- 1Department of Animal Science, Chungbuk National University, Cheongju, Republic of Korea
- 2Department of Poultry Science, University of Georgia (UGA), Athens, GA, United States
In order to make piglet diets more effective, it is necessary to investigate effective methods for breaking down xylan in cereal. The objective of this study was to determine the effects of dietary stimbiotic (STB) supplementation on growth performance, intestinal morphology, immune response and intestinal microbiota in weaned piglets. A total of 24 (Duroc × Yorkshire × Landrace) weaned pigs (initial body weight of 8.01 ± 0.38 kg and 28 ± 3 d old), were assigned to 4 treatments with 6 replicates per treatment. Pigs were housed in individual pens for 17 days, including 5 days adaption period and 12 days after the first Escherichia coli (E. coli) challenge. The experiment was conducted in a 2 × 2 factorial arrangement of treatments consisting of two levels of challenge (challenge and non-challenge) and two levels of STB (0 and 0.5 g/kg diet). Supplementations of STB 0.5 g/kg improved the gain to feed ratio (G:F) (P < 0.05) in piglets challenged with shiga toxigenic E. coli (STEC). STB supplementation decreased (P < 0.05) white blood cells, neutrophils, lymphocytes, and expression levels of tumor necrosis factor-alpha and interleukin-6. Supplementation of STB improved (P < 0.05) the lymphocytes and neutrophils in piglets challenged with STEC on 12 dpi. Supplementation of STB also improved (P < 0.05) the villus height to-crypt depth ratio of ileum in piglets challenged with STEC. Supplementation of STB increased (P < 0.05) the expression levels of claudin-1 of ileum. In genus level, supplementation of STB increased (P < 0.001) the abundance of Prevotella compared to non-supplementation of STB groups in pre-inoculation period. Also, supplementation of STB decreased (P < 0.05) the abundance of Faecalibacterium and Eubacterium_coprostanoligenes_group compared to non-supplementation of STB groups in post-inoculation period. In phylum level, supplementation of STB increased (P < 0.05) the abundance of Desulfobacterota and Fibrobacterota in pre-inoculation period. E. coli challenge increased the abundance of Fibrobacterota compared to non-challenged group in post-inoculation period. In conclusion, these findings indicated that STB supplementation could alleviate a decrease of the performance, immune response, and inflammatory response in piglets induced by the STEC challenge.
Introduction
Commonly, grains in feed contain variable amounts of non-starch polysaccharides (NSP), which can reduce absorption, decrease digestibility of nutrients, and increase digesta viscosity in the small intestines (1). Xylan, the most abundant fiber source in pig diets, is resistant to digestion by endogenous digestive enzymes (2). Additionally, xylan can result in gut leakage and inflammation by viscous digesta (3). To make piglet diets more effective, it is necessary to investigate effective methods for breaking down xylan into cereal.
Xylanase (XYL), a carbohydrase that can degrade NSP, has been used in the diets of monogastric animals to mitigate the growth performance (4). Zheng et al. (5) reported that xylanase supplementation reduced digesta viscosity and improved nutrient digestibility. According to Petry and Patience (6), supplementation of XYL improved growth performance and villus height in weaned piglets challenged with Escherichia coli (E. coli). Similarly, a mixture of XYL and protease supplementation mitigated the proliferation of coliforms in the ceca and improved the growth performance of the broilers (7).
Xylo-oligosaccharides (XOS) are derived from the hydrolysis of xylan and are made up of xylose monomers bonded together with β-(1, 4) linkages (8). In addition, XOS are considered prebiotics which are non-digestible dietary components that selectively fermented in the intestine (9). Such selective fermentation can change the activity of gut microbiome and promote short-chain fatty acid (SCFA) production (10).
Stimbiotic (STB), a complex of XYL and XOS, means “non-digestible but fermentable additive that obviously activates fiber-degrading microbiota to improve fiber fermentability at an insufficient amount” (11). Hence, STB has multi-function that reduces the antinutritive effects of NSP in feed and stimulates the microbiota to produce more SCFA (12). It may be possible to increase the fermentability of NSP by the supplementation of STB.
In the weaning period, piglets face new environments and experience intestinal morphological changes because of solid diet feeding (13). Post-weaning diarrhea (PWD) caused by these stress factors can lead to changes in gastrointestinal microbiology and immunology (14). Weaning stress has a negative impact on reduced feed intake, poor growth performance, and disease susceptibility (15). Especially, E. coli is the main cause of diarrhea, and gut microbiome is associated with diarrhea (16). For an experiment to be successful, it is crucial to develop a model that mimics an outbreak of PWD in a commercial setting. According to our previous studies (11, 14), we determined the optimal dosage of E. coli. Our previous study showed that supplementation of STB 0.5 g/kg and 1 g/kg improved gut health compared with non-supplementation of STB (11). However, supplementation of STB 0.5 g/kg showed a higher improvement in immune response such as pro-inflammatory cytokines compared with supplementation of STB 1 g/kg. However, we conducted the experiment and the effects of dietary STB supplementation of 0.5 g/kg on growth performance, intestinal health, and immune response in weaned piglets challenged with the PWD infection model. Therefore, we hypothesized that (1) experimental induction of PWD could increase the damage of intestinal mucosa and inflammatory response and (2) supplementation of STB 0.5 g/kg could reduce the antinutritive effects of NSP and provide beneficial bacteria which improve gut health and immunity. To test these hypotheses, we induced inflammation and gut damage through oral inoculation of E. coli and then investigated the effects of STB supplementation on gut health.
Materials and methods
Ethics approval and consent to participate
The protocol for this study was reviewed and approved by the Institutional Animal Care and Use Committee of Chungbuk National University, Cheongju, Korea (approval no. CBNUA-1697-22-01).
Bacterial strains, culture, and challenges
Shiga toxin-producing E. coli F18 was provided in stock form. The F18 E. coli expressed heat-labile toxin (LT) and Shiga toxin type 2e (stx2e). In total, 10 μl of thawed E. coli stock was inoculated into 10 ml of nutrient broth and cultured at 37°C for 24 h and then subcultured. Thereafter, the subcultured E. coli was smeared on MacConkey agar to confirm the bacterial enumeration. A final concentration of 1.2 × 1010 CFU/ml was used in this study.
Animals, experimental design, and diets
A total of 24 (Duroc × Yorkshire × Landrace) weaned pigs (initial body weight of 8.01 ± 0.38 kg and 28 ± 3 days old) were assigned to 4 treatments with 6 replicates per treatment. Pigs were housed in individual pens for 17 days, including 5 days of adaptation period and 12 days after the first E. coli challenge (d 0). The experiment was conducted in a 2 × 2 factorial arrangement of treatments consisting of two levels of challenge (challenge and non-challenge) and two levels of STB (0 and 0.5 g/kg diet). Corn and soybean meal basal diets were formulated to meet or exceed the nutrient requirements for the weaned piglets as recommended by NRC (Table 1) (17). STB used in this study was obtained by a commercial company (Eugene-Bio, Suwon, South Korea). The pigs were fed daily at 8:30 and 17:00 h and had ad libitum access to water. Feed residues were removed before the next meal and considered in the calculations. In the E. coli challenge treatments, all pigs were orally inoculated by dividing 10 ml of E. coli F18 for 3 consecutive days. Challenged piglets and non-challenged piglets were housed in a separate room. Strict biosecurity procedures were followed to avoid E. coli contamination of the non-challenged piglets.
Growth performance
All piglets were weighed every week during the experimental period, and feed consumption was recorded to calculate average daily gain (ADG), average daily feed intake (ADFI), and gain-to-feed ratio (G:F).
Diarrhea scores
The diarrhea scores were individually recorded at 08:00 and 17:00 h by the same person during the entire experimental period. The diarrhea score was scored using a method used by Zhao et al. (18). The diarrhea scores were assigned as follows: 0, normal feces; 1, soft feces; 2, mild diarrhea; and 3, severe diarrhea.
Nutrient digestibility
To estimate the digestibility, 0.2% chromium oxide (Cr2O3) was supplemented with diets as an indigestible marker. Pigs were fed diets mixed with chromium oxide for 4 consecutive days from days post-inoculation (DPI) 4 and 12, and fresh excreta samples were collected in that period. At the end of the experiment, fecal samples were stored at −20°C and dried at 70°C for 72 h and then ground to pass through a 1 mm screen. All analysis items (feed and fecal) were analyzed for DM and CP. The procedures utilized for the determination of dry matter (DM) and crude protein (CP) digestibility were conducted with the AOAC methods (19). Chromium was analyzed with an ultraviolet absorption spectrophotometer (UV-1201, Shimadzu, Kyoto, Japan). The digestibility was calculated using the following formula: digestibility (%) = [1–(Nf × Cd)/(Nd × Cf)] × 100, where Nf is the nutrient concentration in feces (% DM), Nd is the nutrient concentration in diet (% DM), Cd is the chromium concentration in diet (% DM), and Cf is the chromium concentration in feces (% DM).
Blood profile
Blood samples were obtained from the jugular vein of 6 pigs, each treatment at dpi 0, dpi 2, dpi 4, dpi 7, and dpi 12. At the time of collection, blood samples were collected into vacuum tubes containing K3EDTA for CBC analysis and non-heparinized tubes for serum analysis, respectively. After collection, blood samples were centrifuged (3,000 × g for 15 min at 4°C). The white blood cells (WBC), basophils, neutrophils, and lymphocyte levels in the whole blood were measured using an automatic blood analyzer (ADVIA 120, Bayer, NY, USA).
Morphological analysis of small intestine
At the end of the experiment (dpi 12), pigs were anesthetized with carbon dioxide gas after blood sampling and euthanized by exsanguination. Intestinal tissues of approximately 10 cm from the ileum (close to the ileocecal junction) were collected and fixed in 10% neutral buffered formalin (NBF; Sigma–Aldrich, St. Louis, MO, United States). After cutting the intestine sample, it was dehydrated and dealcoholized. The samples were, then, installed on slides, treated with paraffin, and stained with hematoxylin and eosin. Villus height (VH) and crypt depth (CD) were measured under the light microscope (OLYMPUS DP71, BX50F-3, Olympus Optical Co. Ltd., Tokyo, Japan). VH was determined by measuring the distance between the tip of the villi to the villus crypt junction, and CD was determined by measuring the distance between adjacent villi.
The hematoxylin-eosin-stained slides were also used for goblet cell counting. In crypts, goblet cells were counted in the five best-oriented crypts/intestinal tract, from crypt mouth to base (adjacent to submucosa). The number of goblet cells is expressed as the mean number per crypt per tract and mean number of goblet cells/100 μm of crypts (mean data of crypt length). This was determined in order to supply the number of cells/crypt (anatomo-functional unit), flanked by number of goblet cells/unit length of epithelium (linear density), that is more comparable with bibliographic data (Obj. 40X). The equation to determine the number of goblet cells/100 μm was: goblet cells/100 μm = number of goblet cells × 100/(crypt depth × 2) (20). In villi, goblet cells were counted in the five best-oriented villi/intestinal tract, from villus tip to base (adjacent to crypt mouth). The number of goblet cells is expressed as the mean number/villus per tract and the mean number of goblet cells/100 μm of villi epithelium (mean data of villus height).
Measurements of pro-inflammatory cytokine and immunoglobulin
The inflammatory biomarkers such as interleukin-6 (IL-6) and tumor necrosis factor α (TNF-α) were measured using commercially available ELISA kits, according to the manufacturer's instructions (R&D Systems, Minneapolis, MN). Immunoglobulin G (IgG) and immunoglobulin A (IgA) levels were gauged using an automatic biochemistry blood analyzer (Hitachi 747; Hitachi, Tokyo, Japan).
Expression of tight junction proteins
The intestinal sample stored at −80°C after sampling was homogenized and used for calprotectin and claudin-1 (CLDN-1) concentration analysis. The concentration of total protein was quantified using a Pierce BCA protein assay kit (#23225, Thermo Fisher Scientific, Waltham, MA, USA). After the homogenized intestinal sample was diluted to reach a working range of 20–2,000 μg/ml, the absorbance was measured at 562 nm. The total protein concentration was calculated as a standard curve and used to normalize the concentrations of calprotectin and CLDN-1. The relative protein expression of calprotectin and CLDN-1 was determined by using commercially available ELISA kits (Cat no. MBS707210, MBS025129; Mybiosource, San Diego, CA, USA). Homogenized intestinal samples were diluted to reach a working range of 0.312–20 ng/ml for calprotectin and 0.5–16 ng/ml for CLDN-1. Both absorbances were measured at 450 nm. The concentrations of calprotectin and CLDN-1 were calculated by the standard curve and described as ng/mg of protein.
16S metagenomic data analysis
Bacterial 16S rRNA sequencing data of the two different metagenomics sequencing methods were analyzed using QIIME2 next-generation microbiome bioinformatics pipeline for comparative metagenomics study. The samples were sent to Sanigen (Anyang, South Korea) for microbial sequencing using the 16s rRNA technique. All raw input data were transformed in the form of QIIME2 artifacts, which contain information about the data types and sources for the downstream processing. From raw sequence data, the amplicon sequence variants (ASVs) were obtained using the Divisive Amplicon Denoising Algorithm 2 (DADA2) within QIIME 2 plugin, which detected and corrected amplicon errors and filtered out the potential base error and chimeric sequences (21, 22). The relative classification frequency table represented differential abundance tests at specific taxonomic levels was created using collapse and feature-table within the QIIME2 plugins. The “diversity” QIIME2 plugin was used to estimate alpha diversity measurements and plots using the R bioinformatics packages. This microbial diversity analysis pipeline was designed to use the ASV table (a higher resolution analog than the traditional OTU table) of the ASV picking step as necessary input data. Analyzing the differences in species richness and evenness scores considering the sampling depth was measured using the observed OTUs and Chao1, Shannon, and Simpson indices. Each index estimates the V3-V4 hypervariable region of the bacterial 16S rRNA gene. In addition, a difference in the relative abundance was analyzed by comparing the average bacterial proportion and composition investigated in each taxonomic ranking. Additionally, according to the different amplicon regions, the bacterial classification accuracy was cross-checked by comparing the taxonomy matching rate of each ASV taxonomy and NCBI bacterial reference genome database at the phylum and genus levels.
Statistical analysis
Statistical analyses and graph construction were performed using JMP Pro 16 (SAS Institute Inc., Cary, NC, United States) and GraphPad Prism (Version 9.1.0; GraphPad Software, San Diego, CA), respectively. Parametric data (growth performance, ileal morphology, blood profile, cytokine level, and TJ proteins) were submitted to two-way ANOVA using the Standard Least Squares model. The statistical model included the effect of the E. coli challenge (chal -, chal +), the effect of STB supplementation (0, 0.5 g/kg), and the interaction between E. coli and STB, and initial body weight at the start of the trial (d 0) was also included as a covariate. The richness and alpha diversity were calculated with raw counts based on Shannon estimators. For quantitative beta diversity measurement, each treatment group was placed as the control group, and treatment groups were compared by using PROC MIXED with Dunnett's post-hoc test. Non-parametric data (diarrhea score) were analyzed using contingency analysis to test the relationship between categorical variables (scores) and the different combinations tested in this study. A chi-square test was performed to determine if the different combinations had an effect on the categorical variables, repartition with significance accepted at P < 0.05.
Results
Growth performance
The effects of STB supplementation on the growth performance of piglets are presented in Table 2. There was an interaction (P < 0.05) between the supplementation of STB and E. coli challenge in BW, ADG, ADFI, and G:F. Piglets supplemented STB5 with E. coli challenge had higher BW on 7 dpi (P = 0.005) and final (P = 0.002) compared with piglets fed STB0 with E. coli challenge.
Incidence of diarrhea
An overview of the incidence of diarrhea is shown in Figure 1. After the E. coli challenge, there were differences in diarrhea scores from 1 to 7 dpi. E. coli challenge increased (P = 0.0004) the average diarrhea scores compared with the non-challenged group. Supplementation of STB5 decreased (P < 0.001) the average diarrhea scores compared with supplementation of STB0. There was an interaction between supplementation of STB and E. coli challenge in diarrhea score on days 0 to 12.
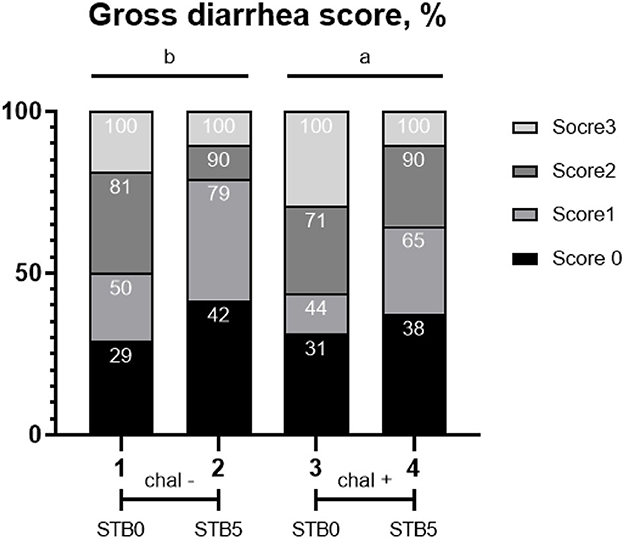
Figure 1. Effects of STB in weaned piglets challenged with E. coli on diarrhea score. χ2 = 19.571, P = 0.0208. Numbers inside the bar indicate the percentage of score out of total (100%) as shown in the legend. a,bMean scores followed by different superscripts in the bar graph indicate statistical significance by Student's t-test (P < 0.05).
Nutrient digestibility
E. coli challenge decreased the CP digestibility on 1w and 2w compared with the non-challenged groups (Table 3). There was an interaction between STB and E. coli challenge in digestibility on 2w. Piglets supplemented with STB5 with E. coli challenge had higher digestibility of CP, DM, and GE on 2w compared with piglets supplemented with STB0 with E. coli challenge.
Blood profile
E. coli challenge increased (P < 0.05) WBC and neutrophils and decreased (P < 0.05) lymphocytes compared with the non-challenged group during the post-inoculation period (Table 4). The piglets fed STB5 had improved (P < 0.05) neutrophils and lymphocytes in 12 dpi compared with STB0. There was an interaction between STB and E. coli challenge in WBC, neutrophils, and lymphocytes. Piglets supplemented STB5 with E. coli challenge improved WBC (P = 0.024), neutrophils (P = 0.002), and lymphocytes (P = 0.002) compared with piglets fed STB0 with E. coli challenge on 12 dpi.
Measurements of pro-inflammatory cytokine
E. coli challenge increased (P < 0.05) TNF-α and IL-6 and decreased (P < 0.05) IgG compared with the non-challenged group during the post-inoculation period (Table 5). Supplementation of STB5 improved (P < 0.05) TNF-α on 2 and 12 dpi compared with STB0. In addition, supplementation of STB5 improved (P < 0.05) IL-6 on 4, 7, and 12 dpi compared with STB0. There was an interaction between STB and E. coli challenge in TNF- α and IL-6. Piglets supplemented with STB5 with E. coli challenge improved (P < 0.05) IL-6 on 4, 7, and 12 dpi compared with piglets fed STB0 with E. coli challenge. In addition, piglets supplemented STB5 with E. coli challenge improved (P < 0.05) TNF-α compared with piglets fed STB0 with E. coli challenge on 12 dpi.
Morphological analysis of the small intestine
There was no interaction between supplementation of STB and E. coli challenge in villus height, crypt depth, and height-to-depth ratio. E. coli challenge decreased (P = 0.021) villus height of the ileum (Figure 2, Table 6). Supplementation of STB increased (P > 0.05) VH and HDR. However, there was no difference between E. coli and supplementation of STB in CD. There was no interaction between supplementation of STB and E. coli challenge in counts of goblet cells. E. coli challenge increased (P = 0.005) the counts of goblet cells in the villus.
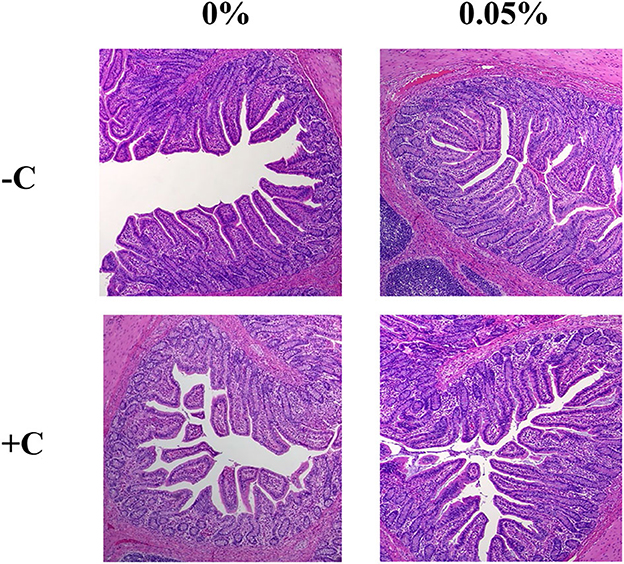
Figure 2. Histological analysis of the intestinal morphology of weaned piglets. Figures display the morphology of the ileum tissue from pigs in four dietary treatments.
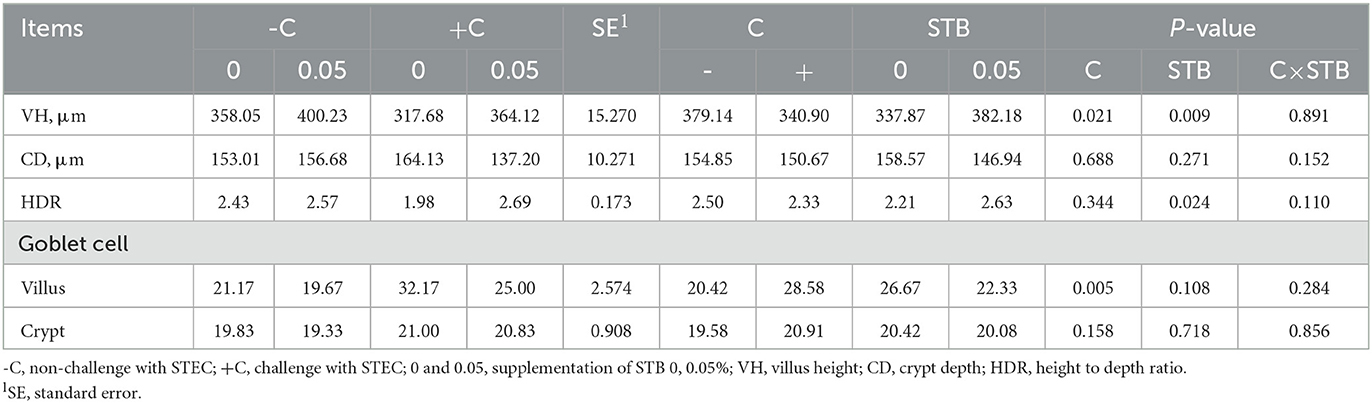
Table 6. Effects of stimbiotic supplementation on villus height and counts of goblet cell in pigs challenged with STEC.
Expression of tight junction proteins
There was no interaction between the supplementation of STB and E. coli challenge in CLDN-1 and calprotectin (Figure 3). E. coli challenge downregulated (P < 0.05) the expression of CLDN-1 while supplementation of STB upregulated (P < 0.05) the expression of CLDN-1. In addition, the E. coli challenge upregulated (P < 0.001) the expression of calprotectin compared with the non-challenged group. However, supplementation of STB did not affect (P > 0.05) the expression of calprotectin.
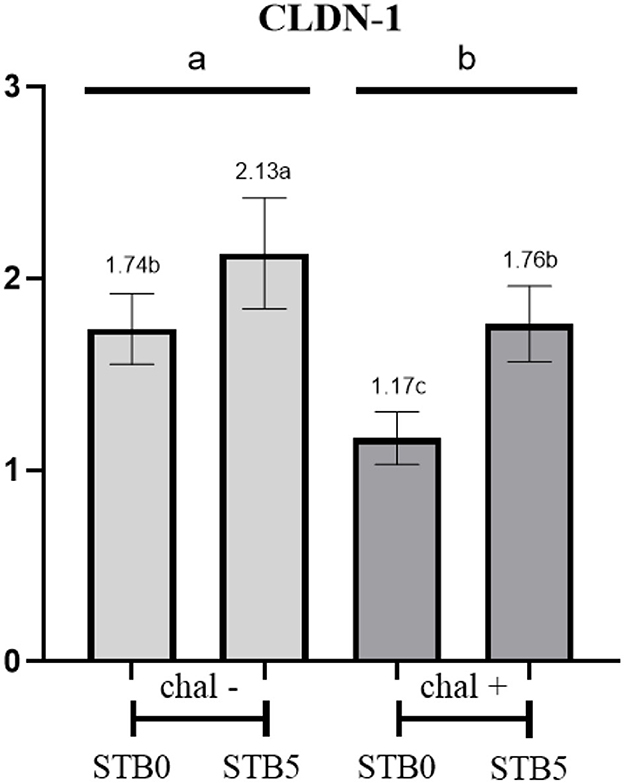
Figure 3. Effects of STB in weaned piglets challenged with E. coli on CLDN-1. a,b,cMean scores followed by different superscripts in the bar graph indicates statistical significance by Student's t-test (P < 0.05).
Alpha diversity of the fecal microbiome
No differences were observed in the alpha diversity parameters including Chao 1, Simpson, and Shannon indices on d 0 and dpi 12.
Beta diversity of the fecal microbiome
No differences were observed in unweighted and weighted unifrac distance to each treatment both on d 0 and dpi 12 (P < 0.05; Figures 4–6).
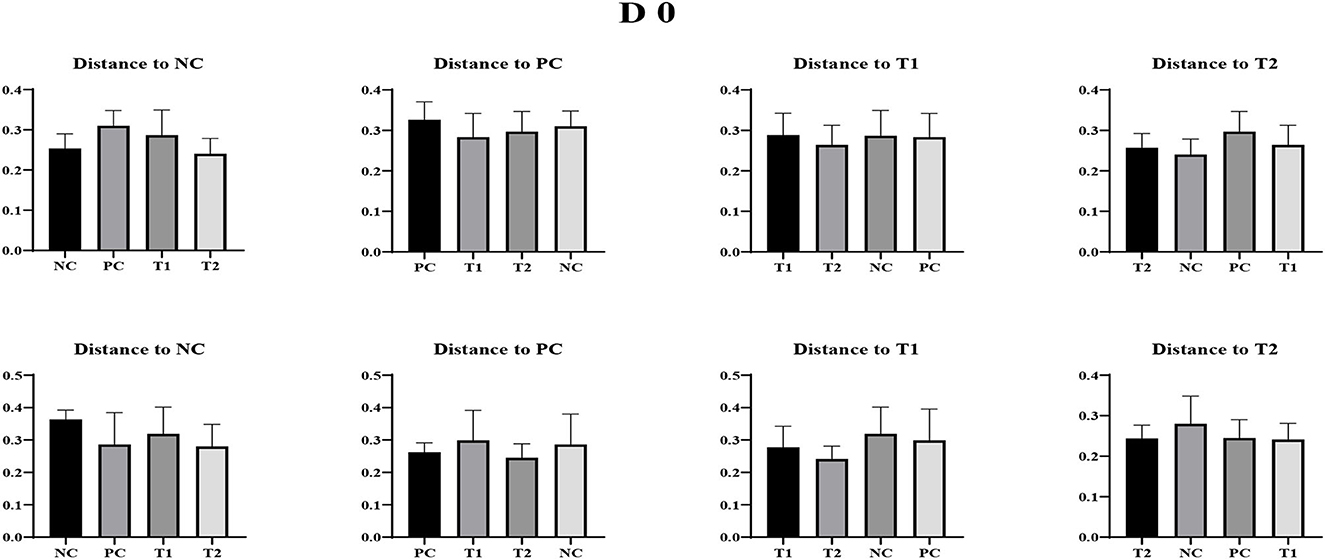
Figure 4. Weighted Unifrac measurement in negative control (NC): basal diet; positive control (PC): NC + E. coli challenge; treatment 1 (T1): NC + stimbiotic 0.5 g/kg; treatment 2 (T2): PC + stimbiotic 0.5 g/kg. Each treatment group was placed as the control group, and treatment groups were compared by using one-way PROC MIXED with Dunnett's post-hoc test.
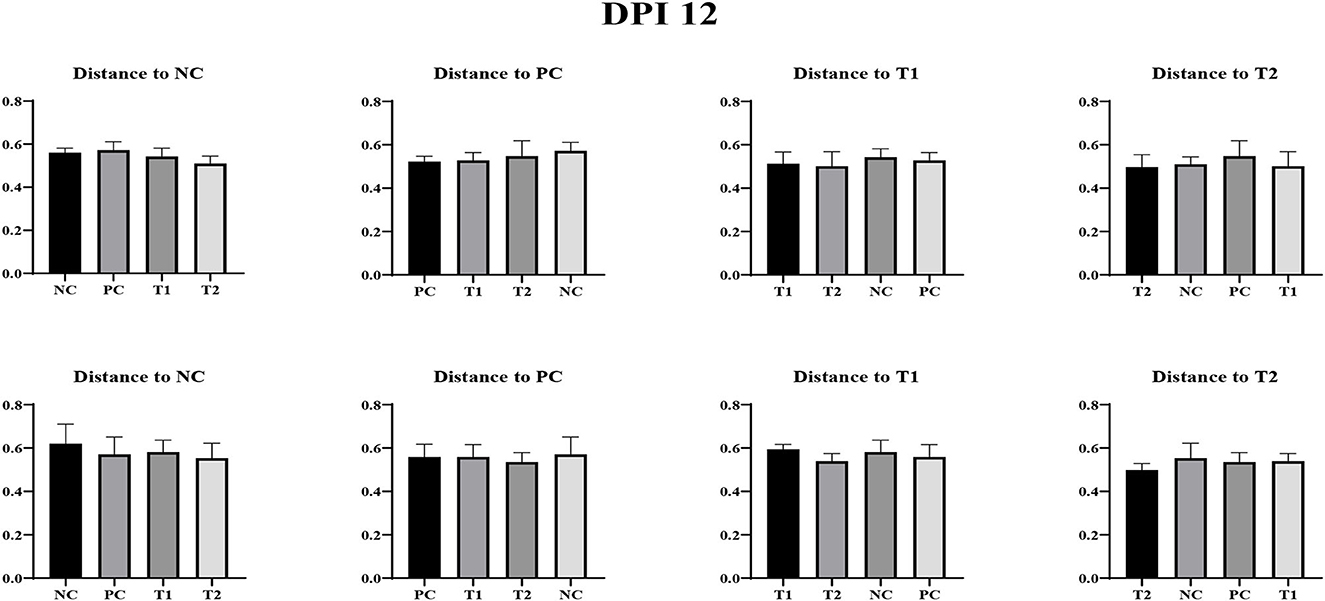
Figure 5. Unweighted unifrac measurement in negative control (NC): basal diet; positive control (PC): NC + E. coli challenge; treatment 1 (T1): NC + stimbiotic 0.5 g/kg; treatment 2 (T2): PC + stimbiotic 0.5 g/kg. Each treatment group was placed as the control group, and treatment groups were compared by using one-way PROC MIXED with Dunnett's post-hoc test.
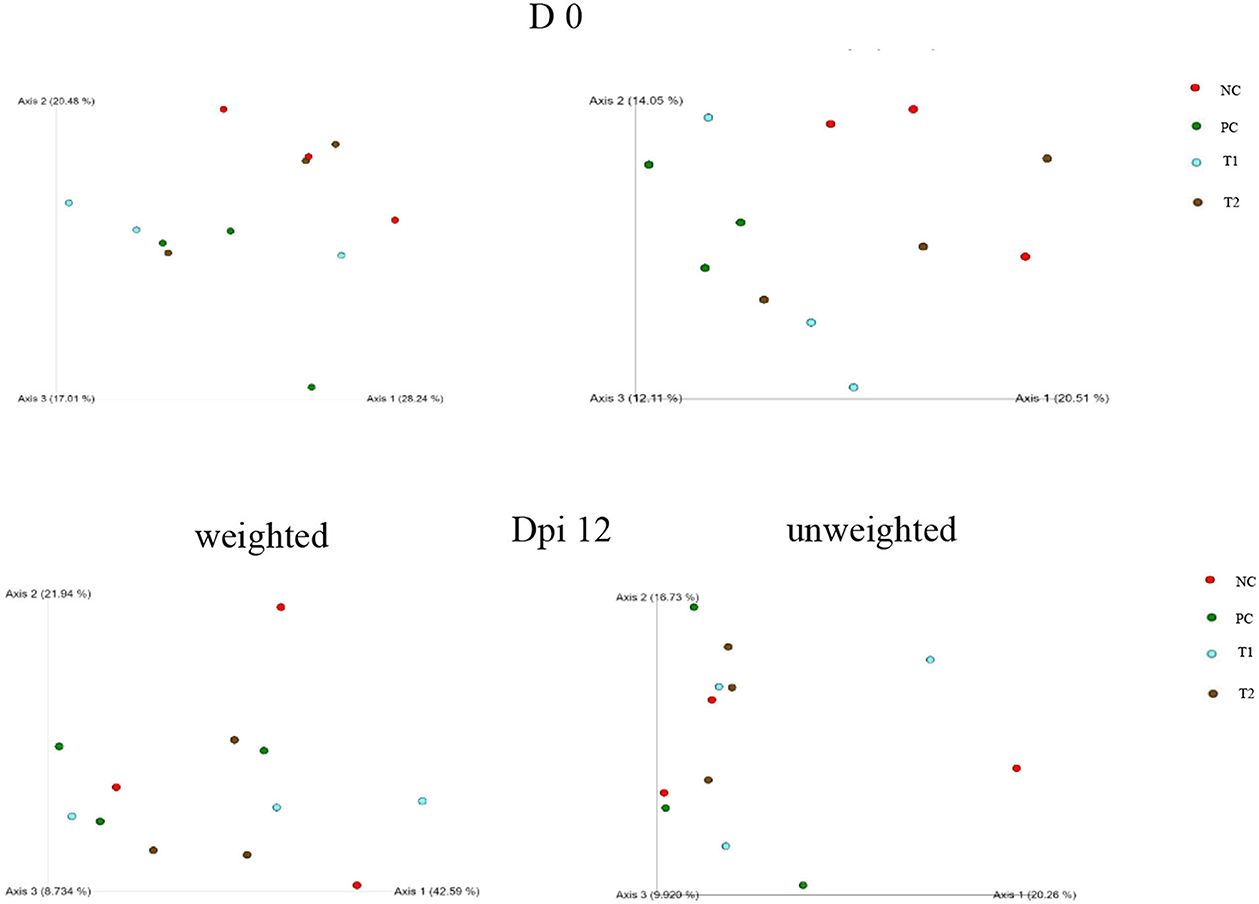
Figure 6. Visualized beta diversity indices including unweighted and weighted emperor in negative control (NC): basal diet; positive control (PC): NC + E. coli challenge; treatment 1 (T1): NC + stimbiotic 0.5 g/kg; treatment 2 (T2): PC + stimbiotic 0.5 g/kg.
Relative abundance
At the genus level, supplementation of STB increased (P < 0.001) the abundance of Prevotella compared with non-supplemented STB groups in the pre-inoculation period (Table 7; Figure 7). In addition, supplementation of STB decreased (P < 0.05) the abundance of the Faecalibacterium and Eubacterium_coprostanoligenes_group compared with the non-supplemented STB groups in the post-inoculation period. E. coli challenge decreased (P < 0.05) the abundance of Clostridium_sensu_stricto_1 and Faecalibacterium compared with the non-challenged groups in the post-inoculation period. There was an interaction between the supplementation of STB and E. coli challenge in the abundance of Muribaculaceae and Faecalibacterium in the post-inoculation period. Piglets supplemented STB5 with E. coli challenge decreased (P < 0.05) the abundance of Muribaculaceae and Faecalibacterium compared with piglets fed STB0 with E. coli challenge.
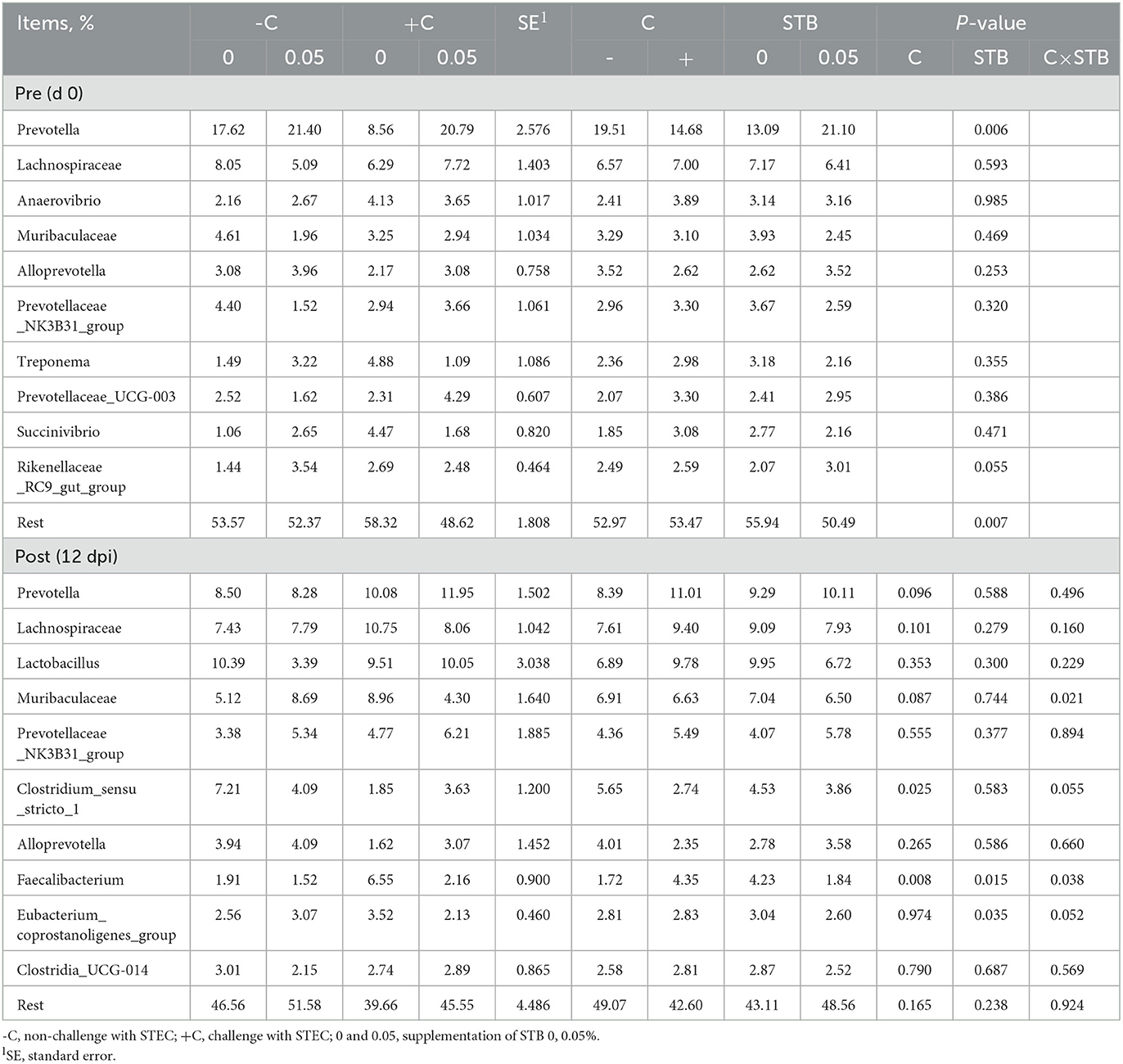
Table 7. Relative abundance of fecal microbiota at the genus level in pigs challenged with STEC on d 0 and 12 dpi and fed diets supplemented with stimbiotic.
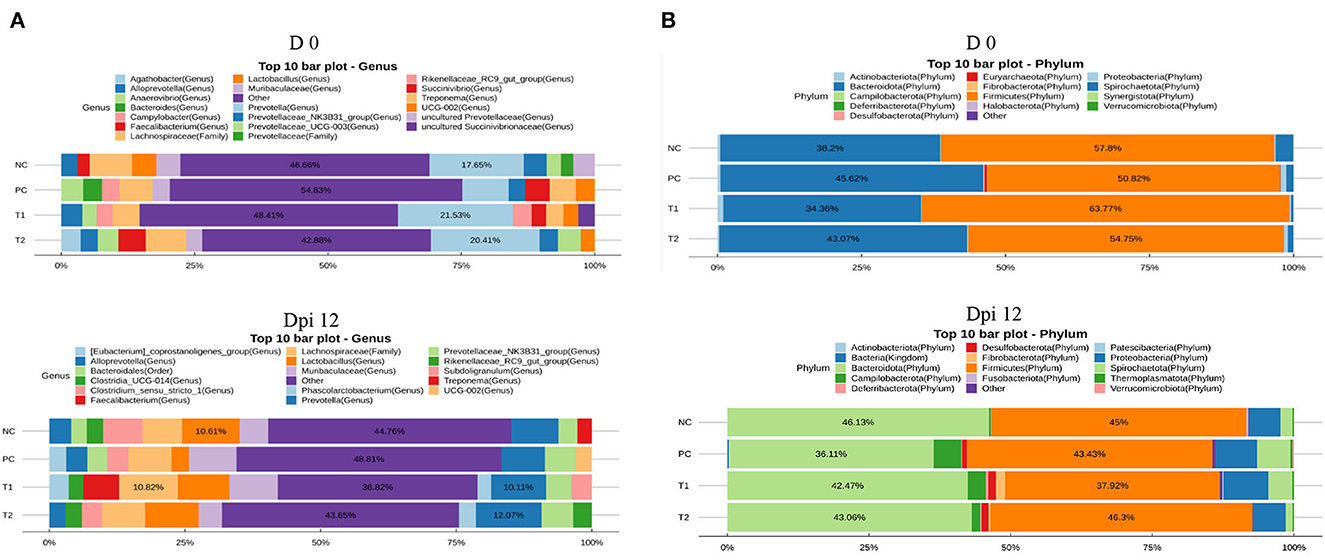
Figure 7. (A) 16S rRNA gene analysis revealed the relative abundance of fecal bacterial community structure at the genus level in piglets challenged with E. coli in negative control (NC): basal diet; positive control (PC): NC + E. coli challenge; treatment 1 (T1): NC + stimbiotic 0.5 g/kg; treatment 2 (T2): PC + stimbiotic 0.5 g/kg. (B) 16S rRNA gene analysis revealed the relative abundance of fecal bacterial community structure at the phylum level in piglets challenged with E. coli in negative control (NC): basal diet; positive control (PC): NC + E. coli challenge; treatment 1 (T1): NC + stimbiotic 0.5 g/kg; treatment 2 (T2): PC + stimbiotic 0.5 g/kg.
In the phylum level, supplementation of STB increased (P < 0.05) the abundance of Desulfobacterota and Fibrobacterota in the pre-inoculation period (Figure 7, Table 8). However, supplementation of STB decreased (P = 0.040) the abundance of Fibrobacterota compared with the non-supplemented group in the post-inoculation period. E. coli challenge increased the abundance of Fibrobacterota compared with the non-challenged group in the post-inoculation period. There was an interaction between the supplementation of STB and E. coli challenge in the abundance of Fibrobacterota. Piglets supplemented STB5 with E. coli challenge decreased (P = 0.010) the abundance of Fibrobacterota compared with piglets supplemented STB0 with E. coli challenge.
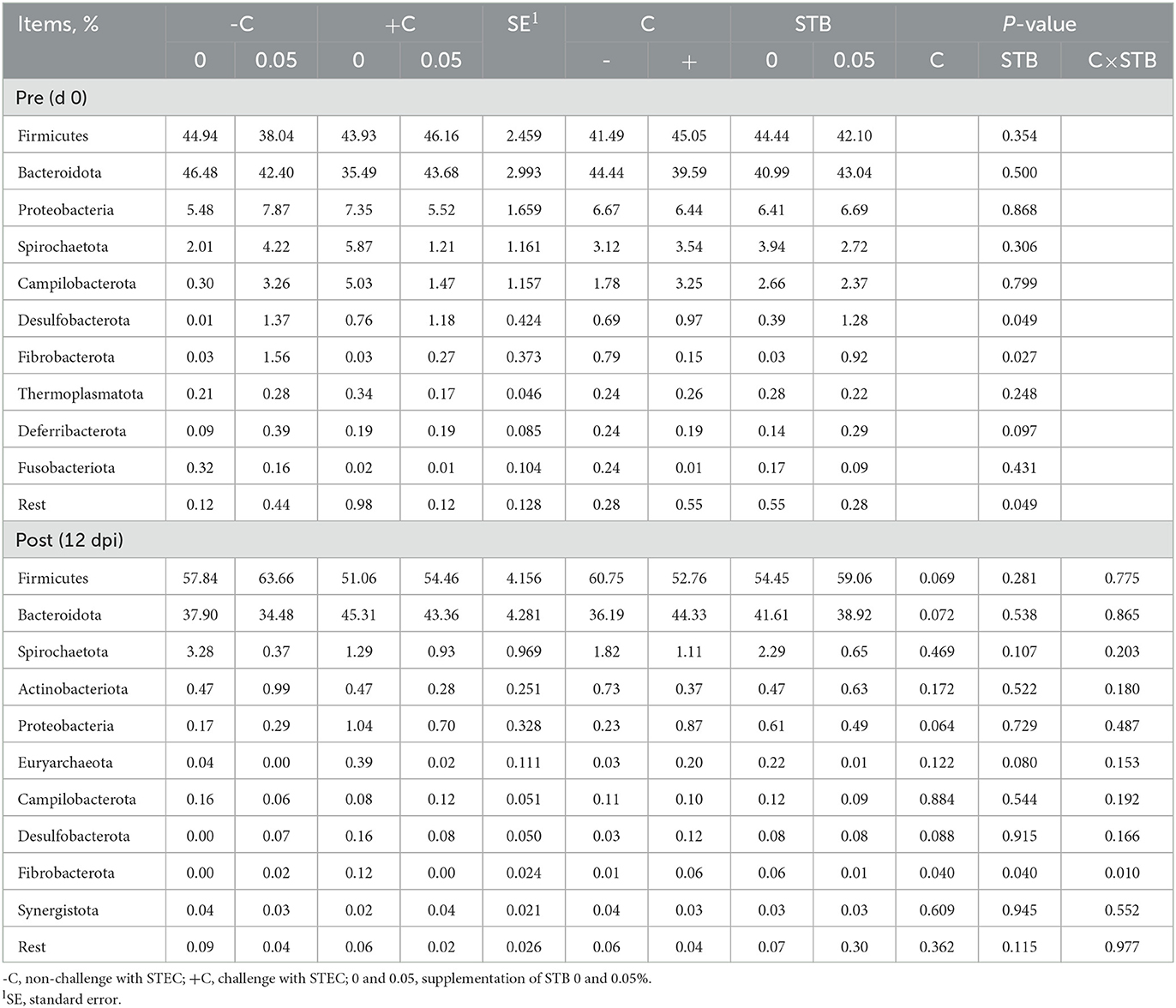
Table 8. Relative abundance of fecal microbiota at the phylum level in pigs challenged with STEC on d 0 and 12 dpi and fed diets supplemented with stimbiotic.
Discussion
Weaned piglets are commonly affected by PWD, which decreases their growth performance and increases their mortality (23). E. coli F18 is a primary pathogen associated with PWD in weaned piglets (24). It is known that E. coli F18 attaches to a specific receptor on the epithelium of the pig's intestinal tract through their fimbriae (25). As a result of colonization of the gut, toxins are produced, causing diarrhea (26). In many research facilities, experimental induction of PWD has been used to test the efficacy of functional additives under commercial PWD conditions (27–29). Furthermore, these studies have evaluated the gut health and immune response in weaned piglets challenged with E. coli.
The results obtained from this study showed that supplementation of 0.5 g/kg of STB could mitigate growth performance, diarrhea rate, intestinal morphology, and index of inflammation of weaned piglets challenged with E. coli infection. These results were consistent with other types of research, indicating an improved immune response after supplementation of STB (11, 30).
In the current study, F18 E. coli infection was accomplished in agreement with our previous studies using an E. coli challenge model in weaned piglets (9, 12). According to Kim et al. (31), heat-labile toxin and Shiga toxin induce gut permeability and inflammation, which, in turn, increase cytokines.
The integrity of the intestinal barrier is strictly regulated by Tight junction (TJ) proteins including CLDN, occludin, and zonula occludin which are continuously threatened by pro-inflammatory stimuli (32). Especially, CLDN-1, a representative TJ protein in mammals, enhances gut barriers to stop the loss of electrolytes (33). As a marker of neutrophilic inflammation in the gut, calprotectin concentrations are associated with the histological activity of inflammatory bowel disease (34). In the present study, supplementation of STB5 decreased the gross diarrhea incidence in E. coli-challenged piglets. This result is similar to those of previous studies showing that supplementing carbohydrase and XOS could alleviate diarrhea incidence in pigs (35, 36). It would be possible that increased expression of CLDN-1 in ileal mucosa fortified the intestinal barrier function in pigs fed STB infected with E. coli. TNF-α is a crucial pro-inflammatory cytokine in reaction to an infection by bacteria which is produced by neutrophils (31). IL-6 is also a pro-inflammatory cytokine expressed widely across vertebrates and plays multiple physiological roles involved in inflammation (37). TNF-α and IL-6 are served as biological indicators of intestinal inflammation in pigs (34, 38). Our observation indicated that supplementation of STB decreased the concentration of TNF-α and IL-6 in piglets infected with E. coli. Similar research reported that feeding STB improved TJ proteins between epithelial cells and reduced the production of pro-inflammatory cytokines such as TNF-α and IL-6 (39). Serum IgG and IgA are known to play an important role in humoral immunity.
The immune system depends on WBC, which includes neutrophils, lymphocytes, basophils, monocytes, and eosinophils, to control infections in the body, and the count of WBC is considered a marker of infection (40). The primary line of protection against bacterial infection is provided by neutrophils, and lymphocytes provide particular cellular and humoral immune responses (41). In the present study, E. coli infection increased counts of WBC and neutrophils, but supplementation of STB decreased the count of WBC and neutrophils in piglets infected with E. coli. In addition, the ratio of neutrophils to lymphocytes as a biomarker of inflammation was increased by E. coli infection. Similarly, the previous study reported that supplementation of mannan-oligosaccharide (MOS) decreased the neutrophils in broilers (42).
VH and CD are markers of enterocyte proliferation and villus damage (43). Shorter VH and deeper CD may indicate the presence of toxins (44). The villus contains enterocytes, goblet cells, and enteroendocrine cells that line the space, and the crypt contains undifferentiated cells and a subset of differentiated secretory cells (5). In our current study, E. coli infection decreased the VH, but supplementation of STB increased the VH, VH:CD, and goblet cells in the villi of piglets infected with E. coli. It has been reported that supplementation of MOS increased the villus height in weaned piglets (45). However, our results showed that supplementation of STB did not affect ileal CD. According to Luise et al. (46), XYL supplementation did not affect jejunal CD in weaned piglets which are genetically susceptible to ETEC. Zhang et al. (47) reported that the morphology of intestinal villi is tightly associated with the absorption of nutrients. Consistent with the results of the morphology of the small intestine, the digestibility of CP was also decreased by E. coli infection. There was an interaction effect between STB and E. coli infection. These findings support that supplementation of STB might mitigate inflammation and improve CP digestibility in E. coli-infected piglets.
Pathogenic challenges impair pig intestinal integrity by disturbing intestine microbial balance (48, 49). At the phylum level, Fibrobacterota is known for degrading lignocellulosic materials in the gut (47). Our study indicated that supplementation of STB increased the relative abundance of Fibrobacterota in the post-inoculation period. Desulfobacterota is associated with inflammation and increased relative abundance of Desulfobacterota, reducing VH and epithelial cells and downregulating the expression of TJ proteins (50). In the current study, the relative abundance of Desulfobacterota increased after E. coli infection. Similar research indicated that the abundance of Desulfobacterota is increased in broilers challenged with C.perfrigens (51).
At the genus level, Prevotella is associated with the production of acetate and butyrate in the small intestine (52). In the current study, supplementation of STB increased the relative abundance of Prevotella. Similar research indicated that the fermentation of xylan leads to the increased production of short-chain fatty acids such as butyrate (53).
Conclusion
The results of this study support the hypothesis that supplementation of STB is capable of alleviating the growth performance and intestinal morphology, immune response, and gut microbiota in weaned piglets infected with E. coli. Our results supported that STB supplementation might increase the fermentability of NSP and reduce the antinutritive effects of NSP. Therefore, STB could be used as an antidiarrheal growth stimulator in weaned piglets.
Data availability statement
The datasets presented in this study can be found in online repositories. The names of the repository/repositories and accession number(s) can be found below: https://www.ncbi.nlm.nih.gov/, SUB12953593.
Ethics statement
The animal study was reviewed and approved by Institutional Animal Care and Use Committee of Chungbuk National University.
Author contributions
DS, WK, and JL conducted the experiment and wrote the manuscript. HO, SC, JA, HC, SP, and KJ helped to conduct animal trial and laboratory work and helped to revise the manuscript. JC was the principal investigator and wrote the last version of the manuscript. All authors read and approved the final manuscript.
Funding
This study was supported by the National Research Foundation of Korea (NRF) grant funded by the Korean government (MSIT) (No. NRF-2021R1I1A3051928).
Conflict of interest
The authors declare that the research was conducted in the absence of any commercial or financial relationships that could be construed as a potential conflict of interest.
Publisher's note
All claims expressed in this article are solely those of the authors and do not necessarily represent those of their affiliated organizations, or those of the publisher, the editors and the reviewers. Any product that may be evaluated in this article, or claim that may be made by its manufacturer, is not guaranteed or endorsed by the publisher.
References
1. Lærke H, Arent S, Dalsgaard S, Bach Knudsen K. Effect of xylanases on ileal viscosity, intestinal fiber modification, and apparent ileal fiber and nutrient digestibility of rye and wheat in growing pigs. J Anim Sci. (2015) 93:4323–35. doi: 10.2527/jas.2015-9096
2. Wang W, Zheng D, Zhang Z, Ye H, Cao Q, Zhang C, et al. Efficacy of combination of endo-xylanase and xylan-debranching enzymes in improving cereal bran utilization in piglet diet. Animal Bioscience. (2022) 35:1733. doi: 10.5713/ab.21.0534
3. Baker JT, Duarte ME, Holanda DM, Kim SW. Friend or Foe? Impacts of dietary xylans, xylooligosaccharides, and xylanases on intestinal health and growth performance of monogastric animals. Animals. (2021) 11:609. doi: 10.3390/ani11030609
4. Cozannet P, Kidd MT, Neto RM, Geraert P-A. Next-generation non-starch polysaccharide-degrading, multi-carbohydrase complex rich in xylanase and arabinofuranosidase to enhance broiler feed digestibility. Poult Sci. (2017) 96:2743–50. doi: 10.3382/ps/pex084
5. Zheng L, Duarte ME, Sevarolli Loftus A, Kim SW. Intestinal health of pigs upon weaning: Challenges and nutritional intervention. Front Vet Sci. (2021) 8:628258. doi: 10.3389/fvets.2021.628258
6. Petry AL, Patience JF. Xylanase supplementation in corn-based swine diets: a review with emphasis on potential mechanisms of action. J Animal Sci. (2020) 98:skaa318. doi: 10.1093/jas/skaa318
7. Barekatain MR, Antipatis C, Rodgers N, Walkden-Brown SW, Iji PA, Choct M. Evaluation of high dietary inclusion of distillers dried grains with solubles and supplementation of protease and xylanase in the diets of broiler chickens under necrotic enteritis challenge. Poult Sci. (2013) 92:1579–94. doi: 10.3382/ps.2012-02786
8. Pang J, Zhou X, Ye H, Wu Y, Wang Z, Lu D, et al. The high level of xylooligosaccharides improves growth performance in weaned piglets by increasing antioxidant activity, enhancing immune function, and modulating gut microbiota. Front Nutr. (2021) 3:8. doi: 10.3389/fnut.2021.764556
9. Wang X, Xiao K, Yu C, Wang L, Liang T, Zhu H, et al. Xylooligosaccharide attenuates lipopolysaccharide-induced intestinal injury in piglets via suppressing inflammation and modulating cecal microbial communities. Anim Nutr. (2021) 7:609–20. doi: 10.1016/j.aninu.2020.11.008
10. Tang S, Chen Y, Deng F, Yan X, Zhong R, Meng Q, et al. Xylooligosaccharide-mediated gut microbiota enhances gut barrier and modulates gut immunity associated with alterations of biological processes in a pig model. Carbohydr Polym. (2022) 294:119776. doi: 10.1016/j.carbpol.2022.119776
11. Song D, Lee J, Kwak W, Song M, Oh H, Kim Y, et al. Stimbiotic supplementation alleviates poor performance and gut integrity in weaned piglets induced by challenge with E. coli. Animals. (2022) 12:1799. doi: 10.3390/ani12141799
12. Morgan NK, Gomes GA, Kim JC. Comparing the efficacy of stimbiotic and a combination of xylanase and beta-glucanase, in broilers fed wheat-barley based diets with high or low AME. Poult Sci. (2021) 100:101383. doi: 10.1016/j.psj.2021.101383
13. Oh HJ, Kim MH, Song MH, Lee JH, Kim YJ, Chang SY, et al. Effects of replacing medical zinc oxide with different ratios of inorganic. organic zinc or reducing crude protein diet with mixed feed additives in weaned piglet diets. Animals. (2021) 11:3132. doi: 10.3390/ani11113132
14. Chang SY, Song MH, Lee JH, Oh HJ, Kim YJ, An JW, et al. Phytogenic feed additives alleviate pathogenic Escherichia coli-induced intestinal damage through improving barrier integrity and inhibiting inflammation in weaned pigs. J Anim Sci Biotechnol. (2022) 13:1–12. doi: 10.1186/s40104-022-00750-y
15. Xiong X, Tan B, Song M, Ji P, Kim K, Yin Y, et al. Nutritional intervention for the intestinal development and health of weaned pigs. Front Vet Sci. (2019) 6:46. doi: 10.3389/fvets.2019.00046
16. Peng S-S, Li Y, Chen Q, Hu Q, He Y, Che L, Jiang PP. Intestinal and mucosal microbiome response to oral challenge of enterotoxigenic escherichia coli in weaned pigs. Pathog. (2022) 11:160. doi: 10.3390/pathogens11020160
18. Zhao PY, Jung JH, Kim IH. Effect of mannan oligosaccharides and fructan on growth performance, nutrient digestibility, blood profile, and diarrhea score in weanling pigs. J Anim Sci. (2012) 90:833–9. doi: 10.2527/jas.2011-3921
19. AOAC International. Official Methods of Analysis of AOAC int. 18th ed. Rev. 2nd ed. In:Hortwitz W and Latimer Jr GW, , editors. Gaithersburg, MD: AOAC International (2007).
20. Tugnoli B, Piva A, Sarli G, Grilli E. Tributyrin differentially regulates inflammatory markers and modulates goblet cells number along the intestinal tract segments of weaning pigs. Livest Sci. (2020) 234:103996. doi: 10.1016/j.livsci.2020.103996
21. Bolyen E, Rideout JR, Dillon MR, Bokulich NA, Abnet CC, Al-Ghalith GA, et al. Reproducible, interactive, scalable, and extensible microbiome data science using QIIME 2. Nat Biotechnol. (2019) 37:852–7. doi: 10.1038/s41587-019-0209-9
22. Callahan BJ, McMurdie PJ, Rosen MJ, Han AW, Johnson AJA, Holmes SP. DADA2. High-resolution sample inference from Illumina amplicon data. Nat Methods. (2016) 13:581–3. doi: 10.1038/nmeth.3869
23. Almeida JAS, Liu Y, Song M, Lee JJ, Gaskins HR, Maddox CW, et al. Escherichia coli challenge and one type of smectite alter intestinal barrier of pigs. J Anim Sci Biotechnol. (2013) 4:1–8. doi: 10.1186/2049-1891-4-52
24. Luise D, Spinelli E, Correa F, Salvarani C, Bosi P, Trevisi P. Effects of E. coli bivalent vaccine and of host genetic susceptibility to E coli on the growth performance and faecal microbial profile of weaned pigs. Livest Sci. (2020) 241:104247. doi: 10.1016/j.livsci.2020.104247
25. Coddens A, Diswall M, Ångström J, Breimer ME, Goddeeris B, Cox E, et al. Recognition of blood group ABH type 1 determinants by the FedF adhesin of F18-fimbriated Escherichia coli. J Biol Chem. (2009) 284:9713–26. doi: 10.1074/jbc.M807866200
26. Coddens A, Loos M, Vanrompay D, Remon JP, Cox E. Cranberry extract inhibits in vitro adhesion of F4 and F18+ Escherichia coli to pig intestinal epithelium and reduces in vivo excretion of pigs orally challenged with F18+ verotoxigenic E. coli. Vet Microbiol. (2017) 202:64–71. doi: 10.1016/j.vetmic.2017.01.019
27. Luise D, Lauridsen C, Bosi P, Trevisi P. Methodology and application of Escherichia coli F4 and F18 encoding infection models in post-weaning pigs. J Anim Sci Biotechnol. (2019) 10:1–20. doi: 10.1186/s40104-019-0352-7
28. Fairbrother JM, Nadeau É, Bélanger L, Tremblay CL, Tremblay D, Brunelle M, et al. Immunogenicity and protective efficacy of a single-dose live non-pathogenic Escherichia coli oral vaccine against F4-positive enterotoxigenic Escherichia coli challenge in pigs. Vaccine. (2017) 35:353–60. doi: 10.1016/j.vaccine.2016.11.045
29. Kim JC, Heo JM, Mullan BP, Pluske JR. Efficacy of a reduced protein diet on clinical expression of post-weaning diarrhoea and life-time performance after experimental challenge with an enterotoxigenic strain of Escherichia coli. Anim Feed Sci Technol. (2011) 170:222–30. doi: 10.1016/j.anifeedsci.2011.08.012
30. Cho HM, Gonzalez-Ortiz G, Melo-Duran D, Heo JM, Cordero G, Bedford MR, et al. Stimbiotic supplementation improved performance and reduced inflammatory response via stimulating fiber fermenting microbiome in weaner pigs housed in a poor sanitary environment and fed an antibiotic-free low zinc oxide diet. PLoS ONE. (2020) 15:e0240264. doi: 10.1371/journal.pone.0240264
31. Kim K, Ehrlich A, Perng V, Chase JA, Raybould H, Li X, et al. Algae-derived β-glucan enhanced gut health and immune responses of weaned pigs experimentally infected with a pathogenic E. coli. Anim Feed Sci Technol. (2019) 248:114–25. doi: 10.1016/j.anifeedsci.2018.12.004
32. Sudan S, Zhan X, Li J. A novel probiotic bacillus subtilis strain confers cytoprotection to host pig intestinal epithelial cells during enterotoxic escherichia coli infection. Microbiol Spectrum. (2022) 10:e01257–21. doi: 10.1128/spectrum.01257-21
33. Splichal I, Donovan SM, Splichalova Z, Neuzil Bunesova V, Vlkova E, Jenistova V, et al. Colonization of germ-free piglets with commensal Lactobacillus amylovorus, Lactobacillus mucosae, and probiotic E. coli Nissle 1917 and their interference with Salmonella Typhimurium. Microorganisms. (2019) 7:273. doi: 10.3390/microorganisms7080273
34. Boeckman JX, Sprayberry S, Korn AM, Suchodolski JS, Paulk C, Genovese K, et al. Effect of chronic and acute enterotoxigenic E. coli challenge on growth performance, intestinal inflammation, microbiome, and metabolome of weaned piglets. Sci Rep. (2022) 12:1–14. doi: 10.1038/s41598-022-08446-z
35. Li Q, Burrough ER, Gabler NK, Loving CL, Sahin O, Gould SA, et al. A soluble and highly fermentable dietary fiber with carbohydrases improved gut barrier integrity markers and growth performance in F18 ETEC challenged pigs. J Anim Sci. (2019) 97:2139–53. doi: 10.1093/jas/skz093
36. González-Solé F, Solà-Oriol D, Ramayo-Caldas Y, Rodriguez-Prado M, González Ortiz G, Bedford MR, et al. Supplementation of xylo-oligosaccharides to suckling piglets promotes the growth of fiber-degrading gut bacterial populations during the lactation and nursery periods. Sci Rep. (2022) 12:1–13. doi: 10.1038/s41598-022-15963-4
37. Felcher CM, Bogni ES, Kordon EC. IL-6 cytokine family: a putative target for breast cancer prevention and treatment. Int J Mol Sci. (2022) 23:1809. doi: 10.3390/ijms23031809
38. Sun Y, Duarte ME, Kim SW. Dietary inclusion of multispecies probiotics to reduce the severity of post-weaning diarrhea caused by Escherichia coli F18+ in pigs. Anim Nutr. (2021) 7:326–33. doi: 10.1016/j.aninu.2020.08.012
39. Tiwari UP, Fleming SA, Rasheed MSA, Jha R, Dilger RN. The role of oligosaccharides and polysaccharides of xylan and mannan in gut health of monogastric animals. J Nutr Sci. (2020) 9:14. doi: 10.1017/jns.2020.14
40. Hong J, Ariyibi S, Antony L, Scaria J, Dilberger-Lawson S, Francis D, Woyengo TA. Growth performance and gut health of Escherichia coli–challenged weaned pigs fed canola meal-containing diet. J Anim Sci. (2021) 99:skab196. doi: 10.1093/jas/skab054.156
41. Kim K, He Y, Jinno C, Kovanda L, Li X, Song M, Liu Y. Trace amounts of antibiotic exacerbated diarrhea and systemic inflammation of weaned pigs infected with a pathogenic Escherichia coli. J. Anim Sci. (2021) 99:skab073. doi: 10.1093/jas/skab073
42. Ghazalah AA, El-Manylawi MAF, Motawe HFA, Khattab M, Youssef Y. Growth performance, nutrient digestibility, biochemical properties, hematological traits, and intestinal histopathology of broiler chicks fed mannan oligosaccharides. World's Vet J. (2021) 11:621–33. doi: 10.54203/scil.2021.wvj79
43. Duarte ME, Kim SW. Significance of mucosa-associated microbiota and its impacts on intestinal health of pigs challenged with F18+ E. coli. Pathog. (2022) 11:589. doi: 10.3390/pathogens11050589
44. Csernus B, Czeglédi L. Physiological, antimicrobial, intestine morphological, and immunological effects of fructooligosaccharides in pigs. Archives Animal Breeding. (2020) 63:325. doi: 10.5194/aab-63-325-2020
45. Agazzi A, Perricone V, Omodei Zorini F, Sandrini S, Mariani E, Jiang X-R, et al. Dietary mannan oligosaccharides modulate gut inflammatory response and improve duodenal villi height in post-weaning piglets improving feed efficiency. Animals. (2020) 10:1283. doi: 10.3390/ani10081283
46. Luise D, Motta V, Boudry C, Salvarani C, Correa F, Mazzoni M, et al. The supplementation of a corn/barley-based diet with bacterial xylanase did not prevent diarrhoea of ETEC susceptible piglets, but favoured the persistence of Lactobacillus reuteri in the gut. Livest Sci. (2020) 240:104161. doi: 10.1016/j.livsci.2020.104161
47. Zhang Z, Huang B, Wang Y, Zhan Y, Zhu M, Wang C. Dynamic alterations in the donkey fecal bacteria community and metabolome characteristics during gestation. Front Microbiol. (2022) 13:561. doi: 10.3389/fmicb.2022.927561
48. Duarte ME, Tyus J, Kim SW. Synbiotic effects of enzyme and probiotics on intestinal health and growth of newly weaned pigs challenged with enterotoxigenic F18+ Escherichia coli. Front Vet Sci. (2020) 7:573. doi: 10.3389/fvets.2020.00573
49. Li Q, Peng X, Burrough ER, Sahin O, Gould SA, Gabler NK, et al. Dietary soluble and insoluble fiber with or without enzymes altered the intestinal microbiota in weaned pigs challenged with enterotoxigenic E. coli F18. Front Microbiol. (2020) 11:1110. doi: 10.3389/fmicb.2020.01110
50. Tang Y, Zhang X, Wang Y, Guo Y, Zhu P, Li G, et al. Dietary ellagic acid ameliorated Clostridium perfringens-induced subclinical necrotic enteritis in broilers via regulating inflammation and cecal microbiota. J Anim Sci Biotechnol. (2022) 13:1–18. doi: 10.1186/s40104-022-00694-3
51. Stanley D, Hughes RJ, Moore RJ. Microbiota of the chicken gastrointestinal tract: influence on health, productivity and disease. Appl Microbiol Biotechnol. (2014) 98:4301–10. doi: 10.1007/s00253-014-5646-2
52. Ivarsson E, Roos S, Liu H, Lindberg J. Fermentable non-starch polysaccharides increases the abundance of Bacteroides–Prevotella–Porphyromonas in ileal microbial community of growing pigs. Animal. (2014) 8:1777–87. doi: 10.1017/S1751731114001827
Keywords: stimbiotic, immune response, gut health, E. coli, intestinal microbiota
Citation: Song D, Lee J, Kwak W, Oh H, Chang S, An J, Cho H, Park S, Jeon K and Cho J (2023) Effects of stimbiotic supplementation on gut health, immune response, and intestinal microbiota in weaned piglets challenged with E. coli. Front. Vet. Sci. 10:1187002. doi: 10.3389/fvets.2023.1187002
Received: 15 March 2023; Accepted: 27 June 2023;
Published: 19 July 2023.
Edited by:
Balamuralikrishnan Balasubramanian, Sejong University, Republic of KoreaReviewed by:
Ilias Giannenas, Aristotle University of Thessaloniki, Greecejiashun chen, Hunan Agricultural University, China
Copyright © 2023 Song, Lee, Kwak, Oh, Chang, An, Cho, Park, Jeon and Cho. This is an open-access article distributed under the terms of the Creative Commons Attribution License (CC BY). The use, distribution or reproduction in other forums is permitted, provided the original author(s) and the copyright owner(s) are credited and that the original publication in this journal is cited, in accordance with accepted academic practice. No use, distribution or reproduction is permitted which does not comply with these terms.
*Correspondence: Jinho Cho, jinhcho@chungbuk.ac.kr
†These authors have contributed equally to this work and share first authorship