- College of Animal Sciences and Technology, Huazhong Agricultural University, Wuhan, China
A well-known milk-derived bioactive tripeptide, VPP (Val-Pro-Pro) has good anti-inflammatory, anti-hypertension, and anti-hydrolysis properties. However, whether VPP can alleviate calf intestinal inflammation is unclear. In this experiment, the effects of VPP on growth, diarrhea incidence, serum biochemical indices, short-chain fatty acids, and fecal microorganisms were examined in pre-weaning Holstein calves. Eighteen calves with similar birth date, body weight, and genetic background were randomly assigned equally to two groups (n = 9). The control group was given 50 mL of phosphate buffer saline before morning feeding, whereas the VPP group received 50 mL of VPP solution (100 mg/kg body weight/d). The study lasted for 17 days, with the first 3 days used for adaptation. Initial and final body weights were determined, and daily dry matter intake and fecal score were recorded throughout the study. Serum hormone levels and antioxidant and immune indices were measured on day 14. Fecal microorganisms were collected on days 0, 7, and 14, and 16S rDNA sequencing was performed. Oral administration of VPP did not significantly affect calf average daily feed intake and body weight, but the growth rate in body weight was significantly higher in the VPP group than in the control group on day 7 (P < 0.05). Compared with the control, VPP significantly decreased serum TNF-α and IL-6 contents (P < 0.05), and concentrations of nitric oxide and IL-1β also decreased but not significantly (0.05 < P < 0.1). After seven days of VPP, relative abundances of g_Lachnoclostridium, uncultured_bacterium_, and g_Streptococcus in fecal samples increased significantly (P < 0.05). Compared with the control, VPP significantly increased concentrations of the fecal short-chain fatty acids n-butyric acid and isovaleric acid (P < 0.05). In conclusion, VPP can relieve intestinal inflammation and alleviate the degree of diarrhea in pre-weaning calves.
1. Introduction
As one of the most common diseases of young calves, diarrhea causes huge economic and productivity losses for cattle breeders (1). According to a survey released in 2018, diarrhea was responsible for 39% of calf deaths during the first 21 days of life (2). Antibiotics have long been used as a therapeutic agent for calf diarrhea, but antibiotic use in domestic animals is associated with many adverse effects, such as interference with host microorganisms, increased antibiotic resistance in hosts against antibiotic-resistant pathogens, and direct harm to the host (3). Therefore, suitable substitutes need to be identified.
A primary cause of diarrhea in newborn calves is enteropathic Escherichia coli infection. Enteropathic (E.) coli infection destroys proteins of intestinal compact junctions, releases cell-damaging toxins, damages small intestinal mucosa, and leads to mucosal inflammation, thus causing diarrhea in calves (4, 5). When pathogenic bacteria invade the intestinal tract of newborn calves, tight junction proteins are destroyed and inflammatory signaling pathways are activated, including those of mitogen-activated protein kinase, IkappaBalpha, and nuclear factor-kappaB. Pathogenic bacteria also induce production of interleukin-8 (IL-8), interleukin-6 (IL-6), and tumor necrosis factor-α (TNF-α) by small intestinal epithelial cells, which causes intestinal inflammatory response of calves (6). In addition, weaning stress may increase serum cortisol concentrations, which can be detrimental to cellular immunity and inhibit many non-specific immune responses, leading to increased disease susceptibility (7). Weaning stress increases contents of neutrophils, TNF-α, IL-6, and interleukin-1β (IL-1β) in blood of calves (8) and expression of apoptosis factors and pro-inflammatory factors including IL-1β, IL-8, interferon-γ, TNF-α, and toll like receptor 4, causing calf intestinal inflammatory response (9). However, a healthy intestinal environment of pre-weaning calves can alleviate weaning stress. Use of anti-inflammatory drugs can reduce the secretion of inflammatory factors and inhibit intestinal inflammatory response and thus alleviate the degree of diarrhea in calves (10).
The milk-derived bioactive peptide VPP (Val-Pro-Pro) has a positive anti-inflammatory effect in animal models of colitis (11). Dietary supplementation of VPP reduces expression of monocyte chemoattractant protein-1 and IL-6 and number of macrophages in inflammatory mice, as well as relieves adipose tissue inflammation through the mitogen-activated protein kinase-c-Jun N-terminal kinase pathway (12). The VPP peptide can also decrease expression of IL-1β and TNF-α, which alleviates inflammation in obese mice by activating angiotensin-converting enzyme (13). The tripeptide is highly resistant to gastric enzymes and pancreatic enzymes and rennet and is difficult to digest by digestive enzymes (14). Therefore, VPP that enters the digestive tract from the mouth can remain intact in the gut until it is absorbed. In addition, VPP can be transported in its intact form through the Caco-2 monolayer by paracellular diffusion, and the maximum concentration of VPP in animal blood can range from 10 to 100 μmol/L (15, 16). Therefore, VPP can be completely absorbed into the portal vein after ingestion and play a role in blood vessels. On the basis of previous research, it was hypothesized that VPP could relieve intestinal inflammation of calves and thus help to solve the problem of diarrhea. Therefore, an experiment was conducted with Holstein calves to determine the effects of VPP.
2. Materials and methods
2.1. Animals and management
The trial was conducted on the Linbo Cattle Farm, Jiangnan District, Nanning City, Guangxi Zhuang Autonomous Region, China, from June to July 2021. Eighteen 1-month-old Holstein calves with similar birth date, weight, and genetic background were randomly assigned equally to two groups (n = 9), with five male and four female calves in each group. Before morning feeding, 50 mL of phosphate buffer saline was given to the control (Ctrl) group and 50 mL of VPP solution (100 mg/kg body weight (BW)/d) was given to VPP group for 14 days. During the experiment, calves were housed in a single cage and fed 4 kg of milk substitute and 250 g of starter food every day, with free intake of food and water.
2.2. Data and sample collection
Electronic scales were used to record daily dry matter intake. Weights of calves were measured with a weighbridge on days 0, 7, and 14. At 2 h after morning feeding on days 10 and 14, 10 mL of blood was collected by the jugular vein. To isolate the serum, blood was centrifuged at 3,000 rpm for 10 min. The upper layer of serum was extracted with a pipetting gun and released into centrifuge tubes, which were stored at −20°C until analysis of serum biochemical indices. On days 0, 7, and 14, after stimulating the end of the rectum to cause defecation, 10 g of fresh feces was collected and packed into 5-mL sterile frozen tubes, which were then stored at −80°C.
2.3. Determination of diarrhea rate
Calves were observed daily for diarrhea, and feces scores were determined according to the four-point system. The fecal scoring criteria were according to Magalhaes et al. (17). When the feces score was ≥3, the diarrhea rate of calves was calculated according to the feces score.
2.4. Serum analyses
Bovine ELISA kits (NanJing JianCheng Bioengineering Institute, Nanjing, China) were used to determine serum biochemical indices, including activities of superoxide dismutase (SOD) and glutathione peroxidase (GSH-Px) and contents of malondialdehyde (MDA), immunoglobulin A (IgA), immunoglobulin G (IgG), IL-6, IL-8, IL-1β, endothelin 1 (ET-1), insulin-like growth factor 1 (IGF-1), TNF-α, and nitric oxide (NO).
2.5. Determination of fecal bacteria and short chain fatty acids
To analyze fecal bacterial composition and diversity, 16S rDNA amplicon sequencing was performed, with PCR amplification of bacterial 16S rDNA V3-V4 regions. After PCR products were evaluated and qualified, the library was constructed and sequenced by Beijing Baimaike Biotechnology Co., Ltd. (Beijing, China). Operational taxonomic unit (OUT) number, abundance, and Alpha diversity indices were obtained by analyzing fecal bacteria and principal coordinate analysis (PCoA) was conducted with OTU number. Referring to the detailed steps described in the literature (18), fecal short-chain fatty acids were analyzed by gas chromatography.
2.6. Statistical analyses
Data were analyzed by t-test, and figures were prepared using GraphPad Prism 9.0 (GraphPad Software, Inc., La Jolla, CA, USA). In all analyses, differences detected at P < 0.05 were considered significant.
3. Results
3.1. Effects of VPP on feed intake and body weight of pre-weaning calves
Feed intake of calves in both Ctrl and VPP groups tended to increase (Figure 1A). Growth rate in body weight was significantly higher in the VPP group than in the Ctrl group only on d 7 (Figure 1B; P < 0.05).
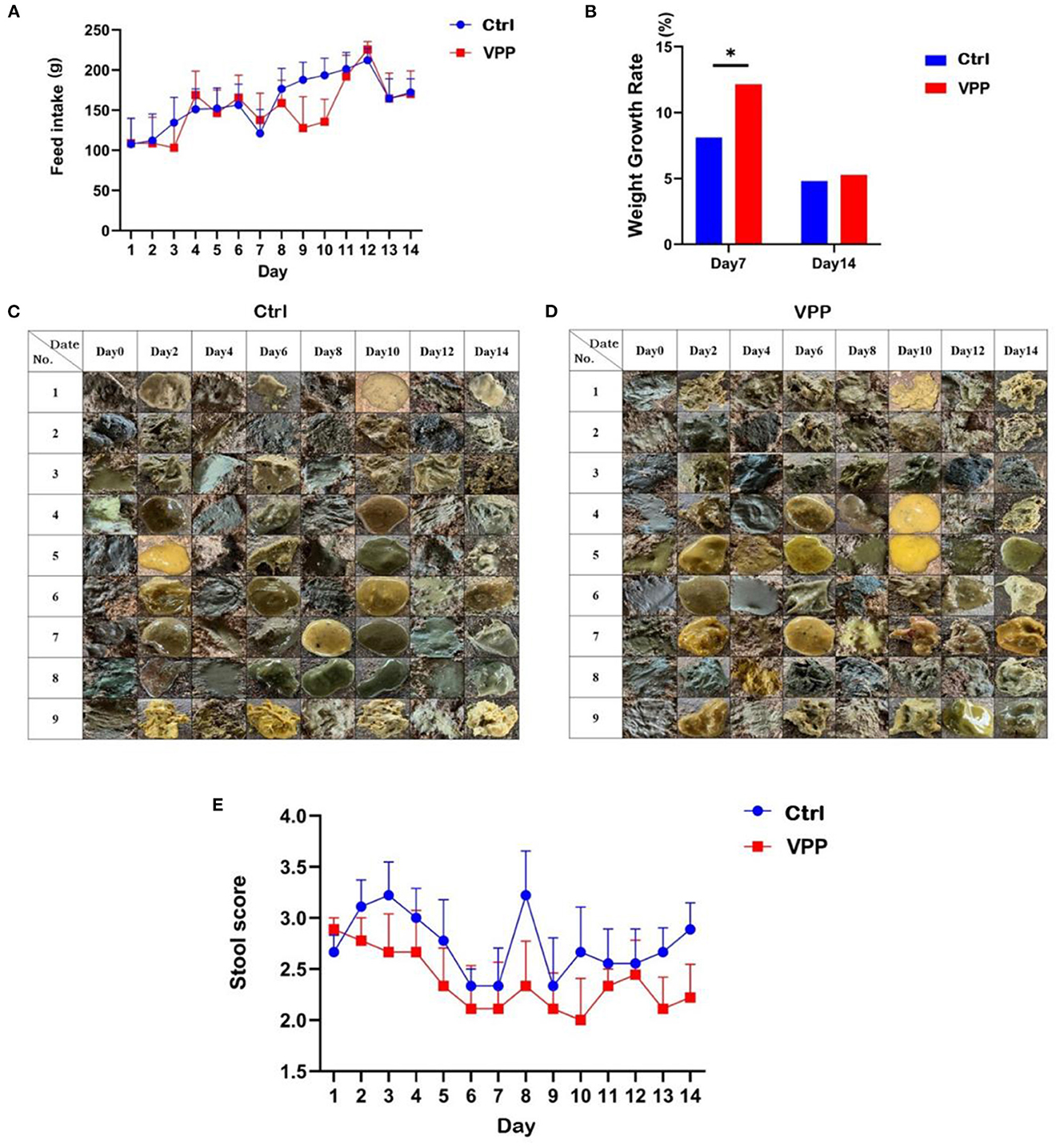
Figure 1. Effects of VPP on feed intake, body weight, and fecal score of pre-weaning calves. (A) Change in feed intake. (B) Growth rate in weight. (C) Control fecal sample. (D) VPP fecal sample. (E) Change in stool score. Values are means ± SD, n = 10. *Significant difference between group (P < 0.05).
3.2. Effects of VPP on fecal score of pre-weaning calves
The effect of VPP on the diarrhea rate of calves was not significant, and compared with the Ctrl group, the fecal score of calves in the VPP group after day 1 was lower throughout the study (Figures 1C–E).
3.3. Effects of VPP on serum biochemical indices of pre-weaning calves
Serum concentration of IGF-1 in the VPP group was not significantly different compared with that in the Ctrl group (Figure 2A), but the concentration of ET-1 decreased, although not significantly (Figure 2B; 0.05 < P < 0.1). Differences in serum concentrations of GSH-P, MDA, and SOD between VPP and Ctrl groups were not significant (Figures 2C–E), although SOD and GSH-Px concentrations were higher in the VPP group. Serum NO concentration was not significantly lower in the VPP group than in the Ctrl group (Figure 2F; P = 0.05). Levels of IL-6 and TNF-α were significantly lower in the VPP group than in the Ctrl group (Figures 2H, I; P < 0.05), and level of IL-1β was also lower in VPP but the difference was not significant (Figure 2G, 0.05 < P < 0.1). Concentrations of IgA, IgG, and IL-8 were not significantly different between groups (Figures 2J–L).
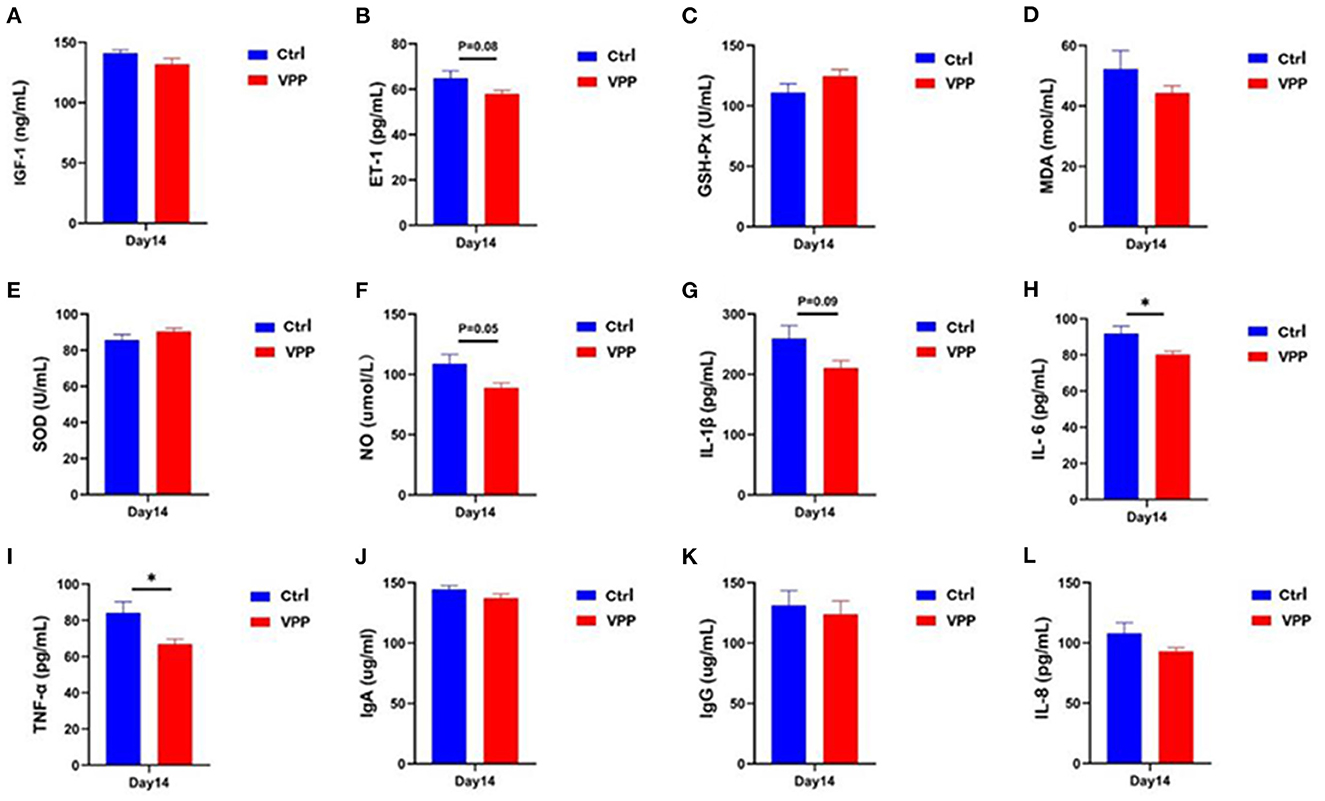
Figure 2. Effects of VPP on serum biochemical indices of pre-weaning calves. Concentrations of (A) insulin-like growth factor 1 (IGF-1), (B) endothelin 1 (ET-1), (C) glutathione peroxidase (GSH-Px), (D) malondialdehyde (MDA), (E) superoxide dismutase (SOD), (F) nitric oxide (NO), (G) interleukin-1β (IL-1β), (H) interleukin-6 (IL-6), (I) tumor necrosis factor-α (TNF-α), (J) immunoglobulin A (IgA), (K) immunoglobulin G (IgG), and (L) interleukin-8 (IL-8). Values are means ± SD, n = 10. *Significant difference between group (P < 0.05).
3.4. Effects of VPP on short-chain fatty acids in pre-weaning calves
Fecal concentrations of butyric acid, isobutyric acid, valeric acid, and isovaleric acid in the VPP group increased throughout the study (Figures 3C–F). Concentrations of isobutyric acid and isovaleric acid were significantly higher in VPP group than in the Ctrl group on day 14 (Figures 3D, F; P < 0.05). On day 7, concentrations of acetic acid and propionic acid were higher in the VPP group than in the Ctrl group, but differences were not significant (Figures 3A, B; 0.05 < P < 0.1). The total short-chain fatty acid concentration was higher in VPP group than in the Ctrl group on days 7 and 14, but there was no significant difference (Figure 3G).
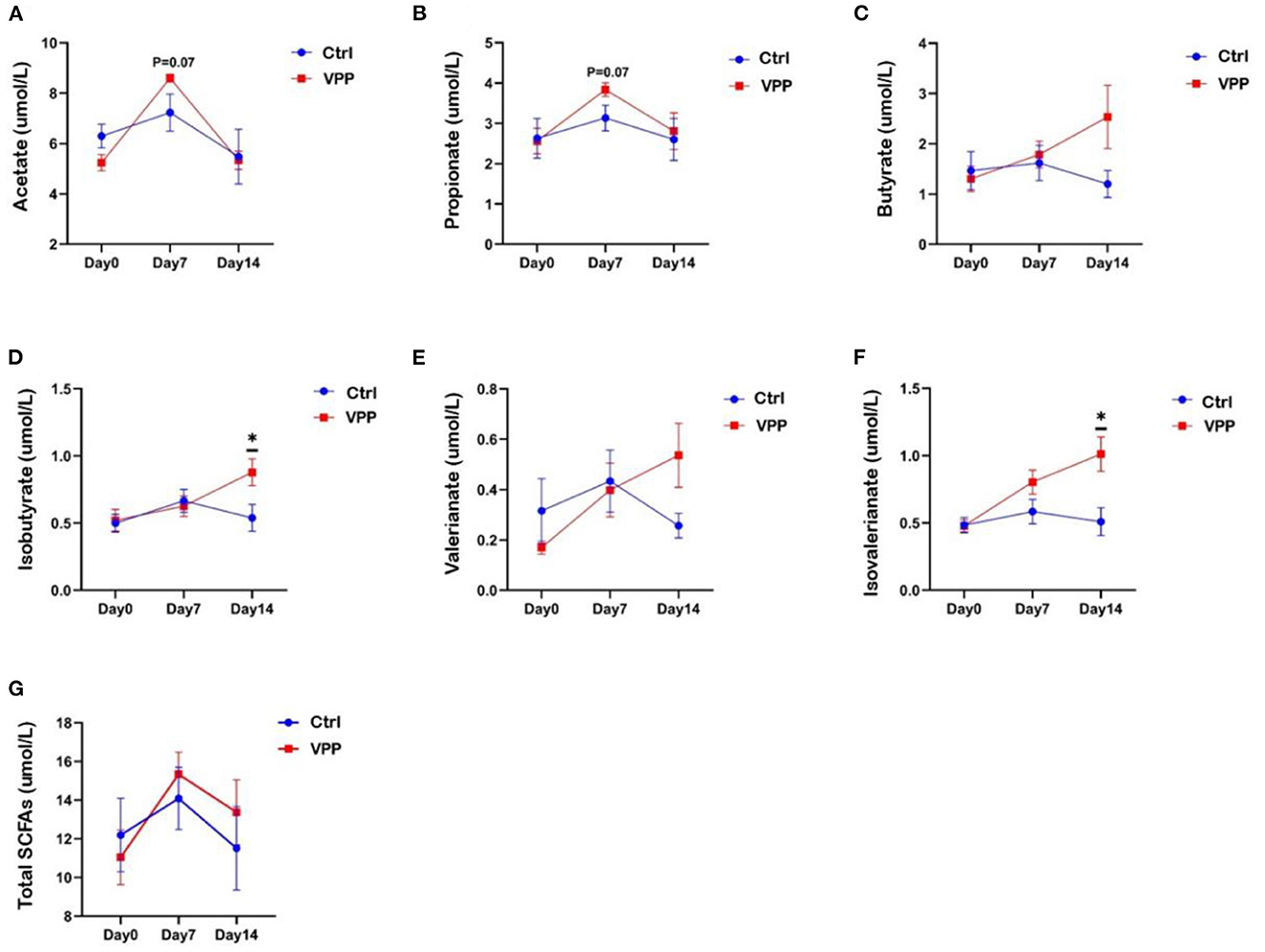
Figure 3. Effects of VPP on short-chain fatty acids in pre-weaning calves. Concentrations of (A) acetate, (B) propionate, (C) butyrate, (D) isobutyrate, (E) valerianate, (F) isovalerianate, and (G) total short-chain fatty acids (SCFAs). Values are means ± SEM, n = 10. *Significant difference between group (P < 0.05).
3.5. Effects of VPP on composition and diversity of fecal bacteria in pre-weaning calves
Composition and diversity of fecal bacteria in each group were analyzed. In Ctrl and VPP groups, there were 519 common OTUs, and there was one unique OTU in the Ctrl group (Figure 4A). In addition, PCoA clearly indicated that composition of fecal bacterial communities was similar in Ctrl and VPP groups on day 14 (Figure 4B). Alpha diversity indices of fecal bacterial communities were compared between Ctrl and VPP groups on day 14, and differences in Chao1, ACE, Simpson, and Shannon indices were not significant, indicating similar species diversity between the groups (Figures 4C–F). Distributions of bacterial taxa at phylum, genus, and species levels were also analyzed. The dominant phyla of intestinal bacteria in Ctrl and VPP groups were Firmicutes, Bacteroidetes, Proteobacteria, Actinobacteria, and Tenericutes. In both groups on all days, the combined relative abundance of Firmicutes and Bacteroidetes was higher than 90%, and the proportion of Firmicutes in the VPP group exceeded 50% on the three sample dates (Figure 4G). The dominant genera of intestinal were Faecalibacterium, Alloprevotella, and Megamonas (Figure 4H), and the dominant species were uncultured bacteria g Faecalibacterium, uncultured bacteria g Alloprevotella and uncultured bacteria g Megamonas (Figure 4I). At different times, non-significant differences were observed between the two treatment groups among the 10 most abundant groups at phylum, genus, and species levels (Figures 4G–I). The 30 most abundant species of bacteria in samples collected on day 7 were analyzed, and relative abundances of uncultured_bacterium, g_Lachnoclostridi, umuncul-tured_bacterium_ and g_Streptococcu were significantly different between Ctrl and VPP groups (Figure 4J; P < 0.05).
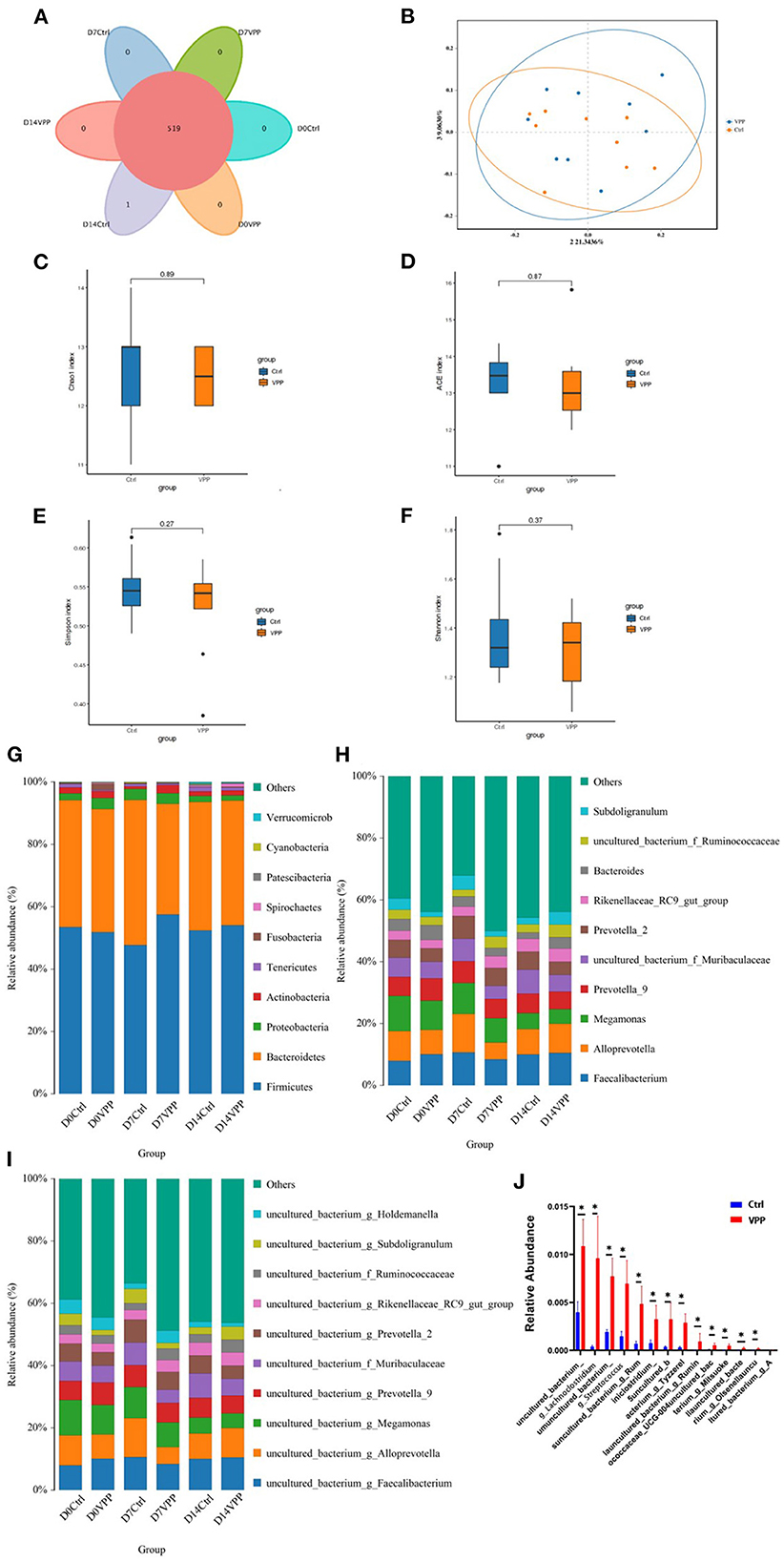
Figure 4. Effects of VPP on fecal bacteria of pre-weaning calves. (A) Venn analysis based on operational taxonomic units. (B) Principal coordinates analysis of fecal bacterial communities. Ctrl, control. Alpha diversity indices: (C) Chao1 index, (D) Ace index, (E) Simpson index, and (F) Shannon index. Composition of bacterial communities (relative abundance): (G) phyla, (H) genera, and (I) species. (J) Relative abundance of species of fecal bacteria.
4. Discussion
Relatively low diarrhea rates and high feed intake and average daily gain in pre-weaning calves indicate good growth performance. In this experiment, oral administration of VPP significantly increased the weight gain rate of calves on day 7 and alleviated the degree of diarrhea. The mechanism for the decrease in diarrhea rate might be that VPP inhibited intestinal pathogenic bacteria and alleviated intestinal inflammation of lactating calves to some extent. In addition, VPP might have entered the blood by mediation of peptide transporter 1, which would improve serum immune indices, and alleviate the intestinal inflammatory response of calves, and thus alleviate the degree of diarrhea. Similarly, intravenous injection of the dipeptide Alanyl-glutamine in calves not only reduces the diarrhea rate caused by weaning but also improves feed intake and daily gain (19). Although not directly comparable with the results of this study, the different effects on feed intake and daily gain might result from differences in the type, function, and amino acid composition of the peptides between the two studies.
Complete forms of milk-derived active peptides can be absorbed and positively affect anti-oxidative, anti-hypertensive, antibacterial, anti-inflammatory, and immunomodulatory health-related properties (20). The pro-inflammatory factors IL-1β (21), IL-6 (22), and TNF-α (23) are involved in the inflammatory response, inducing changes in intestinal epithelial permeability. The serum results of this experiment are consistent with those associated with anti-inflammatory drugs used to relieve diarrhea of calves (10) and the concentrations of IL-6 and IL-8 were also significantly reduced in the study of using dimethylglycine to relieve intestinal damage caused by heat stress (24), indicating that VPP also decreases production of inflammatory factors and alleviates the inflammatory response. In this study, VPP decreased contents of the pro-inflammatory factors IL-1β, IL-6, and TNF-α in the blood of calves, demonstrating good anti-inflammatory effects.
In the PCoA in this study, there was little separation between VPP and Ctrl fecal bacterial communities on day 14, which indicated that VPP did not significantly affect the composition of fecal bacteria in calves. Recent studies have found that patients with inflammatory bowel disease all have an intestinal microbial imbalance. For example, alpha diversity decreases, and relative abundances of pathogenic bacteria, including Gammaproteobacteria, Fusobacterium, Escherichia, Firmicutes, and Proteobacteria, increase, whereas those of other bacteria, including Bacteroides, Firmicutes, Clostridia, Ruminococcaceae, Bifidobacterium, and Lactobacillus, decrease (25, 26). In this study, alpha diversity of fecal bacteria in the VPP group did not decrease significantly compared with that in the Ctrl group, and relative abundances of Firmicutes and Proteobacteria were not affected. In addition, no significant differences in bacteria at phylum, genus, and species levels were detected between groups in fecal samples collected on day 14. In samples collected on day 7, relative abundances of uncultured_bacterium, g_Lachnoclostridium, uncultured_bacterium, g_Streptococcus, and many other species were significantly different between VPP and Ctrl groups, but the differences were not maintained at the end of the experiment. Although some bioactive peptides can restrict harmful bacteria from colonizing intestinal epithelial cells (27) and promote intestinal homeostasis and counteract pathogens (28), in this trial, VPP did not show similar efficacy, which might be due to the strong hydrolytic resistance of VPP in the intestine.
As metabolites of intestinal microbes, short-chain fatty acids are important in interactions between intestinal microflora and the immune system (29). Short-chain fatty acids function in regulating tight junction protein expression, maintaining the activity of mucosal immune cells, lowering gut pH to suppress harmful bacteria, and protecting the intestinal epithelial barrier (30, 31). Short-chain fatty acids can suppress colon inflammation by activating butyric acid receptors and thereby inducing secretion of anti-inflammatory cytokines (32) and also by inhibiting inhibit the pro-inflammatory response of intestinal macrophages (33) and reducing the inflammatory response of peripheral blood mononuclear cells (34). On day 7, levels of propionic acid and acetic acid increased in the VPP group compared with those in the Ctrl group (0.05 < P < 0.1), but on day 14, contents were the same in the two groups. In addition, on day 14, isovaleric acid and isobutyric acid concentrations were significantly higher in the VPP group than in the Ctrl group (P < 0.05). Valeric acid can inhibit the growth of Clostridium difficile and thereby regulate intestinal homeostasis (35), whereas sodium butyrate can reduce IL-6 and TNF-α levels and thereby improve colitis in mice (36). Results of both studies are consistent with those of this test. Therefore, VPP might alleviate intestinal inflammation in diarrheal calves by altering gut flora to increase relative abundances of beneficial bacteria producing n-butyric acid and isovalerate.
5. Conclusions
The tripeptide VPP alleviated the degree of diarrhea in pre-weaning calves, by reducing contents of pro-inflammatory factors in the blood and thus relieving intestinal inflammation.
Data availability statement
The original contributions presented in the study are included in the article/supplementary material, further inquiries can be directed to the corresponding author.
Ethics statement
The animal study was reviewed and approved by Animal Welfare and Ethics Committee of Huazhong Agricultural University.
Author contributions
XZ and YG prepared the original draft. YD, LY, and YG contributed to conception, supervised, data collection, conducted data analysis, and data curation. XZ performed investigation. YG designed methodology. XZ, YD, and QX reviewed and edited the manuscript. JH polished the article and improved the figures. All authors have read and agree to the published version of the manuscript.
Funding
This work was funded by the grants from the National Key R&D Program of China (Nos. 2022YFD1300705 and 2022YFD1301004) and Key Laboratory of Molecular Animal Nutrition of Zhejiang University (KLMAN202101 and KLMAN202205).
Acknowledgments
We thank the staff at the Linbo Cattle Farm for providing us with the infrastructure.
Conflict of interest
The authors declare that the research was conducted in the absence of any commercial or financial relationships that could be construed as a potential conflict of interest.
Publisher's note
All claims expressed in this article are solely those of the authors and do not necessarily represent those of their affiliated organizations, or those of the publisher, the editors and the reviewers. Any product that may be evaluated in this article, or claim that may be made by its manufacturer, is not guaranteed or endorsed by the publisher.
References
1. El-Seedy FR, Abed AH, Yanni HA, Abd El-Rahman SAA. Prevalence of Salmonella and E. coli in neonatal diarrheic calves Beni Suef Univ. J Basic Appl Sci. (2016) 5:45–51. doi: 10.1016/j.bjbas.2015.11.010
2. Kim HS, Whon TW, Sung H, Jeong YS, Jung ES, Shin NR, et al. Longitudinal evaluation of fecal microbiota transplantation for ameliorating calf diarrhea and improving growth performance. Nat Commun. (2021) 12:161. doi: 10.1038/s41467-020-20389-5
3. Ji S, Jiang T, Yan H, Guo C, Liu J, Su H, et al. Ecological restoration of antibiotic-disturbed gastrointestinal microbiota in foregut and hindgut of cows. Front Cell Infect Microbiol. (2018) 8:79. doi: 10.3389/fcimb.2018.00079
4. Foster DM, Smith GW. Pathophysiology of diarrhea in calves. Vet Clin North Am Food Anim Pract. (2009) 25:13–36. doi: 10.1016/j.cvfa.2008.10.013
5. Shang T, Zhang Y, Lin Q, Zhu L. Isolation and identification of the pathogen of Colibacillosis in calves. Prog Vet Med. (2012) 33:143–6. doi: 10.16437/j.cnki.1007-5038.2012.06.036
6. Savkovic SD, Koutsouris A, Hecht G. Activation of NF-k B in intestinal epithelial cells by enteropathogenic Escherichia coli. Am J Physiol. (1997) 273:C1160–7. doi: 10.1152/ajpcell.1997.273.4.C1160
7. Carroll JA, Arthington JD, Chase CC Jr. Early weaning alters the acute-phase reaction to an endotoxin challenge in beef calves. J Anim Sci. (2009) 87:4167–72. doi: 10.2527/jas.2009-2016
8. Kim MH, Yun CH, Lee CH, Ha JK. The effects of fermented soybean meal on immunophysiological and stress-related parameters in Holstein calves after weaning. J Dairy Sci. (2012) 95:5203–12. doi: 10.3168/jds.2012-5317
9. O'Loughlin A, McGee M, Waters SM, Doyle S, Earley B. Examination of the bovine leukocyte environment using immunogenetic biomarkers to assess immunocompetence following exposure to weaning stress. Bmc Vet Res. (2011) 7:45. doi: 10.1186/1746-6148-7-45
10. Todd CG, Millman ST, McKnight DR, Duffield TF, Leslie KE. Nonsteroidal anti-inflammatory drug therapy for neonatal calf diarrhea complex: effects on calf performance. J Anim Sci. (2010) 88:2019–28. doi: 10.2527/jas.2009-2340
11. Chatterton DE, Nguyen DN, Bering SB, Sangild PT. Anti-inflammatory mechanisms of bioactive milk proteins in the intestine of newborns. Int J Biochem Cell Biol. (2013) 45:1730–47. doi: 10.1016/j.biocel.2013.04.028
12. Aihara K, Osaka M, Yoshida M. Oral administration of the milk casein-derived tripeptide Val-Pro-Pro attenuates high-fat diet-induced adipose tissue inflammation in mice. Br J Nutr. (2014) 112:513–9. doi: 10.1017/S0007114514001147
13. Sawada Y, Sakamoto Y, Toh M, Ohara N, Hatanaka Y, Naka A, et al. Milk-derived peptide Val-Pro-Pro (VPP) inhibits obesity-induced adipose inflammation via an angiotensin-converting enzyme (ACE) dependent cascade. Mol Nutr Food Res. (2015) 59:2502–10. doi: 10.1002/mnfr.201500324
14. Ohsawa K, Satsu H, Ohki K, Enjoh M, Takano T, Shimizu M. Producibility and digestibility of antihypertensive β-casein tripeptides, val-pro-pro and ile-pro-pro, in the gastrointestinal tract: analyses using an in vitro model of mammalian gastrointestinal digestion. J Agr Food Chem. (2008) 56:854–8. doi: 10.1021/jf072671n
15. Satake M, Enjoh M, Nakamura Y, Takano T, Kawamura Y, Arai S, et al. Transepithelial transport of the bioactive tripeptide, val-pro-pro, in human intestinal caco-2 cell monolayers. Biosci Biotechnol Biochem. (2002) 66:378–84. doi: 10.1271/bbb.66.378
16. Meyer J, Butikofer U, Walther B, Wechsler D, Sieber R. Hot topic: Changes in angiotensin-converting enzyme inhibition and concentrations of the tripeptides Val-Pro-Pro and Ile-Pro-Pro during ripening of different Swiss cheese varieties. J Dairy Sci. (2009) 92:826–36. doi: 10.3168/jds.2008-1531
17. Magalhaes VJ, Susca F, Lima FS, Branco AF, Yoon I, Santos JE. Effect of feeding yeast culture on performance, health, and immunocompetence of dairy calves. J Dairy Sci. (2008) 91:1497–509. doi: 10.3168/jds.2007-0582
18. Yan Y, Xu B, Yin B, Xu X, Niu Y, Tang Y, et al. Modulation of gut microbial community and metabolism by dietary glycyl-glutamine supplementation may favor weaning transition in piglets. Front Microbiol. (2019) 10:3125. doi: 10.3389/fmicb.2019.03125
19. Zhou Y, Zhang P, Deng G, Liu X, Lu D. Improvements of immune status, intestinal integrity and gain performance in the early-weaned calves parenterally supplemented with L-alanyl-L-glutamine dipeptide. Vet Immunol Immunopathol. (2012) 145:134–42. doi: 10.1016/j.vetimm.2011.10.020
20. Jia L, Wang L, Liu C, Liang Y, Lin Q. Bioactive peptides from foods: production, function, and application. Food Funct. (2021) 12:7108–25. doi: 10.1039/D1FO01265G
21. Migliorini P, Italiani P, Pratesi F, Puxeddu I, Boraschi D. The IL-1 family cytokines and receptors in autoimmune diseases. Autoimmun Rev. (2020) 19:102617. doi: 10.1016/j.autrev.2020.102617
22. Tanaka T, Narazaki M, Kishimoto T. IL-6 in inflammation, immunity, and disease. Cold Spring Harb Perspect Biol. (2014) 6:a016295. doi: 10.1101/cshperspect.a016295
23. Li J, ZHHW. TNF-α Inhibitors with Anti-Oxidative Stress Activity from Natural Products. Curr Top Med Chem. (2012) 12:1408–21. doi: 10.2174/156802612801784434
24. Wang Z, Shao D, Wu S, Song Z, Shi S. Heat stress-induced intestinal barrier damage and dimethylglycine alleviates via improving the metabolism function of microbiota gut brain axis. Ecotoxicol Environ Saf. (2022) 244:114053. doi: 10.1016/j.ecoenv.2022.114053
25. Kostic AD, Xavier RJ, Gevers D. The microbiome in inflammatory bowel disease: current status and the future ahead. Gastroenterology. (2014) 146:1489–99. doi: 10.1053/j.gastro.2014.02.009
26. Mancabelli L, Milani C, Lugli GA, Turroni F, Cocconi D, van Sinderen D, et al. Identification of universal gut microbial biomarkers of common human intestinal diseases by meta-analysis. FEMS Microbiol Ecol. (2017) 93:153. doi: 10.1093/femsec/fix153
27. Sun X, Ganzle M, Wu J. Identification and characterization of glycopeptides from egg protein ovomucin with anti-agglutinating activity against porcine k88 enterotoxigenic escherichia coli strains. J Agric Food Chem. (2017) 65:777–83. doi: 10.1021/acs.jafc.6b04299
28. Napoli MD, Luccia BD, Vitiello G, D'Errico G, Carpentieri A, Pezzella A, et al. Characterisation of EFV12 a bio-active small peptide produced by the human intestinal isolate Lactobacillus gasseri SF1109. Benef Microbes. (2020) 11:815–24. doi: 10.3920/BM2020.0124
29. Arpaia N, Rudensky AY. Microbial metabolites control gut inflammatory responses. Proc Natl Acad Sci U S A. (2014) 111:2058–9. doi: 10.1073/pnas.1323183111
30. Liu P, Wang Y, Yang G, Zhang Q, Meng L, Xin Y, et al. The role of short-chain fatty acids in intestinal barrier function, inflammation, oxidative stress, and colonic carcinogenesis. Pharmacol Res. (2021) 165:105420. doi: 10.1016/j.phrs.2021.105420
31. Zhang Q, Zhang S, Wu S, Madsen MH, Shi S. Supplementing the early diet of broilers with soy protein concentrate can improve intestinal development and enhance short-chain fatty acid-producing microbes and short-chain fatty acids, especially butyric acid. J Anim Sci Biotechnol. (2022) 13:97. doi: 10.1186/s40104-022-00749-5
32. Singh N, Gurav A, Sivaprakasam S, Brady E, Padia R, Shi H, et al. Activation of Gpr109a, receptor for niacin and the commensal metabolite butyrate, suppresses colonic inflammation and carcinogenesis. Immunity. (2014) 40:128–39. doi: 10.1016/j.immuni.2013.12.007
33. Chang PV, Hao L, Offermanns S, Medzhitov R. The microbial metabolite butyrate regulates intestinal macrophage function via histone deacetylase inhibition. Proc Natl Acad Sci U S A. (2014) 111:2247–52. doi: 10.1073/pnas.1322269111
34. Wenzel TJ, Gates EJ, Ranger AL, Klegeris A. Short-chain fatty acids (SCFAs) alone or in combination regulate select immune functions of microglia-like cells. Mol Cell Neurosci. (2020) 105:103493. doi: 10.1016/j.mcn.2020.103493
35. McDonald JAK, Mullish BH, Pechlivanis A, Liu Z, Brignardello J, Kao D, et al. Inhibiting growth of clostridioides difficile by restoring valerate, produced by the intestinal microbiota. Gastroenterology. (2018) 155:1495–507. doi: 10.1053/j.gastro.2018.07.014
Keywords: VPP, gut inflammation, calf, diarrhea, gut microbiome
Citation: Zong X, Gao Y, Du Y, Hou J, Yang L and Xu Q (2023) Effects of milk-derived bioactive peptide VPP on diarrhea of pre-weaning calves. Front. Vet. Sci. 10:1154197. doi: 10.3389/fvets.2023.1154197
Received: 30 January 2023; Accepted: 14 March 2023;
Published: 29 March 2023.
Edited by:
Shourong Shi, Poultry Institute, Chinese Academy of Agricultural Sciences, ChinaReviewed by:
Jiashun Chen, Hunan Agricultural University, ChinaAiwei Guo, Southwest Forestry University, China
Copyright © 2023 Zong, Gao, Du, Hou, Yang and Xu. This is an open-access article distributed under the terms of the Creative Commons Attribution License (CC BY). The use, distribution or reproduction in other forums is permitted, provided the original author(s) and the copyright owner(s) are credited and that the original publication in this journal is cited, in accordance with accepted academic practice. No use, distribution or reproduction is permitted which does not comply with these terms.
*Correspondence: Qingbiao Xu, qbxu@mail.hzau.edu.cn
†These authors have contributed equally to this work