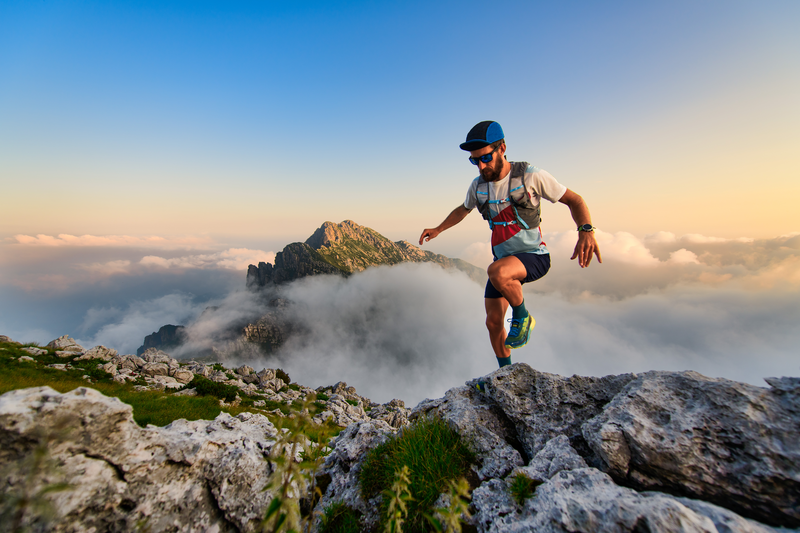
94% of researchers rate our articles as excellent or good
Learn more about the work of our research integrity team to safeguard the quality of each article we publish.
Find out more
ORIGINAL RESEARCH article
Front. Vet. Sci. , 07 September 2023
Sec. Animal Nutrition and Metabolism
Volume 10 - 2023 | https://doi.org/10.3389/fvets.2023.1170488
Information on the availability of Cu from plant feedstuffs for broilers in the presence of phytase is scarce. The present research has been conducted with the objective of evaluating the Cu requirements of broilers when fed corn-soy diets with or without phytase. A total of 640 one-day-old male Cobb x Cobb 500, allocated into 80 battery cages with 8 chicks in each, were fed a low Cu content diet (formulated with 8.58 ± 0.21 mg/kg Cu) without phytase from placement to day 7. Starting on day 8, battery cages were distributed into a 2 × 5 factorial arrangement (phytase-added diets X 5 with graded increases of supplemental Cu) until day 28. Feeding treatments (feeds added or not with phytase and 5 graded increases of Cu) were randomly distributed with 8 cages of 8 chicks. The basal non-supplemented feeds were formulated with corn and soybean meal (SBM) without any other significant Cu contributors. Supplemental Cu was from laboratory-grade Cu sulfate pentahydrate (CuSO5H20) which was increasingly added to the feeds. Phytase was added in excess to the producer recommendation (2,500 FYT) and had average analyzed values of 2,768 ± 135.2 FYT/kg whereas analyzed Cu values were: 8.05 ± 0.25, 11.25 ± 0.15, 14.20 ± 0.40, 16.55 ± 0.05, and 19.45 ± 0.45 mg/kg. Statistics were conducted using linear and quadratic polynomial regression models. No interactions occurred between dietary Cu and phytase (p > 0.05) for any response and no effects were found for the individual factors (phytase or dietary Cu) for Ht, Hb, varus, valgus, rotated tibia, and tibia breaking strength, as well as for Cu contents in breast, gastrocnemius tendon, and kidney (p > 0.05). However, the phytase-added diets led to higher BWG, lower FCR, and increased ileal digestible Cu (p < 0.05). The gradual increase in dietary Cu produced linear increases in Cu content in livers, as well as in excreta and retention (p < 0.05). Supplementing phytase at levels expected to maximize phytate degradation was demonstrated to improve BWG and FCR; however, no effects were observed when dietary Cu was increased to a maximum of 19.45 mg/kg. An increase of 8.8% in ileal digestible Cu was observed when birds were fed phytase.
Routine supplementation of dietary Cu, as well as other trace minerals, is intended to prevent deficiencies that originate from the lack of sufficient amounts of this micromineral in the feeds. Copper sulfate (CuSO4) is traditionally utilized as the source Cu of supplementation in poultry feeds because of its wide availability and low cost (1).
Total supplemental Cu in commercially formulated broiler feeds is variable, as can be observed in the recommendation tables, from as low as 6.5 in the NRC (2) to as high as 15 mg/kg (3) with others standing in between [10.5 mg/kg from Rostagno et al. (4) and 10.0 mg/kg from Aviagen (5)]. Since these are supplemental recommendations, Cu originating from feed ingredients, other than the supplemental source, is usually not taken into consideration for broilers. Copper has also been frequently added to broiler feeds at levels much higher than those suggested, intending to improve performance (6–9). The mode of action of such excessive dietary Cu supplementation is unknown, although it likely relates to antimicrobial properties (10).
Copper has several important functions in poultry, participating in bone formation, Fe transport, and in ceruloplasmin and cytochrome C oxidase and hemoglobin synthesis (11–14). Also, as a component of ferroxidase, Cu is important in the conversion of Fe+2 into Fe+3 (10, 15), which is required for Fe absorption and transport in animal organisms. Dietary Cu deficiency results in reduced blood hemoglobin (Hb), inducing anemia (16) and therefore blood parameters such as hematocrit (Ht) and Hb are frequently used to assess Cu bioavailability (17). As a cofactor of lysyl oxidase, Cu supports the formation of collagen and elastin and its deficiency leads to inadequate cartilage formation (18, 19). However, excess dietary Cu leads to hepatocellular degeneration, necrosis, or visual presence of Kupffer cells (20).
The published bioavailability of Cu for broilers varies from 80% in corn (21) to as low as 40% in soybean meal (SBM) (22). A major concern regarding the availability of Cu is related to its potential chelation to phytic acid (1,2,3,4,5,6 myo-inositol hexakis dihydrogen phosphate), a main storage form of P in plants that is widely distributed in feedstuffs (23–25). Phytic acid has a high density of negatively charged phosphate groups, therefore rendering it highly reactive with di- and trivalent cations. Commercial phytases have been widely added to poultry feeds with successful releases of significant amounts of P from phytic acid (26–30). Demonstrations of increased availability of trace minerals by phytase are much more limited than for Ca and P, even though increased ileal digestibility of Fe (31), Zn, Mn, and Cu have been recently shown (32).
Total Cu in excreta increases with its dietary intake (8, 10, 33, 34). Concerns about the excessive excretion of trace minerals and environmental pollution have been growing around the world, which has led to the establishment of maximum upper limits for total Cu in feeds of 25 ppm in the European Union (35).
The objective of the present study was to assess the availability of Cu for broilers when phytase was added to their feeds. Phytase was added at levels above current market usage such that any significant release of Cu could be detected under a potential extensive degradation of phytate. Supplementation of Cu was done using Cu sulfate such that feeds with values that mimic its contents in commercial corn-soy feeds were reached and therefore no limitations in growth could be attributed to its deficiency.
The Ethics and Research Committee of the Federal University of Rio Grande do Sul, Porto Alegre, Brazil, approved all procedures used in the present study (Protocol number: 40248).
A total of 640 one-day-old male Cobb ×Cobb 500 slow feathering broiler chicks were obtained after incubating eggs in the University hatchery and were allocated into 80 battery cages (experimental units) (0.9 × 0.4 m2). The chicks were vaccinated for Marek’s disease and feather sexed to keep only males. The temperature at placement was 32°C, which was adjusted weekly to maintain bird comfort throughout the study. The chicks had ad libitum access to water and mash feeds. Lighting was provided for 24 h continuously throughout the study. All battery pens were daily checked for sick and dead birds.
The birds were fed a diet of corn-SBM without phytase and not supplemented with Cu (8.58 ± 0.2 ppm analyzed) from day 1 to 7 (Table 1). This was a low Cu diet intending to reduce in Cu stored in the body. A random allocation of birds to treatments was in a 2 vs. 5 factorial: feeds with or without phytase and 5 graded increases of supplemental Cu. Therefore, a total of 10 treatments with 8 replications of 8 birds each were utilized. Birds were kept until 28 days of age. Dietary treatments consisted of corn-SBM feeds with energy and nutrients to optimize live performance as usually formulated in broiler integrations. Feeds were mixed in batches of 400 kg and provided as mash. The phytase utilized in the present study was a commercially available product added at 125 g per ton (Ronozyme HiPhos with 20,000 FYT/g, Novozymes A/S, Bagsvaerd, Denmark), expecting to deliver 2,500 FYT/kg (2,768 ± 135.2 analyzed). The phytase in the present study was added in excess of its commercial recommendation of 1,000 FYT, such that the vast majority of the phytate present in corn and SBM was overwhelmingly degraded. The product was added on top of the feeds without attribution of value for P and Ca in mixes of 1 kg diluted with SBM corresponding to the contents expected in the treatments. Supplementation of Cu was from laboratory-grade sulfate pentahydrate (CuSO5H2O) at 0, 3.0, 6.0, 9.0, and 12.0 mg/kg. Calcium carbonate and phosphoric acid were also laboratory-grade without significant Cu contents. The final formulated Cu in feeding treatments was 8.31, 11.31, 14.31, 17.31, and 20.31 mg/kg. The feeds provided from days 8 to 28 had the inclusion of 1% indigestible marker (as is, Celite, Celite Corp., Lompoc, CA) and had an average geometric diameter of 1.124 μm with a standard deviation of 1.79. The phytase activity in the feeds was measured according to Engelen et al. (36) and expressed in phytase units (FYT, defined as the activity that releases one μmol of inorganic phosphate from 5.0 mM sodium phytate/min at pH 5.5 and 37°C). Analyses of Cu in ingredients and feeds were performed using inductively coupled plasma atomic emission spectroscopy (ICP-Spectro Flamme, Spectro Analytical Instruments, Kleve, Germany) (37).
Table 1. Ingredient and nutrient composition of feeds formulated to evaluate Cu requirements of broilers having phytase.
Body weight gain (BWG), feed intake (FI), and feed conversion ratio corrected for the weight of dead birds (FCR) were measured at the intervals between 8 to 14, 14 to 21, and 21 to 28 d as well as in the overall period (8 to 28 d). From 26 to 28 d, the total amount of feed consumed was recorded and the corresponding excreta were collected. Excreta were pooled by cage and stored frozen at −20°C immediately after collection in plastic containers. Ileal contents were collected from all birds from each cage at 28 d after euthanasia by electrical stunning using 45 V for 3 s. Contents were collected from the terminal two-thirds ileum, which was defined as the region between Meckel’s diverticulum to 2 cm cranial to the ileocecal junction. Contents were flushed with distilled water into plastic containers, pooled by battery cage, immediately frozen in liquid nitrogen, and stored in a freezer at −20°C until lyophilized. Feed samples and ileal contents were analyzed to determine dry matter (DM), gross energy (GE), and total Cu. Calculations of ileal digestible energy (IDE) were done afterward. Apparent ileal digestibility was calculated using the equations from Kong and Adeola (38) as follows: digestibility (%) = [1 – (Mi/Mo) × (Eo/Ei)] × 100, where Mi represents the concentration of acid insoluble ash in the feeds in grams per kilogram of DM; Mo represents the concentration of acid insoluble ash in the ileal digesta in grams per kilogram of DM output; Ei represents the concentration of DM, GE, Cu in the diet in milligrams per kilogram of DM; and Eo represents the concentration of DM, GE, Cu, in the ileal digesta in milligrams per kilogram of DM.
At 26 d, valgus, varus, and rotated tibiae deviations at the tibia metatarsal joints were evaluated in all birds standing in the normal anatomic position by a 3 individual panel, classifying them by the presence or the absence of each deviation. Tibiae-metatarsal joints with valgus deviations were those presenting an outward angulation, whereas the varus deviations were inward (39). Rotated tibiae above 90° were considered abnormal and characterized as a torsional rotation of the shaft of the tibiotarsus of 1 or both legs, causing the metatarsus to point laterally and the broiler to assume a spraddle leg posture (40). Scores taken were averaged for the sake of statistical analysis.
Blood samples were taken by heart punctures prior to slaughter from three broilers randomly selected from each cage. The obtained blood was partially transferred to 0.5 mL test tubes containing EDTA for hematocrit (Ht) and hemoglobin (Hb) analyses. Determination of Ht was done using micro capillaries containing blood centrifuged for 5 min at 15,650–18,510×g, whereas Hb was determined using the cyanmethemoglobin method as described by Crosby et al. (41).
Breast muscle (Pectoralys major), liver, kidney, and gastrocnemius tendon samples were collected from all birds after euthanasia at 28 d. Collected samples were weighed and stored in plastic bags by pen and remained at −20°C until analysis. Samples were further ashed and Cu content was determined as done with the feeds. The left tibiae of all birds from each cage were collected and the surrounding muscle tissue was removed. Samples were frozen until analysis. Tibia breaking strength was evaluated using a texture analyzer (TA.XTPlus, Texture Technologies, Hamilton, MA, United States), according to the method proposed by Shim et al. (42).
Data were tested for homoscedasticity and normality of the variance prior to statistical analyses (43). Data that were not normally distributed were square root transformed prior to analyses; however, real means are presented as results. A two-way ANOVA was used to test for differences in the effects of the independent variables (phytase and dietary Cu) on the dependent variables measured. Analyses were done using the GLM procedure of SAS (44). Significance was accepted at p ≤ 0.05. Data were submitted to a two-way ANOVA and mean differences were separated using Tukey’s HSD test.
Estimation of optimum responses to total dietary Cu was done using linear (L) and quadratic polynomial (QP) regression analyses. The L model (Y = β1 + β2 × X) had Y as the dependent variable, X as the dietary concentration of Cu, β1 as the intercept, and β2 as the linear coefficient. The QP model [Y = β1 + β2 × Cu + β3 × (Cu)2] had Y as the dependent variable as a function of the dietary concentration of Cu, β1 as the intercept, β2 as the linear coefficient, and β3 as the quadratic coefficient. The maximum response for Cu concentration would be found as Cu = −β2 ÷ (2 × β3).
The analyzed Cu in the experimental feeds was similar to that expected from the feed formulation (Table 1) and, therefore, the feeds were considered acceptable for the experimental assessment originally planned. The final formulated Cu in feeding treatments were 8.31, 11.31, 14.31, 17.31, 20.31 mg/kg (analyzed Cu in the feeds without phytase were 7.83 ± 0.2, 11.11 ± 0.4, 14.62 ± 0.1, 16.47 ± 0.2, 18.96 ± 0.1, whereas in the feeds with phytase were 8.28 ± 0.2, 11.36 ± 0.6, 13.77 ± 0.3, 16.63 ± 0.2, 19.94 ± 0.4). The final analyzed phytase in the Cu-supplemented feeds was (from the lowest to the highest Cu content feeds) 2,752 ± 171; 2,864 ± 264; 2,831 ± 391; 2,746 ± 282; and 2,747 ± 149 FYT/kg.
Two-way ANOVA and regression analyses were conducted with the Cu-analyzed data whenever no interaction was found.
There was no mortality and no individuals were detected as sick during the study. No interactions between dietary Cu and phytase were found for any evaluated response throughout the study. Individual factors (phytase and Cu) did not affect Ht, Hb, varus, valgus, rotated tibia, tibia breaking strength, or Cu concentration in breast, gastrocnemius tendon, and kidney (p > 0.05; Table 2). However, broilers fed phytase showed higher BWG and lower FCR, and higher FI (p < 0.01; Table 3), IDE, and ileal digestibility of Cu (p < 0.05; Table 4) when compared to broilers that had no phytase supplementation. Responses to Cu supplementation were only observed for Cu intake, excretion, liver content, and retention. These responses were linearly correlated to the increasing dietary Cu as follows: Cu intake = 2.3618 + 0.8507x, R2 = 0.90, p < 0.001; Cu excreted = 0.2605 + 0.2814x, R2 = 0.82, p < 0.001; 1iver Cu content = 2.3617 + 0.85076x, R2 = 0.73, p < 0.001; and Cu retained = 0.2645 + 0.6849x, R2 = 0.80, p < 0.001. There was no response that could be quadratically adjusted, which prevented estimations of optimum dietary levels of Cu.
Table 2. Blood parameters, leg deviations, tibia breaking strength, and Cu content in tissues of broilers fed diets formulated to evaluate the Cu requirements of broilers receiving phytase.
Table 3. Growth performance of broilers fed diets formulated to evaluate the Cu requirements of broilers receiving phytase.
Table 4. Metabolism responses of broilers fed diets formulated to evaluate Cu requirements (dry matter basis)1 when receiving phytase.
The content of Cu in feedstuffs varies depending on its original area of production (2, 45). Copper has been supplemented to broiler feeds because of uncertainty about its availability in plant feedstuffs and, therefore, to avoid deficiency. Nonetheless, poultry excreta has high Cu regardless of the level utilized in feeds, which eventually leads to increased Cu in soils used for agriculture (8, 46, 47). In the present study, diets fed to broilers without Cu supplementation optimized broiler growth up to 28 d. In parallel, no effects were observed for other responses not directly related to growth, regardless of the addition of phytase. Therefore, the recommendations from the NRC (2), Cobb (3), Rostagno et al. (4), and Aviagen (5) were considered excessive whereas the limit of 25 mg/kg imposed by EFSA (35) is well above broiler needs.
The addition of exogenous phytase in poultry feeds is commercially mandatory currently since it significantly increases P availability from plant feedstuffs, leading to the reduction in feed costs due to a lower inclusion of phosphate sources. The increased availability of Ca, protein, amino acids, and other minerals than P has been shown as a secondary benefit from the use of phytases with a consequent reduction in excreta (48–52). In the present study, broilers fed phytase showed higher BWG and lower FCR when compared to those that were not fed the enzyme. Phytase was added on top of the feeds without attributing any P or Ca values in the formulation, therefore, the improvements were thought to have other origins. The contents of non-phytate P (nPP) and Ca in the feed formulations in the present study were as is usual in commercial integrations and similar to popular allowance recommendations [0.45% nPP and 1.00% Ca from the NRC (2); 0.43% nPP and 95% Ca from FEDNA (53); 0.43% nPP and 0.91% Ca from Rostagno et al. (4); 0.42% nPP and 0.84% Ca from Cobb (3)]. Apart from Cu, all other trace minerals were supplemented. Therefore, Cu was the only trace mineral not supplemented and the benefits of phytase regarding the further availability of Cu could be assessed. Phytase additions in feeds in amounts that surpass optimized phytate degradation and capability to provide P from phytate (super doses) have sometimes been reported as extra phosphoric effects with improvements in the availability of energy, amino acids, and in the performance of broilers (54–59).
Hematological analyses can be efficient clinical tools in the diagnosis of metabolic disorders. Although Cu is not a constituent of hemoglobin itself, Cu is an active participant in the oxygen transport process as it is present in certain plasma proteins that are involved in the transport of Fe (12, 60). When varying levels of dietary Cu were evaluated with broilers and broiler breeders, hemoglobin was markedly lower than in those supplemented with Cu (9, 61). In the present study, there were no differences in the measured blood parameters which suggests that Cu in the non-supplemented feeds had sufficient Cu to maximize those measurements properly inducing red-blood-cell formation. Interestingly, it has been earlier suggested by Hill and Matrone (62) that Cu deficiency alone has little effect on hemoglobin concentration and hematocrit values.
Proper bone mineralization demands Cu, which is ultimately derived from the feed (63, 64). Locomotor limitations are currently important morbidity signals and, therefore, indicators of the welfare of broiler chickens (35, 65). In the present experiment, there were no effects of Cu supplementation in the frequency of varus or valgus deviations, or in tibia rotation due to dietary Cu. Day-old chicks fed Cu deficient feeds with increasing levels of Cu for 21 days showed optimum growth when fed 6–8 mg/kg Cu whereas the requirement for normal cross-link formation and bone mineralization was lower than 2 mg/kg Cu (19). The lack of Cu supplementation effects in the gastrocnemius tendon paralleled the outcomes in bone ash in the present study. This tendon is a sensitive indicator of Cu deficiency (66). Thus, it seems that increasing Cu in corn-SBM feeds through Cu sulfate supplementation does not impact the most prevalent locomotor capabilities of broilers.
According to the present results, there were differences among treatments in liver Cu contents when measured on day 28. Previous studies have reported increased Cu in the liver along with its increase in feeds (7, 13, 67–70). Despite the wide range of dietary Cu concentrations fed to poultry in different commercial settings, its proportional increase in livers seemed not to reach a concentration capable of producing signals of toxicity (71, 72). The increase in Cu excretion as well as in body retention that paralleled liver contents in the present study have been earlier noticed by others (33, 73, 74). The frequent ultimate disposal of poultry excreta as a soil amendment will certainly lead to a steady increase of Cu in the environment. Moreover, the linear excretion of Cu that parallels its content in the feeds is an explicit indicator of its dietary excess when supplemented further than what is provided by commercial corn-SBM feeds.
Broiler feeds supplemented with phytase in a corn-SBM diet presented higher IDE in the present study. Leyva-Jimenez et al. (75) reported that phytase added at 1,000 FYT/Kg led to increased IDE in 24-day-old broilers and Walters et al. (76) observed higher IDE when broilers were fed phytase at 1,000 and 2,000 FTY/kg. Supplementation with phytase allows a reduction of endogenous losses in the gastrointestinal tract, allowing an increase in metabolizable energy and a reduction in energy needed for maintenance. This allows for a greater amount of energy for growth (77). The benefits of using phytase at levels higher than 1,000 FYT have been related to higher absorption of myo-inositol with a parallel increase in the retention of dietary energy (48).
In the present study, the phytase addition led to a higher Cu ileal digestibility when compared to broilers that were not fed the enzyme. To the knowledge of this study’s authors, the benefits of phytase on Cu availability have only been reported in pigs (78). The increase in the ileal digestibility of Cu occurred regardless of the level of Cu in the feeds and, therefore, this seems to have resulted from the release of Cu chelated to phytate. The increased coefficient of digestibility of Cu when broilers were fed a high dose of phytase was small; however, this effect was obtained without assessing phytate degradation directly.
Adding phytase at a dose expected to degrade the vast majority of phytate present in broiler feeds leads to growth performance improvements, which seems to be related to the increased utilization of dietary energy. The effect of phytase on Cu digestibility is significant, but small in value when the total Cu requirement is considered. Increases in Cu supplementation to levels that mimic the total provision of this mineral in commercial feeds have no benefit in responses related to growth or blood, or in the most common locomotor problems. Excess dietary Cu leads to its increased content in the liver as well as in excreta, which may lead to an eventual environmental burden.
The raw data supporting the conclusions of this article will be made available by the authors, without undue reservation.
The animal studies were approved by Comissão de Ética no Uso de Animais—UFRGS. The studies were conducted in accordance with the local legislation and institutional requirements. Written informed consent was obtained from the owners for the participation of their animals in this study.
PS: research and analysis. SV: conceptualization and research. JF: research, writing, and analysis. WA: research. GT: research. All authors contributed to the article and approved the submitted version.
Fundação de Apoio da Universidade Federal do Rio Grande do Sul.
The authors acknowledge the partial funding from Brazilian Research Council (CNPq – Brasilia, DF, Brazil).
The authors declare that the research was conducted in the absence of any commercial or financial relationships that could be construed as a potential conflict of interest.
All claims expressed in this article are solely those of the authors and do not necessarily represent those of their affiliated organizations, or those of the publisher, the editors and the reviewers. Any product that may be evaluated in this article, or claim that may be made by its manufacturer, is not guaranteed or endorsed by the publisher.
1. Pang, Y, and Applegate, T. Effects of copper source and concentration on in vitro phytate phosphorus hydrolysis by phytase. J Agric Food Chem. (2006) 54:1792–6. doi: 10.1021/jf052053b
2. NRC, National Research Council. Nutrient requirements of poultry. 9th ed. Washington, DC: Natl. Acad. Press (1994).
3. Cobb-Vantress. COBB 500 broiler performance & nutrition supplement. Siloam Springs, AR: Cobb-Vantress Inc. (2018).
4. Rostagno, HS, Albino, LFT, Hannas, MI, Donzele, JL, Sakomura, NK, Perazzo, FG, et al. Brazilian tables for poultry and swine: composition of foods and nutritional requirements. 4th ed. Viçosa: UFV (2017).
6. Arias, VJ, and Koutsos, EA. Effects of copper source and level on intestinal physiology and growth of broiler chickens. Poult Sci. (2006) 85:999–1007. doi: 10.1093/ps/85.6.999
7. Ewing, HP, Pesti, GM, Bakalli, RI, and Menten, JF. Studies on the feeding of cupric sulfate pentahydrate, cupric citrate, and copper oxychloride to broiler chickens. Poult Sci. (1998) 77:445–8. doi: 10.1093/ps/77.3.445
8. Pesti, G, and Bakalli, RI. Studies on the feeding of cupric sulfate pentahydrate, cupric citrate, and copper oxychloride to broiler chickens. Poult Sci. (1996) 77:445–8. doi: 10.1093/ps/77.3.445
9. Samanta, B, Biswas, A, and Ghosh, PR. Effects of dietary copper supplementation on production performance and plasma biochemical parameters in broiler chickens. Brit Poult Sci. (2011) 52:573–7. doi: 10.1080/00071668.2011.608649
10. Leeson, S. Reviews: copper metabolism and dietary needs. World Poult Sci J. (2009) 65:353–66. doi: 10.1017/S0043933909000269
11. Capaldi, RA. Structure and function of cytochrome c oxidase. Annu Rev Biochem. (1990) 59:569–96. doi: 10.1146/annurev.bi.59.070190.003033
12. Chen, H, Huang, G, Su, T, Gao, H, Attieh, ZK, McKie, AT, et al. Decreased hephaestin activity in the intestine of copper-deficient mice causes systemic iron deficiency. J Nutr. (2006) 136:1236–41. doi: 10.1093/jn/136.5.1236
13. Karimi, A, Sadeghi, GH, and Vaziry, A. The effect of copper in excess of the requirement during the starter period on subsequent performance of broiler chicks. J Appl Poult Res. (2011) 20:203–9. doi: 10.3382/japr.2010-00290
14. Roychoudhury, S, Nath, S, Massanyi, P, Stawarz, R, Kacaniova, M, and Kolesarova, A. Copper-induced changes in reproductive functions: in vivo and vitro effects. Physiol Res. (2016) 65:11–22. doi: 10.33549/physiolres.933063
15. Grotto, HZW. Fisiologia e metabolismo do ferro. Rev Bras Hematol e Hemoter. (2010) 32:8–17. doi: 10.1590/S1516-84842010005000050
16. Baumgartner, S, Jeannett, D, and Leach, RM. Copper deficiency in the laying hen. J Nutr. (1978) 108:804–11. doi: 10.1093/jn/108.5.804
17. Ledoux, DR, Henry, PR, Ammerman, CB, Rao, PV, and Miles, RD. Estimation of the relative bioavailability of inorganic copper sources for chicks using tissue uptake of copper. J Anim Sci. (1991) 69:215–22. doi: 10.2527/1991.691215x
18. Medeiros, DM. Copper, iron and selenium dietary deficiencies negatively impact skeletal integrity: a review. Exp Biol Med (Maywood). (2016) 241:1316–22. doi: 10.1177/1535370216648805
19. Opsahl, W, Zeronian, H, Ellison, M, Lewis, D, Rucker, RB, and Riggins, RS. Role of copper in collagen cross-linking and its influence on selected mechanical properties of chick bone and tendon. J Nutr. (1982) 112:708–16. doi: 10.1093/jn/112.4.708
20. Bozynski, CC, Evans, TJ, Kim, DY, Johnson, GC, Hughes-Hanks, JM, Mitchell, WJ, et al. Copper toxicosis with hemolysis and hemoglobinuric nephrosis in three adult Boer goats. J Vet Diagnostic Investig. (2009) 21:395–400. doi: 10.1177/104063870902100319
21. Aw-Yong, LM, Sim, JS, and Bragg, DB. Mineral availability of corn, barley, wheat, and triticale for the chick. Poult Sci. (1982) 62:659–64. doi: 10.3382/ps.0620659
22. Aoyagi, S, and Baker, DH. Estimates of copper bioavailability from liver of different animal species and from feed ingredients derived from plants and animals. Poult Sci. (1993) 72:1746–55. doi: 10.3382/ps.0721746
23. Oberleas, D. (1973). Phytates. In Toxicants occurring naturally in foods. 2th ed, by F. M. Strong. National Academic of Science, Washington, DC.
24. Vohra, P., Gray, G. A., and Kratzer, F. H. (1965). Phytic acid-metal. Proc Soc Exp Biol Med 120:447–449. doi: 10.3181/00379727-120-30559
25. Wise, A, and Gilburt, DJ. In vitro competition between calcium phytate and the soluble fraction of rat small intestine contents for cadmium, copper and zinc. Toxicol Lett. (1982) 11:49–54. doi: 10.1016/0378-4274(82)90104-7
26. Cowieson, AJ, Ptak, A, Maćkowiak, P, Sassek, M, Pruszyńska-Oszmałek, E, Żyła, K, et al. The effect of microbial phytase and myo-inositol on performance and blood biochemistry of broiler chickens fed wheat/corn-based diets. Poult Sci. (2013) 92:2124–34. doi: 10.3382/ps.2013-03140
27. Maenz, DD, Engele-Schaan, CM, Newkirk, RW, and Classen, HL. The effect of minerals and mineral chelators on the formation of phytase-resistant and phytase-susceptible forms of phytic acid in solution and in a slurry of canola meal. Anim Feed Sci Technol. (1999) 81:177–92. doi: 10.1016/S0377-8401(99)00085-1
28. Naves, LP, Rodrigues, PB, Meneghetti, C, Bernardino, VMP, Oliveira, DH, Saldanha, MM, et al. Efficiency of microbial phytases in diets formulated with different calcium: phosphorus ratios supplied to broilers from 35 to 42 days of age. J Appl Anim Res. (2016) 44:446–53. doi: 10.1080/09712119.2015.1091324
29. Rutherfurd, SM, Chung, TK, Thomas, DV, Zou, ML, and Moughan, PJ. Effect of a novel phytase on growth performance, apparent metabolizable energy, and the availability of minerals and amino acids in a low-phosphorus corn-soybean meal diet for broilers. Poult Sci. (2012) 91:1118–27. doi: 10.3382/ps.2011-01702
30. Tamin, MN, and Angel, R. Phytate phosphorus hydrolysis as influenced by dietary calcium and micro-mineral source in broiler diets. J Agric Food Chem. (2003) 51:4687–93. doi: 10.1021/jf034122x
31. Akter, M, Iji, PA, and Graham, H. Increased iron level in phytase-supplemented diets reduces performance and nutrient utilization in broiler chickens. Br Poult Sci. (2017) 58:409–17. doi: 10.1080/00071668.2017.1315050
32. Moss, AF, Chrystal, PV, Dersjant-Li, Y, Selle, PH, and Liu, SY. Responses in digestibilities of macro-minerals, trace minerals and amino acids generated by exogenous phytase and xylanase in canola meal diets offered to broiler chickens. Anim Feed Sci Technol. (2018) 240:22–30. doi: 10.1016/j.anifeedsci.2018.03.011
33. Dozier, WA, Davis, AJ, Freeman, ME, and Ward, TL. Early growth and environmental implications of dietary zinc and copper concentrations and sources of broiler chicks. Brit Poult Sci. (2003) 44:726–31. doi: 10.1080/00071660310001643714
34. Pesti, GM, and Bakalli, RI. Studies on the effect of feeding cupric sulfate pentahydrate to laying hens on egg cholesterol content. Poult Sci. (1998) 77:1540–5. doi: 10.1093/ps/77.10.1540
35. EFSA. Revision of the currently authorised maximum copper content in complete feed. EFSA panel on additives and products or substances used in animal feed. EFSA J. (2016) 14:4563. doi: 10.2903/j.efsa.2016.4563
36. Engelen, AJ, Van der Heeft, FC, Randsdorp, PHG, and Smit, ELC. Simple and rapid determination of phytase activity. J AOAC Int. (1994) 77:760–4.
37. Anderson, KA. Analytical techniques for inorganic contaminants. Gaithersburg, MD: AOAC Int. (1999).
38. Kong, C, and Adeola, O. Evaluation of amino acid and energy utilization in feedstuff for swine and poultry diets. Asian-Australasian J Anim Sci. (2014) 27:917–25. doi: 10.5713/ajas.2014.r.02
39. Julian, RJ. Valgus-varus deformity of the intertarsal joint in broiler chickens. Can Vet J. (1984) 25:254–8.
40. Thorp, BH. Skeletal disorders in the fowl: a review. Avian Pathol. (1994) 23:203–36. doi: 10.1080/03079459408418991
41. Crosby, WH, Munn, JI, and Furth, FW. Standardizing a method for clinical hemoglobinometry. U S Armed Forces Med J. (1954) 5:693–703.
42. Shim, MY, Karnuah, AB, Mitchell, AD, Anthony, NB, Pesti, GM, and Aggrey, SE. The effects of growth rate on leg morphology and tibia breaking strength, mineral density, mineral content, and bone ash in broilers. Poult Sci. (2012) 91:1790–5. doi: 10.3382/ps.2011-01968
43. Shapiro, SS, and Wilk, MB. An analysis of variance test for normality (complete samples). Biometrika. (1965) 52:591–611. doi: 10.2307/2333709
44. SAS Institute ed. SAS user’s guide: statistics, version 9. 4th ed. Cary, NC: SAS Institute (2012).
45. Aoyagi, S, and Baker, DH. Effect of microbial phytase and 1,25-dihydroxycholecalciferol on dietary copper utilization in chicks. Poult Sci. (1995) 74:121–6. doi: 10.3382/ps.0740121
46. Brainer, MMA, Menten, JFM, Vale, MM, and Morais, SCD. Cupric citrate as growth promoter for broiler chickens in different rearing stages. Sci Agric. (2003) 60:441–5. doi: 10.1590/S0103-90162003000300004
47. Mohanna, C, and Nys, Y. Influence of age, sex and cross on body concentrations of trace elements (zinc, iron, copper and manganese) in chickens. Br Poult Sci. (1998) 39:536–43. doi: 10.1080/00071669888737
48. Bassi, LS, Teixeira, LV, Sens, RF, Almeida, L, Zavelinski, VAB, and Maiorka, A. High doses of phytase on growth performance, bone mineralization, diet utilization, and plasmatic myo-inositol of Turkey poults. Poult Sci. (2021) 100:101050. doi: 10.1016/j.psj.2021.101050
49. Muszy, S, and Tomaszewska, E. Effect of dietary phytase supplementation on bone and hyaline cartilage development of broilers fed with organically complexed copper in a cu-deficient diet. Biol Trace Elem Res. (2017) 182:339–53. doi: 10.1007/s12011-017-1092-1
50. Pirgozliev, V, Oduguwa, O, Acamovic, T, and Bedford, MR. Effects of dietary phytase on performance and nutrient metabolism in chickens. Br Poult Sci. (2008) 49:144–54. doi: 10.1080/00071660801961447
51. Song, T, Yu, C, Zhao, X, Chen, F, Liu, Y, Yang, C, et al. Effects of thermostable phytase supplemented in diets on growth performance and nutrient utilization of broilers. Anim Sci J. (2021) 92:1–8. doi: 10.1111/asj.13513
52. Woyengo, TA, and Nyachoti, CM. Review: supplementation of phytase and carbohydrases to diets for poultry. Can J Anim Sci. (2010) 91:177–92. doi: 10.4141/cjas10081
53. Fundación Española para el Desarrollo de la Nutrición Animal (FEDNA). Necesidades nutricionales para avicultura: pollos de carne y aves de puesta. Madrid, Spain: FEDNA (2008).
54. Ajuwon, KM, Sommerfeld, V, Paul, V, Däuber, M, Schollenberger, M, Kühn, I, et al. Phytase dosing affects phytate degradation and Muc2 transporter gene expression in broiler starters. Poult Sci. (2020) 99:981–91. doi: 10.1016/j.psj.2019.10.016
55. Cowieson, AJ, Aureli, R, Guggenbuhl, P, and Fru-Nji, F. Possible involvement of myo-inositol in the physiological response of broilers to high doses of microbial phytase. Anim Product Sci. (2014) 55:710–9. doi: 10.1071/AN14044
56. Gautier, AE, Walk, CL, and Dilger, RN. Effects of a high level of phytase on broiler performance, bone ash, phosphorus utilization, and phytate dephosphorylation to inositol. Poult Sci. (2018) 97:211–8. doi: 10.3382/ps/pex291
57. Sommerfeld, V, Kunzel, S, Schollenberger, M, Kuhn, I, and Rodehutscord, M. Influence of phytase or myo-inositol supplements on performance and phytate degradation products in the crop, ileum, and blood of broiler chickens. Poult Sci. (2018) 97:920–9. doi: 10.3382/ps/pex390
58. Walk, CL, and Rama Rao, SV. Increasing dietary phytate has a significant anti-nutrient effect on apparent ileal amino acid digestibility and digestible amino acid intake requiring increasing doses of phytase as evidenced by prediction equations in broilers. Poult Sci. (2020) 99:290–300. doi: 10.3382/ps/pez489
59. Woyengo, TA, and Wilson, J. Enhancing nutrient utilization in maize for broiler chickens by superdosing phytase. Anim Feed Sci Technol. (2019) 252:34–40. doi: 10.1016/j.anifeedsci.2019.04.005
60. Reeves, PG, Demars, LCS, Johnson, WT, and Lukaski, HC. Dietary copper deficiency reduces iron absorption and duodenal enterocyte hephaestin protein in male and female rats. J Nutr. (2005) 135:92–8. doi: 10.1093/jn/135.1.92
61. Berwanger, E, Vieira, SL, Angel, CR, Kindlein, L, Mayer, AN, Ebbing, MA, et al. Copper requirements of broiler breeder hens. Poult Sci. (2018) 97:2785–97. doi: 10.3382/ps/pex437
62. Hill, CH, and Matrone, G. Studies on copper and iron deficiencies in growing chickens. J Nutr. (1961) 73:425–31. doi: 10.1093/jn/73.4.425
63. Güz, BC, Molenaar, R, de Jong, IC, Kemp, B, Van den Brand, H, and Van Krimpen, M. Effects of dietary organic minerals, fish oil, and hydrolyzed collagen on growth performance and tibia characteristics of broiler chickens. Poult Sci. (2019) 98:6552–63. doi: 10.3382/ps/pez427
64. Ji, F, Zhang, S, An, Y, Wang, Z, Shao, Y, du, S, et al. Influence of dietary phosphorus concentrations on the performance of rearing pigeons (Columba livia), and bone properties of squabs. Poult Sci. (2022) 101:101744. doi: 10.1016/j.psj.2022.101744
65. Paz, ICLA, Garcia, RG, Bernardi, R, Seno, LO, Naas, IA, and Caldara, FR. Locomotor problems in broilers reared on new and re-used litter. Ital J Anim Sci. (2013) 12:e45. doi: 10.4081/ijas.2013.e45
66. Harris, ED, Gonnerman, WA, Savage, JE, and O’Dell, BL. Connective tissue amine oxidase II. Purification and partial characterization of lysyl oxidase from chick aorta. Biochim Biophys Acta. (1974) 341:332–44. doi: 10.1016/0005-2744(74)90226-5
67. Güclü, BK, Kara, K, Beyaz, L, Uyanik, F, Eren, M, and Atasever, A. Influence of dietary copper proteinate on performance, selected biochemical parameters, lipid peroxidation, liver, and egg copper content in laying hens. Biol Trace Elem Res. (2008) 125:160–9. doi: 10.1590/1806-9061-2017-0536
68. Jegede, AV, Oduguwa, OO, Bamgbose, AM, Fanimo, AO, and Nollet, L. Growth response, blood characteristics and copper accumulation in organs of broilers fed on diets supplemented with organic and inorganic dietary copper sources. Br Poult Sci. (2011) 52:133–9. doi: 10.1080/00071668.2010.544714
69. Wang, Z, Cerrate, S, Coto, C, Yan, F, and Waldroup, PW. Evaluation of mintrex® copper as a source of copper in broiler diets. Int J Poult Sci. (2007) 6:308–13. doi: 10.3923/ijps.2007.308.313
70. Yang, Z, Qi, XM, Yang, HM, Dai, H, Xu, CX, and Wang, ZY. Effects of dietary copper on growth performance, slaughter performance and nutrient content of fecal in growing goslings from 28 to 70 days of age. Brazil J Poult Sci. (2018) 20:45–52. doi: 10.1590/1806-9061-2017-0536
71. Luo, XG, Ji, F, Lin, YX, Steward, FA, Lu, L, Liu, B, et al. Effect of dietary supplementation with copper sulfate or tribasic copper chloride on broiler performance, relative copper bioavailability, and oxidation stability of vitamin E in feed. Poult Sci. (2005) 84:888–93. doi: 10.1093/ps/84.6.888
72. Nguyen, HTT, Kheravii, SK, Wu, S, Roberts, JR, Swick, RA, and Toghyani, M. Sources and levels of copper afect liver copper profle, intestinal morphology and cecal microbiota population of broiler chickens fed wheat-soybean meal diets. Nature. (2022) 12:2249. doi: 10.1038/s41598-022-06204-9
73. Kwiecień, M, Winiarska-Mieczan, A, Piedra, JV, Bujanowicz-Haras, B, and Chalabis-Mazurek, A. Effects of copper glycine chelate on liver and faecal mineral concentrations, and blood parameters in broilers. Agric Food Sci. (2015) 24:92–103. doi: 10.23986/afsci.49511
74. Nollet, L, Huyghebaert, G, and Spring, P. Effect of different levels of dietary organic (Bioplex) trace minerals on live performance of broiler chickens by growth phases. J Appl Poult Res. (2008) 17:109–15. doi: 10.3382/japr.2007-00049
75. Leyva-Jimenez, H, Alsadwi, AM, Gardner, K, Voltura, E, and Bailey, CA. Evaluation of high dietary phytase supplementation on performance, bone mineralization, and apparent ileal digestible energy of growing broilers. Poult Sci. (2019) 98:811–9. doi: 10.3382/ps/pey389
76. Walters, HG, Coelho, M, Coufal, CD, and Lee, JT. Effects of increasing phytase inclusion levels on broiler performance, nutrient digestibility, and bone mineralization in low-phosphorus diets. J Appl Poult Res. (2019) 28:1210–25. doi: 10.3382/japr/pfz087
77. Wu, D, Wu, SB, Choct, M, and Swick, RA. Comparison of 3 phytases on energy utilization of a nutritionally marginal wheat-soybean meal broiler diet. Poult Sci. (2015) 94:2670–6. doi: 10.3382/ps/pev222
Keywords: broiler, copper, phytase, mineral, requirement
Citation: Soster P, Vieira SL, Feijo JC, Altevogt WE and Tormes GB (2023) Dietary phytase effects on copper requirements of broilers. Front. Vet. Sci. 10:1170488. doi: 10.3389/fvets.2023.1170488
Received: 21 February 2023; Accepted: 21 August 2023;
Published: 07 September 2023.
Edited by:
Arda Yıldırım, Gaziosmanpaşa University, TürkiyeReviewed by:
Tugay Ayasan, Osmaniye Korkut Ata University, TürkiyeCopyright © 2023 Soster, Vieira, Feijo, Altevogt and Tormes. This is an open-access article distributed under the terms of the Creative Commons Attribution License (CC BY). The use, distribution or reproduction in other forums is permitted, provided the original author(s) and the copyright owner(s) are credited and that the original publication in this journal is cited, in accordance with accepted academic practice. No use, distribution or reproduction is permitted which does not comply with these terms.
*Correspondence: Sergio Luiz Vieira, c2x2aWVpcmFAdWZyZ3MuYnI=
Disclaimer: All claims expressed in this article are solely those of the authors and do not necessarily represent those of their affiliated organizations, or those of the publisher, the editors and the reviewers. Any product that may be evaluated in this article or claim that may be made by its manufacturer is not guaranteed or endorsed by the publisher.
Research integrity at Frontiers
Learn more about the work of our research integrity team to safeguard the quality of each article we publish.