- 1College of Veterinary Medicine and Zoonoses Research Institute, Kyungpook National University, Daegu, Republic of Korea
- 2Division of Microbiology, National Center for Toxicological Research, U.S. Food and Drug Administration, Jefferson, AR, United States
- 3Bacterial Disease Division, Animal and Plant Quarantine Agency, Gimcheon-si, Gyeongsangbuk-do, Republic of Korea
Fluoroquinolones are classified as “critically important antimicrobials for human medicine”; however, their extensive use in livestock poses a significant health risk to humans as it leads to the rapid spread of antimicrobial resistance. This study confirmed that 40.0%−71.4% of the farms in three of the five integrated broiler operations were administered ciprofloxacin (CIP). Moreover, preventive purposes (60.9%), veterinarian prescriptions (82.6%), drinking water route (100%), and 1 to 3 days (82.6%) of age were significantly highest (P < 0.05). 194 high-level ciprofloxacin-resistant (HLCR) Enterococcus faecalis (E. faecalis) were found in 65 of 74 farms, and of which, the prevalence of qnrA (63.9%), tetM (60.3%), ermB (64.9%), blaz (38.7%), and catA (34.0%) was significantly highest (P < 0.05). 154 (79.4%) isolates showed MDR, and the distribution of MDR was significantly differences among the operations (P < 0.05). All HLCR E. faecalis possessed double mutations in gyrA and parC, and S83I/S80I (90.7%) mutations were most commonly identified. Interestingly, the distribution of isolates with MICs ≥ 512 for both CIP and moxifloxacin was significantly higher in CIP–administered farms (56.5%) than in non-CIP–administered farms (41.4%) (P < 0.05). Also, the prevalence of strong or moderate biofilm formers in HLCR E. faecalis was significantly higher than that of weak and no biofilm formers (P < 0.05). HLCR E. faecalis were heavily distributed in the broiler farms in Korea; therefore, it is necessary to minimize the prevalence of resistant bacteria via structural management regulations such as cleaning and disinfection of farm environments.
1. Introduction
Antimicrobials play a critical role in the prevention and treatment of disease in humans and animals. However, the extensive use of antimicrobials poses a significant health risk to humans, leading to the rapid spread of antimicrobial resistance (1). Fluoroquinolones, including ciprofloxacin (CIP), are classified as “critically important antimicrobials for human medicine” by the World Health Organization (2), but they have been widely used for treatment and prevention of livestock because of their strong antibacterial activity against gram-negative bacteria, gram-positive bacteria, and mycoplasma (3). Fluoroquinolones inhibit DNA replication by inhibiting bacterial DNA topoisomerase (parC and parE subunits) and DNA gyrase (gyrA and gyrB subunits), which act as essential enzymes in bacteria (4). Therefore, single and double point mutations in the quinolone resistance determining region (QRDR) of these subunits reduce susceptibility and lead to high resistance to fluoroquinolones in the future (5, 6).
Because the risk promoting fluoroquinolones-resistant bacteria could be harmful to humans, US FDA has finally decided to withdraw approval of enrofloxacin for use in poultry in 2005 (7, 8), and in Ireland, fluoroquinolones have been prescribed in < 1% of antimicrobials for livestock since 2009 (9). Moreover, in Australia, fluoroquinolones have never been approved for food production animals due to strict regulations on antimicrobial use. Although the manufacture and import of enrofloxacin in the poultry industry in Korea have been only banned since October 2021 (10), the rate of resistance to CIP, a major metabolic product of enrofloxacin in vivo, increased by ~4.5% in 2021 compared with that in 2020 (10).
In Korea, 96.4% of the broilers are produced in integrated operations (11). However, antimicrobial resistance patterns may differ by operation depending on biosecurity and hygiene practices, vaccine programs, and antimicrobial applications (12). Therefore, this study was conducted to analyze the current status of fluoroquinolones-based prescriptions and characterize high-level CIP-resistant (HLCR) E. faecalis from five major integrated broiler operations which produce 50.2% of chicken meat in Korea (11).
2. Materials and methods
2.1. Data source
A situational analysis of CIP use was performed using a questionnaire on CIP administration patterns during the broiler grow-out period. The questionnaire areas included purpose, proponent, route, age, and duration of CIP administration.
2.2. Sample collection
Fecal and dust samples from 74 farms of five integrated broiler operations were collected in 2021 according to the standard set of Processing and Ingredients Specification of Livestock Products by the Ministry of Food and Drug Safety (2018) (13). Briefly, approximately 10 g of the dust was swabbed using a sterile gauze moistened with sterile double-strength skim milk, and feces were sampled to disposable boots in five different sites from each flock. All samples were placed in whirl pak bags (Whirl-Pak, Nasco, Madison, WI) and transported to the laboratory at 4°C.
2.3. Bacterial identification
The isolation and identification of E. faecalis were performed following the standard microbiological protocols published by the Ministry of Food and Drug Safety (2018). In brief, each dust and fecal samples were cultured in 90 mL of buffered peptone water (BPW; BD Biosciences, San Jose, CA, USA) for 18–24 h at 37°C. The pre-enriched BPW was transferred to Enterococcosel broth (BD Biosciences) at a ratio of 1:10 and streaked onto Enterococcosel agar (BD Biosciences) after incubation at 37 °C for 18–24 h. At least three presumptive Enterococcus spp. colonies were selected from each sample, and E. faecalis was confirmed using polymerase chain reaction (PCR) with a specific primer for ddl, as described previously (14). If two or more isolates from the same farm showed the same antimicrobial susceptibility pattern, only one isolate was selected. A total of 351 E. faecalis isolates were analyzed in this study.
2.4. Antimicrobial susceptibility testing
The minimum inhibitory concentrations (MICs) of CIP and moxifloxacin (MOX) were determined by standard agar dilution methods with Mueller–Hinton agar (BD Biosciences) according to the guidelines of the Clinical and Laboratory Standards Institute (15). The breakpoint for HLCR was set at ≥64 μg/mL against CIP (16). Moreover, based on the guidelines of the Clinical and Laboratory Standards Institute (15), antimicrobial resistance of all HLCR E. faecalis isolates was determined using the disc diffusion method with the following discs (BD Biosciences): ampicillin (10 μg), chloramphenicol (30 μg), ciprofloxacin (CIP, 5 μg), doxycycline (30 μg), erythromycin (15 μg), penicillin (10 units), rifampin (5 μg), tetracycline (30 μg), and vancomycin (30 μg). Multidrug resistance (MDR) was defined as the acquired non-susceptibility to at least one agent in three or more antimicrobial classes (17).
2.5. Identification of mutations in QRDRs
PCR was performed to amplify gyrA and parC in HLCR E. faecalis isolates using primers and conditions described previously (18). The amplified DNA was purified by a PCR purification kit (Bioneer Inc., Seoul, Republic of Korea) and sequenced using an automatic sequencer (Cosmogenetech, Seoul, Republic of Korea). The sequences were confirmed with those in the GenBank nucleotide database using the Basic Local Alignment Search Tool (BLAST) program available at the National Center for Biotechnology Information website (http://www.ncbi.nlm.nih.gov/BLAST).
2.6. Detection of antimicrobial resistance genes
DNA extraction was performed using the boiling method as described previously (19). The presence of resistance genes associated with fluoroquinolones (qnrA, qnrB, qnrD, and qnrS), β-lactams (blaZ, and mecA), phenicols (cfr, catA, and catB), glycopeptides (vanA), macrolides (ermA, ermB, and ermC), tetracyclines (tetL, tetM, and tetO), and rifamycin (rpoB) was detected using PCR as described previously (20–29).
2.7. Biofilm formation assay
All HLCR E. faecalis were determined for biofilm formation by using the standard microtiter plate test, as described previously (30). All HLCR isolates were cultured overnight on Brain–Heart Infusion agar (BD Biosciences, Sparks, MD, USA) at 37°C. Then, 500 μL of bacterial suspension adjusted for turbidity with 0.5 McFarland standard was inoculated into 5 mL of tryptic soy broth (BD Biosciences) with 0.25% (wt/vol) glucose, and 200 μL of the mixture was transferred into three wells of a 96-well microtiter plate. After overnight incubation at 37°C, the 96-well microtiter plates were washed with phosphate–buffered saline (BD Biosciences) to remove the planktonic bacteria. After air-drying, the attached bacteria were fixed with 200 μL of methanol for 15 min, and the bacterial biomass was quantified by measuring the absorbance at 595 nm (OD595) after staining with crystal violet (1% wt/vol) for 10 min and destaining with 50% ethanol-50% glacial acetic acid solution. The ability to form biofilms was classified as negative (OD595 < 0.12), weak (0.12 ≤ OD595 < 0.24), moderate (0.24 ≤ OD595 ≤ 0.48), and strong (OD595 > 0.48). To verify the analysis, E. faecalis OG1RF and E. faecalis ATCC 29212 were used as reference strains for weak and no biofilm formers, respectively. A sterile medium was used as the contamination control as described previously (31, 32).
2.8. Statistical analysis
Statistical analysis was performed using Pearson's chi-square tests and Fisher's exact tests with Bonferroni correction in Statistical Package for the Social Science version 25 (IBM, Korea). Significant differences were considered at P < 0.05.
3. Results
3.1. Analysis of the prescription status of CIP
The situational analysis of the prescription status of CIP in 74 farms of five integrated broiler operations is shown in Table 1. Among the five integrated broiler operations, the prevalence of CIP–administered farms was significantly highest in operation D (71.4%), followed by operations E (50.0%) and B (40.0%) (P < 0.05). No farms administered CIP in operations A and C. In the analysis for the purpose of CIP administration, operation D was significantly higher for the prevention (80.0%) than treatment (20.0%), whereas operation E were significantly higher for the treatment (60.0 %) than prevention (40.0 %) (P < 0.05). There were no significant differences between the rates of prevention and treatment in operation B. CIP administration via veterinarians (82.6%) and drinking water route (100%) was significantly highest (P < 0.05). Moreover, CIP was administered significantly highest at 1 to 3 days of age (82.6%), and significantly higher on duration of CIP administration in 2 days (43.5%) to 3 days (39.1%) (P < 0.05).
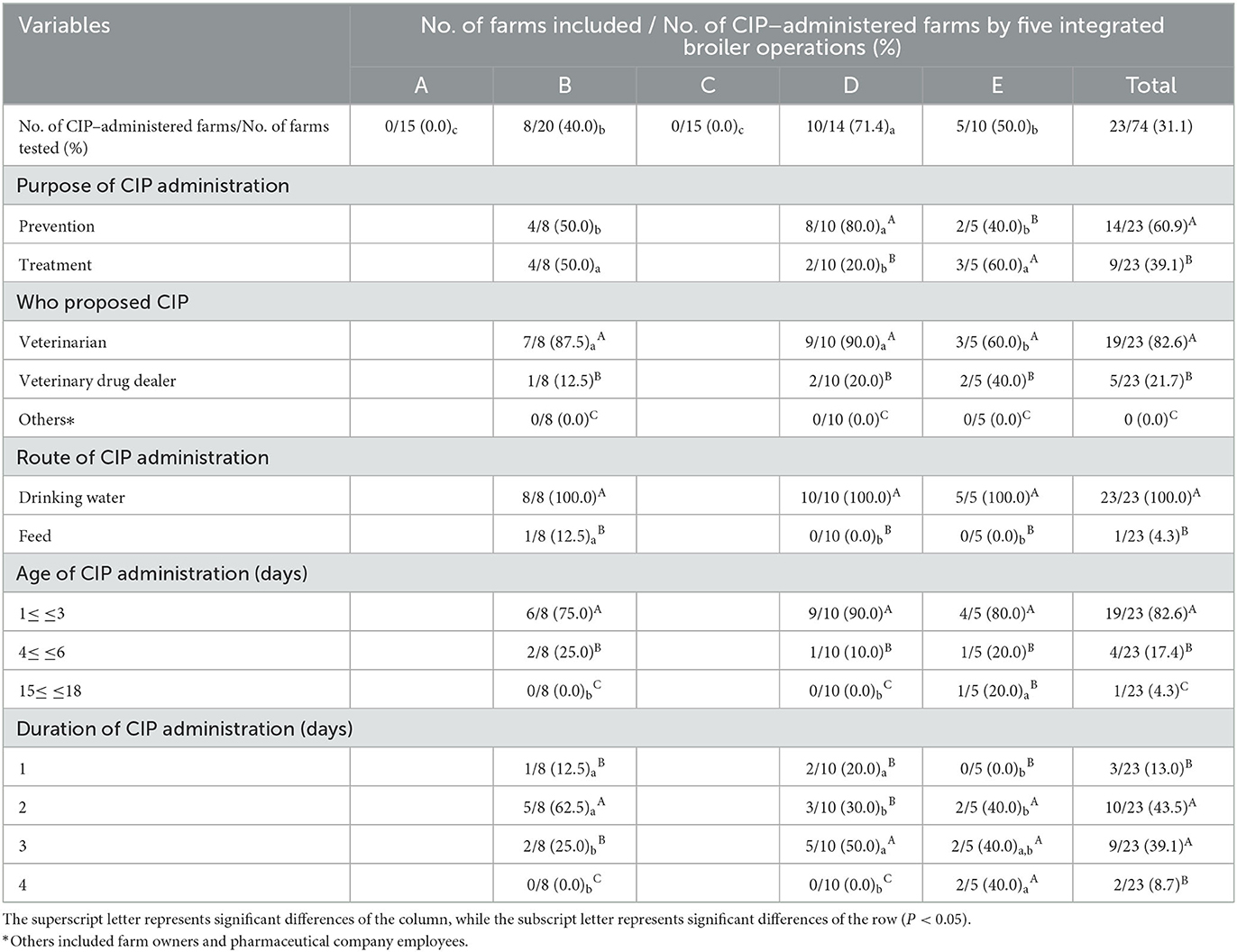
Table 1. Analysis of the prescription status of ciprofloxacin (CIP) by five integrated broiler operations.
3.2. Distribution of HLCR E. faecalis and antimicrobial resistance genes
The distribution of HLCR E. faecalis and antimicrobial resistance genes in 74 farms of five integrated broiler operations are shown in Table 2. HLCR E. faecalis were found in 65 (87.8%) of 74 farms. In particular, HLCR E. faecalis were significantly higher at 95.0% and 100% in farms of operations B and E, respectively (P < 0.05). In addition, HLCR E. faecalis was found in a total of 194 (55.3%) of 351 E. faecalis isolates, which was significantly highest in farms of operation B (P < 0.05). In the distribution of fluoroquinolones resistance genes of HLCR E. faecalis, the prevalence of qnrA (63.9%) and qnrB (57.7%) genes were significantly higher than that of qnrD (20.1%) and qnrS (18.0%) (P < 0.05). In particular, qnrA showed a significantly high prevalence in the farms of four operations A (66.7%), B (60.7%), D (78.9%), and E (73.3%) except for operation C (45.0%) (P < 0.05), and qnrB was found in 50.0%−66.7% of farms in all five operations without significant differences.
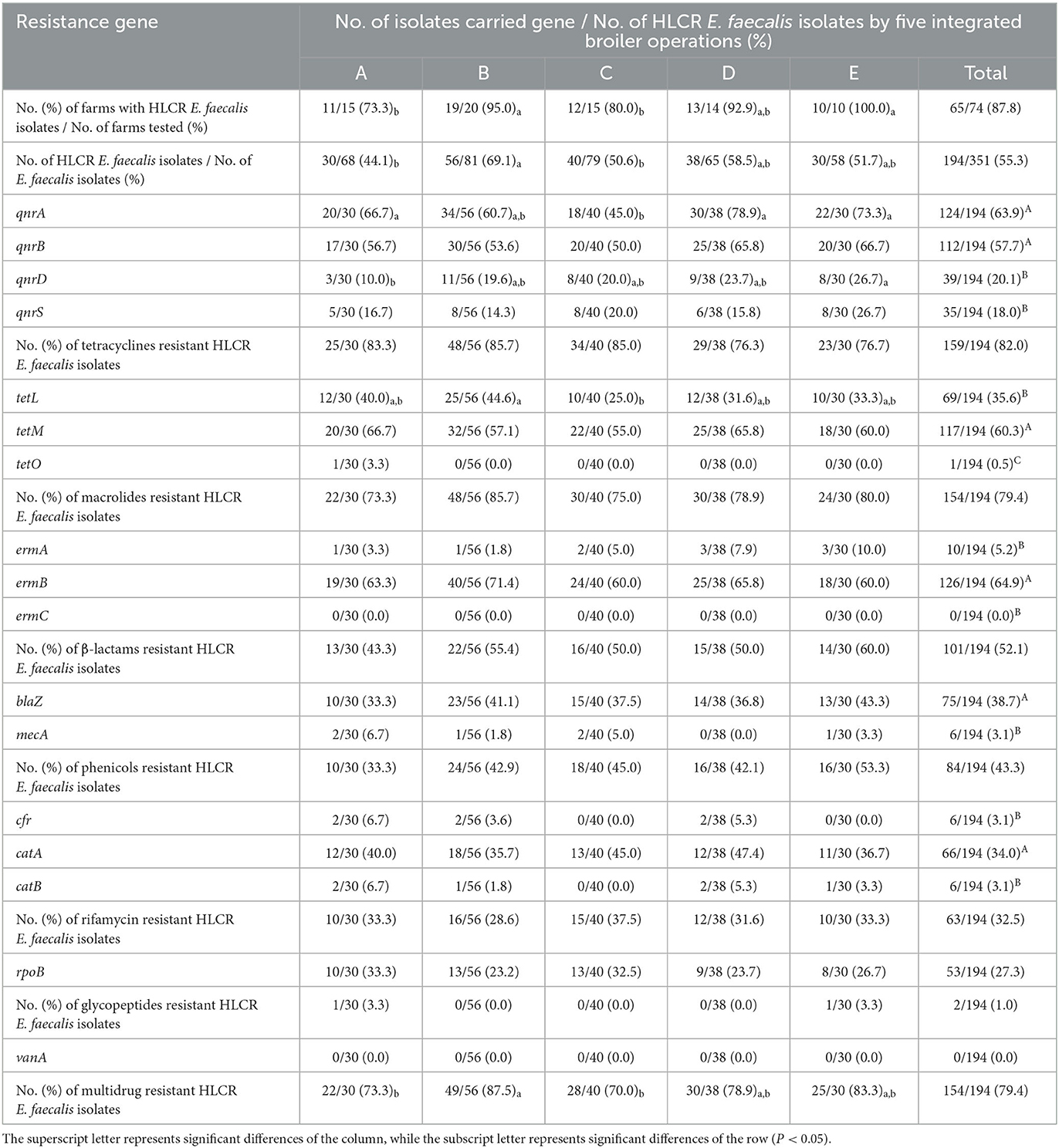
Table 2. Distribution of high-level ciprofloxacin-resistant (HLCR) E. faecalis and antimicrobial resistance genes by five integrated broiler operations.
All HLCR E. faecalis also showed the highest resistance to tetracyclines (82.0%), followed by macrolides (79.4%), β-lactams (52.1%), phenicols (43.3%), rifamycin (32.5%), and glycopeptides (1.0%) without no significant differences among the five integrated broiler operations. Moreover, the prevalence of tetM (60.3%), ermB (64.9%), blaz (38.7%), and catA (34.0%) was significantly highest among tetracyclines, marcrolides, β-lactams and phenicols resistance-related genes, respectively (P < 0.05). MDR was found in 154 (79.4%) of 194 HLCR E. faecalis isolates; however, the distribution of MDR was significantly highest in operation B (87.5%) and lowest in operations A (73.3%) and C (70.0%) (P < 0.05).
3.3. Detection of mutations in the QRDRs of HLCR E. faecalis
The presence of mutations in gyrA and parC and distribution of MICs in 194 HLCR E. faecalis isolates are shown in Table 3. All HLCR E. faecalis isolates possessed double mutations in gyrA and parC. Moreover, S83I/S80I (90.7%) mutations were most commonly identified. The distribution of isolates with MICs ≥ 512 for both CIP and MOX was significantly higher in CIP–administered farms (56.5%) than in non-CIP–administered farms (41.4%) (P < 0.05).
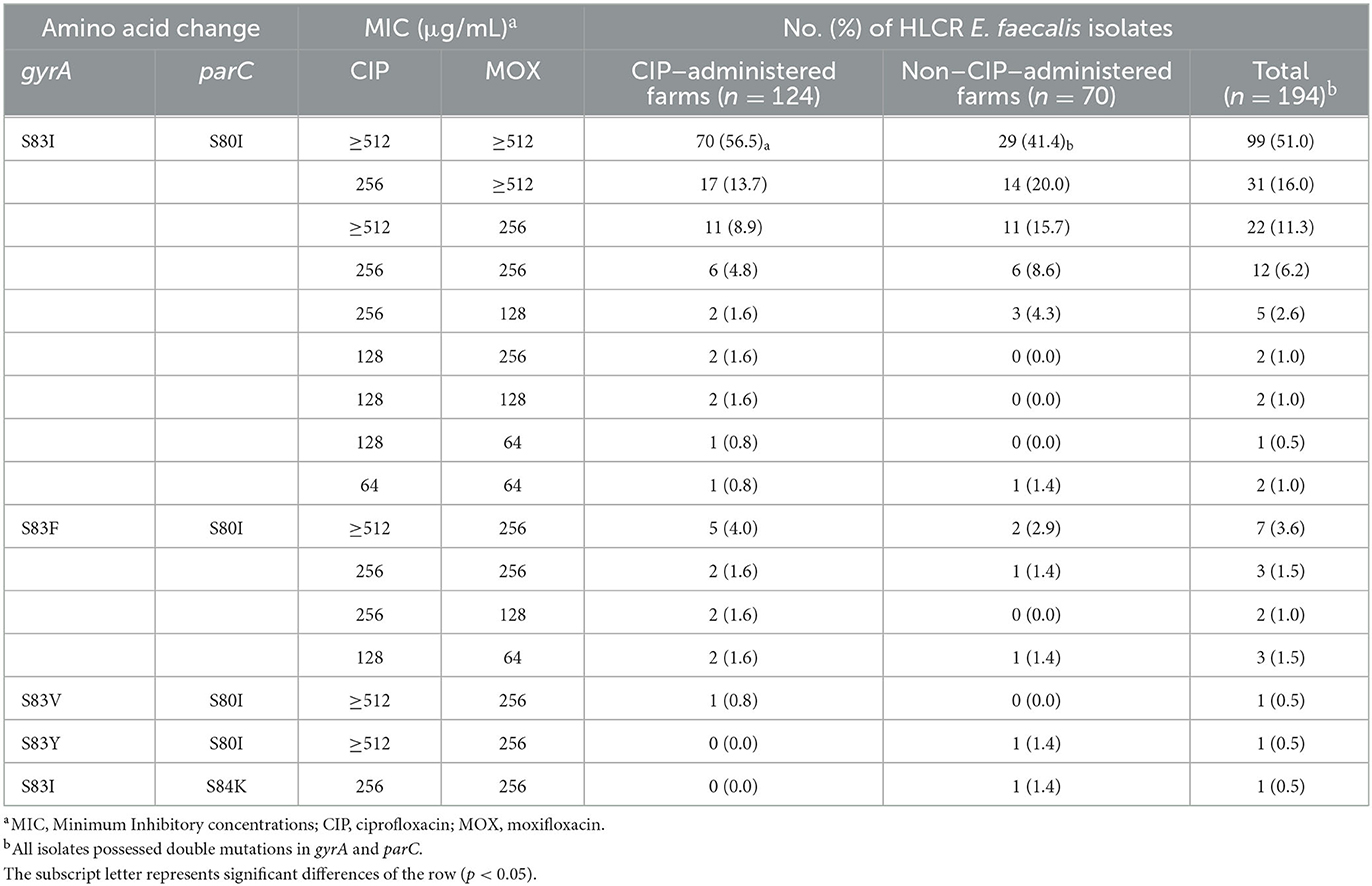
Table 3. Characteristics of amino acid changes in gyrA and parC and distribution of MICs in 194 high-level ciprofloxacin-resistant (HLCR) E. faecalis from 74 broiler farms of five integrated broiler operations.
3.4. Distribution of biofilm-forming ability of HLCR E. faecalis
The distribution of biofilm-forming ability of 194 HLCR E. faecalis isolates is shown in Table 4. Although there were no significant differences between CIP–administered farms and non-CIP–administered farms, the prevalence of strong or moderate biofilm formers (60.5% and 60.0%, respectively) in all HLCR E. faecalis showed significantly higher than that of weak and no biofilm formers (P < 0.05).
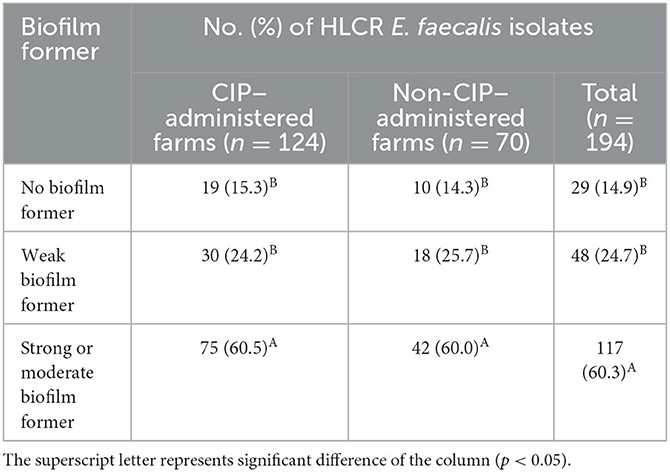
Table 4. Distribution of biofilm-forming ability in 194 high-level ciprofloxacin-resistant (HLCR) E. faecalis from 74 broiler farms of five integrated broiler operations.
4. Discussion
Enterococci are harmless to healthy individuals, but are considered opportunistic pathogens associated with hospital-acquired infections in humans and animals (33). In particular, the ability to cause infection is critical to avoid the action of most commonly used antimicrobials. Recently, antimicrobial-resistant enterococci isolated from poultry farms have become a problem in many countries, including Korea (34–37), because antimicrobial-resistant enterococci can easily spread within pathogenic strains or between pathogenic and non-pathogenic strains (38). Moreover, resistance to fluoroquinolones, which are critically important in human medicine, has been continuously reported worldwide (39).
Antimicrobial resistance in enterococci is described as intrinsic resistance found within a species genome as well as acquired resistance by obtaining new genetic material or acquiring sporadic mutations in intrinsic genes (40, 41). In particular, the prevalence of acquired fluoroquinolones-resistant enterococci has increased with the increased use of fluoroquinolones (36, 42). In this study, CIP was administered in 23 (31.1%) of 74 broiler farms of five integrated broiler operations. Two integrated broiler operations did not use CIP, but the other three used CIP for prevention and treatment, mostly under the proposal of veterinarian. Moreover, the prevalence of 1 to 3 days of age (82.6%) was the highest among the age of CIP administration in the three integrated broiler operations. It is related to the high incidence of colibacillosis in young chicks causing mortality in Korea (43). CIP was also mainly administered via drinking water for 2 or 3 days because it is easier than injection administration, but it has a disadvantage that the time when the drug appears varies depending on individual even within the same flock (44).
In this study, HLCR E. faecalis were isolated in 65 (87.8%) of 74 farms. Farms of operations A and C were not administered CIP at all, but the distribution of HLCR E. faecalis isolates in these two farms showed a high prevalence of 73.3% and 80.0%, respectively. Burbarelli et al. (45) reported that antimicrobial–resistant bacteria could survive and spread throughout the breeding period regardless of CIP administration unless cleaning and disinfection of the broiler environment is followed for farm hygiene. In particular, antimicrobial–resistant bacteria in feces, which are generally considered a natural reservoir, have the potential to remain in the environment or bind to dust and spread throughout the farm (46–48).
Moreover, in this study, the antimicrobial resistance genes qnrA and qnrB, which are related to fluoroquinolones resistance, were present in 63.9% and 57.7%, respectively, of the distribution of HLCR E. faecalis in the five integrated broiler operations. In particular, the prevalence of qnrA in HLCR E. faecalis isolates in operation A was 66.7%, but the prevalence of qnrA in operation C was 45.0%, which was significantly lowest in these operations, even though CIP was not administered in operation A and C. It is thought that environmental cleaning and the use of CIP, and it is necessary to continuously investigate the distribution of these genes in farms that do not use CIP.
The plasmids carrying mobile resistance genes are easily transferable from one bacteria to another by conjugation, sharing genetic information (49, 50). Moreover, the externalization of antimicrobials via efflux pumps responsible for the outflow of the antimicrobials can simultaneously confer resistance to several antimicrobials, and their overexpression can lead to high levels of resistance and MDR (51–53). In this study, HLCR E. faecalis isolated from five broiler integrated operations were mostly resistant to tetracyclines (82.0%), macrolides (79.4%), β-lactams (52.1%), phenicols (43.3%), and rifamycin (32.5%), without significant differences among the operations. In addition, the prevalence of tetM, ermB, blaZ, catA, and rpoB, which are related to resistance to tetracyclines, macrolides, β-lactams, and phenicols, was 60.3%, 64.9%, 38.7%, and 34.0%, respectively, without significant differences among the operations. However, the distribution of MDR was significantly highest in operation B (87.5%) and lowest in operations A (73.3%) and C (70.0%). These results are consistent with the fact that the distribution of HLCR E. faecalis isolates was significantly highest in farms of operation B and lowest in farms of operations A and C. Moreover, this is consistent with the reports that the efflux pump is overexpressed as the number of HLCR isolates increases (54, 55), which is thought to be related to the increased distribution of MDR.
The mechanism of resistance to fluoroquinolones includes the formation of mutations in the two bacterial essential enzymes, DNA gyrase and DNA topoisomerase (56). DNA gyrase comprises gyrA and gyrB subunits, and DNA topoisomerase comprises parC and parE subunits. In particular, HLCR is associated with double mutations of gyrA and parC (57). Kim et al. (36) reported that all tested HLCR enterococci isolates showed double mutations in gyrA and parC, and the double mutations were the most frequently identified in S83I/S80I at 94.1%. Similarly, all HLCR E. faecalis isolates in this study possessed double mutations in gyrA and parC. In particular, 90.7% of HLCR isolates showed S83I/S80I mutations. Moreover, 99 (56.3%) of 176 HLCR E. faecalis with S83I/S80I mutations showed MICs of ≥512 for CIP and MOX. However, the distribution of these HLCR E. faecalis with MIC of ≥512 for both CIP and MOX in S83I/S80I mutations was found to be significantly higher in CIP–administered farms (56.5%) than in non–CIP–administered farms (41.4%). These results indicate that higher CIP administration leads to higher MIC values, just as fluoroquinolones-resistant enterococci increase with increasing fluoroquinolones use.
Biofilms of bacteria protect them from host immunological responses, phagocytosis, and antimicrobials (58). The ability to form biofilms is related to the ability of bacteria to adhere to a surface and form a layer, and the density of the layer indicates the strength of the biofilms (59). In addition, the thicker the layer, the less effective the antimicrobials against bacteria, suggesting a correlation between antimicrobial resistance and biofilm-forming ability (60). Al-Shammary (61) reported that 93.8% of MDR E. faecalis isolates from raw milk samples were biofilm formers. Sadek and Koriem (62) also reported that all MDR E. faecalis isolates from dairy products were biofilm formers. In this study, 165 (85.0%) of 194 HLCR E. faecalis isolates showed strong or moderate biofilm-forming ability. However, there were no significant differences between the isolates from CIP–administered farms and non–CIP–administered farms. Therefore, these results suggest that if already classified as HLCR, isolates can have strong and moderate biofilm-forming ability regardless of CIP administration, and the increased biofilm density also allows infections to persist chronically despite antimicrobial treatment strategies for broiler diseases. In this study, it was confirmed that HLCR E. faecalis were heavily distributed in the broiler operations in Korea, therefore, it is necessary to minimize the prevalence of resistant bacteria via structural management regulation, such as cleaning and disinfection of farm environments, and reduce the use of antimicrobials via prescriptions based on scientific disease evidence rather than disease prevention.
Data availability statement
The original contributions presented in the study are included in the article/supplementary material, further inquiries can be directed to the corresponding author.
Author contributions
YuL, SK-L, and YoL conceived of the presented idea. YuL, H-RJ, and SY developed the theory and performed the experiments. YuL and H-RJ verified the analysis. YuL wrote the manuscript with supervision from S-KL and YoL. All authors contributed to the article and approved the submitted version.
Funding
This work was supported by the Animal and Plant Quarantine Agency, Ministry of Agriculture, Food and Rural affairs, Republic of Korea (grant number Z-1543061-2021-23-02).
Conflict of interest
The authors declare that the research was conducted in the absence of any commercial or financial relationships that could be construed as a potential conflict of interest.
Publisher's note
All claims expressed in this article are solely those of the authors and do not necessarily represent those of their affiliated organizations, or those of the publisher, the editors and the reviewers. Any product that may be evaluated in this article, or claim that may be made by its manufacturer, is not guaranteed or endorsed by the publisher.
References
1. Hidron AI, Edwards JR, Patel J, Horan TC, Sievert DM, Pollock DA, et al. Antimicrobial-resistant pathogens associated with healthcare-associated infections: annual summary of data reported to the national healthcare safety network at the centers for disease control and prevention, 2006–2007. Infect Control Hosp Epidemiol. (2008) 29:996–1011. doi: 10.1086/591861
2. World Health Organization. WHO list of Critically Important Antimicrobials for Human Medicine (WHO CIA list). (2019). Available online at: https://www.who.int/publications/i/%0Aitem/9789241515528 (accessed May 25, 2022).
3. Pham TDM, Ziora ZM, Blaskovich MAT. Quinolone antibiotics. Medchemcomm. (2019) 10:1719–39. doi: 10.1039/c9md00120d
4. Khan T, Sankhe K, Suvarna V, Sherje A, Patel K, Dravyakar B, et al. gyrase inhibitors: Progress and synthesis of potent compounds as antibacterial agents. Biomed Pharmacother. (2018) 103:923–38. doi: 10.1016/j.biopha.2018.04.021
5. Urushibara N, Suzaki K, Kawaguchiya M, Aung MS, Shinagawa M, Takahashi S, et al. Contribution of type II topoisomerase mutations to fluoroquinolone resistance in enterococcus faecium from Japanese clinical setting. Microb Drug Resist. (2018) 24:1–7. doi: 10.1089/mdr.2016.0328
6. Esfahani S, Ahmadrajabi R, Mollaei H, Saffari F. Co-incidence of type II topoisomerase mutations and efflux expression in high fluoroquinolone resistant Enterococcus faecalis isolated from urinary tract infections. Infect Drug Resist. (2020) 13:553–9. doi: 10.2147/IDR.S237299
7. Crawford L. Senior management perceptions of project management competence. Int J Proj Manag. (2005) 23:7–16. doi: 10.1016/j.ijproman.2004.06.005
8. U.S. Food and Drug Administration. Available online at: https://www.fda.gov/animal-veterinary/recalls-withdrawals/withdrawal-enrofloxacin-poultry (accessed May 25, 2022).
9. Beechinor JG. Report on consumption of veterinary antimicrobial drugs in Ireland in 2009. Ir Med J. (2011) 104:1–3.
10. Animal and plant quarantine agency. Enrofloxacin. (2021). Available online at: https://www.qia.go.kr/popup_search/Search.jsp (accessed July 7, 2022).
11. Korea Agricultural Marketing Information Service. Report of Domestic Distribution. Naju: Korea Agro-Fisheries & Food Trade Coporation. (2020). p. 251–77.
12. Davies R, Wales A. Antimicrobial resistance on farms: a review including biosecurity and the potential role of disinfectants in resistance selection. Compr Rev Food Sci Food Saf. (2019) 18:753–74. doi: 10.1111/1541-4337.12438
13. Processing Standards and Ingredient Specifications for Livestock Products. Chongju, Korea. Minist Food Drug Saf. (2020). Available online at: http://www.foodsafetykorea.go.kr/foodcode/01_02.jsp?idx=%0A263 (accessed May 25, 2022).
14. Dutka-Malen S, Evers S, Courvalin P. Detection of glycopeptide resistance genotypes and identification to the species level of clinically relevant enterococci by PCR. J Clin Microbiol. (1995) 33:1434. doi: 10.1128/jcm.33.5.1434-1434.1995
15. CLSI. M100-ED29: 2021 Performance Standards for Antimicrobial Susceptibility Testing, 30th Edition. Wayne, PA: Centers for Disease Control. (2020). p. 50–1.
16. Leavis HL, Willems RJL, Top J, Bonten MJM. High-level ciprofloxacin resistance from point mutations in gyrA and parC confined to global hospital-adapted clonal lineage CC17 of enterococcus faecium. J Clin Microbiol. (2006) 44:1059–64. doi: 10.1128/JCM.44.3.1059-1064.2006
17. Magiorakos AP, Srinivasan A, Carey RB, Carmeli Y, Falagas ME, Giske CG, et al. Multidrug-resistant, extensively drug-resistant and pandrug-resistant bacteria: an international expert proposal for interim standard definitions for acquired resistance. Clin Microbiol Infect. (2012) 18:268–81. doi: 10.1111/j.1469-0691.2011.03570.x
18. El Amin N, Jalal S, Wretlind B. Alterations in gyrA and parC associated with fluoroquinolone resistance in Enterococcus faecium. Antimicrob Agents Chemother. (1999) 43:947–9. doi: 10.1128/aac.43.4.947
19. Bakshi CS, Shah DH, Verma R, Singh RK, Malik M. Rapid differentiation of Mycobacterium bovis and Mycobacterium tuberculosis based on a 127-kb fragment by a single tube multiplex-PCR. Vet Microbiol. (2005) 109:211–6. doi: 10.1016/j.vetmic.2005.05.015
20. Predari SC, Ligozzi M, Fontana R. Genotypic identification of methicillin-resistant coagulase-negative staphylococci by polymerase chain reaction. Antimicrob Agents Chemother. (1991) 35:2568–73. doi: 10.1128/AAC.35.12.2568
21. Okamoto R, Okubo T, Inoue M. Detection of genes regulating β-lactamase production in Enterococcus faecalis and Staphylococcus aureus. Antimicrob Agents Chemother. (1996) 40:2550–4. doi: 10.1128/aac.40.11.2550
22. Aarestrup FM, Agerso Y, Gerner-Smidt P, Madsen M, Jensen LB. Comparison of antimicrobial resistance phenotypes and resistance genes in Enterococcus faecalis and Enterococcus faecium from humans in the community, broilers, and pigs in Denmark. Diagn Microbiol Infect Dis. (2000) 37:127–37. doi: 10.1016/S0732-8893(00)00130-9
23. Kariyama R, Mitsuhata R, Chow JW, Clewell DB, Kumon H. Simple and reliable multiplex PCR assay for surveillance isolates of vancomycin-resistant enterococci. J Clin Microbiol. (2000) 38:3092–5. doi: 10.1128/jcm.38.8.3092-3095.2000
24. Agersø Y, Pedersen AG, Aarestrup FM. Identification of Tn5397-like and Tn916-like transposons and diversity of the tetracycline resistance gene tet(M) in enterococci from humans, pigs and poultry. J Antimicrob Chemother. (2006) 57:832–9. doi: 10.1093/jac/dkl069
25. Robicsek A, Strahilevitz J, Sahm DF, Jacoby GA, Hooper DC. qnr prevalence in ceftazidime-resistant enterobacteriaceae isolates from the United States. Antimicrob Agents Chemother. (2006) 50:2872–4. doi: 10.1128/AAC.01647-05
26. Cavaco LM, Hasman H, Xia S, Aarestrup FM. qnrD, a novel gene conferring transferable quinolone resistance in Salmonella enterica serovar Kentucky and Bovismorbificans strains of human origin. Antimicrob Agents Chemother. (2009) 53:603–8. doi: 10.1128/AAC.00997-08
27. Zenteno-Cuevas R, Zenteno JC, Cuellar A, Cuevas B, Sampieri CL, Riviera JE, et al. Mutations in rpoB and katG genes in mycobacterium isolates from the Southeast of Mexico. Mem Inst Oswaldo Cruz. (2009) 104:468–72. doi: 10.1590/S0074-02762009000300012
28. Di Cesare A, Luna GM, Vignaroli C, Pasquaroli S, Tota S, Paroncini P, et al. Aquaculture can promote the presence and spread of antibiotic-resistant enterococci in marine sediments. PLoS ONE. (2013) 8:e62838. doi: 10.1371/journal.pone.0062838
29. Osman KM, Badr J, Orabi A, Elbehiry A, Saad A, Ibrahim MDS, Hanafy MH. Poultry as a vector for emerging multidrug resistant Enterococcus spp: First report of vancomycin (van) and the chloramphenicol–florfenicol (cat-fex-cfr) resistance genes from pigeon and duck faeces. Microb Pathog. (2019) 128:195–205. doi: 10.1016/j.micpath.2019.01.006
30. Schlüter S, Franz CMAP, Gesellchen F, Bertinetti O, Herberg FW, Schmidt FRJ. The high biofilm-encoding bee locus: a second pilus gene cluster in enterococcus faecalis? Curr Microbiol. (2009) 59:206–11. doi: 10.1007/s00284-009-9422-y
31. Montealegre MC, La Rosa SL, Roh JH, Harvey BR, Murray BE. The Enterococcus faecalis EbpA pilus protein: Attenuation of expression, biofilm formation, and adherence to fibrinogen start with the rare initiation codon ATT. MBio. (2015) 6:15. doi: 10.1128/mBio.00467-15
32. Zheng JX, Bai B, Lin ZW, Pu ZY, Yao WM, Chen Z, et al. Characterization of biofilm formation by Enterococcus faecalis isolates derived from urinary tract infections in China. J Med Microbiol. (2018) 67:60–7. doi: 10.1099/jmm.0.000647
33. Cattoir V. The multifaceted lifestyle of enterococci: genetic diversity, ecology and risks for public health. Curr Opin Microbiol. (2022) 65:73–80. doi: 10.1016/j.mib.2021.10.013
34. Maasjost J, Mühldorfer K, De Jäckel SC, Hafez HM. Antimicrobial susceptibility patterns of enterococcus faecalis and enterococcus faecium isolated from poultry flocks in Germany. Avian Dis. (2015) 59:143–8. doi: 10.1637/10928-090314-RegR
35. Bortolaia V, Espinosa-Gongora C, Guardabassi L. Human health risks associated with antimicrobial-resistant enterococci and Staphylococcus aureus on poultry meat. Clin Microbiol Infect. (2016) 22:130–40. doi: 10.1016/j.cmi.2015.12.003
36. Kim Y. Bin, Seo HJ, Seo KW, Jeon HY, Kim DK, Kim SW, et al. Characteristics of high-Level ciprofloxacin-Resistant enterococcus faecalis and enterococcus faecium from retail chicken meat in Korea. J Food Prot. (2018) 81:1357–63. doi: 10.4315/0362-028X.JFP-18-046
37. Rajendiran S, Veloo Y, Thahir SSA, Shaharudin R. Resistance towards critically important antimicrobials among enterococcus faecalis and e. faecium in poultry farm environments in Selangor, Malaysia. Antibiotics. (2022) 11:1118. doi: 10.3390/antibiotics11081118
38. Sparo M, Delpech G, Allende NG. Impact on public health of the spread of high-level resistance to gentamicin and vancomycin in enterococci. Front Microbiol. (2018) 9:1–10. doi: 10.3389/fmicb.2018.03073
39. Hayer SS, Casanova-Higes A, Paladino E, Elnekave E, Nault A, Johnson T, et al. Global Distribution of fluoroquinolone and colistin resistance and associated resistance markers in Escherichia coli of Swine Origin—A systematic review and meta-analysis. Front Microbiol. (2022) 13:1–13. doi: 10.3389/fmicb.2022.834793
40. Hollenbeck BL, Rice LB. Intrinsic and acquired resistance mechanisms in enterococcus. Virulence. (2012) 3:421–33. doi: 10.4161/viru.21282
41. Rózańska H, Lewtak-Piłat A, Kubajka M, Weiner M. Occurrence of enterococci in mastitic cow's milk and their antimicrobial resistance. J Vet Res. (2019) 63:93–7. doi: 10.2478/jvetres-2019-0014
42. Miller WR, Munita JM, Arias CA. Mechanisms of antibiotic resistance in enterococci. Expert Rev Anti Infect Ther. (2014) 12:1221–36. doi: 10.1586/14787210.2014.956092
43. Oh JY, Kang MS, Kim JM, An BK, Song EA, Kim JY, et al. Characterization of Escherichia coli isolates from laying hens with colibacillosis on 2 commercial egg-producing farms in Korea. Poult Sci. (2011) 90:1948–54. doi: 10.3382/ps.2011-01509
44. Landoni MF, Albarellos G. The use of antimicrobial agents in broiler chickens. Vet J. (2015) 205:21–7. doi: 10.1016/j.tvjl.2015.04.016
45. Burbarelli MF de C, Polycarpo G., do V, Lelis KD, Granghelli CA, Pinho ACC de, Queiroz SRA, et al. The effects of two different cleaning and disinfection programs on broiler performance and microbiological status of broiler houses. Poult Sci. (2017) 96:3188–98. doi: 10.3382/ps/pex153
46. Casey JA, Kim BF, Larsen J, Price LB, Nachman KE. Industrial food animal production and community health. Curr Environ Heal reports. (2015) 2:259–71. doi: 10.1007/s40572-015-0061-0
47. Woolhouse M, Ward M, Van Bunnik B, Farrar J. Antimicrobial resistance in humans, livestock and the wider environment. Philos Trans R Soc B Biol Sci. (2015) 370:83. doi: 10.1098/rstb.2014.0083
48. Yang Y, Xie X, Tang M, Liu J, Tuo H, Gu J, et al. Exploring the profile of antimicrobial resistance genes harboring by bacteriophage in chicken feces. Sci Total Environ. (2020) 700:1–9. doi: 10.1016/j.scitotenv.2019.134446
49. Choi JM, Woo GJ. Transfer of tetracycline resistance genes with aggregation substance in food-borne enterococcus faecalis. Curr Microbiol. (2015) 70:476–84. doi: 10.1007/s00284-014-0742-1
50. Wallace RL, Bulach DM, Jennison A V, Valcanis M, McLure A, Smith JJ, et al. Molecular characterization of Campylobacter spp. recovered from beef, chicken, lamb and pork products at retail in Australia. PLoS ONE. (2020) 15:1–18. doi: 10.1371/journal.pone.0236889
51. Alcalde-Rico M, Hernando-Amado S, Blanco P, Martínez JL. Multidrug efflux pumps at the crossroad between antibiotic resistance and bacterial virulence. Front Microbiol. (2016) 7:1–14. doi: 10.3389/fmicb.2016.01483
52. Masi M, Réfregiers M, Pos KM, Pagès JM. Mechanisms of envelope permeability and antibiotic influx and efflux in Gram-negative bacteria. Nat Microbiol. (2017) 2:1. doi: 10.1038/nmicrobiol.2017.1
53. Vergalli J, Atzori A, Pajovic J, Dumont E, Malloci G, Masi M, et al. The challenge of intracellular antibiotic accumulation, a function of fluoroquinolone influx versus bacterial efflux. Commun Biol. (2020) 3:1–12. doi: 10.1038/s42003-020-0929-x
54. Martins M, Mccusker MP, Viveiros M, Couto I, Fanning S, Pagès J-M, et al. A simple method for assessment of MDR Bacteria for over-expressed efflux pumps. Open Microbiol J. (2013) 3:72–82. doi: 10.2174/1874285801307010072
55. Nishino K, Yamasaki S, Nakashima R, Zwama M, Hayashi-Nishino M. Function and inhibitory mechanisms of multidrug efflux pumps. Front Microbiol. (2021) 12:1–13. doi: 10.3389/fmicb.2021.737288
56. Mohammed HHH, Abuo-Rahma GE-DAA, Abbas SH, Abdelhafez E-SMN. Current trends and future directions of fluoroquinolones. Curr Med Chem. (2019) 26:3132–49. doi: 10.2174/0929867325666180214122944
57. Werner G, Fleige C, Ewert B, Laverde-Gomez JA, Klare I, Witte W. High-level ciprofloxacin resistance among hospital-adapted enterococcus faecium (CC17). Int J Antimicrob Agents. (2010) 35:119–25. doi: 10.1016/j.ijantimicag.2009.10.012
58. González JF, Hahn MM, Gunn JS. Chronic biofilm-based infections: skewing of the immune response. Pathog Dis. (2018) 76:1–7. doi: 10.1093/femspd/fty023
59. Machado TS, Pinheiro FR, Soares L, Andre P, Freire R, Pereira A, et al. virulence factors found in nasal colonization and infection of methicillin-resistant staphylococcus aureus (MRSA) isolates and their ability to form a biofilm. Toxins. (2020) 13:14. doi: 10.3390/toxins13010014
60. Benbelaïd F, Khadir A, Abdoune MA, Bendahou M, Muselli A, Costa J. Antimicrobial activity of some essential oils against oral multidrug-resistant Enterococcus faecalis in both planktonic and biofilm state. Asian Pac J Trop Biomed. (2014) 4:463–72. doi: 10.12980/APJTB.4.2014C1203
61. Al-Shammary AHA. Run-off patterns of vancomycin resistant enterococci (VRE clones) in cows raw milk and imported milk powders at baghdad markets. Iraqi J Vet Med. (2019) 43:65–70. doi: 10.30539/iraqijvm.v43i2.532
Keywords: high level ciprofloxacin resistance, Enterococcus faecalis, broiler, Korea, biofilm, antimicrobial resistance genes, multidrug resistance, mutation
Citation: Lee YJ, Jung H-R, Yoon S, Lim S-K and Lee YJ (2023) Situational analysis on fluoroquinolones use and characterization of high-level ciprofloxacin-resistant Enterococcus faecalis by integrated broiler operations in South Korea. Front. Vet. Sci. 10:1158721. doi: 10.3389/fvets.2023.1158721
Received: 04 February 2023; Accepted: 10 March 2023;
Published: 03 April 2023.
Edited by:
Khalid Mehmood, Islamia University of Bahawalpur, PakistanReviewed by:
Alois Cizek, University of Veterinary and Pharmaceutical Sciences Brno, CzechiaNing Xu, Yangtze River Fisheries Research Institute (CAFS), China
Copyright © 2023 Lee, Jung, Yoon, Lim and Lee. This is an open-access article distributed under the terms of the Creative Commons Attribution License (CC BY). The use, distribution or reproduction in other forums is permitted, provided the original author(s) and the copyright owner(s) are credited and that the original publication in this journal is cited, in accordance with accepted academic practice. No use, distribution or reproduction is permitted which does not comply with these terms.
*Correspondence: Young Ju Lee, eW91bmdqdSYjeDAwMDQwO2tudS5hYy5rcg==