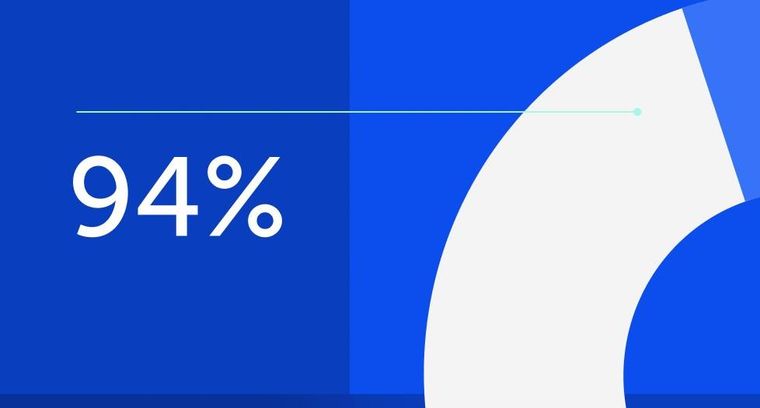
94% of researchers rate our articles as excellent or good
Learn more about the work of our research integrity team to safeguard the quality of each article we publish.
Find out more
ORIGINAL RESEARCH article
Front. Vet. Sci., 18 May 2023
Sec. Veterinary Epidemiology and Economics
Volume 10 - 2023 | https://doi.org/10.3389/fvets.2023.1152246
Introduction: The association between antibiotic use and the occurrence of resistant bacteria is a global health problem and is subject to enormous efforts at national and international levels. Within the scope of the study “KAbMon”, the resistance situation as well as the use of antibiotics in calf rearing farms in Germany was investigated. We hypothesized that the levels of resistance are associated with certain calf keeping farm types, such as pre-weaned calf farms, animal groups, and therapy frequency.
Methods: In total, 95 calf keeping farms were visited between October 2019 and April 2021. At each farm, up to three pooled fecal samples (10 freshly released feces each) were collected. One sample was taken in the youngest calf group, another in the oldest calf group, and one in the hospital box, if available. Escherichia coli was isolated from non-selective MacConkey agar. The therapy frequency reflects the average number of treatment days per calf in a half-year, while the resistance score is the sum of the relative minimum inhibitory concentration per substance over all 10 tested substances.
Results: The 1781 isolates from 178 samples showed high resistance rates against sulfamethoxazole (82%), tetracycline (49%), and ampicillin (40%). High resistance scores were mainly found in pre-weaned calf farms (purchasing calves from 2 weeks of life) and in the youngest animals. The therapy frequency showed an almost linear relationship with the resistance scores, and the age at purchase was negatively related to the resistance score.
Discussion: The high use of antimicrobials of young calves might be associated with a high risk for infectious diseases and might indicate that the current system of crowding 14-day-old calves from different farms in one group is not optimal. Further efforts are necessary to educate and motivate the calf keepers to ensure highest levels of hygiene and management as well as animal welfare conditions and to increase animal health.
The association between antibiotic use and the occurrence of resistant bacteria is a global health problem and is subject to enormous efforts at the national and international levels (1–3). Farm animal husbandry and the veterinary sector are regarded as some of the main drivers, and thus, are supposed to reduce its antibiotic use (4, 5). In Germany, national antibiotic monitoring, including the concept of minimizing antibiotic use in farm animals, has been in force since 2014 (6). Animal keepers for fattening purposes have to report their antibiotic use to a national database twice per year (6). Therapy frequency (TF) is calculated for each farm, and farms above certain thresholds (median and 3rd quartile) need to take action to increase animal health and reduce TF (6).
All fattening calves are compiled within the group “fattening calves up to 8 months of age,” although there are huge differences in the need for antibiotic treatment between different farm types, e.g., dairy farms and calf rearing farms (6). Since the beginning of the monitoring program, the median TF for calves was 0, meaning that at least half of the farms reported no antibiotic use in their calves. However, the third quartile fluctuates around 2, in contrast to fattening bulls older than 8 months, where the third quartile is 0 (7). In Germany, there are about 53,000 dairy farms in which all newborn calves are raised at least up to 14 days of life (8). After 14 days, calves were raised as future dairy cows or sold to specialized calf-rearing farms (Figure 1). Pre-weaned calf farms assemble 14-day-old calves from dairy farms and raise them until slaughter (9, 10). Approximately 300 farms are members of the German veal meat group (11). In addition, there are farms specialized for fattening calves that are slaughtered as cattle at the age of 18 months. It is well known that pre-weaned calves after transport to the rearing farms are of high risk for infectious diseases and therefore, often need antibiotic treatment (12). Thus, the health risk situation between calves in dairy farms and those in rearing farms is quite different, and the antibiotic use between these types of calf husbandry cannot be compared fairly. Therefore, in the future, the group “fattening calves up to 8 months of age” should be split into several groups to ensure comparability (13).
Figure 1. Structure of calf raising systems in Germany. Red, dairy or suckler cow farms (closed farming systems); green, farms raising pre-weaned calves; blue, farms raising weaned calves.
The resistance situation in calf-keeping farms and in contaminated foods derived from calves is alarming. In a report on zoonoses monitoring by the Federal Office of Consumer Protection and Food Safety (BVL), a prevalence of extended-spectrum beta-lactamase and plasmid-mediated AmpC beta-lactamase (ESBL-/pAmpC-) producing Escherichia coli (E. coli) of 70.8% was found in 407 cecum samples from calves in slaughterhouses, and 3.4% of investigated fresh meat samples from retail were contaminated with ESBL-/AmpC-producing E. coli (14).
E. coli is a ubiquitous bacterium some strains of which are pathogenic, others commensal. They are used as indicator bacteria for the assessment of the resistance situation, because they are part of the healthy gut flora and are distributed easily to other animals, animal species and even humans.
The research project “KAbMon” (monitoring of antibiotics use in calves in different rearing types in Germany) monitored antimicrobial usage in different groups of calf keeping farms. The German Federal Ministry for Nutrition and Agriculture funded this study (support code 2818HS014). Within the scope of the study “KAbMon,” among other things, the resistance situation as well as the use of antibiotics in calf rearing farms in Germany was investigated. We hypothesized that the levels of resistance are associated with certain farm types, such as pre-weaned calf farms, animal groups, and TF.
The study farms were stratified for three different farm types (Table 1) as well as for the regions of North Germany (Lower Saxony, North Rhine-Westphalia, and Schleswig-Holstein), East Germany (Brandenburg, Mecklenburg–West Pomerania, and Thuringia), and South Germany (Bavaria). The sample size calculation was based on the hypothesis that there were significant differences between farm types in the percentage of isolates with multiple resistance. Assuming a difference between the three farm types of 20% (50% vs. 70%), a power of 80%, and alpha of 5% requires a group size of 19 farms per group (15). To account for further risk factors, such as region and breed, and multiple comparisons between farm types, we added 4 × 15% = 60%, i.e., 31 farms per farm type (16). Based on the different number of farms per farm type and region, the distribution of the farms was as presented in Table 1.
Farms were recruited by direct contact of their veterinarian for pre-weaned and weaned calf farms. Dairy farms were recruited by announcements at farmers’ assemblies. In total, 95 calf keeping farms were visited between October 2019 and April 2021 (Table 1). At each farm, up to three pooled fecal samples (each pooled sample was composed of 10 freshly released feces) were collected. One of the pooled samples was taken from the youngest calf and the other from the oldest calf group. If available, a third pooled sample was collected from the hospital boxes. The actual age of the calves was unknown and is described in the results part.
To record the use of antimicrobial substances, drug application and dispensing records since January 1, 2018 were either transmitted electronically from the associated veterinary practice (written consent by farmers) or scanned and digitized manually. The treatment frequencies were calculated for half-years from January 2018 to February 2020 according to the common standard (6) (Table 2). For the analysis, the therapy frequency of the farm visit’s half-year was used, i.e., “current therapy frequency.”
After microbiological processing of the pooled fecal samples on non-selective MacConkey agar No. 3 (Fisher Scientific GmbH, Schwerte, Germany), 10 randomly selected isolates of E. coli per sample were confirmed using matrix-assisted laser desorption time of flight (MALDI-TOF Microflex® LT and Biotyper database®; Bruker Daltonics, Bremen, Germany), and antimicrobial susceptibility was tested with minimum inhibitory concentration (MIC) using the EUVSEC format according to BVL zoonoses monitoring with controls according to EUCAST. MIC values were categorized using epidemiological cutoff values following 2013/652/EU. If no cutoff values were available, the respective EFSA recommendations were applied.
The following substances were tested: ampicillin (AMP), azithromycin (AZM), ceftazidime (CAZ), ciprofloxacin (CIP), chloramphenicol (CMP), colistin (COL), cefotaxime (CTX), gentamicin (GEN), meropenem (MER), nalidixic acid (NAL), sulfamethoxazole (SMO), tetracycline (TET), tigecycline (TGC), and trimethoprim (TRP).
In addition, the minimum inhibitory concentration (MIC) that inhibited growth of 50% (MIC50) or of 90% (MIC90) of the isolates were calculated.
The antimicrobial resistance (AMR) score was calculated as follows (17). For each substance, the number of tested concentrations including the categories “below lowest tested concentration” and “above highest tested concentration” was ranked. For example, ampicillin was tested at seven different concentrations: (<1) – 1 – 2 – 4 – 8 – 16 – 32 – 64 – (>64) μg/mL. This resulted in nine possible results, with <1 = range 0 and >64 = range 8. Isolates <1 μg/mL were given 0/8 = 0, and isolates >64 μg/mL were given 8/8 = 1. These values were summed for all substances per isolate.
Meropenem and tigecycline were not considered because all isolates were below the lowest tested concentrations. Ceftazidim was also not considered because of its close relation to cefotaxime; nalidixic acid was excluded for the same reason (related to ciprofloxacin). Thus, only 10 substances were included; the minimum AMR score was 0, and the maximum was 10.
Data were analyzed using R version 4.2.1 (18) and RStudio version 2022.07.1 (19). Descriptive analyzes were carried out using the R packages tidyverse (20), readxl (21), and janitor (22). Generalized additive mixed models were fitted using lme4 (23) and gamm4 (24), allowing polynomial fitting of the relationship between the current therapy frequency and AMR score, as well as between the median age at purchase and AMR score. For this analysis, the AMR score was used at the sample level, that is, the mean AMR score for all isolates per sample.
A total of 178 fecal samples were collected from 95 farms (Table 1). Thereof, 82 samples were derived from the youngest animals, 78 from the oldest animals, and only 18 from the hospital box (17 farms, one dairy farm, 12 pre-weaned calf farms, and 4 weaned calf farms). Sixty-seven farms were located in the Northern region (118 samples), 11 in the Eastern region (24 samples), and 17 in the Southern region (36 samples). All farms that raised weaned calves were located in the Northern region, and pre-weaned calf farms were underrepresented in the Eastern region (Table 3), but the distribution of animal groups between the farm types and between the regions was almost even.
In pre-weaned calf farms, the median age of purchase of the youngest animals was 36 days (interquartile range: 20–41.5), and the oldest animals were on average 208 days old (median, 145–528). In weaned calf farms, the animals were purchased at a median of 149 days (115–174 days) and disposed at a median age of 548.5 days (255–617 days). Only animals up to 243 days of life were considered in the study because older animals do not fall into the category “calves up to 8 months.” Since in dairy farms, the calves were born in that farm and often were not removed from the farm before the age of 8 months, neither age at purchase nor at disposal can be described.
In total, 1781 isolates were investigated for antimicrobial susceptibility (farm types: 440 from dairy farms, 901 from pre-weaned calves, 440 from weaned calf farms; age groups: 820 from the youngest age group, 781 from the oldest age group, and 180 from the hospital box). The distribution of MIC values for each substance and isolate is shown in Table 4. Regarding SMO, more than 50% of the isolates had MIC >1,024, i.e., the highest tested concentration. MIC 50 values below the lowest tested concentrations were found for CAZ, CMP, COL, CTX, GEN, MER, NAL, and TGC.
The results of the qualitative assessment of resistance data are presented in Table 5. SMO showed the highest percentage of resistant isolates (82%), followed by TET (49%), AMP (40%), and TRP (34%). 68 (3.8%) of the isolates were resistant to at least one of the cephalosporins, CTX, and CAZ. Resistance rate against COL was 2.1%, whereas more isolates showed resistance to CIP (16%).
For the following analyzes, the results of the substances CAZ, MER, TGC, and NAL were excluded because they were either all negative (MER, TGC), or were correlated to other tested substances (CAZ to CTX, NAL to CIP). Moreover, 249 (14%) of the isolates were susceptible, 603 (34%) were resistant to one substance, 144 (8%) against two substances, and the remaining 44% of isolates showed resistance to three or more substances, thereof 90 to eight or nine (extensive drug resistant), but none to all 10 substances (pan drug resistant). SMO, TET, and AMP occurred in most of the combinations. Fifty-three isolates (3.0%) were phenotypically ESBLs (resistant to AMP, CAZ, and CTX).
The AMR scores ranged overall from 0.25 to 8.75. Thus, no sample reached a minimum of 0 or maximum of 10.0. The distribution varied between farm types, with higher values in pre-weaned calf farms (Figure 2).
Figure 2. Boxplots of AMR score per isolate in different farm types (n = 1781). The box represents the interquartile range (central 50% of the data), the solid vertical line represents the median, and ◊ represents the mean. Dots are >1.5 standard deviations from the mean.
In dairy and pre-weaned calf farms, the average AMR score was higher in the youngest animals (median 3.9) than in the oldest ones (median 2.0; Figure 3). This effect could not be observed in the weaned calf farms, since the median AMR values in each group were approximately 2.0. However, on weaned farms, the youngest group was older than the youngest group on dairy and pre-weaned calf farms. The range of AMR scores in the youngest pre-weaned calves was very broad, indicating large differences between the farms. Although the median was much smaller in the oldest animals, the range remained wide. On dairy farms, the oldest animals had a median AMR score below 2, and the range was rather small, although outliers ranged up to 7.5 (Figure 3).
Figure 3. Boxplots of AMR score in the youngest and the oldest animal groups of dairy farms [n = 430 isolates (230 youngest, 200 oldest)], pre-weaned calf farms [n = 781 isolates (400 youngest, 381 oldest)], and weaned calf farms [n = 390 isolates (190 youngest, 200 oldest)]. The box represents the interquartile range (central 50% of the data), the solid vertical line represents the median, and ◊ represents the mean. Dots are >1.5 standard deviations from the mean.
Neither in weaned calf farms (median 2.0 in all groups) nor in the pre-weaned calf farms (median 3.2 compared to 4.5 and 2.5 in the animal group), a trend toward higher AMR scores in the hospital boxes could be observed (Figure 4). Since the 10 isolates from hospital boxes in the dairy farms originated from the same farm, this result is not displayed here.
Figure 4. Boxplots of AMR score in the hospital boxes [n = 170 isolates (120 pre-weaned calf, 50 weaned calf farms)]. The box represents the interquartile range (central 50% of the data), the solid vertical line represents the median, and ◊ represents the mean. Dots are values >1.5 standard deviations from the mean.
TF ranged from 0 to 87 (median 11.6) and was lowest in dairy farms (median 1.0) and highest in pre-weaned calf farms (26.5, weaned calf farms 6.5). The association between TF and AMR score was positive and more or less linear in all farm types as well as in the totality of data (Figure 5).
Figure 5. Association between the AMR score and the current therapy frequency: all farms, dairy farms, pre-weaned calf farms, weaned calf farms; n = 178 samples. Displayed is the estimate (blue line) and the 95% confidence intervals (dark gray area).
The association between age at purchase and AMR score was investigated separately for pre-weaned and weaned calf farms (Figure 6). Between 15 and 25 days of life, the AMR score increased but then slightly decreased.
Figure 6. Association between AMR score and median age at purchase in days in farms with pre-weaned calves and weaned calves (n = 134 samples). Displayed is the estimate (blue line) and the 95% confidence intervals (dark gray area).
Our investigation showed that the use of antibiotics and the occurrence as well as rates of phenotypical resistance of E. coli in calf rearing sites were related. This finding correlates with the results of many other studies, not specifically in calf rearing farms, but in general animal husbandry as well as in human medicine (1, 3).
The high resistance rate against SMO is due to its use as a coccidiostat rather than its antimicrobial effect (25). This is in accordance with the EU Summary Report 2021 (3). SMO, TRP, and TET, the substances with the highest resistance rates, are used against enteritis and respiratory infections and can be applied via feed, which makes them a suitable group treatment. GEN is less commonly used because it can only be applied parenterally. AZM, CAZ, CIP, CTX, NAL, and TGC (only humans) are registered mainly for respiratory diseases, while those that can be administered orally are used for group treatments (AZM, CIP, and TGC). COL and AMP have only been registered for the treatment of enteric infections. The resistance rate to AMP was quite high, but that to COL was rather low. CMP has been banned for use in food-producing animals since 1994 (26). It is still routinely tested because the fluorinated derivative florfenicol is widely used against infections of the respiratory tract and can be applied as a long-acting preparation (27). The assessment of the substances used will be discussed elsewhere (28).
All resistance rates were slightly higher in our study compared to the German zoonosis monitoring in 2019 (14), with larger differences concerning GEN (9.7% vs. 2.8%) and CMP (24.3% vs. 10.1%), CTX (3.2 vs. 2.3%), CAZ (3.2 vs. 1.8%), NAL (10.3 vs. 5.5%), CIP (15.5 vs. 12.9%), AMP (39.7 vs. 33.6%), COL (2.1 vs. 0.5%), SMO (82.0% vs. 27.6%), TRP (34.3 vs. 27.2%), TET (49.0 vs. 37.3%), and AZM (5.4 vs. 2.8%). The differences might be due to the sampling material, because for zoonosis monitoring, cecal content from 217 slaughtered animals was investigated, while our study included 1,781 isolates from 178 pooled fecal samples. The investigation of 10 isolates per sample might have detected more levels of resistance than the single isolate per sample that was taken for zoonosis monitoring. The main objective of the zoonosis monitoring is to observe trends over time. Thus, the sample size per animal species and material is not high enough for a proper prevalence estimation.
Compared to the most recent studies on the national zoonosis monitoring, the percentage of completely susceptible isolates was lower in our study than in the recent zoonosis monitoring 13 a (pre-weaned calves), 18 (weaned calves), and 17% (dairy calves) vs. 42 (pre-weaned calves), 46 (weaned calves), and 78% (dairy calves) (29). On the other hand, 23% (95% confidence interval (CI) 13–29%; dairy calves), 42% (95%CI 33–51%, pre-weaned calves), and 36% (95% CI 32–41%, weaned calves) of the isolates in the resistance monitoring showed resistance to three or more substances, but 36% (95% CI 32–41%, dairy calves), 48% (95% CI 45–51%, pre-weaned calves), and 43% (95% CI 38–47%, weaned calves) of the isolates in our study. The 95% CI indicates substantial differences only for dairy farms, where the structures differ widely between farms, and many possible influencing factors, such as detailed husbandry conditions and the presence of other animals, were not investigated in the zoonosis monitoring.
According to previous studies (30–35), the highest AMR scores were observed in pre-weaned calf farms. This is not surprising because this animal group is at the highest risk of infectious diseases in general (33). Calves at the age of 14 days are transported and housed together in groups with calves from other farms, resulting in high infectious pressure. The calf’s immune system is not yet competent, but maternal antibodies from the colostrum are decreasing. Moreover, calves come from many different farms and are exposed to unknown pathogens against which neither maternal nor own antibodies are efficient. This, in combination with transport stress, increases the risk of infection. Calves that are already on the farm for a few weeks become immunocompetent, have adapted to the stable bacterial flora, and have less risk of being infected (36). Thus, the difference between the youngest and oldest animals was most significant in the pre-weaned calf farms, less in the dairy farms, and negligible in the weaned calf farms.
One weakness of the study was that the number of participating farms was different between the farm types; thus, within-group analyzes were not possible. It must also be considered that the actual age of the “youngest” and the “oldest” group differed between the farm types and even within the farm types so that general comparisons cannot be drawn. The effect of age should be assessed by the age at purchase, whereas the effect of the groups must be regarded in terms of infection pressure, as described above.
The analysis of the results from the hospital boxes was biased because the number of animals from which the collective fecal samples were derived varied and sometimes only included one animal. Because the diseases of the animals in the hospital box were not recorded, the heterogeneity of the health status in these hospital boxes might be large and cannot be assessed. This might explain the lack of elevated levels of resistance among the animals in the hospital boxes, as shown in other studies when diseased animals were treated with antibiotic substances (37, 38).
The almost linear relationship between TF and resistance score proves the high association between both parameters and reflects that every use of antibiotics promotes the selection of resistant bacterial strains (30–33, 39–43). Any approach to reduce the resistance pressure in calf-keeping farms must address the need for antibiotic therapy. Thus, it is of utmost importance to improve animal health by reducing the risk of infectious diseases, especially in the period of the immunologic gap between maternal antibodies and their own immunocompetence. In Germany, a new regulation of animal transport came into force in 2022, which allows the transport of calves only from 28 days of life (44). The association between antibiotic use and median age at purchase is well known (31, 36) and is also explained by the high infection pressure and low immunocompetence of younger calves.
In conclusion, the regular use of antimicrobials in calves is associated with the release of AMR into the environment and to humans, and thus, is related to health. Further efforts are necessary to educate and motivate calf keepers to ensure the highest levels of hygiene and management, as well as animal welfare conditions, and to improve animal health (45). Some promising approaches are already published (32, 44–47).
The raw data supporting the conclusions of this article will be made available by the authors, without undue reservation.
RM: conceived and designed the analysis, performed the analysis, and wrote the manuscript. SW: conceived and designed the analysis, collected the data, and performed parts of the analysis. LG: conceived and designed the analysis and collected the data. JB: contributed to the analysis. CR: conceived and designed the analysis and contributed to the analysis AF: conceived and designed the analysis and contributed to the analysis. KJ: performed parts of the analysis. All authors contributed to the article and approved the submitted version.
This study was funded by the German Federal Ministry of Nutrition and Agriculture (grant number 2818HS014).
The authors gratefully acknowledge Uwe Rösler and Kerstin-Elisabeth Müller (Freie Universität Berlin) for their valuable input in the study design and interpretation of the results. They also thank Stefan Lüllmann for actively supporting the study and providing contact with calf-keeping farms. The authors thank the animal owners who participated in the study.
The authors declare that the research was conducted in the absence of any commercial or financial relationships that could be construed as potential conflicts of interest.
All claims expressed in this article are solely those of the authors and do not necessarily represent those of their affiliated organizations, or those of the publisher, the editors and the reviewers. Any product that may be evaluated in this article, or claim that may be made by its manufacturer, is not guaranteed or endorsed by the publisher.
1. World Organisation for Animal Health OIE. Strategic framework for collaboration on antimicrobial resistance–together for one health. Geneva/Switzerland: Food and Agriculture Organization of the United Nations, WHO (2022). 22 p.
3. European Food Safety Authority, European Centre for Disease Prevention and Control. The European Union summary report on antimicrobial resistance in zoonotic and indicator bacteria from humans, animals and food in 2018/2019. EFSA J. (2021) 19:e06490. doi: 10.2903/j.efsa.2021.6490
4. Murphy, D, Ricci, A, Auce, Z, Beechinor, JG, Bergendahl, H, Breathnach, R, et al. EMA and EFSA joint scientific opinion on measures to reduce the need to use antimicrobial agents in animal husbandry in the European Union, and the resulting impacts on food safety (RONAFA). EFSA J. (2017) 15:e04666. doi: 10.2903/j.efsa.2017.4666
5. Landers, TF, Cohen, B, Wittum, TE, and Larson, EL. A review of antibiotic use in food animals: perspective, policy, and potential. Public Health Rep. (2012) 127:4–22. doi: 10.1177/003335491212700103
6. Bundestag. Sechzehntes Gesetz zur Änderung des Arzneimittelgesetzes: 16. AMG-Novelle. Bonn: Bundesanzeiger Verlag (2013).
7. German Federal Ministry for Food and Agriculture. Entwicklung der Kennzahlen zur Therapiehäufigkeit (2022). Available at: https://www.bmel.de/DE/themen/tiere/tierarzneimittel/entwicklung-kennzahlen-therapiehaeufigkeit.html. (Accessed Janauary 22, 2023).
8. DESTATIS. Holdings with cattle and stock of cattle. Available at: https://www.destatis.de/EN/Themes/Economic-Sectors-Enterprises/Agriculture-Forestry-Fisheries/Animals-Animal-Production/Tables/5-holdings-with-cattle-and-stock-of-cattle.html. (Accessed October 28, 2022).
9. Bos, ME, Graveland, H, Portengen, L, Wagenaar, JA, and Heederik, DJ. Livestock-associated MRSA prevalence in veal calf production is associated with farm hygiene, use of antimicrobials, and age of the calves. Prev Vet Med. (2012) 105:155–9. doi: 10.1016/j.prevetmed.2012.01.002
10. Pardon, B, Catry, B, Dewulf, J, Persoons, D, Hostens, M, de Bleecker, K, et al. Prospective study on quantitative and qualitative antimicrobial and anti-inflammatory drug use in white veal calves. J Antimicrob Chemother. (2012) 67:1027–38. doi: 10.1093/jac/dkr570
11. Kontrollgemeinschaft Deutsches Kalbfleisch. E. V. Deutsches Kalbfleisch E. V. Available at: https://www.deutsches-kalbfleisch.de/. (Accessed October 28, 2022).
12. Mitrenga, S, Popp, J, Becker, A, Hartmann, M, Ertugrul, H, Sartison, D, et al. Veterinary drug administration in German veal calves: an exploratory study on retrospective data. Prev Vet Med. (2020) 183:105131. doi: 10.1016/j.prevetmed.2020.105131
13. Deutscher Bundestag. Entwurf eines Gesetzes zur Änderung des Tierarzneimittelgesetzes zur Erhebung von Daten über antibiotisch wirksame Arzneimittel und zur Änderung weiterer Vorschriften. Drucksache 20/3712 (2022).
15. Glaser, S, and Kreienbrock, L. Sample size calculation in veterinary studies. A guideline to estimate the sampe size. Stichprobenplanung bei veterinärmedizinischen Studien. Ein Leitfaden zur Bestimmung des Untersuchungsumfangs. Schlütersche, Hannover. (2011).
16. Dohoo, IR, Martin, W, and Stryhn, H. Methods in epidemiologic research. Charlottetown: VER, Inc. (2012).
17. Ruddat, I, Scholz, B, Bergmann, S, Buehring, AL, Fischer, S, Manton, A, et al. Statistical tools to improve assessing agreement between several observers. Animal. (2014) 8:643–9. doi: 10.1017/S1751731113002450
18. R Core Team. R: A language and environment for statistical computing. Vienna/Austria: R Foundation for Statistical Computing (2022).
20. Wickham, H, Averick, M, Bryan, J, Chang, W, McGowan, L, François, R, et al. Welcome to the tidyverse. J Open Source Softw. (2019) 4:1686. doi: 10.21105/joss.01686
23. Bates, D, Mächler, M, Bolker, B, and Walker, S. Fitting linear mixed-effects models using lme4. J Stat Soft. (2015) 67:67. doi: 10.18637/jss.v067.i01
24. Wood, S, and Gamm, SF. Generalized additive mixed models using 'mgcv' and 'lme4': R package. (2020).
25. Fluckey, WM, Loneragan, WG, Warner, R, and Brashears, MM. Antimicrobial drug resistance of Salmonella and Escherichia coli isolates from cattle feces, hides, and carcasses. J Food Prot. (2007) 70:551–6. doi: 10.4315/0362-028x-70.3.551
26. Poirel, L, Madec, JY, Lupo, A, Schink, AK, Kieffer, N, Nordmann, P, et al. Antimicrobial resistance in Escherichia coli. Microbiol Spectr. (2018) 6:6. doi: 10.1128/microbiolspec.ARBA-0026-2017
27. Federal Ministry of Food and Agriculture. Report of the Federal Ministry of Food and Agriculture on the evaluation of the antibiotics minimisation concept introduced with the 16th act to amend the medicinal products act (16th AMG amendment): Evaluation based on section 58g of the medicinal products act. Bonn, Germany: Federal Ministry of Food and Agriculture (2019).
28. Jensen, CK, Gorisek, L, Weise, S, Baer, J, Friese, A, Robé, C, et al. Antibiotics use differs between types of calves keeping farms in Germany. In preparation. (2023).
29. Bundesamt für Verbraucherschutz und Lebensmittelsicherheit. Zoonosen-Monitoring 2021: BVL-Report 17.3 Berichte zur Lebensmittelsicherheit. Braunschweig. (2022).
30. Ceccarelli, D, Hesp, A, van der Goot, J, Joosten, P, Sarrazin, S, Wagenaar, JA, et al. Antimicrobial resistance prevalence in commensal Escherichia coli from broilers, fattening turkeys, fattening pigs and veal calves in European countries and association with antimicrobial usage at country level. J Med Microbiol. (2020) 69:537–47. doi: 10.1099/jmm.0.001176
31. Gay, E, Bour, M, Cazeau, G, Jarrige, N, Martineau, C, Madec, JY, et al. Antimicrobial usages and antimicrobial resistance in commensal Escherichia coli from veal calves in France: evolution during the fattening process. Front Microbiol. (2019) 10:792. doi: 10.3389/fmicb.2019.00792
32. Schnyder, P, Schönecker, L, Schüpbach-Regula, G, and Meylan, M. Effects of management practices, animal transport and barn climate on animal health and antimicrobial use in Swiss veal calf operations. Prev Vet Med. (2019) 167:146–57. doi: 10.1016/j.prevetmed.2019.03.007
33. Schönecker, L, Schnyder, P, Overesch, G, Schüpbach-Regula, G, and Meylan, M. Associations between antimicrobial treatment modalities and antimicrobial susceptibility in pasteurellaceae and E. coli isolated from veal calves under field conditions. Vet Microbiol. (2019) 236:108363. doi: 10.1016/j.vetmic.2019.07.015
34. Afema, JA, Davis, MA, and Sischo, WM. Antimicrobial use policy change in pre-weaned dairy calves and its impact on antimicrobial resistance in commensal Escherichia coli: a cross sectional and ecological study. BMC Microbiol. (2019) 19:217. doi: 10.1186/s12866-019-1576-6
35. Uyama, T, Renaud, D, LeBlanc, S, McClure, J, Slavic, D, Winder, C, et al. Observational study on antimicrobial resistance in Escherichia coli and Salmonella isolates from Ontario calf samples submitted to a diagnostic laboratory from 2007 to 2020. Can Vet J. (2022) 63:260–8.
36. Fertner, M, Boklund, A, Dupont, N, and Toft, N. Changes in group treatment procedures of Danish finishers and its influence on the amount of administered antimicrobials. Prev Vet Med. (2016) 126:89–93. doi: 10.1016/j.prevetmed.2016.01.034
37. Algammal, AM, El-Sayed, ME, Youssef, FM, Saad, SA, Elhaig, MM, Batiha, GE, et al. Prevalence, the antibiogram and the frequency of virulence genes of the most predominant bacterial pathogens incriminated in calf pneumonia. AMB Express. (2020) 10:99. doi: 10.1186/s13568-020-01037-z
38. Klima, CL, Zaheer, R, Cook, SR, Booker, CW, Hendrick, S, Alexander, TW, et al. Pathogens of bovine respiratory disease in north American feedlots conferring multidrug resistance via integrative conjugative elements. J Clin Microbiol. (2014) 52:438–48. doi: 10.1128/JCM.02485-13
39. Callens, B, Cargnel, M, Sarrazin, S, Dewulf, J, Hoet, B, Vermeersch, K, et al. Associations between a decreased veterinary antimicrobial use and resistance in commensal Escherichia coli from Belgian livestock species (2011-2015). Prev Vet Med. (2018) 157:50–8. doi: 10.1016/j.prevetmed.2017.10.013
40. Dorado-García, A, Mevius, DJ, Jacobs, JJ, van Geijlswijk, IM, Mouton, JW, Wagenaar, JA, et al. Quantitative assessment of antimicrobial resistance in livestock during the course of a nationwide antimicrobial use reduction in the Netherlands. J Antimicrob Chemother. (2016) 71:3607–19. doi: 10.1093/jac/dkw308
41. Becker, J, Perreten, V, Steiner, A, Stucki, D, Schüpbach-Regula, G, Collaud, A, et al. Antimicrobial susceptibility in E. coli and Pasteurellaceae at the beginning and at the end of the fattening process in veal calves: comparing 'outdoor veal calf' and conventional operations. Vet Microbiol. (2022) 269:109419. doi: 10.1016/j.vetmic.2022.109419
42. Ferroni, L, Albini, E, Lovito, C, Blasi, F, Maresca, C, Massacci, FR, et al. Antibiotic consumption is a major driver of antibiotic resistance in calves raised on Italian cow-calf beef farms. Res Vet Sci. (2022) 145:71–81. doi: 10.1016/j.rvsc.2022.01.010
43. Becker, J, Perreten, V, Schüpbach-Regula, G, Stucki, D, Steiner, A, and Meylan, M. Associations of antimicrobial use with antimicrobial susceptibility at the calf level in bacteria isolated from the respiratory and digestive tracts of veal calves before slaughter. J Antimicrob Chemother. (2022) 77:2859–66. doi: 10.1093/jac/dkac246
44. Bundesgesetzblatt. Verordnung zur Änderung der Tierschutz-Hundeverordnung und der Tierschutztransportverordnung. Bundesgesetzblatt. (2022).
45. Dorado-García, A, Graveland, H, Bos, ME, Verstappen, KM, van Cleef, BA, Kluytmans, JA, et al. Effects of reducing antimicrobial use and applying a cleaning and disinfection program in veal calf farming: experiences from an intervention study to control livestock-associated MRSA. PLoS One. (2015) 10:e0135826. doi: 10.1371/journal.pone.0135826
46. Rell, J, Wunsch, N, Home, R, Kaske, M, Walkenhorst, M, and Vaarst, M. Stakeholders’ perceptions of the challenges to improving calf health and reducing antimicrobial use in Swiss veal production. Prev Vet Med. (2020) 179:104970. doi: 10.1016/j.prevetmed.2020.104970
Keywords: antimicrobials, calves, AMR score, therapy frequency, antibiotics use
Citation: Merle R, Weise S, Gorisek L, Baer J, Robé C, Friese A and Jensen KC (2023) The therapy frequency of antibiotics and phenotypical resistance of Escherichia coli in calf rearing sites in Germany. Front. Vet. Sci. 10:1152246. doi: 10.3389/fvets.2023.1152246
Received: 27 January 2023; Accepted: 26 April 2023;
Published: 18 May 2023.
Edited by:
Om P. Dhungyel, The University of Sydney, AustraliaReviewed by:
Lucy Brunton, Royal Veterinary College (RVC), United KingdomCopyright © 2023 Merle, Weise, Gorisek, Baer, Robé, Friese and Jensen. This is an open-access article distributed under the terms of the Creative Commons Attribution License (CC BY). The use, distribution or reproduction in other forums is permitted, provided the original author(s) and the copyright owner(s) are credited and that the original publication in this journal is cited, in accordance with accepted academic practice. No use, distribution or reproduction is permitted which does not comply with these terms.
*Correspondence: Roswitha Merle, cm9zd2l0aGEubWVybGVAZnUtYmVybGluLmRl
Disclaimer: All claims expressed in this article are solely those of the authors and do not necessarily represent those of their affiliated organizations, or those of the publisher, the editors and the reviewers. Any product that may be evaluated in this article or claim that may be made by its manufacturer is not guaranteed or endorsed by the publisher.
Research integrity at Frontiers
Learn more about the work of our research integrity team to safeguard the quality of each article we publish.