- 1Emerging Pathogens Institute, University of Florida, Gainesville, FL, United States
- 2Department of Infectious Diseases and Immunology, College of Veterinary Medicine, University of Florida, Gainesville, FL, United States
- 3Southeastern Center of Excellence in Vector Borne Diseases, Gainesville, FL, United States
- 4Department of Veterinary Pathobiology, School of Veterinary Medicine and Biological Sciences, Texas A&M University, College Station, TX, United States
- 5Laboratory of Parasitology, Federal University of the Agreste of Pernambuco, Garanhuns, PE, Brazil
Filarioid nematodes, which are vector-borne parasites of cosmopolitan distribution, of dogs are medically important. They are represented by species in which microfilariae were found to be circulating in the bloodstream (e.g., Dirofilaria sp., Acanthocheilonema sp., and Brugia sp.) or skin-dwelling (e.g., Cercopithifilaria sp. and Onchocerca sp.). Those species whose microfilariae are detected in blood have been extensively studied, especially Dirofilaria immitis, due to their clinical importance. In recent decades, there has been an increased interest by the scientific community in filarioid nematodes whose microfilariae are detected in the skin because of the zoonotic aspect of Onchocerca lupi. In the United States (US), although D. immitis has been considered the main filarioid infecting dogs, the intense animal movement and global canine filarioid diversity may indicate that the likely presence of cutaneous filarioid nematodes is more common than previously expected. Hence, a question remains: Are these canine filarioid nematodes emerging, neglected, or simply underdiagnosed in the US? In this review, we provide an overview of pertinent information that briefly summarizes the biology of the different canine filarioid nematode species, clinical signs associated with infections, and currently available diagnostic tools using molecular and microscopy-based methods and highlight knowledge gaps where research and surveillance efforts remain necessary. The data herein presented serve as an alert to the scientific community about the importance of filarioid nematodes infecting dogs other than D. immitis. Additionally, the zoonotic potential of several filarioid species reinforces the necessity of a proper diagnosis and the need for broader surveillance to understand their diversity and distribution, to highlight the potential introduction of certain species, and mitigate their establishment in the country and new animal and human cases.
1. Introduction
Parasitic nematodes within the family Onchocercidae (order Spirurida, superfamily Filarioidea) are of significant medical and veterinary importance globally. Species within all genera are transmitted to the vertebrate definitive host via blood-feeding arthropod intermediate hosts including mosquitoes, flies, fleas, lice, and ticks (1–4). A recent study that revisited the phylogenetic relationships among Onchocercidae proposed changes in the previously accepted subfamily-level taxonomic classification (2, 5). The family is composed of 5 distinct clades, namely, ONC-1 to ONC-5. Species within two genera within the ONC3 clade, Dirofilaria and Onchocerca; two genera within ONC4, Acanthocheilonema and Cercopithifilaria; and one genus in ONC5, Brugia, are known to infect domestic dogs (2, 6). From a global perspective, at least 14 onchocercid species are known to infect dogs, including those whose microfilariae are primarily found in the blood, Acanthocheilonema reconditum, Acanthocheilonema dracunculoides, Acanthocheilonema sp. “Ladhki genotype,” Brugia ceylonensis, Brugia malayi, Brugia pahangi, Brugia patei, Dirofilaria sp. “hongkongensis genotype,” Dirofilaria immitis, and Dirofilaria repens, and those with microfilariae are detected in the skin, Cercopithifilaria bainae, Cercopithifilaria grassi, Cercopithifilaria sp. II, and Onchocerca lupi (7–15). Adult nematodes of most species responsible for canine filariosis are found primarily in the subcutaneous and connective tissues and the lymphatic system. Some are also exceptionally adapted to the pulmonary arteries and associated vasculature, as in the case of D. immitis.
Global animal movement and pet travel within and between countries and over large geographic distances have been recognized as a global veterinary and public health concern, causing the dissemination of vectors and potentially zoonotic infections (parasitic, viral, and bacterial) into new areas (16). Dog importation into the United States (US) was estimated at ~1.06 million in 2019, according to a USDA report with ~36% of those imports coming across land borders with Canada (12%) and Mexico (~24%) (17). Following decades of animal movement, importation and considering the global canine filarioid diversity, where are the manifestations of clinical cases in shelter and pet populations, as well as in humans? Are these canine filarioid nematode species emerging, neglected, or simply underdiagnosed in the US?
Active research, scientific awareness, and veterinary medical knowledge of filarioid nematode diversity infecting domestic and wild carnivores in some areas of the Old World, particularly Europe, are much further established than those of the New World, including North, Central, and South America. Among several factors contributing to the lack of knowledge for filarioid species in domestic dogs in the US, is the repeated assumption that heartworm is the only filarioid of clinical importance across the New World. A result of this assumption and an overreliance on heartworm antigen testing was an established perception that testing for microfilariae detection had limited clinical value and was not necessary (18–20). However, in the absence of routine microfilariae testing or an awareness of other canine filarioid nematodes, frontline veterinarians in the US are unlikely to find or suspect any filarioid species other than heartworm in their canine patients, and to a lesser extent, A. reconditum, except for the chance of an incidental finding. A recent example that should cause alarm is that in only one of the 10 US surveys reporting microfilariae testing in dogs (21–27) conducted since 1989 (28, 29), ~2% of dogs and cats in a south Florida shelter had circulating microfilariae morphologically identified as D. repens by veterinary parasitologists performing modified Knott's testing and molecularly confirmed by sequencing, although these sequences were not made available in any repository (23). This finding in a dog and a cat in the same US shelter strongly suggests that local transmission of this zoonotic parasite may be occurring in our highest-risk populations, in an area supporting competent mosquito vectors and where shelter animal evacuations are frequent due to hurricanes. While the origin and detailed travel history of these animals were unknown, it is likely that additional infections with D. repens and other imported filarioid nematodes are present in US shelter, rescue, and pet populations. Previous reports described a dog in New York that was microfilaremic for D. repens, and blood samples from six animals were submitted to a reference lab for microfilariae identification after the heartworm antigen test result was negative (30). The dog had originated in the Czech Republic and moved through the Netherlands and Canada before arriving in New York (30). Animals that are microfilaremic from non-endemic filarioids pose a risk of initiating cycles of autochthonous transmission within the US or non-endemic areas, being detectable only by microfilaria testing or clinical presentation. These examples underscore the need for microfilariae testing and broad awareness of possible emerging parasites among veterinary researchers, veterinarians, and public health entities. Despite advances in molecular diagnostic tools capable of detecting and differentiating filarioid nematodes to species-level, no published D. immitis or blood microfilariae prevalence or research surveys have applied molecular tools alone or in combination with morphological identifications for screening in the US prior to 2020 for sheltered dogs (23–25) and wild canids (31), or prior to 2022 for pet dogs (26, 27, 32).
Historically, A. reconditum and D. immitis have been recognized as endemic in the US, with O. lupi and C. bainae being unequivocally recognized only since 2011 and 2019, respectively (33–35). Most global research and surveillance efforts for filarioid nematodes of dogs have focused on the detection and differentiation of D. immitis, due to its major veterinary importance, or D. repens and multiple Brugia species of zoonotic importance in Europe, south and southeastern Asia, and Africa, where dogs, cats, and other carnivores may serve as reservoirs of infection (26). While generally considered neglected, the subcutaneous canine filarioid nematodes with dermal microfilariae such as O. lupi did not gain research attention until it was attributed to human cases in areas where this parasite is endemic, in particular within the southwestern US (36–39). In addition to O. lupi, research on the presence of Cercopithifilaria in the US has only recently been increasing (35, 40, 41) following the plethora of published research and case reports, originating from Europe, primarily Italy, and the broader Mediterranean region, which has highlighted possible differentials for clinical presentations attributed to these neglected nematodes (42–44).
Awareness of potentially imported or emerging parasites, and particularly canine filarioid nematodes, is lacking among shelter and general practice veterinarians. Active efforts to inform veterinarians of emerging parasitic infections are needed through conferences and continuing education opportunities. Additionally, proactive surveillance is needed by veterinary parasitologists and ideally, medical entomologists or public health offices involved in vector-borne disease (VBD) surveillance of mosquito, biting fly, and tick vectors. It is essential that all relevant case reports of emerging filarioid nematodes from diagnostic and reference laboratories were published or otherwise formally communicated among peers. This is particularly important for species with known zoonotic potential, even without the need for official communication with or reporting to state or federal authorities. The purpose of this review article is to provide an overview of pertinent information that briefly summarizes the biology of the different canine filarioid nematode species, clinical signs associated with infections, and currently available diagnostic tools using molecular and microscopy-based methods, as well as to highlight knowledge gaps where research and surveillance efforts remain necessary.
2. Subcutaneous filarioid nematodes with dermal microfilaria
2.1. Cercopithifilaria spp.
2.1.1. Biology
The genus Cercopithifilaria (Filarioidea, Onchocercidae) is composed of 28 species infecting a variety of mammal definitive hosts, including primates, carnivores, and ungulates (45). In domestic dogs, three species have been described or reported, namely, C. grassi, C. bainae, and a distinct species that has not been formally described, Cercopithifilaria sp. II sensu (42). Adults of Cercopithifilaria species are found in the subcutaneous tissues of dogs (46, 47). The first description of Cercopithifilaria infecting dogs dates from 1907 in Italy when Noè detected microfilariae in skin samples (48). Initially, this species was named Filaria grassii and later transferred to the genus Cercopithifilaria (49). Cercopithifilaria bainae was originally described based on specimens isolated from dogs in Brazil (50), and it has recently been redescribed based on material from dogs in Italy using integrated classical and molecular methods (51).
Although known for a long time, the biology of Cercopithifilaria species has been poorly investigated (52, 53). Knowledge related to the development of this parasite in vertebrate hosts is almost non-existent. Microfilariae are unevenly distributed on the dog's body but seem to have a predilection for the head, the ears, and the neck, which coincidentally are the preferred sites of attachment of the brown dog tick, Riphicephalus sanguineus sensu lato (s.l.), the only proven intermediate host for C. bainae (54). Despite the fact that C. bainae microfilariae occur in the skin, three previous studies have reported the occurrence of its microfilariae in the bloodstream (55–57), a possible introduction during blood collection by venipuncture. While unusual, this finding is important to consider during morphological or molecular diagnosis of filarioid species with blood microfilariae.
The only unequivocally proven intermediate host for C. bainae is the brown dog tick, R. sanguineus (s.l.) (52). A prepatent period of <6 months is suggested (54). It was previously demonstrated experimentally that infection is acquired during the nymphal blood meal and passed by transstadial transmission to adults as the L3 infective stage ~30 days after nymph detachment (52, 53). The most recent update on the biological development in the tick vector for C. bainae originated from researchers who conducted retrieval in dogs from Italy (46, 52). In this study, larvae inside ticks were classified into four different developing types based on their morphometrical features, with sizes ranging from 191.4 μm (±9.1; DL1—developing first stage) to 1,707 μm (±70.5; L3–infective third stage larvae) (52). The potential role of other tick species such as Ixodes ricinus was also assessed but without success (53). More recently, the DNA of C. bainae was detected in Rhipicephalus haemaphysaloides from India, which suggest that ticks from different species or genera may be putative vectors for this nematode (47). However, it is important to highlight that further studies are needed to prove the potential vectorial role of this later species. Despite limited data regarding the adult C. bainae nematodes, these have been found in the subcutaneous tissues of the trunk and forelimbs and less frequently in the perirenal adipose tissue (51).
Various studies suggested that R. sanguineus (s.l.) may comprise a species complex (58, 59). In the US, the presence of two R. sanguineus lineages has been molecularly recognized, a “temperate lineage” and a “tropical lineage,” both with wide and overlapping distributions across the country (60). Thus, far, the DNA of C. bainae has been obtained only from R. sanguineus specimens belonging to the “temperate lineage” (40). It remains to be confirmed whether “tropical lineage” brown dog ticks are also capable of transmitting C. bainae and whether there is any difference in vector competence between lineages.
2.1.2. Geographic distribution
The geographic distribution of C. bainae is vast and overlaps with that of R. sanguineus (s.l.) (52, 53, 61). Overall, Cercopithifilaria species infecting dogs are widespread, being reported on different continents. In the US, there are only three publications reporting the occurrence of C. bainae in dogs or ticks (Table 1). The first report of C. bainae in the US was from a dog from Florida (35), and the second was from shelter dogs and R. sanguineus (s.l.) ticks from Oklahoma (40). Subsequently, an extensive study that molecularly screened R. sanguineus (s.l.) ticks collected from dogs detected DNA of C. bainae in larval, nymphal, and adult brown dog ticks from 11 different American states, including Florida and Oklahoma (41).
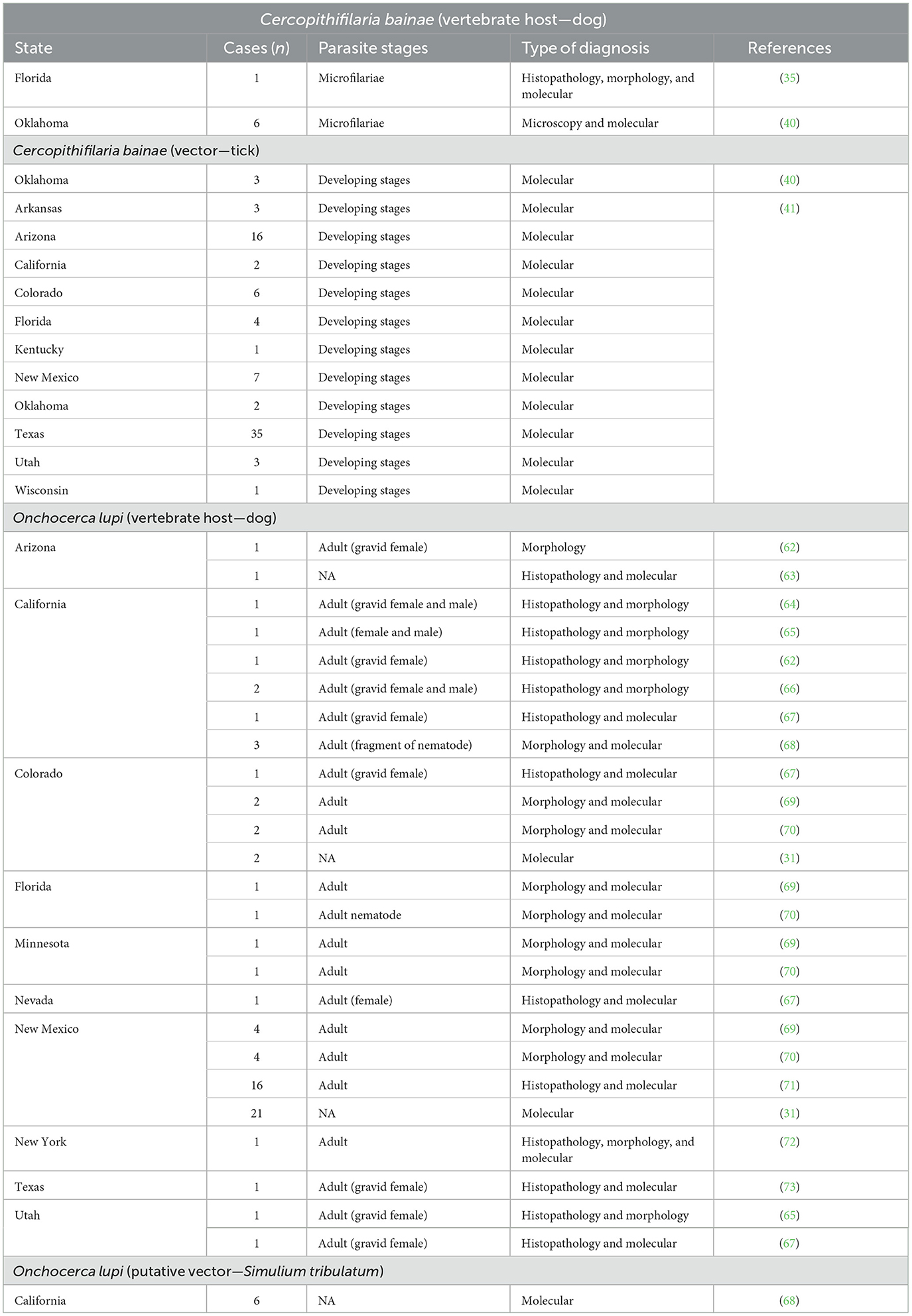
Table 1. Reports of Cercopithifilaria bainae and Onchocerca lupi infection in dogs and vectors in the US.
2.1.3. Pathogenicity, clinical signs, and diagnosis
The pathogenicity of Cercopithifilaria species infecting dogs has not been fully elucidated. Nonetheless, there are few reports in which C. bainae has been suggested as cause of dermatitis and polyarthritis in dogs from Europe (54, 74), dermatitis in the US (35), and more recently to the presence of a giant cutaneous cyst in a dog from Brazil (75). First, skin alterations recorded in a dog in Italy were characterized by a diffuse, erythematous, papular dermatitis (54). During the histological examination, the presence of neutrophils, eosinophils, and lymphocytes was observed in association with microfilariae (54). Afterwards, a 7-year-old dog from the same country presented a history of reluctance to move, lethargy, and lameness. With the exclusion of other potential pathogens, the presence of C. bainae in the synovial fluid was considered an aberrant localization that most likely triggered an inflammatory reaction (74). It is believed that this reaction is similar to that induced by Cercopithifilaria johnstoni in the perivascular connective tissues of infected rodent definitive hosts (76).
In the US, a dog from Florida with no domestic or international travel history presented with an annular erythematous plaque on the head and ulcers on the medial canthi that were unresponsive to antibiotic treatment (35). The histopathological evaluation revealed an eosinophilic to lymphohistiocytic perivascular dermatitis associated with C. bainae microfilariae. The dog was treated with a commercial spot-on formulation containing imidacloprid and moxidectin, and clinical resolution was achieved (35). In Brazil, a recent report describes a 9-year-old male mixed-breed dog who presented with a mass in the lumbosacral region. At the cytological examination, moderate lymphocyte cellularity, foamy macrophages, and erythrophagocytosis were observed. The presence of numerous microfilariae was also detected in the cyst fluid, subsequently identified as C. bainae (75). Although speculative, the large number of microfilariae in the cyst fluid and the absence of coinfection with other pathogens may suggest a potential involvement of this filarioid species. For the first-time subcutaneous effusion and C. bainae microfilariae were noticed together, suggesting an intense immune response against the parasite, with probably fluid sequestration or lymphatic obstruction (75).
The diagnosis of Cercopithifilaria spp. infection in dogs is discussed below, along with that of O. lupi.
2.2. Onchocerca lupi
2.2.1. Biology
The genus Onchocerca comprises more than 30 valid species, the vast majority infecting wild and domestic ungulates, with a few exceptions such as O. lupi, which infects carnivores, and O. volvulus, which infects humans (6). Many Onchocerca species associated with animal hosts have been shown to be zoonotic, including O. lupi and other species associated with ruminants and suid hosts.
Similar to all filarioid nematodes, O. lupi has an indirect life cycle, and dogs seem to be its most common definitive host (77). However, the species was originally described from a wolf (78), and there have been many reports of infection in cats (34, 79, 80), coyotes (Canis latrans) (81), and humans (38, 39, 82, 83). Usually, the adult worm develops within the connective tissues, particularly the ocular conjunctiva, and microfilariae are released near these regions, eventually being detected in the skin of the definitive host. Microfilariae must be ingested by an arthropod intermediate host(s), in which it will develop to the infective third-stage larvae.
The vector or vectors of O. lupi remain unknown, but based on the biology of other Onchocerca species, it is believed that black flies (Diptera, Simuliidae) and/or biting midges (Diptera, Ceratopogonidae) may act as intermediate hosts. Even if not biologically confirmed, this hypothesis is reinforced by the retrieval of O. lupi DNA in Simulium tribulatum from southern California (68), a species vastly distributed across North America (84). Despite not being featured in any peer-reviewed publication, there have been O. lupi sequences isolated from the heads and bodies of Simulium griseum from New Mexico, further supporting the putative role of black flies as suitable intermediate hosts. Several studies in Europe failed in the attempt to confirm the role of black flies and other biting flies as biological vectors of O. lupi, likely due to poor sampling (77, 85, 86). It is known that this parasite species presents a wide geographic distribution; thus, the possibility of multiple arthropod species acting as vectors (e.g., as occurs with Onchocerca volvulus, the agent of river blindness in humans in Africa and Latin America) cannot be ruled out (87). Biting midges (Ceratopogonidae) should also be further investigated as possible vectors since they are known to transmit multiple Onchocerca species in the US including the equine O. cervicalis, and the bovine O. gutturosa (88–91) as well as others worldwide (1, 92). Although a wide range of Culicoides species are present in O. lupi endemic areas of the southwestern US, many of which are documented to feed primarily on humans (93), two subspecies within the Culicoides variipennis complex, Culicoides v. sonorensis and Culidoides v. occidentalis (94), are known to feed on both dogs and humans (93), and Culidoides v. variipennis has been shown to naturally and experimentally transmit O. cervicalis to horses in the US (95, 96). Additionally, the apparent distribution of Culidoides v. occidentalis (94) encompasses the known endemic areas in the southwestern US coinciding with human, companion animal, and coyote cases (70, 81). The combined morphological and phylogenetic evidence suggest that O. lupi has a recent evolution history and clusters with human and bovine Onchocerca spp. (6, 82, 97), and considering that the known bovine-derived Onchocerca spp. (O. ochengi, O. gutturosa, and O. gibsoni) are all vectored by species of Culicoides (88–91, 98), it should provide further support for examination of mammalophilic Culicoides spp. in natural populations or experimentally. Additionally, in the Amazon of South America, presumed infective larvae of an Onchocerca species most closely related to O. lupi based on phylogenetic analysis using the COI gene were found in the labium of the proboscis and head of two sandflies (Psychodidae, Phlebotominae), suggesting this group of biting flies should also be investigated in relevant O. lupi endemic regions (99).
Despite the data presented in clinical reports of onchocercosis in dogs, information about the biological aspects of this nematode in this vertebrate host remains scarce. For instance, the pre-patent period and patency of O. lupi are unknown. Onchocerca lupi microfilariae have been found in higher abundance on the skin of the ears and nose, followed by the forehead and interscapular area of naturally infected dogs (100). It is possible that the choice of anatomic location(s) for skin sampling may impact the prevalence estimate for O. lupi in epidemiological studies. A single study has shown that O. lupi microfilariae were detected in higher abundance in skin samples collected in the afternoon, followed by night and morning (100), likely coinciding with periods of higher activity of suitable vectors. More recently, it has been reported that large black dogs presented a higher risk to develop onchocercosis compared with similar sized brown or white ones (101). It has been well-established that various hematophagous dipteran species, including black flies, are more attracted to darker colors and therefore tend to feed on darker animals (101–103). Regarding a dog's body size, it may be hypothesized that a larger dog likely emits more CO2 than smaller ones, making it more attractive to host-seeking dipterans. On the contrary, there could be various additional factors influencing these variables, including time spent outdoors.
2.2.2. Geographic distribution
After decades from the original description of O. lupi, a few cases of Onchocerca infection were reported in dogs from the US (62, 64). Initially, the identity of the etiological agent was putatively attributed to a species other than O. lupi (e.g., Onchocerca lienalis), as there were no records for the species from the New World. Later, four cases of canine ocular onchocercosis were reported in Hungary, and the morphologically informative features of this nematode allowed species-level confirmation of the agent as O. lupi (104). After the confirmation of O. lupi infection using integrated morphological and molecular methods in dogs, cats, and humans in the US (34, 36, 67), it has been assumed that previous cases of ocular infection in dogs and humans could be attributed to this species. For instance, a revisited study demonstrated that two filarial ocular human cases in Turkey (originally identified as D. repens) and Tunisia (originally identified as D. immitis) were attributed to O. lupi (105). The interest of researchers, veterinarians, and public health professionals has substantially grown in North America and the Old World.
In more recent years, there have been multiple O. lupi reports in companion animals, especially in dogs and less frequently in cats, worldwide, including in North America (34, 64, 66, 67, 70, 73, 106). Coincidentally, the highest incidence of clinical O. lupi infections in dogs is in the southwestern US, in special New Mexico and Arizona (71, 107), where several human cases have been reported (38, 39). In addition, coyotes, which are vastly distributed across North America, were demonstrated as O. lupi hosts and may act as wild reservoirs (81). There has been strong evidence that this filarioid may be undergoing range expansion into new areas, perhaps promoted by the movement of dogs, both domestically and internationally. For instance, two adoption-mediated cases of canine ocular onchocercosis have been reported from Canada (106), with animals coming from endemic areas of the US. Similarly, in the US, there have been increasing reports of ocular onchocercosis in dogs from areas currently considered non-endemic. More recently, an autochthonous case has been reported from south Texas, expanding its known endemic distribution in North America (73). Since this publication, the authors have confirmed additional O. lupi cases in dogs from Texas; nevertheless, its prevalence and distribution across the state remain unknown. Table 1 shows all published reports of O. lupi infection in dogs in the US; however, the authors have confirmed via classical and molecular methods dozens of additional cases.
2.2.3. Pathogenicity, clinical signs, and diagnosis
In dogs, O. lupi is generally responsible for the ocular disease (69, 71, 73), which may be characterized by acute or chronic ocular signs (100). The pathogenicity of this parasite is mainly related to the presence of adult worms in the extraocular tissues, which is usually accompanied by the presence of a mass or swelling in the ocular region (101). In most cases, the swelling occurs due to the presence of epithelioid macrophages and multinucleated giant cells (71), as a result of a host response against the parasite. Several clinical signs may be observed, such as lacrimation, conjunctivitis, exophthalmia, retinal detachment, and the presence of granulomatous nodules, which may lead to blindness (70, 82).
Clinical signs associated with ocular disease may be present in one or both eyes (71); however, data from epidemiological surveys suggest that many animals may remain asymptomatic and would likely go undiagnosed. In addition to the classical ocular presentation of O. lupi infection, a few cases of aberrant localization of O. lupi have been reported, including the respiratory system (108, 109). For instance, in Portugal, in a dog with a history of acute and severe dyspnea and cyanosis, adult nematodes were detected in the laryngeal region (109). Similarly, in Greece, in an animal presenting coughing, difficulty in breathing, and edema near the laryngeal area, microfilariae were detected in buccal lymph nodes (110). More recently, a case of extensive aberrant localization of O. lupi was described in the US, with nematodes or degenerate nematodes detected in different anatomical regions (e.g., episclera, trachea, subcutis around the nares, external ear canals, parietal pleura, pericardium, and laryngeal cartilage) (63). While the pathogenicity of O. lupi is known to be associated with adult nematodes, it remains unclear whether the presence of its skin-dwelling microfilaria may cause dermatitis or any dermatological condition, similar to O. volvulus (111).
Filarioid nematodes whose microfilariae are skin-dwelling have been neglected in veterinary medicine for a long time. Undoubtedly, infections by Onchocerca and Cercopithifilaria are less commonly diagnosed than those by filarioid nematodes whose microfilariae are circulating, such as D. immitis, D. repens, A. reconditum, and A. dracunculoides (112). In this context, it is paramount to increase the awareness of veterinarians and researchers about their veterinary and public health relevance and better inform them on how to diagnose these neglected parasites, which often require integrated classical, microscopy-based methods and molecular tools.
The diagnosis of skin-dwelling filarioid infections can be achieved by morphometric analysis of microfilariae isolated from a skin sample via microscopy (Figures 1A, B). Briefly, skin samples may be collected using a disposable scalpel or a biopsy punch (from 3 to 8 mm) after shaving the hair over the collection area. The skin sample is then soaked in saline solution for 10 min at 37°C or at room temperature overnight. The sediment is collected and examined under light microscopy as is or after adding a drop (50 μl) of methylene blue at 1% (46, 100) and observed under light microscopy at × 100 magnification.
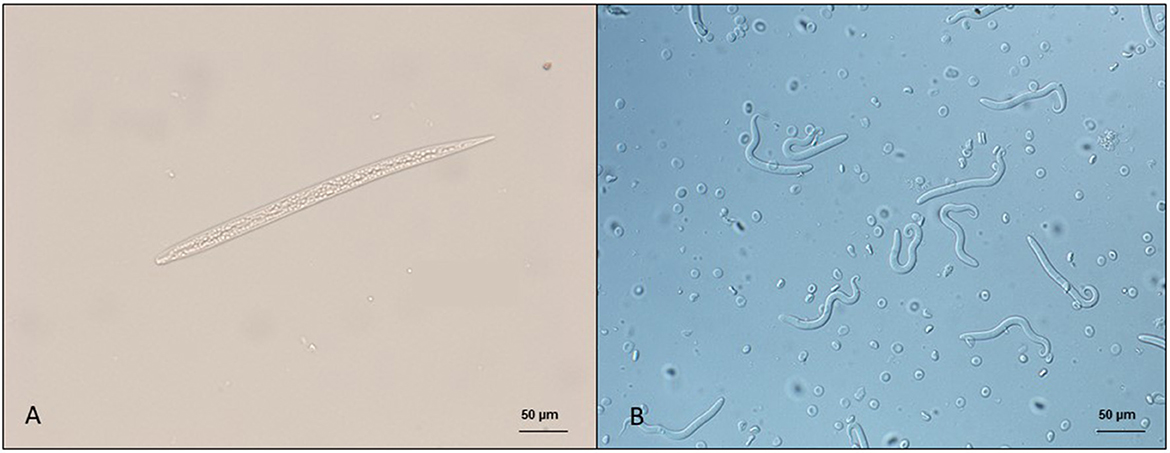
Figure 1. Microfilariae of dermal filarioids in dogs. (A) Cercopithifilaria bainae microfilaria isolated from a skin sample; scale bar: 50 μm. (B) Onchocerca lupi microfilariae recovered from the uterus of an adult nematode; scale bar: 50 μm.
Previous studies demonstrated that both Cercopithifilaria and Onchocerca microfilariae are unevenly distributed on the dog's body, with predominance in specific areas coinciding with vector feeding behavior for C. bainae (54). In dogs, microfilariae have higher frequencies on inter scapular region and on the head (54), known common (i.e., scapular region and head) sites of tick attachment. Hence, the predominance of microfilariae in these anatomical regions may be related to the long feeding period of ticks and consequently to Cercopithifilaria transmission (54). A similar study conducted with O. lupi positive dogs demonstrated a predominance of microfilariae on the nose and ears of dogs (100). Previously, the peri-ocular and umbilical areas were considered preferential sites for skin snipping (104). However, in this preceding research, the number of microfilariae was most likely underestimated as the skin snips were soaked for a short period of time (i.e., 1 h) (104), compared to up to 12 h in the more recent study (100). Data about predilection sites of microfilariae distribution and the time of skin soak described above are extremely useful to veterinarians since may increase the sensitivity of the technique employed. Nonetheless, considering that the collection of skin fragments is an invasive procedure, other factors such as practicability and vascularization of the anatomical region might be considered (100).
Morphometric analysis under microscopy is imperative for genus- or species-level identification of microfilariae recovered from skin snips as well as those circulating in the blood stream. Even if morphometric data of these microfilariae have been provided in previous research, these often do not consider some important aspects including age and origin of the material or detailed fixation or staining methods employed. Both blood and dermal microfilariae may be recovered through different techniques such as modified Knott's, Giemsa or hematoxylin staining of blood smears, saline sedimentation, impression smears, fine needle aspirates, or cytology spin preps. Additionally, reports of molecular detection of C. bainae microfilariae in dog blood (55, 56, 112), although likely introduced from the skin during venipuncture, highlight the importance of an accurate morphometrical analysis of circulating microfilariae to avoid misdiagnosis. Morphological features for all known blood and dermal microfilariae species infecting dogs have been summarized in Table 2 for modified Knott's and saline sedimentation and in Supplementary Table 1 for blood smears.
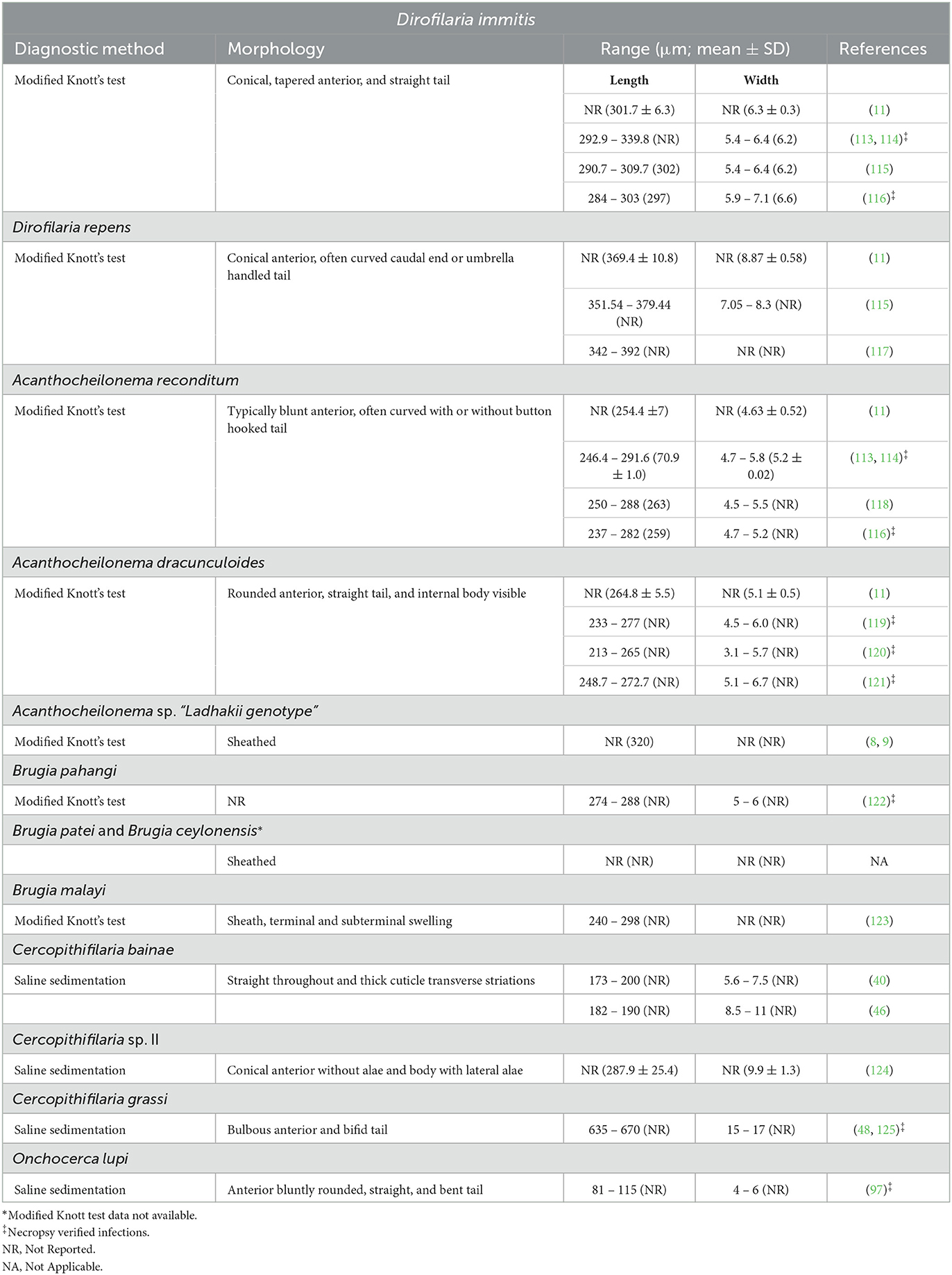
Table 2. Morphological and morphometrical data of microfilariae present in the blood and skin of dogs.
In addition to the research of microfilariae in skin snips, the retrieval of adult specimens (intact or degenerate) of O. lupi from ocular nodules has been a method of diagnosis that is obtained after surgical removal or biopsy of conjunctival tissue, followed by histopathological, morphological, and/or molecular identification (Figures 2A, B) (73, 126). At the histopathological examination, patterns of cuticular ridges of two inner striae within the space between two outer cuticular ridges have been observed and are morphologically compatible with O. lupi (73, 109). This procedure is invasive, and unfortunately, the diagnosis is achieved in animals after the presentation of clinical signs (73). Most often, these studies further confirm the morphological identification with DNA extraction, followed by different molecular tools (126).
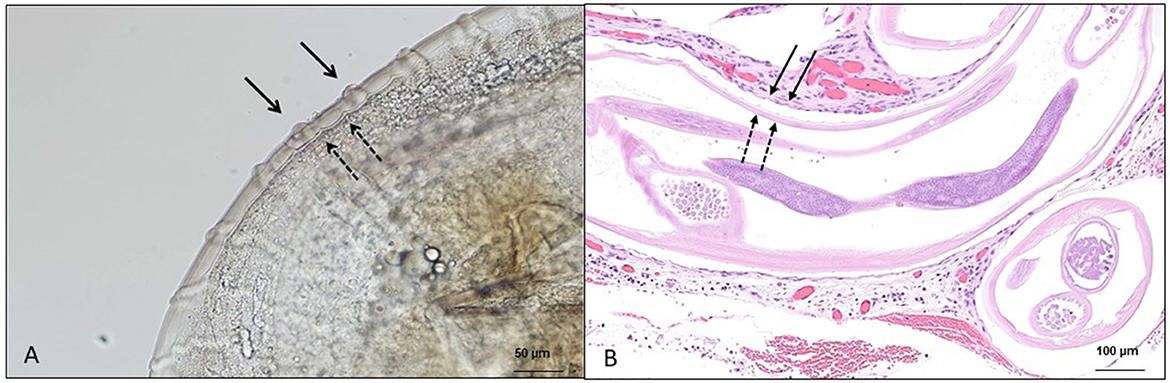
Figure 2. Microscopic features of Onchocerca lupi adults. (A) Multilayered cuticle with prominent annular ridges, external surface (full arrow), and internal layer (dashed arrow); scale bar: 50 μm. (B) Histological section of the ocular tissue of a dog with the presence of O. lupi, external surface (full arrow) and internal layer (dashed arrow); scale bar: 100 μm.
3. Subcutaneous filarioid nematodes with blood microfilariae
3.1. Acanthocheilonema spp.
3.1.1. Biology
The genus Acanthocheilonema has three species known to infect dogs, the most common being Acanthocheilonema reconditum (formerly Dipetalonema reconditum), A. dracunculoides (formerly Dipetalonema dracunculoides), and an undescribed species reported in the blood of dogs in Ladhak, northern India (8, 9). Adult nematodes of A. reconditum are present in the subcutaneous tissues of the trunk, hind limbs and fascial spaces (33, 114, 116, 127) and A. dracunculoides in subcutaneous tissues, peritoneal, thoracic, and abdominal cavities, or hind legs (120, 121, 128, 129). Of these, only A. reconditum has been reported from the United States.
Known arthropod vectors and development times to infective stage (L3) vary for the different canine Acanthocheilonema species. Acanthocheilonema reconditum is vectored by fleas, including Ctenocephalides canis and Ctenocephalides felis, and lice, Heterodoxus spiniger and Linognathus setosus (33, 127, 130). Development times in C. felis maintained on the infected host dog was 7–10 days (116, 131), whereas in off-host lab-maintained fleas fed an artificial bloodmeal, full development took 15 days (132). No development occurs in the brown dog tick, R. sanguineus (s.l) (132). The time of development for A. dracunculoides is unknown in the dog louse fly, Hippobosca longipennis (9, 129), and takes ~7 days in R. sanguineus (s.l.) nymphs (133). Interestingly, no developing forms were seen in the R. sanguineus (s.l.) ticks removed from a microfilaremic dog, which was found to be infected with adults at necropsy and co-infested by H. longipennis (129). In addition, it is unknown whether all members of the species complex of R. sanguineus (s.l.) ticks are equally competent vectors of A. dracunculoides, and this would require biological confirmation through experimental trials. Nevertheless, upon a potential introduction of A. dracunculoides to North America, R. sanguineus would be more likely to vector this species, as H. longipennis is not endemic to this region.
Infective larvae of A. reconditum have been experimentally transmitted via direct penetration of shaved skin and oral mucosa of anesthetized dogs (116, 131, 134). Whether transmission to the canine host occurs during vector blood feeding is uncertain, although it was noted by Pennington (116) that “infective larvae egressing from the intersclerical membranes around the mouth parts” and “infective larvae could be encouraged to come out” by manipulating the “mouthparts forward into a natural feeding position,” allowing escape through this membrane when stretched thin and finally allowing infective larvae to potentially penetrate the skin or bite wound (116). Ingestion of fleas was also suggested to be a more common route of infection (116, 132). It is plausible that multiple routes of infection in dogs may occur naturally.
Upon development and maturation in the subcutaneous tissues, gravid females release microfilariae into surrounding tissues and arrive in blood circulation 67–101-day post-infection for A. reconditum (Figures 3A–C), with a maximum patency period of 795 days (114, 116, 131, 134–136) and 69–76-day post-infection for A. dracunculoides (1, 131, 133, 136). Microfilaremia is often low in natural infections, ranging from 1 to 482 mf/ml of blood for A. reconditum to 5,050 mf/ml for A. dracunculoides. In a rare case, a dog from Spain with 791 adults recovered at necropsy, microfilaremia reached 264,367 mf/ml (121).
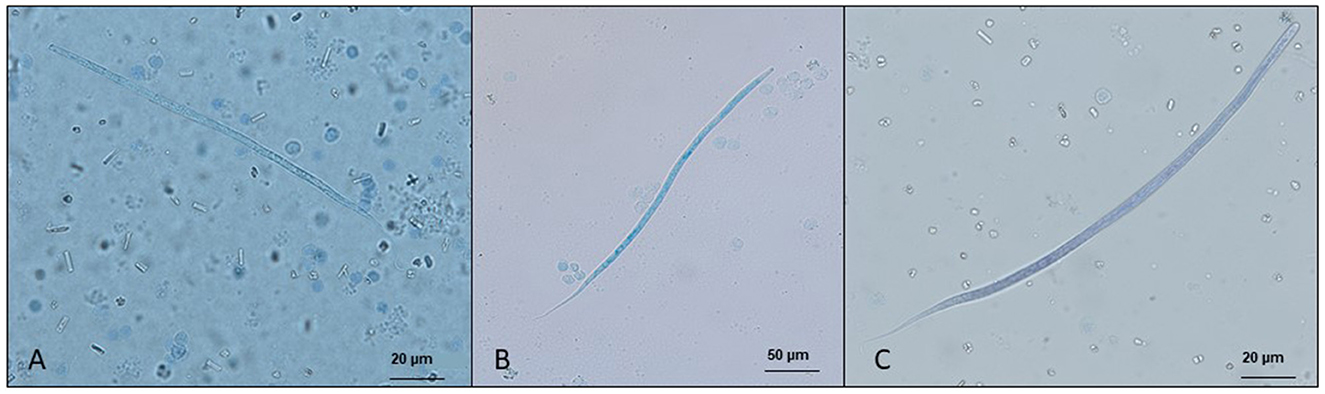
Figure 3. Microfilariae detected in blood samples by a modified Knott's test and stained with methylene blue. (A) Acanthocheilonema reconditum; scale bar: 20 μm. (B) Dirofilaria immitis; scale bar: 50 μm. (C) Dirofilaria repens; scale bar 20 μm.
3.1.2. Geographic distribution
While A. reconditum is considered globally distributed, A. dracunculoides is found in Europe (particularly Spain), Africa, and West Asia. The reported prevalence and distribution of both species are likely underestimated due to low numbers of microfilaremia and limitations of classical and molecular techniques, particularly in cases of co-infections with Dirofilaria species. Most surveillance for A. reconditum occurred prior to the adoption of the heartworm antigen tests in 1985, when clinical signs and identification of microfilariae were required for the diagnosis of heartworm. It has been reported in at least 22 states in the US (27, 137–139). Most likely, the distribution of A. reconditum is more widespread in the country; however, due to the lack of clinical relevance, cases routinely diagnosed by practitioners and diagnostic laboratories are not usually published. Epidemiological surveys and the prevalence of this parasite in Europe were recently summarized, reporting 64 surveys from 1987 to 2019 (140). In contrast, there have been scarce reports from the US during the same period (20, 21), which should underscore the need for more active surveillance using a combination of diagnostic methods, including classical (141), histochemical (142), and molecular methods (143). Despite the need for more active surveillance, the routine use of ectoparasiticide compounds observed in the last decades has likely contributed to the reduction of infection by Acanthocheilonema species in dogs.
3.1.3. Pathogenicity, clinical signs, and diagnosis
While generally considered a non-pathogenic parasite (52, 131), A. reconditum experimentally infected dogs presented with eosinophilia, and in chronic infections, this abnormality was accompanied by proteinuria presumed to be due to microfilariae (131). Newly reported molecularly confirmed cases in Colombia among dogs testing positive for A. reconditum were statistically associated with male dogs showing clinical signs of anemia, including low levels of hemoglobin and hematocrit and elevated levels of plasma proteins (144).
Currently, in vivo diagnosis of Acanthocheilonema spp. is mainly achieved by morphological or molecular identification of the microfilariae in the blood (27, 55). Morphometric differentiation by a modified Knott's test between the two established canine species of Acanthocheilonema is not reliable and requires acid phosphatase staining or molecular differentiation (11, 131). However, with the current assumption that only A. reconditum occurs in the US, it is likely that a potential A. dracunculoides case would be misdiagnosed. The best techniques to detect low microfilaremia, excluding xenodiagnoses (127), include filtration methods (145) and molecular detection of DNA via conventional or qPCR of whole blood samples. When coinfections are present, low Acanthocheilonema microfilaremia may be overlooked among the numerous Dirofilaria microfilariae. This co-infection scenario may also explain the reduced sensitivity of some species-specific PCR assays in cases where the ratio of one species to another is high (24, 146, 147) or the inability to molecularly confirm co-infections of A. reconditum and D. immitis seen following examination of the entire Knott's sediment (24). More accurate diagnosis may be achieved with more sensitive molecular assays such as single or multiplex probe-based PCR protocols (27, 57, 148), accompanied by the sequencing of amplicons as an additional confirmation.
A range of morphologic characteristics, including length, width, the shape of the anterior and posterior extremities, and acid phosphatase somatic staining patterns, were described for microfilariae of A. reconditum (118, 149, 150). This morphological variation for A. reconditum was acknowledged by Courtney et al. (151) in natural populations throughout Florida and by Lindemann et al. (131), who observed more morphometric variability among naturally vs. experimentally infected dogs for diagnostic features such as the blunt head and curved buttonhook tail and found acid phosphatase necessary to determine an identity for microfilariae 270–290 μm in length. Some studies have suggested that these observations are evidence that more than a single Acanthocheilonema species are present within the US, with no additional investigations published (118, 149, 150). Although, these differences in morphology could represent intraspecific variability within A. reconditum, an alternative hypothesis would be that A. dracunculoides may be present in the US, with acid phosphatase staining described by Price (150), matching those described for this species (152, 153). Representative molecular sequences of A. reconditum from the US are currently lacking in Genbank, as are those of A. dracunculoides from other parts of the world. Informative morphological, histochemical, and molecular data from microfilariae surveys in dogs and vectors in the US are lacking. As isolates are obtained, molecular confirmation by multiple gene targets and the addition of the sequences to public repositories are needed. The only published investigation of filarial DNA in the known vector R. sanguineus (s.l.) submitted by veterinarians from pet dogs, identified only in C. bainae, also transmitted by these ticks (40).
4. Other filarioid nematodes with “potential” cutaneous localization
4.1. Brugia spp.
Brugia spp. are filarial nematodes that parasitize the lymphatic system of several mammal species, including dogs and humans (154). Microfilariae are released into the bloodstream and developed into mosquito vectors (Culicidae) (1, 155). The transmission through mosquitoes, which have a broader distribution worldwide, it may contribute for the potential spread of these parasites in areas where vertebrate susceptible hosts are present and mosquito vectors are abundant (156). Despite being composed of at least 10 species, B. pahangi and B. malayi are those more studied due to their occurrence in human populations, especially in Africa and Asia (156, 157). In dogs, the pathogenic importance of these infections has been considered minimal, but in some cases, lymphadenomegaly and lymphedema may be observed (158). Other canine species reported in dogs include B. ceylonensis in India and Sri Lanka, B. patei in Kenya (Pate Island), and Brugia species presenting high similarity to B. malayi and B. pahangi in Chad (159, 160).
In the US, the infection in wild animals for Brugia beaveri, infecting Procyon lotor, Felis lynx, and possibly Neogale vison, and for Brugia lepori, infecting lagomorphs such as Sylvilagus aquaticus and Sylvilagus floridanus, has been documented (161–166), but no case in dogs has been reported. Recently, in Canada, an unusual case of Brugia infection was noticed in a dog presenting with a subcutaneous mass (167). Even if not detected specifically in the US, this case sounds like an alert for veterinarians in North America who might consider the potential for infection in dogs with different Brugia species. Additionally, Brugia infection has been reported in a cat from California (162) and various humans across the US (and Canada) (168–171). The diagnosis of Brugia species has been mentioned in the section that covers the morphological differentiation of microfilariae in the blood and skin of dogs.
4.2. Dirofilaria spp.
The genus Dirofilaria is composed of at least 27 species infecting carnivores, insectivores, and marsupials worldwide (172), with at least three species of importance infecting dogs. Most species within the genus Dirofilaria primarily develop into adults and remain in the subcutaneous tissues except for D. immitis found in the cardiopulmonary vasculature. Additionally, all Dirofilaria species develop up to infective L3 stage generally over 14 days dependent on temperature and are vectored especially by Culex spp., Aedes spp., and Anopheles spp. mosquitoes worldwide except for Dirofilaria ursi infecting bears that are vectored by black flies (Simulium spp.) (18, 173, 174). The life cycle of Dirofilaria species is long, ranging from 6 to 9 months for D. immitis and from 6 to 8 months for D. repens and Dirofilaria sp. (“hongkongensis” genotype) following infection to when microfilaria is seen in the blood stream. Several Dirofilaria species are documented to be zoonotic, and recently, a patent human infection of D. immitis and D. repens was reported in asymptomatic individuals (175).
Dirofilaria repens is the primary species causing canine subcutaneous dirofilariosis, which is endemic in Europe, southeast Asia, and Africa (176, 177). It is primarily of zoonotic importance but can be highly prevalent in dogs (178). Similar to other representatives of Dirofilaria, it can be transmitted by numerous mosquito species and may establish in the same areas suitable for D. immitis due to the possibility of competent vector sharing (173). Additionally, it can be easily confused with D. immitis in geographic areas where endemicity is not recognized. Adults are commonly found under the skin in the fascia sheaths of muscles (179). Despite that most D. repens canine cases infections are asymptomatic, non-specific clinical signs such as pruritus, itching, and asthenia may be present (176). In addition, alopecic areas with hyperpigmentation and an uncommon case of diffused dermatitis associated with D. repens have also been described (180). The recent introduction of non-endemic vector-borne canine pathogens such as D. repens, reported in ~2% of both dogs and cats in South Florida, should not be surprising with requirements for animal importation only requiring vaccination records, a negative rabies titer, or origination from a low-risk country (17). It is important to highlight that no antigen test is available for D. repens, and the only way to reach a differential diagnosis is through the morphometric features of circulating microfilariae or by molecular tools.
Dirofilaria immitis, the causative agent of canine heartworm disease, is regarded as the most important parasite infecting dogs, has a global distribution, and is the primary filarioid of research focus and surveillance in the canine population, particularly in the US. Importantly, the full developmental life cycle in the vertebrate host has been known for a long time (181). While primarily found in the pulmonary arteries, right heart, and associated vasculature, there are increasing case reports and awareness of its presence in other subcutaneous, extravascular, and aberrant locations manifesting in diverse clinical presentations (182–186). Nematodes found incidentally outside the cardiopulmonary vasculature, in nodules, subcutaneous tissues, or body cavities should not be dismissed as heartworm and should be submitted to a veterinary parasitologist or veterinary reference lab for definitive identification. Due to the overreliance on heartworm antigen tests and a lack of microfilaria testing, heartworm prevalence remains underestimated, as recently highlighted in studies using immune complex dissociation (ICD) methods such as acid or heat treatment (20, 24, 186). The pathogenicity of D. immitis infections is a consequence of the anatomical localization of adult parasites as well as the presence of circulating microfilariae (18). The most common clinical presentation is chronic, characterized by dyspnea, weakness, anorexia, and ascites, which may be triggered as a consequence of heart failure (18). Because the pathogenicity of D. immitis infections has been extensively studied, the clinical signs of this parasite will not be discussed in detail here since numerous published resources are available for reference (18).
There is evidence of cryptic diversity among what was thought to be D. repens circulating in dogs (10), causing human cases in south India and Hong Kong (10, 15, 187–189). For example, molecularly, D. repens microfilariae are similar to those of a newly recognized Dirofilaria sp. (“hongkongensis” genotype), with the 18s molecular gene target unable to differentiate the two species, thus appropriate molecular targets should be emphasized (188). Morphological descriptions of the adult worms and microfilariae are lacking for this putative species.
It is not clear if D. repens cross-reacts with the global array of heartworm antigen tests, but it is known to cross-react with a commercial heartworm antigen test following immune complex dissociation (ICD) via heat treatment (107). Differently, A. reconditum and O. lupi do not seem to cross-react with a few commercial heartworm antigen-detection kits that have been tested following this ICD protocol (24, 27, 107).
5. Morphological identification of microfilariae in blood and skin
Most microfilariae testing is focused on detecting the canine heartworm and differentiating it from other globally recognized species with blood microfilariae (Table 2 and Supplementary Table 1). The modified Knott's method (141) is the most commonly used method for morphometric differentiation of unsheathed blood microfilariae based on morphological characteristics (11). While the morphological characteristics of a modified Knott's preparation are preserved over time (11, 190), the time from collection to processing can apparently cause morphology differences within a sample from the same dog (135).
The use of thin and thick blood smears stained by Giemsa or hematoxylin, an alternative differentiation method, is often used in regions where sheathed microfilariae of Brugia species are common together with unsheathed microfilariae. The length of the cephalic space and innenkorper are considered major differentiating morphological features for Brugia species, which also have two distinct and separate nuclei in the tail differentiating this genus from Wuchereria bancrofti in humans (161, 191, 192). In fixed blood smears, D. immitis can be clearly distinguished from D. repens D. striata, and “D. striata-like” which have been rarely reported in dogs from Florida. These microfilariae can easily be distinguished from D. immitis due to the presence of two nuclei distinctly separate from the nuclear column in cephalic space in these latter species (118, 193). Variation in reported length measurements for morphological features observed in blood smears can be caused by the numerous different solvents used for fixing dried blood smears, which causes different degrees of shrinkage of microfilariae. This variability of measurements in the literature for thin and thick smears is generally addressed by comparing percentages between reports, which is possible considering the length of each morphological feature from the anterior end divided by the microfilariae length (194). However, the lack of reported range measurements for each species and the use of different fixatives in the literature potentially complicate an accurate differentiation between species.
Unfortunately, a broad range of morphological measurements exists for the modified Knott's and stained thin or thick blood smears, and measurements from these different methods are often lumped together in reference tables causing some confusion. Clarification of the literature should be considered using results from multiple diagnostic tools (Knotts, Giemsa thin blood and thick blood smears, acid phosphatase, and PCR of multiple gene targets), in a similar fashion recently used to demonstrate B. malayi is indeed infecting dogs in southern India and possibly impacting eradication efforts (14). We have attempted to clarify the reported morphological measurements between the species for modified Knott's with the inclusion of saline sedimentation in Table 2. For techniques using blood smears, consistent reporting of morphological characters or the lack of details on fixation and staining methods in the literature that hindered our efforts, further clarification identifying microfilariae in blood smears may be necessary. Available morphological information using this technique, for which methodology was discernible or where no other data sources were available, is summarized in Supplementary Table 1.
Acid phosphatase staining is a particularly useful tool for differentiating canine microfilariae (Figures 4A–C) (192), although laborious and time-consuming, thus generally restricted to diagnostic labs (11). Although the use of several commercially available kits has been described in the literature, these methods do not seem to work for all species of microfilariae (11, 195). The originally described method produces the most consistent results and is best used on freshly collected samples (142); however, the inclusion of a controlled drug in the veronal buffer hinders its accessible use. Reagents prepared for this technique are recommended to be used fresh but can also be combined as described (142), rapidly aliquoted, and flash frozen for longer shelf life and convenience (131). Using this staining method, D. immitis shows somatic staining at the excretory vesical and anal pore; A. reconditum shows diffuse staining throughout concentrated in the caudal half; A. dracunculoides demonstrates staining at the cephalic vesicle, excretory pore, inner body, and anal pore; and D. repens apparently has two different somatic staining patterns reported in the literature, specifically at the inner body and anal pore or the anal pore only (11, 13, 20, 142). Whether these somatic staining patterns are different between D. repens and the Dirofilaria sp., “hongkongensis genotype” is unknown but should be confirmed along with specific molecular targets along with other identified genotypes possibly distinct from D. repens. Somatic staining patterns for Brugia spp. infecting dogs have been previously discussed elsewhere (13, 14, 159).
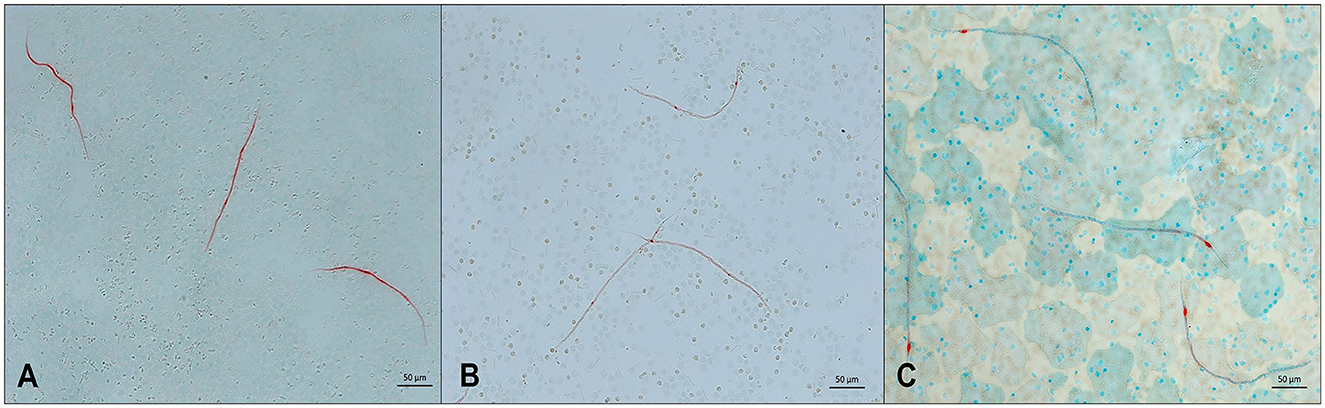
Figure 4. Microfilariae detected in blood samples stained by acid phosphatase (AP) histochemical method. (A) Acantocheilonema reconditum: AP activity through the entire body (red); scale bar: 50 μm. (B) Dirofilaria immitis: AP activity in excretory and anal pores (red); scale bar: 50 μm. (C) Dirofilaria repens: AP activity in anal pores (red); scale bar: 50 μm.
6. Molecular differentiation of microfilariae in blood and skin
Due to the limitations presented by microscopy-based techniques, molecular tools have been extensively used for the diagnosis of filarioid in dogs. These DNA-based techniques provide important genetic data useful for the differentiation and detection of mixed infections (55). Initially employed for blood microfilariae (e.g., Dirofilaria sp. and Acanthocheilonema sp.), these methods allowed specific diagnosis in vertebrate and invertebrate hosts (196, 197) or differentiation between Brugia spp. (160, 198). However, in endemic regions, where more than one filarioid species is detected, the differentiation of these parasites is pivotal (199, 200).
It is indisputable the advances of DNA-based tests over the last years, but in general, these methods were able to differentiate a few filarioid species present in the blood stream (197). With the retrieval of subcutaneous species (Cercopithifilaria sp. and O. lupi) infecting dogs, the molecular methods focusing only on blood parasites (30) became outdated in regions where subcutaneous species were also detected (31, 73). Then, molecular techniques capable of detecting three or more filarioids simultaneously were developed. For example, in 2012, a multiplex PCR for the simultaneous detection and differentiation of Dirofilaria sp., A. reconditum, and Cercopithifilaria sp. was proposed (201). Afterward, a HRM real-time qPCR was successfully developed for the quantification of D. immitis-microfilariae and distinguished filarioid nematodes that were not detected by the other employed assays (55), being a good tool for the detection of samples with low microfilarial concentrations likely missed by the modified Knott's test (145). More recently, a multiplex real-time PCR allowed specific detection of bloodstream filarioid nematodes, in which the authors suggest that this technique can detect occult infection by Dirofilaria spp. when interpreted together with results from blood smears and heartworm antigen testing (148). Additional studies are needed to support claims of molecular detection of occult infection and evaluated against filtration microfilariae testing, necropsy, and recovery of nematodes from tissues (24). Apart from filarial parasites, this tool also targets Wolbachia genotypes, an application important for diagnosis and also follow-up on animals undergoing treatment (148, 202).
The rise in cases of O. lupi infecting dogs and humans in Europe and North America has stimulated the development of novel molecular diagnostic protocols aiming for higher sensitivity and specificity. It is important to note that microfilariae of this species are skin-dwelling (100), and these are likely to go undetected or underdiagnosed as the collection and screening of skin samples are less common than those of blood samples. The development of the molecular diagnosis of cutaneous filarioid species in dogs has expanded given the increased interest in these parasites. In most cases, it is based on the conventional PCR approach followed by sequencing, which is labor- and time-intensive and unpractical for large-scale epidemiological studies. Then, a species-specific qPCR assay developed represents an important resource for the diagnosis of O. lupi and for the detection and quantification of the low number of microfilariae of this nematode (85).
Recently, the mitogenome of O. lupi was provided through the analysis of next-generation sequencing and molecular phylogenetic placement, which revealed that it is composed of 13,766 bp and contains 36 genes (203). These data are useful for studies involving different aspects of this filarioid, including the discovery of new markers important for the development of diagnostic tools. Stimulated by this finding of the mitogenome, a real-time PCR was developed to determine host-to-parasite DNA ratios (Onchocerca lupi vs. Canis lupus), which are essential for knowledge about the whole genome sequence (204), but can also be very important for future diagnostic purposes.
Despite the advances in molecular diagnostics, these tools have only recently been applied for the screening of filarioid species in pet and shelter populations in the US, sometimes in combination with morphological identifications (20, 23–27, 32). Additionally, efforts should be made to design molecular assays that can overcome a dominant species bias, as seen for D. immitis/D. repens coinfections (147) and D. immitis/A. reconditum coinfections (24, 146).
7. Reports of other microfilariae of unknown identity
Increased surveillance in pet and shelter canine populations may occasionally result in the detection of microfilariae that do not fit morphologically with known species (Table 3). In the Americas, microfilariae of diverse morphometry and unknown identity have been sporadically detected in canine skin, tissues, lesions, and blood but are lacking further investigation and description. For example, in Florida, Dirofilaria striata, of wild felids, or D. striata-like microfilariae was reported in 3 dogs (151, 206) during modified Knott's testing, and larger microfilariae similar in size to D. lutrae, which infects otters (199), was reported in 1 dog with only adults of D. immitis at necropsy (24). Only dermal microfilariae were recovered in 10 dogs from Arizona, with dermal lesions (e.g., papules, alopecia, scarring, erythema, ulceration, and crusting) attributed to Acanthocheilonema species based on the recovery of 1 adult female, which emerged from an excised nodule (192). Also, a single dermal microfilaria from the skin of a dog in Oklahoma, shorter than that of C. bainae, were reported in a recent study but could not be molecularly characterized (40). Additionally, in Brazil, microfilariae measuring ~168 μm in length were recovered from skin snips and detected through histopathological examination in corneal biopsies of 8 dogs with ocular keratitis (208).
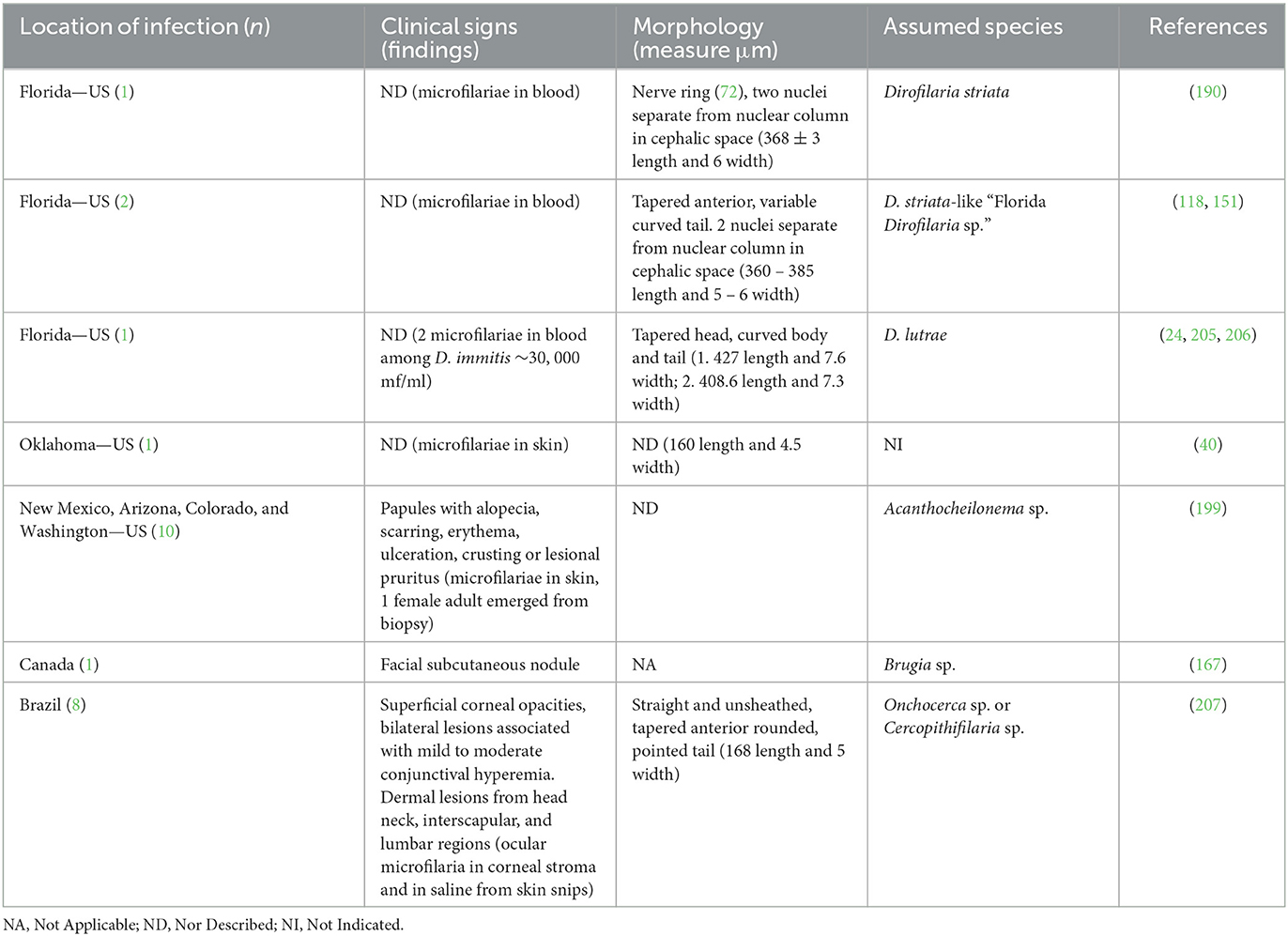
Table 3. Microfilariae that do not fit morphologically with known species of occurrence in the Americas.
Apart from dogs, large microfilariae of unknown morphological and molecular identity were observed in a cat from Florida and in a bobcat from Oklahoma. In both cases, gene sequences differed from those published for D. striata (23, 25). Modified Knott's testing of blood from Florida panthers reported diverse morphology and a wide range of lengths (273–370 μm), many smaller than expected for D. striata (195). Although those microfilariae were found in felids, these examples should emphasize our lack of understanding of filarioid nematodes in domestic and wild animals in the US. When possible, microfilariae of unknown identity or subcutaneous nodules should be investigated with multiple techniques, which could possibly aid in the identification and, ideally, recovery of adult specimens on the occasion of necroscopic examination. When a dog or cat is microfilariae-positive and heartworm antigen-negative, it is strongly encouraged that the sample should be examined in a reference diagnostic laboratory (20), ideally with the capability for molecularly characterizing the sample.
8. Zoonosis and proactive surveillance for filarioids in domestic and wild animals
Nematodes of the genera Brugia and Dirofilaria present in mammals pose the greatest zoonotic risk due to their ability to infect and adapt to a wide range of hosts (45, 209). All species of canine filarioids should be generally suspected of zoonotic potential due to the close association of canids with human communities (8). To date, the seven species that have been implicated in known human cases are A. reconditum, B. pahangi, B. ceylonensis, D. immitis, D. repens, Dirofilaria sp. (hongkongensis genotype), and O. lupi (10, 34, 45, 203, 210, 211). An increased risk of zoonosis, or anthropozoonosis for B. malayi, exists in areas where competent vectors also feed on both humans and infected canid/felid definitive hosts (212).
Surveillance activities, while specifically of veterinary and scientific interest, should also serve a public health interest, integrating into a one-health approach. An example of this approach would be to work with the state's health departments or existing mosquito control districts' arbovirus surveillance efforts to retest nucleic acid from mosquito pools and for the possible use of biting midges and black fly bycatch, while prioritizing geographic areas near identified microfilaremic animals. Alternatively, banked vector lysates from blue tongue, or other animal disease surveillance could also be repurposed. Additionally, population control activities and conservation activities by the state's wildlife departments may offer collaboration opportunities for use of banked samples or collection of carcasses, for skin, blood, tissue, or nematode recovery from wild canids, felids, and other mammals. Trap-neuter-release efforts for feral and free-roaming cats across the US provide an opportunity for blood collection and result in discarded ear tips, indicating vaccination, which could be opportunistically saved and used to screen for microfilariae of filarioids and other vector-borne diseases in cats. Unfortunately, impactful surveillance for these nematodes in arthropod vectors, wildlife, or domesticated hosts is often motivated in reaction to human cases.
Humans are considered “dead end” hosts for D. immitis and for D. repens, which are causative agents of cardiopulmonary and subcutaneous nodules, respectively (18). This established perspective may require additional examination with patent infections of Dirofilaria spp. detected molecularly in ~1% of asymptomatic healthy individuals in Italy (3/397 and 1/397 for D. immitis and D. repens, respectively) (175), and serologically in 6.1% (41/668 for D. immitis and Wolbachia surface protein antibodies) of patients in Portugal (213). Similar investigative studies could be performed on opportunistic blood samples from human populations from across the US, and positive results may spur a public health interest in control efforts targeting these parasites in dog and cat populations (i.e., heartworm prophylaxis). However, subcutaneous dirofilariosis caused by D. repens is the fastest growing zoonosis in Europe (214) in North America subcutaneous dirofilariosis caused by species associated with wildlife has so far only been suggested for D. tenuis, D. ursi, and D. striata (36, 209, 215–218).
The absence of a confirmed vector for O. lupi poses an additional impairment for the control and prevention of this infection in animal and human hosts. However, it is essential the implementation of surveillance activities due to the rise of human cases recently observed in the US (39). After the first case reported in Arizona (36), other states such as New Mexico and Texas diagnosed human patients, totaling 7 notifications in this country, most of them affecting children (6/7) (37–39). Along with the data on natural infection in dogs, the states of New Mexico and Arizona show up as important endemic areas, and the surveillance in these areas is pivotal to better understand the real epidemiological situation of this filarial infection and consequently prevent future cases. The detection of microfilariae of O. lupi is achieved through the examination of skin snips (100) that are less frequently collected than blood samples used for the detection of other filarioid species. Even if not collected routinely, skin fragments are usually obtained by vet dermatologists for diagnostic purposes. These samples might be extremely useful for the surveillance of this infection, especially for the detection of asymptomatic dogs. Currently, the most important source of data about O. lupi infection in endemic regions is veterinary ophthalmologists, since the ocular manifestations are the most common clinical signs (71, 73). To better understand the epidemiology of O. lupi in endemic regions, dogs and other potential hosts should be enrolled and screened despite the presence of clinical ocular disease.
In addition to all the surveillance actions herein reported, the control of filarial infection in dogs is pivotal to preventing human cases. For instance, chemoprophylaxis through the use of lactones macrocyclic (e.g., ivermectin, selamectin, milbemycin oxime, and moxidectin) is recommended in areas of high risk of infection by Dirofilaria spp. due to the microfilaricidal action of these substances (18). Also, the prevention of vector-borne infestations by ticks, fleas, and mosquitoes through the use of repellent and ectoparasiticides compounds has been stimulated everywhere to prevent infection by filarioids and other vector-borne pathogens (26).
9. Gaps in knowledge and future perspectives
The implementation of active surveillance for filarioid nematode infections and co-infections in dogs is necessary. Ideally, this effort should be geographically broad and utilize diagnostic methods that may detect endemic, emerging, and exotic species. Integrated morphological, robust molecular (e.g., multiple genes, mitogenomes, or whole genomes), and histochemical characterization of filarioid nematodes infecting wild animals and their vectors is warranted to better understand filarioid (cryptic) biodiversity, their host range, and geographic distribution (219). Geographic areas where microfilaremic imported animals are identified should be prioritized for surveillance in dogs, cats, and potential vectors. Previously uncharacterized species may occasionally infect dogs, such as the recent Brugia case in Canada, and also cats. These (un)known nematodes may represent a risk for both companion animals and humans, as a great proportion of zoonotic diseases come from wildlife reservoir species (220, 221).
Molecular characterization of all known Dirofilaria species in the New World is still lacking, despite recently added limited data for D. lutrae (207), D. ursi (221), and D. striata (222). For instance, recent studies focused on North American Onchocerca species have revealed various cryptic genetic lineages (223–227). Addressing this need may be important for identifying filarioid species in the future domestic animal and zoonotic cases (207, 228–234) or revisiting archival materials in museum collections that contain formalin-fixed tissues attributed to filarioid nematodes but that have not been unequivocally assigned to species. Serological surveillance for determining exposure or molecular screening for active infection by filarioids in human populations has not yet been examined in the US and represents a major knowledge gap (143).
In addition, some biological aspects of O. lupi and C. bainae life cycle and effective chemoprophylaxis and treatment and reliable diagnostic tools for the detection of infections and co-infections caused by filarioid nematodes occurring in sympatry may be considered important gaps in the knowledge of subcutaneous filarioid nematodes infecting dogs in the US. The research of new molecular markers and techniques, the training of parasitologists for classical microscopical examination, and a broad active surveillance program may improve parasite detection and inform veterinary and public health authorities for implementing intervention strategies for mitigating range expansion and the establishment of endemic and exotic filarioid species in animals and humans. With the exception of D. immitis, all of the filarioid nematode species discussed herein may be considered emerging, neglected, or underdiagnosed.
Author contributions
All authors listed have made a substantial, direct, and intellectual contribution to the work and approved it for publication.
Acknowledgments
The authors would like to thank Dr. Heather Walden for providing C. bainae microfilariae photomicrograph, and Drs. Heather Walden and Lindsay Starkey for providing a photomicrograph of D. repens microfilariae pictures.
Conflict of interest
The authors declare that the research was conducted in the absence of any commercial or financial relationships that could be construed as a potential conflict of interest.
Publisher's note
All claims expressed in this article are solely those of the authors and do not necessarily represent those of their affiliated organizations, or those of the publisher, the editors and the reviewers. Any product that may be evaluated in this article, or claim that may be made by its manufacturer, is not guaranteed or endorsed by the publisher.
Supplementary material
The Supplementary Material for this article can be found online at: https://www.frontiersin.org/articles/10.3389/fvets.2023.1128611/full#supplementary-material
References
1. Anderson RC. Nematode Parasites of Vertebrates. Their Development and Transmission. Wallingford: CABI Publishing (2000). 672 pp.
2. Lefoulon E, Bain O, Bourret J, Junker K, Guerrero R, Cañizales I, et al. Shaking the tree: Multi-locus sequence typing usurps current onchocercid (filarial nematode) phylogeny. PLoS Negl Trop Dis. (2015) 9:e0004233. doi: 10.1371/journal.pntd.0004233
3. Orihel TC, Eberhard ML. Zoonotic filariasis. Clin Microbiol Rev. (1998) 11:366–81. doi: 10.1128/CMR.11.2.366
4. Otranto D, Brianti E, Dantas-Torres F, Miró G, Latrofa MS, Mutafchiev Y, et al. Species diversity of dermal microfilariae of the genus Cercopithifilaria infesting dogs in the Mediterranean region. Parasitology. (2013) 140:99–108. doi: 10.1017/S0031182012001357
5. Bain O. Le genre Onchocerca: hypothèses sur son évolution et clé dichotomique des espèces [The genus Onchocherca: hypothesis on its evolution and a key to the species (author's transl)]. Ann Parasitol Hum Comp. (1981) 56:503–26. doi: 10.1051/parasite/1981565503
6. Lefoulon E, Giannelli A, Makepeace BL, Mutafchiev Y, Townson S, Uni S, et al. Whence River blindness? The domestication of mammals and host-parasite co-evolution in the nematode genus. Onchocerca Int J Parasitol. (2017) 47:457–70. doi: 10.1016/j.ijpara.2016.12.009
7. Foley H. Microfilaria of dogs in the South Oran district. Ann Inst Pasteur Paris. (1921) 35:212–7.
8. Megat Abd Rani PA, Irwin PJ, Gatne M, Coleman GT, McInnes LM, Traub RJ, et al. survey of canine filarial diseases of veterinary and public health significance in India. Parasit Vectors. (2010) 3:30. doi: 10.1186/1756-3305-3-30
9. Rani PA, Coleman GT, Irwin PJ, Traub RJ. Hippobosca longipennis-a potential intermediate host of a species of Acanthocheilonema in dogs in northern India. Parasit Vectors. (2011) 4:143. doi: 10.1186/1756-3305-4-143
10. To KK, Wong SS, Poon RW, Trendell-Smith NJ, Ngan AH, Lam JW, et al. A novel Dirofilaria species causing human and canine infections in Hong Kong. J Clin Microbiol. (2012) 50:3534–41. doi: 10.1128/JCM.01590-12
11. Magnis J, Lorentz S, Guardone L, Grimm F, Magi M, Naucke TJ, et al. Morphometric analyses of canine blood microfilariae isolated by the Knott's test enables Dirofilaria immitis and D. repens species-specific and Acanthocheilonema (syn Dipetalonema) genus-specific diagnosis. Parasit Vectors. (2013) 6:48. doi: 10.1186/1756-3305-6-48
12. Cortes HC, Cardoso L, Giannelli A, Latrofa MS, Dantas-Torres F, Otranto D. Diversity of Cercopithifilaria species in dogs from Portugal. Parasit Vectors. (2014) 7:261. doi: 10.1186/1756-3305-7-261
13. Ravindran R, Varghese S, Nair SN, Balan VM, Lakshmanan B, Ashruf RM, et al. Canine filarial infections in a human Brugia malayi endemic area of India. Biomed Res Int. (2014) 2014:630160. doi: 10.1155/2014/630160
14. Chirayath D, Alex PC, Pillai UN, George S, Ajithkumar S, Panicker VP. Identification of Brugia malayi in dogs in Kerala, India. Trop Biomed. (2017) 34:804–14.
15. Gowrishankar S, Aravind M, Sastya S, Latha BR, Azhahianambi P, Vairamuthu S, et al. Dirofilaria hongkongensis—A first report of potential zoonotic dirofilariosis infection in dogs from Tamil Nadu. Vet Parasitol Reg Stud Rep. (2019) 2019:100326. doi: 10.1016/j.vprsr.2019.100326
16. Tiffin HS, Rajotte EG, Sakamoto JM, Machtinger ET. Tick control in a connected world: Challenges, solutions, and public policy from a United States border perspective. Trop Med Infect Dis. (2022) 7:388. doi: 10.3390/tropicalmed7110388
17. Centers for Disease Control and Prevention. Guidance regarding agency interpretation of “rabies-free” as it relates to the importation of dogs into the United States. Federal Register. (2019) 84:724–30.
18. McCall JW, Genchi C, Kramer LH, Guerrero J, Venco L. Heartworm disease in animals and humans. Adv Parasitol. (2008) 66:193–285. doi: 10.1016/S0065-308X(08)00204-2
19. Atkins CE. Comparison of results of three commercial heartworm antigen test kits in dogs with low heartworm burdens. J Am Vet Med Assoc. (2003) 222:1221–3. doi: 10.2460/javma.2003.222.1221
20. Little S, Saleh M, Wohltjen M, Nagamori Y. Prime detection of Dirofilaria immitis: Understanding the influence of blocked antigen on heartworm test performance. Parasit Vectors. (2018) 11:186. doi: 10.1186/s13071-018-2736-5
21. Yabsley MJ, Dresden-Osborne C, Pirkle EA, Kirven JM, Noblet GP. Filarial worm infections in shelter dogs and cats from northwestern South Carolina, USA. Comp Parasitol. (2004) 71:154–7. doi: 10.1654/4125
22. Donnett U, Hubbard K, Woodruff K, Varela-Stokes A. Prevalence of canine heartworm infection in Mississippi animal shelters. Vet Parasitol. (2018) 259:68–73. doi: 10.1016/j.vetpar.2018.07.007
23. Hays KM, Rodriguez JY, Little SE, Litster AL, Mwacalimba KK, Sundstrom KD, et al. Heartworm prevalence in dogs versus cats: Multiple diagnostic modalities provide new insights. Vet Parasitol. (2020) 277S:100027. doi: 10.1016/j.vpoa.2020.100027
24. Gruntmeir JM, Thompson NM, Long MT, Blagburn BL, Walden HDS. Detection of heartworm antigen without cross-reactivity to helminths and protozoa following heat treatment of canine serum. Parasit Vectors. (2021) 14:71. doi: 10.1186/s13071-020-04573-6
25. Lane JN, Litster A, Little SE, Rodriguez JY, Mwacalimba KK, Sundstrom KD, et al. Optimizing heartworm diagnosis in dogs using multiple test combinations. Parasit Vectors. (2021) 14:224. doi: 10.1186/s13071-021-04715-4
26. Smith R, Murillo DFB, Chenoweth K, Barua S, Kelly PJ, Starkey L, et al. Nationwide molecular survey of Dirofilaria immitis and Dirofilaria repens in companion dogs and cats, United States of America. Parasit Vectors. (2022) 15:367. doi: 10.1186/s13071-022-05459-5
27. Negron V, Saleh MN, Sobotyk C, Luksovsky JL, Harvey TV, Verocai GG. Probe-based qPCR as an alternative to modified Knott's test when screening dogs for heartworm (Dirofilaria immitis) infection in combination with antigen detection tests. Parasit Vectors. (2022) 15:306. doi: 10.1186/s13071-022-05372-x
28. Courtney CH, Zeng QY. The structure of heartworm populations in dogs and cats in Florida. Proc Heartworm Symp. (1989) 89:1–6.
29. Patton S, Faulkner CT. Prevalence of Dirofilaria immitis and Dipetalonema reconditum infection in dogs: 805 cases (1980-1989). J Am Vet Med Assoc. (1992) 200:1533–4.
30. Rishniw M, Barr SC, Simpson KW, Frongillo MF, Franz M, Dominguez Alpizar JL. Discrimination between six species of canine microfilariae by a single polymerase chain reaction. Vet Parasitol. (2006) 135:303–14. doi: 10.1016/j.vetpar.2005.10.013
31. de Oliveira CS, Savadelis MD, McLean NJ, Verocai GG. Assessing the potential cross-reactivity using a commercial heartworm ELISA kits of serum from dogs naturally infected with Onchocerca lupi. Vet Parasitol. (2020) 280:109070. doi: 10.1016/j.vetpar.2020.109070
32. Scavo NA, Zecca IB, Sobotyk C, Saleh MN, Lane SK, Olson MF, et al. High prevalence of canine heartworm, Dirofilaria immitis, in pet dogs in south Texas, USA, with evidence of Aedes aegypti mosquitoes contributing to transmission. Parasit Vectors. (2022) 15:407. doi: 10.1186/s13071-022-05514-1
33. Newton WL, Wright WH. The occurrence of a dog filariid other than Dirofilaria immitis in the United States. J Parasitol. (1956) 42:246–58. doi: 10.2307/3274849
34. Labelle AL, Daniels JB, Dix M, Labelle P. Onchocerca lupi causing ocular disease in two cats. Vet Ophthalmol. (2011) 14(Suppl.1):105–10. doi: 10.1111/j.1463-5224.2011.00911.x
35. Boyd M, Santoro D, Craft WF, Ginn PE, Childress AL, Wellehan JFX, et al. Dermatitis caused by autochthonous Cercopithifilaria bainae from a dog in Florida, USA: Clinical, histological and parasitological diagnosis and treatment. Vet Dermatol. (2019) 30:68–e20. doi: 10.1111/vde.12701
36. Eberhard ML, Ostovar GA, Chundu K, Hobohm D, Feiz-Erfan I, Mathison BA, et al. Zoonotic Onchocerca lupi infection in a 22-month-old child in Arizona: First report in the United States and a review of the literature. Am J Trop Med Hyg. (2013) 88:601–5. doi: 10.4269/ajtmh.12-0733
37. Dudley RWR, Smith C, Dishop M, Mirsky D, Handler MH, Rao S, et al. Cervical spine mass caused by Onchocerca lupi. Lancet. (2015) 386:1372. doi: 10.1016/S0140-6736(14)62255-8
38. Cantey PT, Weeks J, Edwards M, Rao S, Ostovar GA, Dehority W, et al. The emergence of zoonotic Onchocerca lupi infection in the United States—A case-series. Clin Infect Dis. (2016) 62:778–83. doi: 10.1093/cid/civ983
39. Bowers Wu D, Ko B, Lopez Hernandez G, Botros J, Spader H, Sapp S, et al. Neuroinvasive Onchocerca lupi infection in a ten-year-old girl. Case Rep Infect Dis. (2022) 2022:9773058. doi: 10.1155/2022/9773058
40. Lineberry MW, Sundstrom KD, Little SE, Stayton EM, Allen KE. Detection of Cercopithifilaria bainae infection in shelter dogs and ticks in Oklahoma, USA. Parasit Vectors. (2020) 13:216. doi: 10.1186/s13071-020-04089-z
41. Lineberry MW, Sundstrom KD, Little SE, Allen KE. Molecular detection of Cercopithifilaria bainae in brown dog ticks collected from dogs across the United States. Vet Parasitol. (2021) 299:109583. doi: 10.1016/j.vetpar.2021.109583
42. Otranto D, Brianti E, Latrofa MS, Annoscia G, Weigl S, Lia RP, et al. On a Cercopithifilaria sp. transmitted by Rhipicephalus sanguineus: A neglected, but widespread filarioid of dogs. Parasit Vectors. (2012) 5:1. doi: 10.1186/1756-3305-5-1
43. Ramos RA, Giannelli A, Lia RP, Brianti E, Tarallo VD, Breitshwerdt EB, et al. Incidence of Cercopithifilaria bainae in dogs and probability of co-infection with other tick-borne pathogens. PLoS ONE. (2014) 9:e88198. doi: 10.1371/journal.pone.0088198
44. Mutafchiev Y, Dantas-Torres F, Giannelli A, Abramo F, Papadopoulos E, Cardoso L, et al. Redescription of Onchocerca lupi (Spirurida: Onchocercidae) with histopathological observations. Parasit Vectors. (2013) 6:309. doi: 10.1186/1756-3305-6-309
45. Eberhard ML. Dipetalonema (Cercopithifilaria) kenyensis subgen. et sp n (Nematoda: Filarioidea) from African baboons, Papio anubis. J Parasitol. (1980) 66:551–4. doi: 10.2307/3280762
46. Otranto D, Brianti E, Dantas-Torres F, Weigl S, Latrofa MS, Gaglio G, et al. Morphological and molecular data on the dermal microfilariae of a species of Cercopithifilaria from a dog in Sicily. Vet Parasitol. (2011) 182:221–9. doi: 10.1016/j.vetpar.2011.05.043
47. Bezerra-Santos MA, de Macedo LO, Nguyen VL, Manoj RR, Laidoudi Y, Latrofa MS, et al. Cercopithifilaria spp. in ticks of companion animals from Asia: New putative hosts and vectors. Ticks Tick Borne Dis. (2022) 13:101957. doi: 10.1016/j.ttbdis.2022.101957
48. Noè G. Contribuzioni alla sistematica e alla anatomia del genere Filaria. La Filaria grassii. Roma: Istituto di Anatomia Comparata della Regia Università di Roma (1907). p. 236–52.
49. Bain O, Aeschlimann A, Chatelanat P. Présence, chez des tiques de la région de Genève, de larves infestantes qui pourraient se rapporter à la filaire de chien Dipetalonema grassii [Presence, in ticks from the Geneva region, of infective larvae which may be related to the dog filaria Dipetalonema grassii]. Ann Parasitol Hum Comp. (1982) 57:643–6. doi: 10.1051/parasite/1982576643
50. Gouvea de Almeida GL, Vicente J. Cercopithifilaria bainae sp. n. parasita de Canis familiaris (L.) (Nematoda, Filarioidea). Atas da Sociedade de Biologia do Rio de Janeiro. (1985) 1985:24.
51. Otranto D, Varcasia A, Solinas C, Scala A, Brianti E, Dantas-Torres F, et al. Redescription of Cercopithifilaria bainae Almeida & Vicente, 1984 (Spirurida, Onchocercidae) from a dog in Sardinia, Italy. Parasit Vectors. (2013) 6:132. doi: 10.1186/1756-3305-6-132
52. Brianti E, Gaglio G, Napoli E, Giannetto S, Dantas-Torres F, Bain O, et al. New insights into the ecology and biology of Acanthocheilonema reconditum (Grassi, 1889) causing canine subcutaneous filariosis. Parasitology. (2012) 139:530–6. doi: 10.1017/S0031182011002198
53. Ramos RA, Giannelli A, Brianti E, Annoscia G, Cantacessi C, Dantas-Torres F, et al. Tick vectors of Cercopithifilaria bainae in dogs: Rhipicephalus sanguineus sensu lato versus Ixodes ricinus. Parasitol Res. (2013) 112:3013–7. doi: 10.1007/s00436-013-3474-4
54. Otranto D, Brianti E, Abramo F, Gaglio G, Napoli E, Latrofa MS, et al. Cutaneous distribution and localization of Cercopithifilaria sp. microfilariae in dogs. Vet Parasitol. (2012) 190:143–50. doi: 10.1016/j.vetpar.2012.05.016
55. Rojas A, Rojas D, Montenegro VM, Baneth G. Detection of Dirofilaria immitis and other arthropod-borne filarioids by an HRM real-time qPCR, blood-concentrating techniques and a serological assay in dogs from Costa Rica. Parasit Vectors. (2015) 8:170. doi: 10.1186/s13071-015-0783-8
56. Tutija JF, Soares RL, Echeverria JT, Souza MA, Silva TO, Ramos RA, et al. Microfilaremia by Cercopithifilaria bainae in a dog from the central western region of Brazil: Case report. Arq Bras Med Vet Zoo. (2020) 72:312–6. doi: 10.1590/1678-4162-11177
57. Laidoudi Y, Marie JL, Tahir D, Watier-Grillot S, Mediannikov O, Davoust B. Detection of canine vector-borne filariasis and their Wolbachia endosymbionts in French Guiana. Microorganisms. (2020) 8:770. doi: 10.3390/microorganisms8050770
58. Dantas-Torres F, Latrofa MS, Annoscia G, Giannelli A, Parisi A, Otranto D. Morphological and genetic diversity of Rhipicephalus sanguineus sensu lato from the New and Old Worlds. Parasit Vectors. (2013) 6:1–7. doi: 10.1186/1756-3305-6-213
59. Šlapeta J, Chandra S, Halliday B. The “tropical lineage” of the brown dog tick Rhipicephalus sanguineus sensu lato identified as Rhipicephalus linnaei. Int J Parasitol. (2021) 51:431–6. doi: 10.1016/j.ijpara.2021.02.001
60. Jones EO, Gruntmeir JM, Hamer SA, Little SE. Temperate and tropical lineages of brown dog ticks in North America. Vet Parasitol Reg Stud Reports. (2017) 7:58–61. doi: 10.1016/j.vprsr.2017.01.002
61. Latrofa MS, Dantas-Torres F, Giannelli A, Otranto D. Molecular detection of tick-borne pathogens in Rhipicephalus sanguineus group ticks. Ticks Tick Borne Dis. (2014) 5:943–6. doi: 10.1016/j.ttbdis.2014.07.014
62. Eberhard ML, Ortega Y, Dial S, Schiller CA, Sears AW, Greiner E. Ocular Onchocerca infections in two dogs in western United States. Vet Parasitol. (2000) 90:333–8. doi: 10.1016/S0304-4017(00)00252-1
63. Wallitsch K, Jaffey JA, Ferguson S, Verocai GG, Sobotyk C, van Eerde E, et al. Extensive aberrant migration of Onchocerca lupi in a dog. Top Companion Anim Med. (2022) 49:100666. doi: 10.1016/j.tcam.2022.100666
64. Orihel TC, Ash LR, Holshuh HJ, Santenelli S. Onchocerciasis in a California dog. Am J Trop Med Hyg. (1991) 44:513–7. doi: 10.4269/ajtmh.1991.44.513
65. Gardiner CH, Dick EJ Jr, Meininger AC, Lozano-Alarcón F, Jackson P. Onchocerciasis in two dogs. J Am Vet Med Assoc. (1993) 203:828–30.
66. Zarfoss MK, Dubielzig RR, Eberhard ML, Schmidt KS. Canine ocular onchocerciasis in the United States: Two new cases and a review of the literature. Vet Ophthalmol. (2005) 8:51–7. doi: 10.1111/j.1463-5224.2005.00348.x
67. Labelle AL, Maddox CW, Daniels JB, Lanka S, Eggett TE, Dubielzig RR, et al. Canine ocular onchocercosis in the United States is associated with Onchocerca lupi. Vet Parasitol. (2013) 193:297–301. doi: 10.1016/j.vetpar.2012.12.002
68. Hassan HK, Bolcen S, Kubofcik J, Nutman TB, Eberhard ML, Middleton K, et al. Isolation of Onchocerca lupi in dogs and black flies, California, USA. Emerg Infect Dis. (2015) 21:789–96. doi: 10.3201/eid2105.142011
69. Otranto D, Giannelli A, Latrofa MS, Dantas-Torres F, Trumble NS, Chavkin M, et al. Canine infections with Onchocerca lupi nematodes, United States, 2011-2014. Emerg Infect Dis. (2015) 21:868–71. doi: 10.3201/eid2105.141812
70. Otranto D, Giannelli A, Scotty Trumble N, Chavkin M, Kennard G, Latrofa MS, et al. Clinical case presentation and a review of the literature of canine onchocercosis by Onchocerca lupi in the United States. Parasit Vectors. (2015) 8:89. doi: 10.1186/s13071-015-0699-3
71. McLean NJ, Newkirk K, Adema CM. Canine ocular onchocerciasis: A retrospective review of the diagnosis, treatment, and outcome of 16 cases in New Mexico (2011-2015). Vet Ophthalmol. (2017) 20:349–56. doi: 10.1111/vop.12433
72. Edelmann ML, Jager M, Espinheira F, Ledbetter EC. In vivo confocal microscopy for detection of subconjunctival Onchocerca lupi infection in a dog. Vet Ophthalmol. (2018) 21:632–7. doi: 10.1111/vop.12547
73. Verocai GG, Sobotyk C, Lamison A, Borst MM, Edwards EE. Autochthonous, zoonotic Onchocerca lupi in a South Texas dog, United States. Parasit Vectors. (2021) 14:203. doi: 10.1186/s13071-021-04707-4
74. Gabrielli S, Giannelli A, Brianti E, Dantas-Torres F, Bufalini M, Fraulo M, et al. Chronic polyarthritis associated to Cercopithifilaria bainae infection in a dog. Vet Parasitol. (2014) 205:401–4. doi: 10.1016/j.vetpar.2014.06.027
75. Soares RL, Parolin LB, Mateus NLF, de Figueiredo GRD, Rodrigues VR, de Oliveira GG, et al. Giant cutaneous cyst in a dog infected by Cercopithifilaria bainae. Vet Parasitol Reg Stud Reports. (2020) 20:100401. doi: 10.1016/j.vprsr.2020.100401
76. Vuong PN, Spratt D, Wanji S, Aimard L, Bain O. Onchocerca-like lesions induced by the filarioid nematode Cercopithifilaria johnstoni, in its natural hosts and in the laboratory rat. Ann Parasitol Hum Comp. (1993) 68:176–81. doi: 10.1051/parasite/1993684176
77. Otranto D, Dantas-Torres F, Giannelli A, Latrofa MS, Papadopoulos E, Cardoso L, et al. Zoonotic Onchocerca lupi infection in dogs, Greece and Portugal, 2011-2012. Emerg Infect Dis. (2013) 19:2000–3. doi: 10.3201/eid1912.130264
78. Rodonaya TE. A new species of nematode, Onchocerca lupi n. sp, from Canis lupus cubanensis Soobshchenyia. Akad Nuak Gruz Ssr. (1967) 45:715–9.
79. Maia C, Annoscia G, Latrofa MS, Pereira A, Giannelli A, Pedroso L, et al. Onchocerca lupi nematode in cat, Portugal. Emerg Infect Dis. (2015) 21:2252–4. doi: 10.3201/eid2112.150061
80. Tudor P, Iona?cu I, Mateescu CI, Bezerra-Santos MA, Gurău MR, Mateescu RE, et al. Feline ocular onchocercosis by Onchocerca lupi: Phylogenetic insights and implication for veterinary health. Acta Trop. (2023) 237:106723. doi: 10.1016/j.actatropica.2022.106723
81. Roe CC, Yaglom H, Howard A, Urbanz J, Verocai GG, Andrews L, et al. Coyotes as reservoirs for Onchocerca lupi, United States, 2015-2018. Emerg Infect Dis. (2020) 26:2989–93. doi: 10.3201/eid2612.190136
82. Rojas A, Salant H, Yasur-Landau D, Tsarfati H, Baneth G. First report of Onchocerca lupi from Israel and confirmation of two genotypes circulating among canine, feline and human hosts. Parasitology. (2020) 147:1723–7. doi: 10.1017/S0031182020001560
83. Hasanreisoglu M, Halim MS, Latrofa MS, Mendoza-Roldan JA, Ogut B, Yilmaz M, et al. Case report: A human case of Onchocerca lupi mimicking nodular scleritis. Am J Trop Med Hyg. (2021) 105:1782–5. doi: 10.4269/ajtmh.21-0186
84. Adler PH, Currie DC, Wood DM, Idema RM, Zettler LW. The Black Flies (Simuliidae) of North America. New York City, NY: Comstock Pub Associates (2004).
85. Latrofa MS, Annoscia G, Colella V, Cavalera MA, Maia C, Martin C, et al. A real-time PCR tool for the surveillance of zoonotic Onchocerca lupi in dogs, cats and potential vectors. PLoS Negl Trop Dis. (2018) 12:e0006402. doi: 10.1371/journal.pntd.0006402
86. Manoj RRS, Latrofa MS, Cavalera MA, Mendoza-Roldan JA, Maia C, Otranto D. Molecular detection of zoonotic filarioids in Culex spp. from Portugal. Med Vet Entomol. (2021) 35:468–77. doi: 10.1111/mve.12524
87. Gustavsen K, Hopkins A, Sauerbrey M. Onchocerciasis in the Americas: From arrival to (near) elimination. Parasit Vectors. (2011) 4:205. doi: 10.1186/1756-3305-4-205
88. Mellor PS. Studies on Onchocerca cervicalis Railliet and Henry 1910. I. Onchocerca cervicalis in British horses. J Helminthol. (1973) 47:97–110. doi: 10.1017/S0022149X00023774
89. Foil LD, Klei TR, Miller RI, Church GE, Foil CS, French DD, et al. Seasonal changes in density and tissue distribution of Onchocerca cervicalis microfilariae in ponies and related changes in Culicoides variipennis populations in Louisiana. J Parasitol. (1987) 73:320–6. doi: 10.2307/3282085
90. Bain O. Transmission de l'Onchocerque bovine, Onchocerca gutturosa, par Culicoides [Transmission of bovine Onchocerca, O. gutturosa, by Culicoides (author's transl)]. Ann Parasitol Hum Comp. (1979) 54:483–8. doi: 10.1051/parasite/1979544483
91. Higgins JA, Klei TR, Foil LD. Factors influencing the ingestion of Onchocerca cervicalis microfilariae by Culicoides variipennis (Diptera: Ceratopogonidae). J Am Mosq Control Assoc. (1988) 4:242–7.
92. Cambra-Pellejà M, Gandasegui J, Balaña-Fouce R, Muñoz J, Martínez-Valladares M. Zoonotic implications of Onchocerca species on human health. Pathogens. (2020) 9:761. doi: 10.3390/pathogens9090761
93. Phillips RA. Culicoides latreille and Leptoconops skuse biting midges of the southwestern United States with emphasis on the Canyonlands of southeastern Utah (Diptera: Ceratopogonidae). Insecta mundi. (2022) 907:1–214.
94. Shults P, Moran M, Blumenfeld AJ, Vargo EL, Cohnstaedt LW, Eyer PA. Development of microsatellite markers for population genetics of biting midges and a potential tool for species identification of Culicoides sonorensis Wirth & Jones. Parasit Vectors. (2022) 15:69. doi: 10.1186/s13071-022-05189-8
95. Collins RC, Jones RH. Laboratory transmission of Onchocerca cervicalis with Culicoides variipennis. Am J Trop Med Hyg. (1978) 27:46–50. doi: 10.4269/ajtmh.1978.27.46
96. Foil L, Stage D, Klei TR. Assessment of wild-caught Culicoides (Ceratopogonidae) species as natural vectors of Onchocerca cervicalis in Louisiana. Mosq News. (1984) 44:204–6.
97. Egyed Z, Sréter T, Széll Z, Beszteri B, Oravecz O, Márialigeti K, et al. Morphologic and genetic characterization of Onchocerca lupi infecting dogs. Vet Parasitol. (2001) 102:309–19. doi: 10.1016/S0304-4017(01)00541-6
98. Buckley JJ. On Culicoides as a vector of Onchocerca gibsoni (Cleland & Johnston, 1910). J Helminthol. (1938) 16:121–58. doi: 10.1017/S0022149X00018526
99. Brilhante AF, de Albuquerque AL, Rocha ACB, Ayres CFJ, Paiva MHS, de Ávila MM, et al. First report of an Onchocercidae worm infecting Psychodopygus carrerai carrerai sandfly, a putative vector of Leishmania braziliensis in the Amazon. Sci Rep. (2020) 10:15246. doi: 10.1038/s41598-020-72065-9
100. Otranto D, Dantas-Torres F, Giannelli A, Abramo F, Ignjatović Cupina A, Petrić D, et al. Cutaneous distribution and circadian rhythm of Onchocerca lupi microfilariae in dogs. PLoS Negl Trop Dis. (2013) 7:e2585. doi: 10.1371/journal.pntd.0002585
101. Taylor ME, Auten CR, Foster T, Ebbs E, Hofkin BV. Canine ocular onchocerciasis in New Mexico: Risk factors for disease. Vet Ophthalmol. (2021) 24:288–94. doi: 10.1111/vop.12889
102. Green CH, Cosens D. Spectral responses of the tsetse fly, Glossina morsitans morsitans. J Ins Physiol. (1983) 29:795–800. doi: 10.1016/0022-1910(83)90009-4
103. McNeill CA, Pereira RM, Koehler PG, McNeill SA, Baldwin RW. Behavioral responses of nymph and adult Cimex lectularius (Hemiptera: Cimicidae) to colored harborages. J Med Entomol. (2016) 53:760–9. doi: 10.1093/jme/tjw033
104. Széll Z, Erdélyi I, Sréter T, Albert M, Varga I. Canine ocular onchocercosis in Hungary. Vet Parasitol. (2001) 97:243–9. doi: 10.1016/S0304-4017(01)00397-1
105. Otranto D, Dantas-Torres F, Cebeci Z, Yeniad B, Buyukbabani N, Boral OB, et al. Human ocular filariasis: Further evidence on the zoonotic role of Onchocerca lupi. Parasit Vectors. (2012) 5:84. doi: 10.1186/1756-3305-5-84
106. Verocai GG, Conboy G, Lejeune M, Marron F, Hanna P, MacDonald E, et al. Onchocerca lupi nematodes in dogs exported from the United States into Canada. Emerg Infect Dis. (2016) 22:1477–9. doi: 10.3201/eid2208.151918
107. Sobotyk C, Savadelis MD, Verocai GG. Detection and cross-reaction of Dirofilaria repens using a commercial heartworm antigen test kit. Vet Parasitol. (2021) 289:109302. doi: 10.1016/j.vetpar.2020.109302
108. Papaioannou N, Psalla D, Papadopoulos E, Adamama-Moraitou KK, Petanidis T, Rallis T, et al. Obstructive, granulomatous tracheitis caused by Onchocerca sp. in a dog. J Vet Med A Physiol Pathol Clin Med. (2004) 51:354–7. doi: 10.1111/j.1439-0442.2004.00660.x
109. Alho AM, Cruz L, Coelho A, Martinho F, Mansinho M, Annoscia G, et al. Aberrant laryngeal location of Onchocerca lupi in a dog. Parasitol Int. (2016) 65:218–20. doi: 10.1016/j.parint.2015.12.010
110. Vergou M, Diakou A, Pardali D, Tachmazidou A, Timiou D, Adamama-Moraitou KK, et al. What is your diagnosis? Buccal lymph node fine-needle aspirate from a dog. Vet Clin Pathol. (2022) 51:286–8. doi: 10.1111/vcp.13043
111. Sréter T, Széll Z. Onchocercosis: A newly recognized disease in dogs. Vet Parasitol. (2008) 151:1–13. doi: 10.1016/j.vetpar.2007.09.008
112. Laidoudi Y, Bedjaoui S, Medkour H, Latrofa MS, Mekroud A, Bitam I, et al. Molecular approach for the diagnosis of blood and skin canine filarioids. Microorganisms. (2020) 8:1671. doi: 10.3390/microorganisms8111671
113. Lindsey JR. Diagnosis of filarial infections in dogs. I. Microfilarial surveys. J Parasitol. (1961) 47:695–702. doi: 10.2307/3275448
114. Lindsey JR. Diagnosis of filarial infections in dogs. II. Confirmation of microfilarial identifications. J Parasitol. (1962) 48:321–6. doi: 10.2307/3275595
116. Pennington NE. Arthropod Vectors, Cycle Development and Prepatent Period of Dipetalonema reconditum (Grassi) and the Incidence of Canine Filariasis and Ectoparasites in North-central Oklahoma. Stillwater: Oklahoma State University (1971).
117. Giannelli A, Ramos RA, Traversa D, Brianti E, Annoscia G, Bastelli F, et al. Treatment of Dirofilaria repens microfilariaemia with a combination of doxycycline hyclate and ivermectin. Vet Parasitol. (2013) 197:702–4. doi: 10.1016/j.vetpar.2013.05.012
118. Redington BC, Jackson RF, Seymour WG, Otto GF. The various microfilariae found in dogs in the United States. In:GF Otto, , editor, Proceedings of the Heartworm Symposium ‘77, KS. Stillwater, OK: VM Publishing Co., Bonner Springs (1978). p. 14–21.
119. Ortega-Mora LM, Gomez-Bautista M, Rojo-Vazquez FA. The acid phosphatase activity and morphological characteristics of Dipetalonema dracunculoides (Cobbold, 1870) microfilariae. Vet Parasitol. (1989) 33:187–90. doi: 10.1016/0304-4017(89)90066-6
120. Wolfe MS, Aslamkhan M, Sharif M, Pervez E. Acanthocheilonema dracunculoides (Cobbold, 1870) in dogs in Lahore, West Pakistan. J Helminthol. (1971) 45:171–6. doi: 10.1017/S0022149X00007057
121. Muñoz C, Gonzálvez M, Rojas A, Martínez-Carrasco C, Baneth G, Berriatua E, et al. Massive microfilaremia in a dog subclinically infected with Acanthocheilonema dracunculoides. Parasitol Int. (2020) 76:102070. doi: 10.1016/j.parint.2020.102070
122. Schacher JF. Morphology of the microfilaria of Brugia pahangi and of the larval stages in the mosquito. J Parasitol. (1962) 48:679–92. doi: 10.2307/3275257
123. WHO. Bench Aids for the Diagnosis of Filarial Infections. Geneva: World Health Organization (1997).
124. Solinas C, Varcasia A, Brianti E, Giannetto S, Pipia AP, Columbano N, et al. Cercopithifilaria spp. in dogs in Sardinia Island (Italy). Parasitol Res. (2014) 113:675–9. doi: 10.1007/s00436-013-3695-6
125. Noè G. La Filaria grassii (Noè, 1907). Ricerche Laboratorio di Anatomia Normale Regia Università di Roma. (1911) 15:235–52.
126. Deak G, Toader S, Soare DG, Ionică AM, Taulescu M, Mihalca AD. Case report: A new geographic area for the presence of the zoonotic ocular nematode, Onchocerca lupi in Romania. Front Vet Sci. (2022) 9:941303. doi: 10.3389/fvets.2022.941303
127. Nelson GS. Observations on the development of Setaria labiatopapillosa using new techniques for infecting Aedes aegypti with this nematode. J Helminthol. (1962) 36:281–96. doi: 10.1017/S0022149X00023956
128. Cobbold TS. Description of a new generic type of entozoon from the aardwolf (Proteles); with remarks on its affinities, especially in reference to the question of parthenogenesis. Proc Zool Soc Lond. (1870) 1:9–14.
129. Nelson GS. Dipetalonema dracunculoides (Cobbold, 1870), from the dog in Kenya: with a note on its development in the louse-fly, Hippobosca longipennis. J Helminthol. (1963) 37:235–40. doi: 10.1017/S0022149X00003825
130. Pennington NE, Phelps CA. Canine filariasis on Okinawa, Ryukyu Islands. J Med Entomol. (1969) 6:59–67. doi: 10.1093/jmedent/6.1.59
131. Lindemann BA, Evans TL, McCall JW. Clinical responses of dogs to experimentally induced Dipetalonema reconditum infection. Am J Vet Res. (1983) 44:2170–2.
132. Napoli E, Brianti E, Falsone L, Gaglio G, Foit S, Abramo F, et al. Development of Acanthocheilonema reconditum (Spirurida, Onchocercidae) in the cat flea Ctenocephalides felis (Siphonaptera, Pulicidae). Parasitology. (2014) 141:1718–25. doi: 10.1017/S0031182014001000
133. Olmeda-García AS, Rodríguez-Rodríguez JA, Rojo-Vázquez FA. Experimental transmission of Dipetalonema dracunculoides (Cobbold 1870) by Rhipicephalus sanguineus (Latreille 1806). Vet Parasitol. (1993) 47:339–42. doi: 10.1016/0304-4017(93)90034-K
134. Lindemann BA. The Biology of Experimental Dipetalonema reconditum Infections in Beagles. Athens: University of Georgia (1982).
135. Sawyer TK. Molting and ensheathment in vitro of third-stage Dirofilaria immitis. J Parasitol. (1965) 51:1016–7. doi: 10.2307/3275900
136. Farnell DR, Faulkner DR. Prepatent period of Dipetalonema reconditum in experimentally-infected dogs. J Parasitol. (1978) 64:565–7. doi: 10.2307/3279818
137. Pratt SE, Corwin RM, Selby LA, Rhoades JD. Prevalence of Dirofilaria immitis and Dipetalonema reconditum infections in Missouri dogs. J Am Vet Med Assoc. (1981) 179:592–3.
138. Lindemann BA, McCall JW. Experimental Dipetalonema reconditum infections in dogs. J Parasitol. (1984) 70:167–8. doi: 10.2307/3281950
139. Pappas LG, Lunzman AT. Canine heartworm in the domestic and wild canids of southeastern Nebraska. J Parasitol. (1985) 71:828–30. doi: 10.2307/3281722
140. Pacifico L, Ferrari N, Romeo C, Buono F, Varuzza P, Sgroi G, et al. Haematological and biochemical abnormalities in hunting dogs infected with Acanthocheilonema reconditum, associated risk factors, and a European overview. Parasitol Res. (2021) 120:2109–24. doi: 10.1007/s00436-021-07179-8
141. Knott J. A method for making microfilarial surveys on day blood. Trans R Soc Trop Med Hyg. (1939) 33:191–6. doi: 10.1016/S0035-9203(39)90101-X
142. Chalifoux L, Hunt RD. Histochemical differentiation of Dirofilaria immitis and Dipetalonema reconditum. J Am Vet Med Assoc. (1971) 158:601–5.
143. Dantas-Torres F, Otranto D. On the validity of “Candidatus Dirofilaria hongkongensis” and on the use of the provisional status Candidatus in zoological nomenclature. Parasit Vectors. (2020) 13:287. doi: 10.1186/s13071-020-04158-3
144. Espinosa N, Rosero A, Villegas CL, Garcia IC, Gaviria-Cantin T, Nieto AP, et al. First report of Acanthocheilonema reconditum outbreak in canines with clinical signs of anemia from Southwestern Colombia. Pathogens. (2022) 11:1434. doi: 10.3390/pathogens11121434
145. Altman NH, Yarbrough B. A rapid screening program for the diagnosis of Dirofilaria immitis infections in dogs. Lab Anim Sci. (1973) 23:701–3.
146. Otranto D, de Caprariis D, Lia RP, Tarallo V, Lorusso V, Testini G, et al. Prevention of endemic canine vector-borne diseases using imidacloprid 10% and permethrin 50% in young dogs: A longitudinal field study. Vet Parasitol. (2010) 172:323–32. doi: 10.1016/j.vetpar.2010.05.017
147. Ionică AM, Matei IA, D'Amico G, Bel LV, Dumitrache MO, Modrý D, et al. Dirofilaria immitis and D. repens show circadian co-periodicity in naturally co-infected dogs. Parasit Vectors. (2017) 10:116. doi: 10.1186/s13071-017-2055-2
148. Laidoudi Y, Davoust B, Varloud M, Niang EHA, Fenollar F, Mediannikov O. Development of a multiplex qPCR-based approach for the diagnosis of Dirofilaria immitis, D. repens and Acanthocheilonema reconditum. Parasit Vectors. (2020) 13:319. doi: 10.1186/s13071-020-04185-0
149. Rothstein N, Kinnamon KE, Brown ML, Carithers RW. Canine microfilariasis in eastern United States. J Parasitol. (1961) 47:661–5. doi: 10.2307/3275082
150. Price EW. Endemic elephantiasis: Early signs and symptoms, and control. Ethiop Med J. (1983) 21:243–53.
151. Courtney CH, Sundlof SF, Lane TJ. Impact of filariasis on the racing greyhound. J Am An Hosp Assoc. (1985) 21:421–5.
152. Valcárcel F, Ferre I, Gómez-Bautista M, Rojo-Vázquez FA. Diagnóstico de laboratorio de la infestación por Dirofilaria immitis en el perro. Med Vet. (1990) 7:345–53.
153. Schwan EV, Schröter FG. First record of Acanthocheilonema dracunculoides from domestic dogs in Namibia. J S Afr Vet Assoc. (2006) 77:220–1. doi: 10.4102/jsava.v77i4.381
154. Mallawarachchi CH, Chandrasena TGAN, Withanage GP, Premarathna R, Mallawarachchi SMNSM, Gunawardane NY, et al. Molecular characterization of a reemergent Brugia malayi parasite in Sri Lanka, suggestive of a novel strain. Biomed Res Int. (2021) 2021:9926101. doi: 10.1155/2021/9926101
155. Vinnie-Siow WY, Low VL, Tan TK, Wong ML, Leong CS, Ahmad NW, et al. Identification of potential vectors of Dirofilaria immitis and Brugia pahangi (Spirurida: Filariidae): First observation of infective third-stage larva of B. pahangi in Culex quinquefasciatus (Diptera: Culicidae). Pathog Glob Health. (2022) 116:356–64. doi: 10.1080/20477724.2022.2035624
156. Jones RT. Non-endemic cases of lymphatic filariasis. Trop Med Int Health. (2014) 19:1377–83. doi: 10.1111/tmi.12376
157. Supali T, Djuardi Y, Bradley M, Noordin R, Rückert P, Fischer PU. Impact of six rounds of mass drug administration on Brugian filariasis and soil-transmitted helminth infections in eastern Indonesia. PLoS Negl Trop Dis. (2013) 7:e2586. doi: 10.1371/journal.pntd.0002586
158. Rathnayake S, Chandrasena N, Wijerathna T, Mallawarachchi H, Gunathilaka N. Canine filaria species in selected lymphatic filariasis endemic and non-endemic areas in Sri Lanka. Parasitol Res. (2022) 121:2187–91. doi: 10.1007/s00436-022-07555-y
159. Evans C, Pilotte N, Williams S, Moorhead A. Veterinary diagnosis of filarial infection. In: Human and Animal Filariases. Wiley VCH (2022). p. 125–59.
160. Haynes E, Cleveland CA, Garrett KB, Grunert RKA, Bryan JA, Sidouin M, et al. Characterization of the genetics and epidemiology of Brugia sp. in domestic dogs in Chad, Africa. Vet Parasitol Reg Stud Rep. (2022) 35:100784. doi: 10.1016/j.vprsr.2022.100784
161. Ash LR, Little MD. Brugia beaveri sp. n (Nematoda: Filarioidea) from the raccoon (Procyon lotor) in Louisiana. J Parasitol. (1964) 50:119–23. doi: 10.2307/3276044
162. Beaver PC, Wong MM. Brugia sp. from a domestic cat in California. Proc Helminthol Soc Wash. (1988) 55:111–3.
163. Eberhard ML. Brugia lepori sp. n (Filarioidea: Onchocercidae) from rabbits (Sylvilagus aquaticus, S floridanus) in Louisiana. J Parasitol. (1984) 70:576–9. doi: 10.2307/3281408
164. Eberhard ML, Telford SR 3rd, Spielman A. A Brugia species infecting rabbits in the northeastern United States. J Parasitol. (1991) 77:796–8. doi: 10.2307/3282722
165. Harbut CL. The white rat and golden hamster as experimental hosts for Brugia pahangi and subperiodic Brugia malayi. South Asian J Trop Med Public Health. (1973) 4:487–91.
166. Schlesinger JJ, Dubois JG, Beaver PC. Brugia-like filarial infection acquired in the United States. Am J Trop Med Hyg. (1977) 26:204–7. doi: 10.4269/ajtmh.1977.26.2.TM0260020204
167. Kulpa M, Goldsmith D, Verocai GG. An unusual case of Brugia sp. infection in a dog from Alberta, Canada. Vet Parasitol Reg Stud Rep. (2023) 21:100811. doi: 10.1016/j.vprsr.2022.100811
168. Baird JK, Alpert LI, Friedman R, Schraft WC, Connor DH. North American brugian filariasis: Report of nine infections of humans. Am J Trop Med Hyg. (1986) 35:1205–9. doi: 10.4269/ajtmh.1986.35.1205
169. Orihel TC, Beaver PC. Zoonotic Brugia infections in North and South America. Am J Trop Med Hyg. (1989) 40:638–47. doi: 10.4269/ajtmh.1989.40.638
170. Eberhard ML, DeMeester LJ, Martin BW, Lammie PJ. Zoonotic Brugia infection in western Michigan. Am J Surg Pathol. (1993) 17:1058–61. doi: 10.1097/00000478-199310000-00012
171. Elenitoba-Johnson KS, Eberhard ML, Dauphinais RM, Lammie PJ, Khorsand J. Zoonotic Brugian lymphadenitis. An unusual case with florid monocytoid B-cell proliferation. Am J Clin Pathol. (1996) 105:384–7. doi: 10.1093/ajcp/105.4.384
172. Canestri Trotti G, Pampiglione S, Rivasi F. The species of the genus Dirofilaria, Railliet & Henry, 1911. Parassitologia. (1997) 39:369–74.
173. Silaghi C, Beck R, Capelli G, Montarsi F, Mathis A. Development of Dirofilaria immitis and Dirofilaria repens in Aedes japonicus and Aedes geniculatus. Parasit Vectors. (2017) 10:94. doi: 10.1186/s13071-017-2015-x
174. Yamada M, Shishito N, Nozawa Y, Uni S, Nishioka K, Nakaya T. A combined human case of Dirofilaria ursi infection in dorsal subcutaneous tissue and Anisakis simplex sensu stricto (ss) infection in ventral subcutaneous tissue. Trop Med Health. (2017) 45:26. doi: 10.1186/s41182-017-0067-4
175. Mendoza-Roldan JA, Gabrielli S, Cascio A, Manoj RRS, Bezerra-Santos MA, Benelli G, et al. Zoonotic Dirofilaria immitis and Dirofilaria repens infection in humans and an integrative approach to the diagnosis. Acta Trop. (2021) 223:106083. doi: 10.1016/j.actatropica.2021.106083
176. Capelli G, Genchi C, Baneth G, Bourdeau P, Brianti E, Cardoso L, et al. Recent advances on Dirofilaria repens in dogs and humans in Europe. Parasit Vectors. (2018) 11:663. doi: 10.1186/s13071-018-3205-x
177. Geissler N, Ruff J, Walochnik J, Ludwig W, Auer H, Wiedermann U, et al. Autochthonous Human Dirofilaria repens Infection in Austria. Acta Parasitol. (2022) 67:1039–43. doi: 10.1007/s11686-021-00506-0
178. Ferrara M, Maglione R, Ciccarelli D, Mundis SJ, Di Loria A, de Carvalho MP, et al. Prevalence of Dirofilaria repens in dogs living in deltaic coastal plain of the Volturno River (Italy): A geographical risk model of infection. J Helminthol. (2022) 96:62. doi: 10.1017/S0022149X22000062
179. Heisch RB, Nelson GS, Furlong M. Studies in filariasis in East Africa. 1. Filariasis on the Island of Pate, Kenya. Trans R Soc Trop Med Hyg. (1959) 53:41–53. doi: 10.1016/0035-9203(59)90082-3
180. Rocconi F, Di Tommaso M, Traversa D, Palmieri C, Pampurini F, Boari A. Allergic dermatitis by Dirofilaria repens in a dog: Clinical picture and treatment. Parasitol Res. (2012) 111:493–6. doi: 10.1007/s00436-012-2833-x
181. Kotani T, Powers KG. Developmental stages of Dirofilaria immitis in the dog. Am J Vet Res. (1982) 43:2199–206.
182. Theis JH. Public health aspects of dirofilariasis in the United States. Vet Parasitol. (2005) 133:157–80. doi: 10.1016/j.vetpar.2005.04.007
183. Beasley MJ, Hiebert EC, Alcott C, Jeffery N, LaFoon-Jones B, Gambino JM. Verminous myelopathy secondary to aberrant Dirofilaria immitis migrans was detected in the cervical subarachnoid space of four dogs using MRI and CT. Vet Radiol Ultrasound. (2021) 62:299–308. doi: 10.1111/vru.12954
184. Oliveira LB, McHale BJ, Verocai GG, Rissi DR. Subcutaneous and cardiopulmonary dirofilariasis in a dog. Can Vet J. (2021) 62:854–6.
185. Goh YS, Kim HM, Alkathiri B, Chang HS, Yoon YM, Lee SH, et al. Two cases of ectopic dirofilariasis by Dirofilaria immitis in subconjunctival and subcutaneous tissues in dogs. Parasitol Int. (2023) 92:102683. doi: 10.1016/j.parint.2022.102683
186. Velasquez L, Blagburn BL, Duncan-Decoq R, Johnson EM, Allen KE, Meinkoth J, et al. Increased prevalence of Dirofilaria immitis antigen in canine samples after heat treatment. Vet Parasitol. (2014) 206:67–70. doi: 10.1016/j.vetpar.2014.03.021
187. Kwok RP, Chow PP, Lam JK, Fok AC, Jhanji V, Wong VW, et al. Human ocular dirofilariasis in Hong Kong. Optom Vis Sci. (2016) 93:545–8. doi: 10.1097/OPX.0000000000000770
188. Pradeep RK, Nimisha M, Pakideery V, Johns J, Chandy G, Nair S, et al. Whether Dirofilaria repens parasites from South India belong to zoonotic Candidatus Dirofilaria hongkongensis (Dirofilaria sp. hongkongensis)? Infect Genet Evol. (2019) 67:121–5. doi: 10.1016/j.meegid.2018.10.019
189. Xing F, Li X, Lo SKF, Poon RWS, Lau SKP, Woo PCY. Dirofilaria hongkongensis infection presenting as recurrent shoulder mass. Parasitol Int. (2020) 77:102117. doi: 10.1016/j.parint.2020.102117
190. Courtney CH, Zeng QY. Relationship between microfilaria count and sensitivity of the direct smear for diagnosis of canine dirofilariosis. Vet Parasitol. (2001) 94:199–204. doi: 10.1016/S0304-4017(00)00377-0
191. Buckley JJ, Nelson GS, Heisch RB. On Wuchereria patei n. sp from the lymphatics of cats, dogs and genet cats on Pate Island, Kenya. J Helminthol. (1958) 32:73–80. doi: 10.1017/S0022149X00019362
192. Hargis AM, Lewis TP, Duclos DD, Loeffler DG, Rausch RL. Dermatitis Associated With Microfilariae (Filarioidea) in 10 Dogs. Vet Dermatol. (1999) 10:95–107. doi: 10.1046/j.1365-3164.1999.00136.x
193. Liotta JL, Sandhu GK, Rishniw M, Bowman DD. Differentiation of the microfilariae of Dirofilaria immitis and Dirofilaria repens in stained blood films. J Parasitol. (2013) 99:421–5. doi: 10.1645/12-10.1
194. Soulsby EJ. Helminths, Arthropods and Protozoa of Domesticated Animals. 6th ed. Baltimore: Williams and Wilkins Co., (1968). p. 824.
195. Lamm MG, Roelke ME, Greiner EC, Steible CK. Microfilariae in the free-fanging Florida Panther. J Helminthol Soc Washington. (1997) 64:1.
196. Scoles GA, Kambhampati S. Polymerase chain reaction-based method for the detection of canine heartworm (Filarioidea: Onchocercidae) in mosquitoes (Diptera: Culicidae) and vertebrate hosts. J Med Entomol. (1995) 32:864–9. doi: 10.1093/jmedent/32.6.864
197. Favia G, Lanfrancotti A, Della Torre A, Cancrini G, Coluzzi M. Polymerase chain reaction-identification of Dirofilaria repens and Dirofilaria immitis. Parasitology. (1996) 113:567–71. doi: 10.1017/S0031182000067615
198. Xie H, Bain O, Williams SA. Molecular phylogenetic studies on Brugia filariae using Hha I repeat sequences. Parasite. (1994) 1:255–60. doi: 10.1051/parasite/1994013255
199. Orihel TC. Dirofilaria lutrae sp. n (Nematoda: Filarioidea) from otters in the southeast United States. J Parasitol. (1965) 51:409–13. doi: 10.2307/3275965
200. Casiraghi M, Bazzocchi C, Mortarino M, Ottina E, Genchi C. A simple molecular method for discriminating common filarial nematodes of dogs (Canis familiaris). Vet Parasitol. (2006) 141:368–72. doi: 10.1016/j.vetpar.2006.06.006
201. Latrofa MS, Dantas-Torres F, Annoscia G, Genchi M, Traversa D, Otranto D, et al. Duplex real-time polymerase chain reaction assay for the detection of and differentiation between Dirofilaria immitis and Dirofilaria repens in dogs and mosquitoes. Vet Parasitol. (2012) 185:181–5. doi: 10.1016/j.vetpar.2011.10.038
202. Savadelis MD, Day KM, Bradner JL, Wolstenholme AJ, Dzimianski MT, Moorhead AR. Efficacy and side effects of doxycycline vs. minocycline in the three-dose melarsomine canine adulticidal heartworm treatment protocol. Parasit Vectors. (2018) 11:671. doi: 10.1186/s13071-018-3264-z
203. Roe CC, Urbanz J, Andrews L, Verocai GG, Engelthaler DM, Hepp CM, et al. Complete mitochondrial genome of Onchocerca lupi (Nematoda, Onchocercidae). Mitochondrial DNA B Resour. (2021) 6:2572–4. doi: 10.1080/23802359.2021.1960211
204. Roe CC, Urbanz J, Auten C, Verocai GG, Upshaw-Bia K, Holiday O, et al. LupiQuant: A real-time PCR based assay for determining host-to-parasite DNA ratios of Onchocerca lupi and host Canis lupus from onchocercosis samples. PLoS ONE. (2022) 17:e0276916. doi: 10.1371/journal.pone.0276916
205. Sivanandam S, Fredericks HJ. The “Innenkorper” in differentiation between the microfilanriae of Brugia pahangi and B. malayi (sub-periodic form). Med J Malaya. (1966) 20:337–8.
206. Pacheco G, Tulloch GS. Microfilariae of Dirofilaria striata in a dog. J Parasitol. (1970) 56:248. doi: 10.2307/3277650
207. Swanepoel L, Cleveland CA, Olfenbuttel C, Dukes CG, Brown D, Brown JD, et al. Prevalence and genetic characterization of Dirofilaria lutrae Orihel, 1965 in North American river otters (Lontra canadensis). Vet Parasitol Reg Stud Reports. (2018) 14:187–90. doi: 10.1016/j.vprsr.2018.10.007
208. Morales A, Perlmann E, Abelha ANV, Levy CE, de Goes ACA, Safatle AMV. Keratitis due to microfilariae in dogs: A newly recognized disease. Vet Ophthalmol. (2018) 21:305–11. doi: 10.1111/vop.12482
209. Simón F, Siles-Lucas M, Morchón R, González-Miguel J, Mellado I, Carretón E, et al. Human and animal dirofilariasis: The emergence of a zoonotic mosaic. Clin Microbiol Rev. (2012) 25:507–44. doi: 10.1128/CMR.00012-12
210. Buckley JJ. On Brugia gen. nov. for Wuchereria spp. of the “malayi” group, i.e., W. malayi (Brug, 1927), W. pahangi Buckley and Edeson, 1956, and W. patei Buckley, Nelson and Heisch, 1958. Ann Trop Med Parasitol. (1960) 54:75–7. doi: 10.1080/00034983.1960.11685959
211. Huynh T, Thean J, Maini R. Dipetalonema reconditum in the human eye. Br J Ophthalmol. (2001) 85:1391–2. doi: 10.1136/bjo.85.11.1384i
212. Mulyaningsih B, Umniyati SR, Hadisusanto S, Edyansyah E. Fauna associated with Malayan filariasis transmission in Banyuasin, South Sumatra, Indonesia. Vet World. (2021) 14:1954–9. doi: 10.14202/vetworld.2021.1954-1959
213. Fontes-Sousa AP, Silvestre-Ferreira AC, Carretón E, Esteves-Guimarães J, Maia-Rocha C, Oliveira P, et al. Exposure of humans to the zoonotic nematode Dirofilaria immitis in Northern Portugal. Epidemiol Infect. (2019) 147:e282. doi: 10.1017/S0950268819001687
214. Alsarraf M, Baneth G, Bogucka-Kocka A, Ciuca L, Dwuznik-Szarek D, Fuehrer HP, et al. Haplotypes of Dirofilaria repens From Poland and Selected Countries of Central, North-Eastern Europe and the Middle East: An Evaluation on the Relation Between the Genetic Diversity and the Geographic Distribution of the Fast-Spreading Parasite. Available online at: https://ssrn.com/abstract=4191291 (accessed January 10, 2023).
215. Beaver PC, Wolfson JS, Waldron MA, Swartz MN, Evans GW, Adler J. Dirofilaria ursi-like parasites acquired by humans in the northern United States and Canada: Report of two cases and brief review. Am J Trop Med Hyg. (1987) 37:357–62. doi: 10.4269/ajtmh.1987.37.357
216. Horst A. Dirofilaria and dirofilarioses; Introductory remarks. In: Proceedings of Helminthological Colloquium Vienna. Austria (2003).
217. Warthan ML, Warthan TL, Hearne RH, Polk AC, Warthan MM. Human dirofilariasis: Raccoon heartworm causing a leg nodule. Cutis. (2007) 80:125–8.
218. Chandy A, Thakur AS, Singh MP, Manigauha A. A review of neglected tropical diseases: Filariasis. Asian Pac J Trop Med. (2011) 4:581–6. doi: 10.1016/S1995-7645(11)60150-8
219. Cháves-González LE, Morales-Calvo F, Mora J, Solano-Barquero A, Verocai GG, Rojas A. What lies behind the curtain: Cryptic diversity in helminth parasites of human and veterinary importance. Curr Res Parasitol Vector Borne Dis. (2022) 2:100094. doi: 10.1016/j.crpvbd.2022.100094
220. Moraes MFD, da Silva MX, Magalhães-Matos PC, de Albuquerque ACA, Tebaldi JH, Mathias LA, et al. Filarial nematodes with zoonotic potential in ring-tailed coatis (Nasua nasua Linnaeus, 1766, Carnivora: Procyonidae) and domestic dogs from Iguaçu National Park, Brazil. Vet Parasitol Reg Stud Reports. (2017) 8:1–9. doi: 10.1016/j.vprsr.2017.01.003
221. Sophia DC, Aitor C, Claudia UC, Javier C, Delia G, Valeria G, et al. Large-scale survey for canine vector-borne parasites in free-ranging dogs and foxes from six diverse bioclimatic regions of Chile. Vet Parasitol Reg Stud Reports. (2022) 30:100721. doi: 10.1016/j.vprsr.2022.100721
222. Wyatt D, Santoro D, Deabold K, Gruntmeir J, Childress A, Craft WF, et al. Subcutaneous nodules and dermatitis associated with non-immitis non-repens dirofilariosis morphologically consistent with Dirofilaria striata in a 2-year-old male domestic cat in Florida, USA. Vet Q. (2020) 40:215–22. doi: 10.1080/01652176.2020.1814972
223. Verocai GG, Lejeune M, Beckmen KB, Kashivakura CK, Veitch AM, Popko RA, et al. Defining parasite biodiversity at high latitudes of North America: New host and geographic records for Onchocerca cervipedis (Nematoda: Onchocercidae) in moose and caribou. Parasit Vectors. (2012) 5:242. doi: 10.1186/1756-3305-5-242
224. McFrederick QS, Haselkorn TS, Verocai GG, Jaenike J. Cryptic Onchocerca species infecting North American cervids, with implications for the evolutionary history of host associations in Onchocerca. Parasitology. (2013) 140:1201–10. doi: 10.1017/S0031182012001758
225. Verocai GG, Nelson KJ, Callahan RT, Wekesa JW, Hassan HK, Hoberg EP. A cryptic species of Onchocerca (Nematoda: Onchocercidae) in blackflies (Simulium spp) from southern California, USA. Parasit Vectors. (2018) 11:547. doi: 10.1186/s13071-018-3133-9
226. Kulpa M, Nelson KJ, Morales AM, Ryan BM, Koschik ML, Scott JJ, et al. Presence of a cryptic Onchocerca species in black flies of northern California, USA. Parasit Vectors. (2021) 14:478. doi: 10.1186/s13071-021-04990-1
227. Benedict BM, Barboza PS, Crouse JA, Groch KR, Kulpa MR, Thompson DP, et al. Sores of boreal moose reveal a previously unknown genetic lineage of parasitic nematode within the genus Onchocerca. PLoS ONE. (2023) 18:e0278886. doi: 10.1371/journal.pone.0278886
228. Jayewardene LG. Larval development of Brugia ceylonensis Jayewardene, 1962, in Aedes aegypti, with a brief comparison of the infective larva with those of Brugia spp., Dirofilaria repens and Artionema digitata. Ann Trop Med Parasitol. (1963) 57:359–70. doi: 10.1080/00034983.1963.11686188
229. Mar PH, Yang IC, Chang GN, Fei AC. Specific polymerase chain reaction for differential diagnosis of Dirofilaria immitis and Dipetalonema reconditum using primers derived from internal transcribed spacer region 2 (ITS2). Vet Parasitol. (2002) 106:243–52. doi: 10.1016/S0304-4017(02)00032-8
230. Jayewardene LG. On two filarial parasites from dogs in Ceylon, Brugia ceylonensis n. sp and Dipetalonema sp. J Helminthol. (1962) 36:269–80. doi: 10.1017/S0022149X00023944
231. Peribáñez MA, Lucientes J, Arce S, Morales M, Castillo JA, Gracia MJ. Histochemical differentiation of Dirofilaria immitis, Dirofilaria repens and Acanthocheilonema dracunculoides microfilariae by staining with a commercial kit, Leucognost-SP. Vet Parasitol. (2001) 102:173–5. doi: 10.1016/S0304-4017(01)00516-7
232. Michalski ML, Bain O, Fischer K, Fischer PU, Kumar S, Foster JM. Identification and phylogenetic analysis of Dirofilaria ursi (Nematoda: Filarioidea) from Wisconsin black bears (Ursus americanus) and its Wolbachia endosymbiont. J Parasitol. (2010) 96:412–9. doi: 10.1645/GE-2208.1
233. Feng LC. A comparative study of the anatomy of Microfilaria malayi Brug, 1927 and Microfilaria bancrofti Cobbold, 1877. Chin Med J. (1933) 47:1214–46.
Keywords: Onchocerca, Cercopithifilaria, Dirofilaria, diagnosis, vector-borne diseases, zoonosis
Citation: Gruntmeir J, Kelly M, Ramos RAN and Verocai GG (2023) Cutaneous filarioid nematodes of dogs in the United States: Are they emerging, neglected, or underdiagnosed parasites? Front. Vet. Sci. 10:1128611. doi: 10.3389/fvets.2023.1128611
Received: 20 December 2022; Accepted: 30 January 2023;
Published: 23 February 2023.
Edited by:
Yukifumi Nawa, Khon Kaen University, ThailandReviewed by:
Uni Shigehiko, Kobe Women's University, JapanClaudio Genchi, University of Milan, Italy
Ayako Yoshida, University of Miyazaki, Japan
Copyright © 2023 Gruntmeir, Kelly, Ramos and Verocai. This is an open-access article distributed under the terms of the Creative Commons Attribution License (CC BY). The use, distribution or reproduction in other forums is permitted, provided the original author(s) and the copyright owner(s) are credited and that the original publication in this journal is cited, in accordance with accepted academic practice. No use, distribution or reproduction is permitted which does not comply with these terms.
*Correspondence: Guilherme Gomes Verocai, Z3Zlcm9jYWlAY3ZtLnRhbXUuZWR1
†These authors have contributed equally to this work