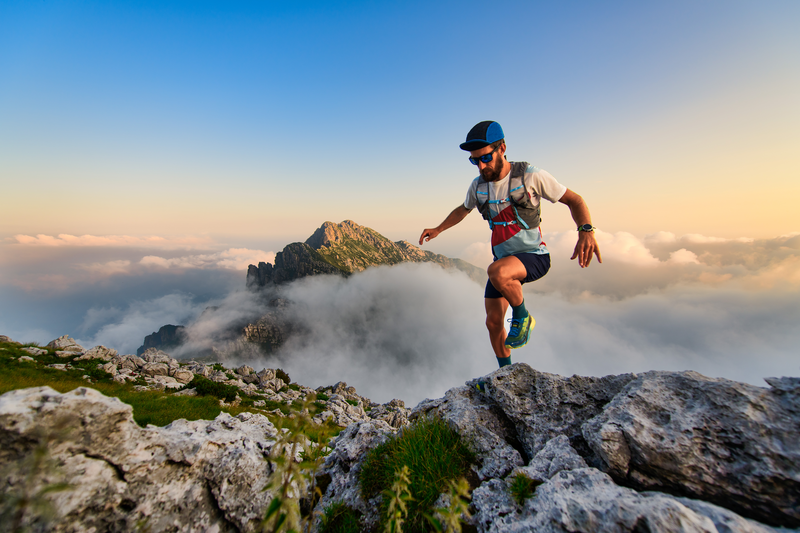
94% of researchers rate our articles as excellent or good
Learn more about the work of our research integrity team to safeguard the quality of each article we publish.
Find out more
EDITORIAL article
Front. Vet. Sci. , 20 March 2023
Sec. Livestock Genomics
Volume 10 - 2023 | https://doi.org/10.3389/fvets.2023.1167079
This article is part of the Research Topic Genomic and Epigenomic Applications in Animal and Veterinary Sciences View all 12 articles
Editorial on the Research Topic
Genomic and epigenomic applications in animal and veterinary sciences
In the last two decades, all biological and health disciplines, from virology to ecology, have been revolutionized by genomics with the advances in sequencing technologies and bioinformatics software development. Animal and veterinary sciences have not been an exception; the impact of genomics, transcriptomics, proteomics, and other “omic” branches on animal selection, breeding, nutrition, and health is evident.
Among the plethora of genomic and epigenomic papers published each year, the proportion of publications involving non-model, domestic, or wild animals is still low. However, the availability of annotated genomes for most domestic and economically relevant species (Table 1 collects genome assembly information for some domestic animals) has made it possible for animal science and veterinary researchers to turn to cutting-edge genomic strategies to better identify genetic markers associated with productive traits, characterize pathologic agents, associate transcriptomic information with physiological responses, and evaluate the effect of epigenetic modifications on gene expression. Even multi-omic data integration approaches are being applied to animal research (11).
Table 1. Assembly information for important domestic species genomes, organized chronologically according to the first assembly release.
This Research Topic, on the application of genomic and epigenomic tools in animal and veterinary sciences, aimed at showcasing research that included genomic and epigenomic tools to solve questions relating to animal and veterinary science. The result is a set of nine original research papers and two review articles that explore a diverse array of applications of genomics to animal health, reproduction, production, and response to stress and pathogens in cattle, yak, Chinese forest musk deer, pig, rat, chicken, and carp.
Two papers focused on measuring microRNA expression in domestic animals: Wang H. et al. evaluated the role of miR-22 in Suhuai pig skeletal muscle and identified a SNP upstream of the miR-22 precursor sequence that correlates with pork color. The target of this microRNA is ELOVL6, an elongase that catalyzes de novo synthesis of fatty acids, previously linked with the regulation of muscle fiber type conversion, and that appears to be over-expressed in white muscle. Their findings provide a basis for future research on the molecular markers of pork color. The paper by Veshkini et al. studied circulating miRNAs in dairy cows to assess their roles in the metabolic adaptations the cow goes through before and after calving. According to their results, calving significantly affects miRNA expression, disturbing signaling pathways related to energy, metabolism, and immunity.
Three additional papers used genomic tools for association and gene expression studies. Khan et al. genotyped TRAPPC9 and CD4 polymorphisms in Chinese Holstein cows and studied their association with milk production and mastitis resistance. They associated the identified SNPs with milk production, protein content, somatic cell count, somatic cell score, and the expression of interleukin 6 (IL-6) and interferon-gamma (IFN-γ), suggesting that polymorphisms in these genes could be useful markers for milk production and mastitis resistance in dairy cattle. The pioneering work by Chen et al. employed Nanopore long-read RNA-Seq to evaluate the genome-wide allelic differential expression in the lung and liver of domesticated cattle-yak hybrids (known as “yattle”). The authors found that genes related to hypoxia adaptation and immune response were predominantly expressed from the yak alleles. In contrast, lipid metabolism and endocrine secretion genes were expressed from the cattle alleles. This analysis of the differential contribution of parental alleles in hybrid animals could enhance our understanding of the genetic basis of hybrid vigor during crossbreeding. Thirdly, a weighted gene co-expression network analysis (WGCNA) was applied by Wang X. et al. to identify key genes involved in the regulation of subcutaneous adipose tissue. They identified 15 gene co-expression modules and selected 3, according to the correlation between modules and phenotype, from which eight hub lipid metabolism genes were identified. Their expression levels were measured in the heart, liver, spleen, lung, kidney, muscle, and adipose tissue. The results provide a theoretical basis for studying beef quality by identifying hub genes that regulate lipid metabolism.
A couple of papers in this Research Topic studied gene expression in brain tissue. Baker et al. explore the pattern of DNA methylation and gene expression in amygdala tissue from Brahman cows exposed to prenatal stress. Although they only found differential methylation in a few individual CpG sites and differentially expressed in two genes, this is one of the first studies on the impact of prenatal stress on cattle brain DNA methylation and transcriptomic profiles. The second paper by Gao et al. measured the protective effects of taurine in rats against the negative effect of formaldehyde, benzene, toluene, and xylene, which are common indoor volatile organic compounds (VOC) and associated them with intellectual and cognitive impairment in children. Taurine protected rats against VOC-induced cognitive-behavioral damage and restored their learning and memory. Therefore, suggesting that it could be a potential treatment for a cognitive behavioral disorder.
Given the association between bacterial infection and pneumonia and the threat, it poses to the endangered Chinese forest musk deer (Moschus berezovskii), Tang et al. relied on ITRAQ-based quantitative proteomics to understand pneumonia pathogenesis in this species. Since the forest musk deer genome is poorly annotated, the researchers used the bovine genome to identify the proteins and found a clear dysregulation of proteins involved in bacterial infection and immunity, in deer suffering from pneumonia. These results shed light on the molecular mechanisms, and pathways underlying pneumonia pathogenesis.
The paper by Xie et al. is one of the first attempts at optimizing common carp (Cyprinus carpio) germ cell culture, which could open new opportunities for the application of surrogate production, a biotechnological strategy that could be valuable in common carp breeding, the restoration and development of lines, and the conservation of genetic resources.
Finally, this Research Topic included two review papers: first, Gul et al. reviewed the recent genetic basis of poultry resistance against microbial pathogens and genomic modifications that increase resistance against pathogens in chickens. Understanding disease resistance genetics would enable the identification of resistance markers and the development of disease resistance breeds, which could reduce the dependency on vaccination and prophylactic antibiotics in the poultry industry. The last paper by Guo et al.'s team reviews the methods and advances in cell immortalization in livestock and poultry. Immortalized cell lines provide a reliable tool for biological research and stable infinite cell lines should guarantee proliferation, while maintaining normal cell function.
NR-O initiated the Research Topic and drafted the manuscript. AB, JF, and NR-O co-edited the Research Topic and participated in the editorial process. All authors revised and approved the manuscript.
JF acknowledges the support of USDA-ARS Biophotonics (grant # 6066-31000-015-00D).
The authors declare that the research was conducted in the absence of any commercial or financial relationships that could be construed as a potential conflict of interest.
All claims expressed in this article are solely those of the authors and do not necessarily represent those of their affiliated organizations, or those of the publisher, the editors and the reviewers. Any product that may be evaluated in this article, or claim that may be made by its manufacturer, is not guaranteed or endorsed by the publisher.
1. Kirkness EF, Bafna V, Halpern AL, Levy S, Remington K, Rusch DB, et al. The dog genome: Survey sequencing and comparative analysis. Science. (2003) 301:1898–903. doi: 10.1126/science.1086432
2. International Chicken Genome Sequencing Consortium. Sequence and comparative analysis of the chicken genome provide unique perspectives on vertebrate evolution. Nature. (2004) 432:695–716. doi: 10.1038/nature03154
3. Pontius JU, Mullikin JC, Smith DR, Team AS, Lindblad-Toh K, Gnerre S, et al. Initial sequence and comparative analysis of the cat genome. Genome Res. (2007) 17:1675–89. doi: 10.1101/gr.6380007
4. Wade CM, Giulotto E, Sigurdsson S, Zoli M, Gnerre S, Imsland F, et al. Genome sequence, comparative analysis, and population genetics of the domestic horse. Science. (2009) 326:865–7. doi: 10.1126/science.1178158
5. Bovine Genome Sequencing and Analysis Consortium, Elsik CG, Tellam RL, Worley KC, Gibbs RA, Muzny DM, Weinstock GM. The genome sequence of taurine cattle: A window to ruminant biology and evolution. Science. (2009) 324:522–8. doi: 10.1126/science.1169588
6. Archibald AL, Bolund L, Churcher C, Fredholm M, Groenen MA, Harlizius B, et al. Pig genome sequence—Analysis and publication strategy. BMC Genomics. (2010) 11:438. doi: 10.1186/1471-2164-11-438
7. Consortium ISG, Archibald AL, Cockett NE, Dalrymple BP, Faraut T, Kijas JW, et al. The sheep genome reference sequence: A work in progress. Anim Genet. (2010) 41:449–53. doi: 10.1111/j.1365-2052.2010.02100.x
8. Dalloul RA, Long JA, Zimin AV, Aslam L, Beal K, Blomber LA, et al. Multi-platform next-generation sequencing of the domestic turkey (Meleagris gallopavo): Genome assembly and analysis. PLoS Biol. (2010) 8:e1000475. doi: 10.1371/journal.pbio.1000475
9. Brawand D, Wagner CE, Li YI, Malinsky M, Keller I, Fan S, et al. The genomic substrate for adaptive radiation in African cichlid fish. Nature. (2014) 513:375–81. doi: 10.1038/nature13726
10. Huang Y, Li Y, Burt DW, Chen H, Zhang Y, Qian W, et al. The duck genome and transcriptome provide insight into an avian influenza virus reservoir species. Nat Genet. (2013) 45:776–83. doi: 10.1038/ng.2657
Keywords: animal genomics, animal epigenomics, domestic animals and wildlife, veterinary research, animal sciences
Citation: Baloch AR, Feugang JM and Rodríguez-Osorio N (2023) Editorial: Genomic and epigenomic applications in animal and veterinary sciences. Front. Vet. Sci. 10:1167079. doi: 10.3389/fvets.2023.1167079
Received: 15 February 2023; Accepted: 01 March 2023;
Published: 20 March 2023.
Edited and reviewed by: Martino Cassandro, University of Padua, Italy
Copyright © 2023 Baloch, Feugang and Rodríguez-Osorio. This is an open-access article distributed under the terms of the Creative Commons Attribution License (CC BY). The use, distribution or reproduction in other forums is permitted, provided the original author(s) and the copyright owner(s) are credited and that the original publication in this journal is cited, in accordance with accepted academic practice. No use, distribution or reproduction is permitted which does not comply with these terms.
*Correspondence: Nélida Rodríguez-Osorio, bmVsaWRhLnJvZHJpZ3VlekB1bm9ydGUuZWR1LnV5
Disclaimer: All claims expressed in this article are solely those of the authors and do not necessarily represent those of their affiliated organizations, or those of the publisher, the editors and the reviewers. Any product that may be evaluated in this article or claim that may be made by its manufacturer is not guaranteed or endorsed by the publisher.
Research integrity at Frontiers
Learn more about the work of our research integrity team to safeguard the quality of each article we publish.