- 1Guangxi Center for Animal Disease Control and Prevention, Nanning, China
- 2College of Animal Science and Technology, Guangxi University, Nanning, China
- 3Longan Center for Animal Disease Control and Prevention, Nanning, China
- 4Guangxi State Farms Yongxin Animal Husbandry Group Xijiang Co., Ltd., Guigang, China
- 5Wenzhou Key Laboratory for Virology and Immunology, Institute of Virology, Wenzhou University, Wenzhou, China
Duck Tembusu virus (DTMUV), duck circovirus (DuCV), and new duck reovirus (NDRV) have seriously hindered the development of the poultry industry in China. To detect the three pathogens simultaneously, a multiplex digital PCR (dPCR) was developed and compared with multiplex qPCR in this study. The multiplex dPCR was able to specifically detect DTMUV, DuCV, and NDRV but not amplify Muscovy duck reovirus (MDRV), Muscovy duck parvovirus (MDPV), goose parvovirus (GPV), H4 avian influenza virus (H4 AIV), H6 avian influenza virus (H6 AIV), and Newcastle disease virus (NDV). The standard curves showed excellent linearity in multiplex dPCR and qPCR and were positively correlated. The sensitivity results showed that the lowest detection limit of multiplex dPCR was 1.3 copies/μL, which was 10 times higher than that of multiplex qPCR. The reproducibility results showed that the intra- and interassay coefficients of variation were 0.06–1.94%. A total of 173 clinical samples were tested to assess the usefulness of the method; the positive detection rates for DTMUV, DuCV, and NDRV were 18.5, 29.5, and 14.5%, respectively, which were approximately 4% higher than those of multiplex qPCR, and the kappa values for the clinical detection results of multiplex dPCR and qPCR were 0.85, 0.89, and 0.86, indicating that the two methods were in excellent agreement.
Introduction
Tembusu virus (TMUV), a positive-sense single-stranded RNA virus belonging to the Flaviviridae family and Flavivirus genus (1), was first detected in the Malaysian Culex mosquito in 1955 and became endemic in duck farms in southeastern coastal China in 2010 (2, 3). DTMUV has a wide range of hosts, such as chickens, ducks, geese, pigeons, and sparrows, and the diseased ducks show a series of symptoms, including ovarian hemorrhage and necrosis, decreased egg production, ataxia, diarrhea, and enlarged spleen and liver, with an incidence of up to 100% and mortality rates between 5 and 30% (4, 5); moreover, younger ducks exhibit more serious pathogenicity (6). Reportedly, DTMUV antibodies have been detected in the serum of duck farm workers (7), indicating that the disease may pose a public safety risk. Duck circovirus (DuCV) is a circular single-stranded DNA virus belonging to the genus Circovirus of the family Circoviridae (8), which first appeared in Germany in 2003 and subsequently spread to China, Korea, and the United States (9, 10). DuCV is highly contagious, mainly through horizontal and vertical transmission (11, 12); ducks of all ages can be infected, and the highest rate of infection is observed 3 to 4 weeks of age (13). Moreover, the incidence of this virus is 10–81.6% in China, and the disease ducks show feathering disorders, stunted growth, reduced production performance, immune organ defects and cell necrosis (14). The abovementioned features seriously affect the development of the duck breeding industry. DuCV-1 and DuCV-2 are prevalent in China, but a recent study revealed the identification of a new genotype (DuCV3) in duck farms in Hunan, China (15), which has attracted attention. Novel duck reovirus (NDRV) belongs to the Orthoreovirus genus in the family Reoviridae and is a double-stranded RNA virus (16). NDRV was first identified in Fujian in 2005 (17) and has become endemic in duck farms in China. NDRV can cause morbidity in ducks and geese, and ducks are most susceptible at 7–14 days of age, with an incidence of 5–35% and mortality of 2–20% (18). Ducks exhibit a pathology characterized by hemorrhagic necrosis of the liver and spleen, diarrhea, and growth retardation, and the disease is also known as spleen necrosis disease or duck hemorrhagic-necrotic hepatitis (19). In summary, DTMUV, DuCV, and NDRV, which are commonly prevalent in the farming industry in China, have a great impact on and causes loss to the poultry farming industry. Therefore, early diagnosis and prevention of the spread of these pathogens in poultry flocks are of great practical importance.
Digital PCR (dPCR) is a nucleic acid absolute quantification technology developed in the late 1990s (20). This technique can be understood as single-molecule-level fluorescent PCR amplification in large-scale parallel microreactors, and after the PCR reaction, the copy number of the target nucleic acid sequence is calculated based on the fluorescent signal and Poisson distribution (21). Due to its advantages of high sensitivity, high accuracy, high tolerance, and absolute quantification (22), dPCR is superior to qPCR technology in clinical settings and has been widely used in genetic mutation diagnosis, copy number variation analysis, and pathogenic microorganism detection. In this study, a new method was developed by applying multiplex dPCR technology for the first time, and this developed method provides a technical tool for the simultaneous detection of DTMUV, DuCV, and NDRV.
Materials and methods
Viral strains and clinical samples
The viral strains included DTMUV (tissue sample), DuCV (tissue sample), NDRV (tissue sample), Muscovy duck reovirus (MDRV, tissue sample), Muscovy duck parvovirus (MDPV, tissue sample), goose parvovirus (GPV, tissue sample), H4 avian influenza virus (H4 AIV, tissue sample), H6 avian influenza virus (H6 AIV, tissue sample), and Newcastle disease virus (NDV, La Sota vaccine strain). A total of 173 clinical tissue samples of ducks were collected from Guangxi in China. The tissues were placed into 2.0-mL centrifuge tubes containing PBS at pH 7.0, ground into a crumbly form using a grinder, and centrifuged at 10,000 rpm for 5 min, and the supernatant was used for the extraction of total nucleic acids using a TaKaRa MiniBEST Viral RNA/DNA Extraction Kit Ver.5.0 (Dalian, China). RNA from clinical samples or vaccine strains was reverse transcribed to cDNA using the PrimeScript™ II 1st Strand cDNA Synthesis Kit (Dalian, China), and the DNA and cDNA were stored at −80°C. All clinical tissue samples were collected from sick and dead ducks transported from farms and stored at the Guangxi Animal Disease Prevention and Control Center in China.
Primers and probes
Primers and probes for multiplex dPCR amplification of the DTMUV (KC990540) C gene, DuCV (MK814589) rep gene, and NDRV (GQ888710) S3 gene were designed using Olign 7.6 (Table 1).
Construction of standard plasmids
PCR amplification was performed with DTMUV, DuCV, NDRV cDNA, or DNA as a template using three pairs of specific primers, and the PCR products were purified by applying the TaKaRa MiniBEST Agarose Gel DNA Extraction Kit Ver.4.0 (Dalian, China) and ligated into the pMD™18-T Vector (China); the constructs were transformed into E. coli DH5α competent cells, coated and incubated at 37°C for 16 h, and the TaKaRa MiniBEST Plasmid Purification Kit Ver. 4.0 (Dalian, China) was applied for extraction of the plasmids, which were named p-DTMUV, p-DuCV, and p-NDRV, respectively, and sequencing. The concentration of each plasmid was determined and normalized using the following formula: plasmid copy number (copies/μL) = plasmid concentration × 10−9 × 6.02 × 1023)/(660 Dalton/bases × DNA length).
Optimization of optimal reaction conditions for multiplex dPCR
The NaicaTM System (Stilla Technologies, Villejuif, France) was applied to optimize the DTMUV, DuCV, and NDRV primer and probe concentrations and annealing temperature (55–60°C). The reaction systems included 12.5 μL of PerfeCTa Multiplex qPCR ToughMix UNG (USA); 2.5 μL of Fluorescein Sodium Salt (Beijing, China); primers and probes for DTMUV, DuCV, and NDRV at concentrations of 600–1,000 nM and 200–400 nM, respectively; 2.5 μL of template; and double distilled water up to 25 μL. The reaction procedure was as follows: Step 1: predenaturation at 95°C for 5 min; Step 2: 45 cycles of denaturation at 95°C for 5 s, annealing at 57°C for 30 s, and 72°C for 30 s; Step 3: 72°C for 5 min. Moreover, the QuantStudio 5 qPCR detection system (USA) was applied to optimize the reaction conditions for qPCR.
Construction of standard curves
Equal amounts of the standard plasmids for the three viruses were mixed and diluted to 1.3 × 105-1.3 × 101 copies/μL via 10-fold serial dilution. The linearity of multiplex dPCR and qPCR was statistically analyzed using SPSS 27.0, the correlation between them was assessed, and standard curves were plotted.
Specificity, sensitivity, and reproducibility assays
Multiplex dPCR amplification was performed using DTMUV, DuCV, NDRV, MDRV, MDPV, GPV, H4 AIV, H6 AIV, and NDV cDNA or DNA as templates and sterilized water as a negative control to assess the specificity.
Equal amounts of standard plasmids for the three viruses were mixed, diluted to 1.3 × 108 copies/μL−1.3 × 100 copies/μL via 10-fold serial dilution and used as templates for multiplex dPCR and multiplex qPCR amplification to evaluate the detection limits.
The standard plasmids for the three viruses were mixed in equal volumes and diluted via 10-fold serial dilution to 1.3 × 105 copies/μL, 1.3 × 104 copies/μL, and 1.3 × 103 copies/μL for use as templates for multiplex dPCR amplification to assess the reproducibility.
Clinical assays for multiplex dPCR and multiplex qPCR
A total of 173 clinical samples were tested by multiplex dPCR to verify their utility. The reaction system consisted of 12.5 μL of PerfeCTa Multiplex qPCR ToughMix UNG (USA), 2.5 μL of Fluorescein Sodium Salt (Beijing, China), 0.9 μL each of DTMUV-Fq and DTMUV-Rq (25 pmol/μL), 0.3 μL of DTMUV-P (25 pmol/μL), 0.8 μL of DuCV-Fq and DuCV-Rq (25 pmol/μL), 0.3 μL of DuCV-P (25 pmol/μL), 0.9 μL each of NDRV-Fq and NDRV-Rq (25 pmol/μL), 0.3 μL of NDRV-P (25 pmol/μL), 2.5 μL of template, and double-distilled water to 25 μL. The reaction procedure was as follows: Step 1: pre-denaturation at 95°C for 5 min; Step 2: 45 cycles of denaturation at 95°C for 5 s, annealing at 57°C for 30 s, and 72°C for 30 s; Step 3: 72°C for 5 min (Table 2). Moreover, multiplex qPCR was applied to examine the same diseased material. Kappa values were calculated to compare the consistency of the two assays (when K < 0, the consistency strength was very poor; 0–0.2, weak; 0.21–0.4 weak; 0.41–0.6, moderate; 0.61–0.8, high; 0.81–1, very strong).
Results
Construction of standard plasmids
Standard plasmids for three viruses were obtained and named p-DTMUV, p-DuCV, and p-NDRV. The constructs were verified by sequencing. The concentrations were calculated using the formula and were found to equal 2.13 × 1010 copies/μL, 1.74 × 1010 copies/μL, and 1.30 × 1010 copies/μL, respectively, and the samples were diluted to obtain the same concentration of 1.30 × 1010 copies/μL.
Optimal reaction conditions
The concentration combined with the high number of microdroplets generated, high fluorescence signal value of positive microdroplets, good microdroplet density, the clear distinction between fluorescence signal value of negative and positive microdroplets, and low number of intermediate diffuse microdroplets were selected as the best reaction conditions. The optimal primer and probe volumes and concentrations for dPCR of DTMUV, DuCV, and NDRV were as follows: 0.9 μL each for DTMUV-Fq and DTMUV-Rq (25 pmol/μL), 0.3 μL for DTMUV-P (25 pmol/μL), 0.8 μL each for DuCV-Fq and DuCV-Rq (25 pmol/μL), 0.3 μL for DuCV-P (25 pmol/μL), 0.9 μL each for NDRV-Fq and NDRV-Rq (25 pmol/μL), and 0.3 μL for NDRV-P (25 pmol/μL). The optimal annealing temperature for the three viruses was 57°C, which produced the highest number of positive and total droplets (Figure 1). Meanwhile, the optimal primer and probe volumes and concentrations for qPCR were as follows: 0.1 μL each for DTMUV-Fq, DTMUV-Rq, and DTMUV-P (25 pmol/μL), 0.2 μL each for DuCV-Fq and DuCV-Rq (25 pmol/μL), 0.3 μL for DuCV-P (25 pmol/μL), and 0.2 μL each for NDRV-Fq, NDRV-Rq, and NDRV-P (25 pmol/μL). The annealing temperature was 57°C.
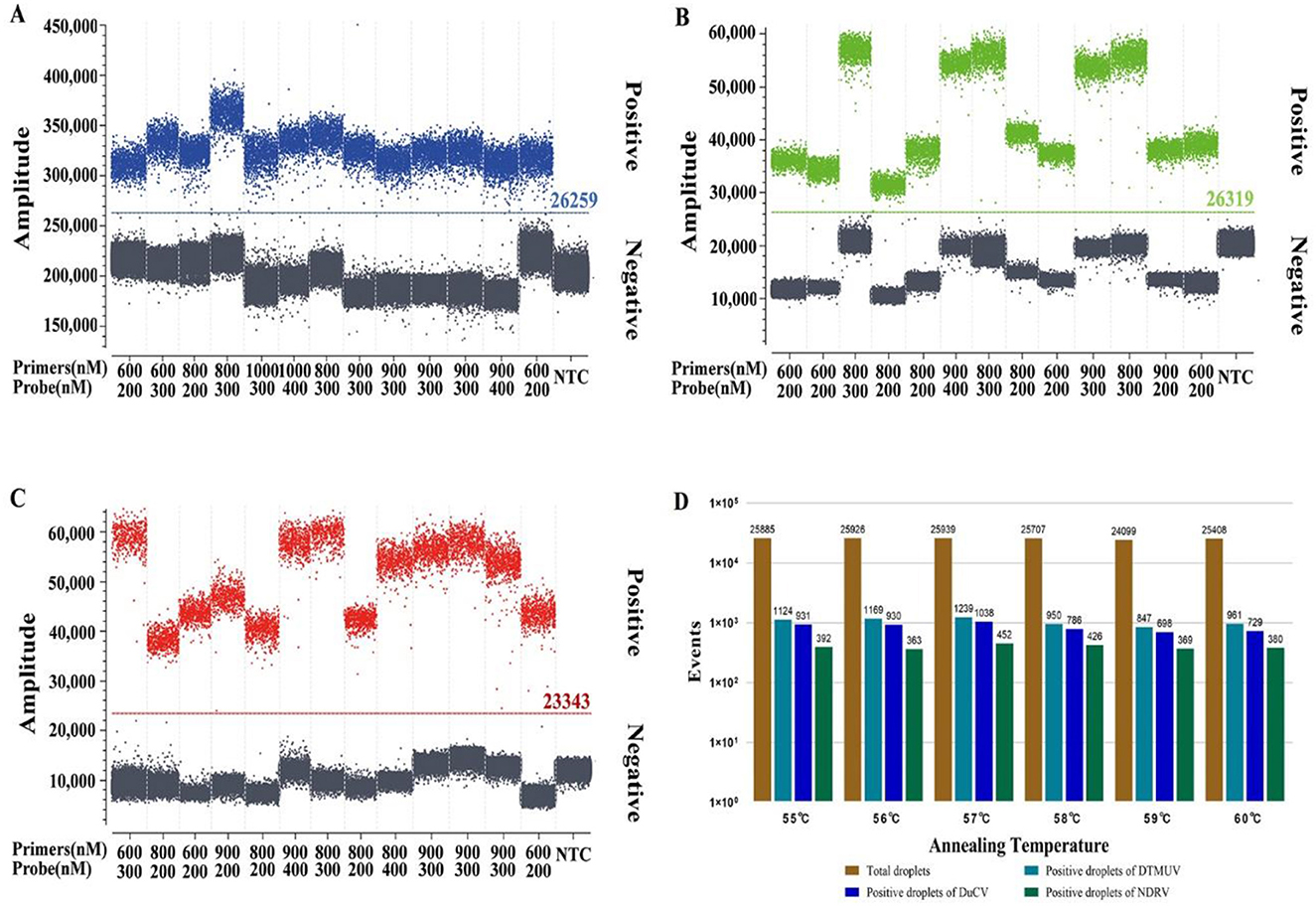
Figure 1. Optimization of the reaction conditions for multiplex dPCR. (A–C) show the optimization results for the multiplex dPCR primer and probe concentrations for duck Tembusu virus, duck circovirus, and new duck reovirus, respectively, and (D) shows the annealing temperature.
Construction of standard curves
The multiplex dPCR standard curve results showed that the R2 and slope values for DTMUV, DuCV, and NDRV were −0.999 and 0.997, 0.999 and 0.978, and 0.999 and 0.981, respectively. The multiplex qPCR results showed that the R2 and slope values for DTMUV, DuCV, and NDRV were 0.999 and −3.3787, 0.997 and −3.4352, and 0.996 and −3.3856, respectively. The Pearson correlation coefficients for multiplex dPCR and qPCR for DTMUV, DuCV, and NDRV were 0.998, 0.998, and 0.995, respectively, indicating a positive correlation between the two methods (Figure 2).
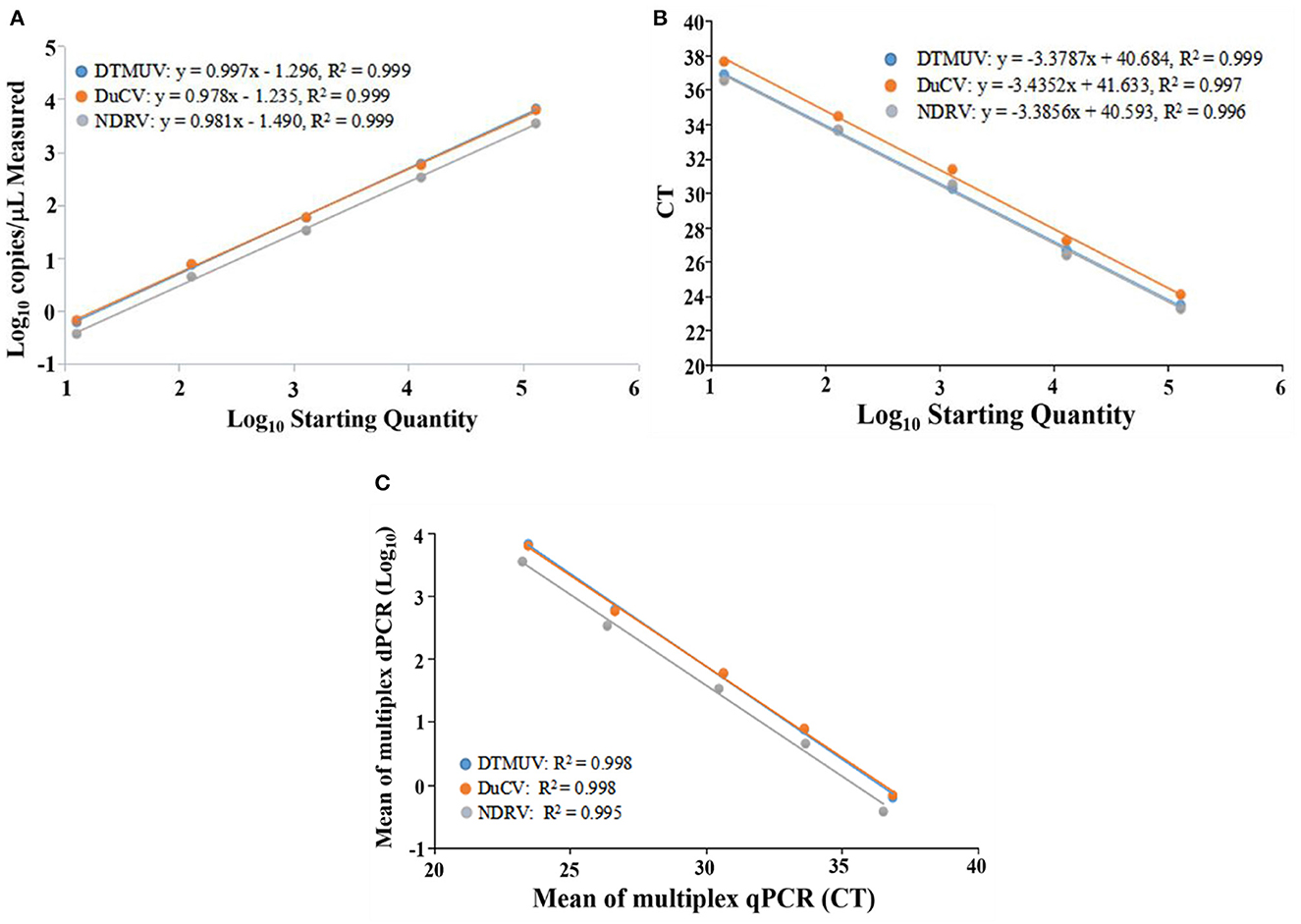
Figure 2. Standard curve of duck Tembusu virus, duck circovirus and new duck reovirus. (A, B) show the standard curves of multiplex dPCR and multiplex qPCR, respectively, and (C) indicates the correlation between them.
Analyses of specificity, sensitivity, and reproducibility
The specificity analysis showed that only DTMUV, DuCV, and NDRV cDNA and DNA showed specific amplification, whereas MDRV, MDPV, GPV, H4 AIV, H6 AIV, and NDV cDNA and DNA and the negative control did not show positive microdroplets (Figure 3). The limit of detection for multiplex dPCR was 1.3 copies/μL, and that for multiplex qPCR was 13 copies/μL (Figure 4). The intra-assay coefficient of variation ranged from 0.06 to 1.35%, and the inter-assay coefficient of variation ranged from 0.23 to 1.94% (Table 3).
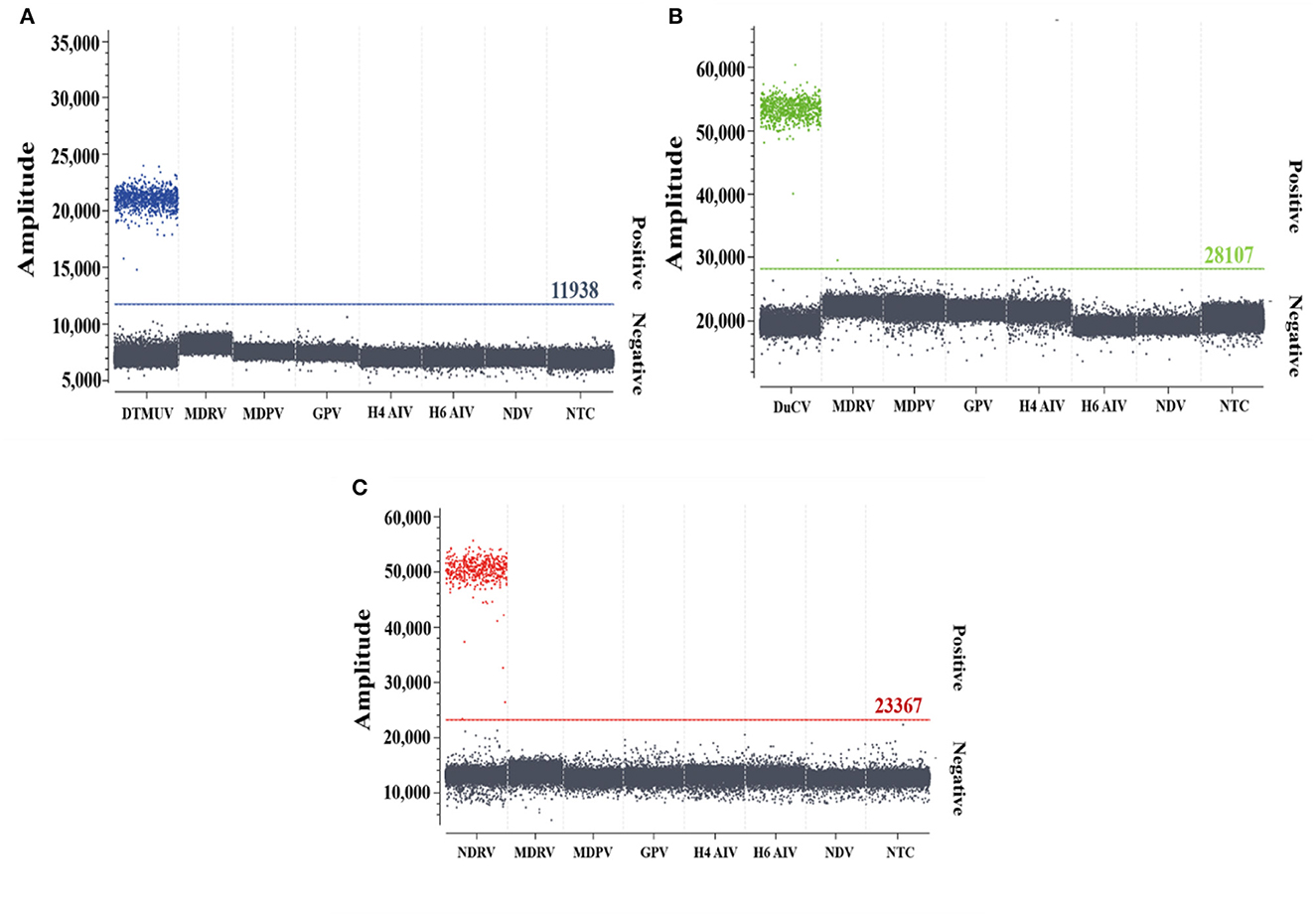
Figure 3. Specificity analysis of multiplex dPCR. (A–C) show the specific amplification of duck Tembusu virus, duck circovirus, and novel duck reovirus by multiplex dPCR, respectively.
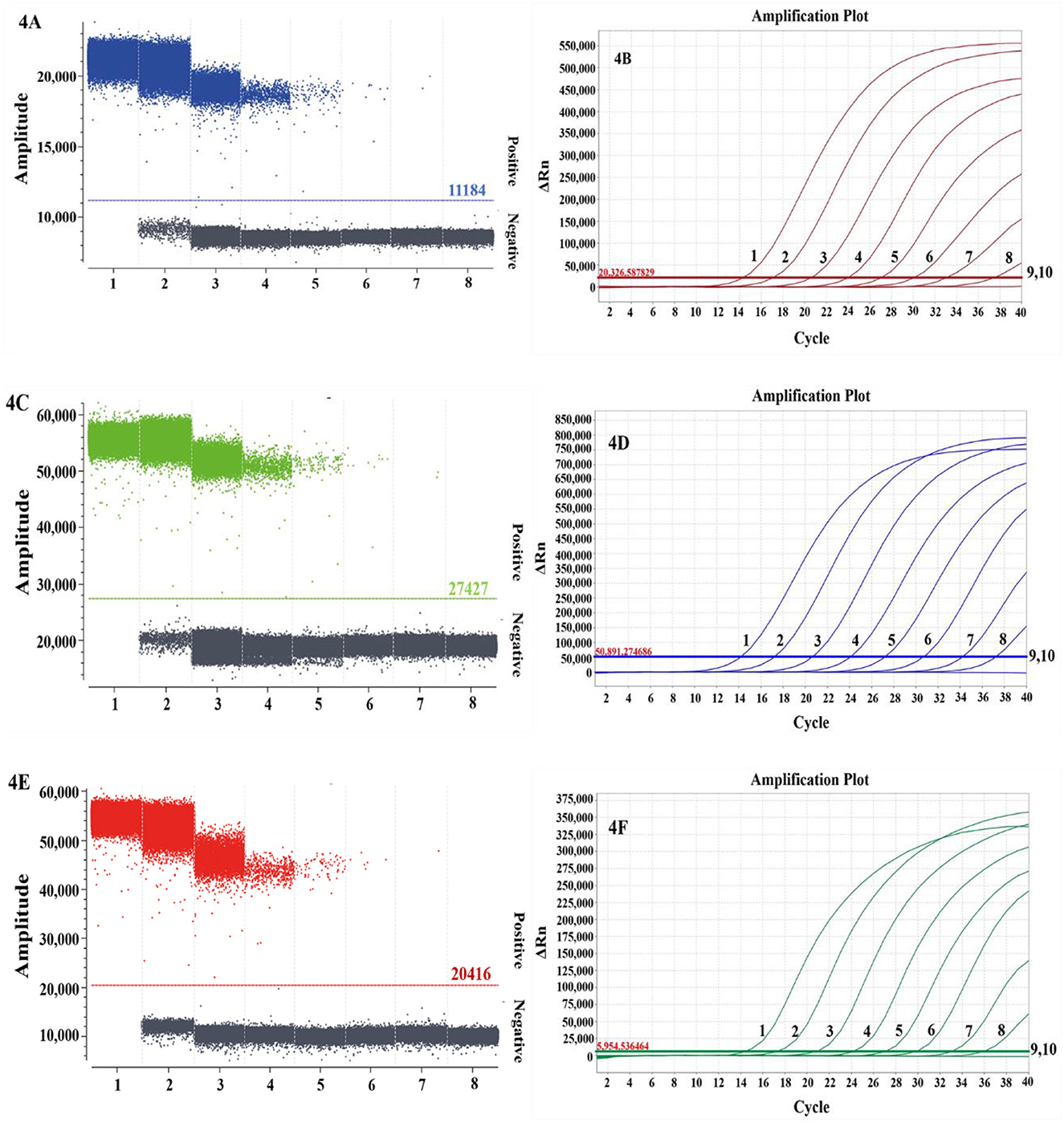
Figure 4. Comparison of the sensitivities of multiplex dPCR (A, C, E) and multiplex qPCR (B, D, F) for duck Tembusu virus (A, B), duck circovirus (C, D), and novel duck reovirus (E, F). In (A, C, E), 1–7 represent 1.30 × 106-1.30 × 100 copies/μL, and 8 represents the negative control; in (B, D, F), 1–9 represent 1.30 × 108-1.30 × 100 copies/μL, and 10 represents the negative control.
Clinical results for multiplex dPCR and multiplex qPCR
A total of 173 clinical samples were tested by multiplex dPCR, and the results showed that the positive detection rates for DTMUV, DuCV, and NDRV were 18.5, 29.5, and 14.5%, respectively, and that there were cases of simultaneous infection by several viruses in the same clinical sample during the testing process. The mixed infection rates for DTMUV+DuCV, DTMUV+NDRV, DuCV+NDRV, and DTMUV+DuCV+NDRV were 5.2% (9/173), 2.9% (5/173), 1.7% (3/173), and 1.2% (2/173), respectively. Meanwhile, multiplex qPCR was used to probe the same diseased material; the DTMUV, DuCV, and NDRV positivity rates were 14.5, 25.4, and 11.0% (Table 4), respectively, and the coinfection rates for DTMUV+DuCV, DTMUV+NDRV, DuCV+NDRV, and DTMUV+DuCV+NDRV were 4.6% (8/173), 1.7% (3/173), 1.7% (3/173), and 1.2% (2/173), respectively. When the results of the two assays were compared, the compliance rates for DTMUV, DuCV, and NDRV were 96, 96, and 97%, respectively, and the kappa values for DTMUV, DuCV, and NDRV were 0.85, 0.89, and 0.86, respectively (Table 5).
Discussion
In 2010, an infectious disease characterized by a sudden decrease in egg production (20–60%) emerged in Zhejiang, Fujian, Guangdong, and Jiangsu in China, and the causative virus was later confirmed to be DTMUV (23, 24). In autumn 2019, a new highly pathogenic DTMUV strain emerged in Anhui, China, belonging to genotype 2.2.1, which is far more pathogenic to geese and ducks than genotype 2.2 and may become the dominant group for DTMUV transmission in China (25). Epidemiological investigations have shown that DTMUV is widely prevalent in Chinese duck breeding farms, and DTMUV, AIV, duck hepatitis A virus, duck distemper virus, GPV, NDV, DuCV, fowl adenovirus, and Avian pathogenic Escherichia coli are the main pathogens that endanger the development of duck breeding industry and are often isolated from sick and dead ducks with serious mixed infections (26–28). The above findings show that DTMUV poses an extremely serious threat to China's waterfowl farming industry, and the infection is particularly complex and not easy to identify, requiring continuous epidemiological investigation and enhanced vaccination.
DuCV is an immunosuppressive virus that mainly affects the growth and development of ducks, attacks the immune system, aggravates the clinical symptoms of sick ducks and increases the mortality of sick ducks. When the immunity of the organism is reduced, opportunistic pathogens attack the ducks, and mixed and secondary infections occur. In China, DuCV has become prevalent, an incidences of infection have been reported in Guangxi (34.38%), Guangdong (25.6%), Yunnan (43.09%), and Shandong (33.29%) (8, 29, 30), with a large number of mixed infections with DuCV and Riemerella anatipestifer, Escherichia coli and duck hepatitis virus detected in duck farms in Shandong, at 8.28% (41/495), 4.85% (24/495), and 7.47% (37/495) (30), respectively. Similar coinfection with DuCV and other bacteria was detected in Korean duck flocks (31). In addition, we noted that mixed infections with DuCV and FAdV-4 may promote viral replication and cause more severe immunosuppression and injury (32). The spread of DuCV in China has caused considerable economic losses, and studies have suggested that migrating wild birds may play an important role in the spread, evolution and reorganization of DuCV (33), which is one of the reasons for the high incidence in China. Coupled with the lack of vaccines, the prevention of DuCV poses a serious challenge, and the key lies in strict testing of ducklings and elimination of positive ducks when introducing ducklings, strengthening feeding management, and improving environmental hygiene.
NDRV infection is an emerging infectious disease with clinical signs extremely similar to those of MDRV, but NDRV is more pathogenic. NDRV can cause immunosuppression, which leads to secondary and multiple infections (34), and most infected ducks die within 72 h (19). It is well known that most bacteria or viruses that attack animals cause damage to the spleen, liver, kidneys, and other organs of the host, thus exhibiting clinically similar symptoms and making it impossible to identify the pathogenic species. Early detection of the prevalence of the above pathogens in duck flocks can help interrupt the spread of the virus and reduce economic losses.
Due to the complexity of pathogenic infections and the immunosuppressive properties of DuCV and NDRV, multiple infections often occur with other viruses or bacteria, which are not easy to diagnose and differentiate. dPCR is a new tool for pathogen detection in the laboratory, has the advantages of absolute quantification without relying on standard curves, higher accuracy, and higher sensitivity, and has been used in medical and microbiological research (35). In this study, we developed a multiplex dPCR for the simultaneous detection of DTMUV, DuCV, and NDRV by optimizing the primers, probe concentration, and annealing temperature; the lowest detection limit reached 1.3 copies/μL, but that of the qPCR method established in this study was only 13 copies/μL; in addition, some qPCRs developed for DTMUV, DuCV, and NDRV have lower limits of detection of 101-102 copies/μL (36–40), which indicates that the sensitivity of our developed dPCR method has been further improved and is more suitable for the detection of pathogens at low concentrations. We also noted that the lower limits of detection of dPCR were 1 copies/μL−25 copies/μL regarding porcine circovirus type 2 (PCV2), porcine circovirus type 3 (PCV3), African swine fever virus (ASFV), classical swine fever virus (CSFV), porcine reproductive and respiratory syndrome (PRRS), and bovine leukemia virus (BLV) (41–44), indicating that the dPCR method we developed still has some advantages. We detected 173 clinical samples by dPCR, and the positive detection rates of DTMUV, DuCV, and NDRV were higher than those of qPCR, further validating the high sensitivity of dPCR. In addition, the established dPCR method is more precise than qPCR and can directly respond to the concentration of DTMUV, DuCV, and NDRV in clinical testing. However, despite the excellent detection sensitivity of dPCR, the clinical applications remain limited for various reasons, such as the limitations of the NaicaTM System, the low volume of the established dPCR method, the difficulty in carrying out pathogen detection on a large scale, and more expensive instrumentation and reagents. Compared with qPCR, the reaction mixture volume is limited, the operation is more tedious, and the risk of contamination is higher; thus, qPCR technology is still the most widely used genetic testing tool. With the update of science and technology, it is believed that these drawbacks of dPCR will be solved, and this method will thus be widely promoted in the field of microbiological research.
In conclusion, we developed a more sensitive multiplex dPCR for the simultaneous detection of DTMUV, DuCV, and NDRV, and this method provides a new tool for microbiological research.
Data availability statement
The original contributions presented in the study are included in the article/supplementary material, further inquiries can be directed to the corresponding authors.
Ethics statement
The animal study was reviewed and approved by Guangxi Center for Animal Disease Control and Prevention. Written informed consent was obtained from the owners for the participation of their animals in this study.
Author contributions
YY and CX designed the research. KS and FL performed the majority of the experiments and were involved in preparation of the manuscript. SF, SQ, WL, MH, and CL analyzed data. WS and ZL revised the manuscript. All authors contributed to the article and approved the submitted version.
Funding
This study was supported by the Guangxi Natural Science Foundation (Youth Science Foundation Project) (2020GXNSFBA297066), China and the Guangxi Agricultural Science and Technology Grant Project (Z201984 and Z202032), China.
Acknowledgments
We thank Guangxi Center for Animal Disease Control and Prevention (CADC) for providing the assay platform and clinical samples.
Conflict of interest
CL is employed by Guangxi State Farms Yongxin Animal Husbandry Group Xijiang Co. Ltd.
The remaining authors declare that the research was conducted in the absence of any commercial or financial relationships that could be construed as a potential conflict of interest.
Publisher's note
All claims expressed in this article are solely those of the authors and do not necessarily represent those of their affiliated organizations, or those of the publisher, the editors and the reviewers. Any product that may be evaluated in this article, or claim that may be made by its manufacturer, is not guaranteed or endorsed by the publisher.
References
1. Cui Y, Pan Y, Guo J, Wang D, Tong X, Wang Y, et al. The evolution, genomic epidemiology, and transmission dynamics of Tembusu virus. Viruses. (2022) 14:1236. doi: 10.3390/v14061236
2. Zhang W, Chen S, Mahalingam S, Wang M, Cheng A. An updated review of avian-origin Tembusu virus: a newly emerging avian Flavivirus. J Gen Virol. (2017) 98:2413–20. doi: 10.1099/jgv.0.000908
3. Huang Y, Chu X, Zhang Y, Yang S, Shi Y, Wu J, et al. Duck Tembusu virus infection causes testicular atrophy. Theriogenology. (2022) 188:52–62. doi: 10.1016/j.theriogenology.2022.05.012
4. Li L, Zhang Y, Dong J, Zhang J, Zhang C, Sun M, et al. The truncated E protein of DTMUV provide protection in young ducks. Vet Microbiol. (2020) 240:108508. doi: 10.1016/j.vetmic.2019.108508
5. Guo H, Cheng A, Zhang X, Pan Y, Wang M, Huang J, et al. DEF cell-derived exosomal miR-148a-5p promotes DTMUV replication by negative regulating TLR3 expression. Viruses. (2020) 12:94. doi: 10.3390/v12010094
6. Ninvilai P, Limcharoen B, Tunterak W, Prakairungnamthip D, Oraveerakul K, Banlunara W et al. Pathogenesis of thai duck Tembusu virus in cherry valley ducks: the effect of age on susceptibility to infection. Vet Microbiol. (2020) 243:108636. doi: 10.1016/j.vetmic.2020.108636
7. Pulmanausahakul R, Ketsuwan K, Jaimipuk T, Smith D R, Auewarakul P, Songserm T. Detection of antibodies to duck tembusu virus in human population with or without the history of contact with ducks. Transbound Emerg Dis. (2022) 69:870–3. doi: 10.1111/tbed.13998
8. Yuan S, Yao X, Yang H, Zhang Y, Liu H, Sun J, et al. Research note: genetic diversity of duck circoviruses circulating in partial areas of Guangdong province, southern China. Poult Sci. (2022) 101:102032. doi: 10.1016/j.psj.2022.102032
9. Hattermann K, Schmitt C. Cloning and sequencing of Duck circovirus (DuCV). Arch Virol. (2003) 148:2471–80. doi: 10.1007/s00705-003-0181-y
10. Wan C, Huang Y, Cheng L, Fu G, Shi S H, Chen H, et al. The development of a rapid SYBR Green I-based quantitative PCR for detection of Duck circovirus. Virol J. (2011) 8:465. doi: 10.1186/1743-422X-8-465
11. Li Z, Wang X, Zhang R, Chen J, Xia L, Lin S, et al. Evidence of possible vertical transmission of duck circovirus. Vet Microbiol. (2014) 174:229–32. doi: 10.1016/j.vetmic.2014.09.001
12. Wang Y, Zhang D, Bai CX, Guo X, Gao WH Li ML, et al. Molecular characteristics of a novel duck circovirus subtype 1d emerging in Anhui, China. Virus Res. (2021) 295:198216. doi: 10.1016/j.virusres.2020.198216
13. Tran GTH, Mai NT, Bui VN, Dao TD, Trinh DQ, Vu TTT, et al. Duck circovirus in northern Vietnam: genetic characterization and epidemiological analysis. Arch Virol. (2022) 167:1871–7. doi: 10.1007/s00705-022-05501-y
14. Hong YT, Kang M, Jang HK. Pathogenesis of duck circovirus genotype 1 in experimentally infected Pekin ducks. Poult Sci. (2018) 97:3050–7. doi: 10.3382/ps/pey177
15. Liao JY, Xiong WJ, Tang H, Xiao CT. Identification and characterization of a novel circovirus species in domestic laying ducks designated as duck circovirus 3 (DuCV3) from Hunan province, China. Vet Microbiol. (2022) 275:109598. doi: 10.1016/j.vetmic.2022.109598
16. Zhang H, Song X, Li T, Wang J, Xing B, Zhai X, et al. DDX1 from Cherry valley duck mediates signaling pathways and anti-NDRV activity. Vet Res. (2021) 52:9. doi: 10.1186/s13567-020-00889-4
17. Kong J, Shao G, Zhang Y, Wang J, Xie Z, Feng K, et al. Molecular characterization, complete genome sequencing, and pathogenicity of Novel Duck Reovirus from South Coastal Area in China. Poult Sci. (2023) 102:102776. doi: 10.1016/j.psj.2023.102776
18. Yu K, Ti J, Lu X, Pan L, Liu L, Gao Y, et al. Novel duck reovirus exhibits pathogenicity to specific pathogen-free chickens by the subcutaneous route. Sci Rep. (2021) 11:11769. doi: 10.1038/s41598-021-90979-w
19. Li Z, Cai Y, Liang G, El-Ashram S, Mei M, Huang W, et al. Detection of Novel duck reovirus (NDRV) using visual reverse transcription loop-mediated isothermal amplification (RT-LAMP). Sci Rep. (2018) 8:14039. doi: 10.1038/s41598-018-32473-4
20. Vogelstein B, Kinzler KW. Digital PCR. Proc Natl Acad Sci U S A. (1999) 96:9236–41. doi: 10.1073/pnas.96.16.9236
21. Quan PL, Sauzade M, Brouzes E. dPCR: a technology review. Sensors (Basel). (2018) 18:1271. doi: 10.3390/s18041271
22. Kuypers J, Jerome KR. Applications of digital PCR for clinical microbiology. J Clin Microbiol. (2017) 55:1621–8. doi: 10.1128/JCM.00211-17
23. Yu K, Sheng ZZ, Huang B, Ma X, Li Y, Yuan X, et al. Structural, antigenic, and evolutionary characterizations of the envelope protein of newly emerging Duck Tembusu Virus. PLoS ONE. (2013) 8:e71319. doi: 10.1371/journal.pone.0071319
24. Lv C, Li R, Liu X, Li N, Liu S. Pathogenicity comparison of duck Tembusu virus in different aged Cherry Valley breeding ducks. BMC Vet Res. (2019) 15:282. doi: 10.1186/s12917-019-2020-8
25. Zhu Y, Hu Z, Lv X, Huang R, Gu X, Zhang C, et al. A novel Tembusu virus isolated from goslings in China form a new subgenotype 211. Transbound Emerg Dis. (2022) 69:1782–93. doi: 10.1111/tbed.14155
26. Liu R, Chen C, Huang Y, Cheng L, Lu R, Fu G, et al. Microbiological identification and analysis of waterfowl livers collected from backyard farms in southern China. J Vet Med Sci. (2018) 80:667–71. doi: 10.1292/jvms.17-0452
27. Niu X, Wang H, Wei L, Zhang M, Yang J, Chen H, et al. Epidemiological investigation of H9 avian influenza virus, Newcastle disease virus, Tembusu virus, goose parvovirus and goose circovirus infection of geese in China. Transbound Emerg Dis. (2018) 65:e304–16. doi: 10.1111/tbed.12755
28. Liu C, Diao Y, Wang D, Chen H, Tang Y, Diao Y. Duck viral infection escalated the incidence of avian pathogenic Escherichia coli in China. Transbound Emerg Dis. (2019) 66:929–38. doi: 10.1111/tbed.13107
29. Liu H, Li LX, Sun WC, Shi N, Sun XT, Jin NY, et al. Molecular survey of duck circovirus infection in poultry in southern and southwestern China during 2018 and 2019. BMC Vet Res. (2020) 16:80. doi: 10.1186/s12917-020-02301-x
30. Zhang X, Jiang S, Wu J, Zhao Q, Sun Y, Kong Y, et al. An investigation of duck circovirus and co-infection in Cherry Valley ducks in Shandong Province, China. Vet Microbiol. (2009) 133:252–6. doi: 10.1016/j.vetmic.2008.07.005
31. Cha S, Song E, Kang M, Wei B, Seo H, Roh J, et al. Prevalence of duck circovirus infection of subclinical pekin ducks in South Korea. J Vet Med Sci. (2014) 76:597–9. doi: 10.1292/jvms.13-0447
32. Shen M, Gao P, Wang C, Li N, Zhang S, Jiang Y, et al. Pathogenicity of duck circovirus and fowl adenovirus serotype 4 co-infection in Cherry Valley ducks. Vet Microbiol. (2023) 279:109662. doi: 10.1016/j.vetmic.2023.109662
33. Niu X, Liu L, Han C, Li J, Zeng X. First findings of duck circovirus in migrating wild ducks in China. Vet Microbiol. (2018) 216:67–71. doi: 10.1016/j.vetmic.2018.02.007
34. Yang Y, Li L, Liu X, Jiang M, Zhao J, Li X, et al. Quantitative proteomic analysis of duck embryo fibroblasts infected with novel duck reovirus. Front Vet Sci. (2020) 7:577370. doi: 10.3389/fvets.2020.577370
35. Mao X, Liu C, Tong H, Chen Y, Liu K. Principles of digital PCR and its applications in current obstetrical and gynecological diseases. Am J Transl Res. (2019) 11:7209–22.
36. Zhang S, Li W, Liu X, Li X, Gao B, Diao Y, et al. A TaqMan-based real-time PCR assay for specific detection of novel duck reovirus in China. BMC Vet Res. (2020) 16:306. doi: 10.1186/s12917-020-02523-z
37. Zhang L, Jiang W, Zhang F, Li Y, Li J, Liang S, et al. Development of a dual-labeled, hydrolysis probe-based, real-time quantitative PCR assay for detection of both genotypes of duck circovirus-1 (DuCV-1) and DuCV-2. Virus Genes. (2021) 57:453–8. doi: 10.1007/s11262-021-01862-9
38. Li H, Wan C, Wang Z, Tan J, Tan M, Zeng Y, et al. Rapid diagnosis of duck Tembusu virus and goose astrovirus with TaqMan-based duplex real-time PCR. Front Microbiol. (2023) 14:1146241. doi: 10.3389/fmicb.2023.1146241
39. Zhang X, Yao M, Tang Z, Xu D, Luo Y, Gao Y, et al. Development and application of a triplex real-time PCR assay for simultaneous detection of avian influenza virus, Newcastle disease virus, and duck Tembusu virus. BMC Vet Res. (2020) 16:203. doi: 10.1186/s12917-020-02399-z
40. Yin YW, Xiong C, Shi KC, Xie SY, Long F, Li J, et al. Development and application of a multiplex qPCR assay for the detection of duck circovirus, duck Tembusu virus, Muscovy duck reovirus, and new duck reovirus. Virus Genes. (2023) 59:91–9. doi: 10.1007/s11262-022-01946-0
41. Zhao S, Lin H, Chen S, Yang M, Yan Q, Wen C, et al. Sensitive detection of Porcine circovirus-2 by droplet digital polymerase chain reaction. J Vet Diagn Invest. (2015) 27:784–8. doi: 10.1177/1040638715608358
42. Liu Y, Meng H, Shi L, Li L. Development of a droplet digital polymerase chain reaction for sensitive and simultaneous identification of porcine circovirus type 2 and 3. J Virol Methods. (2019) 270:34–7. doi: 10.1016/j.jviromet.2019.04.021
43. Long F, Chen Y, Shi K, Yin Y, Feng S, Si H. Development of a multiplex crystal digital RT-PCR for differential detection of classical, highly pathogenic, and NADC30-like porcine reproductive and respiratory syndrome virus. Animals (Basel). (2023) 13:594. doi: 10.3390/ani13040594
Keywords: digital PCR, coefficients of variation, duck Tembusu virus, duck circovirus, new duck reovirus
Citation: Yin Y, Xiong C, Shi K, Long F, Feng S, Qu S, Lu W, Huang M, Lin C, Sun W and Li Z (2023) Multiplex digital PCR: a superior technique to qPCR for the simultaneous detection of duck Tembusu virus, duck circovirus, and new duck reovirus. Front. Vet. Sci. 10:1222789. doi: 10.3389/fvets.2023.1222789
Received: 15 May 2023; Accepted: 24 July 2023;
Published: 10 August 2023.
Edited by:
Valentín Pérez, University of León, SpainReviewed by:
Guanliu Yu, Shandong Normal University, ChinaDongdong Yin, Anhui Academy of Agricultural Sciences (CAAS), China
Copyright © 2023 Yin, Xiong, Shi, Long, Feng, Qu, Lu, Huang, Lin, Sun and Li. This is an open-access article distributed under the terms of the Creative Commons Attribution License (CC BY). The use, distribution or reproduction in other forums is permitted, provided the original author(s) and the copyright owner(s) are credited and that the original publication in this journal is cited, in accordance with accepted academic practice. No use, distribution or reproduction is permitted which does not comply with these terms.
*Correspondence: Wenchao Sun, c3Vud2VuY2hhbzEzMSYjeDAwMDQwOzE2My5jb20=; Zongqiang Li, bGl6cTIwJiN4MDAwNDA7MTYzLmNvbQ==
†These authors have contributed equally to this work and share first authorship