- 1Department of Animal Science, College of Animal Science and Technology, Anhui Agricultural University, Hefei, China
- 2Biotechnology Center, Anhui Agricultural University, Hefei, China
- 3Qinghai Pure Yak Biotechnology Co., LTD., Xining, China
- 4State Key Laboratory of Plateau Ecology and Agriculture, Key Laboratory of Plateau Grazing Animal Nutrition and Feed Science of Qinghai Province, Qinghai Plateau Yak Research Center, Qinghai Academy of Science and Veterinary Medicine, Qinghai University, Xining, China
Introduction: Manganese (Mn) is an essential trace element for livestock, but little is known about the optimal Mn source and level for yak.
Methods: To improve yak’s feeding standards, a 48-h in vitro study was designed to examine the effect of supplementary Mn sources including Mn sulfate (MnSO4), Mn chloride (MnCl2), and Mn methionine (Met-Mn) at five Mn levels, namely 35 mg/kg, 40 mg/kg, 50 mg/kg, 60 mg/kg, and 70 mg/kg dry matter (includes Mn in substrates), on yak’s rumen fermentation.
Results: Results showed that Met-Mn groups showed higher acetate (p < 0.05), propionate, total volatile fatty acids (p < 0.05) levels, ammonia nitrogen concentration (p < 0.05), dry matter digestibility (DMD), and amylase activities (p < 0.05) compared to MnSO4 and MnCl2 groups. DMD (p < 0.05), amylase activities, and trypsin activities (p < 0.05) all increased firstly and then decreased with the increase of Mn level and reached high values at 40–50 mg/kg Mn levels. Cellulase activities showed high values (p < 0.05) at 50–70 mg/kg Mn levels. Microbial protein contents (p < 0.05) and lipase activities of Mn-Met groups were higher than those of MnSO4 and MnCl2 groups at 40–50 mg/kg Mn levels.
Discussion: Therefore, Mn-met was the best Mn source, and 40 to 50 mg/kg was the best Mn level for rumen fermentation of yaks.
1. Introduction
Yak (Poephagus grunniens) is a kind of domestic animal who lives on the Asian plateau between 3,000 and 5,000 meters above sea level (1). Local herdsmen mainly rely on grazing on natural pasture to raise yaks (2), so nutrient intakes of yaks depend heavily on the forage supply. However, forage supply on the Qinghai-Tibet plateau varied with time, which is abundant in the warm season from May to September but extremely deficient in the cold season from October to April (3). Rational feed supplementation can improve the growth performance of yaks (4). However, there is not a good feeding standard for yaks due to the lack of knowledge about mineral requirements. There is an increasing evidence that minerals are important for the health of herbivores (5) since they are key cofactors and components of enzymes in various biological processes (6). Yak’s mineral sources are mainly pasture and shrubs, and sometimes small amounts of minerals are taken from the soil (7, 8). However, pasture in most areas cannot meet the mineral requirements of grazing animals (9) and grazing yaks in the Qinghai-Tibet plateau are deficient in minerals (10). Mineral deficiency can impair growth and reproduction of animals and damage immune function and even lead to death (11).
Manganese (Mn) is an essential trace element and plays an important role in growth, development, and metabolism (12). Mn is an activator of two important enzymes necessary for the synthesis of chondroitin sulfate: polysaccharide polymerase and galactose transferase (13, 14). In vitro culture experiments show that Mn deficiency reduces cellulose digestibility and Mn supplementation improves cellulose digestibility and dry matter digestibility (DMD) (15, 16), whereas, extremely high dose of Mn (300 ppm) inhibits cellulose digestion (16). Moreover, Mn is one of the trace elements essential for certain rumen microorganisms (17). In male lambs, higher Mn intake increases the proportion of large rumen bacteria (18). However, the study of Inner Mongolia grassland of China finds that plants are seriously lacking in Mn, iron, copper, and zinc for ruminants (19). Further, Mn content of various silages is lower than the concentration indicated in the feed ingredient list (20) and lower than the requirement of dairy cows (21). Additionally, diets with high calcium, phosphorus, and iron levels also reduce the availability of Mn (20). In general, Mn is usually supplemented in the form of inorganic Mn such as Mn oxide, Mn chloride (MnCl2), and Mn sulfate (MnSO4) (22) and organic Mn such as Mn-methionine (Mn-Met), Mn-proteinate, and Mn-polysaccharide (23). NRC (1996) (24) recommends 20–40 mg/kg Mn for beef cattle. However, Mn requirement for growing yaks is still unclear.
Therefore, to maximize the positive effects of Mn in yak breeding, it’s necessary to formulate a reasonable Mn supplement scheme and select an appropriate Mn supplemental type and level. Rumen is an essential fermentation tank for the preparation of fermentation end products, especially volatile fatty acids (VFAs) and microbial protein (MCP), which are the main energy and protein sources for the ruminants (25). The higher the rumen efficiency, the better the fermentation end product synthesis (25), which indirectly reflects the productivity of ruminants. This study aimed to dissect the effects of different sources and levels of Mn on rumen fermentation of yaks through in vitro fermentation, and finally provided data support for the reasonable supplementation of Mn in yak feeding.
2. Materials and methods
2.1. Laboratory animals and feeding management
Animal experiments were conducted in accordance with the Guidelines for the Conservation and Utilization of Laboratory Animals of Qinghai Province (Qinghai Bureau of Agriculture and Animal Husbandry, 2002) and were approved by the Professional Committee of Animal Use, Academy of Animal Husbandry and Veterinary Medicine in Qinghai University (QHU20150301). According to the requirements of Chinese Beef Cattle Breeding (NY/T815-2004) (26) and Yak Nutrition Monograph (27), three healthy Datong yaks with permanent rumen fistulas were fed 4 kg/day diet. The ingredients and nutritional levels of the diet were shown in Table 1. Yaks were fed the in the individual pens twice a day (8:00 and 18:00) and had free access to water.
2.2. Determination of Mn content
According to the method described in GB/T13885-2003 (28), 5 mg diet sample was carbonized in a crucible and completely incinerated in a muffle furnace (SX-4-10, Taisite Instrument Company, Tianjin, China) at 550°C for 4 h. The ash was cooled to room temperature and dissolved in 5 mL of 6 mol/L HCl, which was then diluted to 50 mL using a volumetric flask. Subsequently, an atomic absorption spectrophotometer (TAS-990, Beijing Puxi General Instrument Company, Beijing, China) was used to determine the content of trace element Mn in the dilution (29). The parameters for the determination of Mn content in the diet were set as follows: wavelength 279.5 nm, slit width 0.2 nm, lighting mode NON-BGC, minimum lamp current 10 mA. Finally, we obtained the Mn content in the diet was 33.819 mg/kg.
2.3. Experimental design
A 3 × 5 two-factor design with 3 Mn types and 5 Mn levels was adopted for the fermentation in vitro. The 3 Mn types were MnSO4, MnCl2, and Met-Mn (Xingjia Bioengineering Company, Changsha, China). We used the diet as substrate for fermentation in vitro, so Mn content of the basic fermented substrate was also 33.819 mg/kg. Mn sources were added into the fermented substrates to make the final Mn contents reach 35 mg/kg, 40 mg/kg, 50 mg/kg, 60 mg/kg, and 70 mg/kg, respectively.
2.4. Yak’s rumen fermentation in vitro
After 15 days of adaption period, yak’s rumen fluid samples were collected from the fistulas before morning feeding using a vacuum pump (2 XZ-1, Kewei Yongxing Instrument Company, Beijing, China), mixed well, and brought back to the laboratory. After filtration with four layers of gauze, the rumen fluid was kept warm for fermentation in vitro later. Artificial fermentation solutions were prepared according to the formula in Table 2 (30). Then, 667 mL pure water, 0.17 mL trace elements solution A, 333 mL buffer solution B, 333 mL macro element solution C, and 1.7 mL resazurin indicator solution, and 67 mL deoxidizer solution were, successively, added into a 2000 mL glass jar warmed in water bath at 39°C. A magnetic stirrer was used to mix the solutions and CO2 was continuously injected into the mixed solution until it faded and become colorless. Subsequently, 750 mL rumen fluid was added into the mixed solution (31) and CO2 was injected for another 30 min to remove the dissolved air, during which the fermentation fluid was kept in a water bath at 39°C to ensure the activity of rumen microorganisms.
Yak’s diet was physically ground and sifted through a 40-mesh sieve (pore size 0.45 mm), which was used as the fermented substrate. Different types (MnSO4, MnCl2, and Met-Mn) and levels of Mn were added into the fermented substrates to reach the designed Mn levels 35 mg/kg, 40 mg/kg, 50 mg/kg, 60 mg/kg, and 70 mg/kg. Then, 200 mg fermentation substrate was accurately weighed using an analytical balance and loaded into a glass fermentation syringe (Fortuna Häberle Laboratory, Lonsee-Ettlenschie β, Germany) and 30 mL fermentation fluid was added to each fermentation syringe using a liquid separation device (Hirschmann-Laborgeräte, Eberstadt, Germany).
2.5. Determination of total gas production and methane yield
All the fermentation syringes were transferred into a preheated thermostatic artificial rumen incubator (temperature 39 ± 0.5°C, rotation speed 40 r/min) and cultured 48 h to record the total gas production (31). At the midpoint and the end point of fermentation time, the gas produced by fermentation was collected into a vacuum tube (Xinle Company, Shijiazhuang, China) for the determination of methane content in the gas. Methane content was determined using a gas chromatograph (GC-2014, Shimadzu, Kyoto, Japan) with FID detector and capillary column (FFAP30 m × 0.32 mm × 0.5 μm). The temperatures of column, vaporization chamber, and detector were set at 100°C, 100°C, and 110°C, respectively. Carrier gas was high-purity nitrogen (99.99%) with the pressure of 0.7 MPa. Hydrogen pressure and air pressure of detector were both set at 0.4 MPa while capillary column pressure was set at 65 KPa.
2.6. Determination of pH, NH3-N, MCP, DMD, VFAs, and digestive enzyme activities
After 48 h, the fermentation syringes were quickly inserted into the ice-water mixture to stop the fermentation and the pH was measured immediately using a high-precision acidometer (Hanna HI221, Ann Arbor, MI, United States). Ammonia nitrogen (NH3-N) concentration was measured using a spectrophotometry (TU-1810, Beijing Puxi Instrument Company, Beijing, China) according to the method described by Feng and Gao (32). MCP content was detected using a kit (A045-1-1, Nanjing Jiancheng Bioengineering Institute, Nanjing, China) according to the manufacturer’s instruction. The formula was MCP content (g/L) = (Am – Ak)/(As-Ak) × Ck × w, in which Am, Ak, and As were the absorption values of sample tube, blank tube, and standard tube, respectively while Ck was the protein standard concentration of 56.3 g/L and w is the dilution times. The formula of DMD was DMD (%) = (sample substrate weight - sample residue weight + blank tube resides weight)/sample substrate weight × 100.
VFAs levels in the fermented fluid were determined by a Gas Chromatography (GC-2014, Shimadz, Kyoto, Japan) with FID detector and FFAP capillary column (30 m × 0.32 mm × 0.5 μm) using the method described by Zhao et al. (33). The column temperature procedure was initial 60°C, an increasing of 10°C /min to 120°C, holding at 120°C for 3 min, an increasing of 15°C /min to 180°C, and holding at 180°C for 5 min. Both the temperatures of vaporization chamber and detector were set at 250°C. Carrier gas was high-purity nitrogen (99.99%) with the pressure of 0.7 MPa. Hydrogen pressure and air pressure of detector were both set at 0.4 MPa while capillary column pressure was set at 0.6–0.8 MPa. Digestive enzyme activities in the fermented fluid including amylase, lipase, trypsin, and cellulose were detected using commercial amylase kit (C016-1-1), lipase kit (A054-1-1), trypsin kit (A080-2-1), and cellulose kit (A138-1-1), respectively, from Nanjing Jiancheng Bioengineering Institute according to the manufacturer’s instructions (33).
2.7. Statistical analysis
Two-way ANOVA was performed using SPSS 19.0 (SPSS Inc., Chicago, IL, United States) software to analyze the effects of general Mn type, Mn level, and the Mn type & level interaction on fermentation parameters. When the Mn type & level interaction was significant, one-way ANOVA was used to make multiple comparisons among different Mn level groups with the same Mn type and among different Mn type groups at the same Mn level. When the Mn type & Mn level interaction was not significant, Duncan’s range test was used to do multiple comparisons among different Mn type groups (without regard to different Mn levels) and among different Mn level groups (without regard to different Mn types). The orthogonal polynomial contrast method was used to determine whether the effect of Mn on the measured variable is linear or quadratic with the increase of Mn level. All data were expressed as Mean ± SD. p < 0.05 was used to judge significant difference.
3. Results
3.1. Gas production in vitro
Mn level & type interaction (p = 0.006) had a significant effect on total gas production (Table 3). For different Mn levels, when MnCl2 was used as a Mn source, the total gas production in the 50 mg/kg Mn level group was significantly higher than all the other groups (p < 0.05); when MnSO4 or Met-Mn was used as a Mn source, no significant difference (p > 0.05) was observed among different Mn level groups (Table 3). For different Mn types, at 50 mg/kg Mn level, the total gas production of MnCl2 group was significantly higher than those of MnSO4 and Met-Mn groups, and at 70 mg/kg Mn level, the total gas production of MnCl2 group was significantly higher than that of Met-Mn group (p < 0.05, Table 3). Mn source (p = 0.46), Mn level (p = 0.756), or Mn level & type interaction (p = 0.621) had no significant effect on methane production (Table 3).
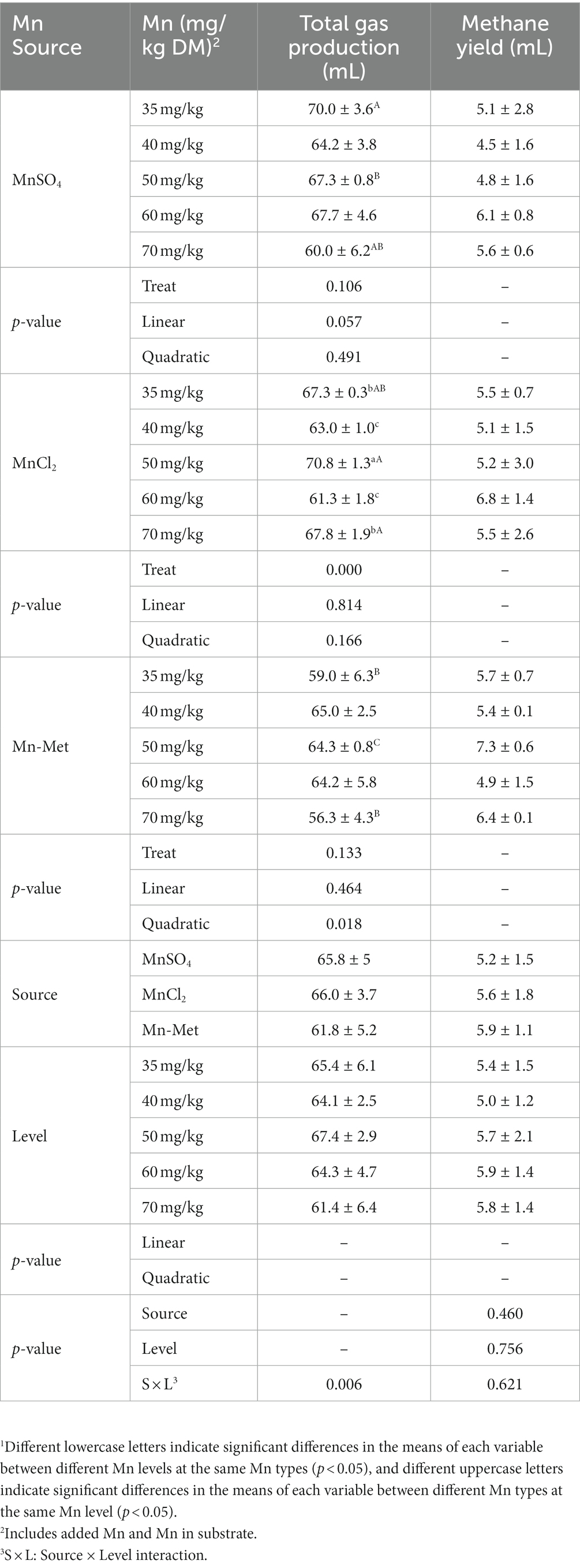
Table 3. In vitro total gas and methane yield from substrate with added manganese (Mn) from different sources at different levels1.
3.2. DMD and pH of fermentation fluid in vitro
Overall, Mn type (p < 0.001) had a significant effect on pH, while Mn level (p = 0.385) and Mn level & type interaction (p = 0.116) had no significant effect (Table 4). For different Mn types, the pH of MnCl2 group was significantly higher than the other two groups (p < 0.05). Both Mn type (p = 0.011) and level (p < 0.001) had significant effects on DMD but no significant (p = 0.326) interaction between Mn level & type (Table 4). The DMD of Met-Mn group was the highest and was significantly higher than that of MnCl2 group (p < 0.05) (Table 4). For different Mn levels, DMD showed a quadratic change trend (p < 0.001) of first increasing and then decreasing with the increase of Mn level and reached the peak value at 50 mg/kg Mn level. The DMD of 50 mg/kg group was significantly higher than those of 35 mg/kg, 40 mg/kg, and 60 mg/kg Mn level groups (p < 0.05, Table 4).
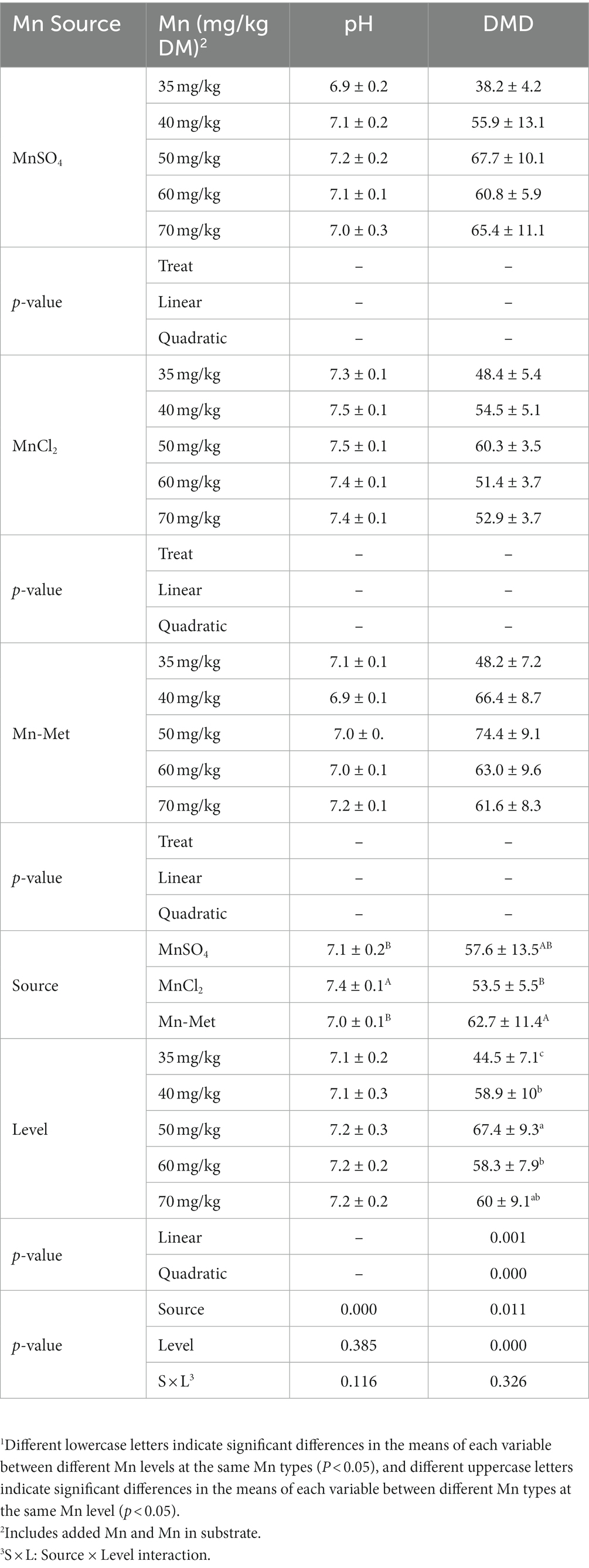
Table 4. In vitro pH and dry matter digestibility (DMD) from substrate with added manganese (Mn) from different sources at different levels1.
3.3. Digestive enzyme activity in vitro
Only the Mn level & type interaction was significant for lipase activity (p = 0.029) but not for amylase (p = 0.209), trypsin (p = 0.721), and cellulose (p = 0.584) activities (Table 5). When MnCl2 was used as a Mn source, Mn level had a significant effect on lipase activity (p = 0.033), and the lipase activity of 50 mg/kg group was significantly higher (p < 0.05) than those of 35 mg/kg, 40 mg/kg, and 70 mg/kg Mn level groups; when Mn-Met was used as a Mn source, Mn level also had a significant effect on lipase activity (p < 0.001), and the lipase activity of 50 mg/kg group was significantly higher (p < 0.05) than the other groups; when MnSO4 was used as a Mn source, no significant difference (p = 0.076) was observed among different Mn level groups (Table 5). For different Mn types, at 50 mg/kg Mn level, the lipase activities of Met-Mn and MnCl2 groups were both higher than that of MnSO4 group. Mn type had a significant effect on amylase activity (p < 0.001) but had no significant effect on trypsin (p = 0.33) and cellulose (p = 0.151) activities (Table 5). The amylase activity of Met-Mn group was higher than those of MnSO4 (p < 0.05) and MnCl2 groups (p < 0.05, Table 5). Mn level showed significant effect on amylase (p = 0.008), trypsin (p < 0.001), and cellulose (p < 0.001) activities for yak’s rumen fermentation in vitro (Table 5). With the increase of Mn level, the activity of trypsin increased firstly and then decreased which showed a quadratic change trend (p < 0.001) while the activity of cellulose linearly increased (p < 0.001). The activities of amylase, trypsin, and cellulose reached the high values at 40 mg/kg, 40–50 mg/kg, and 50–70 mg/kg Mn levels, respectively (Table 5). Therefore, Mn-Met was the optimal Mn type, and the optimal Mn levels for amylase, lipase, trypsin, and cellulase activities were 40 mg/kg, 50 mg/kg, 40–50 mg/kg, and 50–70 mg/kg, respectively.
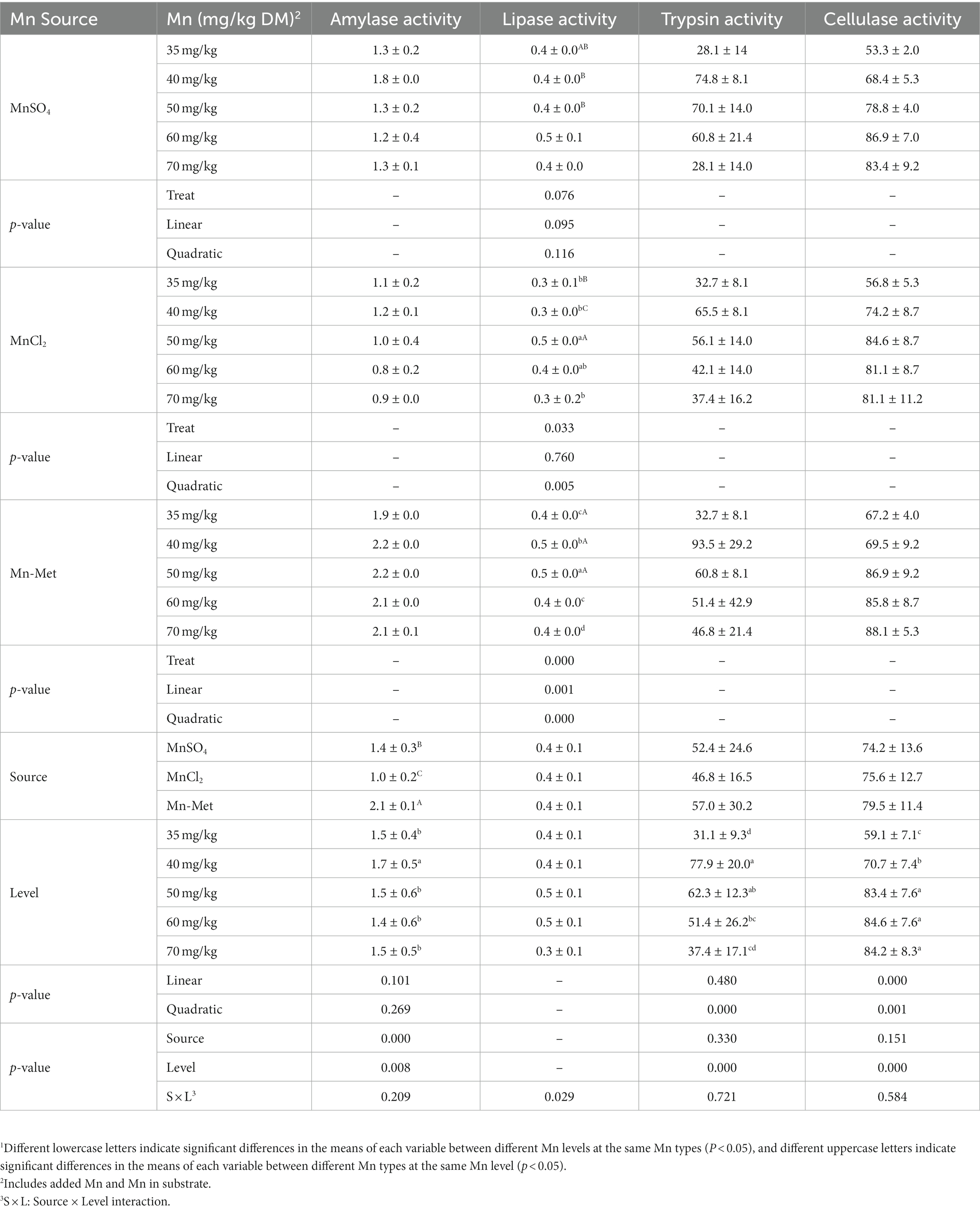
Table 5. In vitro digestive enzyme activities from substrate with added manganese (Mn) from different sources at different levels1.
3.4. VFAs levels in fermentation fluid in vitro
Mn level & type interaction had a significant effect on acetate/propionate (p = 0.033) but had no significant effects on acetate (p = 0.153), propionate (p = 0.122), isobutyrate (p = 0.65), butyrate (p = 0.428), isovalerate (p = 0.592), valerate (p = 0.656), and total VFAs (p = 0.257) levels (Table 6). For different Mn levels, acetate/propionate showed a linear increase (p = 0.01) with the increase of Mn levels when MnCl2 was used as a Mn source, and the acetate/propionate was highest at 50 mg/kg and 70 mg/kg Mn levels, which was higher than that of 35 mg/kg and 40 mg/kg Mn level groups (p < 0.05, Table 6); when MnSO4 or Met-Mn was used as a Mn source, no significant difference (p > 0.05) was observed among different Mn levels. For different Mn types, acetate/propionate in MnCl2 group was significantly higher than that in MnSO4 and Met-Mn groups at the Mn level of 50–70 mg/kg (p < 0.05, Table 6). Mn type only had significant effect on acetate (p = 0.01), propionate (p < 0.001), butyrate (p = 0.023), and total VFAs (p = 0.014) levels (Table 6). The acetate and total VFAs levels of Met-Mn group were higher than those of the other two groups (p < 0.05); the propionate level of Met-Mn group was higher than that of MnCl2 group (p < 0.05) but had no significant difference with that of MnSO4 group (p > 0.05, Table 6). The butyrate level of MnCl2 group was higher than that of MnSO4 group (p < 0.05) but had no significant difference with that of Met-Mn group (p < 0.05, Table 6). Mn level had no significant effects on acetate (p = 0.075) and propionate (p = 0.052), isobutyrate (p = 0.687), butyrate (p = 0.606), isovalerate (p = 0.639), valerate (p = 0.569), and total VFAs (p = 0.163) levels (Table 6).
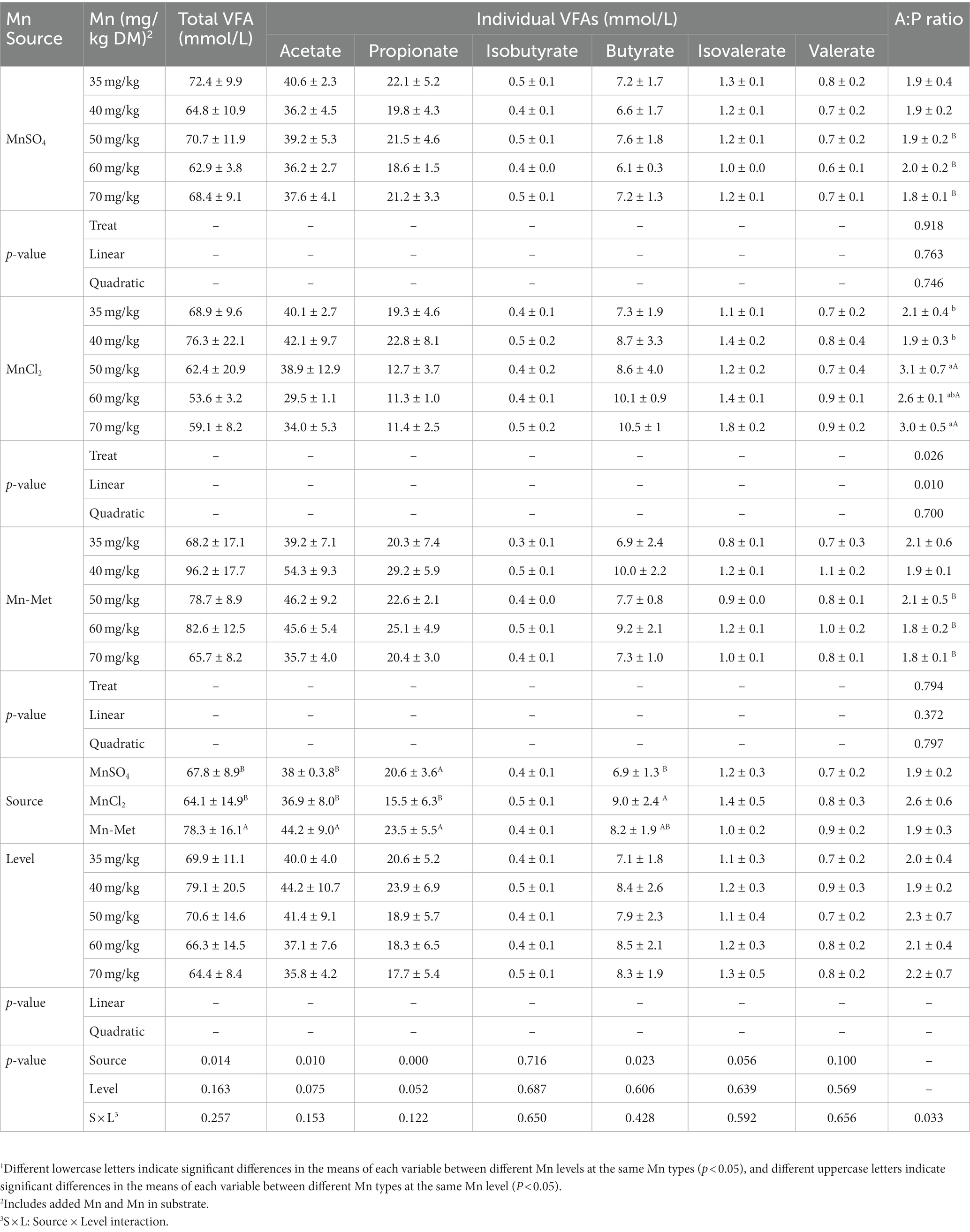
Table 6. In vitro total volatile fatty acids (VFAs) and individual VFAs and acetate/propionate from substrate with added manganese (Mn) from different sources at different levels1.
3.5. NH3-N and MCP levels in fermentation fluid in vitro
Mn type (p < 0.001) had a significant effect on NH3-N concentration while Mn level (p = 0.821) and Mn level & type interaction (p = 0.266) had no significant effect on it (Table 7). For different Mn types, NH3-N concentration of Met-Mn group was significantly higher (p < 0.05) than those of MnSO4 and MnCl2 groups (Table 7). Overview, Mn level & type interaction (p < 0.001) had a significant effect on MCP content (Table 7). With the increasing of Mn level, when MnSO4 or Met-Mn was used as a Mn source, MCP content showed a quadratic change trend (p < 0.001) which increased firstly and then decreased, reaching the highest values at 40 mg/kg and 50 mg/kg Mn levels; when MnCl2 was used as a Mn source, MCP content linearly increased (p < 0.05) and peaked at 70 mg/kg Mn level group, which was significantly higher than those of 35–50 mg/kg Mn level groups (p < 0.05) but was not significantly higher (p > 0.05) than that of 60 mg/kg Mn level group (Table 7). For different Mn types, MCP contents of Met-Mn group were significantly higher than those of MnCl2 groups at 40–50 mg/kg (p < 0.05) Mn levels and were significantly higher than those of MnSO4 groups at 40–70 mg/kg (p < 0.05) Mn levels (Table 7). Taken together, Mn-Met was the optimal Mn type and 40–50 mg/kg was the optimal Mn level for MCP content in yak’s rumen fermentation in vitro.
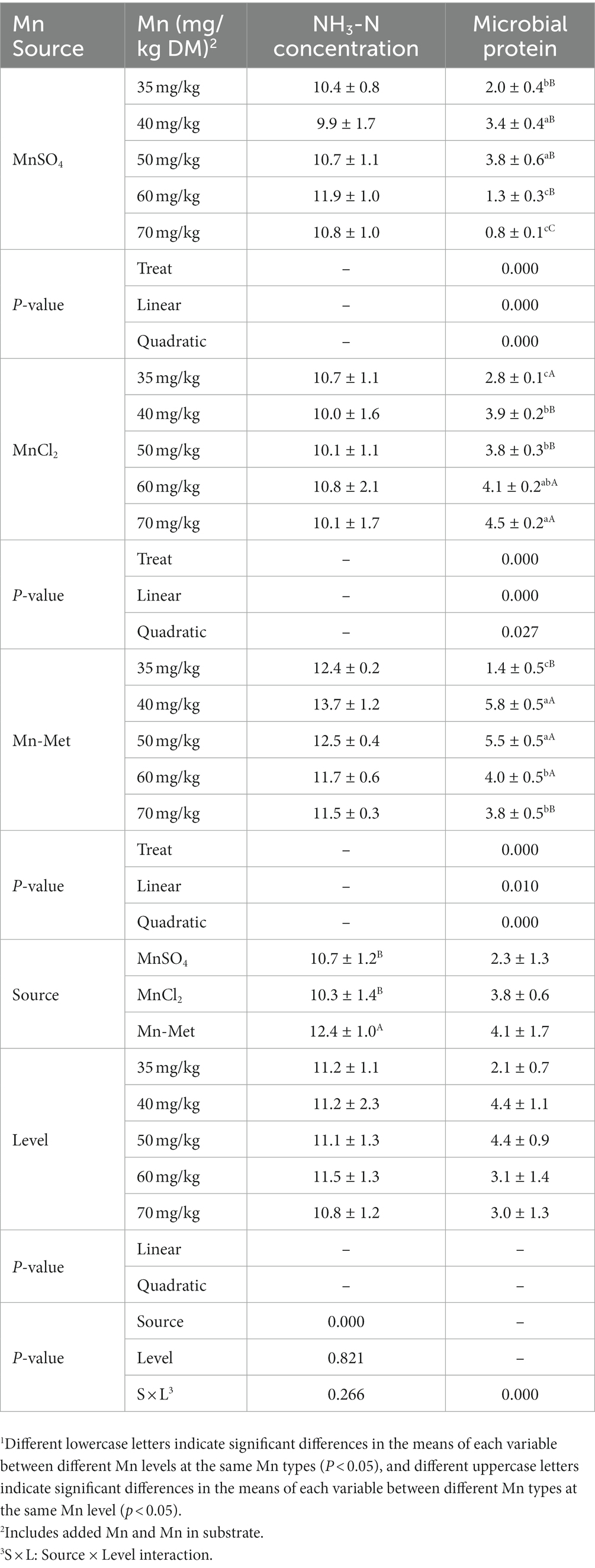
Table 7. In vitro total volatile fatty acids (VFAs) and individual VFAs and acetate/propionate ratio from substrate with added manganese (Mn) from different sources at different levels1.
4. Discussion
Rumen is an important fermenter of ruminants and mainly inhabited by microorganisms such as bacteria, fungi, and protozoa. Microorganisms degrade the feed entering the rumen (34) to synthesize VFAs and MCP (35) and provide energy and protein for animals. Rumen efficiency directly affects fermentation end products (25). Gases are produced when substrate carbohydrates and proteins are fermented into VFAs and ammonia. Total gas production has application value in evaluating the nutritional value of feed chemical components and reflecting the formation of short-chain fatty acids and the growth of microbial biomass synthesis (36). In this study, using MnCl2 as Mn source and 50 mg/kg Mn level were the most benefit for total gas production. Methane emission is a waste of energy, and in cattle up to 10% of dietary energy is lost through methane emission (37). In this study, we found that neither Mn level, Mn type, nor Mn level & type interaction had significant effect on methane yield.
Rumen pH is an important indicator of rumen environmental stability, and its normal level is between 5.5 and 7.5 (38). Rumen pH affects the growth and proliferation of microorganisms, and thus regulates rumen VFAs production (39). In turn, the production of VFAs and other organic acids such as lactate will also reduce rumen pH (40, 41). It has been reported that the optimal pH for maintaining normal cellulose decomposition is between 6.2 and 7.2 (42). When pH is lower than 6.2, cellulose decomposition bacteria are inhibited (43). The pH of Mn-Met group was significantly lower than that of MnCl2 group, which probably caused by the high levels of VFAs in Mn-Met groups (Table 6). Further, the pH of Mn-Met group might be more suitable for microbial biomass and enzyme activities for fiber, starch, and protein breakdown. Trace minerals such as Mn can promote the growth of microorganisms that degrade rumen cellulose, thus improving the fermentability and utilization of organic matter (especially hemicellulose) (17). In vitro experiments, the digestion of rumen cellulose was obviously low without Mn supplementation (44), while the digestion rate of rumen cellulose was increased with Mn supplementation (5–30 μg/mL) (45). However, high doses of inorganic Mn (182.7 mg/kg) or organic Mn (184 mg/kg) supplementation decreased α-amylase activity (46). In the current study, we found Mn-Met was the optimal Mn type and 50 mg/kg was the optimal Mn level for digestive enzyme activities in yak’s rumen fermentation. High digestive enzyme activities might improve the digestibility of feed in the process of fermentation. DMD is one of the best methods to estimate the digestibility of feed (47). Mn supplementation improved DMD in rumen fermentation (17, 45, 48). In this study, DMD was increased firstly and then decreased with the increasing of Mn level and DMD of Mn-Met group was higher than that of MnSO4 and MnCl2 groups (Table 4). The increase in nutrient digestibility related to the addition of Mn might be due to the improvement of metabolic activity of rumen microorganisms or the changes of rumen microbial population (15). Therefore, Mn-Met was the optimal Mn type and 50 mg/kg was the optimal Mn level for feed breakdown in yak’s rumen fermentation.
VFAs are mainly produced by microbial degradation of carbohydrates in the rumen (49) and provide up to 70% of the energy requirements of ruminants (50). Results showed that Mn supplementation had effects on the levels of acetate, propionate, butyrate, total VFAs, and the ratio of acetate to propionate. Acetate is not only used to supply energy through oxidation in tricarboxylic acid cycle but also used to synthesize fatty acids (51). Propionate is the main precursor of gluconeogenesis, while butyrate is mainly converted into β-hydroxybutyrate to provide energy for ruminants (52). Acetate is produced by fiber degradation, while propionate and butyrate are mainly produced by starch degradation (53, 54). Lower acetate/propionate can provide the body with faster and higher energy, which is conducive to improving the growth performance of animals (55). In this study, compared with MnSO4 and MnCl2 groups, VFAs levels were higher in Met-Mn group, while acetate/propionate value were lower at 50–70 mg/kg Mn level. The supplementation of Met-Mn in the diets of cows and buffalo increased the concentration of total VFAs concentrations (56, 57), which were consistent with our results. Taken together, Mn-Met was the optimal Mn type for yak’s rumen fermentation in terms of VFA production.
Rumen microbiota can degrade the proteins and non-protein substances in the feed into oligopeptides, amino acids, and ammonia, which are then converted into MCP with the help of energy generated by feed fermentation (58). NH3-N concentration is the key factor for efficient synthesis of MCP (59), so NH3-N concentration is widely used to evaluate essential nitrogen source (25). Low NH3-N concentration will inhibit microbial growth and productivity (60), limit rumen fermentation, and ultimately affect rumen ecology (25). In this study, we found that the concentration of ammonia nitrogen in Mn-Met group was higher than those in MnSO4 and MnCl2 groups. MCP synthesis is affected by energy substance, protein content, and the number and activity of rumen microorganisms (61, 62). We found MCP content increased firstly and then decreased subsequently with the increasing of Mn levels when MnSO4 or Mn-Met was used as a Mn source. Further, MCP contents of Mn-Met groups were higher than MnSO4 and MnCl2 groups at 40–50 mg/kg Mn levels (Table 7). Therefore, 40–50 mg/kg is the optimal Mn levels and Mn-Met is the optimal Mn type for yak’s rumen fermentation in terms of NH3-N production and MCP synthesis.
Studies found dietary Mn requirement of sheep was about 20 mg/kg in growing and finishing period (63), Mn requirement of sika deer was 80 mg/kg in terms of feed digestibility (64), and the recommended concentration for breeding cattle is 40 mg/kg Mn (19). In this study, it was found that 40–50 mg/kg Mn level was the most beneficial to yak’s rumen fermentation in vitro. The relative utilization rate of different types of Mn in digestive tract of ruminants was different (65). Trace elements in organic form are more beneficial for animals to absorb than inorganic form (66, 67). In a lamb experiment, it was found that the relative bioavailability of Mn-Met was 120% of MnSO4 (68). This study also found that organic form Mn-Met is more suitable as Mn source for yak’s rumen fermentation in vitro.
5. Conclusion
Rumen fermentation in vitro of growing yaks was affected by Mn source and Mn level. DMD, digestive enzyme activities, and MCP content in the fermented fluid all showed relatively high values at 40–50 mg/kg Mn levels; further, amylase activity, VFAs levels, NH3-N concentration, and MCP content in Mn-Met group were mostly better than those of MnSO4 and MnCl2 groups. Therefore, Mn-Met was the optimal Mn source and 40–50 mg/kg was the optimal Mn level for yak’s rumen fermentation.
Data availability statement
The original contributions presented in the study are included in the article/supplementary material, further inquiries can be directed to the corresponding authors.
Ethics statement
The animal study was reviewed and approved by Professional Committee of Animal Use, Academy of Animal Husbandry and Veterinary Medicine in Qinghai University (QHU20150301).
Author contributions
YX, LH, and SL: conceptualization and supervision. YX: methodology. HL, PL, and YX: writing—original draft preparation and validation. HL and PL: visualization. YX, HL, PL, and LH: investigation. HL, PL, YX, XZ, BB, JC, ZZ, and CS: formal analysis. YX and LH: resources, data curation, writing—review and editing, project administration, and funding acquisition. All authors contributed to the article and approved the submitted version.
Funding
This research was funded by the The Open Project of State Key Laboratory of Plateau Ecology and Agriculture (2023-KF-04), AAU Introduction of High-level Talent Funds (RC392107), National Natural Science Foundation of China (32060766), Qinghai Province Key R&D and Transformation Program (2021-NK-126), Key Laboratory of Plateau Grazing Animal Nutrition and Feed Science of Qinghai Province (2022-ZJ-Y17), Top Talent project of “Kunlun Talents–High-level Innovation and Entrepreneurship Talents” in Qinghai Province.
Acknowledgments
The authors thank Changsha Xingjia Bioengineering Company for providing the standard samples of trace element Mn (MnSO4, MnCl2, and Met-Mn).
Conflict of interest
HL, CS, and YX were employed by Qinghai Pure Yak Biotechnology Co., LTD.
The remaining authors declare that the research was conducted in the absence of any commercial or financial relationships that could be construed as a potential conflict of interest.
Publisher’s note
All claims expressed in this article are solely those of the authors and do not necessarily represent those of their affiliated organizations, or those of the publisher, the editors and the reviewers. Any product that may be evaluated in this article, or claim that may be made by its manufacturer, is not guaranteed or endorsed by the publisher.
References
1. Joshi, S, Shrestha, L, Bisht, N, Wu, N, Ismail, M, Dorji, T, et al. Ethnic and cultural diversity amongst yak herding communities in the Asian highlands. Sustainability. (2020) 12:957. doi: 10.3390/su12030957
2. Degen, AA, Kam, M, Pandey, SB, Upreti, CR, Pandey, S, and Regmi, P. Transhumant pastoralism in yak production in the lower Mustang District of Nepal. Nomadic Peoples. (2007) 11:57–85. doi: 10.3167/np.2007.110204
3. Ma, L, Xu, S, Liu, H, Xu, T, and Zhang, X. Yak rumen microbial diversity at different forage growth stages of an alpine meadow on the Qinghai-Tibet plateau. PeerJ. (2019) 7:e7645. doi: 10.7717/peerj.7645
4. Xu, T, Xu, S, Hu, L, Zhao, N, Liu, Z, Ma, L, et al. Effect of dietary types on feed intakes, growth performance and economic benefit in Tibetan sheep and yaks on the Qinghai-Tibet plateau during cold season. PLoS One. (2017) 12:e0169187. doi: 10.1371/journal.pone.0169187
5. Yoshihara, Y, Mizuno, H, Ogura, S, Sasaki, T, and Sato, S. Increasing the number of plant species in a pasture improves the mineral balance of grazing beef cattle. Anim Feed Sci Technol. (2013) 179:138–43. doi: 10.1016/j.anifeedsci.2012.11.009
6. Shannon, MC, and Hill, GM. Trace mineral supplementation for the intestinal health of young Monogastric animals. Front Vet Sci. (2019) 6:73. doi: 10.3389/fvets.2019.00073
7. Jurjanz, S, Collas, C, Lastel, M-L, Godard, X, Archimède, H, Rychen, G, et al. Evaluation of soil intake by growing creole young bulls in common grazing Systems in Humid Tropical Conditions. Animal. (2017) 11:1363–71. doi: 10.1017/S1751731116002755
8. Fan, Q, Wang, Z, Chang, S, Peng, Z, Wanapat, M, Bowatte, S, et al. Relationship of mineral elements in sheep grazing in the Highland agro-ecosystem. Asian Australas J Anim Sci. (2020) 33:44–52. doi: 10.5713/ajas.18.0955
9. Xin, GS, Long, RJ, Guo, XS, Irvine, J, Ding, LM, Ding, LL, et al. Blood mineral status of grazing Tibetan sheep in the northeast of the Qinghai–Tibetan plateau. Livest Sci. (2011) 136:102–7. doi: 10.1016/j.livsci.2010.08.007
10. Fan, Q, Wanapat, M, and Hou, F. Mineral nutritional status of yaks (Bos Grunniens) grazing on the Qinghai-Tibetan plateau. Animals. (2019) 9:468. doi: 10.3390/ani9070468
11. Yu, Y, Mizuno, H, Yasue, H, Purevdorj, N, and Ito, TY. Nomadic grazing improves the mineral balance of livestock through the intake of diverse plant species. Anim Feed Sci Technol. (2013) 184:80–5. doi: 10.1016/j.anifeedsci.2013.06.007
12. Pajarillo, EAB, Lee, E, and Kang, D-K. Trace metals and animal health: interplay of the gut microbiota with Iron, manganese, zinc, and copper. Anim Nutr. (2021) 7:750–61. doi: 10.1016/j.aninu.2021.03.005
13. Leach, R Jr, and Muenster, A-M. Studies on the role of manganese in bone formation: I. effect upon the Mucopolysaccharide content of Chick bone. J Nutr. (1962) 78:51–6. doi: 10.1093/jn/78.1.51
14. Leach, RM, Muenster, AM, and Wien, EM. Studies on the role of manganese in bone formation. Ii. Effect upon chondroitin sulfate synthesis in Chick epiphyseal cartilage. Arch Biochem Biophys. (1969) 133:22–8. doi: 10.1016/0003-9861(69)90483-4
15. Gresakova, L, Venglovska, K, and Cobanova, K. Nutrient digestibility in lambs supplemented with different dietary manganese sources. Livest Sci. (2018) 214:282–7. doi: 10.1016/j.livsci.2018.07.001
16. Hilal, EY, Elkhairey, M, and Osman, A. The role of zinc, Manganse and copper in rumen metabolism and immune function: a review article. Open J Anim Sci. (2016) 6:304–24. doi: 10.4236/ojas.2016.64035
17. Tiwari, S, Jain, R, Mishra, U, Mishra, O, Patel, J, and Rajagopal, S. Effect of trace mineral (mineral capsule) supplementation on nutrient utilization and rumen fermentation pattern in Sahiwal cows (Bos Indicus). Indian J Anim Sci. (2000) 18:409–22. doi: 10.1016/S0739-7240(00)00059-X
18. Sonawane, S, and Arora, S. Influence of zinc supplementation on rumen microbial protein synthesis in in vitro studies [India]. Indian J Anim Sci. (1976) 46:13–8
19. Wang, S, Wang, Y, Hu, Z, Chen, Z, Fleckenstein, J, and Schnug, E. Status of Iron, manganese, copper, and zinc of soils and plants and their requirement for ruminants in Inner Mongolia steppes of China. Commun Soil Sci Plant Anal. (2003) 34:655–70. doi: 10.1081/CSS-120018966
20. Hidiroglou, M. Manganese in ruminant nutrition. Can J Anim Sci. (1979) 59:217–36. doi: 10.4141/cjas79-028
21. Council NR. Nutrient requirements of dairy cattle: 2001. Washington, DC: National Academies Press (2001).
22. Chu, GM, Komori, M, Hattori, R, and Matsui, T. Dietary Phytase increases the true absorption and endogenous fecal excretion of zinc in growing pigs given a corn-soybean meal based diet. Anim Sci J. (2009) 80:46–51. doi: 10.1111/j.1740-0929.2008.00595.x
23. Spears, JW. Trace mineral bioavailability in ruminants. J Nutr. (2003) 133:1506S–9S. doi: 10.1093/jn/133.5.1506s
24. National Academies of Sciences E, Medicine. Nutrient requirements of beef cattle. Washington, DC: The National Academies Press (2016).
25. Wanapat, M. Rumen manipulation to increase the efficient use of local feed resources and productivity of ruminants in the tropics. Asian Australas J Anim Sci. (2000) 13:59–67.
26. Ministry of Agriculture and rural Affairs of the People’s republic of China. Feeding standard of beef cattle (NY/T815-2004). Beijing, China: China Agriculture Press (2004) (In Chinese).
27. Hu, LH. Recent advances in yak nutrition. Xining, China: Qinghai People’s Press (1997) (In Chinese).
28. GB/T13885-2003. Animal feeding stuffs-determination of contents of calcium, copper, Iron, magnesium, manganese potassium, sodium and zinc-method Ssing atomic absorption spectrometry. Geneva, Switzerland: ISO International Standard (2003).
29. Liu, J-F, Feng, Y-D, and Jiang, G-B. Identical flow injection spectrophotometric manifold for determination of protein, phosphorus, calcium, chloride, copper, manganese, Iron, and zinc in feeds or premixes. J AOAC Int. (2001) 84:1179–86. doi: 10.1093/jaoac/84.4.1179
30. Menke, KH, Raab, L, Salewski, A, Steingass, H, and Schneider, W. The estimation of the digestibility and Metabolizable energy content of ruminant Feedingstuffs from the gas production when they are incubated with rumen liquor in vitro. J Agric Sci. (1979) 93:217–22. doi: 10.1017/S0021859600086305
31. Menke, KH. Estimation of the energetic feed value obtained from chemical analysis and in vitro gas production using rumen fluid. Anim Res Dev. (1988) 28:7–55.
32. Feng, ZC, and Gao, M. Improvement of the method for determination of Ammonia nitrogen content in rumen fluid by colorimetry. Anim husb feed sci. (2010) 31:37. doi: 10.16003/j.cnki.issn1672-5190.2010.z1.027. (In Chinese)
33. Zhao, X, Hao, L, Xue, Y, Degen, A, and Liu, S. Effect of source and level of dietary supplementary copper on in vitro rumen fermentation in growing yaks. Fermentation. (2022) 8:693. doi: 10.3390/fermentation8120693
34. Zhou, Z, Fang, L, Meng, Q, Li, S, Chai, S, Liu, S, et al. Assessment of ruminal bacterial and archaeal community structure in yak (Bos Grunniens). Front Microbiol. (2017) 8:179. doi: 10.3389/fmicb.2017.00179
35. Bergmann, GT. Microbial community composition along the digestive tract in forage-and grain-fed Bison. BMC Vet Res. (2017) 13:1–9. doi: 10.1186/s12917-017-1161-x
36. Getachew, G, Blümmel, M, Makkar, H, and Becker, K. In vitro gas measuring techniques for assessment of nutritional quality of feeds: a review. Anim Feed Sci Technol. (1998) 72:261–81. doi: 10.1016/s0377-8401(97)00189-2
37. Blaxter, KL. The energy metabolism of ruminants. Energy Metabolism Ruminants. (1962) 139. doi: 10.1136/vr.139.14.350-a
38. Melo, LQ, Costa, SF, Lopes, F, Guerreiro, MC, and Pereira, MN. Rumen Morphometrics and the effect of Digesta Ph and volume on volatile fatty acid absorption. J Anim Sci. (2013) 91:1775–83. doi: 10.2527/jas.2011-4999
39. Wales, WJ, Kolver, ES, Thorne, PL, and Egan, AR. Diurnal variation in ruminal Ph on the digestibility of highly digestible perennial ryegrass during continuous culture fermentation. J Dairy Sci. (2004) 87:1864–71. doi: 10.3168/jds.S0022-0302(04)73344-5
40. Geishauser, T, Linhart, N, Neidl, A, and Reimann, A. Factors associated with ruminal Ph at herd level. J Dairy Sci. (2012) 95:4556–67. doi: 10.3168/jds.2012-5380
41. Eun, J-S, and Beauchemin, K. Effects of a proteolytic feed enzyme on intake, digestion, ruminal fermentation, and Milk production. J Dairy Sci. (2005) 88:2140–53. doi: 10.3168/jds.s0022-0302(05)72890-3
42. Van Soest, PJ. Nutritional ecology of the ruminant. Ithaca, NY: Cornell University Press (1994).
43. Grant, RH, and Mertens, DR. Influence of buffer Ph and raw corn starch addition on in vitro Fiber digestion kinetics. J Dairy Sci. (1992) 75:2762–8. doi: 10.3168/jds.S0022-0302(92)78039-4
44. Chamberlain, C, and Burroughs, W. Effect of fluoride, magnesium and manganese ions on in vitro cellulose digestion by rumen microorganisms. J Anim Sci. (1962) 21:428–32. doi: 10.1103/PhysRevC.79.054317
45. Martinez, A, and Church, D. Effect of various mineral elements on in vitro rumen cellulose digestion. J Anim Sci. (1970) 31:982–90. doi: 10.2527/jas1970.315982x
46. Kišidayová, S, Pristaš, P, Zimovčáková, M, Blanár Wencelová, M, Homol’ová, L, Mihaliková, K, et al. The effects of high dose of two manganese supplements (organic and inorganic) on the rumen microbial ecosystem. PLoS One. (2018) 13:e0191158. doi: 10.1371/journal.pone.0191158
47. Weiss, WP. Estimation of digestibility of forages by laboratory methods. Forage Quality Eval Utilization. (1994):644–81. doi: 10.2134/1994.foragequality.c16
48. Arelovich, H, Owens, F, Horn, G, and Vizcarra, J. Effects of supplemental zinc and manganese on ruminal fermentation, forage intake, and digestion by cattle fed prairie Hay and urea. J Anim Sci. (2000) 78:2972–9. doi: 10.2527/2000.78112972x
49. He, Y, Wang, H, Yu, Z, Niu, W, Qiu, Q, Su, H, et al. Effects of the gender differences in cattle rumen fermentation on anaerobic fermentation of wheat straw. J Clean Prod. (2018) 205:845–53. doi: 10.1016/j.jclepro.2018.09.156
50. Bergman, E. Energy contributions of volatile fatty acids from the gastrointestinal tract in various species. Physiol Rev. (1990) 70:567–90. doi: 10.1152/physrev.1990.70.2.567
51. Bach, A, Yoon, IK, Stern, MD, Jung, HG, and Chester-Jones, H. Effects of type of carbohydrate supplementation to lush pasture on microbial fermentation in continuous culture. J Dairy Sci. (1999) 82:153–60. doi: 10.3168/jds.S0022-0302(99)75219-7
52. Phillips, RW, and Black, AL. The effect of volatile fatty acids on plasma glucose concentration. Comp Biochem Physiol. (1966) 18:527,IN3,33–32,IN3,36. doi: 10.1016/0010-406X(66)90237-4
53. Janssen, PH. Influence of hydrogen on rumen methane formation and fermentation balances through microbial growth kinetics and fermentation thermodynamics. Anim Feed Sci Technol. (2010) 160:1–22. doi: 10.1016/j.anifeedsci.2010.07.002
54. Giger-Reverdin, S, Rigalma, K, Desnoyers, M, Sauvant, D, and Duvaux-Ponter, C. Effect of concentrate level on feeding behavior and rumen and blood parameters in dairy goats: relationships between behavioral and physiological parameters and effect of between-animal variability. J Dairy Sci. (2014) 97:4367–78. doi: 10.3168/jds.2013-7383
55. Ma, J, Mujtaba Shah, A, Shao, Y, Wang, Z, Zou, H, Hu, R, et al. Effects of yeast Cell Wall on the growth performance, ruminal fermentation, and microbial Community of Weaned Calves. Livest Sci. (2020) 239:104170. doi: 10.1016/j.livsci.2020.104170
56. El-Esawy, GS, Riad, W, El-Dein, AM, Ali, M, and Gaafar, H. Effect of supplementary zinc and manganese methionine chelates on productive and reproductive performance of dairy Friesian cows. Archiva Zootechnica. (2017) 96:289–302. doi: 10.21608/ejar.2018.132846
57. Shakweer, I, Mustafa, G, Ahmed, G, and Ismail, M. Effect of zinc or/and manganese methionine supplements on performance of lactating buffaloes. J Anim Poul Prod. (2010) 1:589–602. doi: 10.21608/jappmu.2010.86271
58. Owens, FN, and Basalan, M. Ruminal Fermentation. Rumenology. (2016):63–102. doi: 10.1007/978-3-319-30533-2_3
59. Stern, M, and Hoover, WH. Methods for determining and factors affecting rumen microbial protein synthesis: a review. J Anim Sci. (1979) 49:1590–603. doi: 10.2527/jas1979.4961590x
60. Satter, L, and Slyter, L. Effect of Ammonia concentration on rumen microbial protein production in vitro. Br J Nutr. (1974) 32:199–208. doi: 10.1079/bjn19740073
61. Davis, C. Some aspects of feeding high producing dairy cows. J Dairy Sci. (1980) 63:873–85. doi: 10.3168/jds.S0022-0302(80)83021-9
62. Clark, J, and Davis, C. Future improvement of Milk production: potential for nutritional improvement. J Anim Sci. (1983) 57:750–64. doi: 10.2527/jas1983.573750x
64. Bao, K, Wang, K, Wang, X, Zhang, T, Liu, H, and Li, G. Effects of dietary manganese supplementation on nutrient digestibility and production performance in male sika deer (Cervus Nippon). Anim Sci J. (2017) 88:463–7. doi: 10.1111/asj.12657
65. Spears, JW. Organic trace minerals in ruminant nutrition. Anim Feed Sci Technol. (1996) 58:151–63. doi: 10.1016/0377-8401(95)00881-0
66. Gresakova, L, Venglovska, K, and Cobanova, K. Dietary manganese source does not affect Mn, Zn and cu tissue deposition and the activity of manganese-containing enzymes in lambs. J Trace Elem Med Biol. (2016) 38:138–43. doi: 10.1016/j.jtemb.2016.05.003
67. Wright, C, Spears, J, and Webb, K Jr. Uptake of zinc from zinc sulfate and zinc Proteinate by ovine ruminal and Omasal epithelia. J Anim Sci. (2008) 86:1357–63. doi: 10.2527/jas.2006-650
Keywords: manganese, rumen fermentation in vitro, digestive enzyme activities, volatile fatty acids, microbial protein
Citation: Lu H, Liu P, Liu S, Zhao X, Bai B, Cheng J, Zhang Z, Sun C, Hao L and Xue Y (2023) Effects of sources and levels of dietary supplementary manganese on growing yak’s in vitro rumen fermentation. Front. Vet. Sci. 10:1175894. doi: 10.3389/fvets.2023.1175894
Edited by:
Mirja Wilkens, Leipzig University, GermanyCopyright © 2023 Lu, Liu, Liu, Zhao, Bai, Cheng, Zhang, Sun, Hao and Xue. This is an open-access article distributed under the terms of the Creative Commons Attribution License (CC BY). The use, distribution or reproduction in other forums is permitted, provided the original author(s) and the copyright owner(s) are credited and that the original publication in this journal is cited, in accordance with accepted academic practice. No use, distribution or reproduction is permitted which does not comply with these terms.
*Correspondence: Lizhuang Hao, bGl6aHVhbmdoYW8xMTIyQGZveG1haWwuY29t; Yanfeng Xue, eHVleWFuZmVuZzE5OTBAMTYzLmNvbQ==
†These authors have contributed equally to this work and share first authorship