis could be used as an effective additive to improve the growth performance and feed conversion ratio and provide immune protection after LPS treatment in juvenile gilthead seabream (Sparus aurata) (119). UA could also effectively inhibit Micropterus salmoides rhabdovirus (MSRV) replication (IC50 = 5.55 μM) in vitro and increase the mortality rate by 12.5% in MSRV-infected largemouth bass (104). This indicates that UA can be considered as an alternative anti-MSRV agent in aquaculture. An extract of O. sanctum containing UA was found to have potential anti-influenza activity (120), and the juice from fresh O. sanctum leaves exhibited antibacterial activity due to the inhibition of the expression of extended-spectrum β-lactamase (ESBL) enzymes produced by E. coli (121). Based on these results, it can be predicted that UA has the potential to be used as an antiviral drug or antibiotic substitute in animal husbandry.
5. Insights and future directions
UA has a typical triterpenoid structure and thus has specific pharmacological characteristics. UA is found in a variety of fruits, spices, and medicinal herbs, such as Lavandula stoechas, apple peel, rosemary, and ligustrine. Numerous investigations have demonstrated that UA has a variety of biological activities, including anti-inflammatory, antioxidant, anti-cancer, and hepatoprotective effects, using both in vitro and in vivo animal model experiments (Figure 4). UA is mainly used in animal husbandry to improve intestinal immunity and for its anti-inflammatory and antiviral activities. Based on its bacteriostatic effects, UA has the potential to be used as a therapeutic agent in farmed species to reduce the use of chemotherapeutics and enhance the move toward sustainability.
However, although ultra-pure and high-dose UA has specific beneficial effects, the economic cost of UA production from plants requires consideration for its widespread use in livestock and poultry production. Furthermore, the toxicology and pharmacokinetics (bioavailability) of UA require further investigation.
Author contributions
GL, PQ, and XC wrote sections of the manuscript. LW and RW made the figures. WG provided the topic of this article. All authors contributed to the article and approved the submitted version.
Funding
This work was supported by the Natural Science Foundation of Hebei Province (NO. C2022402048) and National Natural Science Foundation of China (NO. 31802150).
Acknowledgments
We thank the Hebei Plant Extraction Innovation Center Co., Ltd. for support of this work as well as the support provided through our funding agencies.
Conflict of interest
PQ and XC were employed by Chenguang Biotechnology Group Handan Co., Ltd. LW and WG were employed by Hebei Plant Extraction Innovation Center Co., Ltd.
The remaining authors declare that the research was conducted in the absence of any commercial or financial relationships that could be construed as a potential conflict of interest.
Publisher’s note
All claims expressed in this article are solely those of the authors and do not necessarily represent those of their affiliated organizations, or those of the publisher, the editors and the reviewers. Any product that may be evaluated in this article, or claim that may be made by its manufacturer, is not guaranteed or endorsed by the publisher.
References
1. Cheng, Y, Xue, F, Yu, S, Du, S, and Yang, Y. Subcritical water extraction of natural products. Molecules. (2021) 26:4004. doi: 10.3390/molecules26134004
2. Hu, JF, Garo, E, Goering, MG, Pasmore, M, Yoo, HD, Esser, T, et al. Bacterial biofilm inhibitors from Diospyros dendo. J Nat Prod. (2006) 69:118–20. doi: 10.1021/np049600s
3. Mirza, FJ, Amber, S, Sumera, HD, Ahmed, T, and Zahid, S. Rosmarinic acid and ursolic acid alleviate deficits in cognition, synaptic regulation and adult hippocampal neurogenesis in an Aβ1-42-induced mouse model of Alzheimer's disease. Phytomedicine. (2021) 83:153490. doi: 10.1016/j.phymed.2021.153490
4. Cao, S, Wastney, ME, Lachcik, PJ, Xiao, HH, Weaver, CM, and Wong, MS. Both oleanolic acid and a mixture of oleanolic and ursolic acids mimic the effects of fructus ligustri lucidi on bone properties and circulating 1,25-dihydroxycholecalciferol in ovariectomized rats. J Nutr. (2018) 148:1895–902. doi: 10.1093/jn/nxy242
5. Wu, CR, Hseu, YC, Lien, JC, Lin, LW, Lin, YT, and Ching, H. Triterpenoid contents and anti-inflammatory properties of the methanol extracts of ligustrum species leaves. Molecules. (2010) 16:1–15. doi: 10.3390/molecules16010001
6. Ghiulai, R, Avram, S, Stoian, D, Pavel, IZ, Coricovac, D, Oprean, C, et al. Lemon balm extracts prevent breast cancer progression in vitro and in ovo on chorioallantoic membrane assay. Evid Based Complement Alternat Med. (2020) 2020:6489159. doi: 10.1155/2020/6489159
7. Yamaguchi, H, Noshita, T, Kidachi, Y, Umetsu, H, Hayashi, M, Komiyama, K, et al. Isolation of ursolic acid from apple peels and its specific efficacy as a potent antitumor agent. J Health Sci. (2008) 54:654–60. doi: 10.1248/jhs.54.654
8. Figueroa-Suárez, MZ, González Christen, J, Cardoso-Taketa, AT, Gutiérrez Villafuerte, MDC, and Rodríguez-López, V. Anti-inflammatory and antihistaminic activity of triterpenoids isolated from Bursera cuneata (Schldl.) Engl. J Ethnopharmacol. (2019) 238:111786. doi: 10.1016/j.jep.2019.03.013
9. Hyun, MK, Kim, DH, Park, CH, Noh, SG, Choi, S, Lee, JY, et al. Protective mechanisms of loquat leaf extract and ursolic acid against diabetic pro-inflammation. J Mol Med. (2022) 100:1455–64. doi: 10.1007/s00109-022-02243-x
10. Tshilanda, DD, Onyamboko, DN, Babady-Bila, P, Ngbolua, KT, Tshibangu, DS, Dia Fita Dibwe, E, et al. Anti-sickling activity of ursolic acid isolated from the leaves of ocimum gratissimum L. (Lamiaceae). Nat Prod Bioprospect. (2015) 5:215–21. doi: 10.1007/s13659-015-0070-6
11. Rezaei-Golmisheh, A, Malekinejad, H, Asri-Rezaei, S, Farshid, AA, and Akbari, P. Hawthorn ethanolic extracts with triterpenoids and flavonoids exert hepatoprotective effects and suppress the hypercholesterolemia-induced oxidative stress in rats. Iran J Basic Med Sci. (2015) 18:691–9.
12. Ladda, PL, and Magdum, CS. Antitubercular activity and isolation of chemical constituents from plant Vitex negundo Linn. Iran J Pharm Res. (2018) 17:1353–60.
13. Alves Monteath, SAF, Maciel, MAM, Vega, RG, de Mello, H, de Araújo, MC, Esteves-Souza, A, et al. Ultrasound-assisted extraction of ursolic acid from the flowers of Ixora coccinia Linn (Rubiaceae) and antiproliferative activity of ursolic acid and synthesized derivatives. Pharmacogn Mag. (2017) 13:265–9. doi: 10.4103/0973-1296.204557
14. Acín, S, Muñoz, DL, Guillen, A, Soscue, D, Castaño, A, Echeverri, F, et al. Triterpene-enriched fractions from Eucalyptus tereticornis ameliorate metabolic alterations in a mouse model of diet-induced obesity. J Ethnopharmacol. (2021) 265:113298. doi: 10.1016/j.jep.2020.113298
15. López-Hortas, L, Pérez-Larrán, P, González-Muñoz, MJ, Falqué, E, and Domínguez, H. Recent developments on the extraction and application of ursolic acid. A review. Food Res Int. (2018) 103:130–49. doi: 10.1016/j.foodres.2017.10.028
16. Razborsek, MI, Voncina, DB, Dolecek, V, and Voncina, E. Determination of oleanolic, betulinic and ursolic acid in Lamiaceae and mass spectral fragmentation of their trimethylsilylated derivatives. Chromatographia. (2008) 67:433–40. doi: 10.1365/s10337-008-0533-6
17. Jäger, S, Trojan, H, Kopp, T, Laszczyk, MN, and Scheffler, A. Pentacyclic triterpene distribution in various plants – rich sources for a new group of multi-potent plant extracts. Molecules. (2009) 14:2016–31. doi: 10.3390/molecules14062016
18. Kowalski, R. Studies of selected plant raw materials as alternative sources of triterpenes of oleanolic and ursolic acid types. J Agric Food Chem. (2007) 55:656–62. doi: 10.1021/jf0625858
19. Wang, C, Wang, X, Zhao, S, Sun, W, and Tong, S. Preparative separation of structural isomeric pentacyclic triterpene oleanolic acid and ursolic acid from natural products by pH-zone-refining countercurrent chromatography. RSC Adv. (2019) 9:38860–6. doi: 10.1039/C9RA06082K
20. Aydin, T, Saglamtas, R, Gumustas, M, Genisel, M, Kazaz, C, and Cakir, A. Lavandula stoechas L. subsp. stoechas, a new herbal source for ursolic acid: quantitative analysis, purification and bioactivity studies. Chem Biodivers. (2023) 20:e202300414. doi: 10.1002/cbdv.202300414
21. Palu, D, Bighelli, A, Casanova, J, and Paoli, M. Identification and quantitation of ursolic and oleanolic acids in Ilex aquifolium L. leaf extracts using 13C and 1H-NMR spectroscopy. Molecules. (2019) 24:4413. doi: 10.3390/molecules24234413
22. Zheng, X, Wang, H, Zhang, P, Gao, L, Yan, N, Li, P, et al. Chemical composition, antioxidant activity and α-glucosidase inhibitory activity of chaenomeles speciosa from four production areas in China. Molecules. (2018) 23:2518. doi: 10.3390/molecules23102518
23. Kamatchi, PAC, Maheswaran, R, Sivanandhan, S, Ignacimuthu, S, Balakrishna, K, Reegan, AD, et al. Bioefficacy of ursolic acid and its derivatives isolated from Catharanthus roseus (L) G. Don leaf against Aedes aegypti, Culex quinquefasciatus, and Anopheles stephensi larvae. Environ Sci Pollut Res. (2023) 30:69321–9. doi: 10.1007/s11356-023-27253-1
24. George, J, Meshram, GA, and Patil, VR. Sono-maceration – a rapid and inexpensive method for the isolation of ursolic acid from Neolamarckia cadamba leaves. Nat Prod Res. (2023) 37:494–7. doi: 10.1080/14786419.2021.1971978
25. Bernatoniene, J, Cizauskaite, U, Ivanauskas, L, Jakstas, V, Kalveniene, Z, and Kopustinskiene, DM. Novel approaches to optimize extraction processes of ursolic, oleanolic and rosmarinic acids from Rosmarinus officinalis leaves. Ind Crop Prod. (2016) 84:72–9. doi: 10.1016/j.indcrop.2016.01.031
26. Amidon, GL, Lennernäs, H, Shah, VP, and Crison, JR. A theoretical basis for a biopharmaceutic drug classification: the correlation of in vitro drug product dissolution and in vivo bioavailability. Pharm Res. (1995) 12:413–20. doi: 10.1023/A:1016212804288
27. Lőrincz, A, Mihály, J, Wacha, A, Németh, C, Besztercei, B, Gyulavári, P, et al. Combination of multifunctional ursolic acid with kinase inhibitors for anti-cancer drug carrier vesicles. Mater Sci Eng C Mater Biol Appl. (2021) 131:112481. doi: 10.1016/j.msec.2021.112481
28. Carneiro, SB, Costa Duarte, FÍ, and Heimfarth, L. Cyclodextrin–drug inclusion complexes: in vivo and in vitro approaches. Int J Mol Sci. (2019) 20:642. doi: 10.3390/ijms20030642
29. Luan, T, Jin, C, Jin, CM, Wang, Z, Guo, H, and Zheng, F. Synthesis and biological evaluation of ursolic acid derivatives bearing triazole moieties as potential anti-toxoplasma gondii agents. J Enzyme Inhib Med Chem. (2019) 34:761–72. doi: 10.1080/14756366.2019.1584622
30. Ghasemzadeh, F, Najafpour, GD, and Mohammadi, M. Antiinfective properties of ursolic acid-loaded chitosan nanoparticles against Staphylococcus aureus. Turk J Chem. (2021) 45:1454–62. doi: 10.3906/kim-2104-13
31. Biswas, S, Kar, A, and Sharma, N. Synergistic effect of ursolic acid and piperine in CCl4 induced hepatotoxicity. Ann Med. (2021) 53:2009–17. doi: 10.1080/07853890.2021.1995625
32. Yin, R, Li, T, Tian, JX, Xi, P, and Liu, RH. Ursolic acid, a potential anticancer compound for breast cancer therapy. Crit Rev Food Sci Nutr. (2018) 58:568–74. doi: 10.1080/10408398.2016.1203755
33. Chan, EWC, Soon, CY, Tan, JBL, Wong, SK, and Hui, YW. Ursolic acid: an overview on its cytotoxic activities against breast and colorectal cancer cells. J Integr Med. (2019) 17:155–60. doi: 10.1016/j.joim.2019.03.003
34. Chen, Z, Liu, Q, Zhu, Z, Xiang, F, Zhang, M, Wu, R, et al. Ursolic acid protects against proliferation and inflammatory response in LPS-treated gastric tumour model and cells by inhibiting NLRP3 inflammasome activation. Cancer Manag Res. (2020) 12:8413–24. doi: 10.2147/CMAR.S264070
35. Li, H, Yu, Y, Liu, Y, Luo, Z, Law, BYK, Zheng, Y, et al. Ursolic acid enhances the antitumor effects of sorafenib associated with Mcl-1-related apoptosis and SLC7A11-dependent ferroptosis in human cancer. Pharmacol Res. (2022) 182:106306. doi: 10.1016/j.phrs.2022.106306
36. Kashyap, D, Sharma, A, Tuli, HS, Punia, S, and Sharma, AK. Ursolic acid and oleanolic acid: pentacyclic terpenoids with promising anti-inflammatory activities. Recent Patents Inflamm Allergy Drug Discov. (2016) 10:21–33. doi: 10.2174/1872213X10666160711143904
37. Alam, M, Ali, S, Ahmed, S, Elasbali, AM, Adnan, M, Islam, A, et al. Therapeutic potential of ursolic acid in cancer and diabetic neuropathy diseases. Int J Mol Sci. (2021) 2:12162. doi: 10.3390/ijms222212162
38. Bang, Y, Kwon, Y, Kim, M, Moon, SH, Jung, K, and Choi, HJ. Ursolic acid enhances autophagic clearance and ameliorates motor and non-motor symptoms in Parkinson's disease mice model. Acta Pharmacol Sin. (2023) 44:752–65. doi: 10.1038/s41401-022-00988-2
39. Mirza, FJ, and Zahid, S. Ursolic acid and rosmarinic acid ameliorate alterations in hippocampal neurogenesis and social memory induced by amyloid beta in mouse model of Alzheimer's disease. Front Pharmacol. (2022) 13:1058358. doi: 10.3389/fphar.2022.1058358
40. Guo, H, Callaway, JB, and Ting, JP. Inflammasomes: mechanism of action, role in disease, and therapeutics. Nat Med. (2015) 21:677–87. doi: 10.1038/nm.3893
41. Rai, SN, Zahra, W, Singh, SS, Birla, H, Keswani, C, Dilnashin, H, et al. Anti-inflammatory activity of ursolic acid in MPTP-induced parkinsonian mouse model. Neurotox Res. (2019) 36:452–62. doi: 10.1007/s12640-019-00038-6
42. Zhao, J, Zheng, H, Sui, Z, Jing, F, Quan, X, Zhao, W, et al. Ursolic acid exhibits anti-inflammatory effects through blocking TLR4-MyD88 pathway mediated by autophagy. Cytokine. (2019) 123:154726. doi: 10.1016/j.cyto.2019.05.013
43. Wang, C, Gao, Y, Zhang, Z, Chen, C, Chi, Q, Xu, K, et al. Ursolic acid protects chondrocytes, exhibits anti-inflammatory properties via regulation of the NF-κB/NLRP3 inflammasome pathway and ameliorates osteoarthritis. Biomed Pharmacother. (2020) 130:110568. doi: 10.1016/j.biopha.2020.110568
44. Luo, J, Hu, YL, and Wang, H. Ursolic acid inhibits breast cancer growth by inhibiting proliferation, inducing autophagy and apoptosis, and suppressing inflammatory responses via the PI3K/AKT and NF-κB signaling pathways in vitro. Exp Ther Med. (2017) 14:3623–31. doi: 10.3892/etm.2017.4965
45. Sharma, D, Kaur, S, Kapil, L, Singh, C, and Singh, A. Ursolic acid along with caprylic acid ameliorates pentylenetetrazole induced seizures like behavior in adult zebrafish. Res Sq. (2022):1–31. doi: 10.21203/rs.3.rs-1811314/v1
46. Wei, X, Lan, Y, Nong, Z, Li, C, Feng, Z, Mei, X, et al. Ursolic acid represses influenza a virus-triggered inflammation and oxidative stress in A549 cells by modulating the miR-34c-5p/TLR5 axis. Cytokine. (2022) 157:155947. doi: 10.1016/j.cyto.2022.155947
47. Zhou, JX, and Wink, M. Evidence for anti-inflammatory activity of isoliquiritigenin, 18β glycyrrhetinic acid, ursolic acid, and the traditional chinese medicine plants Glycyrrhiza glabra and Eriobotrya japonica, at the molecular level. Medicines (Basel). (2019) 6:55. doi: 10.3390/medicines6020055
48. Nelson, AT, Camelio, AM, Claussen, KR, Cho, J, Tremmel, L, DiGiovanni, J, et al. Synthesis of oxygenated oleanolic and ursolic acid derivatives with anti-inflammatory properties. Bioorg Med Chem Lett. (2015) 25:4342–36. doi: 10.1016/j.bmcl.2015.07.029
49. Du, C, Zhang, J, and Yu, B. (2014). Preparation of ursolic acid β-D-glucopyranosyl ester as anti-inflammatory agent. CN103739654.
50. Hussain, T, Tan, B, Yin, Y, Blachier, F, Tossou, MC, and Rahu, N. Oxidative stress and inflammation: what polyphenols can do for us? Oxid Med Cell Longev. (2016) 2016:7432797. doi: 10.1155/2016/7432797
51. Do Nascimento, PG, Lemos, TL, Bizerra, AM, Arriaga, ÂM, Ferreira, DA, Santiago, GM, et al. Antibacterial and antioxidant activities of ursolic acid and derivatives. Molecules. (2014) 19:1317–27. doi: 10.3390/molecules19011317
52. Ma, JQ, Ding, J, Xiao, ZH, and Liu, CM. Ursolic acid ameliorates carbon tetrachloride-induced oxidative DNA damage and inflammation in mouse kidney by inhibiting the STAT3 and NF-κB activities. Int Immunopharmacol. (2014) 21:389–95. doi: 10.1016/j.intimp.2014.05.022
53. Bacanlı, M, Başaran, AA, and Başaran, N. The antioxidant, cytotoxic, and antigenotoxic effects of galangin, puerarin, and ursolic acid in mammalian cells. Drug Chem Toxicol. (2017) 40:256–62. doi: 10.1080/01480545.2016.1209680
54. Thiruvengadam, M, Venkidasamy, B, Subramanian, U, Samynathan, R, Ali Shariati, M, Rebezov, M, et al. Bioactive compounds in oxidative stress-mediated diseases: targeting the NRF2/ARE signaling pathway and epigenetic regulation. Antioxidants (Basel). (2021) 10:1859. doi: 10.3390/antiox10121859
55. Ding, H, Wang, H, Zhu, L, and Wei, W. Ursolic acid ameliorates early brain injury after experimental traumatic brain injury in mice by activating the Nrf2 pathway. Neurochem Res. (2017) 42:337–46. doi: 10.1007/s11064-016-2077-8
56. Kim, H, Ramirez, CN, and Su, ZY. Epigenetic modifications of triterpenoid ursolic acid in activating Nrf2 and blocking cellular transformation of mouse epidermal cells. J Nutr Biochem. (2016) 33:54–62. doi: 10.1016/j.jnutbio.2015.09.014
57. Ma, JQ, Ding, J, Zhang, L, and Liu, CM. Protective effects of ursolic acid in an experimental model of liver fibrosis through Nrf2/ARE pathway. Clin Res Hepatol Gastroenterol. (2015) 39:188–97. doi: 10.1016/j.clinre.2014.09.007
58. Lee, SI. Protective effects of ursolic acid on osteoblastic differentiation via activation of IER3/Nrf2. J Dent Hyg Sci. (2019) 19:198–204. doi: 10.17135/jdhs.2019.19.3.198
59. Li, L, Zhang, X, and Cui, L. Ursolic acid promotes the neuroprotection by activating Nrf2 pathway after cerebral ischemia in mice. Brain Res. (2013) 1497:32–9. doi: 10.1016/j.brainres.2012.12.032
60. Naß, J, Abdelfatah, S, and Efferth, T. The triterpenoid ursolic acid ameliorates stress in Caenorhabditis elegans by affecting the depression-associated genes skn-1 and prdx2. Phytomedicine. (2021) 88:153598. doi: 10.1016/j.phymed.2021.153598
61. Li, S, Wu, R, Wang, L, Dina Kuo, HC, Sargsyan, D, Zheng, X, et al. Triterpenoid ursolic acid drives metabolic rewiring and epigenetic reprogramming in treatment/prevention of human prostate cancer. Mol Carcinog. (2022) 61:111–21. doi: 10.1002/mc.23365
62. Wu, S, Zhang, T, and Du, J. Ursolic acid sensitizes cisplatin-resistant HepG2/DDP cells to cisplatin via inhibiting Nrf2/ARE pathway. Drug Des Devel Ther. (2016) 10:3471–81. doi: 10.2147/DDDT.S110505
63. Feng, XM, and Su, XL. Anticancer effect of ursolic acid via mitochondria-dependent pathways. Oncol Lett. (2019) 17:4761–7. doi: 10.3892/ol.2019.10171
64. Luo, F, Zhao, J, Liu, S, Xue, Y, Tang, D, Yang, J, et al. Ursolic acid augments the chemosensitivity of drug-resistant breast cancer cells to doxorubicin by AMPK-mediated mitochondrial dysfunction. Biochem Pharmacol. (2022) 205:115278. doi: 10.1016/j.bcp.2022.115278
65. Lewinska, A, Adamczyk-Grochala, J, Kwasniewicz, E, Deregowska, A, and Wnuk, M. Ursolic acid-mediated changes in glycolytic pathway promote cytotoxic autophagy and apoptosis in phenotypically different breast cancer cells. Apoptosis. (2017) 22:800–15. doi: 10.1007/s10495-017-1353-7
66. Way, TD, Tsai, SJ, Wang, CM, Ho, C, and Chou, C. Chemical constituents of Rhododendron formosanum show pronounced growth inhibitory effect on non-small-cell lung carcinoma cells. J Agric Food Chem. (2014) 62:875–84. doi: 10.1021/jf404243p
67. Gou, W, Luo, N, and Yu, B. Ursolic acid derivative UA232 promotes tumor cell apoptosis by inducing endoplasmic reticulum stress and lysosomal dysfunction. Int J Biol Sci. (2022) 18:2639–51. doi: 10.7150/ijbs.67166
68. Zong, L, Cheng, G, and Zhao, J. Inhibitory effect of ursolic acid on the migration and invasion of doxorubicin-resistant breast cancer. Molecules. (2022) 27:1282. doi: 10.3390/molecules27041282
69. Fogde, DL, Xavier, CPR, and Balnytė, K. Ursolic acid impairs cellular lipid homeostasis and lysosomal membrane integrity in breast carcinoma cells. Cells. (2022) 11:4079. doi: 10.3390/cells11244079
70. Kang, DY, Sp, N, Jang, KJ, Jo, ES, Bae, SW, and Yang, YM. Antitumor effects of natural bioactive ursolic acid in embryonic cancer stem cells. J Oncol. (2022) 2022:6737248. doi: 10.1155/2022/6737248
71. Cheng, J, Liu, Y, and Liu, Y. Ursolic acid alleviates lipid accumulation by activating the AMPK signaling pathway in vivo and in vitro. J Food Sci. (2020) 85:3998–4008. doi: 10.1111/1750-3841.15475
72. Nie, Y, Liu, Q, and Zhang, W. Ursolic acid reverses liver fibrosis by inhibiting NOX4/NLRP3 inflammasome pathways and bacterial dysbiosis. Gut Microbes. (2021) 13:1972746. doi: 10.1080/19490976.2021.1972746
73. Ma, JQ, Ding, J, Zhang, L, and Liu, CM. Ursolic acid protects mouse liver against CCl4-induced oxidative stress and inflammation by the MAPK/NF-κB pathway. Environ Toxicol Pharmacol. (2014) 37:975–83. doi: 10.1016/j.etap.2014.03.011
74. Gan, D, Zhang, W, and Huang, C. Ursolic acid ameliorates CCl4-induced liver fibrosis through the NOXs/ROS pathway. J Cell Physiol. (2018) 233:6799–813. doi: 10.1002/jcp.26541
75. Zhang, W, Gan, D, Jian, J, Huang, C, and Zhu, X. Protective effect of ursolic acid on the intestinal mucosal barrier in a rat model of liver fibrosis. Front Physiol. (2019) 10:956. doi: 10.3389/fphys.2019.00956
76. Furfaro, LL, Payne, MS, and Chang, BJ. Bacteriophage therapy: clinical trials and regulatory hurdles. Front cell infect Microbiol. (2018, 2019) 8:376. doi: 10.3389/fcimb.2018.00376
78. Egodawaththa, NM, Knight, AL, and Ma, J. Synthesis and characterization of ligand-stabilized silver nanoparticles and comparative antibacterial activity against E. coli. Int J Mol Sci. (2022) 23:15251. doi: 10.3390/ijms232315251
79. Manso, T, Lores, M, and de Miguel, T. Antimicrobial activity of polyphenols and natural polyphenolic extracts on clinical isolates. Antibiotics (Basel). (2021) 11:46. doi: 10.3390/antibiotics11010046
80. Biharee, A, Sharma, A, Kumar, A, and Jaitak, V. Antimicrobial flavonoids as a potential substitute for overcoming antimicrobial resistance. Fitoterapia. (2020) 146:104720. doi: 10.1016/j.fitote.2020.104720
81. Sycz, Z, Tichaczek-Goska, D, and Wojnicz, D. Anti-planktonic and anti-biofilm properties of pentacyclic triterpenes-asiatic acid and ursolic acid as promising antibacterial future pharmaceuticals. Biomol Ther. (2022) 12:98. doi: 10.3390/biom12010098
82. Lopes, TS, Fontoura, PS, Oliveira, A, Rizzo, FA, and Streck, AF. Use of plant extracts and essential oils in the control of bovine mastitis. Res Vet Sci. (2020) 131:186–93. doi: 10.1016/j.rvsc.2020.04.025
83. Othman, L, Sleiman, A, and Abdel-Massih, RM. Antimicrobial activity of polyphenols and alkaloids in middle eastern plants. Front Microbiol. (2019) 10:911. doi: 10.3389/fmicb.2019.00911
84. Gurib-Fakim, A. Medicinal plants: traditions of yesterday and drugs of tomorrow. Mol Asp Med. (2006) 27:1–93. doi: 10.1016/j.mam.2005.07.008
85. Kurek, A, Nadkowska, P, Pliszka, S, and Wolska, KI. Modulation of antibiotic resistance in bacterial pathogens by oleanolic acid and ursolic acid. Phytomedicine. (2012) 19:515–9. doi: 10.1016/j.phymed.2011.12.009
86. Sundaramoorthy, NS, Mohan, HM, Subramaniam, S, Raman, T, Selva Ganesan, S, Sivasubamanian, A, et al. Ursolic acid inhibits colistin efflux and curtails colistin resistant Enterobacteriaceae. AMB Express. (2019) 9:27. doi: 10.1186/s13568-019-0750-4
87. Wang, CM, Chen, HT, Wu, ZY, Jhan, YL, Shyu, CL, and Chou, CH. Antibacterial and synergistic activity of pentacyclic triterpenoids isolated from alstonia scholaris. Molecules. (2016) 21:139. doi: 10.3390/molecules21020139
88. Catteau, L, Reichmann, NT, Olson, J, Pinho, MG, Nizet, V, Van Bambeke, F, et al. Synergy between ursolic and oleanolic acids from vitellaria paradoxa leaf extract and β-lactams against methicillin-resistant Staphylococcus aureus: in vitro and in vivo activity and underlying mechanisms. Molecules. (2017) 22:2245. doi: 10.3390/molecules22122245
89. Zhou, L, Ding, Y, Chen, W, Zhang, P, Chen, Y, and Lv, X. The in vitro study of ursolic acid and oleanolic acid inhibiting cariogenic microorganisms as well as biofilm. Oral Dis. (2013) 19:494–500. doi: 10.1111/odi.12031
90. Qian, W, Wang, W, Zhang, J, Wang, T, Liu, M, Yang, M, et al. Antimicrobial and antibiofilm activities of ursolic acid against carbapenem-resistant Klebsiella pneumoniae. J Antibiot (Tokyo). (2020) 73:382–91. doi: 10.1038/s41429-020-0285-6
91. Kefi, S, Essid, R, Papetti, A, Abid, G, Bouslama, L, Aouani, E, et al. Antioxidant, antibacterial and antileishmanial potential of Micromeria nervosa extracts and molecular mechanism of action of the bioactive compound. J Appl Microbiol. (2023) 134:lxad007. doi: 10.1093/jambio/lxad007
92. Wang, WJ, Liu, CC, Li, YT, Li, MQ, Fu, YT, Li, XC, et al. Antifungal and antibiofilm in vitro activities of ursolic acid on cryptococcus neoformans. Curr Microbiol. (2022) 79:293. doi: 10.1007/s00284-022-02992-5
93. Liu, R, Zhang, L, Xiao, S, Chen, H, Han, Y, Niu, B, et al. Ursolic acid, the main component of blueberry cuticular wax, inhibits Botrytis cinerea growth by damaging cell membrane integrity. Food Chem. (2023) 415:135753. doi: 10.1016/j.foodchem.2023.135753
94. Micota, B, Sadowska, B, Podsędek, A, Redzynia, M, and Różalska, B. Leonurus cardiaca L. herb--a derived extract and an ursolic acid as the factors affecting the adhesion capacity of Staphylococcus aureus in the context of infective endocarditis. Acta Biochim Pol. (2014) 61:385–8.
95. Lyu, X, Wang, L, Shui, Y, Jiang, Q, Chen, L, Yang, W, et al. Ursolic acid inhibits multi-species biofilms developed by Streptococcus mutans, Streptococcus sanguinis, and Streptococcus gordonii. Arch Oral Biol. (2021) 125:105107. doi: 10.1016/j.archoralbio.2021.105107
96. Ren, D, Zuo, R, and González Barrios, AF. Differential gene expression for investigation of Escherichia coli biofilm inhibition by plant extract ursolic acid. Appl Environ Microbiol. (2019) 71:4022–34. doi: 10.1128/AEM.71.7.4022-4034.2005
97. Liu, Y, Huang, Y, Fan, C, Chi, M, Bai, M, and Sun, L. Ursolic acid targets glucosyltransferase and inhibits its activity to prevent Streptococcus mutans biofilm formation. Front Microbiol. (2021) 12:743305. doi: 10.3389/fmicb.2021.833726
98. Akbarsha, MA, Palanisamy, M, Murugaian, P, and Lakshmi Latha, PN. Ursolic acid generates symplasts in rat spermatogenic clones. Phytother Res. (1998) 12:32–6. doi: 10.1002/(SICI)1099-1573(19980201)12:1<32::AID-PTR185>3.0.CO;2-4
99. Tu, HY, Huang, AM, Wei, BL, Gan, KH, Hour, TC, Yang, SC, et al. Ursolic acid derivatives induce cell cycle arrest and apoptosis in NTUB1 cells associated with reactive oxygen species. Bioorg Med Chem. (2009) 17:7265–74. doi: 10.1016/j.bmc.2009.08.046
100. Lu, J, Guan, S, and Liu, JB. Acute and genetic toxicity of ursolic acid extract from Ledum pulastre L. Food Sci. (2009) 13:250–2.
101. He, X, Ding, P, Song, ZH, and Zhang, HH. (2023). Application of ursolic acid in broiler breeding and broiler feed containing ursolic acid. CN115669816A.
102. Erdenebayar, O, Liu, DB, Yang, L, Cheng, JY, and Li, QW. The effect of ursolic acid on cryopreservation of boar spermatozoa. Acta Ecologiae Animalis Domastici. (2022) 43:49–53. doi: 10.3969/j.issn.1673-1182.2022.10.008
103. Slate, JR, Chriswell, BO, and Briggs, RE. The effects of ursolic acid treatment on immunopathogenesis following mannheimia haemolytica infections. Front Vet Sci. (2021) 8:782872. doi: 10.3389/fvets.2021.782872
104. Li, BY, Qin, JC, Shen, YF, Yang, F, Wang, T, Ling, F, et al. A therapeutic agent of ursolic acid demonstrates potential application in aquaculture. Virus Res. (2022) 323:198965. doi: 10.1016/j.virusres.2022.198993
105. Sun, X, Chen, X, Wang, S, Zhang, J, Wu, B, and Qin, G. Protective effect of ursolic acid in prunella vulgaris L. on LPS-induced asthenozoospermia via Bcl-2/Bax apoptosis signaling pathway. Curr Pharm Biotechnol. (2021) 22:1953–9. doi: 10.2174/1389201021666201027155413
106. Kang, WY, Song, YL, Ji, ZQ, and Wang, JM. (2010). The antibacterial application of a natural compound ursolic acid. CN101543499B.
107. James, ES, Shen, DD, Juliani, Hector Rodolfo, and Wu, QL. (2012). Oregano and mint anti-inflammatory compositions and methods. US2012135094.
108. Guo, Y, Li, YX, and Cao, YL. (2014). The preparation and application of a kind of ursolic acid saponin against highly pathogenic H5N1 influenza virus. CN101941996B.
109. Zheng, ZJ, Qiao, XD, Zhu, SS, Qiu, ZC, Zhu, WQ, and Li, LY. (2022). The preparation and application of a composite anti-inflammatory nanoparticle. CN114159406B.
110. Zhang, SZ. (2017). The diet for enhancing the immunity of geese by adding garlic powder and Ligustrine powder. CN107259179A.
111. Jung, KY, Hun, JK, Hun, SK, and Won, JK. (2022). Feed additive composition and feed composition comprising the same. AU2019388142B2.
112. Wu, LF, Gao, W, Song, WG, Zhao, PY, and Zhao, J. (2023). A feed additive composition for improving intestinal health of livestock and poultry and its application. CN116268209A.
113. Wang, JB, Gao, F, Han, WC, Cao, RF, and Fu, KQ. Ursolic acid inhibits LPS-induced bovine endometrial cell inflammation by NF-κB signaling. Chin J Anim Sci. (2021) 57:261–4. doi: 10.19556/j.0258-7033.20210617-03
114. Ding, YJ, Sun, CY, Wen, CC, and Chen, YH. Nephroprotective role of resveratrol and ursolic acid in aristolochic acid intoxicated zebrafish. Toxins (Basel). (2015) 7:97–109. doi: 10.3390/toxins7010097
115. Salomón, R, Reyes-López, FE, and Tort, L. Medicinal plant leaf extract from sage and lemon verbena promotes intestinal immunity and barrier function in gilthead seabream (Sparus aurata). Front Immunol. (2021) 12:670279. doi: 10.3389/fimmu.2021.670279
116. Song, G, Shen, X, Li, S, Li, Y, Si, H, Fan, J, et al. Structure-activity relationships of 3-O-β-chacotriosyl ursolic acid derivatives as novel H5N1 entry inhibitors. Eur J Med Chem. (2015) 93:431–42. doi: 10.1016/j.ejmech.2015.02.029
117. Chen, Y, Li, H, Wu, L, Zhang, M, and Chen, J. Ursolic acid derivatives are potent inhibitors against porcine reproductive and respiratory syndrome virus. RSC Adv. (2020) 10:22783–96. doi: 10.1039/D0RA04070C
118. Li, BY, Hu, Y, and Li, J. Ursolic acid from Prunella vulgaris L. efficiently inhibits IHNV infection in vitro and in vivo. Virus Res. (2019) 273:197741. doi: 10.1016/j.virusres.2019.197741
119. Salomón, R, Firmino, JP, Reyes-López, FE, Andree, KB, González-Silvera, D, Esteban, MA, et al. The growth promoting and immunomodulatory effects of a medicinal plant leaf extract obtained from Salvia officinalis and Lippia citriodora in gilthead seabream (Sparus aurata). Aquaculture. (2020) 524:735291. doi: 10.1016/j.aquaculture.2020.735291
120. Jadhav, P, Lal, H, and Kshirsagar, N. Assessment of potency of PC-complexed Ocimum sanctum methanol extract in embryonated eggs against influenza virus (H1N1). Pharmacogn Mag. (2014) 10:S86–91. doi: 10.4103/0973-1296.127352
121. Shrivastav, A, Sharma, RK, Shrivastava, N, Gautam, V, and Jain, SK. Study of inhibitory potential and percent inhibition of oil of Syzygium aromaticum and leaves of Ocimum sanctum on ESBL enzyme from Escherichia coli in broilers of Jabalpur. Indian J Pharmacol. (2019) 51:337–42. doi: 10.4103/ijp.IJP_87_17
Glossary
Keywords: ursolic acid, anti-inflammatory, antioxidative, antimicrobial, hepatoprotective, anticancer, antiviral activity, animal husbandry
Citation: Liu G, Qin P, Cheng X, Wu L, Wang R and Gao W (2023) Ursolic acid: biological functions and application in animal husbandry. Front. Vet. Sci. 10:1251248. doi: 10.3389/fvets.2023.1251248
Edited by:
Hany M. R. Abdel-Latif, Alexandria University, EgyptReviewed by:
Mustafa Shukry, Kafrelsheikh University, EgyptSeyed Pezhman Hosseini Shekarabi, Islamic Azad University, Iran
Copyright © 2023 Liu, Qin, Cheng, Wu, Wang and Gao. This is an open-access article distributed under the terms of the Creative Commons Attribution License (CC BY). The use, distribution or reproduction in other forums is permitted, provided the original author(s) and the copyright owner(s) are credited and that the original publication in this journal is cited, in accordance with accepted academic practice. No use, distribution or reproduction is permitted which does not comply with these terms.
*Correspondence: Wei Gao, Z3dAY2NnYi5jb20uY24=