- College of Animal Science and Technology, Hunan Agricultural University, Changsha, China
The purpose of this study is to reveal the effects of different particle sizes of rice straw on the rumen protozoa count, nutrient disappearance rate, rumen fermentation, and microbial community in a rumen simulation technique (RUSITEC) system. In this experiment, a single-factor random trial design was adopted. According to the different particle sizes of rice straw, there were three treatments with three replies in each treatment. Three kinds of goat total mixed ration (TMR), with the same nutrients were used to carry out a 10 days in vitro fermentation experiment using the rumen simulation system developed by Hunan Agricultural University, including 6 days the pretrial period and 4 days formal period. This study found that the organic matter disappearance rate, concentrations of total volatile fatty acids (VFAs), acetate, propionate, and iso-butyrate were greatest in the 4 mm group (p < 0.05). There were no significant differences in the alpha diversity, among the three groups (p > 0.05). The relative abundance of Treponema and Ruminococcus of the 2 mm group increased; the relative abundance of Butyrivibrio and Prevotella in samples increased in the 4 mm group. In addition, the results of correlation analysis showed that Prevotella and Ruminococcus was positively correlated with butyrate, ammonia-N, dOM and d ADF (p < 0.05) and negatively correlated with valerate (p < 0.05); Oscillospira was positively correlated with valerate (p < 0.01) and negatively correlated with propionate, butyrate, ammonia-N, dOM and dADF (p < 0.05). The present results imply that compared to the other groups, rice straw particle size of 4 mm may improve the disappearance rate of nutrients and promote the production of volatile fatty acids by regulating ruminal microorganisms.
1. Introduction
Rice is the world's third most important particle crop following wheat and corn (1). Rice straw is a crucial residue that is generated in large amounts in Asia. The major proportion of the rice straw is directly burnt in the field, which causes air pollution. However, rice straw can be a crucial source of feed for ruminants. According to statistics, China has 133 million goats and 173 million sheep in 2020 (2). Rational utilization of rice straw resources is of great significance for the development of animal husbandry in China.
The rumen simulation technique (RUSITEC) is an in vitro fermentation system that simulates the physiological functions of the rumen, which can reduce the limitations of animal experiments in vivo. It plays an essential role in studying rumen microorganisms and rumen fermentation mechanisms (3). The RUSITEC used in this study adopts the continuous culture method. Specifically, we can continuously inject buffer solution into the fermentation tank while continuously discharging fermentation products from the fermentation tank to form a continuous and dynamic fermentation system. The standardization of RUSITEC is crucial for the accuracy and comparability of research results on ruminant nutrition.
Currently, there are many studies on technical indicators such as dilution ratio and feed input ratio on RUSITEC (4, 5), while there are few reports on comparative studies of coarse feed particle size. A few in vitro studies have shown that feed particle size can affect the fermentation characteristics of RUSITEC. Smaller feed particle sizes, concentrations of acetic acid, total volatile fatty acids, ammonia nitrogen, and dry matter and crude protein loss rates are lower, but total nitrogen content is higher (6–8). In previous studies, the particle size of the feed used by researchers was highly variable, such as 0.45 (9), 1 (10, 11), 3 (12), 4 (13), and 5 mm (14, 15). The feed particle size differed by nearly 10 fold and the comparability of the test results was poor. Therefore, this experiment used RUSITEC to study the effects of three diets (1, 2, and 4 mm) with relatively similar particle sizes of rice straw on fermentation characteristics and rumen microflora in goats, with a view to providing references for the rational utilization of rice straw resources and the improvement of artificial rumen technical indicators.
2. Materials and methods
2.1. Experimental design and diets
Rice straw was crushed by hammer mill with sieve aperture of 1 mm, 2 mm, and 4 mm, and the crushed rice straw was prepared into three TMR in a 50:50 ratio of concentrate to roughage. The samples were then separately weighed (20 g), stored in a properly sealed plastic bag, refrigerated, and used as the base ration of RUSITEC. The experimental treatments were group 1 mm, group 2 mm, and group 4 mm. The experimental diet and rumen fluid donor goat diet were formulated according to the nutritional requirements of goats in NRC (16).
The RUSITEC consisted of nine fermentation vessels, which were allocated to three groups (three vessels per group); each fermentation vessel was randomly assigned to receive each diet once. Each 10 days period consisted of a 6 days diet adaptation period followed by a 4 days sampling period. The composition and nutritional composition of the diets were shown in Table 1.
2.2. RUSITEC fermentation
Three rumen-fistulated Xiangdong black goats (30 ± 2.5 kg) were used in the experiment. Before morning feeding, contents were collected from the rumen of three goats, mixed and filtered with three layers of gauze. Then the filtered rumen fluid was stored in an insulated container filled with CO2. Buffered rumen fluid was prepared by mixing strained rumen fluid with prewarmed McDougall's (17) buffer at a ratio of 1:1 under a stream of CO2. Then, 20 g of TMR was added to each fermentation vessel and 1,000 ml of rumen fluid mixed with buffer under anaerobic conditions. Fermentation vessel contents were continuously stirred by a central propeller apparatus driven by magnets at the rate of 25 r/min and the temperature of the fermentation vessel was maintained at 39°C through a circulating hot water bath inside the water jacket in the RUSITEC system.
2.3. Sample collection and analysis
2.3.1. Rumen fermentation characteristics
After the start of the test, each day before feeding (8:00 am and 8:00 pm) the contents of fermenters was collected for protozoan counting. First, 5 ml fermentation broth was collected from the sampling port of the fermenter and placed in a 15 ml centrifuge tube. Next, 10 ml of methyl green staining liquor was added and shaken to rest overnight. Then the Protozoan count was performed by Sedgewick-Rafter counting plate and biological microscope.
From the sampling period (day 7), contents of fermenters filtered with three layers of gauze was collected, divided into two tubes with 5 ml frozen storage tube, and stored in a −20°C freezer. Samples were thawed and centrifuged at 15,000 g for 10 min at 4°C, and individual VFAs concentrations were determined by gas chromatography (Agilent 7890A; Agilent Inc.), Wang et al. (18) describe the method. In addition, ammonia in the supernatant was determined colorimetrically according to the method of Wang et al. (19) and Weatherburn (20).
2.3.2. Determination of nutrients disappearance
Before the samples were collected, nitrogen was introduced into the RUSITEC system, the solids and liquids in the fermentation vessel were mixed evenly. Then all the solids and liquids in the vessel were discharged into a 1,000 ml measuring cylinder, the total volume was recorded and the discharged mixture was filtered with a nylon bag, which was cut from nylon cloth with an aperture of 50 μm and a size of 12 cm × 8 cm. The solid effluent of each fermenter within 24 h was collected in the same nylon bag, cleaned, dried, crushed, and stored for determination of routine nutrient content. The contents of dry matter, crude protein, neutral detergent fiber, and acid detergent fiber in feeds and residues were determined according to Van Soest et al. (21).
2.3.3. Microbiota analysis by 16S RNA
Collected the contents of fermenters was collected and put them in the EP tubes after high-temperature sterilization, and then extract total DNA from the contents of fermenters samples using the Qia amp fast DNA Kit (Qiagen, Germany). The DNA extraction was checked on 1% agarose gel, and DNA concentration and purity were determined with Nano Drop 2000 UV-vis spectrophotometer (Thermo Scientific, Wilmington, USA). The hypervariable region V3–V4 of the bacterial16S rRNA gene was amplified by ABI GeneAmp®9700 PCR thermo-nuclear (ABI, CA, USA). The PCR product was removed from 2% agarose gel and purified using the AxyPrep DNA Gel Extraction Kit (Axygen Biosciences, Union City, CA, USA) according to manufacturer's instructions and quantified using Quantus ™Fluorometer (Promega, USA). Paired-end sequencing was performed on the Illumina miseq pe300 platform/novaseq PE 250 platforms (Illumina, San Diego, USA). According to previous studies (22, 23), the original 16S rRNA gene sequencing reads were demultiplexed, quality filtered, and merged. Operational taxonomic units (OTUs) with a 97% similarity cutoff were clustered using UPARSE Version 7.1 (24), and chimeric sequences were identified and removed. ACE and Chao richness estimators, Shannon and Simpson diversity indices were used to assess species diversity complexity (25). Beta diversity was assessed using Principal Component Analysis (PCA). An analysis of similarities (ANOSIM) was used to assess significant differences between samples.
2.4. Statistical analysis
SAS (Version 9.4, USA) software was used for one-way ANOVA. When the difference was significant, Duncan's method was used for multiple comparisons. Using Spearman correlation analysis, the relationship between the bacterial abundance and the disappearance of nutrients, ruminal ammonia-N and VFAs concentrations was examined. The results were presented as the mean and standard error of means (SEM). Statistical difference was respectively declared as significant or highly significant at p < 0.05 or p < 0.01.
3. Results
3.1. Rumen fermentation characteristics
The number of protozoa decreased rapidly from day 0 to day 1, slowly from day 2 to day 4, and tended to be stable from day 6 to day 10 (Figure 1).
The concentration of total VFAs, acetate, propionate, and iso-butyrate were higher in the 4 mm group than others (p < 0.05; Table 2). In addition, the valerate and A/P ratio of the 4 mm group were significantly lower than other groups (p < 0.01).
3.2. Determination of nutrients disappearance
The disappearance rate of OM was higher in the 4 mm group than other groups (Figure 2, p < 0.01), but there was no difference between the 1 mm and 2 mm groups (p > 0.05).
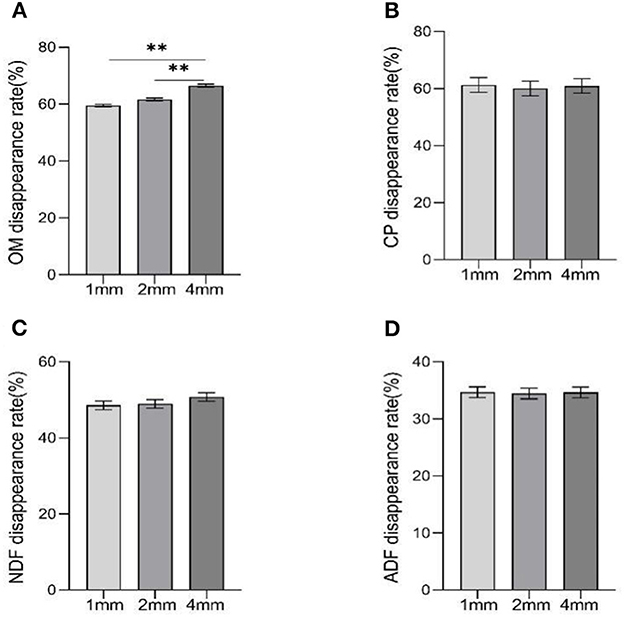
Figure 2. The effects of different straw particle size on disappearance rate of nutrients. Data were shown as means ± SEM, significant (p < 0.05) or highly significant (p < 0.01) statistical differences were represented by * or **. (A–D) are the disappearance rates of OM, CP, NDF, and ADF, respectively.
3.3. Rumen microbial diversity
There were no significant differences in the Ace index, Chao index, Shannon index, Simpson index, among the three groups (Figure 3, p > 0.05).
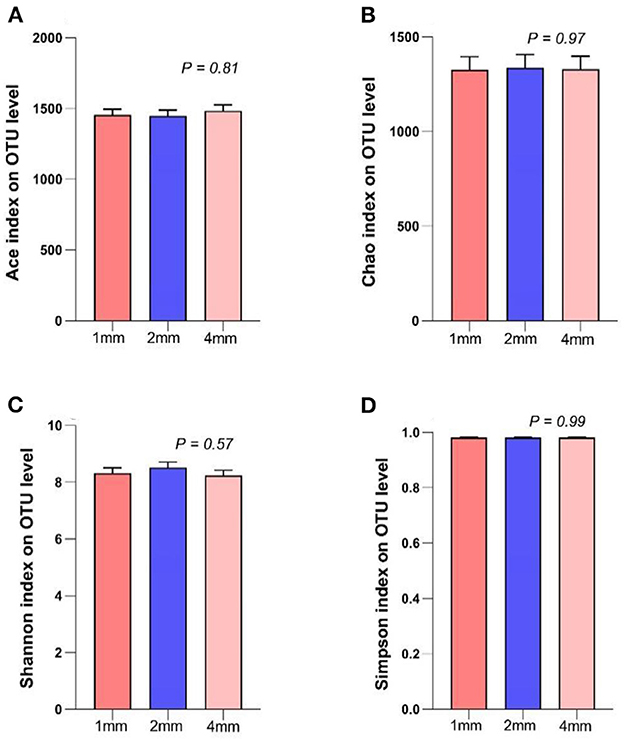
Figure 3. Effects of rice straw particle size on the ruminal microbiota alpha diversity in a rumen simulation technique system. (A) Ace index, (B) Chao index, (C) Shannon index, (D) Simpson index.
The microbial composition of contents of fermenters samples were significantly different in different treatment groups, and the explanation degree of PC1 axis and PC2 axis was 39.95 and 21.34%, respectively (Figure 4).
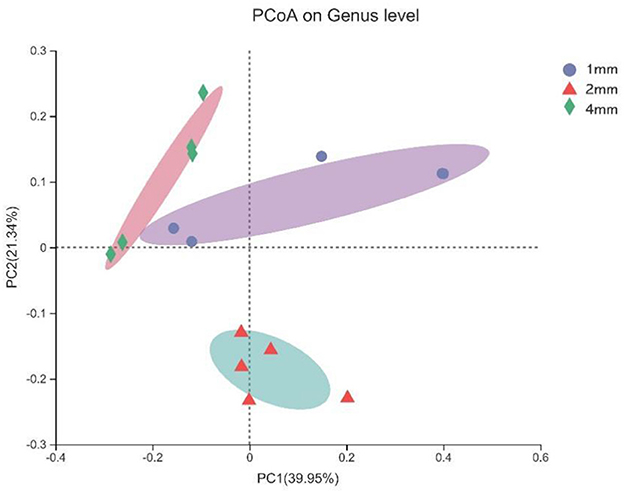
Figure 4. Principle Coordinate Analysis (PCoA) based on Bray-Curtis distance was used to compare and analyze the rumen microbial community composition of three straw particle sizes. The X-axis and Y-axis correspond to principal components 1 (PC1) and 2 (PC2), which explain the highest level of variation.
3.4. Relative abundance of rumen microorganisms
At the phyla level, Bacteroidetes and Firmicutes are the main dominant bacteria. The relative abundance of Bacteroidetes was higher in the 2 and 4 mm groups than that in the 1 mm group (p < 0.05; Table 3). However, the relative abundance of Proteobacteria, Tenericutes, Cyanobacteria, TM7, and WPS-2 were significantly higher in the 1 mm group than others (p < 0.01).
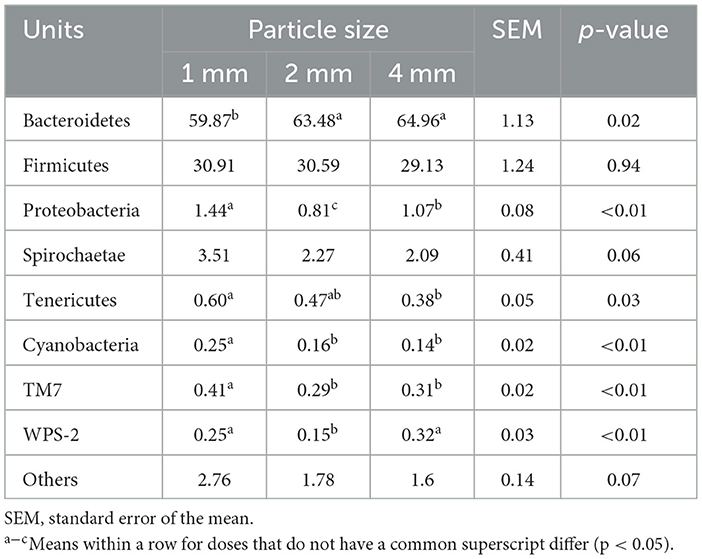
Table 3. Effects of different straw particle sizes on rumen bacterial community at phylum level (%).
At the genus level, the relative abundance of Prevotella, Ruminococcus, and Butyrivibrio were the main dominant bacteria, Prevotella in the 2 and 4 mm groups were significantly higher than that in the 1 mm group (p < 0.01; Table 4). Compared with 1 and 4 mm groups, the relative abundance of Treponema in the 2 mm group increased significantly. Interestingly, the relative abundance of Oscillospira and Mogibacteriaceae decreased with the increase of straw particle sizes (p < 0.01).
Linear Discriminant Analysis Effect Size (LEfSe) analysis was used to identify bacteria that were significantly different at the genus level between the three groups (Figure 5). A total of six genera differed significantly between the three groups. The relative abundance of Succiniclasticun and Oscillospira increased in the 1 mm group. The relative abundance of Treponema and Ruminococcus of 2 mm group increased; the relative abundance of Butyrivibrio and Prevotella increased in the 4 mm group.
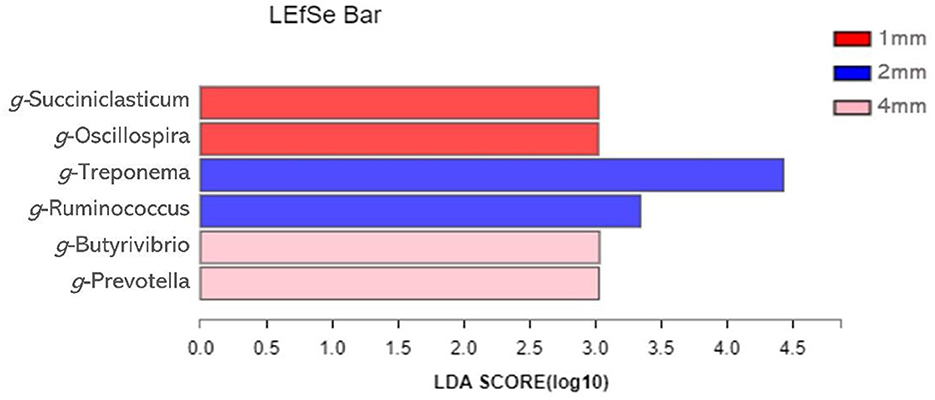
Figure 5. Identification of the most differentially abundant genera in rumen. The plot is generated from Linear Discriminant Analysis Effect Size (LEfSe) analysis with CSS-normalized OTU table and displays taxa with LDA scores above 2 and p-values below 0.05.
3.5. Microbial correlation analysis
To further understand the role of rumen microorganisms in rumen fermentation characteristics and nutrients disappearance rate, six genera with significant differences at the genus level were selected for correlation analysis. As shown in Figure 6, Prevotella and Ruminococcus was positively correlated with butyrate, ammonia-N, dOM, and dADF (p < 0.05) and negatively correlated with valerate (p < 0.05); Treponema was positively correlated with valerate (p < 0.01) and negatively correlated with propionate, butyrate and dOM (p < 0.05); Oscillospira was positively correlated with valerate (p < 0.01) and negatively correlated with propionate, butyrate and ammonia-N (p < 0.05); Butyrivibrio was positively correlated with iso-valerate and valerate (p < 0.05). Treponema was positively correlated with valerate (p < 0.01) and negatively correlated with propionate, butyrate, dOM, and dADF (p < 0.05); Oscillospira was positively correlated with valerate (p < 0.01) and negatively correlated with propionate, butyrate, ammonia-N, dOM, and dADF (p < 0.05); Butyrivibrio was positively correlated with iso-valerate and valerate (p < 0.05).
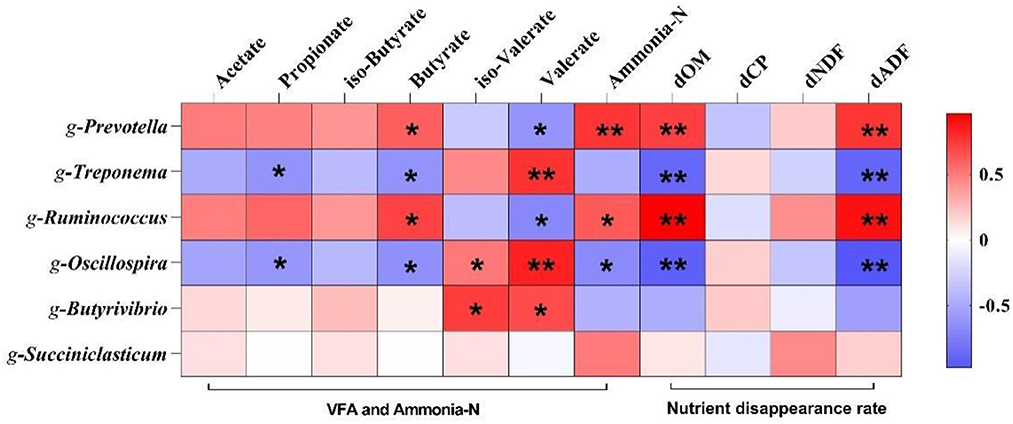
Figure 6. Correlation analysis of volatile fatty acids, ammonia-N and nutrient disappearance rate with and altered microbiota. Significant (p < 0.05) or highly significant (p < 0.01) statistical differences were represented by * or **. dOM, organic matter disappearance rate; dCP, crude protein disappearance rate; dNDF, neutral detergent fiber disappearance rate; dADF, acid detergent fiber disappearance rate.
4. Discussion
Protozoa plays a vital role in the ruminal fermentation of ruminants. Protozoa can phagocytose bacteria, convert dietary fiber and starch into volatile fatty acids, slow down the fermentation rate of carbohydrates in the rumen, and stabilize rumen pH (26). The number of protozoans in this study tended to be stable from day 6 to day 10, which was consistent with the results of Hoover and Knowlton (27). Dietary carbohydrates can produce large amounts of volatile fatty acids under the action of rumen microorganisms. The VFAs are vital energy source for ruminants, providing about 75% of the animal body's energy (28). Previous research results showed that the acetic acid and total VFAs in the large particle size diets group was significantly higher than those in the small particle size diets group in the RUSITEC system (6–8). This was confirmed by the results of the present study, which showed that total VFAs, acetate, propionate, and iso-butyrate contents were higher in the 4 mm group than in the other groups. It may be that a large particle size diets is beneficial to the growth of microorganisms in the rumen, and then promotes the production of volatile fatty acids (28). However, some researchers have found that smaller grain size diets are more conducive to rumen fermentation, which may be closely related to feed and animal species (Table 5).
Hildebrand et al. (7) used the RUSITEC system to study the effect of 1 and 4 mm particle size diets on rumen fermentation characteristics in vitro, and the experimental results showed that the larger particle size diets was more conducive to the fermentation of OM and the decomposition of nutrients. In addition, the results also showed that the content of ammonia-N was higher in the large particle group. Although the results of this experiment also confirmed that larger particle size was beneficial to improving the disappearance rate of OM, but has no effect on the content of ammonia-N. This may be due to the difference research method and diets composition.
Numerous studies have shown that not only nutrients composition of the diets affects the rumen microorganisms, but also the physical structural changes of the diets may alter the interactions between the rumen microorganisms and the animal (34, 35). The different particle sizes of the diets will change the contact area between the diets and rumen microorganisms, which will change the rumen fermentation system (36), then cause the change of rumen microorganisms, and finally affect the rumen fermentation speed and the formation of fermentation products (37). Similar to the results of previous studies (38), this study found that Bacterboidetes and Firmicutes were the dominant phyla. The primary function of Bacteroidetes was to degrade carbohydrates and proteins (39, 40). Kaakoush (41) found that Firmicutes carry many genes, producing many digestive enzymes to help animals digest and absorb nutrients. This study found that the relative abundance of Bacteroidetes increased significantly with the increase of rice straw particle sizes, the disappearance rate of OM in 4 mm group was significantly higher than others, which may be related to the increase of relative abundance of Bacteroidetes. Butyrivibrio was the dominant bacteria in the rumen, which can produce VFAs, CO2 and H2 by fermenting starch and polysaccharides. Prevotella was the main starch degrading bacteria in the rumen, and can decompose and utilize the protein in the diet (39). Fernando et al. (39) have shown that the dominant bacteria in the rumen are not affected by diet, but the results of this study show that the change of diets structure will affect the relative abundance of dominant bacteria genera, such as Prevotella, and the specific reasons need to be further explored.
In this study, LEfSe analysis found that the change in rice straw particle size would affect the composition of microorganisms. With the increase in straw particle size, the relative abundance of Ruminocococus, Butyrivibrio, and Prevotella increase. Ruminococcus and Butyrivibrio are significant cellulolytic bacteria. In the rumen, they play a crucial role in degrading cellulose and producing VFAs (42, 43). The results of correlation analysis between microorganisms and VFAs and nutrients disappearance rate showed that Prevotella was positively correlated with butyrate, ammonia-N, dOM and dADF. Liu et al. (44) found that Prevotella is the main protein hydrolysis bacteria in the rumen. The correlation between Prevotella and ammonia nitrogen and dOM may be due to the role of Prevotella in degrading protein and regulating the rumen environments (45). Ruminocococus is the main butyrate-producing bacteria, positively correlated with butyric acid. However, Ruminocococus is negatively correlated with valerate, which may be caused by competition between Ruminocococus and Butyrivibrio, both cellulolytic bacteria. Liu et al. (46) showed that iso-butyric, valerate, and Iso-valerate were beneficial for the growth of fibro catabolic bacteria. The results of this study also show that Butyrivibrio was positively correlated with isovalerate and valerate. In summary, compared to the other groups, rice straw particle size of 4 mm may improve the disappearance rate of nutrients and promote the production of VFAs by regulating the structure of rumen microorganisms. However, In the future, more targeted studies are needed to determine the appropriate particle size of the diets, to promote the standardization of RUSITEC system trials and enhance the comparability of different studies.
The results of this study can provide RUSITEC reference for the selection of straw particle size of the diet required by RUSITEC in the fermentation process, and can effectively reduce the experimental error caused by the difference in diet structure. In addition, RUSITEC has more advantages in feed evaluation than ruminants, and optimizing the technical index of RUSITEC is of great significance for the efficient utilization of straw resources in ruminants. However, although this experiment compared straw with three-grain sizes of 1, 2, and 4 mm, it is not clear whether there is a more suitable particle size, and further research is needed.
5. Conclusion
The particle sizes of rice straw could significantly affect the nutrients disappearance rate, rumen fermentation, and microbial diversity of the RUSITEC system. This study showed that the nutrient disappearance rate, volatile fatty acids contents, and the relative abundance of beneficial bacteria in the 4 mm group increased compared with other groups. Therefore, compared with other particle sizes, rice straw with a particle size of 4 mm had better fermentation characteristics and nutrients utilization efficiency in vitro under the experimental conditions.
Data availability statement
The datasets presented in this study can be found in online repositories. The names of the repository/repositories and accession number(s) can be found at: https://www.ncbi.nlm.nih.gov/bioproject; PRJNA945584.
Ethics statement
The animal study was reviewed and approved by Hunan Agricultural University Secretary, Animal Care and Use Committee.
Author contributions
ZL, HQ, ZW, and WS designed the research. FW, XL, DX, JH, and ZL conducted the research. ZL and HQ analyzed the data. ZL wrote the paper. All authors approved the final manuscript.
Funding
This work was supported by the Hunan Provincial Science and Technology Department (Grant No. 2021RC4060), Hunan Herbivores Industry Technological System, Hunan Provincial Natural Science Foundation (Grant Nos. 2019JJ50279 and 2021JJ30011), the China Agriculture Research System of MOF and MARA (Grant No. CARS-37), and National Natural Science Foundation of China (Grant No. 32172758).
Acknowledgments
We are grateful to the College of Animal Science and Technology, Hunan Agriculture University for technical support.
Conflict of interest
The authors declare that the research was conducted in the absence of any commercial or financial relationships that could be construed as a potential conflict of interest.
Publisher's note
All claims expressed in this article are solely those of the authors and do not necessarily represent those of their affiliated organizations, or those of the publisher, the editors and the reviewers. Any product that may be evaluated in this article, or claim that may be made by its manufacturer, is not guaranteed or endorsed by the publisher.
References
1. Binod P, Sindhu R, Singhania RR, Vikram S, Devi L, Nagalakshmi S, et al. Bioethanol production from rice straw: an overview. Bioresour Technol. (2010) 101:4767–74. doi: 10.1016/j.biortech.2009.10.079
2. Zheng WX, Xiao HF, Zhang M. Current situation, future development trend and suggestions of wool sheep industry in China. Chin J Anim Sci. (2023) 59:300–6+315.
3. Li L, Zhang PH. Application and progress of artificial rumen research technology. China Dairy Cattle. (2018) 341:11–4.
4. Bao WH, Wang JQ, Bu DP, Jiang YH, Jin EW, Luo QJ. Effect of dilution rate on fermentation effect of a novel solid-liquid-gas shunt rumen simulation system. Chin J Anim Nutr. (2013) 25:1534–40.
5. Bao WH. To Exploration the Optimal Scale of Feeding and the Dilution Rateof Novel Dual-flow Rumen Simulation System [Ph. D. Thesis]. Urumqi: Xinjiang Agricultural University (2013) (in Chine).
6. Rodríguez-Prado M, Calsamiglia S, Ferret A. Effects of fiber content and particle size of forage on the flow of microbial amino acids from continuous culture fermenters. J Dairy Sci. (2004) 87:1413–24. doi: 10.3168/jds.S0022-0302(04)73290-7
7. Hildebrand B, Boguhn J, Rodehutscord M. Effect of maize silage to grass silage ratio and feed particle size on ruminal fermentation in vitro. Animal. (2011) 5:528–36. doi: 10.1017/S1751731110002211
8. Hildebrand B, Boguhn J, Rodehutscord M. Investigations on the effect of forage source, grinding, and urea supplementation on ruminal fermentation and microbial protein flow in a semi-continuous rumen simulation system. Arch Anim Nutr. (2011) 65:402–14. doi: 10.1080/1745039X.2011.609751
9. Qiao FQ. Effect of Steam-flaking on Chemical Compositions, in vitro Ruminal Fermentability or Energetic Values of Maize, Wheat and Rice Grains [Ph. D. Thesis]. Beijing: China Agricultural University (2014) (in Chine).
10. Wischer G, Boguhn J, Steingaß H, Schollenberger M, Rodehutscord M. Effects of different tannin-rich extracts and rapeseed tannin monomers on methane formation and microbial protein synthesis in vitro. Animal. (2013) 7:1796–805. doi: 10.1017/S1751731113001481
11. Newbold CJ, López S, Nelson N, Ouda JO, Wallace RJ, Moss AR. Propionate precursors and other metabolic intermediates as possible alternative electron acceptors to methanogenesis in ruminal fermentation in vitro. Br J Nutr. (2005) 94:27–35. doi: 10.1079/BJN20051445
12. Klevenhusen F, Bernasconi SM, Hofstetter TB, Bolotin J, Kunz C, Soliva CR. Efficiency of monolaurin in mitigating ruminal methanogenesis and modifying C-isotope fractionation when incubating diets composed of either C3 or C4 plants in a rumen simulation technique (RUSITEC) system. Br J Nutr. (2009) 102:1308–17. doi: 10.1017/S0007114509990262
13. Avila-Stagno J, Chaves AV, Ribeiro GJ, Ungerfeld EM, McAllister TA. Inclusion of glycerol in forage diets increases methane production in a rumen simulation technique system. Br J Nutr. (2014) 111:829–35. doi: 10.1017/S0007114513003206
14. Soliva CR, Amelchanka SL, Duval SM, Kreuzer M. Ruminal methane inhibition potential of various pure compounds in comparison with garlic oil as determined with a rumen simulation technique (RUSITEC). Br J Nutr. (2011) 106:114–22. doi: 10.1017/S0007114510005684
15. Martínez ME, Ranilla MJ, Ramos S, Tejido ML, Carro MD. Effects of dilution rate and retention time of concentrate on efficiency of microbial growth, methane production, and ruminal fermentation in RUSITEC fermenters. J Dairy Sci. (2009) 92:3930–8. doi: 10.3168/jds.2008-1975
16. National Research Council. Nutrient Requirements of Goats: Angora, Dairy, and Meat Goats in Temperate and Tropical Countries, Vol. 15. National Academies Press (2012).
17. McDougall EI. The composition and output of sheep's saliva. Biochem J. (1948) 43:99–109. doi: 10.1042/bj0430099
18. Wang M, Janssen PH, Tang Y. Responses of methane production and fermentation pathways to the increased dissolved hydrogen concentration generated by eight substrates in vitro ruminal cultures. Anim Feed Sci Tech. (2014) 194:1–11. doi: 10.1016/j.anifeedsci.2014.04.012
19. Wang M, Wang R, Janssen PH, Zhang XM, Sun XZ, Pacheco D, et al. Sampling procedure for the measurement of dissolved hydrogen and volatile fatty acids in the rumen of dairy cows. J Anim Sci. (2016) 94:1159–69. doi: 10.2527/jas.2015-9658
20. Weatherburn MW. Phenol-hypochlorite reaction for determination of ammonia. Anal Chem. (1967) 39:971–4. doi: 10.1021/ac60252a045
21. Van Soest PJ, Robertson JB, Lewis BA. Methods for dietary fiber, neutral detergent fiber, and nonstarch polysaccharides in relation to animal nutrition. J Dairy Sci. (1991) 74:3583–97. doi: 10.3168/jds.S0022-0302(91)78551-2
22. Magoč T, Salzberg SL. FLASH: fast length adjustment of short reads to improve genome assemblies. Bioinformatics. (2011) 27:2957–63. doi: 10.1093/bioinformatics/btr507
23. Chen S, Zhou Y, Chen Y, Gu J. fastp: an ultra-fast all-in-one FASTQ preprocessor. Bioinformatics. (2018) 34:i884–90. doi: 10.1093/bioinformatics/bty560
24. Edgar RC. UPARSE: highly accurate © sequences from microbial amplicon reads. Nat Methods. (2013) 10:996–8. doi: 10.1038/nmeth.2604
25. Lemieux-Labonté V, Simard A, Willis C, Lapointe FJ. Enrichment of beneficial bacteria in the skin microbiota of bats persisting with white-nose syndrome. Microbiome. (2017) 5:115. doi: 10.1186/s40168-017-0334-y
26. Chaji M, Mohammadabadi T, Aghaei A. Fermenting cell walls of processed sugarcane pith by ruminal bacteria, protozoa and fungi. Int J Agric Biol. (2011) 13:283–6.
27. Hoover WH, Knowlton P. Effects of differential solid-liquid removal rates on protozoa numbers in continuous cultures of rumen contents. J Anim Sci. (1976) 19:93–6.
28. Bergman EN. Energy contributions of volatile fatty acids from the gastrointestinal tract in various species. Physiol Rev. (1990) 70:567–90. doi: 10.1152/physrev.1990.70.2.567
29. Han HZ, Guo YL, Chai SL, Feng BG. Effect of particle size of feed on rumen fermentation of sheep using the rumen simulation technique (RUSITEC). China Anim Husb Vet Med. (2017) 44:1375–81.
30. Hildebrand B, Boguhn J, Rodehutscord M. Effect of maize silage to grass silage ratio and feed particle size on protein synthesis and amino acid profile in different microbial fractions in a semi-continuous rumen simulation. Animal. (2011) 5:537–46. doi: 10.1017/S1751731110002156
31. Bowman JGP, Firkins JL. Effects of forage species and particle size on bacterial cellulolytic activity and colonization in situ. J Anim Sci. (1993) 17:1623–33. doi: 10.2527/1993.7161623x
32. Wang J, Kong LY, Xu JF, Kang J, Shen ZF, Liu T. Effects of particle size on Uraria crinita rumen in-vitro fermentation characteristics and microbial population in lambs. Acta Prataculturae Sin. (2023) 32:224–33.
33. Ueda K, Ichinohe T, Tamura T. Influence of fiber fermentation, size reduction and passage of ruminal particles on fiber digestibility in sheep fed hays. Anim Sci J. (2001) 72:198–208. doi: 10.2508/chikusan.72.198
34. Henderson G, Cox F, Ganesh S, Jonker A, Young W, Janssen PH. Rumen microbial community composition varies with diet and host, but a core microbiome is found across a wide geographical range. Sci Rep. (2016) 6:19175. doi: 10.1038/srep19175
35. David LA, Maurice CF, Carmody RN, Gootenberg DB, Button JE, Wolfe BE, et al. Diet rapidly and reproducibly alters the human gut microbiome. Nature. (2014) 505:559–63. doi: 10.1038/nature12820
36. Gimeno A, Alami AA, Abecia L, Vega AD, Fondevila M, Castrillo C. Effect of type (barley vs. maize) and processing (grinding vs dry rolling) of cereal on ruminal fermentation and microbiota of beef calves during the early fattening period. Anim Feed Sci Tech. (2015) 199:113–26. doi: 10.1016/j.anifeedsci.2014.11.008
37. Kazemi-Bonchenari M, Salem A, López S. Influence of barley grain particle size and treatment with citric acid on digestibility, ruminal fermentation and microbial protein synthesis in Holstein calves. Animal. (2017) 11:1295–302. doi: 10.1017/S1751731116002810
38. Zeng Y, Zeng D, Ni X, Zhu H, Jian P, Zhou Y, et al. Microbial community compositions in the gastrointestinal tract of Chinese Mongolian sheep using Illumina MiSeq sequencing revealed high microbial diversity. AMB Express. (2017) 7:75. doi: 10.1186/s13568-017-0378-1
39. Fernando SC, Purvis HT, Najar FZ, Sukharnikov LO, Krehbiel CR, Nagaraja TG, et al. Rumen microbial population dynamics during adaptation to a high-particle diet. Appl Environ Microbiol. (2010) 76:7482–90. doi: 10.1128/AEM.00388-10
40. Nuriel-Ohayon M, Neuman H, Koren O. Microbial changes during pregnancy, birth, and infancy. Front Microbiol. (2016) 7:1031. doi: 10.3389/fmicb.2016.01031
41. Kaakoush NO. Insights into the role of erysipelotrichaceae in the human host. Front Cell Infect Microbiol. (2015) 5:84. doi: 10.3389/fcimb.2015.00084
42. Tajima K, Aminov RI, Nagamine T, Matsui H, Nakamura M, Benno Y. Diet-dependent shifts in the bacterial population of the rumen revealed with real-time PCR. Appl Environ Microbiol. (2001) 67:2766–74. doi: 10.1128/AEM.67.6.2766-2774.2001
43. Abdul RN, Parks DH, Vanwonterghem I, Morrison M, Tyson GW, Hugenholtz P, et al. Phylogenomic analysis of the bacterial phylum fibrobacteres. Front Microbiol. (2016) 6:1469. doi: 10.3389/fmicb.2015.01469
44. Liu H, Li Z, Pei C, Degen A, Hao L, Cao X, et al. comparison between yaks and Qaidam cattle in in vitro rumen fermentation, methane emission, and bacterial community composition with poor quality substrate. Anim Feed Sci Tech. (2022) 291:115395. doi: 10.1016/j.anifeedsci.2022.115395
45. Chiquette J, Allison MJ, Rasmussen M. Use of Prevotella bryantii 25A and a commercial probiotic during subacute acidosis challenge in midlactation dairy cows. J Dairy Sci. (2012) 95:5985–95. doi: 10.3168/jds.2012-5511
Keywords: rice straw, goat, rumen simulation technique (RUSITEC), rumen microorganisms, rumen fermentation
Citation: Li Z, Qiu H, Lan X, Wang Z, Shen W, Wan F, Xiao D and He J (2023) Appropriate particle size of rice straw promoted rumen fermentation and regulated bacterial microbiota in a rumen simulation technique system. Front. Vet. Sci. 10:1185191. doi: 10.3389/fvets.2023.1185191
Received: 13 March 2023; Accepted: 23 May 2023;
Published: 12 June 2023.
Edited by:
Damiano Cavallini, University of Bologna, ItalyReviewed by:
Giovanni Buonaiuto, University of Bologna, ItalyMuhammad Zahoor, University of Agriculture, Dera Ismail Khan, Pakistan
Copyright © 2023 Li, Qiu, Lan, Wang, Shen, Wan, Xiao and He. This is an open-access article distributed under the terms of the Creative Commons Attribution License (CC BY). The use, distribution or reproduction in other forums is permitted, provided the original author(s) and the copyright owner(s) are credited and that the original publication in this journal is cited, in accordance with accepted academic practice. No use, distribution or reproduction is permitted which does not comply with these terms.
*Correspondence: Zuo Wang, enVvd2FuZ0BodW5hdS5lZHUuY24=; Weijun Shen, c2hlbndlaWp1bkBodW5hdS5lZHUuY24=