- 1Department of Clinical Chemistry, Faculty of Medicine, University of Ioannina, Ioannina, Greece
- 2Division of Obstetrics and Gynecology, Department of Clinical Science, Intervention and Technology, Karolinska Institutet and Karolinska University Hospital, Stockholm, Sweden
- 3Department of Obstetrics and Gynaecology, Faculty of Medicine, School of Health Sciences, University of Thessaly, Larisa, Greece
- 4Division of Endocrinology, Central Drug Research Institute, Lucknow, India
The broad conceptualization of fertility preservation and restoration has become already a major concern in the modern western world since a large number of individuals often face it in the everyday life. Driven by different health conditions and/or social reasons, a variety of patients currently rely on routinely and non-routinely applied assisted reproductive technologies, and mostly on the possibility to cryopreserve gametes and/or gonadal tissues for expanding their reproductive lifespan. This review embraces the data present in human-focused literature regarding the up-to-date methodologies and tools contemporarily applied in IVF laboratories' clinical setting of the oocyte, sperm, and embryo cryopreservation and explores the latest news and issues related to the optimization of methods used in ovarian and testicular tissue cryopreservation.
Routinely applied assisted reproductive technologies for fertility preservation
Increasing evidence demonstrates a global reduction in fertility rates. Unfortunately, infertility now is rising with ~48 million couples and 186 million individuals experiencing infertility or pregnancy failure despite people having frequent and unprotected sexual intercourse (1). This alarming evidence, in combination with the fact that the in vitro fertilization (IVF) success rate is less than the IVF failure rate, may cause “social” (mainly age-related) or medical concerns, such as the potential loss of fertility (2). Under these circumstances, fertility preservation (FP) is considered an option or a necessity. In 2018, the ASRM practice committee accepted social egg freezing as ethically permissible and named it “planned oocyte cryopreservation” (3). For all individuals, cryopreservation of gametes, embryos, and gonadal tissues/cells is the only way to achieve fertility in the future. Performing various cryopreservation programs, either freezing mature or immature oocytes may increase the chances of desired future pregnancies, especially in women of reproductive age that have been diagnosed with cancer and prior to subjection to any toxic chemo-radiotherapy treatments. Moreover, women who prefer oocyte cryopreservation instead of embryo cryopreservation and undergo an IVF program, and women who wish to preserve their ability for a future chance to have a baby for personal (“social”) or medical reasons. Medical reasons include mainly sickle cell anemia, severe endometriosis, diminished ovarian reserve, autoimmune diseases, and ovarian insufficiency risk due to genetic conditions such as the Fragile X premutation, and Turner syndrome (4). Other reasons are the X chromosome deletion and patients that have undergone gender diversity as transgender with affected fertility status (5). Practically, all the concerns regarding embryo cryopreservation, such as ethical, legal, and religious can be challenged by the oocyte cryobanking choice. At present, cryopreservation programs of sperm cells are in operation for supporting FP in men. Semen cryopreservation is one of the main choices where male fertility is compromised, such as for cancer patients who undergo chemo-radiotherapy treatments or vasectomy, HIV-positive, men undergoing gender reassignment since feminizing hormone therapy and orchiectomies can affect their fertility, and last for those with “social” concerns (6). In contrast to gamete cryopreservation, the embryo cryopreservation program is adjustable for having another attempt, after a negative IVF outcome, where spare embryos that have been cryopreserved can be used in a subsequent frozen embryo transfer cycle without repetition of ovarian stimulation and oocyte retrieval. Alternatively to oocyte freezing, embryo freezing can also offer FP in women diagnosed with breast cancer, as it is known that women with breast cancer are subjected to gonadotoxic treatments that may affect their fertility (7, 8). The performance of gametes or embryo freezing, and storage takes place in order to exchange them from donors to recipients after participation in donor programs following eligibility-approved criteria (9–11). Indeed, gamete and embryo cryopreservation are the main implemented practices and well-established techniques for FP, which are widely applied daily in clinics worldwide (Figure 1). A short history of both gamete and embryo cryopreservation, as well as clinical and technical improvements, are indicated in Table 1.
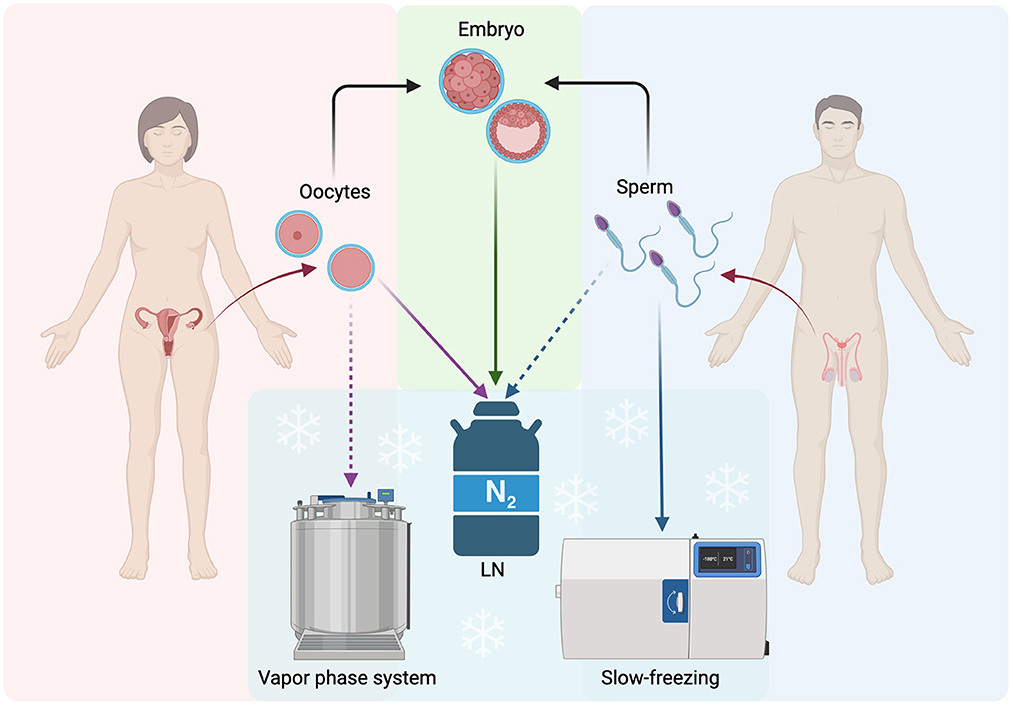
Figure 1. Schematic representation of the main routinely applied procedures for FP: gametes and embryos. LN, Liquid Nitrogen; Solid lines, often used procedure; Dashed lines, not often used procedures. The figure was realized with BioRender.
The current state of human gametes and embryo cryopreservation
Oocytes and embryos
The most applied method of fertility preservation is oocyte cryopreservation and compared to embryo cryopreservation it is technically more challenging since the high-water content is risky for causing cryoinjury (11, 39). Over the last decade, embryo cryopreservation technology has been proven to be safe and effective (40).
Currently, in most embryological laboratories, the traditional methods of freezing and thawing of both human oocytes and embryos have been replaced by vitrification/warming protocols. Mounting evidence has shown the superiority of vitrification/warming over the slow freezing (SF)/thawing protocols, in terms of both the embryological and clinical outcomes (41–43). Vitrification is currently recommended for freezing oocytes and embryos as it has shown remarkably increased live birth rates (LBRs) (44, 45). Vitrification as well as SF, based on standard cryobiology principles, should ensure the accuracy and the success of the method with a minimal negative effect on the quality of the cell during the process of SF/vitrification and thawing/warming. To achieve successful vitrification in IVF it is required the control of three parameters, the concentration of cryoprotectant agents (CPA; viscosity), rapid cooling and warming rates, and media volume for preventing the intracellular crystallization of water (46). Cell stress during cryopreservation on cell/embryo structure is mainly coming from the direct effect of the cooling temperatures. For example, the hardening of zona pellucida due to premature exocytosis of cortical granules, the swelling of mitochondria in oocytes, and, the absence of tight junctions in embryos are some of the ultrastructural cryo damages that have been reported (47, 48). In addition, physical changes in regard to ice formation compromise the viability of the gametes/embryos since supercooled straws can cause various injuries during the ice nucleation phase or “seeding” (49). Noteworthy, cryopreservation indirectly depends on the quality of cryopreserved gametes, which in turn depends on the response to ovarian stimulation treatment (poor, hyper and normo-responders) and the quality of the sperm sample (poly, normo, oligo and azoo-spermia). Embryos coming from gametes of low quality may worsen further after thawing (50, 51).
The current application of ultra-rapid-freezing vitrification procedures involves the exposure of oocytes or embryos to little volumes of high-concentration CPAs (4–8 mol/L) at a very short time (avoiding chemical toxicity by the high concentrations of the cryoprotectants) followed by plunging the straw to liquid nitrogen (LN), in order to achieve solidification (52). During the process, the high osmolarity of the solutions used causes rapid dehydration and the cells are mainly dehydrated prior to freezing. SF dehydration initiates at the equilibration and continues up to −35°C. Afterward, it is taking place the submersion into LN quickly and solidification, so that the remaining intracellular water does not have time for the formation of ice crystals. In a brief time, <2 s, cells are transitioned from −35°C to −196°C, resulting in extremely fast rates of cooling (>10,000°C/min) (53).
The high CPA concentrations used in vitrification are linked with the risk of toxicity in cells (54). Recommendations nowadays are to mix different CPAs in order to avoid the potential toxic risk. The reason is that the combination of CPAs enables the reduction of individual components below their toxic threshold and, reduces to the minimum the time of exposure of oocytes/embryos to the solution (54). To date, the most common freezing solutions used are composed of permeating (e.g., ethylene glycol (EG), glycerol (G), dimethylsulfoxide (DMSO), propylene glycol, acetamide; >4 M) and non-permeating (e.g., sucrose, trehalose; >0.5 M) agents. The most applied protocol for both oocytes and embryos includes the combination of 15% DMSO, 15% EG, and 0.5 M sucrose at the minimum volume of ≤1 μL (17). A recent study showed that in the most used CPAs combination (DMSO and sucrose), while DMSO reduces the solute concentration, sucrose higher proportion has a direct effect on rising the Tg value, therefore increasing the sample safe storage temperature (55). Thus, it is needed to expand the knowledge of each CPAs thermodynamics, in order to find the optimal CPA combination for appropriate vitrification. Macromolecules, such as polyEG, ficoll, or polyvinylpyrrolidone that are used as supplements in the vitrification medium had been found to support vitrification with lower concentrations of CPAs. By further increasing the cooling rate (>10,000°C/min), which is needed for successful oocyte/embryo vitrification, the final volume of the vitrification microdroplet proved to be dramatically reduced, even to 0.1 μL (56).
Regarding oocyte vitrification, several rapid-cooling solutions and protocols have been developed over the years. Variations of the DMSO-based protocol were first introduced in 1998. Except for the DMSO-based protocol, it was developed a vitrification system consisting of a phosphate-buffered medium supplemented with 20% human serum albumin (HSA), and G and/or EG in increasing concentrations (57). For human oocyte and embryo vitrification, both systems are well implemented and represent current and best viable options differencing mainly in the presence or the absence of DMSO. For example, the absence of DMSO allows slower cooling rates, larger volumes of microdroplets, and different carriers. The first IVF cycles with vitrified oocytes have been reported (45), offering also in selected patients a “freeze-all” cycle by freezing all retrieved/collected MII oocytes as an option for fertility preservation (58).
Similarly, identical or slightly modified protocols are used for the rapid-cooling vitrification of cleavage-stage embryos (day 3) and blastocysts (day 5–7). The embryo transfer of frozen cleavage-stage embryos or blastocysts appears to have no difference in pregnancy outcomes (59). Artificial shrinkage of day-5–7 blastocysts has been shown to cause less cryoinjury during freezing/warming (60). Improved survival rates of hatching blastocysts have been found after puncturing manually the trophectoderm with a needle or laser. Notably, in 2014 Parmegiani and colleagues published a pilot study, suggesting a “universal warming protocol” (extracellular CPAs 1–0.5 M) as an efficient protocol for warming (61). Later on, in 2017 they applied this protocol on commercial brand kits (Kitazato and Sega kits containing trehalose and sucrose, respectively), proposing that a combination of different kits is efficient for the vitrification/thawing of embryos (62). Moreover, in regard to embryo vitrification and particularly in re-vitrification, interesting results have been found in the re-vitrification of 8 cells and blastocysts. Indeed, the twice vitrification/warming embryos demonstrated different effects on embryo developmental potential, as re-vitrification at the blastocyst stage followed by previous vitrification at the 8-cell stage decreased the delivery rate. Therefore, it has been recommended to be avoided. Twice-vitrified 8-cell stage achieved comparable pregnancy results to the once-vitrified embryos (63).
Nevertheless, vitrification as a process seems to have technical difficulty during its operation due to the highly concentrated, viscous, and minimal volume of solutions. Therefore, oocytes/embryos require fast handling (<1 min) and only well-trained embryologists can complete this task successfully. Besides, freezing/warming remains a time-consuming process (8–15 min). However, a current study proved that time reduction can be achieved by adding a two-minute dehydration protocol which aims at remaining the critical intracellular concentration needed for successful vitrification. The efficiency of the above protocol was verified by the post-warming survival rates and the ability of the warmed embryos to resume cell cytokinesis (64). Furthermore, in order to accomplish a successful vitrification protocol in combination with the technical difficulties, special carrier systems, open (direct contact of medium with LN) and closed (not direct contact) has been developed. Nowadays, more than 30 different carrier tools have been described, and half of them are commercially available (65). Open-pulled straws, such as Cryoloop and Cryotop were chronologically first introduced and, later on, closed systems were developed, as a more sterile and safe method compared to the open systems (66). Closed cryo devices include the Vitrisafe, the CryoTip, and the high-security vitrification kit (20). Other less usable straws include the Flexipet-denuding pipette, the electron microscopy copper, the gel loading tips, the Vitmaster, the Cryolock, the Cryoleaf, and the Hemi-straw system (56, 66, 67). Notably, when Cryotop was compared to CryoTip, it was found that the closed device demonstrated better survival rates, but the vitrified oocytes appeared with ooplasmic vacuolization, swollen mitochondria, and a high number of dispersed vesicles, possibly due to a less rapid decrease of the temperature in the closed carrier (68). In a recent study using the open Cryotop, and the Vitrolife system against the closed Rapid-i® and the Kitasato system, the latest showed higher survival rates, but lower fertilization rates of the survived oocytes and no difference in the developmental competence compared to the open system (69). Based on the principal concept of cryo devices, in an attempt to minimize the volume of the vitrification solution for increasing cooling/warming rates, the novel device of the Kitasato System is currently under development in mouse embryo trials. This device is similar to the Cryotop device, differing in the possession of a porous membrane that absorbs excess vitrification solution around the embryos, in order to achieve more rapid cooling and warming rates, reaching 683,000°C and 612,000°C/min, respectively (70). Another new Argentinian device, the ZURE® Vitri Carrier is now under construction and the scientific group focuses to obtain preliminary data on recovery and survival rates of vitrified and warmed oocytes (71). To date, current interest focuses on the possible effect of shipment and storage of gametes and blastocysts by comparing vapor and LN phases. The novel vapor phase TMRW platform, which was developed and improved by the use of artificial intelligence, was designed to allow safer handling and a digital chain of custody. Briefly, it consists of the Brooks BioStore III Cryo −190°C System, including a Chart MVE 1500 series tank with custom-designed CryoGrids that hold radiofrequency identification-enabled CryoBeacons serving as vessels for commercially available cryo devices and cryo straws. Evaluation of the data TMRW showed that this system does not have any deleterious effects on the survival rate of sperm, oocytes, and blastocysts. In addition, the storage of embryos in the TMRW vapor phase platform did not have any impact on the post-developmental potential of human blastocysts (72). Modern high-efficiency vapor phase tanks, such as the Chart MVE 1500, allow the storage of specimens in the vapor phase at temperatures below −150°C (73).
However, the Cryotop device, which was first described by Cobo et al. (74), is the most popular and best-selling micro-volume storage device (75). Nonetheless, there is a debate in relation to the sterilization of LN, because gametes and embryos are coming in direct contact with LN, increasing therefore the risk of cross-contamination. The open vitrification system is forbidden in several countries, including France, Belgium, Ireland, and the Czech Republic, despite the existing evidence that the risk is negligible and at a theoretical level (69, 76, 77). Indeed, the virus screening of culture medium and LN for HIV, hepatitis B, and hepatitis C viruses resulted in the absence of the respective viruses in all samples studied which were vitrified by using the open Cryotop device (78). No contamination of bacteria or fungi was observed in both open Cryotop and closed CryoTip carriers, after storing the human genetic material for 1–2 years, while the risk for cross-contamination for bacteria in animals was equal for both carriers (79). The potential contamination risk by the Zika virus was reported in the cryostorage of gametes and embryos, especially in semen. Nevertheless, there is not sufficient data regarding the virus survival in LN, although this probability has been reported by the British Fertility Society. Interestingly, a study published in 2021 comparing the efficacy and safety of closed High-Security Vitrification™ vs. open Cryotop devices, showed that the closed system had comparable survival, developmental, pregnancy, and implantation rates to the open system. According to this finding, the authors suggested the use of closed devices for eliminating the potential risk of viral contamination even during the presence of SARS-CoV-2 (80).
Sperm
The most valuable and popular method for male FP is the cryopreservation of spermatozoa, which is widely used in assisted reproductive technology programs. In contrast to oocyte and embryo cryopreservation, sperm freezing is much simpler due to the small volume of water inside the spermatozoal cells.
SF, rapid freezing, and vitrification are sperm cryopreservation methods, discovered during the performance of several cryo-experimental improvements (81, 82). SF was developed by Behrman and Sawada and includes progressive sperm cooling in two or three steps, either manually or automatically (37, 83). SF was replaced over time by rapid freezing and vitrification protocols since SF is time-consuming and when performed automatically requires an expensive machine as a programmable freezer (84, 85). Optimal sperm survival rates depend strictly on the usage of CPAs and the cryopreservation process itself. Both permeable (e.g., DMSO, G, EG, and 1,2-propanediol) and non-permeable CPAs (carbohydrates such as glucose, sucrose, and trehalose) are routinely used for sperm freezing. Permeable CPAs due to their lipophilic properties can easily cross the sperm cell membrane, something that is associated with increased cell toxicity. The non-permeable CPAs are of high molecular weight and do not cross the membrane, making them effective for increased freezing speeds, and promoting rapid cellular dehydration (86–88).
Conventional SF is performed by using permeable CPAs and is still the most commonly applied technique for sperm freezing (37). SF allows the preservation of a relatively large volume of sperm ejaculate (89) but during the process, almost 50% of spermatozoa are lost by cell lysis caused mainly by the formation of ice crystals. This compromises the semen quality after thawing (90). Indeed, the cooling rate plays a key role in evaluating the extent of sperm cryoinjury since basic morpho-functional abilities of the spermatozoa might have been affected. Thermal shock, formation of intracellular and extracellular ice crystals, changes in membrane permeability, cellular dehydration, and osmotic shock are major cryo damages that may occur during the addition or removal of CPAs (91). In contrast to SF, rapid cooling and vitrification are fast protocols that do not cause ice formation during the process, while the cooling rates reach 3,000°C/min (92, 93). Proposed firstly by Sherman, rapid cooling is performed by freezing sperm in LN vapor, requiring direct contact between the straws/vials and nitrogen vapors (8–10 min), followed by rapid immersion in LN at −196°C (94). At vitrification, as suggested by the WHO manual for sperm analysis (95), the sperm samples are directly plunged/dropped into LN, reaching ultra-rapid cooling rates at seconds and thus preventing any possible formation of ice crystals. In addition, the osmolarity of the media appears to play a crucial role in avoiding membrane damage. Besides the osmolarity, similar importance seems to play the temperature during the thawing/warming process. Sperm vitrification requires the removal of seminal plasma before starting the procedure, while in other sperm freezing techniques, semen removal can be done either before freezing or after thawing (82). Seminal plasma may contain cell leukocytes or other microorganisms with the ability to promote the production of reactive oxygen species (ROS). It was found that either the swim-up or the density gradient centrifugation sperm preparation method, may eliminate the presence of ROS and the possible damage to the spermatozoa (96, 97). Improved sperm recovery rates (in terms of sperm motility) were found in comparison to conventional SF, while the levels of DNA oxidation, and mitochondrial activity remained unchanged (98). In line with the previous conclusion, a recent meta-analysis found better sperm recovery rates with the vitrification method when compared to conventional SF techniques. A drawback of that meta-analysis was the limited number of studies performed with sperm vitrification (84).
As mentioned above, vitrification requires high cooling rates and elevated CPA concentrations in order to bypass the phase of ice crystal formation during glass solidification. Notably, the high permeable CPA concentrations cause damage to spermatozoa. At present, the most commonly used CPAs for sperm vitrification are the permeable CPAs (including DMSO, G, glycol, EG, and methanol), and non-permeable CPAs (including albumins, dextran, and egg yolk citrate) (99). Recent clinical trials investigating the role of various concentrations and mixtures of CPAs at low sperm amounts (at around 20 μL of a sperm suspension drop), are ongoing to optimize the ideal mixture, concentration, and volume (100). Indeed, the investigation is focused mainly on the development of a vitrification protocol CPA-free (101, 102). Isachenko and colleagues were the first who succeed in the development of human sperm vitrification CPA-free (103, 104). In the same line, Slabbert and colleagues performed sperm vitrification CPA-free, suggesting that the CPAs-free technology results in higher mitochondrial membrane potential and lower sperm DNA fragmentation post-thaw when compared to conventional SF (105). Similarly, the permeable CPAs-free vitrification was found to produce better sperm recovery rates, in terms of intact acrosomes, more viable spermatozoa, and of a lower percentage of sperm DNA fragmentation when compared to conventional SF (106).
Although 0.25 mol/L sucrose has been widely used for both rapid freezing and vitrification of human sperm (101, 105), the optimal concentration of trehalose is still under investigation and more trials with different trehalose concentrations are necessary to standardize the rapid freezing and vitrification method. Comparisons between of 0.1 mol/L trehalose and 0.25 mol/L sucrose demonstrated that the first resulted in enhanced sperm motility post-warming when samples were cryopreserved in a closed device system. Moreover, sperm preservation with 0.1 mol/L trehalose showed improved membrane integrity at 0 h post-thaw, while after 6 or 12 h, there were no significant improvements in comparison with sucrose (107). Current trials in optimizing trehalose concentration revealed that the concentration of 0.125 mol/L is more beneficial in comparison to 0.25 mol/L (108). Additionally, combinations of CPAs, such as human tubal fluid, sucrose, and butylhydroxytoluene have been also proposed (103, 109–111).
Over the past years, apart from the indications for different combinations of CPAs, or even the absence of them, it has been also performed analogous testing in various cryo devices. With respect to each device, different volumes/droplets have been tested, but never more than 0.5 ml. Indeed, both rapid cooling and vitrification depend on the volume of sperm suspension, while only small volumes of 1–30 μl were reported in either closed or open systems. The results of every investigation emphasize the need for better protocols following the aseptic cryopreservation (101). Another limitation of the vapor method freezing is that it is unable to control the cooling rate caused by the volatilization of LN (37). A study evaluating sperm motility, fertilization ability, and DNA integrity in a Cryoloop open system using both the vapor and vitrification protocol, showed that there were no differences between the methods tested within the same device (112). When it was evaluated both protocols using cryovials, sperm motility and DNA fragmentation levels were comparable (113). Recently, a study testing six different cryopreservation methods using the novel funnel-shaped device, revealed the superiority of vitrification with 0.3 M sucrose and 20% (v/v) dextran supplement. Moreover, this study revealed optimal results in both conventional SF and vitrification (100). The 0.5 mL straw for rapid freezing and the straw-in-straw for the vitrification system showed no significant differences regarding the percentage of post-thawing sperm DNA damage (114).
Similar to the cryo devices for oocyte and embryo cryopreservation, many also open systems have been developed for sperm vitrification. For example, the 5-mm copper loop, the cryoloop, the open-pulled straw, and the open-standard straw (112, 115). The potential risk of contamination is one of the major disadvantages when freezing semen samples with the open vitrification system due to direct exposure to LN (116). Using a closed carrier during the vitrification process is not always feasible. Moreover, mounting evidence reveals that the storage of sperm samples using the vapor protocol can decrease the risk of viral contamination (and cross-contamination) (117). Apart from the open systems, a variety of closed tools have been proposed and developed in the vitrification process and some of them are the straw-in-straw, the high-security vitrification straw, the Cryotip, the VitriSafe, the Cryopette, the cryptologic, the Rapid-i, the S3 system, and the S3 μS-VTF device (99). Regarding the ultra-rapid vitrification, in an attempt to freeze a small number of spermatozoa, a study suggested a novel approach based on the use of micro-straws (50–100 μl) instead of the traditional straws (0.25 and 0.5 ml) (118). As the micro straws are thin retaining very small cryo-volumes, the freezing rate is faster. These devices showed better sperm motility values after post-thawing maintaining simultaneously the morphology and sperm DNA integrity (38). Other carriers for cryopreserving oligospermic samples and microquantities have been developed, such as the empty zona pellucida, the Volvox globator spheres, the alginate beads, the agarose microspheres, the microdroplets, the straws, the mini-straws and, the open-pulled straws, cryoloop (37, 119). In 2020, a novel sperm tool, named SpermVD, was described as a carrier of high efficiency for freezing a small number of spermatozoa in low-volume droplets. This tool has the advantage of an almost 100% recovery rate post-thawing and eliminates the post-thawing time search for the recovery of the spermatozoa. Moreover, the results obtained by this tool were promising and in particular in men suffering from non-obstructive azoospermia (120). Nowadays, the development and optimization of new devices are based on the progress of 3-D printing technology. This technology makes feasible the production of such advanced freezing devices through complicated designing and printing methods, optimizing and standardizing the accuracy of sperm cryopreservation (121). Nevertheless, the majority of protocols for sperm vitrification are not standardized yet in order to be used in clinical routine. In this context, aseptic techniques can be applied, since the cytoplasmatic membrane of the spermatozoon is exposed to LN, making it more susceptible to the adhesion of microorganisms present in LN during the cryopreservation process. Unlike to the spermatozoon, the cytoplasmatic membrane of the oocytes and embryos is covered by the presence of zona pellucida. Besides, these protocols rarely attain satisfactory post-thawing sperm motility and viability values. Although the above evidence has been recently reported, a survival rate of more than 70% with a new approach (122). The vitrification process is considered more applicable for sperm samples with an extremely low number of spermatozoa, such as those retrieved after testicular surgical sperm extraction. In addition, the storage of vitrified sperm samples appears to have a significant role in cryopreservation strategy, bearing in mind their use in intrauterine insemination protocols.
Not routinely applied assisted reproductive technologies for fertility preservation
In contrast to patients that have the possibility to be subjected either to gamete or embryo cryopreservation procedures in order to preserve their fertility, there is a percentage of patients that cannot undergo the routinely applied processes of fertility preservation. As far as those patients are concerned, gonadal tissue cryopreservation has been indicated as an alternative aiming to safeguard their fertility. Ovarian tissue cryopreservation (OTC) is the only option for girls with cancer at the prepubertal stage since the potential risk of stimulation of estrogen-sensitive cancer can be bypassed (123, 124). Other patients that may benefit from the OTC programs are those having recurrent ovarian cysts, ovarian torsions, and autoimmune diseases as well as women of early reproductive age who wish to delay their menopause (defined as “social freezing”) or to avoid the syndrome of premature ovarian insufficiency. Patients with Turner syndrome or transgender men may also benefit from the OTC program (8, 124–126). The goal of OTC is to maintain the morpho-functional characteristics of the ovary providing in this way a huge supply of primordial follicles, which can be successfully used in the future, preserving their fertility. Systematic reviews in regard to the clinical outcome of ovarian transplantation range between a minimum of 42% and a maximum of 81%, denoting the clinical importance of using this fertility cryopreservation method (127, 128). Noteworthy, 130 healthy babies were born worldwide with the applied OTC (129) two women only worldwide have undergone transplantation with the OTC program which resulted in three live births after transplantation (130, 131). Testicular tissue cryopreservation (TTC) is recommended for boys who had been diagnosed with cancer at the prepubertal stage since 30% of the survivors (a cancer survival rate of 80%) appears to encounter azoospermic issues in the ambient future (132). TTC is indicated also for adolescents diagnosed with testicular cancer, leukemia, or Ewing sarcoma and who have an elevated risk to develop permanent sterility issues after gonadotoxic chemo- or radiotherapies (133). Other patients that may benefit from TTC programs are those having severe autoimmune diseases, genetic and congenital diseases such as those who suffer from the Klinefelter syndrome, and, other life-threatening non-malignant diseases such as drepanocytosis, thalassemia, idiopathic medulla aplasia, and the granulomatous disease. The same group involves patients who are subjected to gonadotoxic therapies, such as full-body radiotherapy, as well as prepubertal boys with a high risk to acquire infertility problems due to bone marrow transplantation (134). However, the clinical application of OTC and TTC is not well-established, and further research and improvements are needed regarding the protocols and the tools used for these occasions (Figure 2). Although improvements in OTC and TTC methods are essential, a major concern is raised in patients affected by oncological pathologies. In this category, it may be an increased risk of reintroducing malignant cells after tissue transplantation. This main drawback is specifically explicated at the end of the following sub-sections.
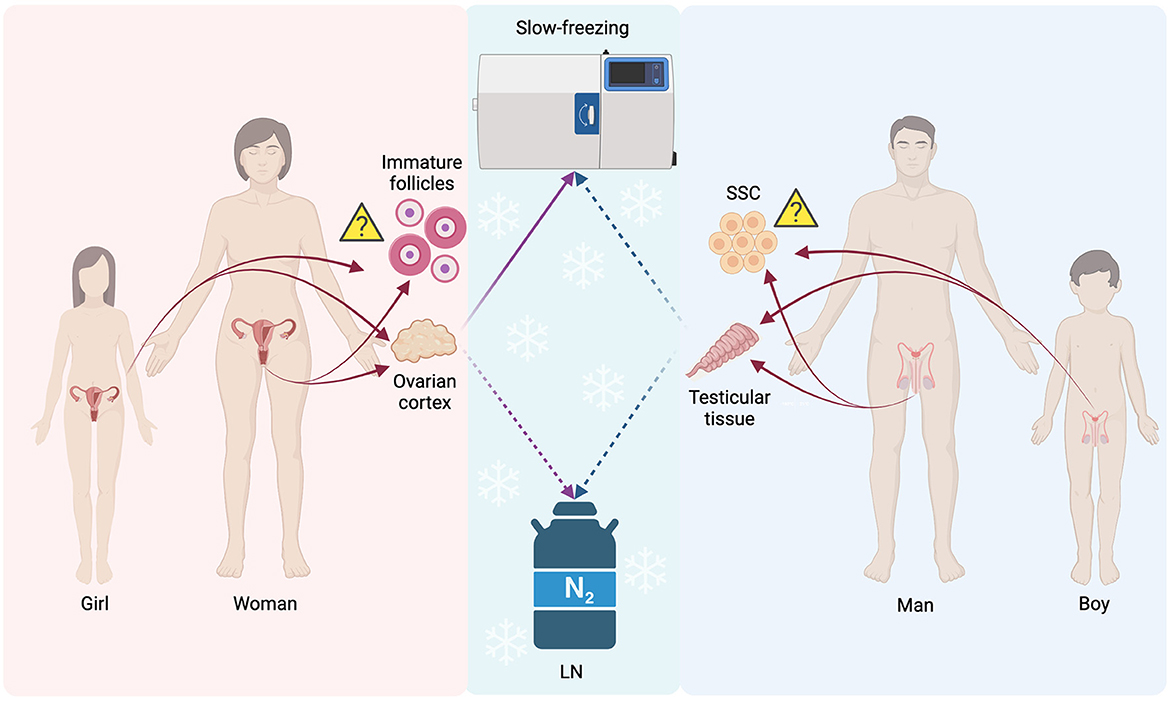
Figure 2. Schematic representation of the main not routinely applied procedures for FP: gonadal tissues, follicles, and spermatogonial stem cells. LN, Liquid Nitrogen; SSC, spermatogonial stem cells; Solid lines, often used procedure; Dashed lines, not often used procedures/experimental; Triangle, still undecided if feasible for FP purposes or not. The figure was realized with BioRender.
Human gonadal tissue cryopreservation: an ongoing process
Ovarian tissue
Nowadays, the OTC programs for fertility preservation procedures are gaining ground in regard to clinical application, holding hope for all patients that couldn't have undergone routine FP programs (8, 129). OTC is applied solely to the ovarian cortex area. Specifically, a 1 mm area is surgically removed from the ovary and cryopreserved from a surface area that ranges from 2 × 2 to 5 × 5 mm (135). Thus, after collecting the cortical ovarian tissue, the somatic and germ cells are frozen with the ultimate scope to preserve early-stage follicles for either future autologous transplantation or in vitro oocyte maturation (136, 137). Autologous ovarian tissue transplantation (OTT) can be orthotopic, meaning that it will be reimplanted in the pelvic cavity or in the ovarian medulla. In a recent study, the ovarian function was restored with 90% success (124). When OTT is heterotopic, the biopsy may be reimplanted outside the peritoneal cavity (138). In comparison with other FP procedures, the OTC process is of increased complexity, owing to the presence of multiple and diverse cell populations. It is known that the presence of these cells may negatively affect the permeation of cryoprotectants, thus also affecting the survival of the follicles (81). In contrast to oocyte vitrification, OTC showed a decreased LBRs trend, but still, today remains the only promising choice for some of the aforenamed patients (124). Notwithstanding, both OTC LBRs and OTT outcomes, could be largely improved by ameliorating the revascularization of the reimplanted ovarian tissue with angiogenic and antiapoptotic factors (129). Interestingly, there is no global consensus about the cryopreservation protocol regarding OTC, even if several trials have been made to develop a whole ovary cryopreservation method (139, 140).
In this matter, two are the procedures for ovarian tissue freezing. The preferable one is the SF method. In this method of paramount importance is the choice of the permeable CPAs (propanediol, DMSO, or EG in a concentration of 1.5 M) in combination with the non-permeable sucrose (~0.1 M) (141). The main factors affecting the SF procedure are the different compositions of the cell types and the complicated extracellular matrix composition. Both these factors should be taken under serious consideration in order for cryoprotectants to gain access to the inner part of the tissue. Toward this, it is essential to separate the ovarian tissue into thin strips or small squares at the initiation of the procedure (135). In 1994, Gosden and colleagues developed for the first time a protocol for OTC. Based on this protocol all the subsequent protocols developed are deviating either in time or in the temperature of the CPAs (142). Briefly, after the equilibration of ovarian tissue with CPAs at 0°C, the tissue stripes are inserted in a programmable freezer where the temperature is gradually reduced to −7°C (for manual seeding) followed by another reduction of the temperature up to −140°C. Finally, the ovarian tissue stripes are cryopreserved and stored in LN for long-term storage (141). In late past years, many investigations have tried to improve the SF protocol, mainly focusing on the notion to reduce the formation of ice crystals that may damage the tissue. The long-time cooling of the ovarian cortex before OTC seems to decrease the translocation of phosphatidylserine in the tissue (143), but a recent application of this protocol found that 24 h cooling of human ovarian tissue at 5°C increased the viability of cells post-thawing (144). Moreover, the use of 20% DMSO concentration allowed a decrease in ice formation, however, it did not increase the survival of the follicles after xenograft (145). Interestingly, promising research has been conducted on sheep ovaries, but until now it was not tested on human ovaries (146), emphasizing once more the lack of evidence for a unanimous OTC protocol.
Despite the SF as a method that is currently the golden standard for OTC, much progress has been made in ovarian tissue vitrification (OTV), aiming to improve the survival of the tissue after warming (147). In Japan, two babies have been born by this method (148, 149). The vitrification procedure is mostly based on the balance between the ultrafast cooling rate and the high concentrations of CPAs. Bearing in mind that high concentrations of CPAs are toxic, then the combination of two or more CPAs seems to be essential because on one hand enhances the support of the tissue and on the other hand decreases the toxicity of the CPAs (150). The small volume during OTV appears to influence negatively the success of OTV. The absence almost of liquids and the difficulty to form ice crystals are attributed to the small volume during vitrification (149). In an attempt to avoid the larger volumes of CPAs, various tools have been described as for instance the solid surface (151), the medium droplets (152), the plastic straws (153), the open Cryo Type M, the closed CryoSheet device (154), and the silver closed vitrification system (155). Interestingly, the use of the stepped vitrification device with high DMSO concentrations resulted in reduced follicle membrane disintegration (156). Additionally, in an OTC protocol through the method of slush nitrogen vitrification which increases the cooling rates and reduces the so-called “Leidenfrost effect”, it was observed better results in terms of follicle ultrastructures, viability, and stromal cell integrity (157, 158).
Due to the lack of consensus regarding standard protocols for the OTC, there are still a few matters that make the FP process more challenging. Among them, follicle survival as well as the size of the ovarian cortex for re-transplantation are major issues that need to be addressed. Recently, Kristensen et al. proposed the vital dye-neutral red method as a quantitative approach for the evaluation of follicle survival after thawing (159). This study, conducted in a non-clinical setting, pointed out the great follicle survival (average of 84%) in ovarian tissue strips cryopreserved through the SF method. Nevertheless, the main issue remains unresolved, since it is impossible to transplant to the patient exactly the same ovarian strip which was intended for investigation. The size of the re-transplanted ovarian cortex is playing also a major role in FP procedures. Large cortical pieces demonstrate greater follicular activity (160, 161), while small pieces (of 1 mm3 approximately) show reduced follicle growth (162).
Other alternatives to OTT, whenever this procedure is not feasible (e.g., cancer patients, transgender women, or polycystic ovary syndrome patients), are the in vitro maturation (IVM), the maturation of small immature follicles, and the use of “artificial ovaries” (163). The follicles are extracted from the tissue and matured in vitro for obtaining MII oocytes for further assisted reproductive technologies processing (164, 165). Similarly, a multistep culture technique has been developed for the in vitro activation of follicles from the primordial to the antral follicle stage, followed by the retrieval of the oocyte for the subsequent IVM procedure (166, 167). Experimental studies of this technique on human primordial follicles showed promising results up to the retrieval of the MII-phase oocytes (168). Although those studies are in the early experimental phase, huge hopes rely on the creation of a biodegradable scaffold, the so-called “artificial ovary”, that support the growth and development of human follicles, either in vitro or after autologous transplantation (169, 170). Toward this, multiple types of scaffolds have been developed, such as fibrin-based (171–173), 3D printed microporous hydrogel (174) and decellularized ovaries (175–177). Most of them have been used for follicle culture in animals and humans followed by transplantation in sterilized mice with promising results. Indeed, primary results have been obtained in mouse models. The experimental achievement regarding the transplantation of artificial ovaries in ovariectomized mice may shed light on the path of using OTC for the purposes of FP, reducing also the potential risks for women (178).
Patients suffering from hematological diseases (such as leukemias), neuroblastoma, or Ewing's sarcomas should be handled with extra caution, because of the potential presence of cancer cells in the vasculature or in the soft tissue that will be cryopreserved. Much attention has been given to cases of cancer patients during OTT because in some cases there is a major risk to reintroduce malignant cells into the ovarian tissue (179). Immunohistochemistry, molecular analysis, and investigation of the ovarian biopsy after xenotransplantation in mice models have been applied clinically in order to detect the presence of residual malignant cells in the tissue. However, the aforementioned tests require the analysis of the ovarian tissue, making it impossible to transplant the same ovarian strip in the patient (180). A possible treatment of the ovarian tissue before transplantation is the so-called “ovarian purging”. This method involves the treatment of the tissue with inhibitors that affects exclusively the malignant cells, leaving untouched both follicles and stromal compartments (181). Several ex vivo studies on human cryopreserved ovarian tissues from patients diagnosed with leukemia showed that the treatment of the tissue with tumor-specific inhibitors, such as DARP in-toxin fusion proteins, Verteporfin, Everolimus, and Aurora kinase B/C inhibitor, successfully reduced the cancer cells without impairing the survival rate or the growth potential of early-stage follicles (182–185). Thus, the application of this method may diminish the risk of the reintroduction of tumor cells to the ovarian tissue that will be transplanted, thus enhancing the possibility of FP in cancer patients.
Testicular tissue
Although sperm cryopreservation appears to be the optimal choice for male FP, a percentage of men with infertility should preserve their fertility with different methods since they do not have spermatozoa in their ejaculate. These methods include the isolation of spermatogonial stem cells (SSC), testicular cell suspension (TCS), and testicular tissue cryopreservation (TTC) (134). Among these procedures, TTC is the preferable option since cryopreserves SSCs with the surrounding supportive microenvironment, allowing in this way the transplantation of only either the SSC or the whole tissue, which in turn allows performing in vitro spermatogenesis after thawing (186). To date, the methods that exist for TTC are the controlled and uncontrolled SF (CSF and USF, respectively). Last, the vitrification option is currently applied in animals but is still in an experimental phase in humans (187–189). As far as TTC is concerned, the SF method (particularly the CSF) is considered to be the golden standard, in terms of optimal tissue preservation and prevention of ice crystal formation (134, 189). Data regarding the TTC method showed significant differences between the DMSO-based medium for immature TTC and the G-base medium for mature TTC (190, 191). Similar to ovarian tissue, when performing TTC needs to be taken into consideration factors such as the presence of several different cell types, the CPAs permeation time, the temperature as well as the size of testicular tissue, which range from 1 to 9 mm3 (192, 193). While it has been described SF and vitrification methods regarding the survival rates of human SSC in xenografted tissues (134, 191, 194), it is obvious that additional studies are still necessary for improving testicular cryopreservation methods in order to be applied in clinical settings.
Although CSF is currently recognized as the most preferable and efficient method for TTC (195, 196), the scientific community did have not yet reached a consensus on a standard protocol between CSF and USF. Methodologically the difference relies on the control of the cooling rate. While CSF requires the use of a programmable freezer to reach a controlled cooling rate of around −8°C, followed by manual seeding, the USF method involves the use of an isopropyl alcohol container (generally the Mr. Frosty Freezing Container) placed overnight at a −80°C freezer with an uncontrolled cooling rate of around 1°C/min (187). Pioneer studies point to the use of combined culture media with DMSO favoring the permeable CPAs in combination with the non-permeable sucrose (195, 197–200). When CSF was performed in the presence of DMSO, studies evidence the better-cryopreserved ultrastructure and higher survival rates of Leydig and spermatogonial cells after a 12- and 24-day ex vivo culture (192, 198, 201). Additionally, the effect of Leibovitz L-15 in the culture medium when compared to phosphate-buffered saline showed no sign of tissue damage, neither in terms of morphological features nor in spermatogonial cell survival rate (202). Interestingly, CSF when was applied to TCS the results were comparable with the TTC, pointing out that TCS cryopreservation may have a potential role at a clinical application level. Nevertheless, further studies are required for the confirmation of these preliminary data (203, 204).
The vitrification of testicular tissue as an ultra-rapid freezing technique is still in an experimental stage, therefore is not applied for any FP procedures. Until today, only a few investigations have been conducted in humans for the development of a testicular tissue vitrification (TTV) protocol. The results were not so convincing in order to promote the use of TTV over the standard method of CSF. TTV was investigated in young-derived testicular tissue in which the tissue was directly plunged into LN within cryo straws, and compared to CSF followed by short-term culture (205) or long-term xenografting (194) after warming/thawing. In all experimental studies, when comparing the CSF and the TTV protocols, the authors denoted a similar morphology, by using hematoxylin-eosin, and a comparable presence of proliferative spermatogonial cells, by using the MAGE-A4 and Ki67 markers (194, 205). Concomitantly, Baert and colleagues explored two different approaches for TTV, solid-surface vitrification (SSV) and direct cover vitrification (DCV), and compared them with CSF and USF methods. In brief, SSV was performed by placing the tissue on aluminum floaters partially immersed in LN, while in the DCV method, the tissue is placed in a cryovial and plunged into LN (187). Despite the fact that in the SSV method, a minimum of spermatogonial-cell ultrastructural damages was found, the number of SSCs recovered after thawing was drastically reduced in comparison to the CSF protocol. These results indicate that vitrification is the best cryopreservation protocol for SSCs but only in the cells that are able to survive the ultra-rapid freezing process. Finally, the scientific background regarding vitrification at TCSs is relatively poor, therefore it cannot be considered as a valuable option besides the TTC method. Only two papers have been published, in which they show a higher survival rate of vitrified TCS in comparison to the CSF one (206, 207). Besides, both publications evaluate exclusively the quality of cell integrity and the survival rate, but not their functionality. Further studies are needed also to identify the ideal TTC procedure for FP protocols in order to use it in clinical practice.
Due to the lack of a standard TTC protocol, it is worth mentioning the different devices that may help during cryopreservation, especially in the TTV methods. Among the opened options, the open-pulled straws have been tested on TTV. The results were very encouraging as far as integrity and functionality are concerned (194), although the open device jeopardize the tissue due to the possible risk of contamination and cross-contamination by the LN of the tank (previously described in this review). The issue of contamination and cross-contamination is not encountered when closed devices (such as cryovials) are used, or during the application of SSV. These methods are extensively studied in small and large animals (208–211) but lack an optimization regarding human testicular tissue (187).
The main drawback of TTC and testicular tissue transplantation (TTT) in cancer patients is the potential risk of reintroducing malignant tumor cells to the implanted tissue. This issue has been given much attention in both TCS and testicular tissue samples (212–214), although in some human experimental settings the authors were able to sort malignant leukemic cells out of the TCS (215, 216). Altogether these results raise concerns about the safety of TTT in cancer patients, highlighting the need for further studies.
Accessibility considerations to fertility preservation: recent guidelines and programs for patients' recruitment
The exponential need for FP in the whole gamma of patients mentioned in the text makes the need of presenting detailed guidelines for the standardization of protocols in order to be applied to IVF clinics either inside the European context or outside. These rules should cover several aspects of FP programs, including medical indications, or any ethical, social, and legal considerations. Whenever applicable, the first line of action for standard-of-care patients in order to preserve their fertility is gamete and embryo freezing (3, 66, 217–219). For instance, at first, FP in patients undergoing gonadotoxic treatments is either the retrieval of oocytes (preferably mature) and spermatozoa from ejaculation or surgically recovered before cancer therapy treatment, or alternatively, the cryopreservation of embryos after an IVF cycle, as reported in the guidelines applied recently (3, 220). In addition, oocyte cryopreservation has been extensively used and recommended for social freezing reasons, today referred to as “planned oocyte cryopreservation”, and for the establishment of donor egg banks (5, 220, 221).
One of the main and still unresolved issues is the lack of recommendations regarding the accessibility of patients to specific FP programs. The discrepancies between the countries are mainly wrapped around the ethical statements and considerations of these procedures. A paradigm of the above discrepancy is the case of the OTC. This FP process is a prerequisite in hormone-sensitive cancer patients, in prepubertal girls that cannot yet produce oocytes, and in patients that undergo ovariectomy for health and social reasons. For these patients, in 2018–2019, a highly qualified ethical and practice committee (organized by ASRM), granted a green flag to specialized centers to undergo OTC in a clinical standardized environment (3, 222). On the other hand, the European perspective is still reluctant on moving forward. The current European guidelines and country-specific Fertility and Oncology Societies built up a consensus regarding the recommendation and the relative criteria for OTC as a FP process. Nevertheless, the approval of the protocol from the Institutional Review Board of the centers is still mandatory (220, 223, 224). Another issue concerns the use of TTC as a potential procedure in young boys with an increased risk of infertility, and/or with a genetic predisposition for testicular tissue degeneration. In fact, multiple European and American centers offer cryopreservation of immature testicular tissue (to date ~1,033), even though this procedure is still considered experimental (225). This still unsolved matter stresses the need for more clinical trials and follow-ups reporting data on the safety and efficacy of fertility restoration after TTC/TTT.
Notably, the discrepancies regarding the cultures and societies are based mainly on the existing regulation of patients' access to FP programs (226). The vast guidelines (covered mainly by US, European and Australian landscapes) that concern FP programs highlight the progression of legislation and the improvement of the governmental issues regarding access to FP programs. As far as Asia guidelines are concerned, a scarce number of guidelines are coming from Japanese Society, followed by Korean and Chinese Societies (the main different FP programs, divided according to the geographical sources are summarized in Table 2).
Finally, it is of paramount importance the commitment of all specialized centers and all experts in the field that are involved in communication with the patients, to promote and suggest, all the necessary information for the options of FP programs, the feasibility, and the safety of the guidelines (248).
Conclusions and future perspectives
In humans, oocyte and embryo vitrification is a method of FP that is routinely applied. Although vitrification is well-established offering a broad vista in patients undergoing assisted reproductive technologies, its application to other more complex biological materials, such as tissues, is still under investigation. Indeed, oocyte and embryo vitrification have shown increased clinical outcomes. In particular, several IVF centers prefer the “freeze-all” protocol anticipating better IVF outcomes (45, 58). The cryopreservation of embryos, either at the cleavage stage or blastocysts, has already reached the maximum outcome through the vitrification process. However, nowadays, challenges are encountered in developing more standardized, optimal, and successful protocols for the cryopreservation of oocytes and embryos, aiming at maintaining integrity in their structure and function post-thawing. Sperm vitrification is currently applied in samples with reduced sperm concentration and volume, while with increased sperm volumes the choice of SF sounds better. In several cases with a limited number of viable spermatozoa, such as epididymal and testicular samples, the conventional SF method is inappropriate. Nevertheless, single-sperm freezing technology such as the use of empty zona pellucida and the novel SpermVD device gives hope to such patients (249, 250). The existing methods concerning sperm cryopreservation need to be validated in terms of new CPAs or even antioxidants. In addition, the future of sperm cryopreservation might benefit from personalized and individually designed cryopreservation approaches. However, established cryopreservation programs with highly developed protocols and appropriate devices can better support FP procedures and may reshape the cryo-banking landscape of gametes and embryos. Especially in vitrification, there is an urge for established recommendations and guidelines regarding the handling and storing of gametes and embryos. Additionally, the contribution of artificial intelligence and genetic analysis via next-generation sequencing could enhance the success of various cryopreservation programs.
Even if OTC and TTC bio-banking is not routinely applied for patients' FP, the cryopreservation methods are a matter of debate. SF of the OTC cortex is the most applied method, whilst OTV is still clinically uncertain, due to the limited number of babies born. The optimization and standardization of cryopreservation protocols for OTC and OTT are currently ongoing. The health of oncological patients is of pivotal importance in this matter, demanding the development of standard-of-care procedures for improving their safety and fertility lifespan. On the other hand, TTC has been experimentally applied to animal models, but the clinical data reported are not valid. For the optimization of OTV or the standardization of TTC procedures, it is required a deep understanding of freezing/thawing biochemistry in order to improve the safety or to establish the efficiency of the applied techniques. Although TTC is still at an experimental stage and not yet established at a clinical phase, it seems to be a promising FP technique. Once TTC will be refined and validated via appropriate algorithms, it will gain ground for future fertility restoration, something that seems to be an important scientific and clinical achievement for young patients. Most clinical human trials are based on the notion to retain the cryopreserved cells or tissues intact. Concomitantly, it is crucial the development of more accurate freezing protocols and cryostorage systems, which will ensure the correct preservation of tissue materials.
It is of great importance to underline that also the guidelines for gametes, embryos, and tissue cryopreservation have a key role for both providers and patients to decrease the risks linked to an unsafe FP choice and cryostorage. The international reproductive medicine community should make a joint effort to have as a main goal the establishment of global registries regarding FP techniques and their short-/long-term outcomes. This eventually will allow overpassing cultural, societal, and geographical discrepancies and will increase the resonance of counseling, thus empowering the patients' choice for FP programs, and ameliorating the global bioethical harmony.
Author contributions
Conceptualization: GA. Writing—original draft preparation: SA, VD, and CM. Writing—review and editing: AD, SR, and GA. Supervision: AD and GA. All authors have read and agreed to the version of the manuscript.
Conflict of interest
The authors declare that the research was conducted in the absence of any commercial or financial relationships that could be construed as a potential conflict of interest.
Publisher's note
All claims expressed in this article are solely those of the authors and do not necessarily represent those of their affiliated organizations, or those of the publisher, the editors and the reviewers. Any product that may be evaluated in this article, or claim that may be made by its manufacturer, is not guaranteed or endorsed by the publisher.
Abbreviations
CPAs, cryoprotectant agents; CSF, controlled slow freezing; DCV, direct cover vitrification; DMSO, dimethylsulfoxide; EG, ethylene glycol; FP, fertility preservation; G, glycerol; HSA, human serum albumin; IVF, in vitro fertilization; IVM, in vitro maturation; LBRs, live birth rates; LN, liquid nitrogen; OTC, ovarian tissue cryopreservation; OTT, ovarian tissue transplantation; OTV, ovarian tissue vitrification; ROS, reactive oxygen species; SF, slow freezing; SSC, spermatogonial stem cells; SSV, solid-surface vitrification; TCS, testicular cell suspension; TTC, testicular tissue cryopreservation; TTT, testicular tissue transplantation; TTV, testicular tissue vitrification; USF, uncontrolled slow freezing.
References
1. World Health Organization (WHO). ICD-11 International Classification of Diseases 11th Revision. (2022). Available online at: https://icd.who.int/en (accessed December 30, 2022).
2. Thoma M, Fledderjohann J, Cox C, Kantum Adageba R. Biological and Social Aspects of Human Infertility: A Global Perspective. In: Oxford Research Encyclopedia of Global Public Health. Oxford: Oxford University Press (2021) doi: 10.1093/acrefore/9780190632366.013.184
3. Ethics Committee of the American Society for Reproductive Medicine. Fertility preservation and reproduction in patients facing gonadotoxic therapies: an Ethics Committee opinion. Fertil Steril. (2018) 110:380–386. doi: 10.1016/j.fertnstert.2018.05.034
4. Condorelli M, Demeestere I. Challenges of fertility preservation in non-oncological diseases. Acta Obstet Gynecol Scand. (2019) 98:638–46. doi: 10.1111/aogs.13577
5. Pai H, Baid R, Palshetkar N, Pai A, Pai R, Palshetkar R. Oocyte cryopreservation-current scenario and future perspectives: a narrative review. J Hum Reprod Sci. (2021) 14:340–9. doi: 10.4103/jhrs.jhrs_173_21
6. Grin L, Girsh E, Harlev A. Male fertility preservation–Methods, indications and challenges. Andrologia. (2021) 53:e13635. doi: 10.1111/and.13635
7. Warner E, Glass K, Foong S, Sandwith E. Update on fertility preservation for younger women with breast cancer. CMAJ. (2020) 192:E1003–9. doi: 10.1503/cmaj.200245
8. Choi JY, Kim TJ. Fertility preservation and reproductive potential in transgender and gender fluid population. Biomedicines. (2022) 10:2279. doi: 10.3390/biomedicines10092279
9. Practice Committee of the American Society for Reproductive Medicine and the Practice Committee for the Society for Assisted Reproductive Technology. Guidance regarding gamete and embryo donation. Fertil Steril. (2021) 115:1395–1410. doi: 10.1016/j.fertnstert.2021.01.045
10. Antonouli S, Papatheodorou A, Panagiotidis Y, Petousis S, Prapas N, Nottola SA, et al. The impact of sperm DNA fragmentation on ICSI outcome in cases of donated oocytes. Arch Gynecol Obstet. (2019) 300:207–15. doi: 10.1007/s00404-019-05133-9
11. Cobo A, García-Velasco JA, Coello A, Domingo J, Pellicer A, Remohí J. Oocyte vitrification as an efficient option for elective fertility preservation. Fertil Steril. (2016) 105:755–64.e8. doi: 10.1016/j.fertnstert.2015.11.027
12. Chen C. Pregnancy after human oocyte cryopreservation. Lancet. (1986) 327:884–6. doi: 10.1016/S0140-6736(86)90989-X
13. Kazem R, Thompson LA, Srikantharajah A, Laing MA, Hamilton MPR, Temple A. Cryopreservation of human oocytes and fertilization by two techniques: in-vitro fertilization and intracytoplasmic sperm injection. Hum Reprod. (1995) 10:2650–4. doi: 10.1093/oxfordjournals.humrep.a135761
14. Bernard A, Fuller BJ. Cryopreservation of human oocytes: a review of current problems and perspectives. Hum Reprod Update. (1996) 2:193–207. doi: 10.1093/humupd/2.3.193
15. Porcu E, Fabbri R, Seracchioli R, Ciotti PM, Magrini O, Flamigni C. Birth of a healthy female after intracytoplasmic sperm injection of cryopreserved human oocytes. Fertil Steril. (1997) 68:724–6. doi: 10.1016/s0015-0282(97)00268-9
16. Kuleshova L, Gianaroli L, Magli C, Ferraretti A, Trounson A. Birth following vitrification of a small number of human oocytes. Hum Reprod. (1999) 14:3077–9. doi: 10.1093/humrep/14.12.3077
17. Kuwayama M, Vajta G, Kato O, Leibo SP. Highly efficient vitrification method for cryopreservation of human oocytes. Reprod Biomed Online. (2005) 11:300–8. doi: 10.1016/S1472-6483(10)60837-1
18. Jain JK, Paulson RJ. Oocyte cryopreservation. Fertil Steril. (2006) 86:1037–46. doi: 10.1016/j.fertnstert.2006.07.1478
19. Grifo JA, Noyes N. Delivery rate using cryopreserved oocytes is comparable to conventional in vitro fertilization using fresh oocytes: potential fertility preservation for female cancer patients. Fertil Steril. (2010) 93:391–6. doi: 10.1016/j.fertnstert.2009.02.067
20. Masrat-Un-Nisa, Malik AA, Sofi KA, Khatun A, Yousuf N. Recent advancements in vitrification cryodevices for gamete and gonadal tissue. Cryoletters. (2022) 43:129–39. doi: 10.54680/fr22310110112
21. Abdullah KAL, Atazhanova T, Chavez-Badiola A, Shivhare SB. Automation in ART: Paving the Way for the Future of Infertility Treatment. Reprod Sci. (2022). doi: 10.1007/s43032-022-00941-y
22. Trounson A, Mohr L. Human pregnancy following cryopreservation, thawing and transfer of an eight-cell embryo. Nature. (1983) 305:707–9. doi: 10.1038/305707a0
23. Zeilmaker GH, Alberda AT, van Gent I, Rijkmans CM, Drogendijk AC. Two pregnancies following transfer of intact frozen-thawed embryos. Fertil Steril. (1984) 42:293–6. doi: 10.1016/S0015-0282(16)48029-5
24. Sakkas D, Shoukir Y, Chardonnens D, Bianchi PG, Campana A. Early cleavage of human embryos to the two-cell stage after intracytoplasmic sperm injection as an indicator of embryo viability. Hum Reprod. (1998) 13:182–7. doi: 10.1093/humrep/13.1.182
25. Lane M, Schoolcraft WB, Gardner DK, Phil D. Vitrification of mouse and human blastocysts using a novel cryoloop container-less technique. Fertil Steril. (1999) 72:1073–8. doi: 10.1016/S0015-0282(99)00418-5
26. Mukaida T, Nakamura S, Tomiyama T, Wada S, Kasai M, Takahashi K. Successful birth after transfer of vitrified human blastocysts with use of a cryoloop containerless technique. Fertil Steril. (2001) 76:618–20. doi: 10.1016/S0015-0282(01)01968-9
27. Roy TK, Brandi S, Tappe NM, Bradley CK, Vom E, Henderson C, et al. Embryo vitrification using a novel semi-automated closed system yields in vitro outcomes equivalent to the manual Cryotop method. Hum Reprod. (2014) 29:2431–8. doi: 10.1093/humrep/deu214
28. Polge C, Smith A, Parkes A. Revival of spermatozoa after vitrification and dehydration at low temperatures. Nature. (1949) 164:666. doi: 10.1038/164666a0
29. Bunge R, Sherman J. Fertilizing capacity of frozen human spermatozoa. Nature. (1953) 172:767–8. doi: 10.1038/172767b0
30. Bunge R, Keettel W, Sherman J. Clinical use of frozen semen—report of four cases. Fertil Steril. (1954) 5:520–9. doi: 10.1016/S0015-0282(16)31802-7
31. Sherman JK. Preservation of bull and human spermatozoa by freezing in liquid nitrogen vapour. Nature. (1962) 194:1291–2. doi: 10.1038/1941291a0
32. Park NC. Sperm bank: from laboratory to patient. World J Mens Health. (2018) 36:89–91. doi: 10.5534/wjmh.182002
33. Devroey P, Silber S, Nagy Z, Liu J, Tournaye H, Joris H, et al. Ongoing pregnancies and birth after intracytoplasmic sperm injection with frozen-thawed epididymal spermatozoa. Hum Reprod. (1995) 10:9–12. doi: 10.1093/oxfordjournals.humrep.a136058
34. Gil-Salom M, Romero J, Mínguez Y, Rubio C, de los Santos M, Remohí J, et al. Pregnancies after intracytoplasmic sperm injection with cryopreserved testicular spermatozoa. Hum Reprod. (1996) 11:1309–13. doi: 10.1093/oxfordjournals.humrep.a019377
35. Brotherton J. Cryopreservation of human semen. Arch Androl. (1990) 25:181–95. doi: 10.3109/01485019008987609
36. Storey BT, Noiles EE, Thompson KA. Comparison of glycerol, other polyols, trehalose, and raffinose to provide a defined cryoprotectant medium for mouse sperm cryopreservation. Cryobiology. (1998) 37:46–58. doi: 10.1006/cryo.1998.2097
37. di Santo M, Tarozzi N, Nadalini M, Borini A. Human sperm cryopreservation: Update on techniques, effect on DNA integrity, and implications for ART. Adv Urol. (2012) 2012:854837. doi: 10.1155/2012/854837
38. Liu F, Zou SS, Zhu Y, Sun C, Liu YF, Wang SS, et al. novel micro-straw for cryopreservation of small number of human spermatozoon. Asian J Androl. (2017) 19:326–9. doi: 10.4103/1008-682X.173452
39. Hudson JN, Stanley NB, Nahata L, Bowman-Curci M, Quinn GP. New promising strategies in oncofertility. Expert Rev Qual Life Cancer Care. (2017) 2:67–78. doi: 10.1080/23809000.2017.1308808
40. Bedoschi G, Oktay K. Current approach to fertility preservation by embryo cryopreservation. Fertil Steril. (2013) 99:1496–502. doi: 10.1016/j.fertnstert.2013.03.020
41. Edgar DH, Gook DA, A. critical appraisal of cryopreservation (slow cooling versus vitrification) of human oocytes and embryos. Hum Reprod Update. (2012) 18:536–54. doi: 10.1093/humupd/dms016
42. Levi Setti PE, Porcu E, Patrizio P, Vigiliano V, de Luca R, D'Aloja P, Spoletini R, Scaravelli G. Human oocyte cryopreservation with slow freezing versus vitrification. Results from the National Italian Registry data, 2007–2011. Fertil Steril. (2014) 102:90–95.e2. doi: 10.1016/j.fertnstert.2014.03.052
43. Li Z, Wang Y, Ledger W, Edgar D, Sullivan E. Clinical outcomes following cryopreservation of blastocysts by vitrification or slow freezing: a population-based cohort study. Hum Reprod. (2014) 29:2794–801. doi: 10.1093/humrep/deu246
44. Rienzi L, Gracia C, Maggiulli R, Labarbera AR, Kaser DJ, Ubaldi FM, et al. Oocyte, embryo and blastocyst cryopreservation in art: systematic review and meta-Analysis comparing slow-freezing versus vitrification to produce evidence for the development of global guidance. Hum Reprod Update. (2017) 23:139–55. doi: 10.1093/humupd/dmw038
45. Nagy ZP, Anderson RE, Feinberg EC, Hayward B, Mahony MC. The human oocyte preservation experience (HOPE) registry: Evaluation of cryopreservation techniques and oocyte source on outcomes. Reprod Biol Endocrinol. (2017) 15:10. doi: 10.1186/s12958-017-0228-7
46. Vanderzwalmen P, Ectors F, Panagiotidis Y, Schuff M, Murtinger M, Wirleitner B. The evolution of the cryopreservation techniques in reproductive medicine—exploring the character of the vitrified state intra- and extracellularly to better understand cell survival after cryopreservation. Reprod Med. (2020) 1:142–57. doi: 10.3390/reprodmed1020011
47. Khalili MA, Maione M, Palmerini MG, Bianchi S, Macchiarelli G, Nottola SA. Ultrastructure of human mature oocytes after vitrification. Eur J Histochem. (2012) 56:236–42. doi: 10.4081/ejh.2012.e38
48. Escribá MJ, Escobedo-Lucea C, Mercader A. de los Santos MJ, Pellicer A, Remohí J. Ultrastructure of preimplantation genetic diagnosis-derived human blastocysts grown in a coculture system after vitrification. Fertil Steril. (2006) 86:664–71. doi: 10.1016/j.fertnstert.2006.01.033
49. Elder K, Dale B. Cryopreservation of Gametes and Embryos. In:Elder K, Dale B, , editors. In-Vitro Fertilization. Cambridge: Cambridge University Press (2020). p. 254–283 doi: 10.1017/9781108611633.013
50. Nichi M, de Cassia Sávio Figueira R, de Almeida Ferreira Braga DP, Setti AS, Iaconelli A, Borges E. Decreased fertility in poor responder women is not related to oocyte morphological status. Arch Med Sci. (2011) 7:315–20. doi: 10.5114/aoms.2011.22084
51. McLachlan RI. Approach to the patient with oligozoospermia. J Clin Endocrinol Metab. (2013) 98:873–80. doi: 10.1210/jc.2012-3650
52. Fahy GM, Rall WF. Vitrification: an overview. In:Tucker M, Liebermann J, , editors. Vitrification in Assisted Reproduction. London: CRC Press (2013). p. 1–20 doi: 10.3109/9780203090022.001
53. Balaban B, Urman B, Ata B, Isiklar A, Larman MG, Hamilton R, et al. randomized controlled study of human Day 3 embryo cryopreservation by slow freezing or vitrification: Vitrification is associated with higher survival, metabolism and blastocyst formation. Hum Reprod. (2008) 23:1976–82. doi: 10.1093/humrep/den222
54. Warner RM, Ampo E, Nelson D, Benson JD, Eroglu A, Higgins AZ. Rapid quantification of multi-cryoprotectant toxicity using an automated liquid handling method. Cryobiology. (2021) 98:219–32. doi: 10.1016/j.cryobiol.2020.10.017
55. Faltus M, Bilavcik A, Zamecnik J. Vitrification ability of combined and single cryoprotective agents. Plants. (2021) 10:2392. doi: 10.3390/plants10112392
56. Konc J, Kanyó K, Kriston R, Somoski B, Cseh S. Cryopreservation of embryos and oocytes in human assisted reproduction. Biomed Res Int. (2014) 2014:307268. doi: 10.1155/2014/307268
57. Stachecki JJ, Garrisi J, Sabino S, Caetano JPJ, Wiemer KE, Cohen J, et al. new safe, simple and successful vitrification method for bovine and human blastocysts. Reprod Biomed Online. (2008) 17:360–7. doi: 10.1016/S1472-6483(10)60219-2
58. Celada P, Bosch E. Freeze-all, for whom, when, and how. Ups J Med Sci. (2020) 125:104–11. doi: 10.1080/03009734.2020.1746870
59. Belva F, Bonduelle M, Roelants M, Verheyen G, van Landuyt L. Neonatal health including congenital malformation risk of 1072 children born after vitrified embryo transfer. Hum Reprod. (2016) 31:1610–20. doi: 10.1093/humrep/dew103
60. Mukaida T, Oka C. Vitrification of oocytes, embryos and blastocysts. Best Pract Res Clin Obstet Gynaecol. (2012) 26:789–803. doi: 10.1016/j.bpobgyn.2012.07.001
61. Parmegiani L, Garello C, Revelli A, Criscuoli L, Dabizzi S, Gualtieri R, et al. Efficacy and efficiency of the “universal warming protocol”: multicenter randomized controlled study on human slow frozen oocytes. Fertil Steril. (2014) 102:e122. doi: 10.1016/j.fertnstert.2014.07.419
62. Parmegiani L, Arnone A, Bernardi S, Filicori M, Cognigni G, A. single “universal warming protocol” for vitrified human embryos: a randomized controlled study. Fertil Steril. (2017) 108:e173. doi: 10.1016/j.fertnstert.2017.07.515
63. Li J, Xiong S, Zhao Y, Li C, Han W, Huang G. Effect of the re-vitrification of embryos at different stages on embryonic developmental potential. Front Endocrinol (Lausanne). (2021) 12:653310. doi: 10.3389/fendo.2021.653310
64. Gallardo M, Saenz J, Risco R. Human oocytes and zygotes are ready for ultra-fast vitrification after 2 minutes of exposure to standard CPA solutions. Sci Rep. (2019) 9:15986. doi: 10.1038/s41598-019-52014-x
65. Vajta G, Rienzi L, Ubaldi FM. Open versus closed systems for vitrification of human oocytes and embryos. Reprod Biomed Online. (2015) 30:325–33. doi: 10.1016/j.rbmo.2014.12.012
66. Practice Committees of the American Society for Reproductive Medicine and Society of ReproductiveBiologists and Technologists. A review of best practices of rapid-cooling vitrification for oocytes and embryos: a committee opinion. Fertil Steril. (2021) 115:305–10. doi: 10.1016/j.fertnstert.2020.11.017
67. Quinn P. Vitrification of human oocytes with different tools. In:Chian RC, Quinn P, , editors. Fertility Cryopreservation. Cambridge: Cambridge University Press (2010). p. 131–143. doi: 10.1017/CBO9780511730207.017
68. Bonetti A, Cervi M, Tomei F, Marchini M, Ortolani F, Manno M. Ultrastructural evaluation of human metaphase II oocytes after vitrification: closed versus open devices. Fertil Steril. (2011) 95:928–35. doi: 10.1016/j.fertnstert.2010.08.027
69. Pujol A, Zamora MJ, Obradors A, Garcia D, Rodriguez A, Vassena R. Comparison of two different oocyte vitrification methods: a prospective, paired study on the same genetic background and stimulation protocol. Hum Reprod. (2019) 34:989–97. doi: 10.1093/humrep/dez045
70. Momozawa K, Matsuzawa A, Tokunaga Y, Abe S, Koyanagi Y, Kurita M, et al. Efficient vitrification of mouse embryos using the Kitasato Vitrification System as a novel vitrification device. Reprod Biol Endocrinol. (2017) 15:29. doi: 10.1186/s12958-017-0249-2
71. Marchetti IA, Cullere M, Beltramo M, Paturlanne JM, Martínez VS, Saggiorato L, et al. Preliminary development results of a new vitrification device for oocytes and embryos: Vitri Carrier from ZURE? Fertil Steril. (2022) 118:e125. doi: 10.1016/j.fertnstert.2022.08.371
72. Logsdon DM, Grimm CK, Schoolcraft WB, McCormick S, Schlenker T, Swain JE, et al. Evaluation of the TMRW vapor phase cryostorage platform using reproductive specimens and in vitro extended human embryo culture. F&S Sci. (2021) 2:268–77. doi: 10.1016/j.xfss.2021.06.005
73. MVE Cryopreservation for Life Science Storage and Transport Systems for MVE Cryopreservation Available online at: http://files.chartindustries.com/www.chartbiomed.com (accessed December 30, 2022).
74. Cobo A, Kuwayama M, Pérez S, Ruiz A, Pellicer A, Remohí J. Comparison of concomitant outcome achieved with fresh and cryopreserved donor oocytes vitrified by the Cryotop method. Fertil Steril. (2008) 89:1657–64. doi: 10.1016/j.fertnstert.2007.05.050
75. Gook DA. History of oocyte cryopreservation. Reprod Biomed Online. (2011) 23:281–9. doi: 10.1016/j.rbmo.2010.10.018
76. de Munck N, Vajta G. Safety and efficiency of oocyte vitrification. Cryobiology. (2017) 78:119–27. doi: 10.1016/j.cryobiol.2017.07.009
77. Anifandis G, Taylor TH, Messini CI, Chatzimeletiou K, Daponte A, Ioannou D, et al. The impact of SARS-CoV-2 on sperm cryostorage, theoretical or real risk? Medicina (B Aires). (2021) 57:946. doi: 10.3390/medicina57090946
78. Cobo A, Bellver J, de Los Santos MJ, Remohí J. Viral screening of spent culture media and liquid nitrogen samples of oocytes and embryos from hepatitis B, hepatitis C, and human immunodeficiency virus chronically infected women undergoing in vitro fertilization cycles. Fertil Steril. (2012) 97:74–8. doi: 10.1016/j.fertnstert.2011.10.006
79. Molina I, Mari M, Martínez JV, Novella-Maestre E, Pellicer N, Pemán J. Bacterial and fungal contamination risks in human oocyte and embryo cryopreservation: open vs. closed vitrification systems. Fertil Steril. (2016) 106:127–32. doi: 10.1016/j.fertnstert.2016.03.024
80. Porcu E, Tranquillo ML, Notarangelo L, Ciotti PM, Calza N, Zuffa S, et al. High-security closed devices are efficient and safe to protect human oocytes from potential risk of viral contamination during vitrification and storage especially in the COVID-19 pandemic. J Assist Reprod Genet. (2021) 38:681–8. doi: 10.1007/s10815-021-02062-y
81. Rosen M, Yang X, Marsh P, Runge A, Olivera G, Ribeiro S, et al. Gamete and embryo manipulation. In:Strauss JF III, Barbieri RL, , editors. Yen & Jaffe's Reproductive Endocrinology: Physiology, Pathophysiology, and Clinical Management: Eighth Edition. Amsterdam, Netherlands: Elsevier (2019). p. 823-856.e14 doi: 10.1016/B978-0-323-47912-7.00032-9
82. Rios AP, Botella IM. Description and outcomes of current clinical techniques for sperm cryopreservation. EMJ Repro Health. (2019) 7:79–92. doi: 10.33590/emjreprohealth/10310343
83. Behrman SJ, Sawada Y. Heterologous and homologous inseminations with human semen frozen and stored in a liquid-nitrogen refrigerator. Fertil Steril. (1966) 17:457–66. doi: 10.1016/S0015-0282(16)36003-4
84. Li Y, Zhou L, Lv M, Ge P, Liu Y, Zhou D. Vitrification and conventional freezing methods in sperm cryopreservation: a systematic review and meta-analysis. Eur J Obstet Gynecol Reprod Biol. (2019) 233:84–92. doi: 10.1016/j.ejogrb.2018.11.028
85. Huang WJ, Zhang D, Hong ZW, Chen ZB, Dong LH, Zhang Y, et al. Sequential interval micro-droplet loading in closed hemi-straw carrier system: a convenient and efficient method for ultra-rapid cryopreservation in extreme oligozoospermia. Cryobiology. (2020) 93:75–83. doi: 10.1016/j.cryobiol.2020.02.005
86. Paoli D, Lombardo F, Lenzi A, Gandini L. Sperm cryopreservation: Effects on chromatin structure. Advances in Experimental Medicine and Biology. New York: Springer New York LLC (2014). p. 137–150 doi: 10.1007/978-1-4614-7783-9_9
87. Sutton RL. Critical cooling rates for aqueous cryoprotectants in the presence of sugars and polysaccharides. Cryobiology. (1992) 29:585–98. doi: 10.1016/0011-2240(92)90063-8
88. Alvarez JG, Storey BT. Differential incorporation of fatty acids into and peroxidative loss of fatty acids from phospholipids of human spermatozoa. Mol Reprod Dev. (1995) 42:334–46. doi: 10.1002/mrd.1080420311
89. Hammadeh ME, Askari AS, Georg T, Rosenbaum P, Schmidt W. Effect of freeze-thawing procedure on chromatin stability, morphological alteration and membrane integrity of human spermatozoa in fertile and subfertile men. Int J Androl. (1999) 22:155–62. doi: 10.1046/j.1365-2605.1999.00162.x
90. Said TM, Gaglani A, Agarwal A. Implication of apoptosis in sperm cryoinjury. Reprod Biomed Online. (2010) 21:456–62. doi: 10.1016/j.rbmo.2010.05.011
91. Stanic P, Tandara M, Sonicki Z, Simunic V, Radakovic B, Suchanek E. Comparison of protective media and freezing techniques for cryopreservation of human semen. Eur J Obstet Gynecol Reprod Biol. (2000) 91:65–70. doi: 10.1016/S0301-2115(99)00255-9
92. Morris GJ. Rapidly cooled human sperm: no evidence of intracellular ice formation. Hum Reprod. (2006) 21:2075–83. doi: 10.1093/humrep/del116
93. Schulz M, Risopatrón J, Uribe P, Isachenko E, Isachenko V, Sánchez R. Human sperm vitrification: a scientific report. Andrology. (2020) 8:1642–50. doi: 10.1111/andr.12847
94. Sherman J. Cryopreservation of human semen. In:Keel BA, Webster BW, , editors. Handbook of the Laboratory Diagnosis and Treatment of Infertility. Boca Raton, FL: (1990). p. 229–260
95. WHO laboratory manual for the examination and processing of human semen - Sixth Edition. (2021). Available online at: https://www.who.int/publications/i/item/9789240030787 (accessed February 13, 2023).
96. Li X, Ni M, Xing S, Yu Y, Zhou Y, Yang S, et al. Reactive oxygen species secreted by leukocytes in semen induce self-expression of interleukin-6 and affect sperm quality. Am J Mens Health. (2020) 14:1557988320970053. doi: 10.1177/1557988320970053
97. Castleton PE, Deluao JC, Sharkey DJ, McPherson NO. Measuring reactive oxygen species in semen for male preconception care: a scientist perspective. Antioxidants. (2022) 11:264. doi: 10.3390/antiox11020264
98. Pabón D, Meseguer M, Sevillano G, Cobo A, Romero JL, Remohí J. de los Santos MJ. A new system of sperm cryopreservation: evaluation of survival, motility, DNA oxidation, and mitochondrial activity. Andrology. (2019) 7:293–301. doi: 10.1111/andr.12607
99. Tao Y, Sanger E, Saewu A, Leveille MC. Human sperm vitrification: the state of the art. Reprod Biol Endocrinol. (2020) 18:17. doi: 10.1186/s12958-020-00580-5
100. O'Neill HC, Nikoloska M, Ho HT, Doshi A, Maalouf W. Improved cryopreservation of spermatozoa using vitrification: comparison of cryoprotectants and a novel device for long-term storage. J Assist Reprod Genet. (2019) 36:1713–20. doi: 10.1007/s10815-019-01505-x
101. Isachenko V, Rahimi G, Mallmann P, Sanchez R, Isachenko E. Technologies of cryoprotectant-free vitrification of human spermatozoa: asepticity as criterion of effectiveness. Andrology. (2017) 5:1055–63. doi: 10.1111/andr.12414
102. Agha-Rahimi A, Khalili MA, Nottola SA, Miglietta S, Moradi A. Cryoprotectant-free vitrification of human spermatozoa in new artificial seminal fluid. Andrology. (2016) 4:1037–44. doi: 10.1111/andr.12212
103. Isachenko E, Isachenko V, Weiss JM, Kreienberg R, Katkov II, Schulz M, et al. Acrosomal status and mitochondrial activity of human spermatozoa vitrified with sucrose. Reproduction. (2008) 136:167–73. doi: 10.1530/REP-07-0463
104. Isachenko E, Isachenko V, Katkov II, Dessole S, Nawroth F. Vitrification of mammalian spermatozoa in the absence of cryoprotectants: From past practical difficulties to present success. Reprod Biomed Online. (2003) 6:191–200. doi: 10.1016/S1472-6483(10)61710-5
105. Slabbert M, du Plessis SS, Huyser C. Large volume cryoprotectant-free vitrification: An alternative to conventional cryopreservation for human spermatozoa. Andrologia. (2015) 47:594–9. doi: 10.1111/and.12307
106. Aizpurua J, Medrano L, Enciso M, Sarasa J, Romero A, Fernández MA, et al. New permeable cryoprotectant-free vitrification method for native human sperm. Hum Reprod. (2017) 32:2007–15. doi: 10.1093/humrep/dex281
107. Schulz M, Risopatrón J, Matus G, Pineda E, Rojas C, Isachenko V, et al. Trehalose sustains a higher post-thaw sperm motility than sucrose in vitrified human sperm. Andrologia. (2017) 49:e12757. doi: 10.1111/and.12757
108. Liu J, Tanrikut C, Wright DL, Lee GY, Toner M, Biggers JD, et al. Cryopreservation of human spermatozoa with minimal non-permeable cryoprotectant. Cryobiology. (2016) 73:162–7. doi: 10.1016/j.cryobiol.2016.08.004
109. Merino O, Aguagüiña WE, Esponda P, Risopatrón J, Isachenko E, Isachenko V, et al. Protective effect of butylated hydroxytoluene on sperm function in human spermatozoa cryopreserved by vitrification technique. Andrologia. (2015) 47:186–93. doi: 10.1111/and.12246
110. Isachenko V, Isachenko E, Petrunkina AM, Sanchez R. Human spermatozoa vitrified in the absence of permeable cryoprotectants: birth of two healthy babies. Reprod Fertil Dev. (2012) 24:323–6. doi: 10.1071/RD11061
111. Isachenko V, Maettner R, Petrunkina AM, Sterzik K, Mallmann P, Rahimi G, et al. Vitrification of human ICSI/IVF spermatozoa without cryoprotectants: new capillary technology. J Androl. (2012) 33:462–8. doi: 10.2164/jandrol.111.013789
112. Isachenko V, Isachenko E, Katkov II, Montag M, Dessole S, Nawroth F, et al. Cryoprotectant-free cryopreservation of human spermatozoa by vitrification and freezing in vapor: effect on motility, DNA integrity, and fertilization ability. Biol Reprod. (2004) 71:1167–73. doi: 10.1095/biolreprod.104.028811
113. Agha-Rahimi A, Khalili MA, Nabi A, Ashourzadeh S. Vitrification is not superior to rapid freezing of normozoospermic spermatozoa: effects on sperm parameters, DNA fragmentation and hyaluronan binding. Reprod Biomed Online. (2014) 28:352–8. doi: 10.1016/j.rbmo.2013.11.015
114. Hu H, Liu R, Shi X, Ji G, Zhang J, Zhang H, et al. Comparison of rapid freezing and vitrification for human sperm cryopreservation using trehalose as a cryoprotective agent. Open J Obstet Gynecol. (2019) 09:1407–18. doi: 10.4236/ojog.2019.910136
115. Isachenko V, Isachenko E, Montag M, Zaeva V, Krivokharchenko I, Nawroth F, et al. Clean technique for cryoprotectant-free vitrification of human spermatozoa. Reprod Biomed Online. (2005) 10:350–4. doi: 10.1016/S1472-6483(10)61795-6
116. Joaquim DC, Borges ED, Viana IGR, Navarro PA, Vireque AA. Risk of contamination of gametes and embryos during cryopreservation and measures to prevent cross-contamination. Biomed Res Int. (2017) 2017:1840417. doi: 10.1155/2017/1840417
117. Hu J, Zhao S, Xu C, Zhang L, Lu S, Cui L, et al. Liquid nitrogen vapor is comparable to liquid nitrogen for storage of cryopreserved human sperm: evidence from the characteristics of post-thaw human sperm. Fertil Steril. (2015) 104:1253–7.e2. doi: 10.1016/j.fertnstert.2015.07.1140
118. Paffoni A, Palini S. There is another new method for cryopreserving small numbers of human sperm cells. Ann Transl Med. (2019) 7:S17. doi: 10.21037/atm.2019.01.52
119. Abdelhafez F, Bedaiwy M, El-Nashar SA, Sabanegh E, Desai N. Techniques for cryopreservation of individual or small numbers of human spermatozoa: a systematic review. Hum Reprod Update. (2009) 15:153–64. doi: 10.1093/humupd/dmn061
120. Berkovitz A, Miller N, Silberman M, Belenky M, Itsykson P. A novel solution for freezing small numbers of spermatozoa using a sperm vitrification device. Hum Reprod. (2018) 33:1975–83. doi: 10.1093/humrep/dey304
121. Hu E, Childress W, Tiersch TR. 3-D printing provides a novel approach for standardization and reproducibility of freezing devices. Cryobiology. (2017) 76:34–40. doi: 10.1016/j.cryobiol.2017.03.010
122. Shah D. Rasappan, Shila, Gunasekaran K. A simple method of human sperm vitrification. MethodsX. (2019) 6:2198–204. doi: 10.1016/j.mex.2019.09.022
123. Ladanyi C, Mor A, Christianson MS, Dhillon N, Segars JH. Recent advances in the field of ovarian tissue cryopreservation and opportunities for research. J Assist Reprod Genet. (2017) 34:709–22. doi: 10.1007/s10815-017-0899-1
124. Gellert SE, Pors SE, Kristensen SG, Bay-Bjørn AM, Ernst E, Yding Andersen C. Transplantation of frozen-thawed ovarian tissue: an update on worldwide activity published in peer-reviewed papers and on the Danish cohort. J Assist Reprod Genet. (2018) 35:561–70. doi: 10.1007/s10815-018-1144-2
125. Amorim CA, Leonel ECR, Afifi Y, Coomarasamy A, Fishel S. Cryostorage and retransplantation of ovarian tissue as an infertility treatment. Best Pract Res Clin Endocrinol Metab. (2019) 33:89–102. doi: 10.1016/j.beem.2018.09.002
126. Dolmans MM, Donnez J. Fertility preservation in women for medical and social reasons: Oocytes vs ovarian tissue. Best Pract Res Clin Obstet Gynaecol. (2021) 70:63–80. doi: 10.1016/j.bpobgyn.2020.06.011
127. Sheshpari S, Shahnazi M, Mobarak H, Ahmadian S, Bedate AM, Nariman-Saleh-Fam Z, et al. Ovarian function and reproductive outcome after ovarian tissue transplantation: a systematic review. J Transl Med. (2019) 17:396. doi: 10.1186/s12967-019-02149-2
128. Diaz AA, Kubo H, Handa N, Hanna M, Laronda MM. A systematic review of ovarian tissue transplantation outcomes by ovarian tissue processing size for cryopreservation. Front Endocrinol (Lausanne). (2022) 13:918899. doi: 10.3389/fendo.2022.918899
129. Donnez J, Dolmans M-M. Fertility preservation in women. New Engl J Med. (2017) 377:1657–65. doi: 10.1056/NEJMra1614676
130. Demeestere I, Simon P, Dedeken L, Moffa F, Tsépélidis S, Brachet C, et al. Live birth after autograft of ovarian tissue cryopreserved during childhood. Hum Reprod. (2015) 30:2107–9. doi: 10.1093/humrep/dev128
131. Matthews SJ, Picton H, Ernst E, Andersen CY. Successful pregnancy in a woman previously suffering from β-thalassemia following transplantation of ovarian tissue cryopreserved before puberty. Minerva Ginecol. (2018) 70:432–5. doi: 10.23736/S0026-4784.18.04240-5
132. Thomson AB, Campbell AJ, Kelnar CJH, Wallace WHB. Semen quality and spermatozoal DNA integrity in survivors of childhood cancer: a case-control study. Lancet. (2002) 360:361–7. doi: 10.1016/S0140-6736(02)09606-X
133. Tournaye H, Dohle GR, Barratt CLR. Fertility preservation in men with cancer. Lancet. (2014) 384:1295–301. doi: 10.1016/S0140-6736(14)60495-5
134. Onofre J, Baert Y, Faes K, Goossens E. Cryopreservation of testicular tissue or testicular cell suspensions: a pivotal step in fertility preservation. Hum Reprod Update. (2016) 22:744–61. doi: 10.1093/humupd/dmw029
135. Oktay KH, Yih M. Preliminary experience with orthotopic and heterotopic transplantation of ovarian cortical strips. Semin Reprod Med. (2002) 20:63–74. doi: 10.1055/s-2002-23520
136. Angarita AM, Johnson CA, Fader AN, Christianson MS. Fertility preservation: a key survivorship issue for young women with cancer. Front Oncol. (2016) 6:102. doi: 10.3389/fonc.2016.00102
137. McLaren JF, Bates GW. Fertility preservation in women of reproductive age with cancer. Am J Obstet Gynecol. (2012) 207:455–62. doi: 10.1016/j.ajog.2012.08.013
138. Donnez J, Dolmans MM. Ovarian cortex transplantation: 60 reported live births brings the success and worldwide expansion of the technique towards routine clinical practice. J Assist Reprod Genet. (2015) 32:1167–70. doi: 10.1007/s10815-015-0544-9
139. Bromer JG, Patrizio P. Fertility preservation: the rationale for cryopreservation of the whole ovary. Semin Reprod Med. (2009) 27:465–71. doi: 10.1055/s-0029-1241056
140. Arav A, Patrizio P. Techniques of cryopreservation for ovarian tissue and whole ovary. Clin Med Insights Reprod Health. (2019) 13:117955811988494. doi: 10.1177/1179558119884945
141. Rivas Leonel EC, Lucci CM, Amorim CA. Cryopreservation of human ovarian tissue: a review. Transfus Med Hemother. (2019) 46:173–81. doi: 10.1159/000499054
142. Gosden RG, Baird DT, Wade JC, Webb R. Restoration of fertility to oophorectomized sheep by ovarian autografts stored at −196°C. Hum Reprod. (1994) 9:597–603. doi: 10.1093/oxfordjournals.humrep.a138556
143. Isachenko V, Todorov P, Isachenko E, Rahimi G, Tchorbanov A, Mihaylova N, et al. Long-time cooling before cryopreservation decreased translocation of phosphatidylserine (Ptd-L-Ser) in human ovarian tissue. PLoS ONE. (2015) 10:e0129108. doi: 10.1371/journal.pone.0129108
144. Wang W, Salama M, Todorov P, Spitkovsky D, Isachenko E, Bongaarts R, et al. New method of FACS analyzing and sorting of intact whole ovarian fragments (COPAS) after long time (24 h) cooling to 5°C before cryopreservation. Cell Tissue Bank. (2021) 22:487–98. doi: 10.1007/s10561-020-09898-1
145. Gallardo M, Paulini F, Corral A, Balcerzyk M, Lucci CM, Ambroise J, et al. Evaluation of a new freezing protocol containing 20% dimethyl sulphoxide concentration to cryopreserve human ovarian tissue. Reprod Biomed Online. (2018) 37:653–65. doi: 10.1016/j.rbmo.2018.09.012
146. Maffei S, Pennarossa G, Brevini TAL, Arav A, Gandolfi F. Beneficial effect of directional freezing on in vitro viability of cryopreserved sheep whole ovaries and ovarian cortical slices. Hum Reprod. (2014) 29:114–24. doi: 10.1093/humrep/det377
147. Shi Q, Xie Y, Wang Y, Li S. Vitrification versus slow freezing for human ovarian tissue cryopreservation: a systematic review and meta-anlaysis. Sci Rep. (2017) 7:8538. doi: 10.1038/s41598-017-09005-7
148. Kawamura K, Cheng Y, Suzuki N, Deguchi M, Sato Y, Takae S, et al. Hippo signaling disruption and Akt stimulation of ovarian follicles for infertility treatment. Proc Natl Acad Sci U S A. (2013) 110:17474–9. doi: 10.1073/pnas.1312830110
149. Suzuki N, Yoshioka N, Takae S, Sugishita Y, Tamura M, Hashimoto S, et al. Successful fertility preservation following ovarian tissue vitrification in patients with primary ovarian insufficiency. Hum Reprod. (2015) 30:608–15. doi: 10.1093/humrep/deu353
150. Amorim CA, David A, van Langendonckt A, Dolmans MM, Donnez J. Vitrification of human ovarian tissue: effect of different solutions and procedures. Fertil Steril. (2011) 95:1094–7. doi: 10.1016/j.fertnstert.2010.11.046
151. Huang L, Mo Y, Wang W, Li Y, Zhang Q, Yang D. Cryopreservation of human ovarian tissue by solid-surface vitrification. Eur J Obstet Gynecol Reprod Biol. (2008) 139:193–8. doi: 10.1016/j.ejogrb.2008.03.002
152. Li Y-B, Zhou C-Q, Yang G-F, Qiong W, Yu D. Modified vitrification method for cryopreservation of human ovarian tissues. Chin Med J. (2007) 120:110–4. doi: 10.1097/00029330-200701020-00007
153. Gandolfi F, Paffoni A, Papasso Brambilla E, Bonetti S, Brevini TAL, Ragni G. Efficiency of equilibrium cooling and vitrification procedures for the cryopreservation of ovarian tissue: comparative analysis between human and animal models. Fertil Steril. (2006) 85:1150–6. doi: 10.1016/j.fertnstert.2005.08.062
154. Sugishita Y, Taylan E, Kawahara T, Shahmurzada B, Suzuki N, Oktay K. Comparison of open and a novel closed vitrification system with slow freezing for human ovarian tissue cryopreservation. J Assist Reprod Genet. (2021) 38:2723–33. doi: 10.1007/s10815-021-02297-9
155. Xiao Z, Zhang Y, Fan W. Cryopreservation of human ovarian tissue using the silver closed vitrification system. J Assist Reprod Genet. (2017) 34:1435–44. doi: 10.1007/s10815-017-1004-5
156. Leonel ECR, Corral A, Risco R, Camboni A, Taboga SR, Kilbride P, et al. Stepped vitrification technique for human ovarian tissue cryopreservation. Sci Rep. (2019) 9:20008. doi: 10.1038/s41598-019-56585-7
157. Barbato V, Gualtieri R, Capriglione T, Pallotta MM, Braun S, di Nardo M, et al. Slush nitrogen vitrification of human ovarian tissue does not alter gene expression and improves follicle health and progression in long-term in vitro culture. Fertil Steril. (2018) 110:1356–66. doi: 10.1016/j.fertnstert.2018.08.020
158. Talevi R, Barbato V, Fiorentino I, Braun S, de Stefano C, Ferraro R, Sudhakaran S, Gualtieri R. Successful slush nitrogen vitrification of human ovarian tissue. Fertil Steril. (2016) 105:1523–31.e1. doi: 10.1016/j.fertnstert.2016.01.043
159. Kristensen SG, Liu Q, Mamsen LS, Greve T, Pors SE, Bjørn AB, et al. simple method to quantify follicle survival in cryopreserved human ovarian tissue. Hum Reprod. (2018) 33:2276–84. doi: 10.1093/humrep/dey318
160. Meirow D, Levron J, Eldar-Geva T, Hardan I, Fridman E, Zalel Y, et al. Pregnancy after transplantation of cryopreserved ovarian tissue in a patient with ovarian failure after chemotherapy. N Engl J Med. (2005) 353:318–21. doi: 10.1056/NEJMc055237
161. Silber SJ, Derosa M, Goldsmith S, Fan Y, Castleman L, Melnick J. Cryopreservation and transplantation of ovarian tissue: results from one center in the USA. J Assist Reprod Genet. (2018) 35:2205–13. doi: 10.1007/s10815-018-1315-1
162. Lunding SA, Pors SE, Kristensen SG, Landersoe SK, Jeppesen JV, Flachs EM, et al. Biopsying, fragmentation and autotransplantation of fresh ovarian cortical tissue in infertile women with diminished ovarian reserve. Hum Reprod. (2019) 34:1924–36. doi: 10.1093/humrep/dez152
163. Anderson RA, Wallace WHB, Telfer EE. Ovarian tissue cryopreservation for fertility preservation: clinical and research perspectives. Hum Reprod Open. (2017) 2017:hox001. doi: 10.1093/hropen/hox001
164. Huang JYJ, Tulandi T, Holzer H, Tan SL, Chian RC. Combining ovarian tissue cryobanking with retrieval of immature oocytes followed by in vitro maturation and vitrification: an additional strategy of fertility preservation. Fertil Steril. (2008) 89:567–72. doi: 10.1016/j.fertnstert.2007.03.090
165. Smitz I, Dolmans MM, Donnez J, Fortune JE, Hovatta O, Jewgenow K, et al. Current achievements and future research directions in ovarian tissue culture, in vitro follicle development and transplantation: Implications for fertility preservation. Hum Reprod Update. (2010) 16:395–414. doi: 10.1093/humupd/dmp056
166. Xiao S, Zhang J, Romero MM, Smith KN, Shea LD, Woodruff TK. In vitro follicle growth supports human oocyte meiotic maturation. Sci Rep. (2015) 5:17323. doi: 10.1038/srep17323
167. Telfer EE, Andersen CY. In vitro growth and maturation of primordial follicles and immature oocytes. Fertil Steril. (2021) 115:1116–25. doi: 10.1016/j.fertnstert.2021.03.004
168. McLaughlin M, Albertini DF, Wallace WHB, Anderson RA, Telfer EE. Metaphase II oocytes from human unilaminar follicles grown in a multistep culture system. Mol Hum Reprod. (2018) 24:135–42. doi: 10.1093/molehr/gay002
169. Dolmans M-M, Amorim CA. Construction and use of artificial ovaries. Reproduction. (2019) 158:F15–25. doi: 10.1530/REP-18-0536
170. Chen J, Torres-de la Roche LA, Kahlert UD, Isachenko V, Huang H, Hennefründ J, et al. Artificial ovary for young female breast cancer patients. Front Med (Lausanne). (2022) 9:837022. doi: 10.3389/fmed.2022.837022
171. Luyckx V, Dolmans MM, Vanacker J, Legat C, Fortuño Moya C, Donnez J, et al. new step toward the artificial ovary: Survival and proliferation of isolated murine follicles after autologous transplantation in a fibrin scaffold. Fertil Steril. (2014) 101:1149–56. doi: 10.1016/j.fertnstert.2013.12.025
172. Chiti MC, Dolmans MM, Orellana R, Soares M, Paulini F, Donnez J, et al. Influence of follicle stage on artificial ovary outcome using fibrin as a matrix. Hum Reprod. (2016) 31:427–35. doi: 10.1093/humrep/dew254
173. Paulini F, Vilela JMV, Chiti MC, Donnez J, Jadoul P, Dolmans MM, et al. Survival and growth of human preantral follicles after cryopreservation of ovarian tissue, follicle isolation and short-term xenografting. Reprod Biomed Online. (2016) 33:425–32. doi: 10.1016/j.rbmo.2016.05.003
174. Laronda MM, Rutz AL, Xiao S, Whelan KA, Duncan FE, Roth EW, et al. bioprosthetic ovary created using 3D printed microporous scaffolds restores ovarian function in sterilized mice. Nat Commun. (2017) 8:15261. doi: 10.1038/ncomms15261
175. Laronda MM, Jakus AE, Whelan KA, Wertheim JA, Shah RN, Woodruff TK. Initiation of puberty in mice following decellularized ovary transplant. Biomaterials. (2015) 50:20–9. doi: 10.1016/j.biomaterials.2015.01.051
176. Hassanpour A, Talaei-Khozani T, Kargar-Abarghouei E, Razban V, Vojdani Z. Decellularized human ovarian scaffold based on a sodium lauryl ester sulfate (SLES)-treated protocol, as a natural three-dimensional scaffold for construction of bioengineered ovaries. Stem Cell Res Ther. (2018) 9:252. doi: 10.1186/s13287-018-0971-5
177. Pors SE, Ramløse M, Nikiforov D, Lundsgaard K, Cheng J, Yding Andersen C, et al. Initial steps in reconstruction of the human ovary: Survival of pre-antral stage follicles in a decellularized human ovarian scaffold. Hum Reprod. (2019) 34:1523–35. doi: 10.1093/humrep/dez077
178. Donfack NJ, Alves KA, Araújo VR, Cordova A, Figueiredo JR, Smitz J, et al. Expectations and limitations of ovarian tissue transplantation. Zygote. (2017) 25:391–403. doi: 10.1017/S0967199417000338
179. Eijkenboom L, Saedt E, Zietse C, Braat D, Beerendonk C, Peek R. Strategies to safely use cryopreserved ovarian tissue to restore fertility after cancer: a systematic review. Reprod Biomed Online. (2022) 45:763–78. doi: 10.1016/j.rbmo.2022.05.020
180. Diáz-Garciá C, Herraiz S, Such E, Andrés MDM, Villamón E, Mayordomo-Aranda E, et al. Dexamethasone does not prevent malignant cell reintroduction in leukemia patients undergoing ovarian transplant: Risk assessment of leukemic cell transmission by a xenograft model. Hum Reprod. (2019) 34:1485–93. doi: 10.1093/humrep/dez115
181. Peek R, Bastings L, Westphal JR, Massuger LFAG, Braat DDM, Beerendonk CCM, et al. preliminary study on a new model system to evaluate tumour-detection and tumour-purging protocols in ovarian cortex tissue intended for fertility preservation. Hum Reprod. (2015) 30:870–6. doi: 10.1093/humrep/dev013
182. Eijkenboom L, Palacio-Castañeda V, Groenman F, Braat D, Beerendonk C, Brock R, et al. Assessing the use of tumor-specific DARPin-toxin fusion proteins for ex vivo purging of cancer metastases from human ovarian cortex before autotransplantation. F&S Science. (2021) 2:330–44. doi: 10.1016/j.xfss.2021.09.004
183. Mulder CL, Eijkenboom LL, Beerendonk CCM, Braat DDM, Peek R. Enhancing the safety of ovarian cortex autotransplantation: Cancer cells are purged completely from human ovarian tissue fragments by pharmacological inhibition of YAP/TAZ oncoproteins. Hum Reprod. (2019) 34:506–18. doi: 10.1093/humrep/dey384
184. Peek R, Eijkenboom LL, Braat DDM, Beerendonk CCM. Complete purging of ewing sarcoma metastases from human ovarian cortex tissue fragments by inhibiting the mtorc1 signaling pathway. J Clin Med. (2021) 10:4362. doi: 10.3390/jcm10194362
185. Eijkenboom L, Mulder C, van der Reijden B, van Mello N, van Leersum J, Koorenhof-Scheele T, et al. Purging human ovarian cortex of contaminating leukaemic cells by targeting the mitotic catastrophe signalling pathway. J Assist Reprod Genet. (2021) 38:1571–88. doi: 10.1007/s10815-021-02081-9
186. Tran KTD, Valli-Pulaski H, Colvin A, Orwig KE. Male fertility preservation and restoration strategies for patients undergoing gonadotoxic therapies. Biol Reprod. (2022) 107:382–405. doi: 10.1093/biolre/ioac072
187. Baert Y, van Saen D, Haentjens P. In't Veld P, Tournaye H, Goossens E. What is the best cryopreservation protocol for human testicular tissue banking? Hum Reprod. (2013) 28:1816–26. doi: 10.1093/humrep/det100
188. Moraveji SF, Esfandiari F, Sharbatoghli M, Taleahmad S, Nikeghbalian S, Shahverdi A, et al. Optimizing methods for human testicular tissue cryopreservation and spermatogonial stem cell isolation. J Cell Biochem. (2019) 120:613–21. doi: 10.1002/jcb.27419
189. Kabiri D, Safrai M, Gropp M, Hidas G, Mordechai-Daniel T, Meir K, et al. Establishment of a controlled slow freezing-based approach for experimental clinical cryopreservation of human prepubertal testicular tissues. F S Rep. (2021) 3:47–56. doi: 10.1016/j.xfre.2021.11.001
190. Picton HM, Wyns C, Anderson RA, Goossens E, Jahnukainen K, Kliesch S, et al. A European perspective on testicular tissue cryopreservation for fertility preservation in prepubertal and adolescent boys. Hum Reprod. (2015) 30:2463–75. doi: 10.1093/humrep/dev190
191. Pourhabibi Zarandi N, Galdon G, Kogan S, Atala A, Sadri-Ardekani H. Cryostorage of immature and mature human testis tissue to preserve spermatogonial stem cells (SSCs): A systematic review of current experiences toward clinical applications. Stem Cells Cloning. (2018) 11:23–38. doi: 10.2147/SCCAA.S137873
192. Keros V, Hultenby K, Borgström B, Fridström M, Jahnukainen K, Hovatta O. Methods of cryopreservation of testicular tissue with viable spermatogonia in pre-pubertal boys undergoing gonadotoxic cancer treatment. Hum Reprod. (2007) 22:1384–95. doi: 10.1093/humrep/del508
193. Wyns C, Curaba M, Martinez-Madrid B, van Langendonckt A, François-Xavier W, Donnez J. Spermatogonial survival after cryopreservation and short-term orthotopic immature human cryptorchid testicular tissue grafting to immunodeficient mice. Hum Reprod. (2007) 22:1603–11. doi: 10.1093/humrep/dem062
194. Poels J, van Langendonckt A, Many MC, Wese FX, Wyns C. Vitrification preserves proliferation capacity in human spermatogonia. Hum Reprod. (2013) 28:578–89. doi: 10.1093/humrep/des455
195. Ho WLC, Bourne H, Gook D, Clarke G, Kemertzis M, Stern K, et al. A short report on current fertility preservation strategies for boys. Clin Endocrinol (Oxf). (2017) 87:279–85. doi: 10.1111/cen.13377
196. Valli-Pulaski H, Peters KA, Gassei K, Steimer SR, Sukhwani M, Hermann BP, et al. Testicular tissue cryopreservation: 8 years of experience from a coordinated network of academic centers. Hum Reprod. (2019) 34:966–77. doi: 10.1093/humrep/dez043
197. Bahadur G, Chatterjee R, Ralph D. Testicular tissue cryopreservation in boys. Ethical and legal issues. Hum Reprod. (2000) 15:1416–20. doi: 10.1093/humrep/15.6.1416
198. Keros V, Rosenlund B, Hultenby K, Aghajanova L, Levkov L, Hovatta O. Optimizing cryopreservation of human testicular tissue: comparison of protocols with glycerol, propanediol and dimethylsulphoxide as cryoprotectants. Hum Reprod. (2005) 20:1676–87. doi: 10.1093/humrep/deh797
199. Ginsberg JP Li Y, Carlson CA, Gracia CR, Hobbie WL, Miller VA, Mulhall J, et al. Testicular tissue cryopreservation in prepubertal male children: an analysis of parental decision-making. Pediatr Blood Cancer. (2014) 61:1673–8. doi: 10.1002/pbc.25078
200. Pietzak EJ, Tasian GE, Tasian SK, Brinster RL, Carlson C, Ginsberg JP, et al. Histology of testicular biopsies obtained for experimental fertility preservation protocol in boys with cancer. J Urol. (2015) 194:1420–4. doi: 10.1016/j.juro.2015.04.117
201. Wyns C, van Langendonckt A, Wese FX, Donnez J, Curaba M. Long-term spermatogonial survival in cryopreserved and xenografted immature human testicular tissue. Hum Reprod. (2008) 23:2402–14. doi: 10.1093/humrep/den272
202. Kvist K, Thorup J, Byskov AG, Høyer PE, Møllgård K, Yding Andersen C. Cryopreservation of intact testicular tissue from boys with cryptorchidism. Hum Reprod. (2006) 21:484–91. doi: 10.1093/humrep/dei331
203. Brook PF, Radford JA, Shalet SM, Joyce AD, Gosden RG. Isolation of germ cells from human testicular tissue for low temperature storage and autotransplantation. Fertil Steril. (2001) 75:269–74. doi: 10.1016/S0015-0282(00)01721-0
204. Pacchiarotti J, Ramos T, Howerton K, Greilach S, Zaragoza K, Olmstead M, et al. Developing a clinical-grade cryopreservation protocol for human testicular tissue and cells. Biomed Res Int. (2013) 2013:930962. doi: 10.1155/2013/930962
205. Curaba M, Poels J, van Langendonckt A, Donnez J, Wyns C. Can prepubertal human testicular tissue be cryopreserved by vitrification? Fertil Steril. (2011) 95:2123.e9–2123.e12. doi: 10.1016/j.fertnstert.2011.01.014
206. Sá R, Cremades N, Malheiro I, Sousa M. Cryopreservation of human testicular diploid germ cell suspensions. Andrologia. (2012) 44:366–72. doi: 10.1111/j.1439-0272.2012.01290.x
207. Unni S, Kasiviswanathan S, D'Souza S, Khavale S, Mukherjee S, Patwardhan S, Bhartiya D. Efficient cryopreservation of testicular tissue: effect of age, sample state, and concentration of cryoprotectant. Fertil Steril. (2012) 97:200–8.e1. doi: 10.1016/j.fertnstert.2011.10.018
208. Abrishami M, Anzar M, Yang Y, Honaramooz A. Cryopreservation of immature porcine testis tissue to maintain its developmental potential after xenografting into recipient mice. Theriogenology. (2010) 73:86–96. doi: 10.1016/j.theriogenology.2009.08.004
209. Kaneko H, Kikuchi K, Tanihara F, Noguchi J, Nakai M, Ito J, et al. Normal reproductive development of pigs produced using sperm retrieved from immature testicular tissue cryopreserved and grafted into nude mice. Theriogenology. (2014) 82:325–31. doi: 10.1016/j.theriogenology.2014.04.012
210. Yokonishi T, Sato T, Komeya M, Katagiri K, Kubota Y, Nakabayashi K, et al. Offspring production with sperm grown in vitro from cryopreserved testis tissues. Nat Commun. (2014) 5:4320. doi: 10.1038/ncomms5320
211. Dumont L, Arkoun B, Jumeau F, Milazzo JP, Bironneau A, Liot D, et al. Assessment of the optimal vitrification protocol for pre-pubertal mice testes leading to successful in vitro production of flagellated spermatozoa. Andrology. (2015) 3:611–25. doi: 10.1111/andr.12042
212. Hou M, Andersson M, Zheng C, Sundblad A, Söder O, Jahnukainen K. Immunomagnetic separation of normal rat testicular cells from Roser's T-cell leukaemia cells is ineffective. Int J Androl. (2009) 32:66–73. doi: 10.1111/j.1365-2605.2007.00819.x
213. Hou M, Andersson M, Zheng C, Sundblad A, Söder O, Jahnukainen K. Decontamination of leukemic cells and enrichment of germ cells from testicular samples from rats with Roser's T-cell leukemia by flow cytometric sorting. Reproduction. (2007) 134:767–79. doi: 10.1530/REP-07-0240
214. Kilcoyne KR, Mitchell RT. Testicular transplantation for fertility preservation: clinical potential and current challenges. Reproduction. (2019) 158:F1–F14. doi: 10.1530/REP-18-0533
215. Dovey SL, Valli H, Hermann BP, Sukhwani M, Donohue J, Castro CA, et al. Eliminating malignant contamination from therapeutic human spermatogonial stem cells. J Clin Investig. (2013) 123:1833–43. doi: 10.1172/JCI65822
216. Sadri-Ardekani H, Homburg CH, van Capel TMM, van den Berg H, van der Veen F, van der Schoot CE, van Pelt AMM, Repping S. Eliminating acute lymphoblastic leukemia cells from human testicular cell cultures: A pilot study. Fertil Steril. (2014) 101:1072-1078.e1. doi: 10.1016/j.fertnstert.2014.01.014
217. The Practice Committees of the American Society for Reproductive Medicine and the Society for AssistedReproductive Technology. Mature oocyte cryopreservation: a guideline. Fertil Steril. (2013) 99:37–43. doi: 10.1016/j.fertnstert.2012.09.028
218. The Practice Committee of the American Society for Reproductive Medicine. Fertility preservation in patients undergoing gonadotoxic therapy or gonadectomy: a committee opinion. Fertil Steril. (2013) 100:1214–23. doi: 10.1016/j.fertnstert.2013.08.012
219. Bosch E, de Vos M, Humaidan P. The future of cryopreservation in assisted reproductive technologies. Front Endocrinol (Lausanne). (2020) 11:67. doi: 10.3389/fendo.2020.00067
220. Anderson RA, Amant F, Braat D, D'Angelo A, Chuva de Sousa Lopes SM, Demeestere I, et al. ESHRE guideline: female fertility preservation. Hum Reprod Open. (2020) 2020:hoaa052. doi: 10.1093/hropen/hoaa052
221. Walker Z, Lanes A, Ginsburg E. Oocyte cryopreservation review: outcomes of medical oocyte cryopreservation and planned oocyte cryopreservation. Reprod Biol Endocrinol. (2022) 20:10. doi: 10.1186/s12958-021-00884-0
222. Practice Committee of the American Society for Reproductive Medicine. Fertility preservation in patients undergoing gonadotoxic therapy or gonadectomy: a committee opinion. Fertil Steril. (2019) 112:1022–33. doi: 10.1016/j.fertnstert.2019.09.013
223. Martinez F, Andersen CY, Barri PN, Brannigan R, Cobo A, Donnez J, et al. Update on fertility preservation from the Barcelona International Society for Fertility Preservation–ESHRE–ASRM 2015 expert meeting: indications, results and future perspectives. Fertil Steril. (2017) 108:407–15.e11. doi: 10.1016/j.fertnstert.2017.05.024
224. Santaballa A, Márquez-Vega C, Rovirosa R, Vázquez L, Zeberio-Etxetxipia I, Andrés M, et al. Multidisciplinary consensus on the criteria for fertility preservation in cancer patients. Clin Transl Oncol. (2022) 24:227–43. doi: 10.1007/s12094-021-02699-2
225. Goossens E, Jahnukainen K, Mitchell R, van Pelt A, Pennings G, Rives N, et al. Fertility preservation in boys: recent developments and new insights. Hum Reprod Open. (2020) 2020:hoaa016. doi: 10.1093/hropen/hoaa016
226. Chen MX, Zhang Y, Cai QQ, Zhang S, Xu R, Chen H, et al. How fertility preservation guidelines have progressed worldwide: potential implications and inspiration. Reprod Dev Med. (2022) 6:34–41. doi: 10.1097/RD9.0000000000000010
227. Centrum voor Reproductieve Geneeskunde (CRG) Oncofertility - Home. (2022). Available online at: http://www.brusselsoncofertility.be/about_us (accessed December 30, 2022).
228. Edinburgh Fertility & Reproductive Endocrine Centre. (2022). Available online at: https://www.edinburgh-fertility-preservation.ed.ac.uk (accessed December 30, 2022).
229. von Wolff M, Montag M, Dittrich R, Denschlag D, Nawroth F, Lawrenz B. Fertility preservation in women - a practical guide to preservation techniques and therapeutic strategies in breast cancer, Hodgkin's lymphoma and borderline ovarian tumours by the fertility preservation network FertiPROTEKT. Arch Gynecol Obstet. (2011) 284:427–35. doi: 10.1007/s00404-011-1874-1
230. Jegaden M, Bouhnik AD, Préau M, Bendiane MK, Peretti-Watel P, Mancini J, Courbiere B. Fertility status perception, fertility preservation and desire to have children in cancer survivors: French VICAN survey. Future Sci OA. (2018) 4:FSO343. doi: 10.4155/fsoa-2018-0018
231. Moussaoui D, Surbone A, Adam C, Diesch-Furlanetto T, Girardin C, Bénard J, et al. Testicular tissue cryopreservation for fertility preservation in prepubertal and adolescent boys: a year experience from a Swiss multi-center network. Front Pediatr. (2022) 10:909000. doi: 10.3389/fped.2022.909000
232. IVI fertility clinics - FP. (2022). Available online at: https://ivi-fertility.com/assisted-reproduction-treatments/preservation-fertility/ (accessed December 30, 2022).
233. MediPass - FP Greece. (2022). Available online at: https://medipass.site/ (accessed December 30, 2022).
234. Nordfertil – Nordic centre for fertility preservation. (2022). Available online at: https://nordfertil.org/ (accessed December 30, 2022).
235. Lambertini M, Anserini P, Fontana V, Poggio F, Iacono G, Abate A, et al. The PREgnancy and FERtility (PREFER) study: an Italian multicenter prospective cohort study on fertility preservation and pregnancy issues in young breast cancer patients. BMC Cancer. (2017) 17:346. doi: 10.1186/s12885-017-3348-8
236. Sveafertil Homepage. (2022). Available online at: https://sveafertil.ki.se/en/home/ (accessed December 30, 2022).
237. García AM, Candás G, Bemi A, Daniel H, Korbenfeld E, Isetta J, et al. Survey of professionals on breast cancer, fertility preservation and pregnancy in Argentina. Ecancermedicalscience. (2021) 15:1183. doi: 10.3332/ecancer.2021.1183
238. Berton CZ, Brogliato C, Yoshida IH, Vellez LT, Suganuma CH, Cordts EB, et al. Cancer fertility preservation: a report from a Brazilian social program. JBRA Assist Reprod. (2020) 24:302–4. doi: 10.5935/1518-0557.20190089
239. Magee-Womens Research Institute and Foundation - Fertility Preservation Program (FPP). (2022). Available online at: https://mageewomens.org/for-researchers/research-centers/fertility-preservation-program-fpp (accessed December 30, 2022).
240. Mayo Clinic offers appointments in Arizona, Florida and Minnesota and at Mayo Clinic Health System locations. (2022). Available online at: https://www.mayoclinic.org/departments-centers/childrens-center/overview/specialty-groups/fertility-preservation (accessed December 30, 2022).
241. McGill University Health Centre - Fertility Preservation. (2022). Available online at: https://muhc.ca/reproductivecentre/page/fertility-preservation (accessed December 30, 2022).
242. Li W, Chian R-C. On behalf of the Fertility Preservation Committee of Chinese Maternal and ChildAssociation. Chinese expert consensus on clinical practice of female fertility preservation. Reprod Dev Med. (2022) 6:42–50. doi: 10.1097/RD9.0000000000000006
243. Centre for Fertility Preservation, Kasturba Medical College, Manipal University. (2022). Available online at: https://manipal.edu/mu/sdg/sdg-3/story-2.html (accessed December 30, 2022).
244. Harada M, Kimura F, Takai Y, Nakajima T, Ushijima K, Kobayashi H, et al. Japan Society of Clinical Oncology Clinical Practice Guidelines 2017 for fertility preservation in childhood, adolescent, and young adult cancer patients: part 1. Int J Clin Oncol. (2022) 27:265–80. doi: 10.1007/s10147-021-02081-w
245. Choi YJ, Hong YH, Kim S, Kim SK, Lee JR, Suh CS. The experience of fertility preservation in a single tertiary center in Korea. Front Endocrinol (Lausanne). (2022) 13: 845051. doi: 10.3389/fendo.2022.845051
246. Medical Services Advisory Committee Australian Government. Public Summary Document: Application No. 1435—Processing and cryopreservation of male and female gonadal tissue and gametes prior to or after gonadotoxic treatment to preserve fertility for the future. (2017). Available online at: http://www.msac.gov.au/internet/msac/publishing.nsf/Content/1435-public
247. Salama M, Ataman L, Taha T, Azmy O, Braham M, Douik F, et al. Building oncofertility core competency in developing countries: experience from Egypt, Tunisia, Brazil, Peru, and Panama. JCO Glob Oncol. (2020) 6:360–8. doi: 10.1200/GO.22.00006
248. Moravek MB, Appiah LC, Anazodo A, Burns KC, Gomez-Lobo V, Hoefgen HR, et al. Development of a pediatric fertility preservation program: a report from the pediatric initiative network of the oncofertility consortium. J Adolesc Health. (2019) 64:563–73. doi: 10.1016/j.jadohealth.2018.10.297
249. Liu S, Li F. Cryopreservation of single-sperm: where are we today? Reprod Biol Endocrinol. (2020) 18:41. doi: 10.1186/s12958-020-00607-x
Keywords: fertility preservation, cryopreservation, gametes, embryo, gonadal tissue
Citation: Antonouli S, Di Nisio V, Messini C, Daponte A, Rajender S and Anifandis G (2023) A comprehensive review and update on human fertility cryopreservation methods and tools. Front. Vet. Sci. 10:1151254. doi: 10.3389/fvets.2023.1151254
Received: 25 January 2023; Accepted: 29 March 2023;
Published: 18 April 2023.
Edited by:
Cristina Soriano-Úbeda, Universidad de León, SpainReviewed by:
Raul Sanchez, University of La Frontera, ChileAlberto Sola-Leyva, University of Granada, Spain
Copyright © 2023 Antonouli, Di Nisio, Messini, Daponte, Rajender and Anifandis. This is an open-access article distributed under the terms of the Creative Commons Attribution License (CC BY). The use, distribution or reproduction in other forums is permitted, provided the original author(s) and the copyright owner(s) are credited and that the original publication in this journal is cited, in accordance with accepted academic practice. No use, distribution or reproduction is permitted which does not comply with these terms.
*Correspondence: George Anifandis, ganif@med.uth.gr