- 1College of Animal Science and Technology, Yunnan Agricultural University, Kunming, Yunnan, China
- 2College of Animal Science and Veterinary Medicine, Yunnan Vocational and Technical College of Agriculture, Kunming, Yunnan, China
- 3Kunming CHIA TAI Co., Ltd., Kunming, Yunnan, China
With the rapid development of poultry industry and the highly intensive production management, there are an increasing number of stress factors in poultry production. Excessive stress will affect their growth and development, immune function, and induce immunosuppression, susceptibility to a variety of diseases, and even death. In recent years, increasing interest has focused on natural components extracted from plants, among which plant polysaccharides have been highlighted because of their various biological activities. Plant polysaccharides are natural immunomodulators that can promote the growth of immune organs, activate immune cells and the complement system, and release cytokines. As a green feed additive, plant polysaccharides can not only relieve stress and enhance the immunity and disease resistance of poultry, but also regulate the balance of intestinal microorganisms and effectively alleviate all kinds of stress faced by poultry. This paper reviews the immunomodulatory effects and molecular mechanisms of different plant polysaccharides (Atractylodes macrocephala Koidz polysaccharide, Astragalus polysaccharides, Taishan Pinus massoniana pollen polysaccharide, and alfalfa polysaccharide) in poultry. Current research results reveal that plant polysaccharides have potential uses as therapeutic agents for poultry immune abnormalities and related diseases.
1. Introduction
The poultry industry is perennially plagued by many diseases, including infectious bursal disease, Salmonella, avian influenza, Newcastle disease, black lung, and mixed infections of various diseases, with a new disease observed in China's poultry industry almost every two years (1–4). In addition, poultry farming faces a considerable number of environmental stresses daily, including stressors, toxic substances, drug effects, inadequate nutritional factors, viruses, and diseases, which lead to low immune levels or dysplastic immune organs, resulting in abnormal immune function (5–8). Owing to imperfections in the immune system, the immune barrier of the body is easily broken and certain pathogenic factors can easily invade the body (9, 10). The widespread prevalence of many epidemic diseases, limited knowledge on their pathogenesis, and high feeding density of rural chicken farms have led to the abuse of antibiotics, which has led to unhealthy practices in the poultry industry and huge economic losses (11–13).
With prohibitions on antibiotics use and the promotion of green farming, replacing antibiotics with green additives has become a research hotspot at home and abroad. Many studies have shown that plant polysaccharides can regulate the growth and development of immune organs and enhance the body's resistance to bacteria and viruses (14, 15). Polysaccharides are key factors in cell surface signal recognition, cell-to-cell signal transmission, and immune-related responses, and they can stimulate innate and cellular immunity through interactions with immune cells, regulate the immune response in an appropriate manner, and promote the immune system against a variety of adverse factors (16).
The immune organs in poultry are mainly composed of the thymus, spleen, and bursa of Fabricius. Immune organs are sites where various immune cells differentiate, mature, and produce an immune response. These immune cells include macrophages, dendritic cells, T lymphocytes, and B lymphocytes (17, 18). Immune cells produce a variety of cytokines and chemokines that enhance the immune response and protect the body (19). In addition, improving immunity is an effective strategy to reduce disease susceptibility and enhance defense against pathogens, and stimulating macrophages, lymphocytes, dendritic cells, and other immune cells plays a vital role in enhancing immunity (20, 21). Many previous studies have shown that plant polysaccharides can regulate immunity by increasing the index of immune organs and activating cellular and humoral immunity (22). In addition, plant polysaccharides can alleviate oxidative stress, liver injury, and immunosuppression induced by various adverse conditions. This study mainly reviewed the effect of plant polysaccharides on poultry immune regulation and the underlying molecular mechanisms to provide a reference basis for the application of these substances in poultry breeding.
2. Plant polysaccharide
2.1. Atractylodes macrocephala koidz polysaccharide
The polysaccharide of the traditional Chinese medicine Atractylodes macrocephala Koidz (PAMK) is an important component of this plant. The main extraction methods are hot water extraction, alcohol precipitation, and ultrasonicassisted enzymatic extraction (23, 24). Different extraction methods produce different components, including arabinose, glucose, galactose, and mannose (25, 26). PAMK is considered a potential therapeutic drug for various diseases in livestock industry because of its biological activities, such as the promotion of immunomodulation (27), anti-tumor (28), anti-inflammation (29), and hypoglycemic effects (30) (Table 1). Importantly, recent studies have found that PAMK plays a useful role in poultry breeding, including alleviating immunosuppression, reducing heat stress damage, maintaining immune organs, liver functions, and intestinal barriers, and improving vaccine efficacy (Figure 1).
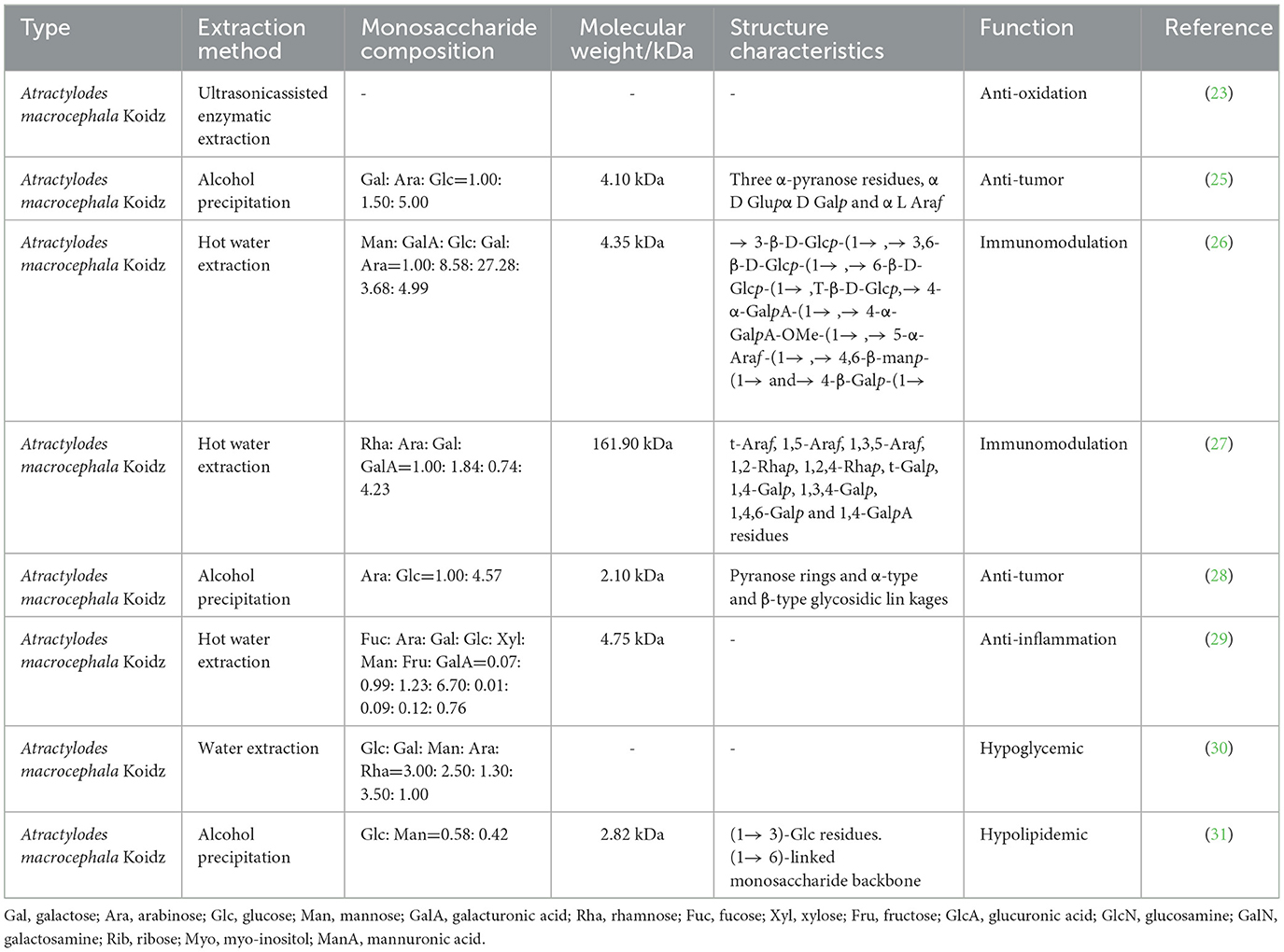
Table 1. Structure and structure-activity-relationship of polysaccharide from Atractylodes macrocephala Koidz.
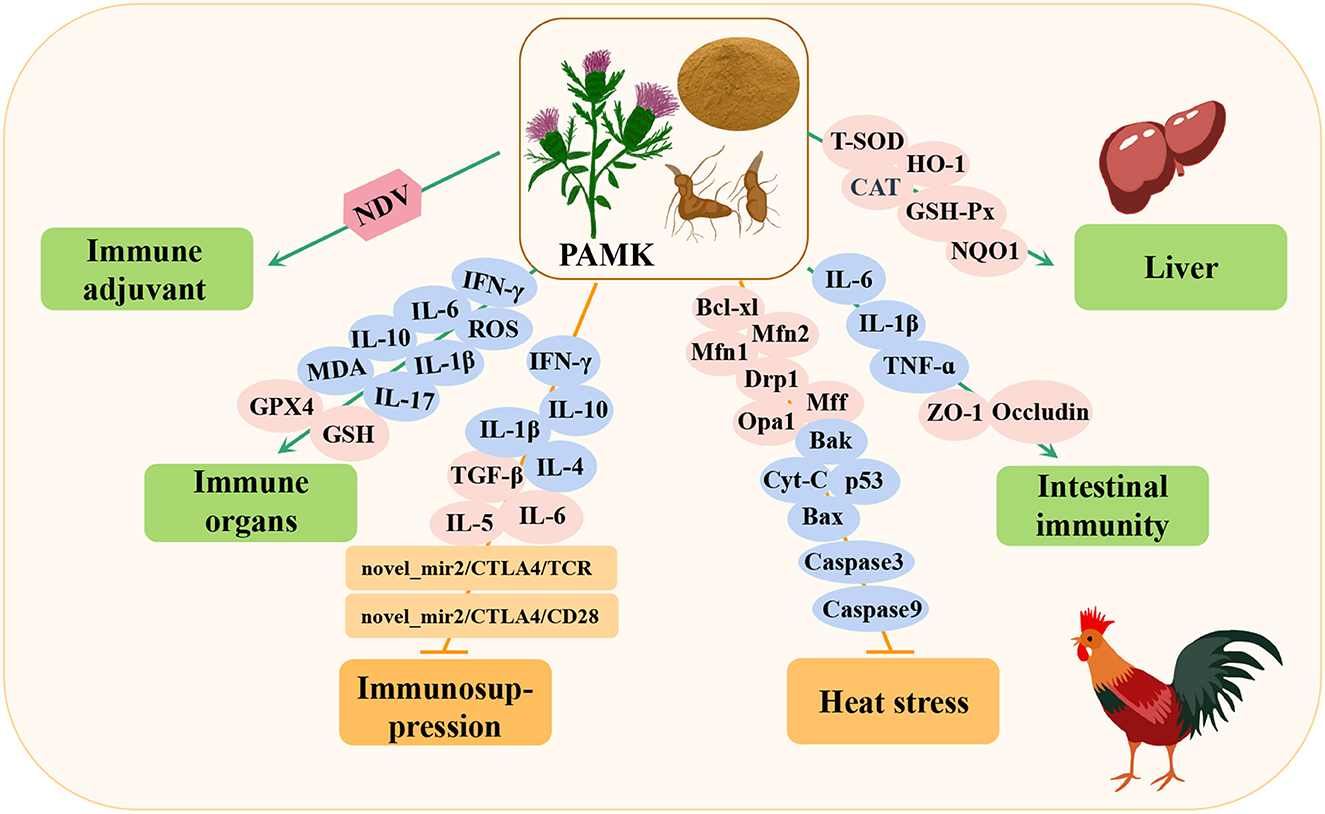
Figure 1. Immunomodulation and protection of immune organs by Atractylodes macrocephala Koidz polysaccharide (PAMK) in poultry. NDV, newcastle disease virus; IFN-γ, interferon-gamma; IL-6, interleukin-6; IL-10, interleukin-10; MDA, malonaldehyde; ROS, reactive oxygen species; IL-1β, interleukin 1 beta; IL-17, interleukin-17; GSH, glutathione; GPX4, glutathione peroxidase 4; IL-4, interleukin-4; TGF-β, transforming growth factor-β; IL-5, interleukin-5; Bcl-xl, BCL2-like 1; Mfn1, mitofusin 1; Mfn2, mitofusin 2; Drp1, dynamin-related protein 1; Opa1, mitochondrial dynamin like GTPase; Mff, mitochondrial fission factor; Bak, BCL2 antagonist/killer 1; Bax, BCL2-associated x; Cyt-C, cytoplasmic cytochrome c; p53, tumor protein p53; TNF-α, tumor necrosis factor-α; ZO-1, zonula occludes protein; CAT, catalase; HO-1, heme oxygenase 1; GSH-Px, glutathione peroxidase; NQO1, NAD(P)H quinone dehydrogenase 1; T-SOD, total superoxide dismutase.
Studies have shown that immunosuppression in poultry is very common and usually caused by infection, stress, and abuse of antibiotics and chemicals. In the poultry immunosuppression model, PAMK can reduce damage to immune organs, alleviate the decreased function and imbalances of immune cells, maintain the balance of immune cytokines, and help restore the morphology of immune organs (32–34). In addition, heat stress can lead to immune organ dysfunction, apoptosis, and oxidative damage in poultry, resulting in decreased immunity. However, PAMK can improve immune system dysfunction and oxidative damage caused by heat stress by increasing the level of cytokines, reducing oxidative stress, enhancing mitochondrial function, and inhibiting apoptosis (35–37). Studies have shown that PAMK can alleviate the injury to immune organs and enteritis-associated inflammation induced by lipopolysaccharides (LPSs), reduce the level of inflammatory factors, and improve disorders among intestinal flora (31, 38). In addition, the poultry liver is the largest reticular endothelial cell phagocytosis system, and it plays an irreplaceable role in immune function through phagocytosis, isolation, and elimination of invasive and endogenous antigens. However, PAMK can improve liver injury by regulating the activity of antioxidant enzymes and liver lipid metabolism (39). Studies have also shown that PAMK can increase the antibody titer of poultry vaccinated with the Newcastle disease (ND) vaccine (40, 41).
At the molecular level, PAMK can activate T lymphocytes in the thymus through the novel_mir2/CTLA4/TCR and novel_mir2/CTLA4/CD28 signaling pathways and inhibit the transcription of proinflammatory cytokines, such as interleukin 1 beta (IL-1β), interferon-gamma (IFN-γ), interleukin-4 (IL-4), and interleukin-10 (IL-10). Increased expression of cytokines, such as transforming growth factor-β (TGF-β), interleukin-6 (IL-6), and interleukin-5 (IL-5), maintains the balance of cytokines in the body to alleviate immunosuppression (32–34). In a heat stress model, PAMK was shown to maintain the number of mitochondria and reduce apoptosis by increasing the expression of the Mff, Drp1, Opa1, Mfn1, and Mfn2 genes (35). Moreover, the expression of p53, Cyt-C, Bax, Bak, Caspase3, and Caspase9 proapoptotic genes increased the expression of anti-apoptotic Bcl-xL gene and alleviated the expression of heat shock proteins, such as heat shock protein 60 (HSP60) and HSP70, to prevent further injury caused by heat stress, relieve the state of heat stress, and improve immunity (36, 37). In addition, PAMK scavenges lipid peroxidation reactive oxygen species (ROS) and malonaldehyde (MDA) by increasing the expression of GPX4, increasing the content of its cofactor glutathione (GSH), and reducing the expression of proinflammatory cytokines IFN-γ, IL-1β, IL-6, IL-10, and IL-17, thereby improving the antioxidant capacity of the body and reducing immune organ damage caused by LPSs (31). Similarly, PAMK increases tight junction occlusal proteins and ZO-1, reduces the levels of endotoxins, CRP, and proinflammatory factors (IL-1β, TNF-α, and IL-6), and improves the stability of intestinal flora abundance, thereby alleviating intestinal damage and microbial disorders caused by LPSs (38). In addition, Miao et al. demonstrated that PAMK can reduce liver injury by reducing oxidative stress-related insulin resistance or by directly changing the expression of genes involved in liver lipid metabolism, such as catalase (CAT), heme oxygenase 1 (HO-1), glutathione peroxidase (GSH-Px), and NQO1 (39). These studies demonstrated that PAMK plays an important role in immune regulation in poultry and mediates protection against immune organ injury.
2.2. Astragalus polysaccharide
Astragalus polysaccharide (APS) is one of the most important natural active components in Astragalus, and it is a water-soluble heteropolysaccharide extracted from the stem or dry root of Astragalus and one of the most important natural active components of this plant. APS extraction methods mainly include hot water extraction, cold water extraction, alcohol precipitation and enzyme-assisted extraction (42, 43). The components of different extraction methods are also slightly different, including mannose, glucose, xylose, arabinose, galactose, glucuronic acid, and rhamnose (42, 44). APS has many beneficial properties, such as anti-tumor (44), antioxidant (45), immunomodulatory (46, 47), anti-inflammatory (48), hypoglycemic (49), and other biological activities (Table 2). At present, the immunomodulatory function of APS has received increasing research attention, especially in poultry breeding (Figure 2).
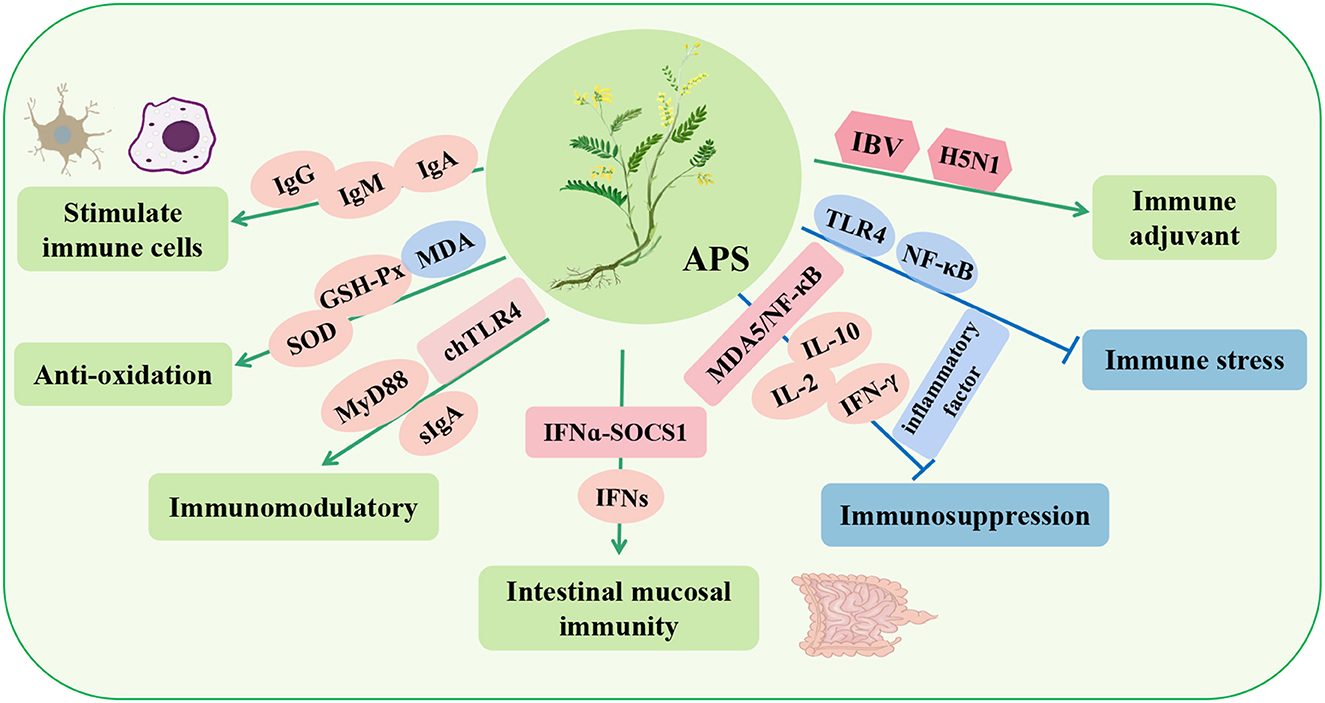
Figure 2. Immunomodulatory effects and mechanisms of Astragalus polysaccharide (APS) in poultry. IgA, immunoglobulin A; IgM, immunoglobulin M; IgG, immunoglobulin G; SOD, superoxide dismutase; MyD88, myeloiddifferentiationfactor88; chTLR4, toll-like receptor 4; sIgA, secretory immunoglobulin A; IFNs, type-I-interferon; NF-κB, nuclear factor-κB; MDA5, interferon induced with helicase C domain 1; IL-2, interleukin-2; IBV, infectious bronchitis virus; H5N1, avian influenza.
Studies have shown that APS can stimulate the proliferation of T and B lymphocytes, promote the maturation of macrophages (50) and dendritic cells (51), and exert immune functions. Similarly, dietary APS significantly increases the activities of superoxide dismutase (SOD) and GSH-Px; increases the levels of serum immunoglobulin G (IgG), immunoglobulin M (IgM), and immunoglobulin A (IgA); and decreases the content of MDA, thus improving the immune response of broilers (52). Zhang et al. (53) found that APS upregulated chTLR4 in the bursa, MyD88-independent genes in downstream molecules, and secretory immunoglobulin A (sIgA) in the gut, thus demonstrating that APS can play an immunoregulatory role by activating the chTLR4 pathway (53). Interestingly, continuous inclusion in the male parent diet of APS (10 g/kg/d) could upregulate the serum level of type-I-interferon (IFNs) in the offspring, and APS could induce the intergenerational endotoxin tolerance-like effect and enhance the intestinal mucosal immunity of the offspring by activating the IFNα-SOCS1 pathway (54, 55).
In addition, in a poultry immunosuppression model induced by the heavy metal cadmium (Cd), APS effectively alleviated the immune imbalance induced by Cd by regulating the MDA5/NF-κB signaling pathway, reducing the expression of proinflammatory cytokines (IL-1β, IL-6, and TNF-α), and increasing the activities of antioxidant enzymes (SOD and GSH-Px) (56). Similarly, APS can stimulate the proliferation of lymphocytes and goblet cells of the intestinal epithelium in poultry, upregulate the mRNA expression of IL-2, IL-10, and IFN-γ, and increase the height of villi and the content of lgA, thus alleviating the immunosuppression of the intestine and body (57, 58). Liu et al. (59) also found that APS can reduce the transcription of TLR4 and NF-κB to inhibit the expression of proinflammatory cytokines, thereby improving the immune stress response (59). In addition, APS can be used as a vaccine adjuvant for avian infectious bronchitis virus (IBV) and H5N1 avian influenza. It can increase the titer of specific antibodies and the number of lymphocytes and increase the mRNA expression levels of IL-1β, IL-2, IL-8, and TNF-α in a dose-dependent manner to better prevent virus infection (60, 61). These studies showed that APS can enhance immune regulation, improve immunosuppression, and enhance vaccine titers in poultry.
2.3. Taishan Pinus massoniana pollen polysaccharide
Taishan Pinus massoniana pollen polysaccharide (TPPPS) is obtained from Taishan Pinus massoniana pollen via water extraction and ethanol precipitation, and it has multiple effects. It is composed of mannose, ribose, xylose, glucuronic acid, galacturonic acid, glucose, galactose, and arabinose (62). Many studies have shown that TPPPS has a wide range of biological activities, such as antioxidation, immune regulation, antivirus, and liver protection activities (63, 64) (Table 3). In addition, an increasing number of studies has found that TPPPS has a variety of beneficial effects on immune regulation in poultry (Figure 3).
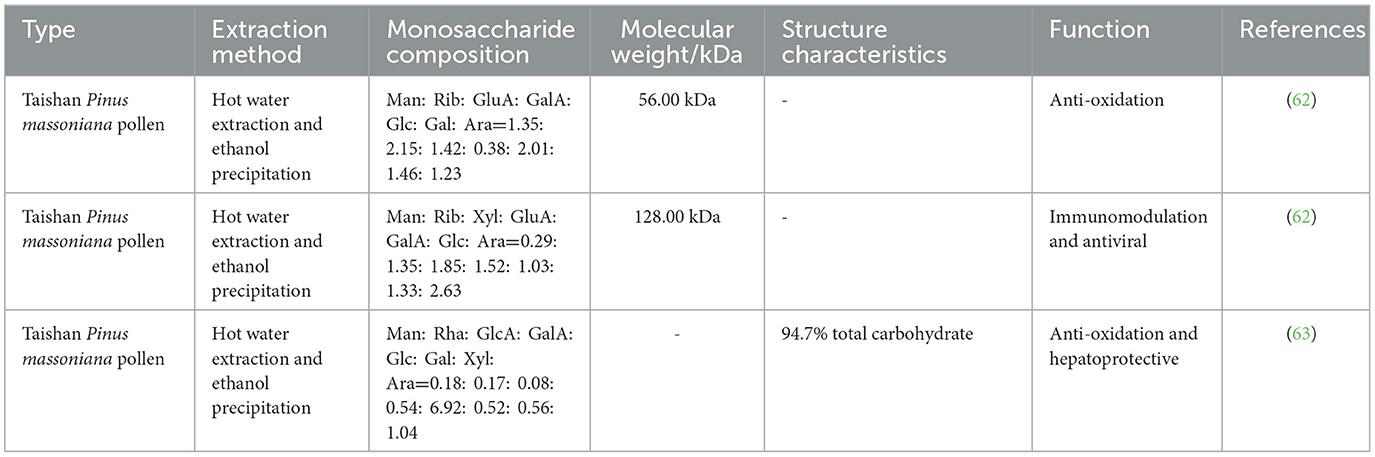
Table 3. Structure and structure-activity-relationship of polysaccharide from Taishan Pinus massoniana pollen.
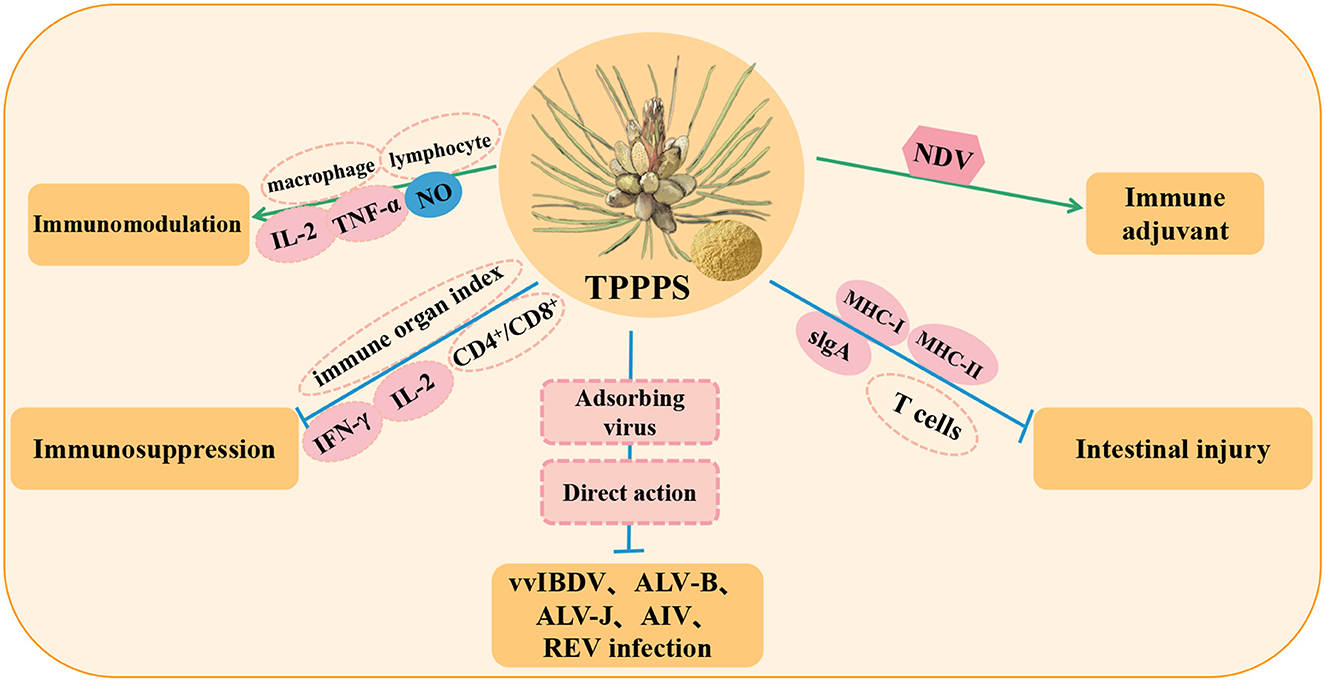
Figure 3. Immunomodulation and antiviral effects of Taishan Pinus massoniana pollen polysaccharide (TPPPS) in poultry. NO, nitrous oxide; vvIBDV, very virulent infectious bursal disease virus; ALV-B, avian leukosis virus subgroup B; ALV-J, subgroup J avian leucosis virus; AIV, avian influenza virus; REV, reticuloendotheliosis virus; MHC-I, major histocompatibility complex class I; MHC-II, major histocompatibility complex class II.
A study reported that TPPPS can promote the proliferation and activation of poultry macrophages and enhance poultry resistance to pathogens by stimulating and activating lymphocytes and immunomodulatory functions by releasing cytokines IL-2 and TNF-α and increasing the chemical signaling mediator NO (65). Simultaneously, a proteomic study on peripheral blood lymphocytes of chickens treated with TPPPS showed that the differentially expressed proteins in the TPPPS group were related to host innate immune response and stress-induced immune response (66). The study also showed that in the model of poultry immunosuppression, TPPPS significantly enhanced the immune organ index of chickens, which enhanced the immune ability, promoted the differentiation and proliferation of CD4+ and CD8+T lymphocytes, and significantly increased the levels of cytokines IL-2 and IFN-γ to mediate the immune response and enhance the immune system function of chickens (67, 68). In addition, in an intestinal injury model, TPPPS promoted the renewal of intestinal epithelial cells and induced intestinal mucosal immunity to produce a large amount of slgA to form a protective barrier and activate the intestinal mucosal immune system. The antigen presentation molecule MHC can present antigens to helper T cells and activate adaptive immunity, while TPPPS promotes the increase of MHC-I genes (BF1 and BF2) and MHC-II genes (BLA, BLB1, and BLB2) in intestinal tissue. Thus, upregulated MHC promotes antigen presentation, drives the activation of immature T cells (naive T), and rapidly differentiates effector T cells, regulatory T cells, cytotoxic T cells, and memory T cells to induce an immune response, thereby alleviating intestinal injury in poultry (69).
In addition, TPPPS can effectively inhibit infection by a variety of poultry viruses and bacteria, including infectious bursal disease virus (vvIBDV) (70), avian leukosis virus subgroup B (ALV-B) (62), subgroup J avian leukosis virus (ALV-J) (71–73), H9N2 subtype avian influenza virus (AIV) (74), reticuloendotheliosis virus (REV) (67), Proteus mirabilis (75), and Bordetella avium (76). Many studies have shown that TPPPS can effectively inhibit viral infection and host cell replication and significantly reduce virus shedding and viral load in immune organs. The antiviral mechanism may be the binding of TPPPS to the adsorption site of virus particles on the cell surface, the inhibition of virus adsorption to cells by the direct interaction between the virus and polysaccharide, or both effects at the same time. TPPPS can also be used as a vaccine adjuvant to improve the antibody titer of poultry vaccinated with ND vaccine (68). Moreover, TPPPS mixed with pathogen vaccine and inoculated into chicken significantly increased the serum antibody response and immune protection against bacterial infection (75). These results suggest that TPPPS can improve the immune system, promote the immune response, and enhance the activity of lymphocytes, thus indicating that it is a potential immune enhancer against viral and bacterial infections.
2.4. Alfalfa polysaccharide
Alfalfa polysaccharide (AP) is a bioactive compound extracted from alfalfa, and it can be extracted by hot water extraction, alcohol precipitation and complex enzyme-assisted extraction (77–79). The components extracted by each method are slightly different and include caramel, fucose, arabinose, glucose, galactose, glucuronic acid, rhamnose, and mannose. AP has many biological functions, such as immune regulation (77), anti-inflammation (80), antioxidation (79), anticancer (81), and neuroprotective (82) (Table 4). AP has been reported to promote the proliferation of lymphocytes and dendritic cells and enhance the killing activity of NK cells in mice (83). It can also enhance the immune function of macrophages by promoting their proliferation and stimulating the secretion of NO, TNF-α, and IL-6 through the MAPK and NF-κB signaling pathways (84). Similarly, AP can activate B cells through the TLR4-MyD88 signaling pathway and increase B cell IgM production through the MAPK/p38 pathway (85). Recently, many researchers have reported that AP has a significant effect on poultry (Figure 4).
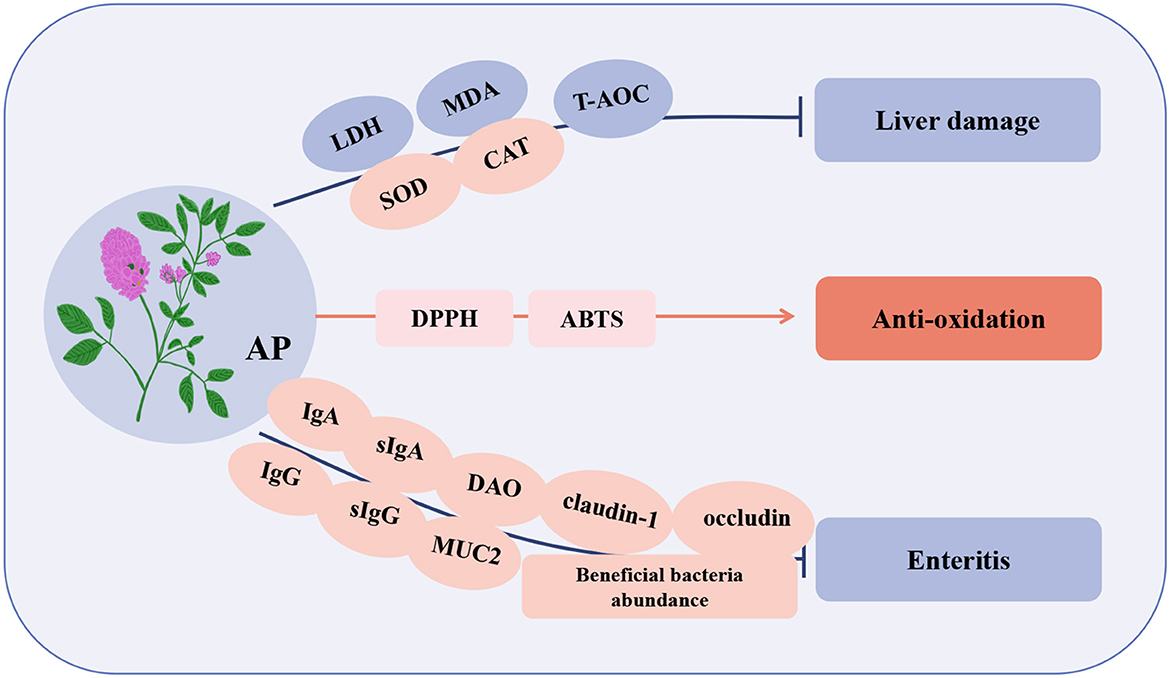
Figure 4. Immunomodulatory effects of Alfalfa polysaccharide (AP) in poultry. LDH, lactate dehydrogenase; T-AOC, total antioxidant capacity; DPPH, 2,2-diphenyl-1-picrylhydrazyl; ABTS, 3-ethylbenzothiazoline-6-sulfonate; sIgG, secretory immunoglobulin G; DAO, diamine oxidase; MUC2, mucin 2.
A study reported that AP can significantly promote the proliferation of lymphocytes in blood and spleen of broilers, and the effect of 20 μg/mL is the best. Compared with the control group, the T and B lymphocytes of 42-day-old spleen increased by 38.37 and 19.89%, respectively (86). In poultry breeding, Salmonella causes enteritis, decreased immunity, and organ damage in poultry and is also a zoonotic pathogen that is very harmful to the poultry industry (87). After poultry infected with Salmonella were fed AP, the contents of IgG and IgA in serum, sIgA and sIgG in duodenal mucosa, the activity of diamine oxidase (DAO) in intestine and the expression of tight junction protein (claudin-1, occludin, and MUC2) in jejunum increased, indicating that AP improved the immune status and intestinal barrier function of broilers. In addition, AP promotes the abundance of intestinal beneficial bacteria (Bacteroides, Pasteurella, Butyricomonas, and Puccinellidae) increased, the number of core bacteria related to body weight increased, and the intestinal morphology and intestinal mucosal barrier function were improved, thus improving the overall resistance of poultry (88). Studies have shown that the liver is an important organ for detoxification and immune regulation, and its health is a fundamental indicator of poultry health. An oxidative stress model of hepatocytes induced by hydrogen peroxide (H2O2) in vitro showed that lactate dehydrogenase (LDH) and MDA are sensitive indicators of oxidative damage in hepatocytes while AP induces a decrease in LDH and MDA, thus protecting the integrity of the hepatocyte membrane and normal oxidative state. At the same time, AP overcomes the oxidative damage induced by H2O2 by increasing the enzyme activities of SOD and CAT and the non-enzymatic total antioxidant capacity (T-AOC) of hepatocytes, thus protecting the hepatocytes of laying hens (89). Studies have shown that the antioxidant activity of natural compounds can be reflected by the scavenging of DPPH and ABTS free radicals. However, AP has strong scavenging ability for DPPH and ABTS free radicals, indicating that AP has high antioxidant activity (79). In summary, AP has the advantages of enhancing immune regulation and promoting growth performance and antioxidation, and it has no toxicity or other side effects in animals (83). Thus, it can be used as a potential natural substitute for antibiotics.
2.5. Epimedium polysaccharide
Epimedium polysaccharide (EPS) is one of the main active components of Epimedium and can be extracted by hot water extraction, alcohol precipitation and ethanol extraction (90, 91). It is mainly composed of mannose, rhamnose, glucuronic acid, galactosamine, glucose, galactose, arabinose, and fructose (92). EPS has many beneficial properties, such as antioxidation (93), anti-tumor (94), immunomodulation (95), antimicrobial (93), and anti-inflammation (96) (Table 5). Studies have shown that EPS has an immunomodulatory effect on poultry and can alleviate immunosuppression (Figure 5).
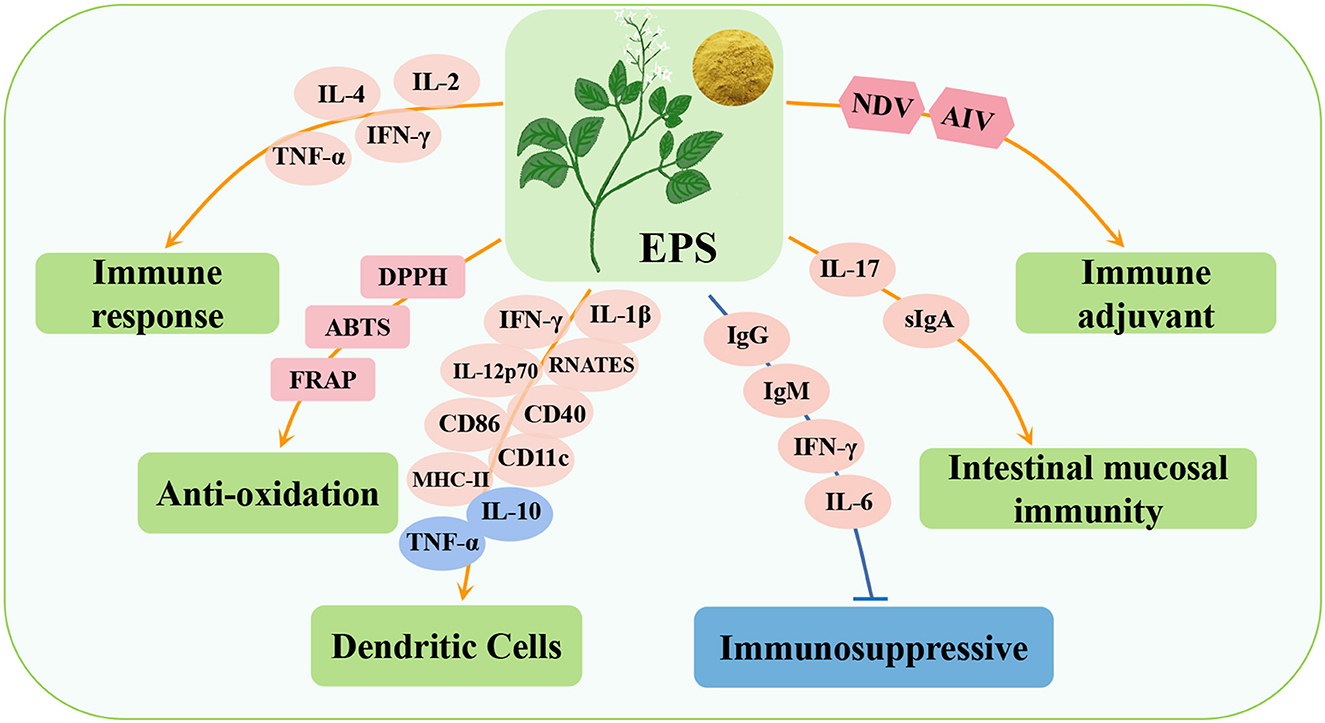
Figure 5. Immunomodulatory effects of Epimedium polysaccharide (EPS) in poultry. FRAP, ferric ion reducing antioxidant power; IL-12p70, interleukin-12p70; RNATES, regulated on activation normal T-cells expressed and secreted; CD86, CD86 molecule; CD40, CD40 molecule; CD11c, integrin subunit alpha X.
Studies have shown that EPS can activate specific and non-specific immune responses in poultry. It promotes the proliferation of spleen lymphocytes and the secretion of immune cytokines (IL-2, IL-4, IFN-γ, and TNF-α), thus enhancing the specific immune response (95). Simultaneously, EPS can promote the maturation of the most powerful antigen-presenting dendritic cells in non-specific immunity, regulate the secretion of related factors (increasing the secretion of IIL-1β, IL-12p70, IFN-γ, and RNATES, and inhibiting the secretion of IL-10 and TNF-α), and promote cell maturation by increasing the expression of key surface molecules (MHC-II, CD40, and CD86) and inhibiting dendritic cell phagocytosis (97). In addition, EPS has strong scavenging ability for DPPH and ABTS free radicals and strong iron reduction ability in ferric ion reducing antioxidant power (FRAP) experiments, indicating that EPS has strong antioxidant ability and has the potential to be developed as a natural antioxidant (90, 91). Studies have also shown that EPS can enhance the immunity of intestinal mucosal cells by promoting IL-17 and sIgA secretion. IL-17 plays an important role in protection from both extracellular bacteria and fungi, as well as viruses that infect cells of the mucosal tracts. sIgA is the main immunoglobulin in the intestinal mucosa, which can kill harmful bacteria, neutralize the virus and prevent the virus from adsorbing to susceptible cells (97, 98). In addition, in the immunosuppressive model, the synergistic immune effect of the mixture of Epimedium polysaccharides and propolis flavonoids (EPA) was higher than that of propolis flavonoids alone, which could promote lymphocyte proliferation, up-regulate the titers of antibody, IFN-γ, IL-6, IgG and IgM, and promote the development of immune organs of CTX immunosuppressed chicks (99). Similarly, a large number of studies have shown that EPA can significantly inhibit Newcastle disease virus (NDV) and AIV infections in poultry cells and improve the antibody titer of the vaccine (100–102). These findings suggest that EPS has a significant effect on immune regulation in poultry.
2.6. Salvia miltiorrhiza polysaccharides
Salvia miltiorrhiza polysaccharides (SMPs) are among the main active components of the dried roots and rhizomes of Salvia miltiorrhiza and can be extracted by hot water extraction, ultrasound-assisted complex enzyme method, and microwave-assisted extraction (103–105). The components of the different extraction methods are slightly different and mainly composed of natural polymers, such as glucose, galactose, fructose, arabinose, mannose, and galacturonic acid (103, 105). SMPs are widely used to treat many diseases because of their various biological activities such as antivirus (106), intestinal immunity (105), anti-inflammation (107), anti-tumor (104), anti-oxidation (108), and liver protection (109) (Table 6). Studies have shown that SMPs can promote the proliferation of mouse T lymphocytes by activating the TLR-mediated MAPK and NF-κB signaling pathways and play an immunomodulatory role (110, 111). In addition, SMPs can improve rat mastitis induced by Staphylococcus aureus by inhibiting the activation of the NF-κB and MAPK signaling pathways (107). In recent years, an increasing number of researchers have studied the role of SMPs in the immune regulation of poultry and their potential mechanism (Figure 6).
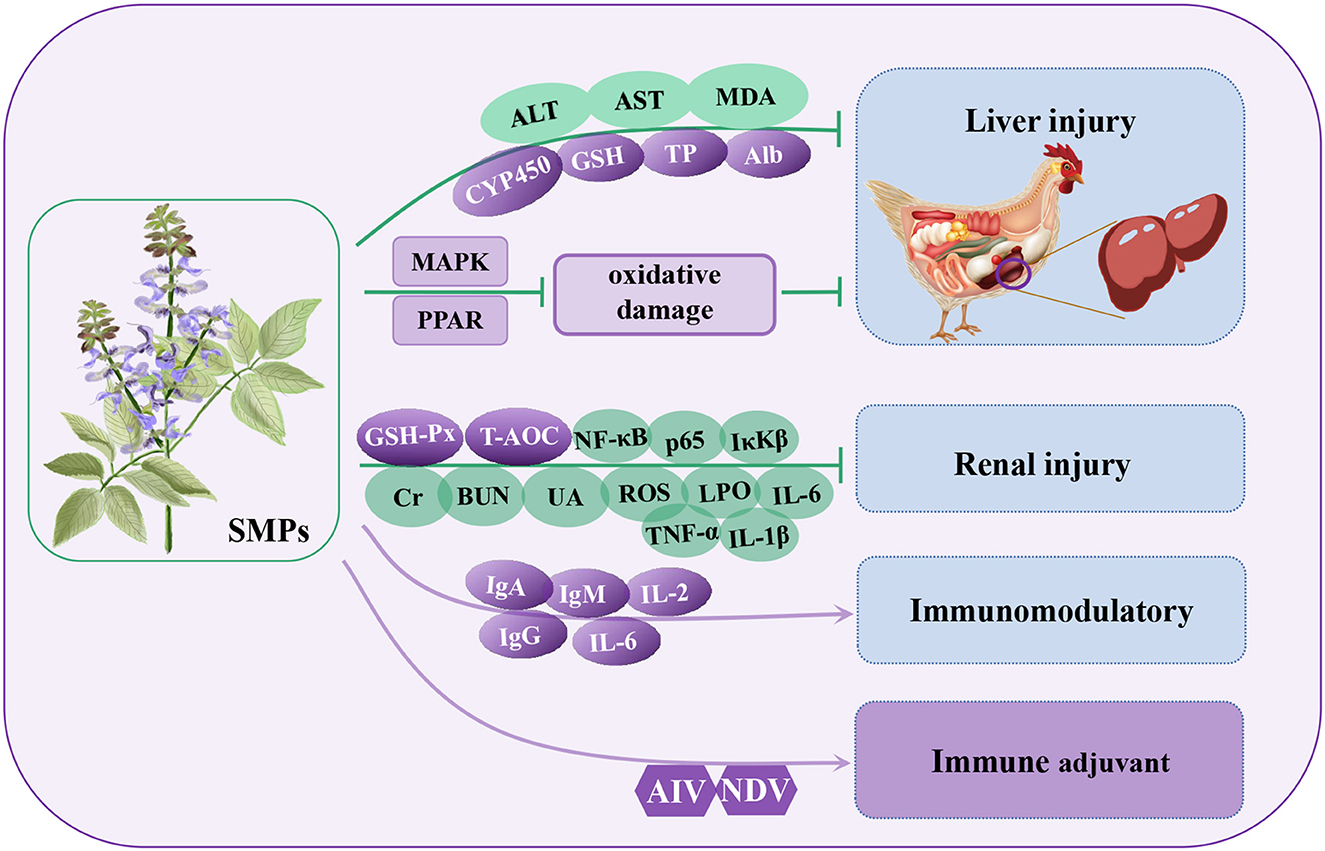
Figure 6. Regulatory mechanisms of Salvia miltiorrhiza polysaccharides (SMPs) in organ protection in poultry. ALT, glutamic pyruvic transaminase; AST, glutamic oxaloacetic transaminase; CYP450, cytochrome P450; TP, total protein; Alb, albumin; MAPK, mitogen activated kinase-like protein; PPAR, peroxisome proliferator activated receptor alpha; BUN, blood urea nitrogen; Cr, creatinine; UA, uric acid; LPO, lipid peroxide; IκKβ, inhibitor kappa B kinaseβ.
In a poultry kidney injury model, SMPs can alleviate inflammation by reducing the phosphorylation levels of NF-κB, p65, and IκKβ proteins and reducing the secretion of inflammatory factors (TNF-α, IL-1β, and IL-6) (112). Similarly, after feeding broilers with SMPs (0.50%), the content of immunoglobulin (IgG, IgM, and IgA) in serum increased, promoted the secretion of cytokines IL-2 and IL-6, and the antibody levels of ND and AI increased by 28.87 and 43.59%, respectively, thus improving the immune function of chicks (113). In a poultry hepatocyte injury model, SMPs can alleviate liver injury and metabolic disorders through drug metabolism induced by cytochrome P450 and the glycine, serine, and threonine metabolism signaling pathways (114). Through transcriptome and proteome studies, Geng et al. (115) found that SMPs alleviate oxidative stress and injury in broiler livers through the PPAR signaling pathway, MAPK signaling pathway, and glutathione metabolism (115). After poultry liver injury, the contents of total protein (TP), albumin (Alb), and GSH increased significantly, while after SMPs treatment, the contents of ALT, AST, and MDA decreased significantly, indicating that SMPs have a good protective effect on poultry liver injury in vivo and in vitro (115, 116). In addition, the strength of immunity is closely related to that of the kidneys. In a renal injury model, SMPs alleviated renal injury by regulating blood urea nitrogen (BUN), creatinine (Cr), uric acid (UA), upregulating antioxidant enzymes GSH-Px and serum T-AOC, rapidly eliminating lipid peroxide (LPO) and ROS, and reducing oxidative stress in the kidney (117). Other studies have shown that SMPs combined with florfenicol (FFC) can improve the growth performance of broilers, increase the number of leukocyte subtypes in blood, increase the number of immunoglobulins (IgG, IgM, and IgA) and cytokines (IFN-γ and IL-2) in serum, and stimulate T cell proliferation and differentiation to activate cellular immunity. It can also increase antibody titers against ND and AI (118). These findings suggest that SMPs have a significant ability to protect the liver and can be used as potential therapeutic drugs for the treatment of liver disease in poultry.
2.7. Agaricus blazei Murill polysaccharides
Agaricus blazei Murill polysaccharides (ABPs) are among the main bioactive components of Agaricus blazei Murrill, and their functional properties have been recognized worldwide. These polysaccharides can be extracted by alcohol precipitation, microwave extraction, and hot water extraction (119–121). The types of polysaccharides produced by different extraction methods vary and mainly include glucose, mannose, galactose, rhamnose, arabinose (122, 123). Several experiments have shown that ABPs has many biological functions, such as anti-tumor (124), anti-inflammation (125), anti-aging (123), anti-oxidation (126), hypolipidemia (122), and anti-parasite (127) functions (Table 7). ABPs can significantly enhance immunity by activating and strengthening all types of immune cells, repairing abnormal cells, increasing the activity of various biological enzymes in cells, and improving the detoxification ability of the liver (128). Recent studies have shown that ABPs can play a positive role in the immune regulation of poultry via many pathways (Figure 7).
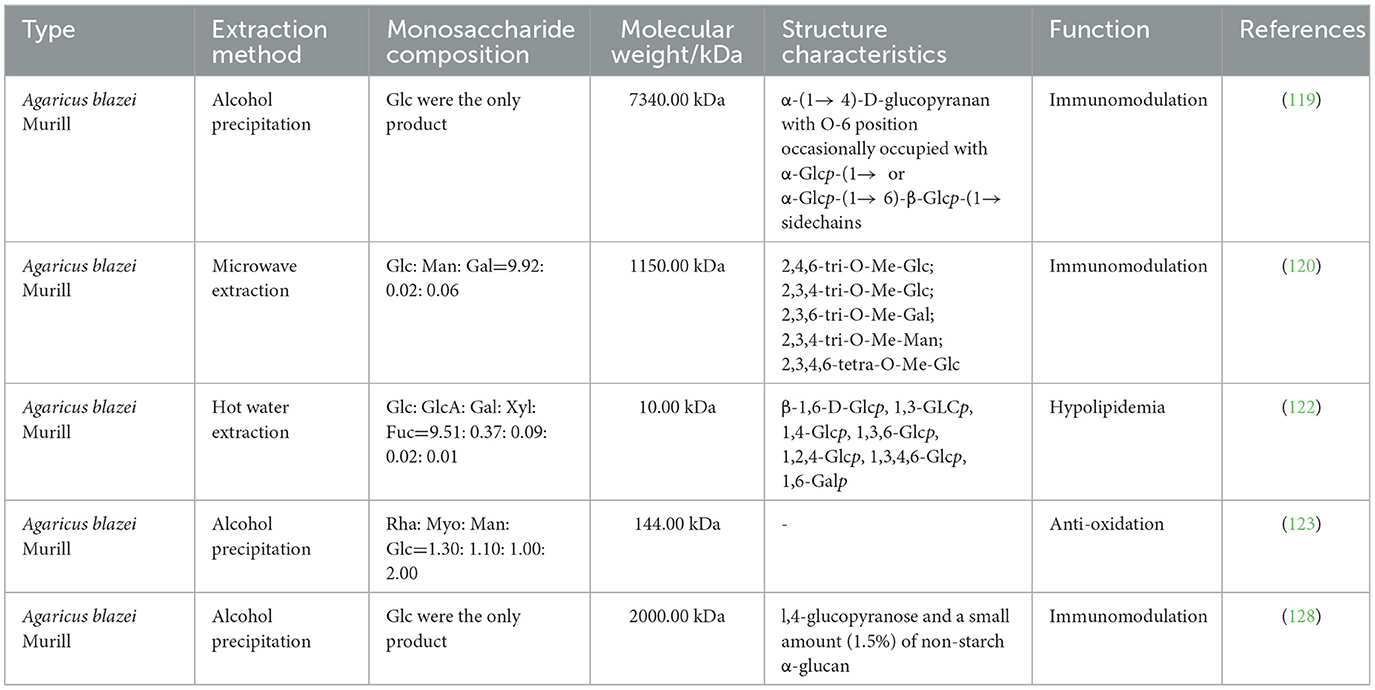
Table 7. Structure and structure-activity-relationship of polysaccharide from Agaricus blazei Murill.
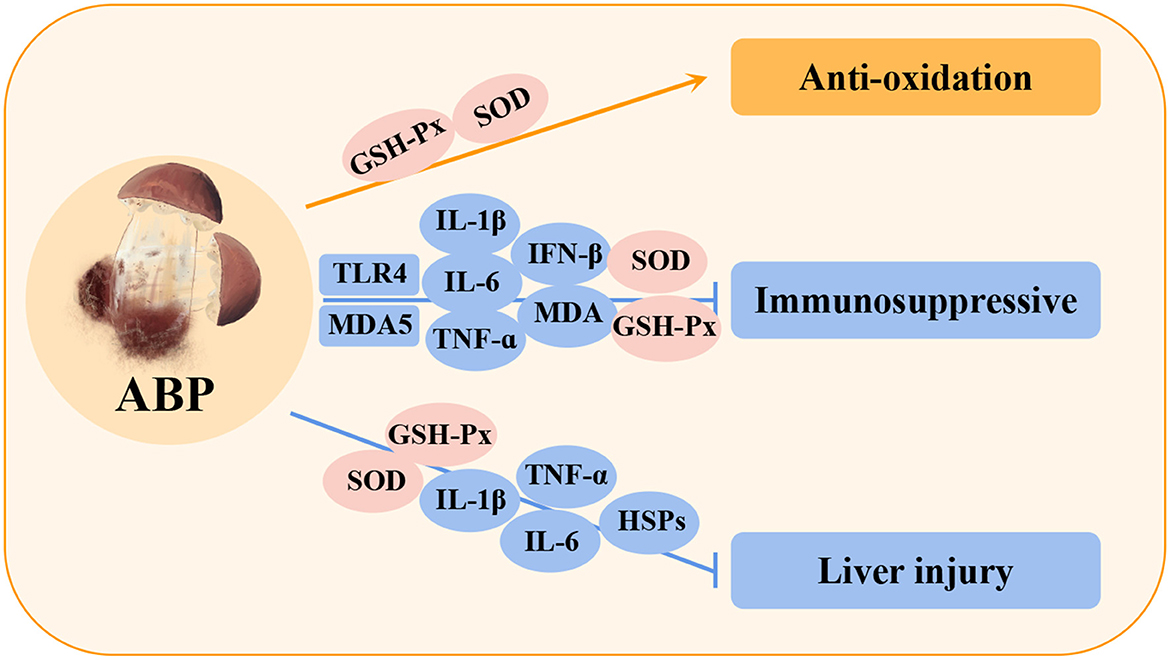
Figure 7. Immunomodulatory and hepatoprotective effects of Agaricus blazei Murill polysaccharides (ABPs) in poultry. C4, complement component 4; IFN-β, interferon-beta; HSPs, heat shock proteins.
Studies have shown that ABPs can significantly increase the contents of GSH-Px and SOD in poultry lymphocytes, indicating that ABPs helps to improve the antioxidant capacity and resistance of the body (129). In addition, in the immunosuppressive model of Cd, ABPs were shown to reduce the accumulation of Cd in chicken serum and immune organs and reduce Cd-induced damage by inhibiting MDA5 and TLR4 signaling pathways (130), which reduces the expression of downstream inflammatory factors (IL-1β, IL-6, TNF-α, and IFN-β), and ABP can promote the expression of anti-apoptosis protein (Bcl-2) and reduce the expression of caspase-3 and pro-apoptosis protein (Bax). It also decreased the levels of heat shock proteins (HSP60, HSP70, and HSP90) in spleen and increased the activity of antioxidant enzymes (SOD, and GSH-Px), indicating that ABP could improve the immunotoxicity induced by Cd (131, 132). In a model of liver injury, ABPs significantly reduced inflammatory cytokines (TNF-α, IL-1β, and IL-6) and HSPs (HSP27, HSP40, HSP60, HSP70, and HSP90) by increasing the activities of SOD and GSH-PX antioxidant enzymes, indicating that ABPs can effectively reduce oxidative stress and inflammation in liver injury by improving the antioxidant capacity (133). In summary, these studies demonstrated that ABPs exert their biological activity by regulating the immune system in poultry and mediating the protection of various organs (134).
2.8. Enteromorpha polysaccharide
Enteromorpha polysaccharide (EP) is one of the main active components of Enteromorpha, which is a natural wild green algae that is widely distributed in the East China Sea and South China Sea and represents a problematic genus along the coastal areas of China (135). In recent years, studies have found that Enteromorpha species have a variety of uses showed that EP has a variety of biological functions, including antiviral (136), anti-tumor (137), immune regulation (138), anti-oxidation (139), anti-diabetes (140), and blood lipid reduction (141). EP is mainly extracted by acid extraction (142), hot water extraction (143) and microwave-assisted extraction (139), and it is mainly composed of rhamnose, glucuronic acid, glucose, galactose, xylose, and mannose. EP structure is rich in sulfate (Table 8). In recent years, researchers have found that EP enhances immune regulation and disease resistance in poultry (Figure 8).
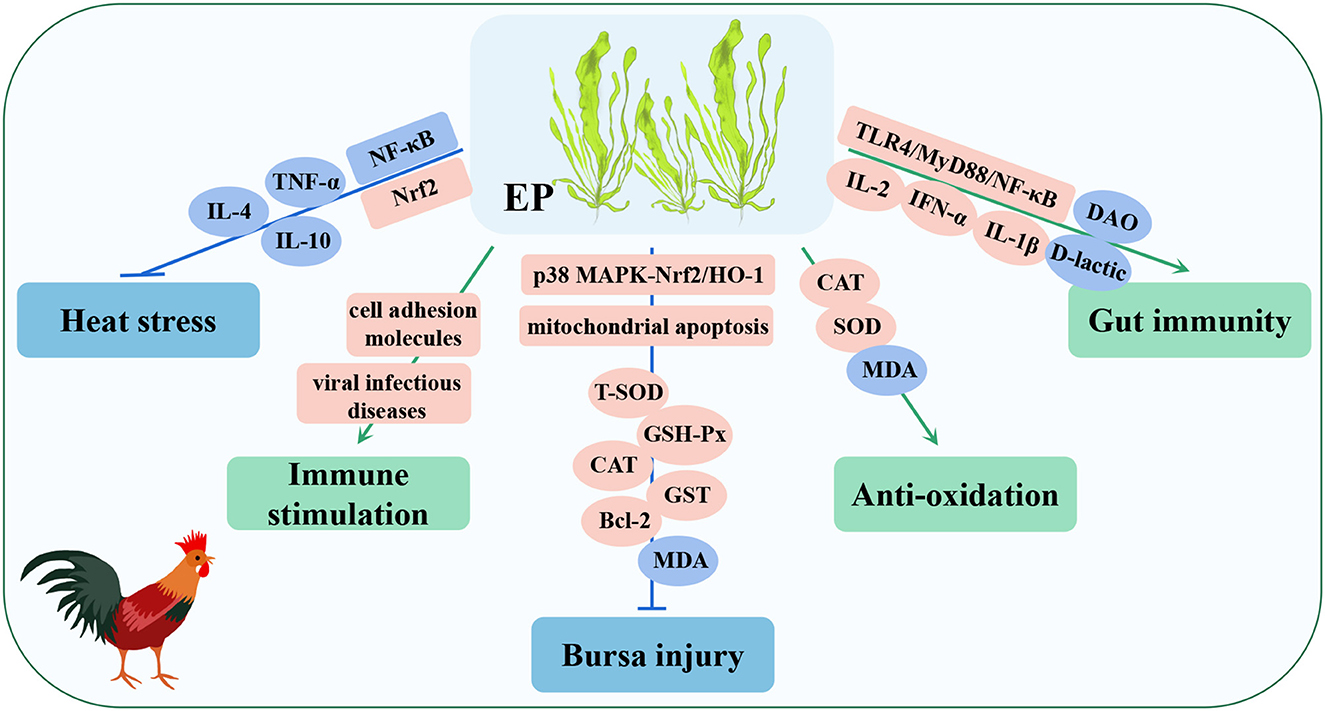
Figure 8. Immunomodulatory and disease-resistant effects of Enteromorpha polysaccharide (EP) in poultry. Nrf2, nuclea factor erythroid-2-related factor 2; Bcl-2, B-cell lymphoma-2; GST, glutathione-S-transferase; IFN-α, interferon-α.
The results showed that the addition of EP to the diet of poultry increased the activity of SOD in the liver and CAT in the serum, decreased the level of MDA in the serum and liver, and enhanced the ability of antioxidation (145, 146). Similarly, after including EP in the diet of poultry, the pectoral muscles and bursa of Fabricius were sampled for transcriptome sequencing (RNA-seq), and a GO analysis showed that the differentially expressed genes (DEGs) were mainly enriched in immune-related signaling pathways, while a KEGG analysis indicated that they were enriched in viral infectious disease, cell adhesion molecule, and other immune signaling pathways, and these findings indicated that EP mainly plays an immunostimulatory role in poultry (147, 148). Moreover, EP can increase the mRNA expression of IL-2, IFN-α, and IL-1β in intestinal tissue by activating the TLR4/MyD88/NF-κB signaling pathway, and significantly decrease the level of D-lactic acid and the activity of DAO in serum, both of which reduce intestinal barrier functions and permeability. Simultaneously, EP also increased the abundance of Bacteroides and Lactobacillus. The short-chain volatile fatty acids (SCFAs) produced by these bacteria are involved in the immune response and intestinal barrier function of the body, indicating that EP can improve the intestinal immune response and integrity of poultry (149, 150). In a heat stress model, EP alleviated the imbalance of Th1/Th2 cells by activating the Nrf2 signaling pathway, inhibiting the NF-κB signaling pathway, and reducing the expression of proinflammatory cytokines (TNF-α, IL-4, and IL-10), thus improving the inflammatory response and injury of immune organs induced by heat stress (144, 151). In addition, in a poultry immune organ bursa oxidative injury model, EP reduced bursa cell necrosis by activating the p38 MAPK-Nrf2/HO-1 and mitochondrial apoptosis signaling pathways, increased the activities of antioxidant enzymes (T-SOD, GSH-Px, CAT, and GST), upregulated the expression of antioxidant and apoptosis-related proteins (Nrf2, HO-1, p38 MAPK, Bcl-2), and decreased the content of MDA. As a result, the antioxidant capacity of the bursa of Fabricius was enhanced and injury was reduced (152). These studies showed that EP has strong immunomodulatory and antioxidant capacities and can be used as a nutritional supplement as an antioxidant and immune enhancer, thereby providing theoretical support for the development and utilization of Enteromorpha resources.
2.9. Glycyrrhiza polysaccharides
Glycyrrhiza polysaccharides (GPs) are among the main bioactive components of licorice, and they are mainly composed of arabinose, glucose, galactose, rhamnose, mannose, xylose, and galacturonic acid in different proportions and with different types of glycosidic bonds (153–155). GPs is considered a potential immune enhancer for poultry because of its biological activities, such as antioxidation (156, 157), immune regulation (158, 159), anti-tumor (160), and intestinal flora regulation (161) (Table 9; Figure 9).
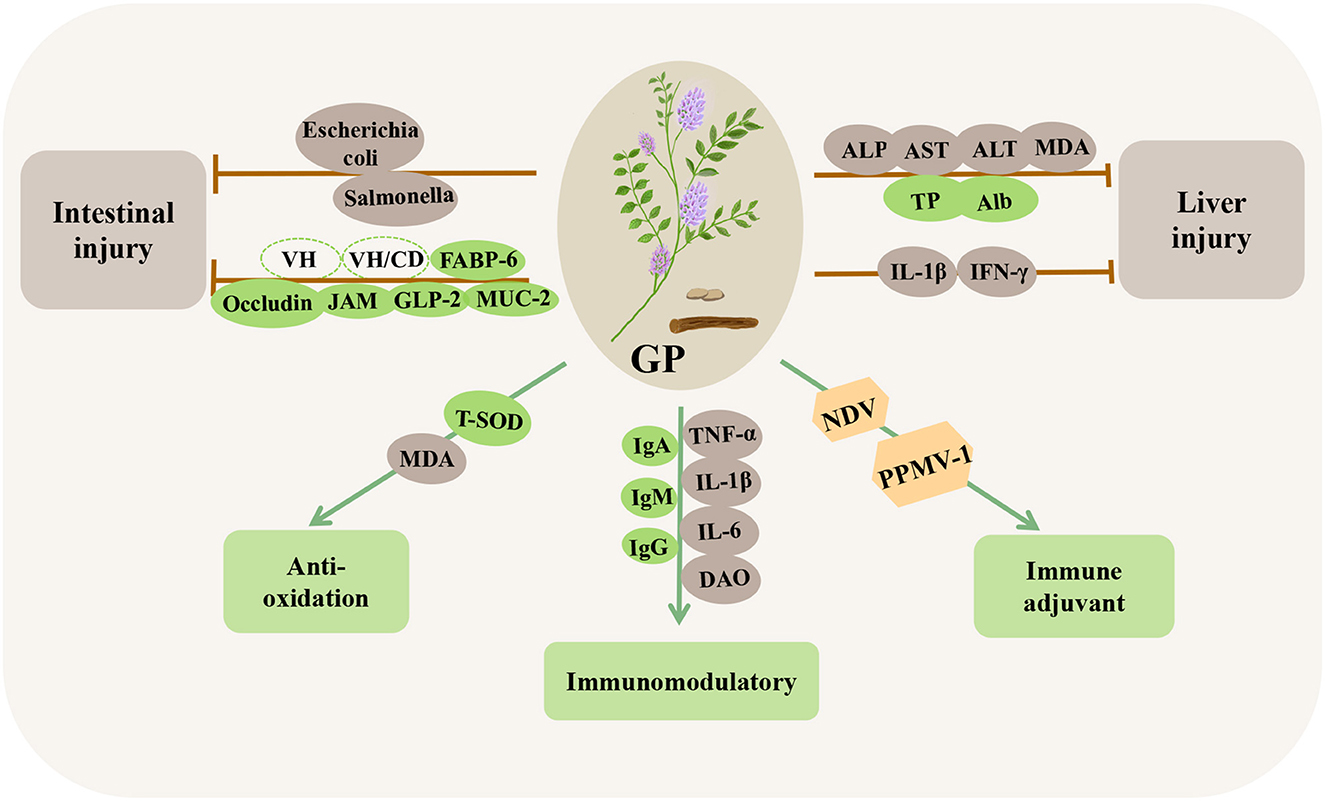
Figure 9. Immunomodulatory and disease-resistant effects of Glycyrrhiza polysaccharides (GPs) in poultry. VH, villus height; VH/CD, villus height/crypt depth; FABP-6, fatty acid binding protein 6; Occludin, tight junction proteins; JAM, junction adhesion molecules; GLP-2, glucagon-like peptide-2; MUC-2, mucin-2; PPMV-1, paramyxovirus type 1; ALP, alkaline phosphatase.
Studies have shown that GPs can promote the development of immune organs and stimulate the continuous proliferation of spleen macrophages, significantly increase the levels of IgA, IgM, and IgG in the serum, and significantly reduce the levels of TNF-α, IL-1β, IL-6, and DAO to induce an immune response, thereby improving the immune function of the body (162–164). In a study on the antioxidation capacity of GPs, the direct addition of 1000 or 1500 mg/kg GPs to chicken diets significantly increased the T-SOD activity and decreased the serum MDA content (165). Similarly, the antioxidant effect of GPs plays a significant role in reversing oxidative stress induced by LPS and can reduce the increase in IL-1β and IFN-γ in the liver induced by LPS (166). In addition, GPs significantly alleviated oxidative stress in broilers induced by mycotoxins, significantly decreased the activities of ALP, AST, and ALT and the concentration of MDA, and increased the concentration of serum total protein and albumin, thus alleviating liver injury (167). In intestinal immunity, dietary GPs can significantly increase the villus height and the ratio of villus height to crypt depth (163, 165) and significantly upregulate the mRNA expression of tight junction proteins (occludin), junction adhesion molecules (JAM), glucagon-like peptides-2 (GLP-2), fatty acid binding protein 6 (FABP-6), and mucin-2 (MUC-2) in the intestine (142, 164). Similarly, GPs can significantly reduce the number of Escherichia coli and Salmonella in poultry intestines, thereby enhancing intestinal immunity and maintaining healthy intestinal microorganisms (161). In addition, GPs can be used as a vaccine adjuvant, which can significantly increase chicken lymphocytes and reduce the copy number of viral RNA, thus enhancing the titer of NDV and paramyxovirus type 1 (PPMV-1) vaccines (168–170). These results suggested that GPs have immunomodulatory activity and can be used as potential innate immunomodulators.
2.10. Others
In addition to the above plant polysaccharides, other polysaccharides also have important immune regulatory activities in poultry (Table 10). Recent studies have shown that the addition of Lycium barbarum polysaccharide (LBP) to poultry diets can increase the enzyme activity of SOD and GSH-Px, reduce the content of MDA, increase the content of IgG and IgA in serum, and increase the cytokine levels of TNF-α, IL-4, IL-6, and IFN-γ to improve the antioxidation ability and enhance immune function (171). Similarly, Acanthopanax senticosus polysaccharide (ASPS) can improve the activities of SOD and GSH-Px in serum, decrease the content of MDA, and significantly increase the content of IgA and IgM in serum, thereby improving the antioxidant capacity and immune status (172). In addition, ASPS can promote lymphocyte proliferation, increase the CD4+ and CD8+T lymphocyte proportion, and promote IFN-γ and IL-2 secretion, indicating that ASPS can enhance immune activity in poultry (173). In addition, Yingshan yunwu tea polysaccharide (YYTP) could increase the immune organ index and the secretion of immunoglobulin (IgA and IgG) in poultry. YYTP has strong DPPH radical, superoxide anion, and hydroxyl scavenging activities and enhances the T-AOC, SOD, and GSH-Px activity. These results indicate that YYTP has strong antioxidant capacity, can be used as a natural antioxidant, and has a positive role in promoting immune response (174, 175). A previous study reported that ginseng polysaccharide (GPS) stimulates immune cells to secrete cytokines (IL-2, IL-10, IFN-β, and TNF-α) and increases antibody titers of H5N1 avian influenza virus vaccine, thus demonstrating its potential in improving the immune response (176). Similarly, Mulberry leaf polysaccharide (MLP) can significantly stimulate lymphocyte proliferation and promote the secretion of IL-2, IFN-γ, sIgA, IgG, and IgA, thereby improving the antibody titer of ND in poultry serum. Moreover, it can be used as a potential immune adjuvant for ND (177, 178).
In addition, Artemisia ordosica polysaccharide (AOP) can alleviate LPS-induced oxidative stress injury in poultry by activating the Nrf2/Keap1 signaling pathway, inhibiting the TLR4/NF-κB pathway, increasing the activity of antioxidant enzymes (T-AOC, SOD, CAT, and GPX), and reducing the expression of proinflammatory cytokines (IL-1β, IL-6, and TNF-α), thus indicating that AOP has antioxidant and anti-inflammatory effects (179). Shu et al. found that Polygonatum sibiricum polysaccharide (PP) can effectively alleviate immunosuppression injury by increasing the antibody content of IgG and IgM, stimulating the proliferation of T lymphocytes in peripheral blood, and regulating the expression levels of IL-2, IL-6, and IFN-γ (180). In addition, Gan et al. found that bush sophora root polysaccharide (BSRPS) could alleviate hepatocyte injury by improving the expression of SOD2 and downregulating CYP450 1A5 (181). In immune cells, Echinacea purpurea polysaccharide (EPP) can increase the expression of CD80 surface markers in poultry dendritic cells, increase the levels of IL-2 and IFN-γ, and stimulate immune cell proliferation, indicating that EPP can drive dendritic cells to mature and play an immunomodulatory role (182). Similarly, Platycodon grandiflorum polysaccharide (PGPS) can increase the proliferation of T lymphocytes and macrophages, increase the phagocytosis rate of macrophages and the expression of maturation markers (CD80 and CD86), and stimulate macrophages to produce the cytokines TNF-α, IL-1β, and IL-6, indicating that PGPS has significant immune activity (183, 184). In addition, Paulownia fortunei flower polysaccharide (PFFPS) can significantly increase leukocytes and lymphocytes, increase the contents of IL-2, IFN, and SIgA in the duodenum, and increase the antibody titer of ND (185). Taken together, plant polysaccharides have many beneficial effects in poultry immune regulation, such as enhancing immunity, anti-oxidation, anti-inflammation, resisting immunosuppression, and improving vaccine titer, but their potential regulatory pathways are still not completely clear.
3. Conclusions
The above data indicate that the molecular weight of PAMK is smaller than other polysaccharides, but similar to APS, it has more bioactive functions. TPPPS is widely studied in poultry antivirus and is a potential vaccine adjuvant. AP and SMPs have good protective effects on liver injury in poultry. Glucose plays an important role in immune function of ABPs. EP are different from the polysaccharides of terrestrial plants, their structure is rich in sulfate, and their biological functions are mainly sulfated polysaccharides. In general, plant polysaccharides play many important roles in poultry immune regulation, such as regulating the body's immune function, improving the antioxidant capacity, relieving immunosuppression, promoting the recovery and regeneration of immune organs, improving the regulation of cytokines, improving intestinal flora, and acting as potential vaccine adjuvants. Hence, plant polysaccharides represent a good and safe method of improving the immune regulatory function of poultry; moreover, plant polysaccharides as green additives may be used as potential therapeutic candidates to treat poultry-related diseases. However, certain concerns remain to be addressed:(1) Different plant polysaccharides have different origins, and the efficiency of immune regulation by polysaccharides from different origins must be compared and investigated. (2) The biological activity mechanism, dosage, effect, and higher-order structure of plant polysaccharides are unknown, which limits their development and utilization. (3) A single polysaccharide should be strengthened and improved for different purposes and combined in different proportions into a complex polysaccharide with multiple functions to improve the utilization rate and functional development of plant polysaccharides. (4) The immune regulatory mechanism of plant polysaccharides involves different receptors on various immune cells and has a regulatory effect on intercellular messenger molecules. Therefore, its mechanism of action must be explored at multiple levels. (5) The bioactive function and precise targeting of different modified plant polysaccharides and drug carrier systems (such as liposomes and nanoparticle carriers) should be improved to improve the therapeutic effect and reduce the dosage and side effects. Such improvements represent an important direction for future research on plant polysaccharides. (6) The potential application of plant polysaccharides in treating poultry diseases needs to be further explored. The current findings and future studies could provide more information on the regulatory and molecular mechanisms of plant polysaccharides in the development, regulation, and function of immune-related diseases of the poultry immune system.
Author contributions
R-HZ: conceptualization, project administration, visualization, and writing—original draft. F-XY: project administration, visualization, and writing—original draft. Y-CB: project administration and visualization. J-YZ, MH, and X-YZ: project administration. T-FD: project administration and supervision. J-JJ: conceptualization, funding acquisition, supervision, and writing—review and editing. All authors contributed to the article and approved the submitted version.
Funding
This work was jointly supported by the Yunnan Province Qiu Shengxiang Expert Workstation (202005AF150039) and the Yunnan Fundamental Research Projects (202201AT070260).
Acknowledgments
We would like to thank Editage (www.editage.cn) for English language editing.
Conflict of interest
Y-CB is employed by the Kunming CHIA TAI Co., Ltd.
The remaining authors declare that the research was conducted in the absence of any commercial or financial relationships that could be construed as a potential conflict of interest.
Publisher's note
All claims expressed in this article are solely those of the authors and do not necessarily represent those of their affiliated organizations, or those of the publisher, the editors and the reviewers. Any product that may be evaluated in this article, or claim that may be made by its manufacturer, is not guaranteed or endorsed by the publisher.
References
1. Alkie TN, Rautenschlein S. Infectious bursal disease virus in poultry: current status and future prospects. Vet Med. (2016) 7:9–18. doi: 10.2147/VMRR.S68905
2. Kumar Y, Singh V, Kumar G, Gupta NK, Tahlan AK. Serovar diversity of Salmonella among poultry. Indian J Med Res. (2019) 150:92–5. doi: 10.4103/ijmr.IJMR_1798_17
3. Mayers J, Mansfield KL, Brown IH. The role of vaccination in risk mitigation and control of Newcastle disease in poultry. Vaccine. (2017) 35:5974–80. doi: 10.1016/j.vaccine.2017.09.008
4. Jiang W, Hou G, Li J, Peng C, Wang S, Liu S. Q, Prevalence of H7N9 subtype avian influenza viruses in poultry in China, 2013-2018. Transbound Emerg Dis. (2019) 66:1758–61. doi: 10.1111/tbed.13183
5. Abdel-Moneim AE, Shehata AM, Khidr RE, Paswan VK, Ibrahim NS, El-Ghoul AA. Nutritional manipulation to combat heat stress in poultry - A comprehensive review. J Therm Biol. (2021) 98:102915. doi: 10.1016/j.jtherbio.2021.102915
6. Naveed M, Haleem KS, Ghazanfar S, Tauseef I, Bano N, Adetunji CO, et al. Quantitative estimation of aflatoxin level in poultry feed in selected poultry farms. Biomed Res Int. (2022) 2022:5397561. doi: 10.1155/2022/5397561
7. Zhai S, Zhu Y, Feng P, Li M, Wang W, Yang L, et al. Ochratoxin A: its impact on poultry gut health and microbiota, an overview. Poult Sci. (2021) 100:101037. doi: 10.1016/j.psj.2021.101037
8. Dhama K, Chakraborty S, Verma AK, Tiwari R, Barathidasan R, Kumar A, et al. Fungal/mycotic diseases of poultry-diagnosis, treatment and control: a review. Pak J Biol Sci. (2013) 16:1626–40. doi: 10.3923/pjbs.2013.1626.1640
9. Gimeno IM, Schat KA. Virus-Induced Immunosuppression in Chickens. Avian Dis. (2018) 62:272–85. doi: 10.1637/11841-041318-Review.1
10. P Wigley. Immunity to bacterial infection in the chicken. Dev Comp Immunol. (2013) 41:413–7. doi: 10.1016/j.dci.2013.04.008
11. Bilal RM, Hassan FU, Farag MR, Nasir TA, Ragni M, Mahgoub HAM, et al. Thermal stress and high stocking densities in poultry farms: potential effects and mitigation strategies. J Therm Biol. (2021) 99:102944. doi: 10.1016/j.jtherbio.2021.102944
12. Hofmann T, Schmucker S, Grashorn M, Stefanski V. Short- and long-term consequences of stocking density during rearing on the immune system and welfare of laying hens. Poult Sci. (2021) 100:101243. doi: 10.1016/j.psj.2021.101243
13. Yang Y, Qiu W, Li Y, Liu L. Antibiotic residues in poultry food in Fujian Province of China. Food Addit Contam Part B Surveill. (2020) 13:177–84. doi: 10.1080/19393210.2020.1751309
14. Wan X, Yin Y, Zhou C, Hou L, Cui Q, Zhang X, et al. Polysaccharides derived from Chinese medicinal herbs: a promising choice of vaccine adjuvants. Carbohydr Polym. (2022) 276:118739. doi: 10.1016/j.carbpol.2021.118739
15. Li CX, Liu Y, Zhang YZ, Li JC, Lai J. Astragalus polysaccharide: a review of its immunomodulatory effect. Arch Pharm Res. (2022) 45:367–89. doi: 10.1007/s12272-022-01393-3
16. Gong H, Li W, Sun J, Jia L, Guan Q, Guo Y, et al. A review on plant polysaccharide based on drug delivery system for construction and application, with emphasis on traditional Chinese medicine polysaccharide. Int J Biol Macromol. (2022) 211:711–28. doi: 10.1016/j.ijbiomac.2022.05.087
17. Stewart CR, Keyburn AL, Deffrasnes C, Tompkins SM. Potential directions for chicken immunology research. Dev Comp Immunol. (2013) 41:463–8. doi: 10.1016/j.dci.2013.05.011
18. Hofmann T, Schmucker SS, Bessei W, Grashorn M, Stefanski V. Impact of housing environment on the immune system in chickens: a review. Animals. (2020) 10:123. doi: 10.3390/ani10071138
19. Nawab A, An L, Wu J, Li G, Liu W, Zhao YQ, et al. Chicken toll-like receptors and their significance in immune response and disease resistance. Int Rev Immunol. (2019) 38:284–306. doi: 10.1080/08830185.2019.1659258
20. Klasing KC. Avian macrophages: regulators of local and systemic immune responses. Poult Sci. (1998) 77:983–9. doi: 10.1093/ps/77.7.983
21. Erf GF. Cell-mediated immunity in poultry. Poult Sci. (2004) 83:580–90. doi: 10.1093/ps/83.4.580
22. Yin M, Zhang Y, Li H. Advances in research on immunoregulation of macrophages by plant polysaccharides. Front Immunol. (2019) 10:145. doi: 10.3389/fimmu.2019.00145
23. Pu JB, Xia BH, Hu YJ, Zhang HJ, Chen J, Zhou J, et al. Multi-optimization of ultrasonic-assisted enzymatic extraction of atratylodes macrocephala polysaccharides and antioxidants using response surface methodology and desirability function approach. Molecules. (2015) 20:22220–35. doi: 10.3390/molecules201219837
24. Li X, Rao Z, Xie Z, Qi H, Zeng N. Isolation, structure and bioactivity of polysaccharides from atractylodes macrocephala: a review. J Ethnopharmacol. (2022) 296:115506. doi: 10.1016/j.jep.2022.115506
25. Feng YY, Ji HY, Dong XD, Yu J, Liu AJ. Polysaccharide extracted from Atractylodes macrocephala Koidz (PAMK) induce apoptosis in transplanted H22 cells in mice. Int J Biol Macromol. (2019) 137:604–11. doi: 10.1016/j.ijbiomac.2019.06.059
26. Xue W, Gao Y, Li Q, Lu Q, Bian Z, Tang LY et al. Immunomodulatory activity-guided isolation and characterization of a novel polysaccharide from Atractylodis macrocephalae Koidz. Int J Biol Macromol. (2020) 161:514–24. doi: 10.1016/j.ijbiomac.2020.06.003
27. Cui YS, Li YX, Jiang SL, Song AN, Fu Z, Dong X, et al. Isolation, purification, and structural characterization of polysaccharides from Atractylodis Macrocephalae Rhizoma and their immunostimulatory activity in RAW264.7 cells. Int J Biol Macromol. (2020) 163:270–8. doi: 10.1016/j.ijbiomac.2020.06.269
28. Feng YY, Ji HY, Dong XD, Liu AJ. An alcohol-soluble polysaccharide from Atractylodes macrocephala Koidz induces apoptosis of Eca-109 cells. Carbohydr Polym. (2019) 226:115136. doi: 10.1016/j.carbpol.2019.115136
29. Guo S, Li W, Chen F, Yang S, Huang Y, Tian YD, et al., Polysaccharide of Atractylodes macrocephala Koidz regulates LPS-mediated mouse hepatitis through the TLR4-MyD88-NFkappaB signaling pathway. Int Immunopharmacol. (2021) 98:107692. doi: 10.1016/j.intimp.2021.107692
30. Shan JJ, Tian GY. Studies on physico-chemical properties and hypoglycemic activity of complex polysaccharide AMP-B from Atractylodes macrocephala Koidz. Yao Xue Xue Bao. (2003) 38:438–41.
31. Li W, Zhou X, Xu S, Cao N, Li B. Lipopolysaccharide-induced splenic ferroptosis in goslings was alleviated by polysaccharide of atractylodes macrocephala koidz associated with proinflammatory factors. Poult Sci. (2022) 101:101725. doi: 10.1016/j.psj.2022.101725
32. Li W, Xu D, Li B, Cao N, Guo S, Jiang Q, et al. The polysaccharide of Atractylodes macrocephala koidz (PAMK) alleviates cyclophosphamide-mediated immunosuppression in geese, possibly through novel_mir2 targeting of CTLA4 to upregulate the TCR-NFAT pathway. RSC Adv. (2018) 8:26837–48. doi: 10.1039/C8RA00368H
33. Li W, Xiang X, Cao N, Chen W, Tian Y, Zhang X, et al. Polysaccharide of atractylodes macrocephala koidz activated T lymphocytes to alleviate cyclophosphamide-induced immunosuppression of geese through novel_mir2/CD28/AP-1 signal pathway. Poult Sci. (2021) 100:101129. doi: 10.1016/j.psj.2021.101129
34. Li W, Guo S, Xu D, Li B, Cao N, Tian Y, et al. Polysaccharide of Atractylodes macrocephala Koidz (PAMK) relieves immunosuppression in cyclophosphamide-treated geese by maintaining a humoral and cellular immune balance. Molecules. (2018) 23:932. doi: 10.3390/molecules23040932
35. Xu D, Li B, Cao N, Li W, Tian Y, Huang Y. The protective effects of polysaccharide of Atractylodes macrocephala Koidz (PAMK) on the chicken spleen under heat stress via antagonizing apoptosis and restoring the immune function. Oncotarget. (2017) 8:70394–405. doi: 10.18632/oncotarget.19709
36. Xu D, Li W, Huang Y, He J, Tian Y. The effect of selenium and polysaccharide of Atractylodes macrocephala Koidz. (PAMK) on immune response in chicken spleen under heat stress. Biol Trace Elem Res. (2014) 160:232–7. doi: 10.1007/s12011-014-0056-y
37. Xu D, Tian Y. Selenium and polysaccharides of atractylodes macrocephala koidz play different roles in improving the immune response induced by heat stress in chickens. Biol Trace Elem Res. (2015) 168:235–41. doi: 10.1007/s12011-015-0351-2
38. Li W, Xiang X, Li B, Wang Y, Qian L, Tian Y, et al. PAMK relieves LPS-induced enteritis and improves intestinal flora disorder in goslings. Evid Based Complement Alternat Med. (2021) 2021:9721353. doi: 10.1155/2021/9721353
39. Miao YF, Gao XN, Xu DN, Li MC, Gao ZS, Tang ZH, et al. Protective effect of the new prepared Atractylodes macrocephala Koidz polysaccharide on fatty liver hemorrhagic syndrome in laying hens. Poult Sci. (2021) 100:938–48. doi: 10.1016/j.psj.2020.11.036
40. Liu J, Chen X, Yue C, Hou R, Chen J, Lu Y, et al. Effect of selenylation modification on immune-enhancing activity of Atractylodes macrocephala polysaccharide. Int J Biol Macromol. (2015) 72:1435–40. doi: 10.1016/j.ijbiomac.2014.10.022
41. Zhao X, Sun W, Zhang S, Meng G, Qi CW, Fan Y, et al. The immune adjuvant response of polysaccharides from Atractylodis macrocephalae Koidz in chickens vaccinated against Newcastle disease (ND). Carbohydr Polym. (2016) 141:190–6. doi: 10.1016/j.carbpol.2016.01.013
42. Jiang Y, Qi X, Gao K, Liu W, Li NN, Cheng G, et al. Relationship between molecular weight, monosaccharide composition and immunobiologic activity of Astragalus polysaccharides. Glycoconj J. (2016) 33:755–61. doi: 10.1007/s10719-016-9669-z
43. Chen H, Zhou X, Zhang J. Optimization of enzyme assisted extraction of polysaccharides from Astragalus membranaceus. Carbohydr Polym. (2014) 111:567–75. doi: 10.1016/j.carbpol.2014.05.033
44. Liu AJ, Yu J, Ji HY, Zhang HC, Zhang Y, Liu HP. Extraction of a novel cold-water-soluble polysaccharide from Astragalus membranaceus and its antitumor and immunological activities. Molecules. (2017) 23:62. doi: 10.3390/molecules23010062
45. Wang JM, Sun XY, Ouyang JM. Structural characterization, antioxidant activity, and biomedical application of astragalus polysaccharide degradation products. Int J Polym Sci. (2018) 2018:1–13. doi: 10.1155/2018/5136185
46. Liao J, Li C, Huang J, Liu W, Chen H, Liao S, et al. Structure characterization of honey-processed astragalus polysaccharides and its anti-inflammatory activity in vitro. Molecules. (2018) 23:68. doi: 10.3390/molecules23010168
47. Li K, Cao YX, Jiao SM, Du GH, Du YG, Qin XM. Structural characterization and immune activity screening of polysaccharides with different molecular weights from astragali radix. Front Pharmacol. (2020) 11:582091. doi: 10.3389/fphar.2020.582091
48. Wu J, Li C, Bai L, Wu J, Bo R, Ye M, et al. Structural differences of polysaccharides from Astragalus before and after honey processing and their effects on colitis mice. Int J Biol Macromol. (2021) 182:815–24. doi: 10.1016/j.ijbiomac.2021.04.055
49. Zhang Z, Zhang L, Xu H. Effect of Astragalus polysaccharide in treatment of diabetes mellitus: a narrative review. J Tradit Chin Med. (2019) 39:133–8. doi: 10.19852/j.cnki.jtcm.2019.01.017
50. Fan W, Zhang S, Pan H, Zheng P, Zhao X. Structure characterization of three polysaccharides and a comparative study of their immunomodulatory activities on chicken macrophage. Carbohydr Polym. (2016) 153:631–40. doi: 10.1016/j.carbpol.2016.07.116
51. Lin J, Kang H, Liang J, Fu J, Yu Q, Yang Q. CpG oligonucleotides and Astragalus polysaccharides are effective adjuvants in cultures of avian bone-marrow-derived dendritic cells. Br Poult Sci. (2015) 56:30–8. doi: 10.1080/00071668.2014.981146
52. Wu S. Effect of dietary Astragalus membranaceus polysaccharide on the growth performance and immunity of juvenile broilers. Poult Sci. (2018) 97:3489–93. doi: 10.3382/ps/pey220
53. Zhang R, Yu Q, Shi G, Liu R, Zhang W, Zhao X, et al. chTLR4 pathway activation by Astragalus polysaccharide in bursa of Fabricius. BMC Vet Res. (2017) 13:119. doi: 10.1186/s12917-017-1039-y
54. Li Y, Lei X, Yin Z, Guo W, Wu S, Yang X. Transgenerational effects of paternal dietary Astragalus polysaccharides on spleen immunity of broilers. Int J Biol Macromol. (2018) 115:90–7. doi: 10.1016/j.ijbiomac.2018.04.009
55. Li Y, Lei X, Guo W, Wu S, Duan Y, Yang X, et al. Transgenerational endotoxin tolerance-like effect caused by paternal dietary Astragalus polysaccharides in broilers' jejunum. Int J Biol Macromol. (2018) 111:769–79. doi: 10.1016/j.ijbiomac.2018.01.095
56. Xie W, Ge M, Li G, Zhang L, Tang Z, Li R, et al. Astragalus polysaccharide protect against cadmium-induced cytotoxicity through the MDA5/NF-κB pathway in chicken peripheral blood lymphocytes. Molecules. (2017) 22:610. doi: 10.3390/molecules22101610
57. Li S, Wang XF, Ren LN, Li JL, Zhu XD, Xing T, et al. Protective effects of gamma-irradiated Astragalus polysaccharides on intestinal development and mucosal immune function of immunosuppressed broilers. Poult Sci. (2019) 98:6400–10. doi: 10.3382/ps/pez478
58. Li S, Ren L, Zhu X, Li J, Zhang L, Wang X, et al. Immunomodulatory effect of gamma-irradiated Astragalus polysaccharides on immunosuppressed broilers. Anim Sci J. (2019) 90:117–27. doi: 10.1111/asj.13133
59. Liu L, Shen J, Zhao C, Wang X, Yao J, Gong Y, et al. Dietary Astragalus polysaccharide alleviated immunological stress in broilers exposed to lipopolysaccharide. Int J Biol Macromol. (2015) 72:624–32. doi: 10.1016/j.ijbiomac.2014.08.057
60. Zhang P, Wang J, Wang W, Liu X, Liu H, Li X, et al. Astragalus polysaccharides enhance the immune response to avian infectious bronchitis virus vaccination in chickens. Microb Pathog. (2017) 111:81–5. doi: 10.1016/j.micpath.2017.08.023
61. Zhang P, Liu X, Liu H, Wang W, Liu X, Li X, et al. Astragalus polysaccharides inhibit avian infectious bronchitis virus infection by regulating viral replication. Microb Pathog. (2018) 114:124–8. doi: 10.1016/j.micpath.2017.11.026
62. Yang S, Wei K, Jia F, Zhao X, Cui G, Guo F, et al. Characterization and biological activity of Taishan Pinus massoniana pollen polysaccharide in vitro. PLoS ONE. (2015) 10:e0115638. doi: 10.1371/journal.pone.0115638
63. Zhou C, Yin S, Yu Z, Feng Y, Wei K, Ma W, et al. Preliminary characterization, antioxidant and hepatoprotective activities of polysaccharides from taishan pinus massoniana pollen. Molecules. (2018) 23:281. doi: 10.3390/molecules23020281
64. Peng J, Yuan Y, Du Y, Wu J, Li B, Li J, et al. Potentiation of Taishan Pinus massoniana pollen polysaccharide on the immune response and protection elicited by a highly pathogenic porcine reproductive and respiratory syndrome virus glycoprotein 5 subunit in pigs. Mol Cell Probes. (2016) 30:83–92. doi: 10.1016/j.mcp.2016.01.008
65. Dong W, Zhang H, Huang H, Zhou J, Hu L, Lian A, et al. Chicken IgY Fc linked to bordetella avium ompa and taishan pinus massoniana pollen polysaccharide adjuvant enhances macrophage function and specific immune responses. Front Microbiol. (2016) 7:1708. doi: 10.3389/fmicb.2016.01708
66. Yang S, Zhao Z, Zhang A, Jia F, Song M, Huang Z, et al. Proteomics analysis of chicken peripheral blood lymphocyte in taishan pinus massoniana pollen polysaccharide regulation. PLoS ONE. (2018) 13:e0208314. doi: 10.1371/journal.pone.0208314
67. Li B, Wei K, Yang S, Yang Y, Zhang Y, Zhu F, et al. Immunomodulatory effects of Taishan Pinus massoniana pollen polysaccharide and propolis on immunosuppressed chickens. Microb Pathog. (2015) 78:7–13. doi: 10.1016/j.micpath.2014.11.010
68. Yang S, Li G, Zhao Z, Huang Z, Fu J, Song M, et al. Taishan pinus massoniana pollen polysaccharides enhance immune responses in chickens infected by avian leukosis virus subgroup B. Immunol Invest. (2018) 47:443–56. doi: 10.1080/08820139.2018.1435689
69. Truong AD, Hong Y, Ly VD, Nguyen HT, Nguyen CT, Vu HT, et al. Interleukin-dependent modulation of the expression of MHC class I and MHC class II genes in chicken HD11 cells. Dev Comp Immunol. (2020) 110:103729. doi: 10.1016/j.dci.2020.103729
70. Wang SJ, Wei K, Huang H, Zhang H, Wang HN, Ma LL, et al. The mechanism of resistance tovery virulent infectious bursal disease virus by Taishan Pinus massoniana pollen polysaccharide in vitro. Chin J Vet Sci. (2018) 38:1670–6. doi: 10.16303/j.cnki.1005-4545.2018.09.06
71. Wang Q, Miao Y, Xu Y, Meng X, Cui W, Wang Y, et al. Taishan Pinus Massoniana pollen polysaccharide inhibits the replication of acute tumorigenic ALV-J and its associated tumor growth. Vet Microbiol. (2019) 236:108376. doi: 10.1016/j.vetmic.2019.07.028
72. Yu C, Wei K, Liu L, Yang S, Hu L, Zhao P, et al. Taishan Pinus massoniana pollen polysaccharide inhibits subgroup J avian leucosis virus infection by directly blocking virus infection and improving immunity. Sci Rep. (2017) 7:44353. doi: 10.1038/srep44353
73. Li Y, Meng F, Cui S, Fu J, Wang Y, Cui Z, et al. Cooperative effects of immune enhancer TPPPS and different adjuvants on antibody responses induced by recombinant ALV-J gp85 subunit vaccines in SPF chickens. Vaccine. (2017) 35:1594–8. doi: 10.1016/j.vaccine.2017.02.022
74. Shang H, Sha Z, Wang H, Miao Y, Zhu R. Taishan Pinus massoniana pollen polysaccharide inhibits H9N2 subtype influenza virus infection both in vitro and in vivo. Vet Microbiol. (2020) 248:108803. doi: 10.1016/j.vetmic.2020.108803
75. Zhang Y, Yang S, Dai X, Liu L, Jiang X, Shao M, et al. protective immunity induced by the vaccination of recombinant proteus mirabilis OmpA expressed in Pichia pastoris. Protein Expr Purif. (2015) 105:33–8. doi: 10.1016/j.pep.2014.10.001
76. Liu L, Yu C, Wang C, Shao M, Yan Z, Jiang X, et al. Immuno-enhancement of taishan pinus massoniana pollen polysaccharides on recombinant bordetella avium ompa expressed in pichia pastoris. Microb Pathog. (2016) 95:54–61. doi: 10.1016/j.micpath.2016.03.002
77. Zhang C, Li Z, Zhang ZY, Li M, Lee Y, Zhang GG. Extract methods, molecular characteristics, and bioactivities of polysaccharide from alfalfa (Medicago sativa L.). Nutrients. (2019) 11:181. doi: 10.3390/nu11051181
78. Wang S, Dong X, Tong J. Optimization of enzyme-assisted extraction of polysaccharides from alfalfa and its antioxidant activity. Int J Biol Macromol. (2013) 62:387–96. doi: 10.1016/j.ijbiomac.2013.09.029
79. Gao P, Bian J, Xu S, Liu C, Sun Y, Zhang G, et al. Structural features, selenization modification, antioxidant and anti-tumor effects of polysaccharides from alfalfa roots. Int J Biol Macromol. (2020) 149:207–14. doi: 10.1016/j.ijbiomac.2020.01.239
80. Zhang CY, Gan LP, Du MY, Shang QH, Xie H, Zhang GG. Effects of dietary supplementation of alfalfa polysaccharides on growth performance, small intestinal enzyme activities, morphology, and large intestinal selected microbiota of piglets. Livestock Sci. (2019) 223: 47–52. doi: 10.1016/j.livsci.2019.01.027
81. Zhang T, Yang Y, Liang Y, Jiao X, Zhao C. Beneficial effect of intestinal fermentation of natural polysaccharides. Nutrients. (2018) 10:55. doi: 10.3390/nu10081055
82. Liu X, Xu S, Ding X, Yue D, Gao P. Structural characteristics of Medicago Sativa L. Polysaccharides and Se-modified polysaccharides as well as their antioxidant and neuroprotective activities. Int J Biol Macromol. (2019) 147:78. doi: 10.1016/j.ijbiomac.2019.10.078
83. Li J, Tang Y, Meng X, Guan N, Yu Y. The proliferative effects of alfalfa polysaccharides on the mouse immune cells. Life Sci J. (2013) 10:868–73.
84. Xie Y, Wang L, Sun H, Wang Y, Yang Y, Zhang G, et al. Polysaccharide from alfalfa activates RAW 264.7 macrophages through MAPK and NF-κB signaling pathways. Int J Biol Macromol. (2019) 126: 960–8. doi: 10.1016/j.ijbiomac.2018.12.227
85. Xie Y, Wang L, Sun H, Shang Q, Wang Y, Zhang G, et al. A polysaccharide extracted from alfalfa activates splenic B cells by TLR4 and acts primarily via the MAPK/p38 pathway. Food Funct. (2020) 11:9035–47. doi: 10.1039/D0FO01711F
86. Zhang SY, Xu CY, Dong XF, Tong JM, Zhang Q. Effects of water soluble alfalfa and astragalus polysaccharide on proliferation of lymphocyte in broilers. Chinese J Anim Nutr. (2010) 22:670–4. doi: 10.3969/j.issn.1006-267x.2010.03.022
87. Antunes P, Mourão J, Campos J, Peixe L. Salmonellosis: the role of poultry meat. Clin Microbiol Infect. (2016) 22:110–21. doi: 10.1016/j.cmi.2015.12.004
88. Li Z, Zhang C, Li B, Zhang S, Haj FG, Zhang Y, et al. The modulatory effects of alfalfa polysaccharide on intestinal microbiota and systemic health of Salmonella serotype (ser) Enteritidis-challenged broilers. Sci Rep. (2021) 11:10910. doi: 10.1038/s41598-021-90060-6
89. Wang S, Dong X, Ma H, Cui Y, Tong Y. Purification, characterisation and protective effects of polysaccharides from alfalfa on hepatocytes Carbohydr Polym. (2014) 112:608–14. doi: 10.1016/j.carbpol.2014.06.047
90. Cheng H, Feng S, Jia X, Li Q, Zhou Y, Ding C. Structural characterization and antioxidant activities of polysaccharides extracted from Epimedium acuminatum Carbohydr Polym. (2013) 92:63–8. doi: 10.1016/j.carbpol.2012.09.051
91. Li B, Zhang N, Wang DX, Jiao L, Tan Y, Wang J, et al. Structural analysis and antioxidant activities of neutral polysaccharide isolated from Epimedium koreanum Nakai. Carbohydr Polym. (2018) 196:246–53. doi: 10.1016/j.carbpol.2018.05.037
92. Xiang Y, Ju Z, Li H, Wang Q, Huang Z. Epimedium polysaccharide alleviates polyglutamine-induced neurotoxicity in caenorhabditis elegans by reducing oxidative stress. Rejuvenation Res. (2017) 20:1830. doi: 10.1089/rej.2016.1830
93. Cheng H, Feng S, Shen S, Zhang L, Yang R, Zhou Y, et al. Extraction, antioxidant and antimicrobial activities of Epimedium acuminatum Franch. Polysaccharide. Carbohydr Polym. (2013) 96:101–8. doi: 10.1016/j.carbpol.2013.03.072
94. Wang C, Feng L, Su J, Cui L, Dan L, Yan J, et al. Polysaccharides from Epimedium koreanum Nakai with immunomodulatory activity and inhibitory effect on tumor growth in LLC-bearing mice. J Ethnopharmacol. (2017) 207:8–18. doi: 10.1016/j.jep.2017.06.014
95. Wu Y, Li Y, Liu C, Li E, Gao ZC, Liu W et al. Structural characterization of an acidic Epimedium polysaccharide and its immune-enhancement activity. Carbohydr Polym. (2016) 138:134–42. doi: 10.1016/j.carbpol.2015.11.014
96. Oh YC, Jeong YH, Cho WK, Ha JH, Lee SJ, Ma JY. Inhibitory effects of epimedium herb on the inflammatory response in vitro and in vivo. Am J Chin Med. (2015) 43:953–68. doi: 10.1142/S0192415X1550055X
97. Qin T, Ren Z, Yi L, Liu X, Luo YY, Long S, et al. Immunological modulation effects of an acid Epimedium polysaccharide on immune response in chickens. Int Immunopharmacol. (2019) 70:56–66. doi: 10.1016/j.intimp.2019.02.009
98. Chen X, Chen X, Qiu S, Hu Y, Jiang C, Wang D, et al. Effects of epimedium polysaccharide-propolis flavone oral liquid on mucosal immunity in chickens. Int J Biol Macromol. (2014) 64:6–10. doi: 10.1016/j.ijbiomac.2013.11.015
99. Fan Y, Lu Y, Wang D, Liu J, Song X, Zhang W, et al. Effect of epimedium polysaccharide-propolis flavone immunopotentiator on immunosuppression induced by cyclophosphamide in chickens. Cell Immunol. (2013) 281:37–43. doi: 10.1016/j.cellimm.2013.01.008
100. Fan Y, Hu Y, Wang D, Guo Z, Zhao X, Guo L, et al. Epimedium polysaccharide and propolis flavone can synergistically stimulate lymphocyte proliferation in vitro and enhance the immune responses to ND vaccine in chickens. Int J Biol Macromol. (2010) 47:87–92. doi: 10.1016/j.ijbiomac.2010.05.017
101. Fan Y, Liu J, Wang D, Hu Y, Yang SJ, Wang L, et al. Epimedium polysaccharide and propolis flavone can synergistically inhibit the cellular infectivity of NDV and improve the curative effect of ND in chicken. Int J Biol Macromol. (2011) 48:439–44. doi: 10.1016/j.ijbiomac.2011.01.005
102. Fan Y, Wang D, Liu J, Hu Y, Zhao X, Han G. Adjuvanticity of epimedium polysaccharide-propolis flavone on inactivated vaccines against AI and ND virus. Int J Biol Macromol. (2012) 51: 1028–32. doi: 10.1016/j.ijbiomac.2012.08.025
103. Meng H, Wu J, Shen L, Chen G, Jin L, Yan M, et al. Microwave assisted extraction, characterization of a polysaccharide from Salvia miltiorrhiza Bunge and its antioxidant effects via ferroptosis-mediated activation of the Nrf2/HO-1 pathway. Int J Biol Macromol. (2022) 215:398–412. doi: 10.1016/j.ijbiomac.2022.06.064
104. Wang X, Gao A, Jiao Y, Zhao Y, Yang X. Antitumor effect and molecular mechanism of antioxidant polysaccharides from Salvia miltiorrhiza Bunge in human colorectal carcinoma LoVo cells. Int J Biol Macromol. (2018) 108:625–34. doi: 10.1016/j.ijbiomac.2017.12.006
105. Jiang YY, Li YB, Yu J, Chen H, Zhou J, Wang L, et al. Preliminary structure and bioactivities of polysaccharide SMWP-U&E isolated from Salvia miltiorrhiza Bunge Residue. Int J Biol Macromol. (2020) 157:434–43. doi: 10.1016/j.ijbiomac.2020.04.092
106. Liang YY, Li KW, Niu FJ, Li Y, Wei HC, Dai YL, et al. Br polysaccharides (SPP) against RSV (respiratory syncytial virus) infection: antiviral effect and mechanisms of action. Biomed Pharmacother. (2021) 141:111843. doi: 10.1016/j.biopha.2021.111843
107. Zhang D, Jin G, Liu W, Dou M, Wang X, Shi W, et al. Salvia miltiorrhiza polysaccharides ameliorates Staphylococcus aureus-induced mastitis in rats by inhibiting activation of the NF-κB and MAPK signaling pathways. BMC Vet Res. (2022) 18:201. doi: 10.1186/s12917-022-03312-6
108. Jiang Y, Wang L, Zhang L, Wang T, Zhou Y, Ding C, et al. Optimization of extraction and antioxidant activity of polysaccharides from Salvia miltiorrhiza Bunge residue. Int J Biol Macromol. (2015) 79:533–41. doi: 10.1016/j.ijbiomac.2015.05.024
109. Wang X, Han C, Qin J, Wei Y, Qian X, Bao Y, et al. Pretreatment with salvia miltiorrhiza polysaccharides protects from lipopolysaccharides/d-Galactosamine-induced liver injury in mice through inhibiting tlr4/myd88 signaling pathway. J Interferon Cytokine Res. (2019) 39:495–505. doi: 10.1089/jir.2018.0137
110. Chen Y, Li H, Li M, Niu S, Wang JH, Shao T, et al. Salvia miltiorrhiza polysaccharide activates T Lymphocytes of cancer patients through activation of TLRs mediated -MAPK and -NF-κB Signaling Pathways. J Ethnopharmacol.. (2017) 200:165–73. doi: 10.1016/j.jep.2017.02.029
111. Chen D, Yang L, Yang F, Pei Q, Lu L, Huang X, et al. Salvia miltiorrhiza polysaccharide activated macrophages and improved the disease resistance of sturgeon against Aeromonas hydrophila. Fish Shellfish Immunol. (2022) 127:594–603. doi: 10.1016/j.fsi.2022.06.062
112. Lu CY, Zhang L, Geng YM, Li SY, Shi WY, Zhai XH. Protective effect of Salvia miltiorrhiza polysaccharides on drug-induced renal injury in broilers. Chin J Vet Sci. (2022) 42:337–43. doi: 10.16303/j.cnki.1005-4545.2022.02.21
113. Y. M. W. Li. Effect of different levels of Salvia miltiorrhiza extract on growth performance, immune function and antioxidant capacity of white feathered broilers. Feed Research. (2021) 44:55–8. doi: 10.13557/j.cnki.issn1002-2813.2021.12.013
114. Liu W, Liu Y, Fang S, Yao W, Wang X, Bao Y, et al. Salvia miltiorrhiza polysaccharides alleviates florfenicol-induced liver metabolic disorder in chicks by regulating drug and amino acid metabolic signaling pathways. Poult Sci. (2022) 101:101989. doi: 10.1016/j.psj.2022.101989
115. Geng Y, Lu C, Jin G, Li S, Cui Y, Han C, et al. Study on the mechanism of Salvia miltiorrhiza polysaccharides in relieving liver injury of broilers induced by florfenicol. Environ Sci Pollut Res Int. (2022) 29:3372–85. doi: 10.1007/s11356-021-15687-4
116. Han C, Wei Y, Wang X, Ba C, Shi W. Protective effect of Salvia miltiorrhiza polysaccharides on liver injury in chickens. Poult Sci. (2019) 98:3496–503. doi: 10.3382/ps/pez153
117. Lu C, Zhang L, Cui Y, Jin G, Bao Y, Shi W. Proteome and transcriptome explore the mechanism of Salvia miltiorrhiza polysaccharides to relieve florfenicol-induced kidney injury in broilers. Environ Sci Pollut Res Int. (2022) 29:45872–84. doi: 10.1007/s11356-022-19114-0
118. Han C, Wang X, Zhang D, Wei Y, Bao Y. Synergistic use of florfenicol and Salvia miltiorrhiza polysaccharide can enhance immune responses in broilers. Ecotoxicol Environ Saf. (2021) 210:111825. doi: 10.1016/j.ecoenv.2020.111825
119. Zhang A, Deng J, Liu X, He P, He L, Zhang F, et al. Structure and conformation of α-glucan extracted from Agaricus blazei Murill by high-speed shearing homogenization. Int J Biol Macromol. (2018) 113:558–64. doi: 10.1016/j.ijbiomac.2018.02.151
120. Zhang Y, Liu D, Fang L, Zhao X, Zhou A, Xie J. A galactomannoglucan derived from Agaricus brasiliensis: purification, characterization and macrophage activation via MAPK and IκB/NFκB pathways. Food Chem. (2018) 239:603–11. doi: 10.1016/j.foodchem.2017.06.152
121. Liu J, Shu M, Wen X, Sun Y. Optimization of polysaccharides (ABP) extraction from the fruiting bodies of Agaricus blazei Murill using response surface methodology (RSM). Carbohydr Polym. (2009) 78:704–9. doi: 10.1016/j.carbpol.2009.06.003
122. Li Y, Sheng Y, Lu X, Guo X, Du P, Isolation and purification of acidic polysaccharides from Agaricus blazei Murill and evaluation of their lipid-lowering mechanism. Int J Biol Macromol. (2020) 157:190. doi: 10.1016/j.ijbiomac.2020.04.190
123. Zhang J, Gao Z, Li S, Gao S, Ren Z, Jing H, et al. Characterization, antioxidation, and antiaging properties of exopolysaccharides and endopolysaccharides of the royal sun medicinal mushroom, Agaricus brasiliensis (Agaricomycetes). Int J Med Mushrooms. (2016) 18:1071–81. doi: 10.1615/IntJMedMushrooms.v18.i12.20
124. Angeli J, Ribeiro LR, Bellini MF, Mantovani MS. Beta-glucan extracted from the medicinal mushroom Agaricus blazei prevents the genotoxic effects of benzo[a]pyrene in the human hepatoma cell line HepG2. Arch Toxicol. (2009) 83:81–6. doi: 10.1007/s00204-008-0319-5
125. Wang P, Li XT, Sun L, Shen L. Anti-Inflammatory Activity of Water-Soluble Polysaccharide of Agaricus blazei Murill on Ovariectomized Osteopenic Rats. Evid Based Complement Alternat Med. (2013) 2013:164817. doi: 10.1155/2013/164817
126. Jia S, Li F, Liu Y. Effects of extraction methods on the antioxidant activities of polysaccharides from Agaricus blazei Murrill. Int J Biol Macromol. (2013) 62:66–9. doi: 10.1016/j.ijbiomac.2013.08.031
127. Lin MH, Lee KM, Hsu CY, Peng SY, Lin CN, Chen CC. Immunopathological effects of Agaricus blazei Murill polysaccharides against Schistosoma mansoni infection by Th1 and NK1 cells differentiation. Int Immunopharmacol. (2019) 73:502–14. doi: 10.1016/j.intimp.2019.05.045
128. Cheng F, Yan X, Zhang M, Chang M, Yun S, Meng J, et al. Regulation of RAW 264. 7 cell-mediated immunity by polysaccharides from Agaricus blazei Murill via the MAPK signal transduction pathway. Food Function. (2017) 8:1475–80. doi: 10.1039/C6FO01332E
129. Lv A, Ge M, Hu X, Liu W, Li G, Zhang R. Effects of Agaricus blazei Murill polysaccharide on cadmium poisoning on the MDA5 signaling pathway and antioxidant function of chicken peripheral blood lymphocytes. Biol Trace Elem Res. (2018) 181:122–32. doi: 10.1007/s12011-017-1012-4
130. Li R, Zhang L, Tang Z, Li T, Li G, Zhang R, et al. Effects of fungal polysaccharide on oxidative damage and TLR4 pathway to the central immune organs in cadmium intoxication in chickens. Biol Trace Elem Res. (2019) 191:464–73. doi: 10.1007/s12011-018-1627-0
131. Xie W, Lv A, Li R, Tang Z, Ma D, Huang X, et al. Agaricus blazei Murill polysaccharides protect against cadmium-induced oxidative stress and inflammatory damage in chicken spleens. Biol Trace Elem Res. (2018) 184:247–58. doi: 10.1007/s12011-017-1189-6
132. Liu W, Ge M, Hu X, Lv A, Ma D, Huang X, et al. the effects of agaricus blazei murill polysaccharides on cadmium-induced apoptosis and the tlr4 signaling pathway of peripheral blood lymphocytes in chicken. Biol Trace Elem Res. (2017) 180:153–63. doi: 10.1007/s12011-017-0969-3
133. Hu X, Zhang R, Xie Y, Wang H, Ge M. The protective effects of polysaccharides from agaricus blazei murill against cadmium-induced oxidant stress and inflammatory damage in chicken livers. Biol Trace Elem Res. (2017) 178:117–26. doi: 10.1007/s12011-016-0905-y
134. Song Y, Zhang R, Wang H, Yan Y, Ming G. Protective effect of agaricus blazei polysaccharide against cadmium-induced damage on the testis of chicken. Biol Trace Elem Res. (2018) 184:491–500. doi: 10.1007/s12011-017-1196-7
135. Ren CG, Liu ZY, Zhong ZH, Wang XL, Qin S. Integrated biotechnology to mitigate green tides. Environ Pollut. (2022) 309:119764. doi: 10.1016/j.envpol.2022.119764
136. Lopes N, Ray S, Espada SF, Bomfim WA, Ray B, Faccin-Galhardi LC, et al. Green seaweed Enteromorpha compressa (Chlorophyta, Ulvaceae) derived sulphated polysaccharides inhibit herpes simplex virus. Int J Biol Macromol. (2017) 102:605–12. doi: 10.1016/j.ijbiomac.2017.04.043
137. Jiao L, Li X, Li T, Jiang P, Zhang L, Wu M, et al. Characterization and anti-tumor activity of alkali-extracted polysaccharide from Enteromorpha intestinalis. Int Immunopharmacol. (2009) 9:324–9. doi: 10.1016/j.intimp.2008.12.010
138. Zhang Z, Wang X, Zhao M, Yu S, Qi H. The immunological and antioxidant activities of polysaccharides extracted from Enteromorpha linza. Int J Biol Macromol. (2013) 57:45–9. doi: 10.1016/j.ijbiomac.2013.03.006
139. Li B, Liu S, Xing R, Li K, Li R, Qin Y, et al. Degradation of sulfated polysaccharides from Enteromorpha prolifera and their antioxidant activities. Carbohydr Polym. (2013) 92:1991–6. doi: 10.1016/j.carbpol.2012.11.088
140. Yuan X, Zheng J, Ren L, Jiao S, Feng C, Du Y, et al. Enteromorpha prolifera oligomers relieve pancreatic injury in streptozotocin (STZ)-induced diabetic mice. Carbohydr Polym. (2019) 206:403–11. doi: 10.1016/j.carbpol.2018.11.019
141. Ren R, Yang Z, Zhao A, Huang Y, Lin S, Gong J, et al. Sulfated polysaccharide from Enteromorpha prolifera increases hydrogen sulfide production and attenuates non-alcoholic fatty liver disease in high-fat diet rats. Food Funct. (2018) 9:4376–83. doi: 10.1039/C8FO00518D
142. Chi Y, Li Y, Zhang G, Gao Y, Ye H, Gao J, et al. Effect of extraction techniques on properties of polysaccharides from Enteromorpha prolifera and their applicability in iron chelation. Carbohydr Polym. (2018) 181:616–23. doi: 10.1016/j.carbpol.2017.11.104
143. Li J, Chi Z, Yu L, Jiang F, Liu C. Sulfated modification, characterization, and antioxidant and moisture absorption/retention activities of a soluble neutral polysaccharide from Enteromorpha prolifera. Int J Biol Macromol. (2017) 105:1544–53. doi: 10.1016/j.ijbiomac.2017.03.157
144. Liu WC, Zhuang DP, Zhao Y, Balasubramanian B, Zhao ZH. Seaweed-derived polysaccharides attenuate heat stress-induced splenic oxidative stress and inflammatory response via regulating Nrf2 and NF-κB signaling pathways. Mar Drugs. (2022) 20:358. doi: 10.3390/md20060358
145. Guo Y, Zhao ZH, Pan ZY, An LL, Balasubramanian B, Liu WC, New insights into the role of dietary marine-derived polysaccharides on productive performance, egg quality, antioxidant capacity, and jejunal morphology in late-phase laying hens. Poultry Sci. (2020) 992:100–7 doi: 10.1016/j.psj.2019.12.032
146. Liu WC, Guo Y, Zhao ZH, Jha R, Balasubramanian B. Algae-derived polysaccharides promote growth performance by improving antioxidant capacity and intestinal barrier function in broiler chickens. Front Vet Sci. (2020) 7:601336. doi: 10.3389/fvets.2020.601336
147. Qiu SJ, Zhang R, Guo Y, Zhao Y, Zhao ZH, Liu WC. Transcriptome analysis reveals potential mechanisms of the effects of dietary Enteromorpha polysaccharides on bursa of Fabricius in broilers. Vet Med Sci. (2021) 7:1881–9. doi: 10.1002/vms3.573
148. Zhao Y, Balasubramanian B, Guo Y, Qiu SJ, Jha R, Liu WC. Dietary enteromorpha polysaccharides supplementation improves breast muscle yield and is associated with modification of mrna transcriptome in broiler chickens. Front Vet Sci. (2021) 8:337. doi: 10.3389/fvets.2021.663988
149. Wassie T, Lu Z, Duan X, Xie C, Gebeyew K, Yumei Z, et al. Dietary enteromorpha polysaccharide enhances intestinal immune response, integrity, and caecal microbial activity of broiler chickens Front Nutr.(2021) 8:783819. doi: 10.3389/fnut.2021.783819
150. Yu C, Zhou C, Tan Z, Cai X, Wang S. Effects of Enteromorpha polysaccharide dietary addition on the diversity and relative abundance of ileum flora in laying hens. Microb Pathog. (2021) 158:105004. doi: 10.1016/j.micpath.2021.105004
151. Liu WC, Ou BH, Liang ZL, Zhang R, Zhao ZH. Algae-derived polysaccharides supplementation ameliorates heat stress-induced impairment of bursa of Fabricius via modulating NF-κB signaling pathway in broilers. Poult Sci. (2021) 100:101139. doi: 10.1016/j.psj.2021.101139
152. Guo Y, Balasubramanian B, Zhao ZH, Liu WC. Marine algal polysaccharides alleviate aflatoxin B1-induced bursa of Fabricius injury by regulating redox and apoptotic signaling pathway in broilers. Poult Sci. (2021) 100:844–57. doi: 10.1016/j.psj.2020.10.050
153. Zhang CH, Yu Y, Liang YZ, Chen XQ. Purification, partial characterization and antioxidant activity of polysaccharides from Glycyrrhiza uralensis. Int J Biol Macromol. (2015) 79:681–6. doi: 10.1016/j.ijbiomac.2015.05.060
154. Zhang X, Kong X, Hao Y, Zhang X, Zhu Z. Chemical structure and inhibition on α-glucosidase of polysaccharide with alkaline-extracted from glycyrrhiza inflata residue. Int J Biol Macromol. (2020) 147:1125–35. doi: 10.1016/j.ijbiomac.2019.10.081
155. Rozi P, Abuduwaili A, Ma S, Bao X, Xu H, Zhu J, et al. Isolations, characterizations and bioactivities of polysaccharides from the seeds of three species Glycyrrhiza. Int J Biol Macromol. (2020) 145:364–71. doi: 10.1016/j.ijbiomac.2019.12.107
156. Pan LC, Zhu YM, Zhu ZY, Xue W, Liu CY, Sun HQ, et al. Chemical structure and effects of antioxidation and against α-glucosidase of natural polysaccharide from Glycyrrhiza inflata Batalin. Int J Biol Macromol. (2020) 155:560–71. doi: 10.1016/j.ijbiomac.2020.03.192
157. Mutaillifu P, Bobakulov K, Abuduwaili A, Huojiaaihemaiti H, Nuerxiati R, Aisa HA, et al. Structural characterization and antioxidant activities of a water soluble polysaccharide isolated from Glycyrrhiza glabra. Int J Biol Macromol. (2020) 144:751–9. doi: 10.1016/j.ijbiomac.2019.11.245
158. Aipire A, Yuan P, Aimaier P, Cai S, Mahabati M, Lu L, et al. Preparation, characterization, and immuno-enhancing activity of polysaccharides from Glycyrrhiza uralensis. Biomolecules. (2020) 10:159. doi: 10.3390/biom10010159
159. Wu Y, Zhou H, Wei K, Zhang T, Che Y, Nguy?n AD, et al. Structure of a new glycyrrhiza polysaccharide and its immunomodulatory activity. Front Immunol. (2022) 13:1007186. doi: 10.3389/fimmu.2022.1007186
160. Zhang X, Zhao S, Song X, Jia J, Zhang Z, Zhou H, et al. Inhibition effect of glycyrrhiza polysaccharide (GCP) on tumor growth through regulation of the gut microbiota composition. J Pharmacol Sci. (2018) 137:324–32. doi: 10.1016/j.jphs.2018.03.006
161. Reda FM, El-Saadony MT, El-Rayes TK, Farahat M, Attia G, Alagawany M. Dietary effect of licorice (Glycyrrhiza glabra) on quail performance, carcass, blood metabolites and intestinal microbiota. Poult Sci. (2021) 100:101266. doi: 10.1016/j.psj.2021.101266
162. Zhang S, Zhu C, Xie H, Wang L, Hu J. Effect of Gan Cao (Glycyrrhiza uralensis Fisch) polysaccharide on growth performance, immune function, and gut microflora of broiler chickens. Poult Sci. (2022) 101:102068. doi: 10.1016/j.psj.2022.102068
163. Qiao Y, Liu C, Guo Y, Zhang W, Guo W, Oleksandr K, et al. Polysaccharides derived from Astragalus membranaceus and Glycyrrhiza uralensis improve growth performance of broilers by enhancing intestinal health and modulating gut microbiota. Poult Sci. (2022) 101:101905. doi: 10.1016/j.psj.2022.101905
164. Ibrahim D, Sewid AH, Arisha AH, Abd El-Fattah AH, Abdelaziz AM, Al-Jabr OA, et al. Influence of glycyrrhiza glabra extract on growth, gene expression of gut integrity, and campylobacter jejuni colonization in broiler chickens. Front Vet Sci. (2020) 7:612063. doi: 10.3389/fvets.2020.612063
165. Abo-Samaha MI, Alghamdi YS, El-Shobokshy SA, Albogami S, El-Maksoud EM, Farrag F, et al. licorice extract supplementation affects antioxidant activity, growth-related genes, lipid metabolism, and immune markers in broiler chickens. Life. (2022) 12:914. doi: 10.3390/life12060914
166. Zhang C, Li CX, Chen WB, Ma YB. Effects of Glycyrrhiza polysaccharide in diet on growth performance, serum antioxidant capacity, and biochemistry of broilers. Poultry Sci. (2020) 100:25. doi: 10.1016/j.psj.2020.12.025
167. Rashidi N, Khatibjoo A, Taherpour K, Akbari-Gharaei M, Shirzadi H. Effects of licorice extract, probiotic, toxin binder and poultry litter biochar on performance, immune function, blood indices and liver histopathology of broilers exposed to aflatoxin-B1 - ScienceDirect. Poult Sci. (2020) 99:5896–906. doi: 10.1016/j.psj.2020.08.034
168. Wu Y, Li N, Zhang T, Che Y, Duan K, Wang Y, et al. Glycyrrhiza polysaccharides can improve and prolong the response of chickens to the Newcastle disease vaccine. Poultry Sci. (2021) 101:549. doi: 10.1016/j.psj.2021.101549
169. Dziewulska D, Stenzel T, Smiałek M, Tykałowski B, Koncicki A. The impact of Aloe vera and licorice extracts on selected mechanisms of humoral and cell-mediated immunity in pigeons experimentally infected with PPMV-1. BMC Vet Res. (2018) 14:148. doi: 10.1186/s12917-018-1467-3
170. Dziewulska D, Stenzel T, Smialek M, Tykalowski B, Koncicki A. An evaluation of the impact of aloe vera and licorice extracts on the course of experimental pigeon paramyxovirus type 1 infection in pigeons. Poult Sci. (2018) 97:470–6. doi: 10.3382/ps/pex341
171. Long LN, Kang BJ, Jiang Q, Chen JS. Effects of dietary Lycium barbarum polysaccharides on growth performance, digestive enzyme activities, antioxidant status, and immunity of broiler chickens. Poult Sci. (2020) 99:744–51. doi: 10.1016/j.psj.2019.10.043
172. Yang S, Shan C, Ma X, Qin Y, Ju A, Duan A. Immunomodulatory effect of Acanthopanax senticosus polysaccharide on immunosuppressed chickens. Poult Sci. (2021) 100:623–30. doi: 10.1016/j.psj.2020.11.059
173. Long LN, Zhang HH, Wang F, Yin YX, Yang LY, Chen JS. Research Note: Effects of polysaccharide-enriched Acanthopanax senticosus extract on growth performance, immune function, antioxidation, and ileal microbial populations in broiler chickens. Poult Sci. (2021) 100:101028. doi: 10.1016/j.psj.2021.101028
174. Li X, Chen S, Li JE, Wang N, Liu X, An Q, et al. Chemical Composition and Antioxidant Activities of Polysaccharides from Yingshan Cloud Mist Tea. Oxid Med Cell Longev. (2019) 2019:1915967. doi: 10.1155/2019/1915967
175. Xiang L, Si C, Zhao ZT, Meng Z, Yi H, Ye XM, et al. Effects of polysaccharides from Yingshan Yunwu tea on meat quality, immune status and intestinal microflora in chickens. Int J Biol Macromol. (2020) 155:61–70. doi: 10.1016/j.ijbiomac.2020.03.198
176. Abdullahi AY, Kallon S, Yu X, Zhang Y, Li G. Vaccination with astragalus and ginseng polysaccharides improves immune response of chickens against H5N1 avian influenza virus. Biomed Res Int. (2016) 2016: 1510264. doi: 10.1155/2016/1510264
177. Chen X, Yang H, Jia J, Chen Y, Wang J, Chen H. Mulberry leaf polysaccharide supplementation contributes to enhancing the respiratory mucosal barrier immune response in Newcastle disease virus-vaccinated chicks. Poult Sci. (2021) 100:592–602. doi: 10.1016/j.psj.2020.11.039
178. Chen X, Sheng Z, Qiu S, Yang H, Jia J, Wang J, et al. characterization and in vitro and in vivo immune enhancement of polysaccharides from mulberry leaves. PLoS One. (2019) 14:e0208611. doi: 10.1371/journal.pone.0208611
179. Xing YY, Zheng YK, Yang S, Zhang LH, Guo SW, Shi LL, et al. Artemisia ordosica Polysaccharide Alleviated Lipopolysaccharide-induced Oxidative Stress of Broilers via Nrf2/Keap1 and TLR4/NF-κB Pathway. Ecotoxicol Environ Saf. (2021) 223:112566. doi: 10.1016/j.ecoenv.2021.112566
180. Shu G, Xu D, Zhao J, Yin L, Lin J, Fu H, et al. Protective effect of Polygonatum sibiricum polysaccharide on cyclophosphamide-induced immunosuppression in chickens. Res Vet Sci. (2021) 135:96–105. doi: 10.1016/j.rvsc.2020.12.025
181. Gan F, Yang Y, Chen Y, Che C, Pan C, Huang K. Bush sophora root polysaccharide could help prevent aflatoxin B1-induced hepatotoxicity in the primary chicken hepatocytes. Toxicon. (2018) 150:180–7. doi: 10.1016/j.toxicon.2018.05.019
182. Yao L, Bai L, Tan Y, Sun J, Qu Q, Shi D, et al. The immunoregulatory effect of sulfated Echinacea purpurea polysaccharide on chicken bone marrow-derived dendritic cells. Int J Biol Macromol. (2019) 139:1123–32. doi: 10.1016/j.ijbiomac.2019.08.028
183. Zhao X, Wang Y, Yan P, Cheng G, Wang C, Geng N. Effects of polysaccharides from platycodon grandiflorum on immunity-enhancing activity in vitro. Molecules. (2017) 22:918. doi: 10.3390/molecules22111918
184. Zheng P, Fan W, Wang S, Hao P, Wang YH, Wan Z, et al. Characterization of polysaccharides extracted from Platycodon grandiflorus (Jacq) ADC affecting activation of chicken peritoneal macrophages. Int J Biol Macromol. (2017) 96:775–85. doi: 10.1016/j.ijbiomac.2016.12.077
Keywords: plant polysaccharides, immune regulation, intestinal health, immune adjuvant, poultry
Citation: Zhao R-H, Yang F-X, Bai Y-C, Zhao J-Y, Hu M, Zhang X-Y, Dou T-F and Jia J-J (2023) Research progress on the mechanisms underlying poultry immune regulation by plant polysaccharides. Front. Vet. Sci. 10:1175848. doi: 10.3389/fvets.2023.1175848
Received: 28 February 2023; Accepted: 28 March 2023;
Published: 17 April 2023.
Edited by:
Hetron M. Munang'andu, Nord University, NorwayReviewed by:
Zhenguang Liu, Nanjing Agricultural University, ChinaYun Peng Fan, Northwest A&F University, China
Copyright © 2023 Zhao, Yang, Bai, Zhao, Hu, Zhang, Dou and Jia. This is an open-access article distributed under the terms of the Creative Commons Attribution License (CC BY). The use, distribution or reproduction in other forums is permitted, provided the original author(s) and the copyright owner(s) are credited and that the original publication in this journal is cited, in accordance with accepted academic practice. No use, distribution or reproduction is permitted which does not comply with these terms.
*Correspondence: Jun-Jing Jia, anVuamluZ2xpMjAwOSYjeDAwMDQwO2hvdG1haWwuY29t; Teng-Fei Dou, dGVuZ2ZlaWRvdSYjeDAwMDQwO3NpbmEuY29t
†These authors have contributed equally to this work