- 1College of Animal Science, Wenzhou Vocational College of Science and Technology, Wenzhou, China
- 2College of Veterinary Medicine, Huazhong Agricultural University, Wuhan, China
- 3Punjab Health Department, Punjab, Pakistan
- 4Department of Pathobiology, Faculty of Veterinary Sciences, Bahauddin Zakariya University, Multan, Pakistan
Pesticides are widely used to control crop diseases, which have made an important contribution to the increase of global crop production. However, a considerable part of pesticides may remain in plants, posing a huge threat to animal safety. Thiram is a common pesticide and has been proven that its residues in the feed can affect the growth performance, bone formation, and intestinal health of chickens. However, there are few studies on the liver metabolism of chickens exposed to thiram. Here, the present study was conducted to investigate the effect of thiram exposure on liver metabolism of chickens. Metabolomics analysis shows that 62 metabolites were down-regulated (ginsenoside F5, arbekacin, coproporphyrinogen III, 3-keto Fusidic acid, marmesin, isofumonisin B1, 3-Hydroxyquinine, melleolide B, naphazoline, marmesin, dibenzyl ether, etc.) and 35 metabolites were up-regulated (tetrabromodiphenyl ethers, deoxycholic acid glycine conjugate, L-Palmitoylcarnitine, austalide K, hericene B, pentadecanoylcarnitine, glyceryl palmitostearate, quinestrol, 7-Ketocholesterol, tetrabromodiphenyl ethers, etc.) in thiram-induced chickens, mainly involved in the metabolic pathways including glycosylphosphatidylinositol (GPI)-anchor biosynthesis, porphyrin and chlorophyll metabolism, glycerophospholipid metabolism, primary bile acid biosynthesis and steroid hormone biosynthesis. Taken together, this research showed that thiram exposure significantly altered hepatic metabolism in chickens. Moreover, this study also provided a basis for regulating the use and disposal of thiram to ensure environmental quality and poultry health.
Introduction
Increasing evidence indicated that pesticides play a vital role in agricultural production. Statistical analysis indicated that China is the main consumer of pesticides, using 1.8 million tons per year, followed by the America (1–3). At present, pesticides have been listed as priority pollutants by the United Nations Environment Protection Agency (UNEP) (4, 5). Although, the use of pesticides has effectively increased crop yield and reduced disease. However, the extensive use of pesticides will also cause serious environmental pollution, posing a serious threat to food security and animal health (6). In addition, some pesticides may remain in plants and be introduced into nearby waters after rainfall, endangering the health of aquatic animals and causing drinking water safety problems (7–16). Moreover, aerial spray of pesticides may cause the pollution of nearby or distant areas through transboundary movement (17). It is worth noting that humans and animals may also ingest plants containing pesticides through the food chain, seriously endangering public health and human security (18–21).
Poultry including chickens, ducks and geese are the largest livestock species. These species developed rapidly in the past few decades, effectively solving the problem of protein shortage. Among the above-mentioned poultry, chickens are widely farmed because of their fast growth and low price (22, 23). Consequently, any factors that endanger chickens should be given enough attention. However, chickens are likely to be exposed to feed containing pesticide residues (24–27). Previous studies have indicated that most pesticides could accumulate in multiple tissues and inhabit the exposed organisms from few months to several years, thus even very low concentration is also harmful to health (5, 28, 29). Liver is the vital metabolic and alexipharmic organ in the animal and humans, which is considered as one of the primary target organs for various hazardous substances such as pesticides and heavy metal (30–32). Therefore, the intake of feed containing pesticide residues will inevitably affect the liver of broilers.
Thiram is one of the common pesticides, mainly used to increase crop yield and reduce disease (33, 34). However, the abuse of thiram not only cause pesticide residues, but also pose a serious threat to the safety of humans and animals (35–37). Previous studies have shown that thiram exposure causes abnormal bone development and reduced growth performance in chickens (38–40). In addition, thiram exposure has been demonstrated to cause intestinal flora imbalance and liver histopathology injuries in chickens (18, 26). However, studies regarding the influences of thiram exposure on liver metabolism in chickens remain scarce. Taking advantage of this gap, we explored the effect of thiram exposure on liver metabolism in chickens.
Materials and methods
Animal experiments and sample acquisition
A group of 60 one-day-old healthy Arbor Acres chickens were purchased from a commercial hatchery and maintained under the standard ambient temperature, sanitary condition and illumination as previously described. Prior to the experiment, all the subjects were performed physical examinations to avoid deformity and other congenital diseases. After acclimatization for 3 days, an equal number of chickens (n = 30) regardless of sex were divided into control and thiram-treated groups. Throughout the trial, the control chickens were provided sufficient feed and water. Moreover, the chickens in thiram-treated group received same diet as controls but supplemented with thiram (50 mg/kg) purchased from Macklin Biochemical Co., Ltd. (Shanghai, China) in feed as suggested by previous research from days 3–7 (39). All chickens were euthanized and liver tissue was collected on days 18 of the experimental study. The achieved samples were snap-frozen utilizing liquid nitrogen and stored at −80°C for further study.
Sample preparation
The metabolomic procedure was conducted based on the previous protocols with minor improvements (41, 42). Briefly, the acquired liver samples (~100 mg) were triturated in methanol and then centrifuged for 15 min at 14,000×g. The supernatant of mixture was collected and stored in Eppendorf tubes for 10 min. Subsequently, the deionized water (400 μl) was added to the obtained supernatant and kept at −80°C for further study. The extract (100 μl) of each sample was mixed for preparing quality control (QC) sample and QC samples were performed testing between every five samples. The 0.22 μm membranes were applied to filter the supernatant and then the filtered supernatant was performed UPLC-QTOF/MS (Waters, USA) analysis. The condition of UPLC was determined as described previously (42). Moreover, the reagents used in this study were HPLC grade.
Differential metabolite analysis
The original mass spectrometry was subjected to process using Marker View 1.1 (AB SCIEX, USA). Subsequently, PCA and PLS-DA were performed by importing metabolomics data into SIMCA (version 14.1, Umetrics, Sweden). The determination of differential metabolites was based on the variable weight value (VIP) and p-value obtained from the OPLS-DA model. To obtain pathways involved in differential metabolites, MetaboAnalyst and KEGG database (https://www.kegg.jp/kegg/pathway.html) was used to perform cluster analysis and metabolic pathway annotation of differential metabolites.
Results
Thiram exposure disrupts liver metabolism
The plots of PCA analysis showed that the samples in the thiram-exposed group were clustered closely and separated from the control group, indicating that thiram exposure significant changes in liver metabolome (Figures 1A, B). To further reveal the alterations of liver metabolome during thiram exposure, OPLS-DA score plots was applied for pattern discriminant analysis. Results indicated that there was a clear separation between both groups and no fitting occur (Figures 1C–F).
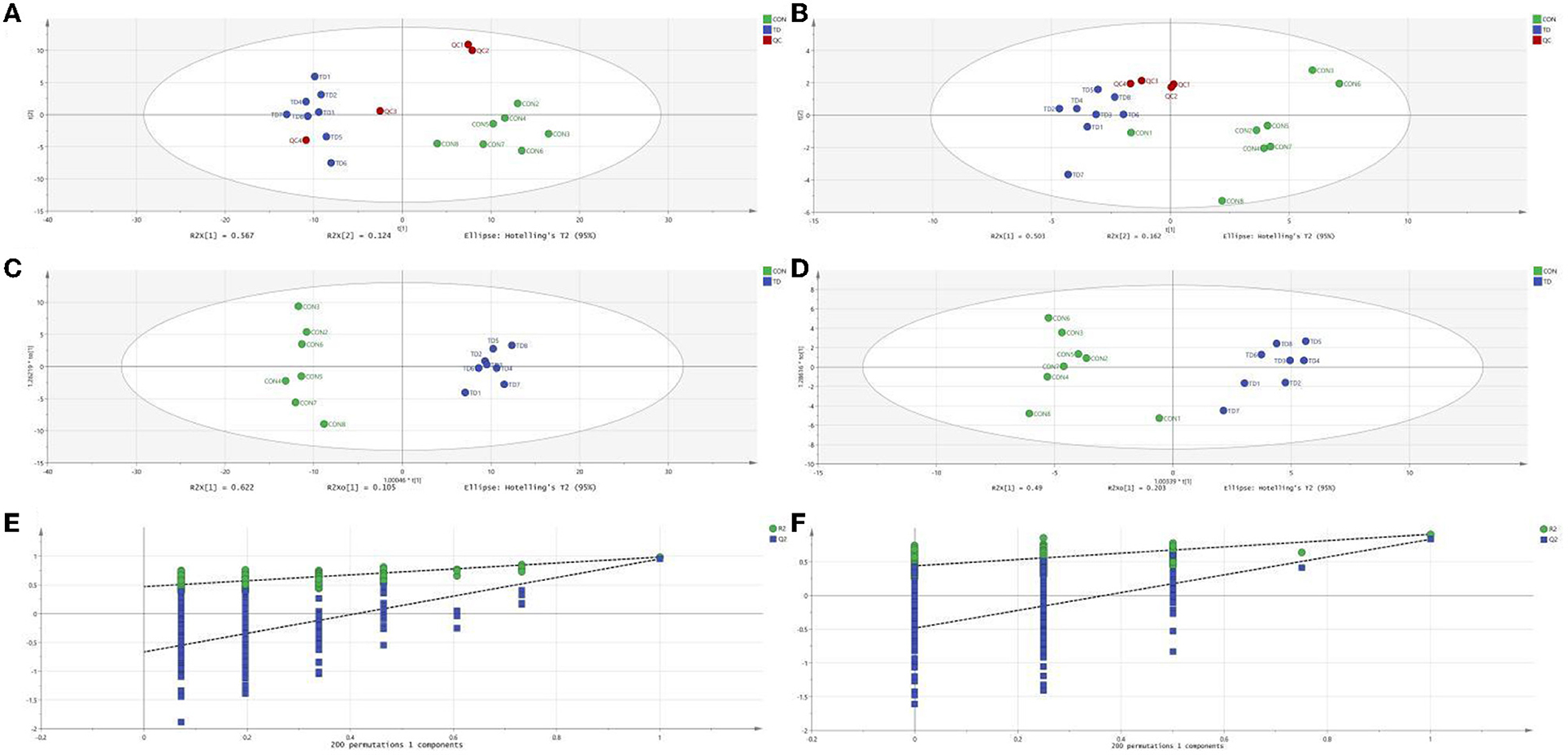
Figure 1. Thiram exposure altered liver metabolism. (A, B) PCA score plots based on positive-ion mode and negative-ion mode, respectively. (C, D) OPLS-DA plot based on positive-ion mode and negative-ion mode, respectively. (E, F) Permutation tests based on positive-ion mode and negative-ion mode, respectively.
Identification of metabolites associated with thiram exposure
The differential metabolites were recognized based on the criterion of VIP > 1, P < 0.05. Results indicated that a total of 97 differential metabolites were detected between both groups (Table 1). Among significantly different metabolites, 62 metabolites (ginsenoside F5, arbekacin, coproporphyrinogen III, 3-keto Fusidic acid, marmesin, etc.) were down-regulated, whereas 35 metabolites (L-Palmitoylcarnitine, quinestrol, 7-Ketocholesterol, tetrabromodiphenyl ethers, etc.) were up-regulated in thiram-induced chickens. Moreover, the alternations of metabolites also could be observed in the heatmap (Figure 2).

Table 1. Statistical analysis of differential metabolites between thiram-exposed and control groups.
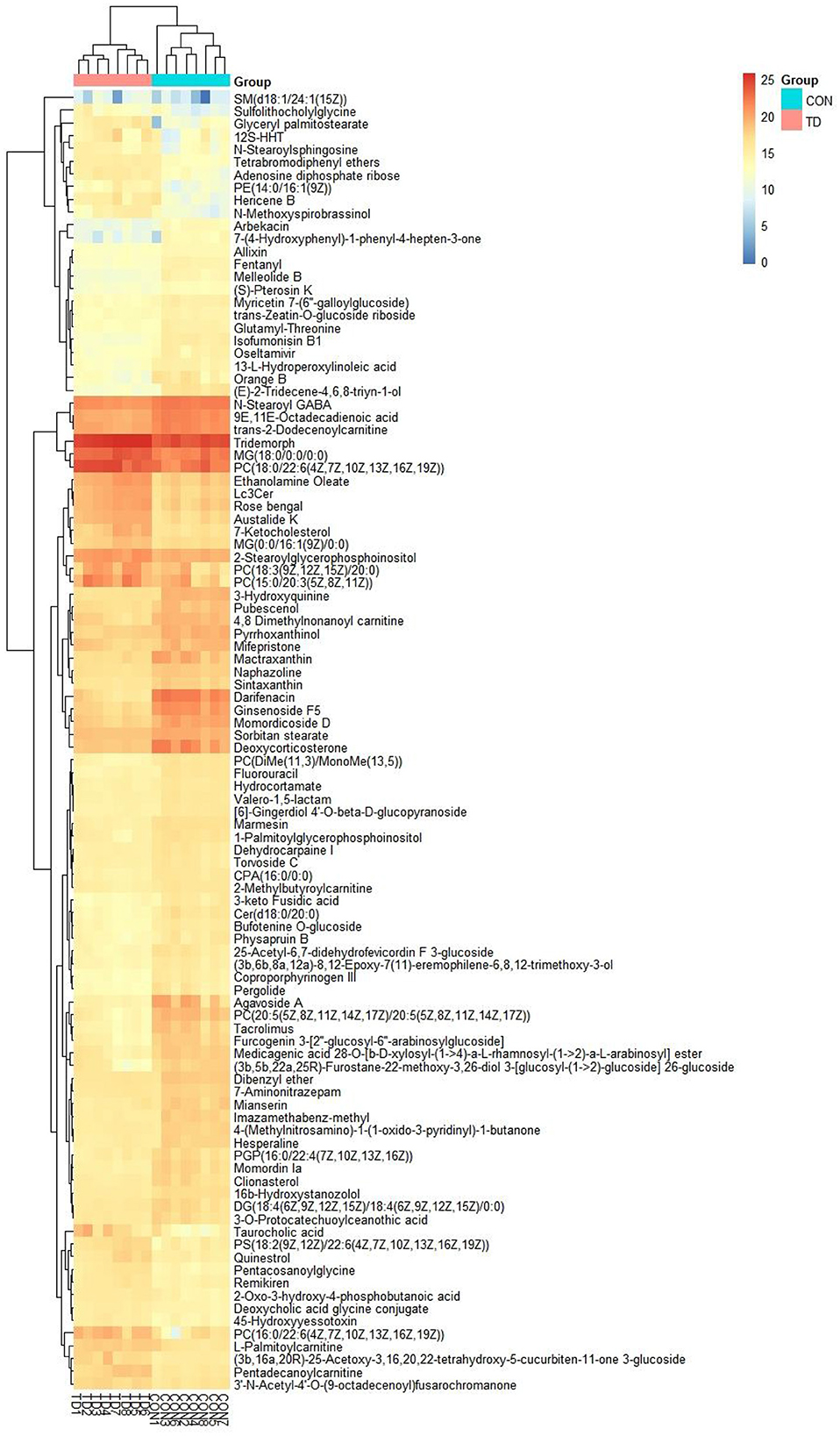
Figure 2. Heatmap revealed the differential metabolites in liver exposed to thiram. The color in the heatmap indicates the normalized relative abundance of each metabolite.
Metabolic pathway analysis
The differential metabolites were subjected to pathway analysis by utilizing MetaboAnalyst 4.0 and results indicated that 13 metabolic pathways (linoleic acid metabolism, glycerophospholipid metabolism, taurine and hypotaurine metabolism, vitamin B6 metabolism, alpha-Linolenic acid metabolism, glycosylphosphatidylinositol (GPI)-anchor biosynthesis, sphingolipid metabolism, porphyrin and chlorophyll metabolism, arachidonic acid metabolism, fatty acid degradation, primary bile acid biosynthesis, purine metabolism and steroid hormone biosynthesis) involved in hepatotoxicity induced by thiram (Figure 3). Among above-mentioned differential pathways, 5 pathways with highest pathway impact value were the glycosylphosphatidylinositol (GPI)-anchor biosynthesis, porphyrin and chlorophyll metabolism, glycerophospholipid metabolism, primary bile acid biosynthesis and steroid hormone biosynthesis. The metabolic diagram in the intestine is shown in Figure 4.
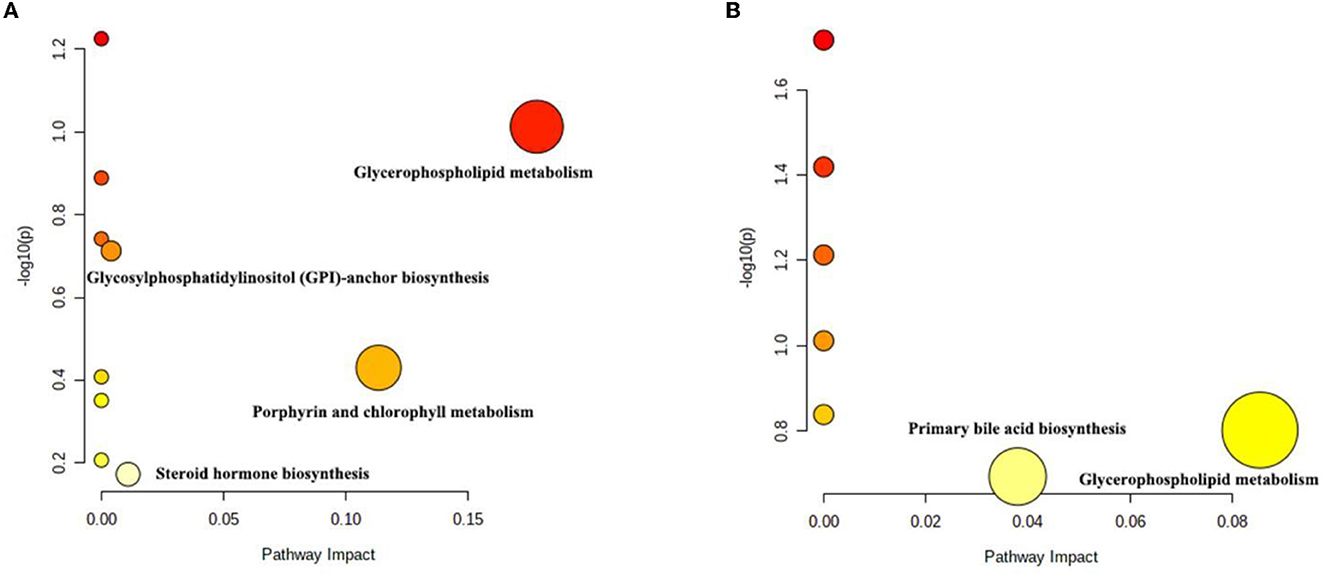
Figure 3. Differential metabolic pathway analysis based on the positive-ion mode (A) and the negative-ion mode (B). Each circle represents a metabolic pathway.
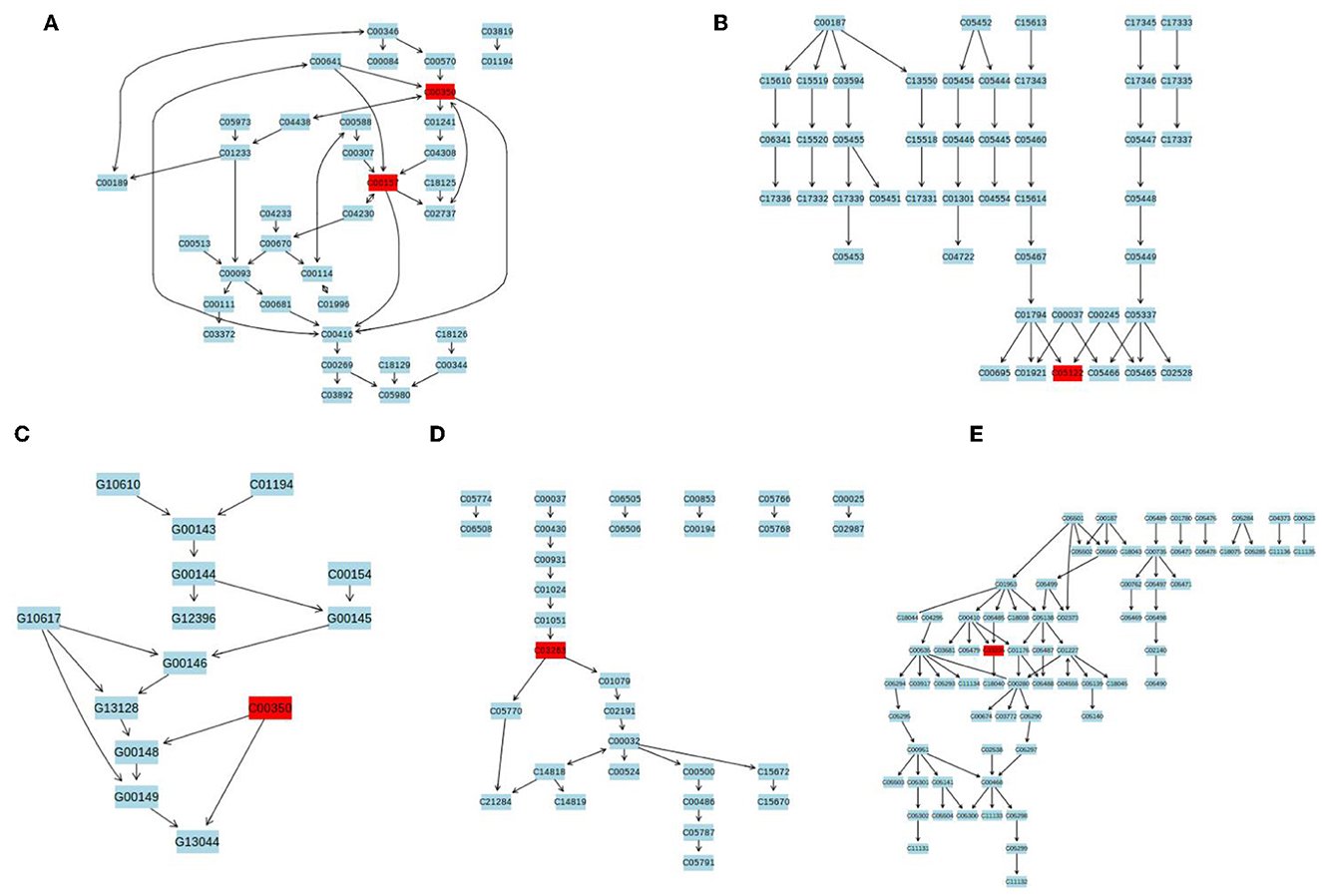
Figure 4. The representative schematic diagram of liver metabolic exposed to thiram. (A) Glycerophospholipid metabolism. (B) Primary bile acid biosynthesis. (C) Glycosylphosphatidylinositol (GPI)-anchor biosynthesis. (D) Porphyrin and chlorophyll metabolism. (E) Steroid hormone biosynthesis. The red boxes represent the differential metabolites associated with thiram exposure.
Discussion
Thiram is widely used in agricultural production and is likely to accumulate in plants (43–45). Some plant-sourced feeds that accumulate pesticides are likely to enter poultry farming through the food chain, posing a serious threat to the health of poultry (18, 38, 39). At present, the harm of thiram exposure to various species such as mice, chickens and fish has been widely confirmed. For instance, thiram has been shown to dramatically affect the respiratory tract, central nervous system, stimulate skin and restrain the formation of white blood cells (34, 46, 47). Furthermore, some studies have also demonstrated the role of thiram exposure in the induction of lipid metabolism (18). The liver is an important metabolic and detoxifying organ in animals and humans, which is regarded as one of the main target organs for multiple stimulations including pesticides, heavy metals and various environmental pollutants (48–50). Therefore, pesticide residues in feed are likely to affect liver health, which will cause great damage to poultry production. However, study on thiram toxicities to liver of chicken is still lacking. In this study, we explored the effect of thiram exposure on liver metabolism in chickens.
In this study, 97 differential metabolites were totally recognized, which was closely related to multiple metabolic pathways including glycerophospholipid metabolism, porphyrin and chlorophyll metabolism, primary bile acid biosynthesis, steroid hormone biosynthesis and glycosylphosphatidylinositol (GPI)-anchor biosynthesis. These metabolic pathways may play an important role in the hepatotoxicity induced by thiram. Remarkably, some of the decreased metabolites including ginsenosides, arbekacin, coproporphyrinogen III, Fusidic acid, marmesin and fluorouracil play important roles in antioxidant capacity, anti-cancer and oxygen transport. Ginsenosides were widely recognized because of multiple beneficial effects, such as inhibiting the growth of cancer cells, inducing tumor cell apoptosis, reversing the abnormal differentiation of tumor cells, and anti-tumor metastasis (51). Moreover, ginsenoside has been demonstrated to improve immunity and antioxidant capacity of host (52). Zhang et al. revealed that the concentrations of aspartate aminotransferase and alanine aminotransferase in thiram-induced chickens significantly increased, but antioxidant enzyme dramatically decreased, suggesting liver injury and antioxidant dysfunction (53). Therefore, we speculated that the decreased ginsenoside may be one of the important pathways for thiram exerts its toxic effects and cause antioxidant dysfunction. Previous research indicated that arbekacin have an inhibitory effect on multiple pathogens such as Pseudomonas aeruginosa, Klebsiella pneumonia and Acinetobacter baumannii (54). Moreover, arbekacin can be used for treating multiple drug resistant pneumonia and septicemia as well as infections caused by resistant Staphylococcus Aureus (55, 56). Coproporphyrinogen III play a key role in the production of heme (57, 58). Heme is also an important component of hemoglobin, which plays a key role in the transport of oxygen. Oxygen has been demonstrated to play key roles in blood vessel development and bone formation (59). Previous studies indicated that the chickens exposed to thiram showed weight loss, accompanied by angiogenesis disorder and tibial dyschondroplasia (60, 61). Therefore, decreased coproporphyrinogen III may be one of the causes of angiogenesis disorder and abnormal bone development of chickens.
Fusidic acid can treat infections induced by methicillin-susceptible and methicillin-resistant Staphylococcus aureus (62). Marmesinpossess multiple pharmacological functions including anti-inflammatory, antihepatotoxic and antitumor activities (63, 64). Fluorouracil has anti-cancer effects (65). Moreover, we observed increased levels of L-palmitoylcarnitine, quinestrol, 7-ketocholesterol, and tetrabromodiphenyl ether during thiram exposure. L-palmitoylcarnitine is an ester derivative of carnitine, which participated in fatty acids metabolism and its abundance increased during hepatic lipid accumulation (66, 67). Consistent with this study, Sheng et al. indicated that the abundance of L-palmitoylcarnitine increased significantly in zebrafish exposed to organic pollutants (68). Moreover, increased L-palmitoylcarnitine was closely related to poorer prognosis in patients with chronic heart failure (69). Quinestrol can disrupt internal secretion and cause fertility disorders by inducing testicular damage (70). Moreover, quinestrol can increase the levels of serum MDA and aggravate the oxidative damage of cells (71). As a pro-oxidant and pro-inflammatory molecule, 7-ketocholesterol not only induces inflammation and nerve cell damage, but also affects membrane permeability and causes oxidative stress (72). Tetrabromodiphenyl ether is known to possess reproductive toxicity, which weaken sperm activity and increase the quantity of abnormal sperm (73). Moreover, tetrabromodiphenyl ether has also been demonstrated to induce liver inflammation and promote the expression of inflammatory genes including IL-6, TNF-α and IL-l β (74). Increasing evidence demonstrated that long-term pesticide exposure can result in cancer and reproductive disorders. In this study, we observed significant changes in metabolites associated with anti-cancer, oxidative stress and reproductive function, indicating that thiram may also be a potential cancer-inducing factor. Previous study indicated that thiram exposure could induce liver autophagy and apoptosis. Notably, some studies also showed that oxidative stress could cause the initiation and development of apoptosis and autophagy. Therefore, thiram induced liver apoptosis and autophagy may be mediated by differential metabolites related to oxidative stress.
In conclusion, this study investigated the effect of thiram exposure on liver metabolism in chickens. Results showed that thiram exposure can significantly alter liver metabolism, characterized by significant changes in some metabolites and metabolic pathways. These results filled in the blank of thiram exposure on liver metabolism characteristics of chickens, and conveyed an important message that hepatic metabolic disorder may be one of the important ways thiram affects broiler liver metabolism. Moreover, this study will help prevent and control the effects of thiram on liver metabolism in chickens from the perspective of liver metabolism.
Data availability statement
The original contributions presented in the study are included in the article/supplementary material, further inquiries can be directed to the corresponding author.
Ethics statement
The study was performed under the instructions and approval of Ethics Committee of the Wenzhou Vocational College of Science and Technology.
Author contributions
MW and LW conceived, designed the experiments, and wrote the manuscript. DZ, HLu, HLi, ZL, XS, CW, and YZ contributed sample collection and reagents preparation. MW analyzed the data. SS and MS revised the manuscript. All authors reviewed the manuscript.
Funding
This study was supported by Teacher Professional Development Project (FX2022194), Zhejiang Provincial Basic Public Welfare Research Project (LGN20C170001), Zhejiang Provincial Key Research and Development Project (2020C02032), and Wenzhou New poultry variety breeding cooperation group project (2019ZX005).
Conflict of interest
The authors declare that the research was conducted in the absence of any commercial or financial relationships that could be construed as a potential conflict of interest.
Publisher's note
All claims expressed in this article are solely those of the authors and do not necessarily represent those of their affiliated organizations, or those of the publisher, the editors and the reviewers. Any product that may be evaluated in this article, or claim that may be made by its manufacturer, is not guaranteed or endorsed by the publisher.
References
1. Zhang H, Mehmood K, Jiang X, Yao W, Iqbal M, Waqas M, et al. Effect of tetramethyl thiuram disulfide (thiram) in relation to tibial dyschondroplasia in chickens. Environ Sci Pollut Res Int. (2018) 25:28264–74. doi: 10.1007/s11356-018-2824-2
2. Palumbi SR. Humans as the world's greatest evolutionary force. Science. (2001) 293:1786–90. doi: 10.1126/science.293.5536.1786
3. de Albuquerque N, Carrao DB, Habenschus MD, de Oliveira A. Metabolism studies of chiral pesticides: A critical review. J Pharm Biomed Anal. (2018) 147:89–109. doi: 10.1016/j.jpba.2017.08.011
4. Wang Y, Li Z, Barnych B, Huo J, Wan D, Vasylieva N, et al. Investigation of the Small Size of Nanobodies for a Sensitive Fluorescence Polarization Immunoassay for Small Molecules: 3-Phenoxybenzoic Acid, an Exposure Biomarker of Pyrethroid Insecticides as a Model. J Agric Food Chem. (2019) 67:11536–41. doi: 10.1021/acs.jafc.9b04621
5. Zhang JJ, Yang H. Metabolism and detoxification of pesticides in plants. Sci Total Environ. (2021) 790:148034. doi: 10.1016/j.scitotenv.2021.148034
6. Parween T, Jan S, Mahmooduzzafar S, Fatma T, Siddiqui ZH. Selective effect of pesticides on plant—a review. Crit Rev Food Sci Nutr. (2016) 56:160–79. doi: 10.1080/10408398.2013.787969
7. Golovko O, Orn S, Sorengard M, Frieberg K, Nassazzi W, Lai FY, et al. Occurrence and removal of chemicals of emerging concern in wastewater treatment plants and their impact on receiving water systems. Sci Total Environ. (2021) 754:142122. doi: 10.1016/j.scitotenv.2020.142122
8. Munz NA, Burdon FJ, de Zwart D, Junghans M, Melo L, Reyes M, et al. Pesticides drive risk of micropollutants in wastewater-impacted streams during low flow conditions. Water Res. (2017) 110:366–77. doi: 10.1016/j.watres.2016.11.001
9. Akram R, Iqbal R, Hussain R, Jabeen F, Ali M. Evaluation of Oxidative stress, antioxidant enzymes and genotoxic potential of bisphenol A in fresh water bighead carp (Aristichthys nobils) fish at low concentrations. Environ Pollut. (2021) 268:115896. doi: 10.1016/j.envpol.2020.115896
10. Aziz S, Abdullah S, Anwar H, Latif F, DNA. Damage and Oxidative Stress in Economically Important Fish, Bighead Carp (Hypophthalmichthys nobilis) Exposed to Engineered Copper Oxide Nanoparticles. Pak Vet J. (2022) 42:1–08. doi: 10.29261/pakvetj/2022.002
11. Li XW, Naseem S, Hussain R, Ghaffar A, Li K, Khan A. Evaluation of DNA Damage, Biomarkers of Oxidative Stress, and Status of Antioxidant Enzymes in Freshwater Fish (Labeo rohita) Exposed to Pyriproxyfen. Oxid Med Cell Longev. (2022) 2022:5859266. doi: 10.1155/2022/5859266
12. Naseem S, Ghaffar A, Hussain R, Khan A. Inquisition of Toxic Effects of Pyriproxyfen on Physical, Hemato-Biochemical and Histopathological Parameters in Labeo rohita Fish. Pak Vet J. (2022) 42:308–15.
13. Wang JQ, Hussain R, Ghaffar A, Afzal G, Saad AQ, Ahmad N, et al. Clinicohematological, mutagenic, and oxidative stress induced by pendimethalin in freshwater fish bighead carp (Hypophthalmichthys nobilis). Oxid Med Cell Longev. (2022) 2022:2093822. doi: 10.1155/2022/2093822
14. Akram R, Ghaffar A, Hussain R, Khan I, de Assis Santana VL, Mehmood K, et al. Hematological, serum biochemistry, histopathological and mutagenic impacts of triclosan on fish (Bighead carp). Agrobiological Records. (2021) 7:18–28.
15. Jabeen G. Manzoor F, Arshad M, BI Barbol. Effect of cadmium exposure on hematological, nuclear and morphological alterations in erythrocyte of fresh water fish (Labeo rohita). Continental Vet J. (2021) 1:20–4.
16. Tahir R, Ghaffar A, Abbas G, Turabi TH, Kausar S, Du XX, et al. Pesticide induced hematological, biochemical and genotoxic changes in fish: A review. Agrobiol Rec. (2021) 3:41–57. doi: 10.47278/journal.abr/2021.005
17. Rasheed T, Rizwan K, Bilal M, Sher F, Iqbal H. Tailored functional materials as robust candidates to mitigate pesticides in aqueous matrices-a review. Chemosphere. (2021) 282:131056. doi: 10.1016/j.chemosphere.2021.131056
18. Kong A, Zhang C, Cao Y, Cao Q, Liu F, Yang Y, et al. The fungicide thiram perturbs gut microbiota community and causes lipid metabolism disorder in chickens. Ecotoxicol Environ Saf. (2020) 206:111400. doi: 10.1016/j.ecoenv.2020.111400
19. Ahmad L, Gul ST, Saleemi MK, Hussain R, Rehman AU, Naqvi SNH, et al. The effect of different repeated doses of cypermethrin on the behavioral and histological alterations in the brain of rabbits (Oryctolagus cuniculi). Int J Vet Sci Med. (2021) 10:347–54. doi: 10.47278/journal.ijvs/2021.092
20. Namratha ML, Lakshman M, Jeevanalatha M, Kumar BA. Hematological alterations induced by glyphosate and ameliorative effect of ascorbic acid in wistar rats. Continental Vet J. (2021) 1:32–6. doi: 10.5455/ijlr.20191012074803
21. Taha MG, El-Hamamsy SMA, Ahmed NS, Ali MM. Amelioration effect of Carica papaya fruit extracts on doxorubicin – induced cardiotoxicity in rats. Int J Vet Sci. (2020) 9:349–54. doi: 10.37422/IJVS/032
22. Cui L, Zhang X, Cheng R, Ansari AR, Elokil AA, Hu Y, et al. Sex differences in growth performance are related to cecal microbiota in chicken. Microb Pathog. (2021) 150:104710. doi: 10.1016/j.micpath.2020.104710
23. Zhang X, Akhtar M, Chen Y, Ma Z, Liang Y, Shi D, et al. Chicken jejunal microbiota improves growth performance by mitigating intestinal inflammation. Microbiome. (2022) 10:107. doi: 10.1186/s40168-022-01299-8
24. Nordborg M, Davis J, Cederberg C, Woodhouse A. Freshwater ecotoxicity impacts from pesticide use in animal and vegetable foods produced in Sweden. Sci Total Environ. (2017) 581–2:448–59. doi: 10.1016/j.scitotenv.2016.12.153
25. Mahugija J, Chibura PE, Lugwisha EH. Residues of pesticides and metabolites in chicken kidney, liver and muscle samples from poultry farms in Dar es Salaam and Pwani, Tanzania. Chemosphere. (2018) 193:869–74. doi: 10.1016/j.chemosphere.2017.11.094
26. Wu Z, Su R. Pesticide thiram exposure alters the gut microbial diversity of chickens. Front Microbiol. (2022) 13:966224. doi: 10.3389/fmicb.2022.966224
27. Liu YM, Han MY, Liu CS, Tang YX, Jia M, Chen XJ, et al. Subchronic toxicity of oral deltamethrin in laying chickens. Front Vet Sci. (2022) 9:1079580. doi: 10.3389/fvets.2022.1079580
28. Kahunyo JM Maitai CK, Froslie A, Organochlorine pesticide-residues in chicken fat—a survey. Poult Sci. (1986) 65:1084–89. doi: 10.3382/ps.0651084
29. Aulakh RS, Gill J, Bedi JS, Sharma JK, Joia BS, Ockerman HW. Organochlorine pesticide residues in poultry feed, chicken muscle and eggs at a poultry farm in Punjab, India. J Sci Food Agric. (2006) 86:741–44. doi: 10.1002/jsfa.2407
30. Zhong G, Wan F, Lan J, Jiang X, Wu S, Pan J, et al. Arsenic exposure induces intestinal barrier damage and consequent activation of gut-liver axis leading to inflammation and pyroptosis of liver in ducks. Sci Total Environ. (2021) 788:147780. doi: 10.1016/j.scitotenv.2021.147780
31. Akbulut S, Isik B, Mehdi UM, Dogan UG, Sahin TT, Sarici KB, et al. Tissue Heavy Metals in Liver Diseases. Transplant Proc. (2022) 54:1859–64. doi: 10.1016/j.transproceed.2022.05.029
32. Li W, Xiao H, Wu H, Xu X, Zhang Y. Organophosphate pesticide exposure and biomarkers of liver injury/liver function. Liver Int. (2022) 42:2713–23. doi: 10.1111/liv.15461
33. Liu K, Li Y, Iqbal M, Tang Z, Zhang H. Thiram exposure in environment: A critical review on cytotoxicity. Chemosphere. (2022) 295:133928. doi: 10.1016/j.chemosphere.2022.133928
34. Radko L, Cybulski W, Rzeski W. The protective effects of silybin on the cytotoxicity of thiram in human, rat and chicken cell cultures. Pestic Biochem Physiol. (2017) 143:154–60. doi: 10.1016/j.pestbp.2017.08.003
35. Xu X, Mathieu C, Berthelet J, Duval R, Bui LC, Busi F, et al. Human Arylamine N-Acetyltransferase 1 Is Inhibited by the Dithiocarbamate Pesticide Thiram. Mol Pharmacol. (2017) 92:358–65. doi: 10.1124/mol.117.108662
36. Vasamsetti B, Chon K, Kim J, Oh JA, Yoon CY, Park HH. Developmental Toxic Effects of Thiram on Developing Zebrafish (Danio rerio) Embryos. Toxics. (2022) 10:369. doi: 10.3390/toxics10070369
37. Long TE. Repurposing thiram and disulfiram as antibacterial agents for multidrug-resistant Staphylococcus aureus infections. Antimicrob Agents Chemother. (2017) 61:e00898–17. doi: 10.1128/AAC.00898-17
38. Chen SM, Jahejo AR, Nabi F, Ahmed S, Zhao JF Yu J, et al. Janus kinase/signal transducer and activator of transcription signaling pathway-related genes STAT3, SOCS3 and their role in thiram induced tibial dyschondroplasia chickens. Res Vet Sci. (2021) 136:25–31. doi: 10.1016/j.rvsc.2021.01.024
39. Liu Y, Yi J, Li Y, Hussain R, Zhu S, Li Y, et al. Residue of thiram in food, suppresses immune system stress signals and disturbs sphingolipid metabolism in chickens. Vet Immunol Immunopathol. (2022) 247:110415. doi: 10.1016/j.vetimm.2022.110415
40. Zhang C, Xu T, Lin L, Shaukat A, Tong X, Yue K, et al. Morinda officinalis polysaccharides ameliorates bone growth by attenuating oxidative stress and regulating the gut microbiota in thiram-induced tibial dyschondroplasia chickens. Metabolites. (2022) 12:958. doi: 10.3390/metabo12100958
41. Lin S, Qiao N, Chen H, Tang Z, Han Q, Mehmood K, et al. Integration of transcriptomic and metabolomic data reveals metabolic pathway alteration in mouse spermatogonia with the effect of copper exposure. Chemosphere. (2020) 256:126974. doi: 10.1016/j.chemosphere.2020.126974
42. Li Q, Liao J, Lei C, Shi J, Zhang H, Han Q, et al. Metabolomics analysis reveals the effect of copper on autophagy in myocardia of pigs. Ecotoxicol Environ Saf. (2021) 213:112040. doi: 10.1016/j.ecoenv.2021.112040
43. Maximiano EM, de Lima F, Cardoso C, Arruda GJ. Modification of carbon paste electrodes with recrystallized zeolite for simultaneous quantification of thiram and carbendazim in food samples and an agricultural formulation. Electrochim Acta. (2018) 259:66–76. doi: 10.1016/j.electacta.2017.10.162
44. Liu K, Jin Y, Wu Y, Liang JG. Simple and rapid colorimetric visualization of tetramethylthiuram disulfide (thiram) sensing based on anti-aggregation of gold nanoparticles. Food Chem. (2022) 384:132223. doi: 10.1016/j.foodchem.2022.132223
45. Feng LX, Duan JL, Wang K, Huang L, Xiao GN. Robotic written silver ink on photographic paper for detection of thiram residues in fruits. Spectrochim Acta a Mol Biomol Spectrosc. (2022) 268:120724. doi: 10.1016/j.saa.2021.120724
46. Shukla Y, Baqar SM, Mehrotra NK. Carcinogenic and co-carcinogenic studies of thiram on mouse skin. Food Chem Toxicol. (1996) 34:283–89. doi: 10.1016/0278-6915(95)00113-1
47. Cereser C, Boget S, Parvaz P, Revol A. An evaluation of thiram toxicity on cultured human skin fibroblasts. Toxicology. (2001) 162:89–101. doi: 10.1016/S0300-483X(01)00347-X
48. Liao J, Liu Y, Yi J, Li Y, Li Q, Li Y, et al. Gut microbiota disturbance exaggerates battery wastewater-induced hepatotoxicity through a gut-liver axis. Sci Total Environ. (2022) 809:152188. doi: 10.1016/j.scitotenv.2021.152188
49. Yi SJ, Xiong YW, Zhu HL, Dai LM, Cao XL, Liu WB, et al. Environmental cadmium exposure during pregnancy causes diabetes-like phenotypes in mouse offspring: Association with oxidative stress in the fetal liver. Sci Total Environ. (2021) 777:146006. doi: 10.1016/j.scitotenv.2021.146006
50. Wu S, Wang Y, Iqbal M, Mehmood K, Li Y, Tang Z, et al. Challenges of fluoride pollution in environment: Mechanisms and pathological significance of toxicity - A review. Environ Pollut. (2022) 304:119241. doi: 10.1016/j.envpol.2022.119241
51. Lev-Ari S, Starr AN, Vexler A, Kalich-Philosoph L, Yoo HS, Kwon KR, et al. Rh2-enriched Korean ginseng (Ginseng Rh2+) inhibits tumor growth and development of metastasis of non-small cell lung cancer. Food Funct. (2021). doi: 10.1039/D1FO00643F
52. Kwan K, Yun H, Dong T, Tsim K. Ginsenosides attenuate bioenergetics and morphology of mitochondria in cultured PC12 cells under the insult of amyloid beta-peptide. J Ginseng Res. (2021) 45:473–81. doi: 10.1016/j.jgr.2020.09.005
53. Zhang H, Mehmood K, Li K, Rehman MU, Jiang X, Huang S, et al. Icariin Ameliorate Thiram-Induced Tibial Dyschondroplasia via Regulation of WNT4 and VEGF Expression in Broiler Chickens. Front Pharmacol. (2018) 9:123. doi: 10.3389/fphar.2018.00123
54. Panchal G, Pandit R, Trailokya A, Sharma A. Arbekacin - A Novel Antibiotic for Critical Infections. J Assoc Physicians India. (2019) 67:93–7.
55. Matsumoto T. Arbekacin: another novel agent for treating infections due to methicillin-resistant Staphylococcus aureus and multidrug-resistant Gram-negative pathogens. Clin Pharmacol. (2014) 6:139–48. doi: 10.2147/CPAA.S44377
56. Hotta K, Kondo S. Kanamycin and its derivative, arbekacin: significance and impact. J Antibiot (Tokyo). (2018) 71:417–24. doi: 10.1038/s41429-017-0017-8
57. Ji X, Mo T, Liu WQ, Ding W, Deng Z, Zhang Q. Revisiting the mechanism of the anaerobic coproporphyrinogen III oxidase HemN. Angew Chem Int Ed Engl. (2019) 58:6235–38. doi: 10.1002/anie.201814708
58. Choby JE, Skaar EP. Staphylococcus aureus Coproporphyrinogen III Oxidase Is Required for Aerobic and Anaerobic Heme Synthesis. Msphere. (2019) 4:e00235–19. doi: 10.1128/mSphere.00235-19
59. Huang S, Tong X, Rehman MU, Wang M, Zhang L, Wang L, et al. Oxygen supplementation ameliorates tibial development via stimulating vascularization in tibetan chickens at high altitudes. Int J Biol Sci. (2017) 13:1547–59. doi: 10.7150/ijbs.22670
60. Mehmood K, Zhang H, Jiang X, Yao W, Tong X, Iqbal MK, et al. Ligustrazine recovers thiram-induced tibial dyschondroplasia in chickens: Involvement of new molecules modulating integrin beta 3. Ecotoxicol Environ Saf. (2019) 168:205–11. doi: 10.1016/j.ecoenv.2018.10.080
61. Zhang H, Mehmood K, Jiang X, Li Z, Yao W, Zhang J, et al. Identification of differentially expressed MiRNAs profile in a thiram-induced tibial dyschondroplasia. Ecotoxicol Environ Saf. (2019) 175:83–9. doi: 10.1016/j.ecoenv.2019.03.043
62. Hajikhani B, Goudarzi M, Kakavandi S, Amini S, Zamani S, van Belkum A, et al. The global prevalence of fusidic acid resistance in clinical isolates of Staphylococcus aureus: a systematic review and meta-analysis. Antimicrob Resist Infect Control. (2021) 10:75. doi: 10.1186/s13756-021-00943-6
63. Dong L, Xu WW Li H, Bi KH. In vitro and in vivo anticancer effects of marmesin in U937 human leukemia cells are mediated via mitochondrial-mediated apoptosis, cell cycle arrest, and inhibition of cancer cell migration. Oncol Rep. (2018) 39:597–602. doi: 10.3892/or.2017.6147
64. Kim JH, Kim MS, Lee BH, Kim JK, Ahn EK, Ko HJ, et al. Marmesin-mediated suppression of VEGF/VEGFR and integrin beta1 expression: Its implication in non-small cell lung cancer cell responses and tumor angiogenesis. Oncol Rep. (2017) 37:91–7. doi: 10.3892/or.2016.5245
65. Prompipak J, Senawong T, Sripa B, Ketterman AJ, Utaiwat S, Woranam K, et al. Anticancer effects of the combined Thai noni juice ethanolic extracts and 5-fluorouracil against cholangiocarcinoma cells in vitro and in vivo. Sci Rep. (2021) 11:14866. doi: 10.1038/s41598-021-94049-z
66. Hu W, Dong T, Wang L, Guan Q, Song L, Chen D, et al. Obesity aggravates toxic effect of BPA on spermatogenesis. Environ Int. (2017) 105:56–65. doi: 10.1016/j.envint.2017.04.014
67. Bjorndal B, Alteras EK, Lindquist C, Svardal A, Skorve J, Berge RK. Associations between fatty acid oxidation, hepatic mitochondrial function, and plasma acylcarnitine levels in mice. Nutr Metab (Lond). (2018) 15:10. doi: 10.1186/s12986-018-0241-7
68. Sheng C, Zhang S, Zhang Y. The influence of different polymer types of microplastics on adsorption, accumulation, and toxicity of triclosan in zebrafish. J Hazard Mater. (2021) 402:123733. doi: 10.1016/j.jhazmat.2020.123733
69. Ueland T, Svardal A, Oie E, Askevold ET, Nymoen SH, Bjorndal B, et al. Disturbed carnitine regulation in chronic heart failure—increased plasma levels of palmitoyl-carnitine are associated with poor prognosis. Int J Cardiol. (2013) 167:1892–99. doi: 10.1016/j.ijcard.2012.04.150
70. Guo M, Lin Q, Xu Z, Zhang C, Zhao X, Tang T. Adsorption-desorption behavior of the endocrine-disrupting chemical quinestrol in soils. Sci Rep. (2020) 10:13273. doi: 10.1038/s41598-020-70300-x
71. Sidhu A, Singla N, Lonare M, Mahal AK. Effect of quinestrol on body weight, vital organs, biochemicals and genotoxicity in adult male lesser bandicoot rat, Bandicota bengalensis. Pestic Biochem Physiol. (2020) 165:104544. doi: 10.1016/j.pestbp.2020.02.010
72. Mahalakshmi K, Parimalanandhini D, Sangeetha R, Cathrene ML, Beulaja M, Thiagarajan R, et al. Influential role of 7- Ketocholesterol in the progression of Alzheimer's disease. Prostaglandins Other Lipid Mediat. (2021) 156:106582. doi: 10.1016/j.prostaglandins.2021.106582
73. Xu L, Gao S, Zhao H, Wang L, Cao Y, Xi J, et al. Integrated Proteomic and Metabolomic Analysis of the Testes Characterizes BDE-47-Induced Reproductive Toxicity in Mice. Biomolecules. (2021) 11:821. doi: 10.3390/biom11060821
74. Tang J, Hu B, Zheng H, Qian X, Zhang Y, Zhu J, et al. 2,2′,4,4′-Tetrabromodiphenyl ether (BDE-47) activates Aryl hydrocarbon receptor (AhR) mediated ROS and NLRP3 inflammasome/p38 MAPK pathway inducing necrosis in cochlear hair cells. Ecotoxicol Environ Saf. (2021) 221:112423. doi: 10.1016/j.ecoenv.2021.112423
Keywords: thiram, liver, metabolism, chicken, pesticides
Citation: Wang M, Wang L, Shabbir S, Zhou D, Shahid MA, Luo H, Li H, Li Z, Sun X, Wu C and Zhao Y (2023) Effects of thiram exposure on liver metabolism of chickens. Front. Vet. Sci. 10:1139815. doi: 10.3389/fvets.2023.1139815
Received: 07 January 2023; Accepted: 06 February 2023;
Published: 28 February 2023.
Edited by:
Isa Ozaydin, Kafkas University, TürkiyeReviewed by:
Ahrar Khan, Shandong Vocational Animal Science and Veterinary College, ChinaZhiyuan Fang, Jilin University, China
Copyright © 2023 Wang, Wang, Shabbir, Zhou, Shahid, Luo, Li, Li, Sun, Wu and Zhao. This is an open-access article distributed under the terms of the Creative Commons Attribution License (CC BY). The use, distribution or reproduction in other forums is permitted, provided the original author(s) and the copyright owner(s) are credited and that the original publication in this journal is cited, in accordance with accepted academic practice. No use, distribution or reproduction is permitted which does not comply with these terms.
*Correspondence: Yan Zhao, yanzhao_wk@163.com
†These authors have contributed equally to this work