- 1Department of Agricultural and Environmental Sciences - Production, Landscape, Agroenergy, University of Milan, Milan, Italy
- 2Department of Agricultural, Food, Environmental and Animal Sciences, University of Udine, Udine, Italy
- 3Department of Veterinary Medicine and Animal Sciences, University of Milan, Lodi, Italy
Both condensed and hydrolysable tannins (CTs and HTs, respectively) have the ability to reduce enteric CH4 production in ruminants. However, the precise mechanism of action is not fully understood. Among the proposed hypotheses are the reduction of ruminal digestibility, direct control action on protozoa, reduction of archaea, and a hydrogen sink mechanism. In this in vitro study, which simulated rumen fermentation, two additives, one containing CTs (70% based on DM) from quebracho and one with HTs (75% based on DM) from chestnut, at four levels of inclusion (2, 4, 6, 8% on an as-fed basis) were added to the fermentation substrate and tested against a negative control. Both types of tannins significantly reduced total gas (GP) and CH4 (ml/g DM) production during the 48 h of incubation. The lower GP and CH4 production levels were linked to the reduction in dry matter digestibility caused by CTs and HTs. Conversely, no significant differences were observed for the protozoan and archaeal populations, suggesting a low direct effect of tannins on these rumen microorganisms in vitro. However, both types of tannins had negative correlations for the families Bacteroidales_BS11 and F082 and positive correlations for the genera Prevotella and Succinivibrio. Regarding the fermentation parameters, no differences were observed for pH and total volatile fatty acid production, while both CTs and HTs linearly reduced the NH3 content. CTs from quebracho were more effective in reducing CH4 production than HTs from chestnut. However, for both types of tannins, the reduction in CH4 production was always associated with a lower digestibility without any changes in archaea or protozoa. Due to the high variability of tannins, further studies investigating the chemical structure of the compounds and their mechanisms of action are needed to understand the different results reported in the literature.
1. Introduction
The livestock sector contributes to approximately 14.5% of anthropogenic greenhouse gas (GHG) emissions, and one of the most important GHGs is methane (CH4) (1). Ruminants contribute significantly to CH4 emissions due to enteric fermentation, accounting for 17% of global CH4 emissions (2). Moreover, ruminants release nitrogen into the environment in the form of ammonia (NH3) and nitrous oxide (N2O), another powerful GHG. Both CH4 and nitrogen emissions are not only related to environmental problems but are also associated with energy and organic matter losses that hamper the efficiency and productivity of farms (3).
Among the different strategies to reduce the environmental impact of ruminants, tannins have been evaluated for their potential in reducing enteric methanogenesis (4). Tannins are polyphenolic substances with great chemical variability, and in terrestrial plants, they are classified into two groups: condensed tannins (CTs) and hydrolysable tannins (HTs). Condensed tannins are polymers of flavonoids with high molecular weights, while HTs are polyesters of gallic acid and various individual sugars and are characterized by lower molecular weights than CT (5). Condensed tannins are mostly found in the vacuole and in the epidermal or subepidermal layers of leaves and fruit, while HTs accumulate in the cell wall. However, tannins are also frequently found in other plant tissues, such as bark, wood, roots, seeds and fruits. Furthermore, tannin concentrations vary according to the plant species and genotype, tissue developmental stage, and environmental conditions (6).
As reported by Mueller-Harvey et al. (7), despite the widespread occurrence of tannins (both CTs and HTs) in the plant kingdom, there are still large gaps in our knowledge regarding their role in plants and their impact on animals. In the diets of ruminants, it is possible to include tannins due to the use of naturally tannin-rich forages, such as some legumes, including sulla (Hedysarum coronarium), birdsfoot trefoil (Lotus corniculatus) and sainfoin (Onobrychis viciifolia), or due to the addition of purified tannin extract. Some of the most popular purified tannin extracts come from mimosa (Mimosa tenuiflora) and quebracho (Schinopsis balansae) for CTs, while a common HTs source is chestnut (Castanea sp.) (8).
Depending mostly on their concentrations in the diet but also on animal species and other factors (such as the chemical structure of the tannins, dietary composition and interaction with other compounds, adaptation of the ruminant to the presence of tannins, etc.), tannins could have both adverse and positive effects on ruminants (9).
One of the positive effects of tannins is the reduction of CH4 emitted from ruminal fermentation (3, 10, 11). This effect has been observed in several in vitro and in vivo trials, but there are some aspects that need to be explored, such as the effect of different dietary concentrations and the effect of different sources and types of tannins. In this regard, Jayanegara et al. (8) found a greater and more constant effect of HTs in comparison with CTs in reducing CH4 emissions with less adverse effects on digestibility. As highlighted by several authors (4, 5, 12, 13), it is necessary to investigate whether the reduction in CH4 emissions is linked to a reduction in the digestibility of organic matter, particularly fiber. Moreover, tannins can exert a control action on both archaea and protozoa, which are related to methane production in the rumen (14–18).
Due to their high affinity for proteins and the formation of tannin-protein complexes, tannins can also cause a reduction in protein degradability in the rumen (19). The consumption of CTs and HTs by ruminants can reduce the production of ruminal ammonia, lowering urinary nitrogen excretion and increasing faecal nitrogen excretion (7, 20, 21). This shift can improve the soil nitrogen status and reduce N2O emissions and nitrogen leachate into groundwater.
The negative effects include reduced palatability of the diet associated with the reduction of dry matter intake but also the reduction of feed digestibility. Additionally, when tannins are fed in high concentrations, they can be toxic to the animal (22).
A common generalization is that with tannin concentrations lower than 5% of the feed dry matter, there are mainly positive effects on ruminants, while with higher concentrations, negative effects prevail. However, it is important to consider the type of tannin, the plant from which it derives, as well as the type of diet and the species of ruminant (e.g., goats are more adapted to tannins compared to cows) (23). Furthermore, high concentrations of tannins in the diet would be difficult to apply commercially due to the high cost of tannin extracts.
The study aims to determine the effects of the dietary inclusion level of purified CTs and HTs on total gas and CH4 production, ruminal fermentation parameters and rumen microbial population by an in vitro method. The use of several regular dose levels for both types of tannins in this paper aims to allow a regression study that could help predict the effects of tannins at any low practical dietary dosage.
2. Materials and methods
2.1. Experimental design: samples and tannins tested
In this study, we evaluated the effects of the inclusion of commercial purified extracts of CTs (Silvafeed® Q powder, Silvateam, Mondovì, Italy; minimum tannin content of 70% based on DM, ISO 14088) from quebracho (Schinopsis lorentzii Engl.) and HTs (Silvafeed® ENC powder, Silvateam, Mondovì, Italy; minimum tannin content of 75% based on DM, ISO 14088) from chestnut (Castanea sativa Mill.) in vitro. Both types of tannin extracts were added to the fermentation substrate at different levels of inclusion: 2, 4, 6, and 8% on an as-fed basis. The fermentation substrate was composed (g/kg) of second-cut meadow hay (447), pelleted alfalfa hay (147), pea protein seeds (147), commercial concentrate mix (17% CP on DM, 220), and a vitamin–mineral mix (9) and contained 159, 391, 17.4 and 76.9 g/kg DM of CP, aNDFom, EE, and ash, respectively.
All treatments were tested versus a negative control (substrate without CT or HT addition). All treatments were tested in triplicate in two incubation runs.
2.2. In vitro gas production technique
Animal procedures were conducted under the approval of the University of Milan Ethics Committee for animal use and care and with the authorization of the Italian Ministry of Health, authorization no. 904/2016-PR.
Ruminal fluid was collected from two fistulated dry Italian Friesian dairy cows fed a diet composed of 66% hay and 34% concentrate. Depending on the treatments, 300 mg of fermentation substrate and the relative quantity of tannin inclusion (0–2–4–6–8%) were weighed into 120 ml serum bottles.
The fermentation medium was prepared according to Menke and Steingass (24) following the procedures previously described by Pirondini et al. (25). After the addition of 40 ml of the fermentation medium, the headspace of the incubation was flushed with N2, and then the incubation vials were hermetically closed with rubber tops and placed in a shaking water bath (80 RPM) at 39.5°C for 48 h.
Gas production (GP) was determined by measuring the headspace pressure in the incubation vials (26). The pressure was recorded after 24 and 48 h of incubation using a digital manometer (model 840,082, Sper Scientific, Scottsdale, AZ, United States). After the headspace pressure was measured, at each reading time, 5 ml of gas was collected from each vial using a gastight syringe and stored in airtight vials for subsequent methane analysis. At the 24-h incubation interval, after gas collection, the pressure inside each vial was brought back to the atmospheric value using needles to avoid high headspace pressures that could compromise normal microbial activity (26).
2.3. Gas production calculation and methane measurement
The gas pressure data recorded at 24 and 48 h were converted into ml of gas produced using the ideal gas law. The values of each treatment were subsequently corrected for their respective blanks.
The methane concentration at 24 and 48 h of incubation was determined by injecting 250 μl of gas into a Varian CP-3800 gas chromatograph (Varian Chromatography Systems, Walnut Creek, CA, United States) equipped with a Supelco (2.3 m length x 2.1 mm internal diameter) stainless steel column packed with 60/80 mesh Carboxen™-1,000 stationary phase (Supelco, Bellefonte, PA, United States), using He as the carrier. The CH4 volume (ml) produced during the first (0–24 h) and second (24–48 h) phases of fermentation and the final cumulative volume were calculated as reported by Tavendale and colleagues (16).
2.4. Ruminal fermentation parameters, protozoal count, and microbiota analysis
At the end of the incubation, all vials were put into an ice bath to stop the fermentation, and the pH was recorded. Two of the three replicates per treatment were intended for the determination of VFA production, NH3 concentration and dry matter digestibility (DMD), while the remaining replicate was intended for the count of protozoa and the study of the microbiota.
Two of the three replicates per treatment were individually transferred into 50 ml Falcon tubes and centrifuged at 10,000 × g for 15 min at 4°C. After this first centrifugation, 5 and 30 ml of supernatant were sampled for subsequent VFA and NH3 analyses, respectively. The incubation vials were carefully washed with distilled water to remove all substrate residuals, and the contents were transferred into the respective Falcon tubes for a second centrifugation at 10,000 × g for 5 min at 4°C. After the second centrifugation, the supernatants were discharged, and the Falcon tubes with the precipitated residue were placed in an oven at 60°C until constant weight for the determination of the undigested DM.
For VFA determination, 1 ml of 25% meta-phosphoric acid was added to 5 ml of supernatant as described by Colombini et al. (27). After 30 min, the mixture was centrifuged again at 3,500 × g for 10 min. VFA analysis was performed by injecting 2 μl of the supernatant into a Varian CP-3800 gas chromatograph (Varian Chromatography Systems, Walnut Creek, CA, United States) using a Nukol fused silica capillary column (30 m length; 0.25 mm diameter; 0.25 μm film thickness; Supelco) as reported by Pirondini et al. (28).
The NH3 concentration was determined with the Kjeldahl method, with only the distillation and titration, using a Raypa NP-1500-MP Kjeldahl distiller (Raypa, Terrassa, Spain).
At the end of the incubation, the third replicate was used for the protozoal count and the microbiota analysis. Each vial was shaken to obtain a homogeneous solution, and then, for the protozoal count, 5 ml of fermentation medium was sampled, added to 5 ml of formalin and stored at room temperature, while for the microbiota analysis, 10 ml of fermentation medium was sampled and stored at −80°C pending extraction.
The protozoal count was performed as described by Dehority (29).
The DNA from the rumen fluid was extracted using the NucleoSpin Soil kit (Macherey-Nagel, Germany) following the procedures and using the reagents suggested by the kit manufacturer. Amplification of the V4 region of the 16S gene was conducted as described by Parada et al. (30). DNA was diluted at 10 ng/μl. For amplification, the following primers were used: 515F-Y: 5′-GTGYCAGCMGCCGCGGTAA and 926R: 5′-CCGYCAATTYMTTTRAGTTT. The amplifications were performed using 5 μl of the extracted DNA in a final reaction volume of 25 μl using Platinum Taq DNA polymerase high fidelity (Thermo-Fisher, MA, United States) following the manufacturer’s instructions. The amplifications were performed for 27 cycles using 55°C as the annealing temperature. The libraries were purified with Beads Amplure XP 0.8X and amplified with Indexes Nextera XT Illumina; they were normalized, mixed, and loaded on the MiSeq platform using the 2 × 300 bp (paired-end) approach to generate a minimum of 50,000 sequences (±20%). The raw sequences R1 and R2 (raw reads) were verified and filtered by quality, trimmed by the primers, and fused by QIIME2 v8 software. DADA2 (Qiime2) software isolated the amplicon sequence variants (formerly operational taxonomic units), whose sequences were compared against the SILVA Database SSU r123 to obtain the taxonomic assignment.
2.5. Statistical analysis
The data were statistically analyzed by the MIXED procedure of SAS 9.4 (SAS Institute Inc., Cary, NC, United States), with the run as a random effect, using the following factorial model:
where Yijk is the dependent variable, μ is the overall mean, Ti is the tannin type effect (i = CTs from quebracho or HTs from chestnut), Lj is the level effect (j = 0, 2, 4, 6, 8%), T×L is the interaction between the fixed effects, Rk is the random effect of the run (k = 1, 2), and eijk is the residual error. Least squares mean estimates are reported. Differences between least square means were evaluated using Tukey’s method for comparison when the p value of the interaction T×L was <0.10.
The linear effect was tested separately for each type of tannin using the MIXED procedure of SAS 9.4 as described by St-Pierre (31), applying the following model:
where a represents the overall intercept, b is the overall regression coefficient, Li is the level of tannin inclusion (i = 0, 2, 4, 6, 8), Rj is the random effect of the run (j = 1,2), and eij is the residual error. The coefficient of determination (R2) and root mean square error (RMSE) were derived from single factor regression analysis between the adjusted values derived from the mixed model and the measured values (31, 32).
The quadratic and cubic effects of tannin inclusion were also tested separately for each type of tannin; however, these effects, which were not always significant, are not reported in the tables.
3. Results
3.1. Gas and methane production
The values of total gas and CH4 produced and the DMD are reported in Table 1, while in Tables 2, 3, the relationship between the level of tannin inclusion and the total gas and CH4 productions and DMD are reported for each type of tannin, CTs and HTs.
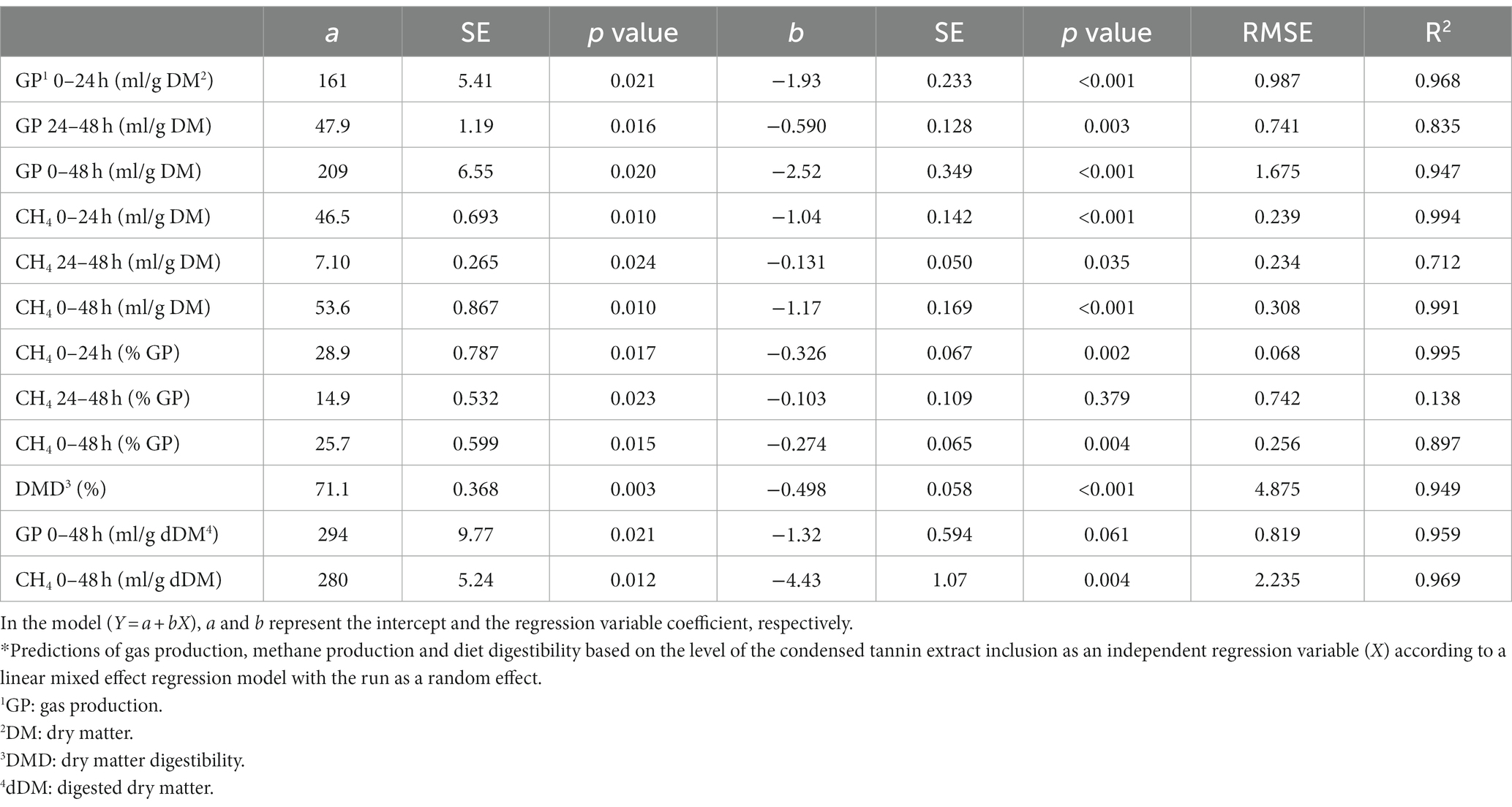
Table 2. Prediction equations of the gas production based on the inclusion level of the condensed tannin.*
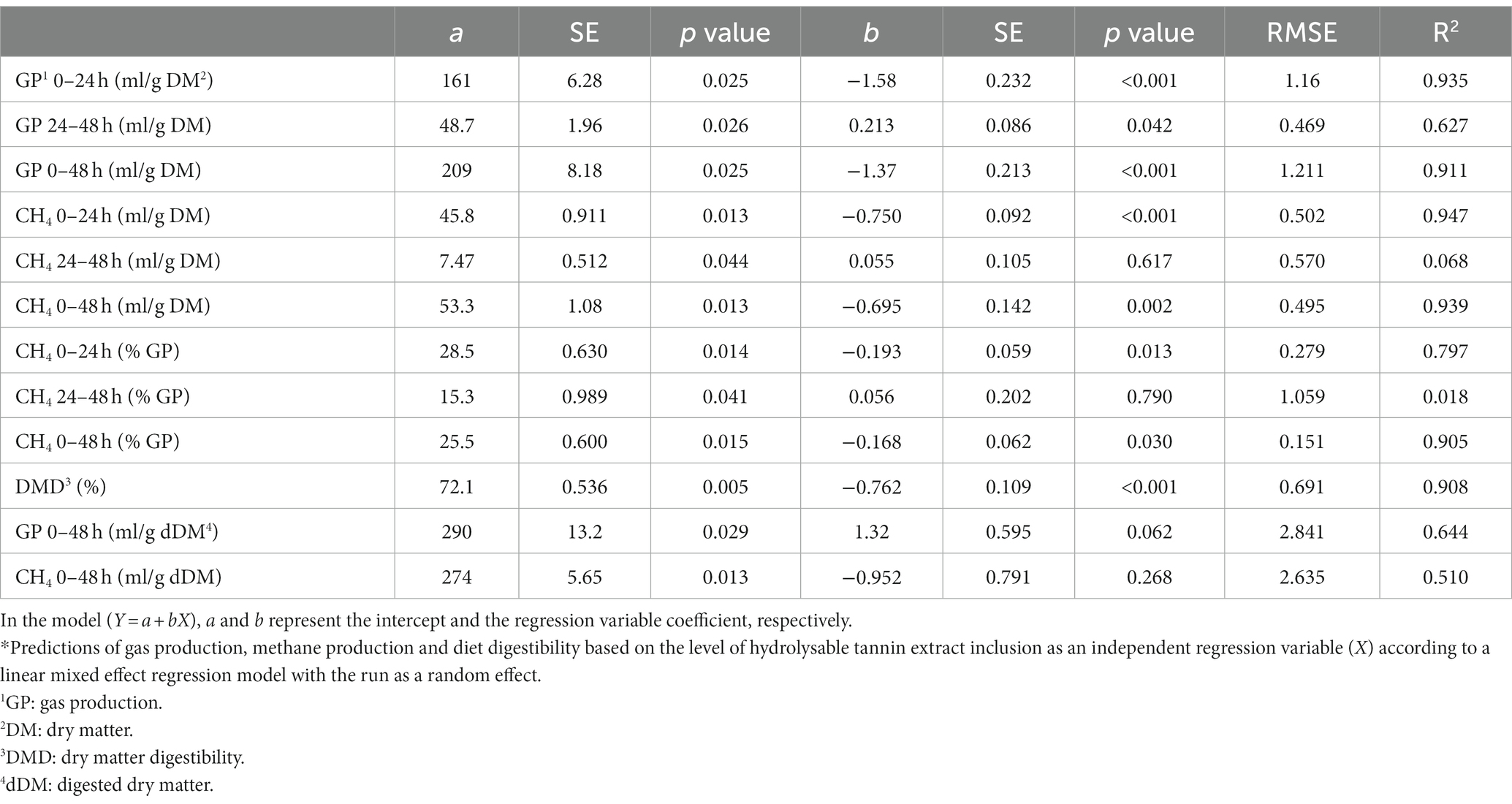
Table 3. Prediction equations of the gas production based on the inclusion level of the hydrolysable tannin.*
The GP from 0 to 24 h of incubation was significantly reduced by the level of inclusion of the tannins (p < 0.001), showing a linear reduction of the GP (Tables 2, 3) as the level of inclusion for both types of tannins increased.
During the time interval between 24 and 48 h of incubation, CTs continued to reduce the GP, while HTs had the opposite trend, numerically increasing the GP compared to the control, as shown by the different signs of the slope values in Tables 2, 3 (−0.590 and 0.213 for CTs and HTs, respectively; p = 0.003 and p = 0.042 for CTs and HTs, respectively).
Considering the total GP during the 48 h of incubation and comparing the two treatments at the different levels of inclusion, CTs had a greater effect in reducing GP than HTs (p = 0.066). This was confirmed by the slope values of the two equations of prediction (−2.52 and − 1.37 for CTs and HTs, respectively, for both types of tannins p < 0.001, Table 2).
The CH4 production (ml/g DM) at 0–24 and 0–48 h of incubation was reduced as the level of inclusion increased for both types of tannins (p < 0.001). However, during the second day of incubation (24–48 h), only CTs linearly reduced CH4 production (slope value of −0.131, p = 0.035, Table 2).
The percentage of CH4 in total GP was significantly lowered by the level of inclusion of both types of tannins at both 0–24 h (p = 0.010) and 0–48 h of incubation (p = 0.021; Table 1). The methanogenesis reduction effect was more pronounced with CTs than with HTs, as can be observed by the slope values of the two tannins for the percentage of CH4 against total GP (0–48 h; −0.274 for CTs, p = 0.004 and − 0.168 for HTs, p = 0.030).
The DMD was significantly reduced as the level of tannin inclusion increased for both CTs and HTs (p < 0.001). From the two regression equations (Tables 2, 3), it is possible to calculate that the addition of one percentage point of tannins caused a DMD reduction of 0.498% (for CTs) and 0.762% (for HTs).
3.2. Ruminal fermentation parameters
The ruminal fermentation parameters are reported in Table 4, while in Tables 5, 6, the relationships (expressed as a linear regression) between the level of tannin inclusion and the fermentation parameters are reported for each type of tannin, CTs and HTs.
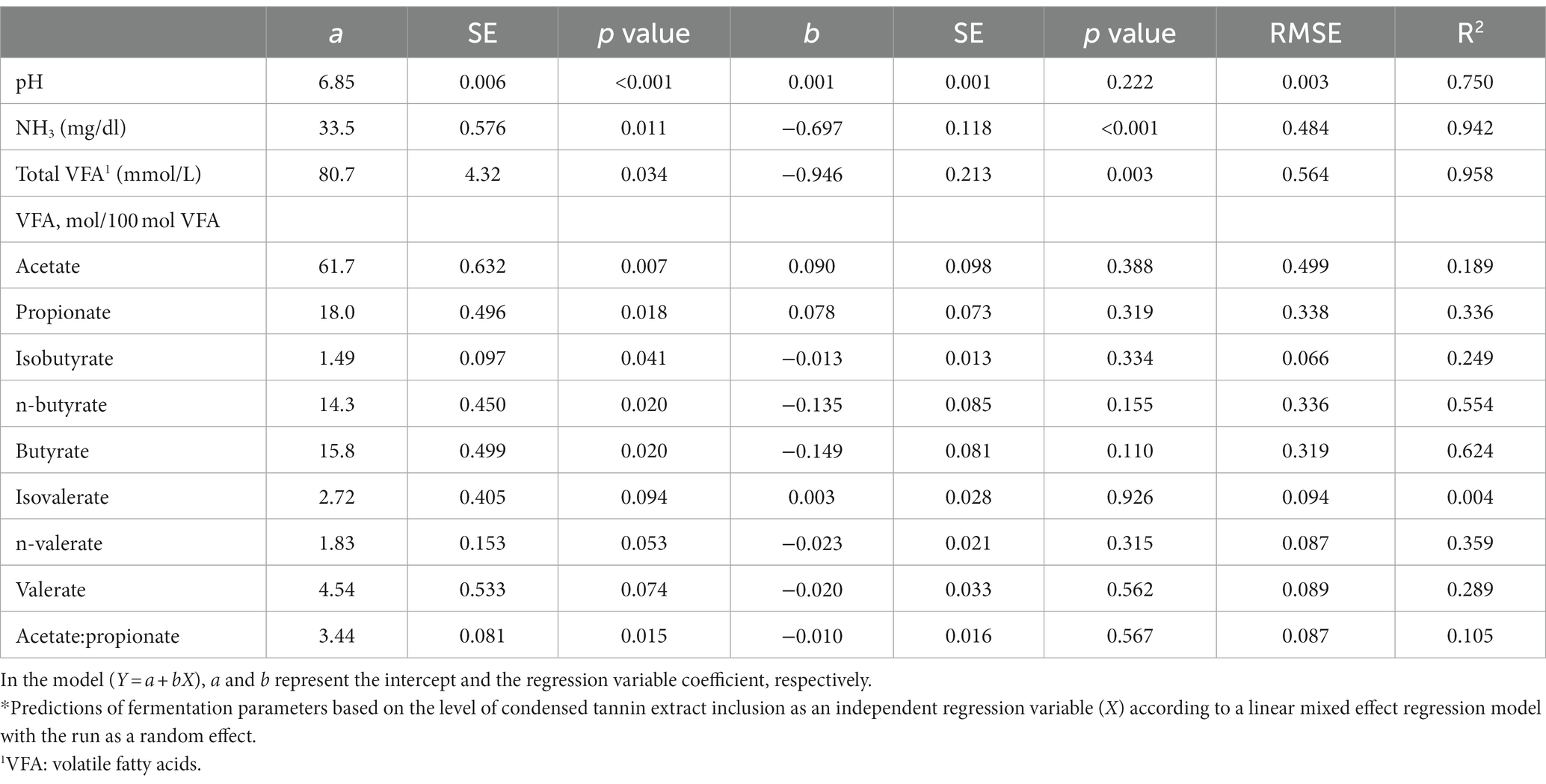
Table 5. Prediction equations of the fermentation parameters based on the inclusion level of the condensed tannin.*
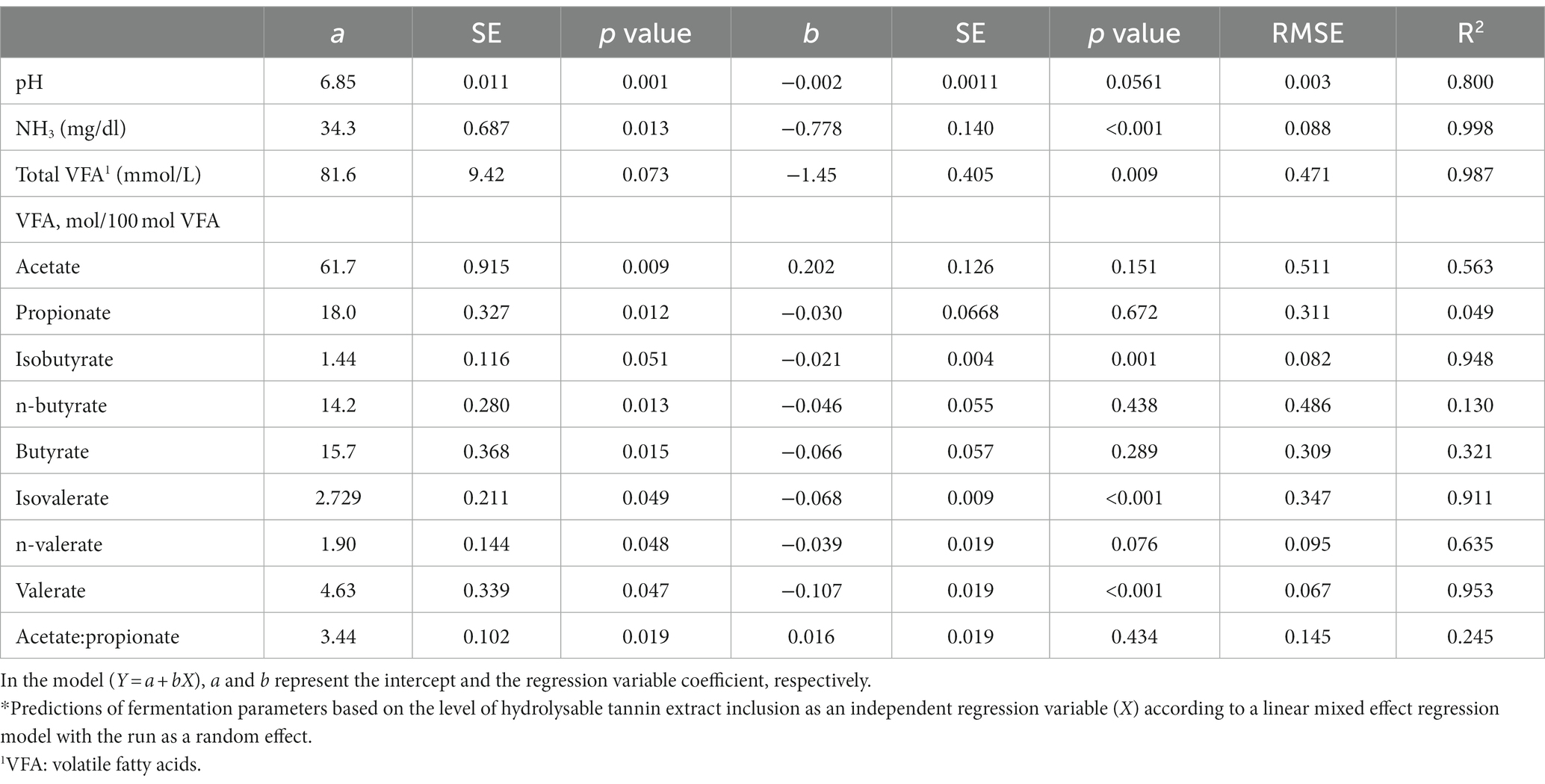
Table 6. Prediction equations of the fermentation parameters based on the inclusion level of the hydrolysable tannin.*
Regarding the pH, a significant effect of the treatment (6.86 vs. 6.84 for CTs and HTs, respectively; p < 0.001) was recorded; however, the difference was very little.
The NH3 concentration was significantly reduced (p = 0.002) with increasing tannin level for both types of tannins, as also shown by the slope coefficients (−0.697 and −0.778 for CTs and HTs, p < 0.001 for both types of tannins).
When all treatments were studied together, no differences were recorded for the total VFA production between the type of tannin and the level of inclusion. However, considering CTs and HTs separately, a linear reduction of the total VFA production for both types of tannins was observed (b coefficient − 0.946, p = 0.003, R2 = 0.958; for CTs; b coefficient − 1.45, p = 0.009, R2 = 0.987 for HTs).
No differences among treatments were observed for the contents of acetate, propionate, and butyrate expressed as a percentage of total VFA. However, isovalerate was significantly affected by the type of tannin (2.73 vs. 2.43 for CTs and HTs, p = 0.032), and a treatment trend was found for isobutyrate and valerate: HTs, unlike CTs, linearly reduced isobutyrate, isovalerate, n-valerate, and valerate as the level of inclusion increased, as can be observed from the slope coefficients in Tables 5, 6.
3.3. Protozoal count and microbiota analysis
In Table 7, the number of total protozoa and the percentages of Entodinium and Holotricha are reported. No level of inclusion, for both CTs and HTs, significantly influenced the number of protozoa compared to the control; however, CTs significantly increased the number of total protozoa compared to HTs (383 vs. 331 × 103 cell/ml, p = 0.034, for CTs and HTs, respectively). The inclusion of tannins did not affect the percentage composition of protozoa.
After sequencing, 130,841 ± 24,058 reads were obtained per sample, with a minimum of 101,733 reads and a maximum of 183,156. These values ensure that the results show complete representativeness of the microbiome. The mean number of OTUs detected (standardized value at 130,000 reads) was 8,800 ± 1,100 with a minimum of 7,500 and a maximum of 11,990. Shannon’s diversity index, whose value in the various experiments was between 8.26 and 9.48, was not significantly affected by the tannins.
Globally, bacterial species belonging to 19 phyla have been identified (Supplementary Table 1). However, only 4 of these phyla (Bacteroidota, Firmicutes, Verrucomicrobiota, and Proteobacteria) were present in a percentage greater than 1% in at least all concentrations tested in a single treatment (CTs or HTs); finally, these 4 phyla always covered at least 94.1% of the bacterial population (Figure 1).
Archaea were present in the different samples to varying degrees between 0.21 and 1.21% of the total microbiota (data not shown); however, their presence was not affected by the treatments. Analysis of similarities (ANOSIM) detected no significant differences in bacterial diversity between treatments.
The analysis of the relationship between the level of tannins and the abundance of the phyla highlighted a positive relationship for Proteobacteria (R2 = 0.959 and R2 = 0.672 for CTs and HTs, respectively; Figure 2).
The analysis of the microbiota at the class level showed the identification of bacteria belonging to 123 different classes. However, only 16 of these were present in a percentage higher than 0.5% in all treatments (Table 1). These bacterial classes covered a variable percentage between 73.2 and 85% of the entire microbial population.
Regarding the correlation between the treatments and the percentage presence of the different microorganisms, some of them showed a close correlation between treatment and the percentage presence, and it is possible to highlight negative correlations for the families Bacteroidales_BS11 and F082 (Figures 3A,B) and positive correlations for the genera Prevotella and Succinivibrio (Figures 3C,D) valid for both types of tannins (CTs or HTs).
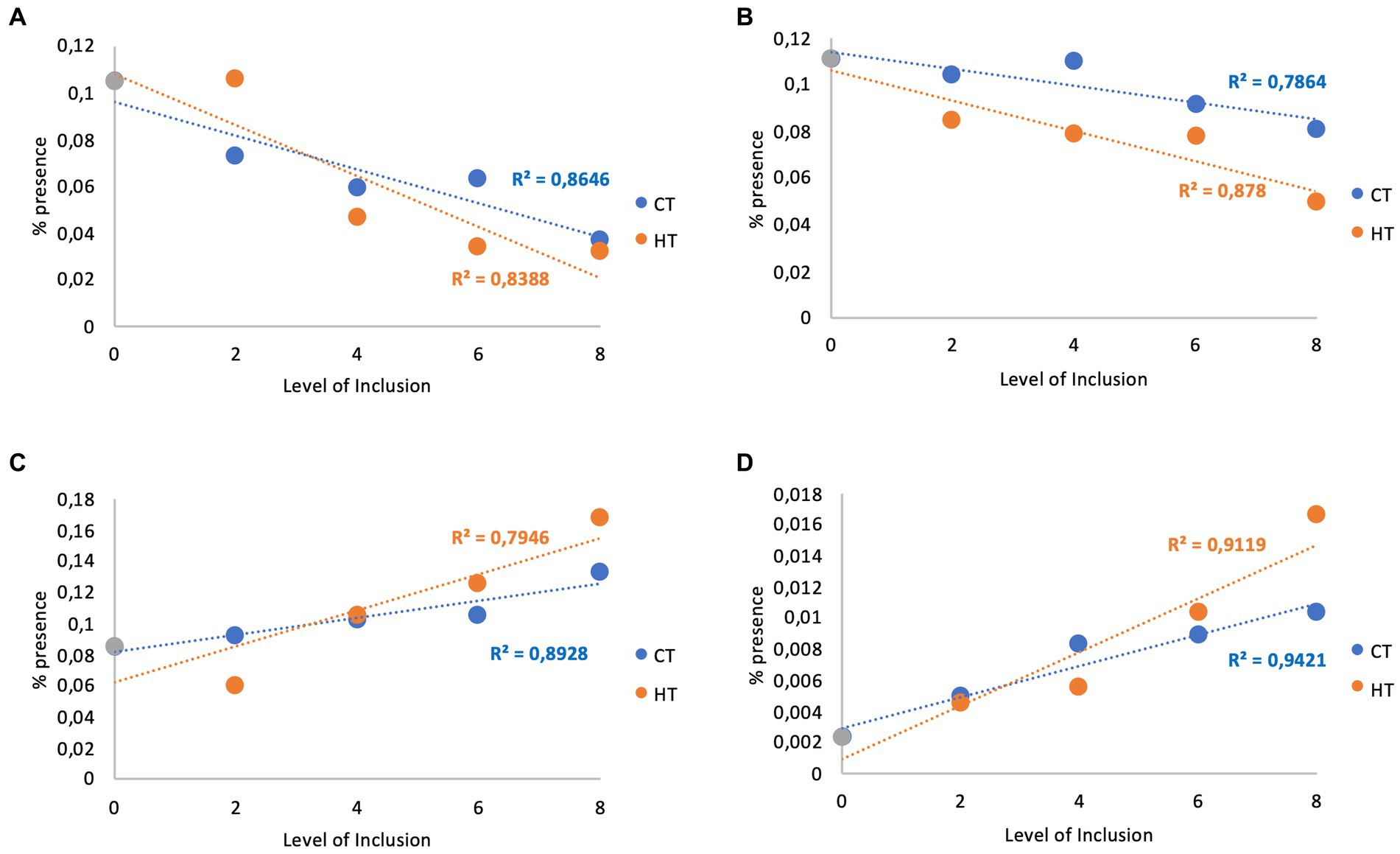
Figure 3. Correlation between tannins and families Bacteroidales_BS11 and F082 and genera Prevotella and Succinivibrio*. *: Correlation between the level of inclusion of condensed (CT) and hydrolysable (HT) tannin extracts and the percentage of the presence of families Bacteroidales_BS11 (A) and F082 (B) and genera Prevotella (C) and Succinivibrio (D).
4. Discussion
In this in vitro experiment, which simulated ruminal fermentation, we tested both CTs and HTs purified extracts from quebracho and chestnut, respectively, at different levels of inclusion. Both types of tannins reduced the GP expressed as ml/g DM (0–48 h). The percentage reduction induced by both types of tannins at the different levels of inclusion was between 1.91 and 8.61% for CTs and between 1.44 and 5.74% for HTs. The reduction percentages of GP obtained in this study are similar to those observed by Jayanegara et al. (8) with tannins extracted from chestnut and quebracho at concentrations of 3.95, 5.92, and 7.89%, except for CTs at 8% for which level of inclusion we obtained an 8.61% reduction of GP while Jayanegara et al. (8) obtained a 13.4% reduction. Condensed tannins at 6% inclusion tended to reduce the GP by approximately 8.61% compared to the control, while HTs did not differentiate significantly from the control after Tukey’s adjustment.
Both types of tannins significantly reduced CH4 production at 24 h. Interestingly, during the second day of incubation (24–48 h), CTs continued to reduce CH4 production differently than HTs, with the result that CTs were more effective in reducing CH4 production than HTs during the 48 h of incubation. This can be due to the greater ruminal degradability of HTs compared to CTs (8), which leads to a reduction in the effect of HTs with the time of incubation. As reported by McSweeney et al. (19), HTs are more susceptible to microbial hydrolysis than condensed tannins, which agrees with the results of the present study. Overall, considering the regression equation for CTs (Table 3) and HTs (Table 4), the reduction in CH4 production (ml/g DM) during the 48 h of incubation was 17.5 and 10.4%, respectively, when tannins were added at 8% compared to the 0 level of inclusion. The same higher effect of CTs compared to HTs on CH4 production in vitro was observed by Bhatta et al. (17) but not by Hassanat and Benchaar (33), who achieved similar reductions in CH4 production when CTs from quebracho and HTs from chestnut were included at concentrations higher than 10% of the diet.
The reduction of rumen methanogenesis due to the presence of tannins can be caused by the following four mechanisms of action: direct effect on rumen methanogens, direct control of the protozoan population, reduction of diet digestibility, especially fiber degradability, and the sink action of hydrogen (18, 23, 34, 35). In our study, the reduction in CH4 production is probably due to the reduction in DMD more than the other proposed mechanisms; however, although at minor extent, the other proposed mechanisms of action cannot be excluded.
Indeed, in this experiment, archaeal and protozoan populations were not affected by the treatments; although, as report by Aboagye and Beauchemin (22), tannins can reduce their activity. Protozoa and methanogens have a symbiotic relationship in the rumen, and a high number of protozoa is often associated with higher CH4 production (36–38) since approximately 37% of total rumen methanogenesis is produced by protozoa-associated methanogens (39).
In the literature, it is possible to find different responses to the presence of tannins for both methanogens and protozoa, with variation due to the type and source of tannins; in addition, the substrates used could explain the different results reported despite the similar dose of tannins (17, 34, 40, 41). In this sense, concentrated rich substrates encourage the growth of protozoa (42, 43). Sarnataro and Spanghero (44) reported a depressive effect of chestnut tannins on protozoa when the substrate was ground corn meal but not when the substrate was a mixture of feeds, simulating a total mixed ration for ruminants. In our experiment, the significant effect of the type of tannin on total protozoa without an effect due to the level of inclusion is unclear.
Regarding the type of tannin, generally, it is stated that HTs has a greater effect on CH4 reduction compared to CTs due to the supposed different mechanism of action: HTs act directly on methanogens, inhibiting their growth or their activity, while CTs reduce CH4 more through the indirect effect caused by the reduction in fiber degradability (22, 45). In an in vitro study, Jayanegara et al. (8) compared two different HTs, chestnut and sumach tannins, with two CTs, mimosa and quebracho tannins, and contrary to our work, they observed a greater reduction in CH4 production with the addition of HTs. However, similar to our results, Jayanegara et al. (8) did not observe any significant differences in methanogen populations between the types of tannins, suggesting that a discrepancy may exist between CH4 production, the number of methanogens, their activity, and the number of protozoa. This discrepancy could also be explained by the reduction of the DMD, particularly of the fibrous fraction. In a recent paper, Foggi et al. (46) tested in vitro CTs from quebracho and HTs from chestnut (inclusion level of 2%), finding a CH4 reduction of approximately 10% for both CTs and HTs, without a significant reduction in the number of protozoa but accompanied by a reduction in digestibility.
Regarding the bacterial population, the increase in the genus Prevotella in the presence of tannins can be explained by its ability to tolerate tannins (47, 48) and also to a lower presence of competitors. Moreover, species of Prevotella are hydrogen-consuming bacteria that can produce propionate via the succinate pathway from the fermentation of sugar or via the acrylate pathway through the fermentation of lactate (49, 50). The reduction in CH4 production induced by tannins can lead to an increase in hydrogen concentration, which could promote the development of Prevotella. Additionally, species of Prevotella are noncellulolytic bacteria known to use ammonia for amino acid synthesis (51). Similar to what we have observed, Sarnataro and Spanghero (44) observed an increase in P. ruminicola in vitro associated with a decrease in rumen NH3 when chestnut HTs were added to the substrate. In line with these results, de Sant’ana et al. (52) reported an increase in the genus Prevotella when dairy goats were fed a diet including tannins. However, Carrasco et al. (14) observed a reduction effect of quebracho and chestnut tannins on Prevotella in an in vivo experiment with Holstein steers, although with a high degree of variance among animals.
We also observed an increase in the genus Succinivibrio, which belongs to the Succinivibrionaceae family and is responsible for the utilization of hydrogen to produce succinate, with tannin supplementation. Succinivibrionaceae and methanogens are mutually exclusive and could represent a potential target for a CH4 mitigation strategy (53). Contrary to what was reported in several works (8, 22, 45), the present study did not reveal a significant difference between tannin sources (CTs and HTs) when added at the same concentration in terms of negative effects on DMD and VFA production. Hassanat and Benchaar (33) reported a significant reduction (−6%) in VFA production with quebracho and chestnut tannins when added at concentrations higher than 5%. In our study, we recorded a linear reduction in total VFA production for both types of tannins. Several in vitro studies (17, 33, 54, 55) have reported a reduction in branched VFAs (isobutyrate, isovalerate and valerate) caused by CTs and HTs. Branched chain volatile fatty acids are a byproduct of amino acid deamination in the rumen, and in our experiment, the reduction in these branched VFAs was caused mainly by HTs. This observation is supported by the reduction in NH3 concentration that we registered for both CTs and HTs. According to the work of Hassanat and Benchaar (33), the NH3 concentration in the fermentation buffer at the end of the incubation was linearly reduced as the level of both quebracho and chestnut tannins increased, reaching a reduction of almost 17 and 18% with the inclusion level at 8% for CTs and HTs, respectively. Similar results were found by Foggi et al. (46), who observed a reduction in NH3 concentration of 13 and 10% for CTs and HTs, respectively, when included at 2%.
The reduction of GP, DMD and VFA, without changes in the relative quantities of acetate and propionate, could indicate, for both types of tannin, an effect of reducing the degradability more generally of the organic matter rather than the fiber. This is also to be seen in relation to changes in the microbiota, especially in the population of cellulolytic bacteria.
5. Conclusion
In this study, condensed tannins from quebracho were more effective in reducing CH4 production compared to HTs from chestnut. However, no significant changes in protozoan and archaeal populations were observed. Negative correlations with both CTs and HTs were observed with the families Bacteroidales_BS11 and F082, while the correlations were positive for the genera Prevotella and Succinivibrio. Moreover, both types of tannins caused a reduction in GP, DMD and NH3 and linearly lowered VFA production as the level of tannins increased. This leads us to assume that the reduction in CH4 production is more related to the reduction in DMD caused by tannins than to their direct action on methanogens and protozoa. This could be detrimental to feed efficiency. On the other hand, the lower NH3 concentration observed with tannins may suggest a lower ruminal protein degradation, which would lead to a reduction of urinary nitrogen excretion and an increase in the faecal one, with favorable environmental and agronomic repercussions.
Due to the high variability of CTs and HTs, further studies investigating the chemical structure of the compounds and their mechanisms of action are needed to understand the different results observed in the literature.
Finally, in vivo studies will show possible long-term effects such as ruminal microbial adaptation.
Data availability statement
The datasets presented in this study regarding the DNA sequences can be found in online repositories. The names of the repository/repositories and accession number(s) can be found at: https://doi.org/10.13130/RD_UNIMI/YYXP4V.
Ethics statement
The animal study was reviewed and approved by University of Milan Ethics Committee for animal use and care and with the authorization of the Italian Ministry of Health, authorization no. 904/2016-PR.
Author contributions
MB, SC, and LR: conceptualization. MB, SC, LR, PP, GG, and GMC: methodology and investigation. DP: cow surgery cannulation and animal care. MB and LR: validation and formal analysis. GMC, PP, and LR: resources. MB, SC, PP, GMC, and LR: data curation. MB and PP: writing – original draft preparation. MB, GC, SC, GG, MS, PP, SAZ, and LR: writing – review and editing. GMC and LR: supervision. LR and MM: project administration and funding acquisition. All authors contributed to the article and approved the submitted version.
Funding
Project support was provided by the Regione Lombardia - PSR 2014–2020 Operazione 16.1.01 Gruppi Operativi PEI – d.d.s. 2951/2018.
Acknowledgments
We acknowledge the valuable help of Paolo Roveda with the in vitro experiment and of Vincenzo D’Ardes with the chemical analyses.
Conflict of interest
The authors declare that the research was conducted in the absence of any commercial or financial relationships that could be construed as a potential conflict of interest.
Publisher’s note
All claims expressed in this article are solely those of the authors and do not necessarily represent those of their affiliated organizations, or those of the publisher, the editors and the reviewers. Any product that may be evaluated in this article, or claim that may be made by its manufacturer, is not guaranteed or endorsed by the publisher.
Supplementary material
The Supplementary material for this article can be found online at: https://www.frontiersin.org/articles/10.3389/fvets.2023.1178288/full#supplementary-material
References
1. Gerber, PJ, Steinfeld, H, Henderson, B, Mottet, A, Opio, C, Dijkman, J, et al. Tackling climate change through livestock – A global assessment of emissions and mitigation opportunities. Rome: Food and Agriculture Organization of the United Nations (FAO) (2013).
2. Knapp, JR, Laur, GL, Vadas, PA, Weiss, WP, and Tricarico, JM. Invited review: enteric methane in dairy cattle production: quantifying the opportunities and impact of reducing emissions. J Dairy Sci. (2014) 97:3231–61. doi: 10.3168/jds.2013-7234
3. Niderkorn, V, Barbier, E, Macheboeuf, D, Torrent, A, Mueller-Harvey, I, and Hoste, H. In vitro rumen fermentation of diets with different types of condensed tannins derived from sainfoin (Onobrychis viciifolia Scop.) pellets and hazelnut (Corylus avellana L.) pericarps. Anim Feed Sci Technol. (2020) 259:114357. doi: 10.1016/j.anifeedsci.2019.114357
4. Beauchemin, KA, Ungerfeld, EM, Abdalla, AL, Alvarez, C, Arndt, C, Becquet, P, et al. Invited review: current enteric methane mitigation options. J Dairy Sci. (2022) 105:9297–326. doi: 10.3168/jds.2022-22091
5. Jayanegara, A, Leiber, F, and Kreuzer, M. Meta-analysis of the relationship between dietary tannin level and methane formation in ruminants from in vivo and in vitro experiments. J Anim Physiol Anim Nutr. (2012) 96:365–75. doi: 10.1111/j.1439-0396.2011.01172.x
6. Barbehenn, RV, and Peter Constabel, C. Tannins in plant–herbivore interactions. Phytochemistry. (2011) 72:1551–65. doi: 10.1016/j.phytochem.2011.01.040
7. Mueller-Harvey, I, Bee, G, Dohme-Meier, F, Hoste, H, Karonen, M, Kölliker, R, et al. Benefits of condensed tannins in forage legumes fed to ruminants: importance of structure, concentration, and diet composition. Crop Sci. (2019) 59:861–85. doi: 10.2135/cropsci2017.06.0369
8. Jayanegara, A, Goel, G, Makkar, HPS, and Becker, K. Divergence between purified hydrolysable and condensed tannin effects on methane emission, rumen fermentation and microbial population in vitro. Anim Feed Sci Technol. (2015) 209:60–8. doi: 10.1016/j.anifeedsci.2015.08.002
9. Makkar, HPS. Effects and fate of tannins in ruminant animals, adaptation to tannins, and strategies to overcome detrimental effects of feeding tannin-rich feeds. Small Rumin Res. (2003) 49:241–56. doi: 10.1016/S0921-4488(03)00142-1
10. Bueno, ICS, Brandi, RA, Franzolin, R, Benetel, G, Fagundes, GM, Abdalla, AL, et al. In vitro methane production and tolerance to condensed tannins in five ruminant species. Anim Feed Sci Technol. (2015) 205:1–9. doi: 10.1016/j.anifeedsci.2015.03.008
11. Jayanegara, A, Goel, G, Makkar, H, and Becker, K. Reduction in methane emissions from ruminants by plant secondary metabolites: effects of polyphenols and saponins In: NE Odongo, M Garcia, and GJ Viljoen, editors. Sustainable improvement of animal production and health. Rome: Food and Agriculture Organization of the United Nations (FAO) (2010). 151–7.
12. Patra, AK, and Saxena, J. Exploitation of dietary tannins to improve rumen metabolism and ruminant nutrition. J Sci Food Agric. (2010) 91:24–37. doi: 10.13130/RD_UNIMI/YYXP4V
13. Bodas, R, Prieto, N, García-González, R, Andrés, S, Giráldez, FJ, and López, S. Manipulation of rumen fermentation and methane production with plant secondary metabolites. Anim Feed Sci Technol. (2012) 176:78–93. doi: 10.1016/j.anifeedsci.2012.07.010
14. Carrasco, JMD, Cabral, C, Redondo, LM, Viso, NDP, Colombatto, D, Farber, MD, et al. Impact of chestnut and quebracho tannins on rumen microbiota of bovines. Biomed Res Int. (2017) 2017:1–11. doi: 10.1155/2017/9610810
15. Patra, AK, and Saxena, J. Dietary phytochemicals as rumen modifiers: a review of the effects on microbial populations. Antonie Van Leeuwenhoek. (2009) 96:363–75. doi: 10.1007/s10482-009-9364-1
16. Tavendale, MH, Meagher, LP, Pacheco, D, Walker, N, Attwood, GT, and Sivakumaran, S. Methane production from in vitro rumen incubations with Lotus pedunculatus and Medicago sativa, and effects of extractable condensed tannin fractions on methanogenesis. Anim Feed Sci Technol. (2005) 123-124:403–19. doi: 10.1016/j.anifeedsci.2005.04.037
17. Bhatta, R, Uyeno, Y, Tajima, K, Takenaka, A, Yabumoto, Y, Nonaka, I, et al. Difference in the nature of tannins on in vitro ruminal methane and volatile fatty acid production and on methanogenic archaea and protozoal populations. J Dairy Sci. (2009) 92:5512–22. doi: 10.3168/jds.2008-1441
18. Saminathan, M, Sieo, CC, Gan, HM, Abdullah, N, Wong, CMVL, and Ho, YW. Effects of condensed tannin fractions of different molecular weights on population and diversity of bovine rumen methanogenic archaea in vitro, as determined by high-throughput sequencing. Anim Feed Sci Technol. (2016) 216:146–60. doi: 10.1016/j.anifeedsci.2016.04.005
19. McSweeney, CS, Palmer, B, McNeill, DM, and Krause, DO. Microbial interactions with tannins: nutritional consequences for ruminants. Anim Feed Sci Technol. (2001) 91:83–93. doi: 10.1016/S0377-8401(01)00232-2
20. Waghorn, G. Beneficial and detrimental effects of dietary condensed tannins for sustainable sheep and goat production—Progress and challenges. Anim Feed Sci Technol. (2008) 147:116–39. doi: 10.1016/j.anifeedsci.2007.09.013
21. Grainger, C, Clarke, T, Auldist, MJ, Beauchemin, KA, McGinn, SM, Waghorn, GC, et al. Potential use of Acacia mearnsii condensed tannins to reduce methane emissions and nitrogen excretion from grazing dairy cows. Can J Anim Sci. (2009) 89:241–51. doi: 10.4141/CJAS08110
22. Aboagye, IA, and Beauchemin, KA. Potential of molecular weight and structure of tannins to reduce methane emissions from ruminants: a review. Animals. (2019) 9:856. doi: 10.3390/ani9110856
23. Naumann, HD, Tedeschi, LO, Zeller, WE, and Huntley, NF. The role of condensed tannins in ruminant animal production: advances, limitations and future directions. Rev Bras Zootec. (2017) 46:929–49. doi: 10.1590/s1806-92902017001200009
24. Menke, K, and Steingass, H. Estimation of the energetic feed value obtained from chemical analysis and in vitro gas production using rumen fluid. Anim Res Dev. (1988) 28:7–55.
25. Pirondini, M, Malagutti, L, Colombini, S, Amodeo, P, and Crovetto, GM. Methane yield from dry and lactating cows diets in the Po plain (Italy) using an in vitro gas production technique. Ital J Anim Sci. (2012) 11:e61. doi: 10.4081/ijas.2012.e61
26. Theodorou, MK, Williams, BA, Dhanoa, MS, McAllan, AB, and France, J. A simple gas production method using a pressure transducer to determine the fermentation kinetics of ruminant feeds. Anim Feed Sci Technol. (1994) 48:185–97. doi: 10.1016/0377-8401(94)90171-6
27. Colombini, S, Graziosi, AR, Parma, P, Iriti, M, Vitalini, S, Sarnataro, C, et al. Evaluation of dietary addition of 2 essential oils from Achillea moschata, or their components (bornyl acetate, camphor, and eucalyptol) on in vitro ruminal fermentation and microbial community composition. Anim Nutr. (2021) 7:224–31. doi: 10.1016/j.aninu.2020.11.001
28. Pirondini, M, Colombini, S, Malagutti, L, Rapetti, L, Galassi, G, Zanchi, R, et al. Effects of a selection of additives on in vitro ruminal methanogenesis and in situ and in vivo NDF digestibility. Anim Sci J. (2015) 86:59–68. doi: 10.1111/asj.12249
30. Parada, AE, Needham, DM, and Fuhrman, JA. Every base matters: assessing small subunit rRNA primers for marine microbiomes with mock communities, time series and global field samples. Environ Microbiol. (2016) 18:1403–14. doi: 10.1111/1462-2920.13023
31. St-Pierre, NR. Invited review: integrating quantitative findings from multiple studies using mixed model methodology. J Dairy Sci. (2001) 84:741–55. doi: 10.3168/jds.S0022-0302(01)74530-4
32. Rapetti, L, Colombini, S, Galassi, G, Crovetto, GM, and Malagutti, L. Relationship between milk urea level, protein feeding and urinary nitrogen excretion in high producing dairy goats. Small Rumin Res. (2014) 121:96–100. doi: 10.1016/j.smallrumres.2014.04.006
33. Hassanat, F, and Benchaar, C. Assessment of the effect of condensed (acacia and quebracho) and hydrolysable (chestnut and valonea) tannins on rumen fermentation and methane production in vitro. J Sci Food Agric. (2013) 93:332–9. doi: 10.1002/jsfa.5763
35. Becker, PM, van Wikselaar, PG, Franssen, MCR, de Vos, RCH, Hall, RD, and Beekwilder, J. Evidence for a hydrogen-sink mechanism of (+)catechin-mediated emission reduction of the ruminant greenhouse gas methane. Metabolomics. (2014) 10:179–89. doi: 10.1007/s11306-013-0554-5
36. Morgavi, DP, Forano, E, Martin, C, and Newbold, CJ. Microbial ecosystem and methanogenesis in ruminants. Animal. (2010) 4:1024–36. doi: 10.1017/S1751731110000546
37. Animut, G, Puchala, R, Goetsch, AL, Patra, AK, Sahlu, T, Varel, VH, et al. Methane emission by goats consuming diets with different levels of condensed tannins from lespedeza. Anim Feed Sci Technol. (2008) 144:212–27. doi: 10.1016/j.anifeedsci.2007.10.014
38. Spanghero, M, Braidot, M, Fabro, C, and Romanzin, A. A meta-analysis on the relationship between rumen fermentation parameters and protozoa counts in in vitro batch experiments. Anim Feed Sci Technol. (2022) 293:115471. doi: 10.1016/j.anifeedsci.2022.115471
39. Finlay, BJ, Esteban, G, Clarke, KJ, Williams, AG, Embley, TM, and Hirt, RP. Some rumen ciliates have endosymbiotic methanogens. FEMS Microbiol Lett. (1994) 117:157–61. doi: 10.1111/j.1574-6968.1994.tb06758.x
40. Patra, A, Park, T, Kim, M, and Yu, Z. Rumen methanogens and mitigation of methane emission by anti-methanogenic compounds and substances. J Anim Sci Biotechnol. (2017) 8:13. doi: 10.1186/s40104-017-0145-9
41. Cieslak, A, Szumacher-Strabel, M, Stochmal, A, and Oleszek, W. Plant components with specific activities against rumen methanogens. Animal. (2013) 7:253–65. doi: 10.1017/S1751731113000852
42. Franzolin, R, and Dehority, BA. Effect of prolonged high-concentrate feeding on ruminal protozoa concentrations. J Anim Sci. (1996) 74:2803–9. doi: 10.2527/1996.74112803x
43. Hook, SE, Steele, MA, Northwood, KS, Wright, ADG, and McBride, BW. Impact of high-concentrate feeding and low ruminal pH on methanogens and protozoa in the rumen of dairy cows. Microb Ecol. (2011) 62:94–105. doi: 10.1007/s00248-011-9881-0
44. Sarnataro, C, and Spanghero, M. In vitro rumen fermentation of feed substrates added with chestnut tannins or an extract from Stevia rebaudiana Bertoni. Anim Nutr. (2020) 6:54–60. doi: 10.1016/j.aninu.2019.11.009
45. Goel, G, and Makkar, HPS. Methane mitigation from ruminants using tannins and saponins. Trop Anim Health Prod. (2012) 44:729–39. doi: 10.1007/s11250-011-9966-2
46. Foggi, G, Terranova, M, Conte, G, Mantino, A, Amelchanka, SL, Kreuzer, M, et al. In vitro screening of the ruminal methane and ammonia mitigating potential of mixtures of either chestnut or quebracho tannins with blends of essential oils as feed additives. Ital J Anim Sci. (2022) 21:1520–32. doi: 10.1080/1828051X.2022.2130832
47. Nelson, KE, Pell, AN, Doane, PH, Giner-Chavez, BI, and Schofield, P. Chemical and biological assays to evaluate bacterial inhibition by tannins. J Chem Ecol. (1997) 23:1175–94. doi: 10.1023/B:JOEC.0000006394.06574.f4
48. Li, ZP, Liu, HL, Li, GY, Bao, K, Wang, KY, Xu, C, et al. Molecular diversity of rumen bacterial communities from tannin-rich and fiber-rich forage fed domestic sika deer (Cervus nippon) in China. BMC Microbiol. (2013) 13:151. doi: 10.1186/1471-2180-13-151
49. Purushe, J, Fouts, DE, Morrison, M, White, BA, Mackie, RI, Coutinho, PM, et al. Comparative genome analysis of Prevotella ruminicola and Prevotella bryantii: insights into their environmental niche. Microb Ecol. (2010) 60:721–9. doi: 10.1007/s00248-010-9692-8
50. Flint, HJ, Bayer, EA, Rincon, MT, Lamed, R, and White, BA. Polysaccharide utilization by gut bacteria: potential for new insights from genomic analysis. Nat Rev Microbiol. (2008) 6:121–31. doi: 10.1038/nrmicro1817
51. Atasoglu, C, Valdés, C, Walker, ND, Newbold, CJ, and Wallace, RJ. De novo synthesis of amino acids by the ruminal bacteria Prevotella bryantii B14, Selenomonas ruminantium HD4, and Streptococcus bovis ES1. Appl Environ Microbiol. (1998) 64:2836–43. doi: 10.1128/AEM.64.8.2836-2843.1998
52. Silva de Sant'ana, A, Ribeiro Silva, AP, Oliveira do Nascimento, SP, Araújo Moraes, A, Fonseca Nogueira, J, Moreira Bezerra, FC, et al. Tannin as a modulator of rumen microbial profile, apparent digestibility and ingestive behavior of lactating goats: a preliminary metagenomic view of goats adaptability to tannin. Res Vet Sci. (2022) 145:159–68. doi: 10.1016/j.rvsc.2022.02.002
53. Mizrahi, I, Wallace, RJ, and Moraïs, S. The rumen microbiome: balancing food security and environmental impacts. Nat Rev Microbiol. (2021) 19:553–66. doi: 10.1038/s41579-021-00543-6
54. Vasta, V, Makkar, HPS, Mele, M, and Priolo, A. Ruminal biohydrogenation as affected by tannins in vitro. Br J Nutr. (2008) 102:82–92. doi: 10.1017/S0007114508137898
55. Pellikaan, WF, Stringano, E, Leenaars, J, Bongers, DJGM, Schuppen, SVLV, Plant, J, et al. Evaluating effects of tannins on extent and rate of in vitro gas and CH4 production using an automated pressure evaluation system (APES). Anim Feed Sci Technol. (2011) 166-167:377–90. doi: 10.1016/j.anifeedsci.2011.04.072
Keywords: condensed tannins, hydrolysable tannins, methane, microbiota, digestibility, ruminants
Citation: Battelli M, Colombini S, Parma P, Galassi G, Crovetto GM, Spanghero M, Pravettoni D, Zanzani SA, Manfredi MT and Rapetti L (2023) In vitro effects of different levels of quebracho and chestnut tannins on rumen methane production, fermentation parameters, and microbiota. Front. Vet. Sci. 10:1178288. doi: 10.3389/fvets.2023.1178288
Edited by:
Damiano Cavallini, University of Bologna, ItalyReviewed by:
Arianna Buccioni, University of Florence, ItalyMohamed El-Sherbiny, National Research Centre, Egypt
Copyright © 2023 Battelli, Colombini, Parma, Galassi, Crovetto, Spanghero, Pravettoni, Zanzani, Manfredi and Rapetti. This is an open-access article distributed under the terms of the Creative Commons Attribution License (CC BY). The use, distribution or reproduction in other forums is permitted, provided the original author(s) and the copyright owner(s) are credited and that the original publication in this journal is cited, in accordance with accepted academic practice. No use, distribution or reproduction is permitted which does not comply with these terms.
*Correspondence: Luca Rapetti, luca.rapetti@unimi.it