- 1The Russian State Center for Animal Feed and Drug Standardization and Quality (VGNKI), Moscow, Russia
- 2The Lopukhin Federal Research and Clinical Center of Physical-Chemical Medicine of FMBA of Russia, Moscow, Russia
Over the past 10 years, immunization of cattle in Russia has been performed using vaccines from Brucella abortus strains 82, 19 and 75/79. To prevent brucellosis in small ruminants, two vaccines have been used, from the Brucella melitensis strain REV-1 and the B. abortus strain 19; note that twice as many animals have been immunized with the former vaccine than with the latter vaccine. The disadvantage of using these preparations is the formation of prolonged post-vaccination seropositivity, which is especially pronounced in animals after immunization with vaccines from B. abortus strain 19 and B. melitensis strain REV-1. This study aims to perform the whole genome sequencing of Brucella vaccine strains from the Russian collection. A bioinformatics analysis of the genomic data proved that the vaccine strains 75/79AB, 82, R-1096, and the KV 17/100 belong to ST-2, 104 M to ST-1, KV 13/100 to ST-5. This analysis allowed us to characterize vaccine strains’s phylogenetic relationships and to prove the close relation of vaccine strains 75/79AB, 82, R-1096. Also, we defined candidate mutations in genes pmm, wbdA, wbkA, wboA, and eryB, which could be responsible for the attenuated virulence of vaccine strains. The complete genomic sequences of B. abortus strains make further studies of bacterial pathogenicity determinants and virulence phenotype feasible, as well as their use in quality control of animal medicines.
Introduction
The development of measures for specific prophylaxis of brucellosis in the world began almost immediately from the moment of discovery of its causative agent. It was started by Bang who for the first time isolated microorganisms from the amniotic fluid of a cow that suffered abortion, selected the correct medium and determined optimal conditions for their cultivation (1). By infecting animals, he also proved that the isolated microorganisms are the causative agent of infectious abortion; he later noted that infected cows acquired certain immunity to this disease. It was further evidenced by observing cases of self-recovery of animals from infectious abortion. Based on this, Bang concluded that it is possible and necessary to develop measures for specific disease prophylaxis. Many researchers began to work in this direction, and, first of all, Bang himself.
In 1906, Bang reported the results of experiments which allowed him to form an immunity in pregnant sheep, goats and cows by inoculating them with live virulent cultures of Brucella. In 1909, he for the first time in the field experiments performed the intravenous administration of live Brucella broth cultures to animals, as a result of which they developed a high degree of immune response, which, however, was accompanied by signs of anaphylactic shock.
Since 1910, in England, Germany, Denmark, United States, and Argentina, agar swabs of Brucella cultures were used for mass vaccination of young and non-pregnant cows. Although the number of abortions in animals was slightly decreasing, the damage from the infection was about the same as in the natural course of the disease. The widespread use of virulent cultures caused abortions in pregnant animals and essentially led to their massive artificial infection. Thus, vaccinated animals presented an epizootic and epidemic danger.
Due to this, later on strains with attenuated virulence were used for the production of vaccines. Attenuated virulence was achieved by treating virulent cultures of Brucella with chemical, physical and biological methods. Some of the researchers were able to achieve significant success.
In 1934, Corner et al. (2) worked with a culture of Brucella abortus strain 19 isolated by Buck in 1923 from the milk of a cow of the third calving and found that after storage for one year at room temperature the culture spontaneously reduced its virulence. The authors were able to select an immunogenic and stable strain which subsequently was named Buck-19 [B-19] in honor of the author of the original culture. From that moment on, a new stage in the development of anti-brucellosis vaccines has begun, while the vaccine from strain 19 remains the standard of immunogenicity until now.
Brucella species are characterized by high invasiveness which allows them to successfully multiply in macrophages and lymphocytes (3). At the same time, Brucella lack virulence factors such as the capsule, flagella, fimbriae, pili, plasmids, toxins, exotoxins, cytolysins, and secreted proteases found in other bacteria (4, 5). Various virulence factors, the mechanism of evasion from the host defense systems and the method of intracellular survival of Brucella were reviewed in detail in (6). It was shown that the virulence of Brucella can be determined by: lipopolysaccharide (LPS) (7), β-glucan (β-cyclic glucan) (8, 9), BvrS/BvrR, BacA (10), outer membrane proteins (Omps) (11), BmaC (12), SagA (13), BtaE (14), BetB (15), MucR and T4SS genes found in virB operon (16). The vital virulence factor, LPS, determines the morphology of Brucella colonies in culture. The smooth phenotype (S-form) is formed due to complete LPS, consisting of lipid A, core oligosaccharide, and O-side chains of the polysaccharide. The strains of Brucella with attenuated virulence often form rough colonies (R-form) due to a deficiency of O-side chains of the polysaccharide (17).
In accordance with the List of Prokaryotic Names with Standing in Nomenclature (LPSN), the causative agent of brucellosis is assigned to the genus Brucella, family Brucellaceae, order Rhisobailes, class Alphaproteobacteria. The genus Brucella consists of 13 independent species. A total of six typical and seven new Brucella species were identified in a wide range of susceptible hosts. There are seven species that infect terrestrial animals, including B. abortus, Brucella melitensis, Brucella suis, Brucella ovis, Brucella canis, Brucella neotomae, and Brucella microti (18); two more species, Brucella ceti and Brucella pinnipedialis, infect marine mammals (19). Brucella papionis was isolated from baboons and Brucella vulpis from red foxes (20). Seven biovars have been recognized for B. abortus, three for B. melitensis and five for B. suis. Other species are not subdivided into biovars. Brucella nomenclature is based on the species of the main hosts (21). As the list of species grows, it is important to identify more effective prophylaxis measures to control the spread of the disease in humans.
Considering the complexity of differentiation of various species of Brucella bacteria, including using 16sRNA analysis for typing and comparison with other strains, we carried out whole genome sequencing and phylogenetic analysis by single nucleotide polymorphisms (SNPs) in order to comparatively assess the molecular-genetic characteristics of strains and analyze alternative options for the production of vaccines in Russia.
Materials and methods
Object of study
The strains for this study were obtained from the All-Russian State Collection of Microorganisms Used in Veterinary Medicine and Animal Husbandry of VGNKI: B. abortus 82, B. abortus 75/79-AB, B. abortus 104 M, B. abortus KV 17/100, B. abortus R-1096, B. abortus KV 13/100. To construct a phylogenetic tree based on SNP loci, we selected genomes of vaccine strains and field isolates from the NCBI database that were assembled completely or at the scaffold level (Table 1).
Microbiological cultivation methods
Bacteria were cultivated on Brucella agar at 37°C for 48 h. A microbial suspension with a concentration of 1.7 × 109 CFU/mL was disinfecte—d by adding sodium merthiolate (0.01%) and incubating at 56°C for 30 min. Additional incubation with CO2 was not performed.
Method for studying immunogenicity
The immunogenicity of the microorganism strains was studied in guinea pigs by introducing 10–15 minimal infectious doses of the culture of the control virulent strain (B. abortus 54 VGNKI).
Extraction of genomic DNA
Total genomic DNA was isolated using the DNA-sorb-V Kit (Central Research Institute for Epidemiology, Russia) in accordance with the manufacturer’s instructions. DNA concentration was measured on a Quantus fluorimeter (Promega, United States) using the QuantiFluor®ONE dsDNA System Kit (Promega, United States).
Genome sequencing, bioinformatics analysis
DNA libraries were prepared using the Nextera XT DNA Sample Preparation Kit in accordance with the manufacturer’s instructions. Whole genome sequencing was performed on a MiSeq system (Illumina, United States) in accordance with the standard operating procedure.
For bioinformatics analysis of data of whole genome sequencing and de novo genome assembly, the following programs were used: FastQC 0.11.17 (31), Trimmomatic v.0.36 (32), SPAdes 2.11.1 (33), QUAST 4.6.3 (34), MAUVE v.20150226 (35). Bacterial species identification and multilocus sequence typing (MLST) were performed via the online service of the Center for Genomic Epidemiology of the Danish University of Technology (CGE) using the KmerFinder server (version 3.0.2) and MLST server (version 2.0.4) (36, 37). Annotation of bacterial genomes was performed using the RAST server (38).
The search for antibiotic resistance genes was carried out using the ResFinder 4.1 online service (39) as well as the Arg-ANNOT, CARD and NCBI BARRGD databases (40). Identification was carried out with the ABRicate program (41) using BLASTN and BLASTX against nucleotide and amino acid sequences from various databases. To search for the main virulence factors in bacterial genomes, the Virulence Factor Database (VFDB) (42) and Victors database were used (43); to search for integrons, the IntegronFinder v5 program was used (44). For phylogenetic analysis, the kSNP v.3.1.2 program was used (45). For visualization, the service iTOL v.6.4.3 (Interactive Tree of Life) was used (46).
Results and discussion:
An analysis of epizootic data from 1946 to the present day shows that brucellosis in cattle on the territory of Russia was not regx+istered only in the Kamchatka. Circulation of a total of 6 species of Brucella was detected: B. abortus, B. melitensis, B. suis, B. neotomae, B. ovis and B. canis. The most virulent for humans are B. melitensis, B. abortus and B. suis, and to a lesser extent B. canis, B. ceti and B. pinnipedialis. Therefore, when conducting laboratory diagnostics for brucellosis, it is important to perform not only the isolation of the culture of the pathogen, but also the identification of the specific species. To use as part of the composition for specific prophylaxis in animals, different authors proposed 25 strains of microorganisms of the genus Brucella, mainly B. abortus, B. melitensis and rarely B. suis. So far, however, only 9 of them were used in the composition of vaccines that were used to treat farm animals (Table 2).
For the mass prophylaxis of brucellosis in cattle, live vaccines from B. abortus strains 19, 82 and 75/79-AB were used in different volumes, in small ruminants—from B. melitensis strain Rev-1 and B. abortus strain 19. It is important to note that there exist no Brucella vaccines for medical and veterinary use guaranteed to protect all those immunized. The degree of their protection depends on the virulence and dose of field Brucella cultures (56). Until 1952, the measures against brucellosis in the Russia consisted of conducting diagnostic studies and removing sick animals from herds, without the use of anti-brucellosis vaccines. Due to the aggravation of the epizootic situation in 1953, the system of anti-brucellosis measures was expanded: it included immunization of animals with a vaccine from the B. abortus strain 19. This vaccine was used until 1975. During the period of active implementation of the vaccine, the disease was eliminated from many farms and even entire regions. However, in regions with a wide spread of brucellosis, the effectiveness of these sanation measures was insufficient. This was primarily due to the high agglutinogenicity of the vaccine. Agglutinins and complement-binding antibodies are preserved in the bodies of repeatedly immunized animals for up to 5–8 years, which makes it extremely difficult to differentiate such animals from those with brucellosis.
According to official data (57), in the period of 2010–2021 three vaccines were used in Russia for immunization of cattle (Figure. 1), the main of which was the vaccine from the B. abortus strain 82. To prevent brucellosis in small ruminants, two vaccines were used (Figure. 2); among them, the vaccine from the B. melitensis REV-strain 1 was used to immunize twice as many animals as the vaccine from the B. abortus strain 19.
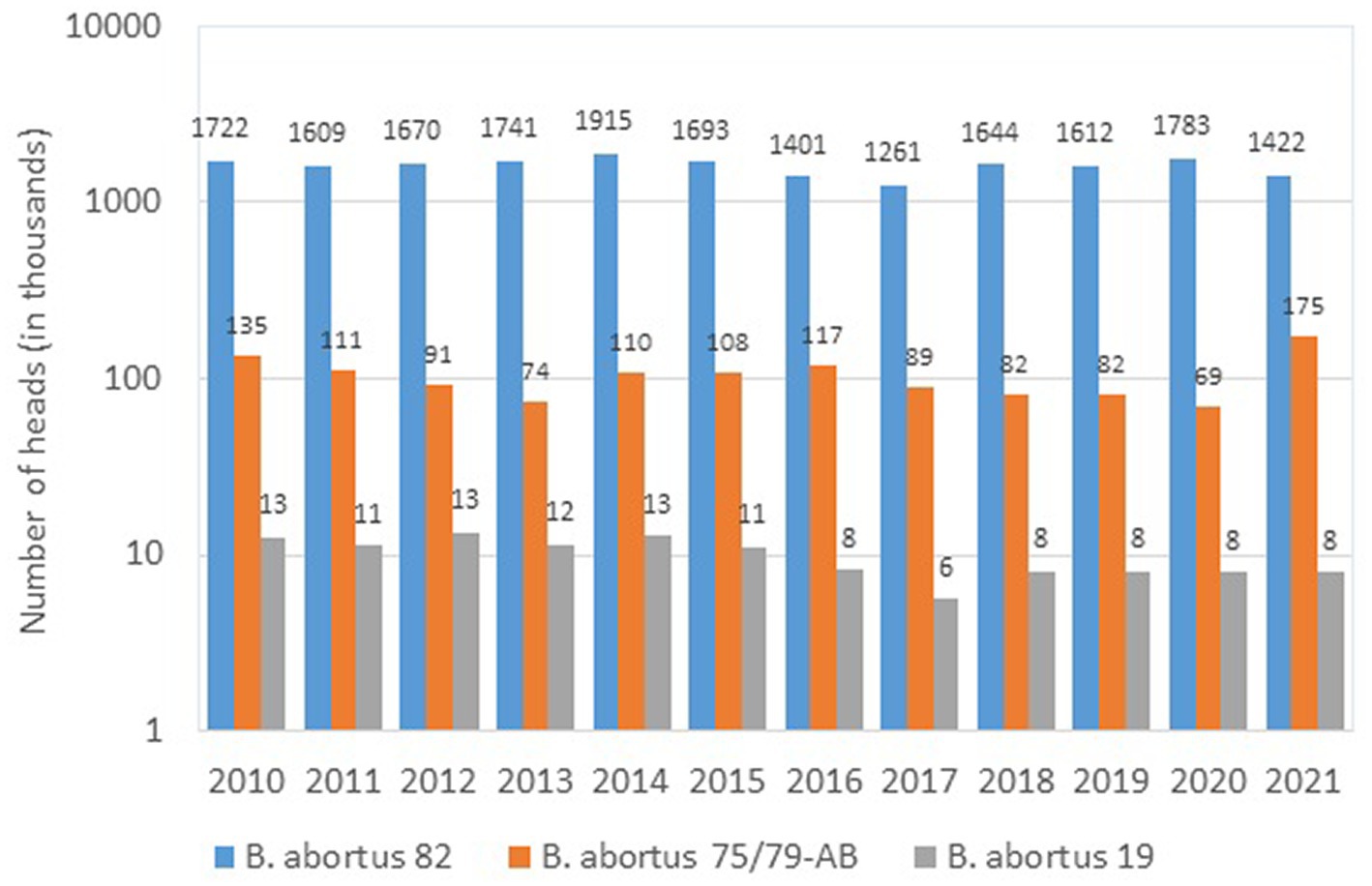
Figure 1. Brucella strains in the composition of vaccines used for the prophylaxis of brucellosis in cattle in Russia (2010–2021, log scale).
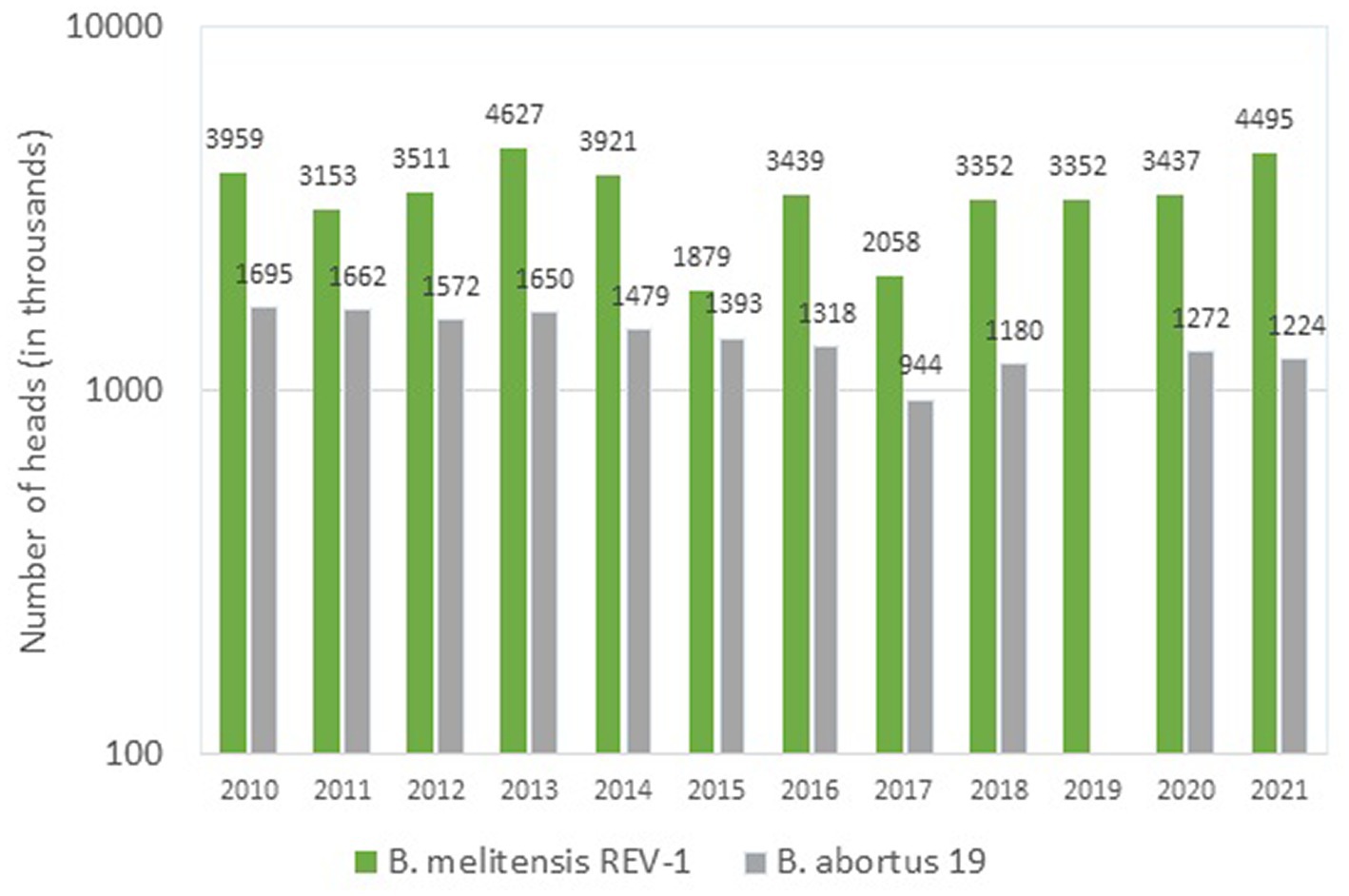
Figure 2. Brucella strains in the composition of vaccines used for the prophylaxis of brucellosis in small ruminants in Russia (2010–2021, log scale).
As seen from the Figures 1, 2, over the past 11 years up to two million heads of cattle have been immunized annually with a vaccine from the B. aborus strain 82 (from 1,261.3 thousand heads in 2017 to 1,915.3 thousand heads in 2014). Despite that, today this vaccine, as almost any other live vaccine, does not fully meet the requirements of veterinary practice due to its abortogenic properties. Nonetheless, to a large extent, this problem can be solved by immunizing heifers at the age of 4–6 and/or 12–14 months. Subsequently, sensitized animals can be immunized against brucellosis during pregnancy, but only if the infection with field cultures of Brucella is completely ruled out. However, the bulk of the breeding stock of cattle is immunized after calving.
The B. abortus strain KV 17/100-VGNKI was obtained by targeted selection for cultural, morphological, biochemical and antigenic properties is very promising for the production of an inactivated vaccine with an adjuvant when constructing and testing a mini-batch (58). This vaccine does not induce the synthesis of S-Brucella antibodies in diagnostic titers in animals not infected with Brucella but induces it in cattle with a latent form of brucellosis, which allows, due to the rapid removal of such animals from herds, to accelerate the recovery of affected farms from brucellosis. At the same time, it does not have abortogenic properties and can be used for immunization of pregnant cows and heifers. Moreover, since it is in an inactivated state, it does not pose an environmental hazard.
Next, we performed whole genome sequencing of six vaccine strains: Brucella abortus strain 82, B. abortus strain 75/79-AB and B. abortus strain R-1096; B. abortus strain KV 17/100 and B. abortus strain 104 M used as an antigen to provoke latent forms of brucellosis; and B. abortus strain KV 13/100 used to control the immunogenic activity of inactivated vaccines (Table 3).
The bioinformatics analysis of genomic data of Brucella abortus strains
The genomes of all Brucella species have the same size and genome map (59). The average size of the genome which consists of two ring chromosomes is approximately 3.29 Mb.
The quality of sequencing data (FASTQ files) was assessed using the FastQC_0.11.17. The removal of technical sequences and low-quality nucleotides was performed in the Trimmomatic v.0.36 with the following ILLUMINACLIP parameters: NexteraPE-PE.fa: 2:30:10, SLIDINGWINDOW: 4:15, MINLEN: 50. The de novo assembly of bacterial genomes was performed using SPAdes 2.11.1 with the sequencing error correction and automatic selection of k-mer length (21, 33, 55, 77, 99). Contigs shorter than 500 bp were excluded from further analysis. The assembly with the smallest number of contigs and the largest N50 value was chosen as the best one. The main characteristics of the assembly were obtained using the QUAST 4.6.3 and are presented in Table 4.
To identify a bacterial species using the assembled contigs, we used the method of searching for common k-mers. Multilocus typing of the strain was carried out for the aroA, cobQ, dnaK, gap, glk, gyrB, int_hyp, omp25, and trpE loci. The MLST profiles and loci sequences were obtained from the PubMLST database (23). Genotyping data are shown in Table 5.
Annotation of genomes was performed using the RAST server on the SEED, an open platform for comparative analysis of genomes. The preliminary contigs were ordered using the MAUVE v.20150226 relative to the corresponding sequences indicated in Table 5 in the KmerFinder column.
To analyze contig sequences for the presence of various antibiotic resistance genes, the following criteria were used: >95% identity, >80% minimum intersection length. No antibiotic resistance genes were identified in any of the strain. Genome analysis did not reveal the presence of integrons in any of the strains.
Phylogenetic analysis of Brucella abortus vaccine strains
For typing and comparison with other strains, a phylogenetic analysis was carried out using single nucleotide substitutions.
To construct a phylogenetic tree based on SNP loci, we selected the genomes of vaccine strains and field isolates from the NCBI database that were assembled completely or at the scaffold level and added 6 vaccine strains from the All-Russian State Collection of Microorganisms Used in Veterinary Medicine and Animal Husbandry. The list of strains from the NCBI database is presented in Table 1. Pan-genome analysis was carried out using the program kSNP v.3.1. In total, we identified 14,768 SNPs, of which 11,510 are core SNPs. The rooted tree of all SNPs was constructed using the maximum parsimony method (Figure. 3). When constructing the tree, the genome of Brucella melitensis bv. 1 str. 16 M (NC_003317.1) was used as an outgroup.
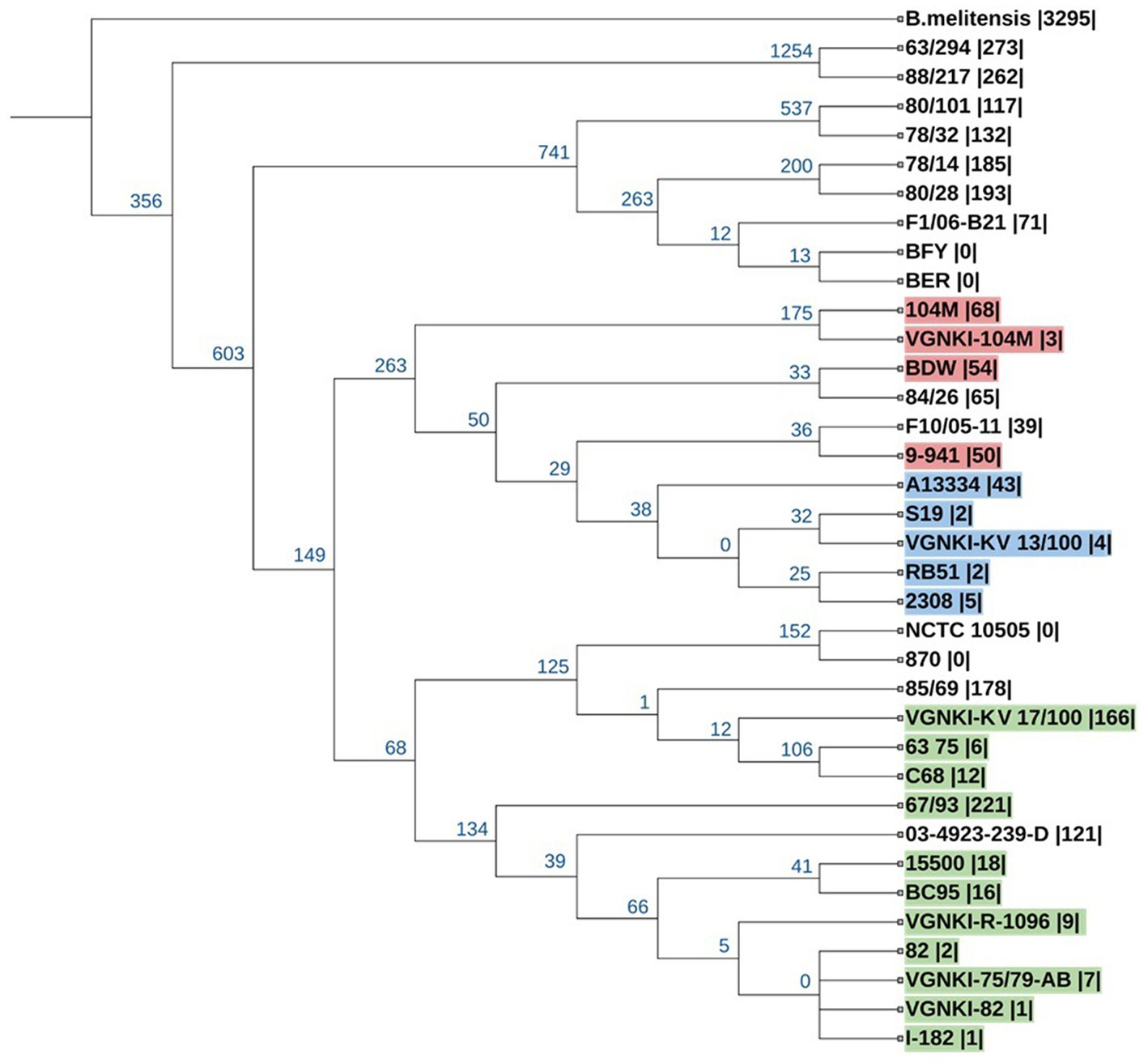
Figure 3. Phylogenetic tree of Brucella abortus for 11,510 SNPs. The prefix “VGNKI” indicates strains sequenced in this study. Numbers in blue at the roots of nodes show the number of SNPs that are shared exclusively among the descendants of each node. The number of unique SNPs for the genome is given in parentheses. Strains of ST-1 are highlighted in red, ST-2—in green, and ST-5—in blue.
Vaccine strain KV 13/100 (ST-5) belong to the same clade as vaccine strain S19 and it is a close relative to the vaccine strain RB-51. Vaccine strain 104 M (ST-1) is more distant relative to afore mentioned vaccine strains. Vaccine strains R-1096, 75/79AB and 82 (ST-2) belong to the same clade and very similar to each other. They are related distantly to vaccine strain KV 17/100-VGNKI (ST-2). It should be noted that the vaccine strain 104 M transferred from Soviet Russia to China 60 years ago (60) has acquired more than 60 unique SNPs compared to the Russian collection strain.
Analysis of mutations in the virulence genes of industrial Brucella abortus strains
The complete genome sequence of B. abortus provides an important resource for future studies of pathogenicity determinants and virulence phenotypes of these bacteria, as well as serves as a basis for quality control of medicinal products for animals (29).
We analyzed the nucleotide sequence of virulence genes in our vaccine strains using the list of Brucella virulence genes from VFDB and the Victors database. For every SNP, we estimated its possible contribution to protein function using SIFT (61), then additionally checked the protein conservation level in the mutation site using UniProt (62). We found alterations and mutations that could explain the attenuated virulence in all six vaccine strains (Table 6).
So, the virulence genes wbdA and wboA is absent in the vaccine strain R-1096. The IS711-like insertion sequence destroys the pmm gene in the vaccine strain 13/100. The eryB gene in strain 104 M codes a non-functional truncated protein because of nonsense mutation W215X. The attenuated phenotype of the strains KV 17/100, 75/79-AB, and 82 can be explained by missense mutations that occur in conservative sites of functional domains in virulence proteins. The strain KV 17/100 has a substitution mutation G255V in a very conservative site of the glycosyl transferase domain of the wbkA protein (Supplementary Figure S1). Both strains 75/79-AB and 82 have a substitution W219L located in a conservative site of the glycosyl transferase domain of the wboA protein (Supplementary Figure S2). The strain KB 17/100 has two substitutions N255K in the pmm gene and A321 in the wbkA gene, which can also negatively impact the function of corresponding proteins because they are in semi-conservative sites (Supplementary Figures S3, S4).
Thus, the five vaccine strains analyzed here are deficient in classical virulence genes responsible for the biosynthesis of LPS O-chain: pmm, wbdA, wbkA, wboA (63). These mutations can be responsible for these vaccine strains’ R-form type of colonies (Table 3). The deficiency of the wboA gene observed here in strains R-1096, 75/79-AB, and 82 was reported earlier for vaccine strain RB51 (64).
Yu et al. (22) described a set of candidate genes associated with virulence attenuation in vaccine strain 104 M. We complement this set with gene eryB, whose function is impaired by preliminary stop codon W215X. The gene eryB is a part of operon eryABCD encoding the erythritol dissimilative pathway (65, 66) reported that the growth of vaccine strain B19 was inhibited by erythritol due to mutations in genes eryC and eryD.
Conclusion
This study represents a comprehensive bioinformatics analysis of genomes of 6 Brucella vaccine strains. The results of our study serve as a prerequisite for improving the diagnostics of brucellosis in animals and will allow to reduce the risks associated with the spread of this infection in Russia, as well as to improve the control over the quality and safety of anti-brucellosis immunobiological agents.
Data availability statement
The datasets presented in this study can be found in online repositories. The name of the repository and accession number can be found below: NCBI; PRJNA932701, https://www.ncbi.nlm.nih.gov/.
Ethics statement
The study was approved by the Ethics Committee of VGNKI.
Author contributions
AP: project management and securing funding. OS and AM: conceptualization and methodology. IS and IT: molecular genetic studies. AB and VG: bioinformatics analysis. OP, EK, and OS: writing and editing. All authors contributed to the article and approved the submitted version.
Funding
This work was supported by the grant # 075-15-2021-1053 from the Ministry of Science and Higher Education of the Russian Federation.
Acknowledgments
The authors express their gratitude to the staff of the All-Russian State Collection of Microorganism Used in Veterinary Medicine and Animal Husbandry for providing materials for the study.
Conflict of interest
The authors declare that the research was conducted in the absence of any commercial or financial relationships that could be construed as a potential conflict of interest.
Publisher’s note
All claims expressed in this article are solely those of the authors and do not necessarily represent those of their affiliated organizations, or those of the publisher, the editors and the reviewers. Any product that may be evaluated in this article, or claim that may be made by its manufacturer, is not guaranteed or endorsed by the publisher.
Supplementary material
The Supplementary material for this article can be found online at: https://www.frontiersin.org/articles/10.3389/fvets.2023.1154520/full#supplementary-material
References
1. Bang, B., and Stribold,. The etiology of contagious abortion Zeitschr.f. Tiermed, (1897). 1:103–105.
2. Corner, LA, and Alton, GG. Persistence of Brucella abortus strain 19 infection in adult cattle vaccinated with reduced doses. Res Vet Sci. (1981) 31:342–4. doi: 10.1016/S0034-5288(18)32468-8
3. Smith, R, Smoll, F, and Schutz, R. Measurement and correlates of sport-specific cognitive and somatic trait anxiety: the sport anxiety scale. Anxiety Res. (1990) 2:263–80. doi: 10.1080/08917779008248733
4. DelVecchio, VG, Kapatral, V, Redkar Guy Patra, RJ, Mujer, C, Los, T, Ivanova, N, et al. The genome sequence of the facultative intracellular pathogen Brucella melitensis. Proc Natl Acad Sci. (2002) 99:443–8. doi: 10.1073/pnas.22157539
5. Richard, C, Essenberg, RS, Nelson, K, and Paulsen, I. Sugar metabolism by Brucellae. Vet Microbiol. (2002) 90:249–61. doi: 10.1016/S0378-1135(02)00212-2
6. Gopalakrishnan, A, Dimri, U, Saminathan, M, Yatoo, MY, Priya, BG, Gopinath, D, et al. Virulence factors, intracellular survivability and mechanism of evasion from host immune response by Brucella: an overview. J Anim Plant Sci. (2016) 26:1542–55.
7. Lapaque, N, Moriyon, I, Moreno, E, and Gorvel, J-P. Brucella lipopolysaccharide acts as a virulence factor. Curr Opin Microbiol. (2005) 8:60–6. doi: 10.1016/j.mib.2004.12.003
8. Martirosyan, A, Moreno, E, and Gorvel, J. An evolutionary strategy for a stealthy intracellular Brucella pathogen. Immunol Rev. (2011) 240:211–34. doi: 10.1111/j.1600-065X.2010.00982.x
9. Martirosyan, A, Pérez-Gutierrez, C, Banchereau, R, Dutartre, H, Lecine, P, Dullaers, M, et al. Brucella β 1,2 cyclic glucan is an activator of human and mouse dendritic cells. PLoS Pathog. (2012) 8:e1002983. doi: 10.1371/journal.ppat.1002983
10. Martin, R. Regional economic resilience, hysteresis and recessionary shocks. J Econ Geogr. (2012) 12:1–32. doi: 10.1093/jeg/lbr019
11. Lim, JJ, Kim, DH, Lee, JJ, Kim, DG, Min, W, Lee, HJ, et al. Evaluation of recombinant 28 kDa outer membrane protein of Brucella abortus for the clinical diagnosis of bovine brucellosis in Korea. J Vet Med Sci. (2012) 74:687–91. doi: 10.1292/jvms.11-0512
12. Posadas, DM, Ruiz-Ranwez, V, Bonomi, HR, Martín, FA, and Zorreguieta, A. BmaC, a novel autotransporter of Brucella suis, is involved in bacterial adhesion to host cells. Cell Microbiol. (2012) 14:965–82. doi: 10.1111/j.1462-5822.2012.01771.x
13. Del Giudice, MG, Ugalde, JE, and Czibener, C. A lysozyme-like protein in Brucella abortus is involved in the early stages of intracellular replication. Infect Immun. (2013) 81:956–64. doi: 10.1128/IAI.01158-12
14. Ruiz-Ranwez, V, Posadas, DM, Estein, SM, Abdian, PL, Martin, FA, and Zorreguieta, A. The BtaF trimeric autotransporter of Brucella suis is involved in attachment to various surfaces, resistance to serum and virulence. PLoS One. (2013) 8:e79770. doi: 10.1371/journal.pone.0079770
15. Lee, JJ, Kim, JH, Kim, DG, Kim, DH, Simborio, HL, Min, WG, et al. Characterization of betaine aldehyde dehydrogenase (BetB) as an essential virulence factor of Brucella abortus. Vet Microbiol (2014); 168, 131–140. doi: 10.1016/j.vetmic.2013.10.007
16. Mirabella, A, Terwagne, M, Zygmunt, MS, Cloeckaert, A, De Bolle, X, and Letesson, JJ. Brucella melitensis MucR, an orthologue of Sinorhizobium meliloti MucR, is involved in resistance to oxidative, detergent, and saline stresses and cell envelope modifications. J Bacteriol. (2013) 195:453–65. doi: 10.1128/JB.01336-12
17. Mancilla, M. Smooth to rough dissociation in Brucella: the missing link to virulence/M. Mancilla. Front Cell Infect Microbiol. (2016) 5:98. doi: 10.3389/fcimb.2015.00098
18. Scholz, HC, Hubalek, Z, Sedlácek, I, Vergnaud, G, Tomaso, H, Al Dahouk, S, et al. Brucella microti sp. nov., isolated from the common vole Microtus arvalis. Int J Syst Evol Microbiol. (2008) 58:375–82. doi: 10.1099/ijs.0.65356-0
19. Foster, G, Osterman, BS, Godfroid, J, Jacques, I, and Cloeckaert, A. Brucella ceti sp. nov. and Brucella pinnipedialis sp. nov. for Brucella strains with cetaceans and seals as their preferred hosts. Int J Syst Evol Microbiol. (2007) 57:2688–93. doi: 10.1099/ijs.0.65269-0
20. Scholz, HC, Revilla-Fernández, S, Dahouk, SA, Hammerl, JA, Zygmunt, MS, Cloeckaert, A, et al. Brucella vulpis sp. nov., isolated from mandibular lymph nodes of red foxes (Vulpes vulpes). Int J Syst Evol Microbiol. (2016) 66:2090–8. doi: 10.1099/ijsem.0.000998
21. Verger, JM, Grimont, F, Grimont, PA, and Grayon, M. Taxonomy of the genus Brucella. Ann Inst Pasteur Microbiol. (1987) 138:235–8. doi: 10.1016/0769-2609(87)90199-2
22. Yu, D, Hui, Y, Zai, X, Xu, J, Liang, L, Wang, B, et al. Comparative genomic analysis of Brucella abortus vaccine strain 104M reveals a set of candidate genes associated with its virulence attenuation. Virulence. (2015) 6:745–54. doi: 10.1080/21505594.2015.1038015
23. Halling, SM, Peterson-Burch, BD, Bricker, BJ, Zuerner, RL, Qing, Z, Li, LL, et al. Completion of the genome sequence of Brucella abortus and comparison to the highly similar genomes of Brucella melitensis and Brucella suis. J Bacteriol. (2005) 187:2715–26. doi: 10.1128/JB.187.8.2715-2726.2005
24. Minogue, TD, Daligault, HA, Davenport, KW, Bishop-Lilly, KA, Broomall, SM, Bruce, DC, et al. Whole-genome sequences of 24 Brucella strains. Genome Announc. (2014) 2:e00915–4. doi: 10.1128/genomeA.00915-14
25. Paradiso, R, Orsini, M, Criscuolo, D, Borrelli, R, Valvini, O, Cammà, C, et al. Complete genome sequencing of 10 Brucella abortus Biovar 3 strains isolated from water Buffalo. Genome Announc. (2018) 6:e00180–18. doi: 10.1128/genomeA.00180-18
26. Shevtsov, A, Tarlykov, P, Zholdybayeva, E, Shevtsova, E, Momynkulov, D, Sytnik, I, et al. Draft genome sequence of the live vaccine strain Brucella abortus 82. Genome Announc. (2013) 1:e01101–13. doi: 10.1128/genomeA.01101-13
27. Bricker, B, Goonesekere, N, Bayles, D, Alt, D, Olsen, S, and Vrentas, C. Genome report—a genome sequence analysis of the RB51 strain of Brucella abortus in the context of its vaccine properties. G3: genes, genomes. Genetics. (2020) 10:1175–81. doi: 10.1534/g3.119.400964
28. Kim, H, Jeong, W, Jeoung, HY, Song, JY, Kim, JS, Beak, JH, et al. Complete genome sequence of Brucella abortus A13334, a new strain isolated from the fetal gastric fluid of dairy cattle. J Bacteriol. (2012) 194:5444. doi: 10.1128/JB.01124-12
29. Crasta, OR, Folkerts, O, Fei, Z, Mane, SP, Evans, C, Martino-Catt, S, et al. Genome sequence of Brucella abortus vaccine strain S19 compared to virulent strains yields candidate virulence genes. PLoS One. (2008) 3:e2193. doi: 10.1371/journal.pone.0002193
30. Chain, PS, Comerci, DJ, Tolmasky, ME, Larimer, FW, Malfatti, SA, Vergez, LM, et al. Whole-genome analyses of speciation events in pathogenic Brucellae. Infect Immun. (2005) 73:8353–61. doi: 10.1128/IAI.73.12.8353-8361.2005
31. Andrews, S (2010). FastQC: a quality control tool for high throughput sequence data. Available at: http://www.bioinformatics.babraham.ac.uk/projects/fastqc/
32. Bolger, AM, Lohse, M, and Usadel, B. Trimmomatic: a flexible trimmer for Illumina sequence data. Bioinformatics. (2014) 30:2114–20. doi: 10.1093/bioinformatics/btu170
33. Bankevich, A, Nurk, S, Antipov, D, Gurevich, AA, Dvorkin, M, Kulikov, AS, et al. SPAdes: a new genome assembly algorithm and its applications to single-cell sequencing. J Comput Biol. (2012) 19:455–77. doi: 10.1089/cmb.2012.0021
34. Gurevich, A, Saveliev, V, Vyahhi, N, and Tesler, G. QUAST: quality assessment tool for genome assemblies. Bioinformatics. (2013) 29:1072–5. doi: 10.1093/bioinformatics/btt086
35. Darling, AC, Mau, B, Blattner, FR, and Perna, NT. Mauve: multiple alignment of conserved genomic sequence with rearrangements. Genome Res. (2004) 14:1394–403. doi: 10.1101/gr.2289704
36. Hasman, H, Saputra, D, Sicheritz-Ponten, T, Lund, O, Svendsen, CA, Frimodt-Møller, N, et al. Rapid whole-genome sequencing for detection and characterization of microorganisms directly from clinical samples. J Clin Microbiol. (2014) 52:139–46. doi: 10.1128/JCM.02452-13
37. Larsen, MV, Cosentino, S, Rasmussen, S, Friis, C, Hasman, H, Marvig, RL, et al. Multilocus sequence typing of total-genome-sequenced bacteria. J Clin Microbiol. (2012) 50:1355–61. doi: 10.1128/JCM.06094-11
38. Brettin, T, Davis, JJ, Disz, T, Edwards, RA, Gerdes, S, Olsen, GJ, et al. RASTtk: a modular and extensible implementation of the RAST algorithm for building custom annotation pipelines and annotating batches of genomes. Sci Rep. (2015) 10:8365. doi: 10.1038/srep08365
39. Zankari, E, Hasman, H, Cosentino, S, Vestergaard, M, Rasmussen, S, Lund, O, et al. Identification of acquired antimicrobial resistance genes. J Antimicrob Chemother. (2012) 67:2640–4. doi: 10.1093/jac/dks261
40. Gupta, SK, Padmanabhan, BR, Diene, SM, Lopez-Rojas, R, Kempf, M, Landraud, L, et al. ARG-ANNOT, a new bioinformatic tool to discover antibiotic resistance genes in bacterial genomes. Antimicrob Agents Chemother. (2014) 58:212–20. doi: 10.1128/AAC.01310-13
41. Available at: https://github.com/tseemann/abricate (дата обращения 20 11 2022).
42. Liu, B, Zheng, D, Jin, Q, Chen, L, and Yang, J. VFDB 2019: a comparative pathogenomic platform with an interactive web interface. Nucleic Acids Res. (2019) 47:D687–92. doi: 10.1093/nar/gky1080
43. Sayers, S, Li, L, Ong, E, Deng, S, Fu, G, Lin, Y, et al. Victors: a web-based knowledge base of virulence factors in human and animal pathogens. Nucleic Acids Res. (2019) 47:D693–700. doi: 10.1093/nar/gky999
44. Cury, J, Jové, T, Touchon, M, Néron, B, and Rocha, EP. Identification and analysis of integrons and cassette arrays in bacterial genomes. Nucleic Acids Res. (2016) 44:4539–50. doi: 10.1093/nar/gkw319
45. Gardner, SN, Slezak, T, and Hall, BG. kSNP3. 0: SNP detection and phylogenetic analysis of genomes without genome alignment or reference genome. Bioinformatics. (2015) 31:2877–8. doi: 10.1093/bioinformatics/btv271
46. Letunic, I, and Bork, P. Interactive tree of life (iTOL) v5: an online tool for phylogenetic tree display and annotation. Nucleic Acids Res. (2021) 49:W293–6. doi: 10.1093/nar/gkab301
47. Buck, JM. Studies of vaccination during calfhood to prevent bovine infectious abortion. J Agric Res. (1930) 41:667–89.
48. Elberg, SS, Steiner, PE, and Doll, JP. Immunization against Brucella infection. V. Histopathologic appraisal of immunity induced in mice by a streptomycin-dependent mutant of Brucella melitensis. Am J Pathol. (1955) 31:1065–75.
49. Schurig, GG, Roop, RM II, Bagchi, T, Boyle, S, Buhrman, D, and Sriranganathan, N. Biological properties of RB51; a stable rough strain of Brucella abortus. Vet Microbiol. (1991) 28:171–88. doi: 10.1016/0378-1135(91)90091-S
50. Shumilov, KV. Biological properties of the vaccine strain B. abortus 104 M. Proc VIEV. (1983) 57:42–7.
52. RU2113857 C1Nikiforov, I.P., Shumilov, K. V., Kalmykov, V.V., Klimanov, A.I., Shumilov, K. V., and Shumilov, K. V.. Vaccine against brucellosis in cattle. (1997).
53. RU 2130068 C1Kalmykov, V.V., and Shumilov, K. V.. (1999). Brucella abortus strain used for the manufacture of diagnostic and preventive biological preparations against animal brucellosis, Antrag Nr. 97118640/13 vom 12. Nov. 1997.
54. RU 2149184 C1Kalmykov, V.V.. (2000). A method is known for evaluating the immunogenicity of anti-brucellosis vaccine strains by determining the rate of elimination from the body of immunized animals of the Brucella control strain B. abortus KB 13/100-DEPT.
55. SU 946039 АSalmakov, К.М. (1979). A method for preparing an antigen for the differential serological diagnosis of brucellosis in animals, including obtaining a bacterial mass of the B. abortus R-1096 strain and inactivating it by gamma irradiation.
56. Khurana, SK, Sehrawat, A, Tiwari, R, Prasad, M, Gulati, B, Shabbir, MZ, et al. Bovine brucellosis—a comprehensive review. Vet Q. (2021) 41:61–88. doi: 10.1080/01652176.2020.1868616
58. Shumilov, KV, Sklyarov, O, and Klimanov, A. Designing vaccines against cattle brucellosis. Vaccine. (2010) 28:F31–4, ISSN 0264-410X, doi: 10.1016/j.vaccine.2010.03.049
59. Seleem, M, Boyle, S, and Sriranganathan, N. Brucellosis: a re-emerging zoonosis. Vet Microbiol. (2009) 140:392–8. doi: 10.1016/j.vetmic.2009.06.021
60. Li, ZQ, Shi, JX, Fu, WD, Zhang, Y, Zhang, J, Wang, Z, et al. A Brucella melitensis M5-90 wboA deletion strain is attenuated and enhances vaccine efficacy. Mol Immunol. (2015) 66:276–83. doi: 10.1016/j.molimm.2015.04.004
61. Ng, PC, and Henikoff, S. SIFT: predicting amino acid changes that affect protein function. Nucleic Acids Res. (2003) 31:3812–4. doi: 10.1093/nar/gkg509
62. UniProt Consortium. UniProt: a hub for protein information. Nucleic Acids Res. (2015) 43:D204–12. doi: 10.1093/nar/gku989
63. Delrue, RM, Lestrate, P, Tibor, A, Letesson, JJ, and De Bolle, X. Brucella pathogenesis, genes identified from random large-scale screens. FEMS Microbiol Lett. (2004) 231:1–12. doi: 10.1016/S0378-1097(03)00963-7
64. Vemulapalli, R, McQuiston, JR, Schurig, GG, Sriranganathan, N, Halling, SM, and Boyle, SM. Identification of an IS 711 element interrupting the wboA gene of Brucella abortus vaccine strain RB51 and a PCR assay to distinguish strain RB51 from other Brucella species and strains. Clin Diagn Lab Immunol. (1999) 6:760–4. doi: 10.1128/CDLI.6.5.760-764.1999
65. Sangari, FJ, Agüero, J, and García-Lobo, JM. The genes for erythritol catabolism are organized as an inducible operon in Brucella abortus. Microbiology. (2000) 146:487–95. doi: 10.1099/00221287-146-2-487
Keywords: vaccines, brucellosis, strains, selection, NGS, bioinformatics analysis annotation
Citation: Prasolova O, Krylova E, Bogomazova A, Soltynskaya I, Sklyarov O, Gordeeva V, Timofeeva I, Motorygin A and Panin A (2023) Russian collection of Brucella abortus vaccine strains: annotation, implementation and genomic analysis. Front. Vet. Sci. 10:1154520. doi: 10.3389/fvets.2023.1154520
Edited by:
Shengqing Yu, Chinese Academy of Agricultural Sciences, ChinaReviewed by:
Muhammad Saqib, University of Agriculture, PakistanRosa Estela Quiroz Castañeda, Instituto Nacional de Investigaciones Forestales, Agrícolas y Pecuarias (INIFAP), Mexico
Copyright © 2023 Prasolova, Krylova, Bogomazova, Soltynskaya, Sklyarov, Gordeeva, Timofeeva, Motorygin and Panin. This is an open-access article distributed under the terms of the Creative Commons Attribution License (CC BY). The use, distribution or reproduction in other forums is permitted, provided the original author(s) and the copyright owner(s) are credited and that the original publication in this journal is cited, in accordance with accepted academic practice. No use, distribution or reproduction is permitted which does not comply with these terms.
*Correspondence: Olga Prasolova, o.prasolova@vgnki.ru