- 1State Key Laboratory of Plateau Ecology and Agriculture, Qinghai University, Xining, China
- 2College of Agriculture and Animal Husbandry, Qinghai University, Xining, China
- 3Qinghai Provincial Key Laboratory of Pathogen Diagnosis for Animal Diseases and Green Technical Research for Prevention and Control, Qinghai University, Xining, China
Dermacentor nuttalli has been a focus of study because tick-borne pathogens have been widely identified in this tick from northern and southwestern China. The aim of this study was to characterize the life cycle of D. nuttalli under laboratory conditions and to detect spotted fever group (SFG) Rickettsia in the midgut and salivary glands of both field-collected and first laboratory generation adults. D. nuttalli ticks were collected in the field on the Qinghai-Tibetan Plateau from March to April 2021 and their life cycle was studied under laboratory conditions. Tick identify was molecularly confirmed, and SFG Rickettsia were detected in the midgut and salivary glands of males and females by PCR targeting different rickettsial genes. The results showed that the life cycle of D. nuttalli under laboratory conditions was completed in an average of 86.1 days. High positivity of Rickettsia spp. was detected in the midgut and salivary glands of both males (92.0%) and females (93.0%) of field-collected D. nuttalli ticks. However, a relatively lower positivity (4.0–6.0%) was detected in first laboratory generation adults. Furthermore, sequencing analysis showed that the Rickettsia sequences obtained in this study shared 98.6 to 100% nucleotide identity with Rickettsia slovaca and Rickettsia raoultii isolated from Dermacentor spp. in China. Phylogenetic analysis of Rickettsia spp. based on the gltA, ompA, ompB and sca4 genes revealed that the Rickettsia sequences obtained could be classified as belonging to R. slovaca and R. raoultii clades. This study described for the first time the life cycle of D. nuttalli from the Qinghai-Tibetan Plateau under laboratory conditions. Two species of SFG Rickettsia were detected in the midgut and salivary glands of males and females in both field-collected and first laboratory-generation adults of D. nuttalli. Our study provides new insights into pathogen detection in ticks in the Qinghai-Tibet Plateau, and the relationships among hosts, ticks, and pathogens.
Introduction
Ticks are vectors transmitting a large number of pathogenic bacteria, protozoa, and viruses to humans and animals that cause serious diseases (1, 2). Species belonging to the genus Dermacentor (Acari: Ixodidae), including D. marginatus, D. nuttalli and D. silvarum are well known as vectors for a great variety of infectious pathogens (3, 4). Among Dermacentor spp., D. nuttalli and D. silvarum are widely distributed in northern China, Mongolia, and Russia (5–9). D. nuttalli as a major vector for transmitting various diseases including tick-borne rickettsiosis, Q fever, anaplasmosis, and tick-borne encephalitis virus, has been reported in Europe and some countries in Asia (3, 9–16). In China, it was highlighted because the genus Rickettsia has been widely identified in D. nuttalli in northern and southwestern regions of the country (8, 16–29).
Tick-borne rickettsiosis, which is caused by species of spotted fever group (SFG) Rickettsia, poses a serious threat to the health of humans and animals (30–32). The SFG Rickettsia species, including R. raoultii, R. slovaca, R. sibirica, R. conorii, R. rickettsii, R. heilongjiangensis, R. aeschlimannii, R. felis, R. massiliae, and R. monacensis, have been identified in ticks, humans, and animals in China (23, 25, 33–38). Among them, R. raoultii and R. heilongjiangensis were identified in tick vectors and humans, and are pathogenic factors of human rickettsiosis in China (39–41).
The salivary glands and midgut in ticks, as specific and major barriers to efficient pathogen transmission, are the main factors influencing the acquisition, maintenance, colonization, and transmission of pathogens by ticks (42). The tick's salivary glands can transmit pathogens to the host during blood-feeding along with salivary secretions, and the midgut determines pathogen colonization and survival in ticks (42–45). The influences of salivary glands and the midgut on pathogen transmission and survival is not independent, rather there is a pathogenic life cycle: the salivary glands are infected by the ingestion of the infected host blood, the pathogens colonize and multiply after they are transported into the midgut along with the blood, pathogens are exchanged between the midgut and salivary glands, and the salivary glands transmit the pathogens to another host when the ticks blood-feed again (42). Studies of the genus Rickettsia in both the midgut and salivary glands of D. nuttalli are limited. The aim of this study was to characterize the life cycle of D. nuttalli under laboratory conditions, and to detect SFG Rickettsia in the midgut and salivary glands of D. nuttalli males and females in both field-collected and first laboratory-generation adults.
Materials and methods
Sample collection of ticks
Adult ticks were collected on vegetation from 8 counties in Haidong city (102°09′-102°47′ E and 36°16′-36°40′ N, average elevation of more than 2,500 m above sea level) of Qinghai Province (89°35–103°04′ E and 31°36′-39°19′N) on the Qinghai-Tibetan Plateau (73°19′-104°47′ E and 26°00′-39°47′ N) in northwestern China from March to April 2021 (Figure 1). All ticks were placed in sterile glass bottles, sent to Qinghai University within one day, and reared in an incubator at 25–30°C and 60–90% humidity.
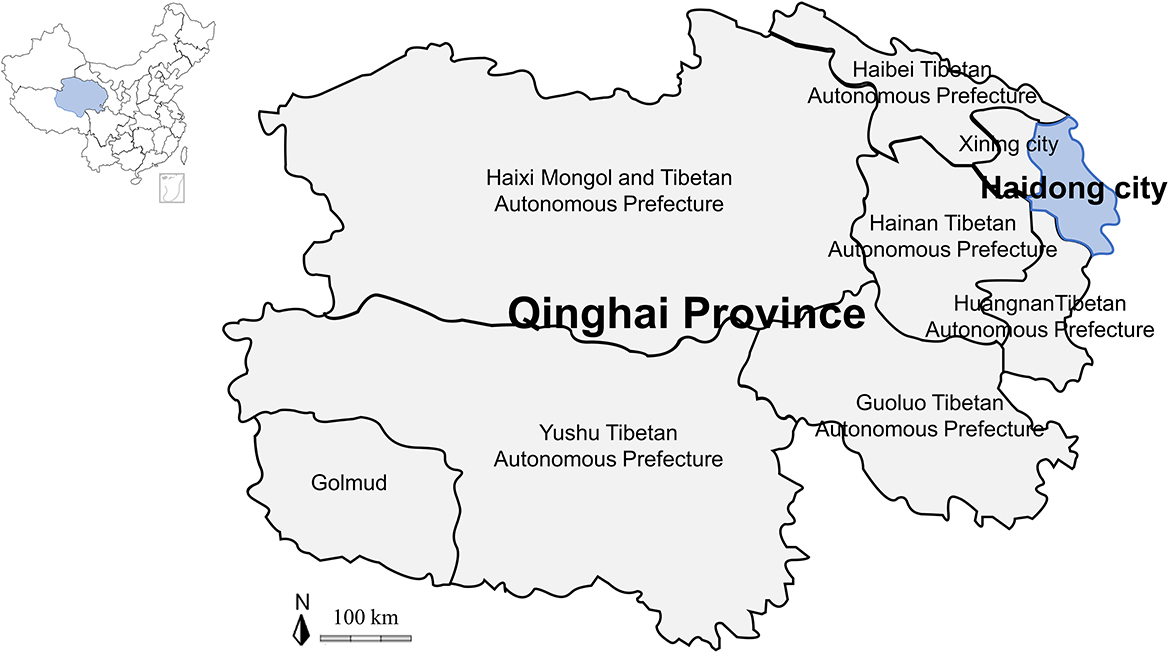
Figure 1. Map of the Qinghai-Tibetan Plateau area and Qinghai Province showing the sampling area in this study.
Morphological identification of tick species and laboratory-rearing of ticks
Microscopy identification of tick species and sex was performed at Qinghai University. After morphological identification (46), 200 males and 200 females of D. nuttalli were selected for laboratory rearing.
To characterize the life-cycle of D. nuttalli, New Zealand white rabbits (specific-pathogen-free, female, 9-weeks-old, purchased from Yifengda, Xi'an, China) housed in the animal facility of Qinghai University at a temperature of 25 ± 2°C and humidity of 40% under controlled lighting (i.e., period of light from 6:00 to 19:00 h) were used to feed the adult ticks. After a few days, engorged ticks were weighed, placed in a sterile glass bottle, and reared in an incubator at 25 ± 2°C and 80% humidity. After tick eggs were laid and hatched, a total of 500 larvae were allowed to refeed on the ears of rabbits within 3–4 days after hatching all eggs. The engorged ticks were collected for incubation until molting as nymphs and then allowed to feed on the rabbit ears again within 12–14 days after molting all larvae. During these periods, the processes of egg laying, hatching, and molting and the viability of the ticks were regularly observed. All animal procedures were carried out according to the ethical guidelines of Qinghai University (2020017 and SL-2022007).
DNA extraction and molecular characterization of ticks
In this study, after morphological identification of the field-collected D. nuttalli, we randomly selected five females and males to extract DNA for molecular identification of ticks. And for the first-laboratory generation, we also randomly selected five males and five females for molecular identification. DNA from the field-collected and first-laboratory generation adult males and females was extracted using the QIAamp DNA Mini Kit (QIAGEN, Germany) according to the manufacturer's manual. The DNA concentration was determined using a NanoDrop 2000 (Thermo Fisher Scientific, USA), and the DNA was stored at−80°C until further use. To further confirm the tick species, PCRs based on the 12S ribosomal RNA (12S rRNA), 16S ribosomal RNA (16S rRNA), cytochrome c oxidase subunit I (COI), and internal transcribed spacer 2 (ITS2) genes were conducted, respectively. All primers are listed in Table 1. The PCR assay volume was 10 μl including 2 μl of DNA, 0.5 μl of each forward and reverse primer (100 μM), 0.2 μl of deoxyribonucleotide triphosphate (200 μM, New England BioLab, USA), 1 μl of 10× ThermoPol Reaction Buffer (New England BioLab, USA), 0.1 μl of Taq polymerase (0.5 U, New England BioLab, USA) and enough double-distilled water to reach a final volume of 10 μl. Double-distilled water was used as a negative control and D. nuttalli DNA stored in our laboratory was used as a positive control.
Detection and identification of SFG Rickettsia
To identify SFG Rickettsia in the midgut and salivary glands in both field-collected and first-laboratory generation males and females of D. nuttalli in the Qinghai-Tibetan Plateau area, organ DNA from 100 original males, 100 original females, 100 first-laboratory generation males and 116 first-laboratory generation females chosen randomly was extracted and screened using previously established PCR assays based on the citrate synthase gene (gltA). To further confirm SFG Rickettsia species, all samples positive for the gltA gene were tested for the outer membrane protein A (ompA) gene, outer membrane protein B (ompB) gene, and surface cell antigen 4 (sca4) gene. All primers for the four genes are listed in Table 1. The PCRs were performed as described above.
Sequence analysis
For the confirmation of ticks and detected pathogens, at least one positive sample of each tissue per sex was selected for sequencing and molecular characterization. PCR products of the positive samples were purified using a QIAquick Gel Extraction Kit (QIAGEN, Germany). Purified PCR products was cloned into E. coli DH5α using the PMDTM 19-T Vector Cloning Kit (TaKaRa, Japan). At least three positive clones were sequenced by Sangon Biotech (Shanghai) Co., Ltd. The obtained sequences were confirmed by a BLASTn search in GenBank.
Phylogenetic analyses
Phylogenetic trees were constructed using the maximum likelihood statistical method and bootstrap analysis with 500 replications in MEGA7: Molecular Evolutionary Genetics Analysis version 7.0 for larger datasets (51).
Statistical analysis
The chi-squared test was used to compare proportions of detected sample positivity in different regions and among different animals via Prism 8 software. Observed differences were considered to be statistically significant when the resulting P-values were <0.05.
Results
Morphological identification and molecular characterization of D. nuttalli
In this study, D. nuttalli ticks were preliminarily morphologically identified. As shown in Figure 2, the dorsal view of the female D. nuttalli is about 5.1 × 3.0 mm with an elliptical scutum of the specimen, which only occupies a small part of the front of the body (Figure 2A). And the genital pore of the female has a wing-like protrusion (Figure 2B). The male D. nuttalli is about 5.8 × 4.0 mm. Its scutum on the back almost covers the entire back of the body and has a lighter enamel color on its back (Figure 2C). In the ventral view of male D. nuttalli, the paraproct is very obvious (Figure 2D). Moreover, the capitulum of the male and female D. nuttalli is rectangular and the overall color is dark brown. The anal groove presents an inverted “U” shape in front of the anus.
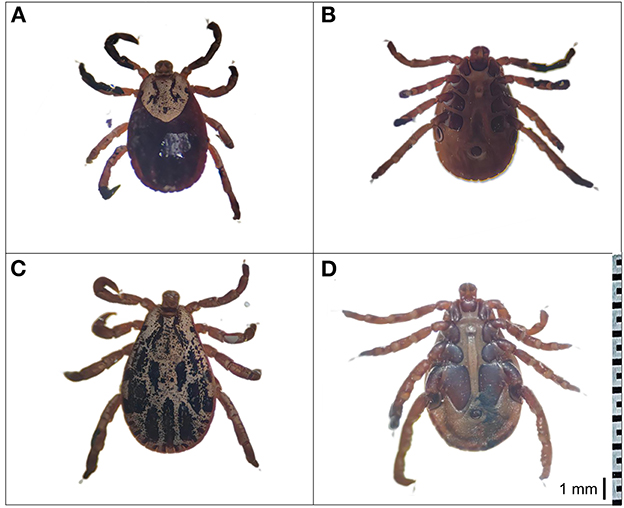
Figure 2. Morphological characterization of D. nuttalli. The dorsal view and ventral view of D. nuttalli. All figures used the same ruler (1 mm). (A) Dorsal view of female. (B) Ventral view of female. (C) Dorsal view of male. (D) Ventral view of male.
Furthermore, the species identify was confirmed by molecular analysis of the PCR assay based on the 12S rRNA, 16S rRNA, COI, and ITS2 genes. The sequencing of positive samples showed that the length of the obtained tick sequences varied from 386 to 1,210 bp (Table 2). The BLASTn analysis of two D. nuttalli 12S rRNA sequences in this study showed identities ranging from 98.7 to 99.5% with reference sequences in China from GenBank (Table 2). The 16S rRNA sequences of D. nuttalli obtained in this study showed 99.8% identity with each other and 98.2–99.0% identity with the sequences from Dermacentor spp. in China. Sequence analysis revealed that the nucleotide sequence of COI in this study shared 99.0 to 99.7% identity with Dermacentor spp. sequences from China and 99.0–99.7% identity with Dermacentor spp. sequences from other countries from GenBank. Moreover, the BLASTn analysis of the ITS2 gene revealed that the three sequences obtained in this study had 98.7 to 99.2% identity with each other, and 97.5–99.2% identity with the sequences of Dermacentor spp. from China. Additionally, the phylogenetic analysis of Dermacentor spp. based on the 12S rRNA, 16S rRNA, COI, and ITS2 genes revealed that tick sequences obtained from this study grouped with the D. nuttalli clades (Figure 3).
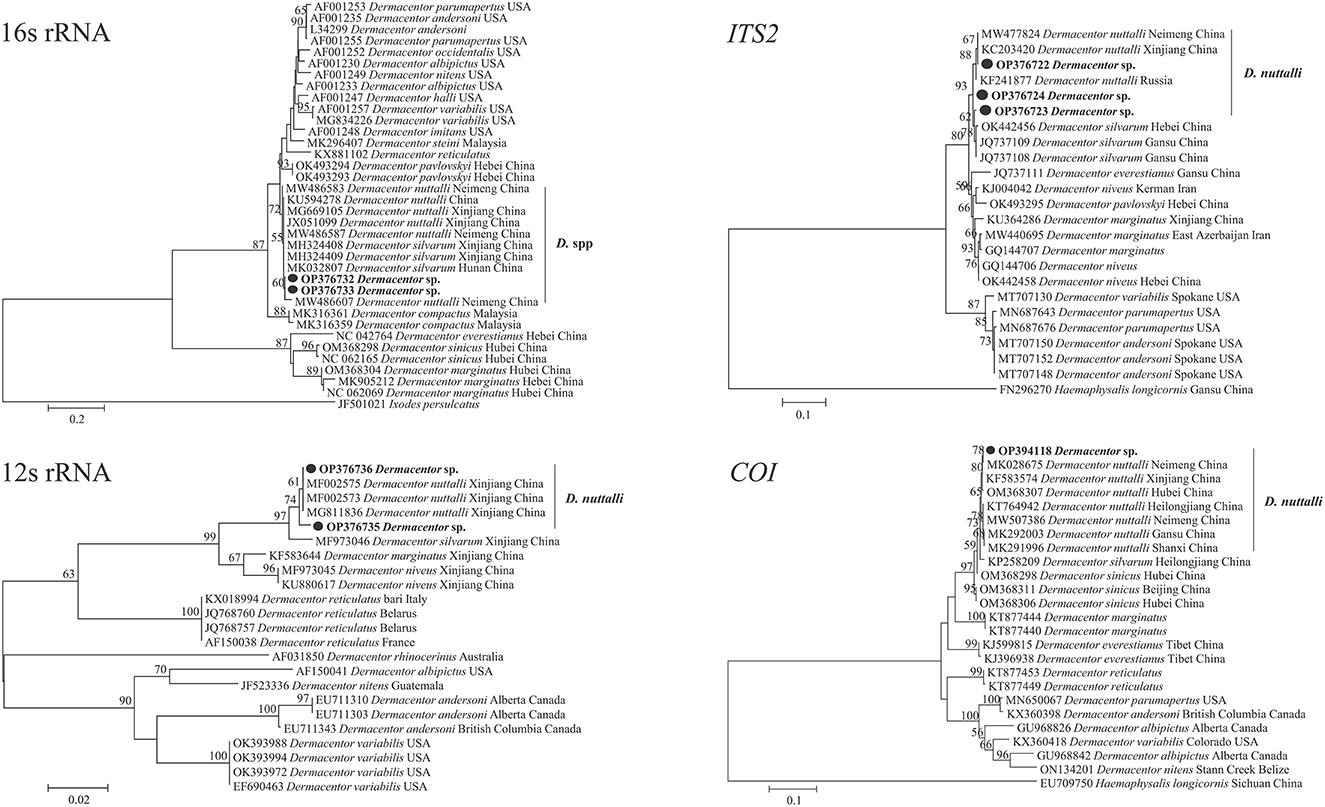
Figure 3. Molecular phylogenetic analysis by the maximum likelihood method based on the 12S rRNA, 16S rRNA, COI, and ITS2 genes of D. nuttalli. The sequences obtained in this study are shown in bold font. The numbers at the nodes represent bootstrap support values.
Laboratory-rearing of D. nuttalli
For laboratory-rearing of D. nuttalli and detection of Rickettsia colonization in first-laboratory generation D. nuttalli, 200 D. nuttalli males and 200 females were randomly chosen to generate next-generation adults by blood-biting in rabbits and rearing in an incubator at 25 ± 2°C and 80% humidity. The results showed that the first laboratory-generation of adults was completed within an average of 86.1 days (Figure 4; Table 3). The average weight of engorged females was 656.0 mg, which was 74.5 times the weight of unfed females (Table 4). No significant difference in average weight was found between field-collected and first-laboratory generation female adults, while the first-generation males produced in the laboratory weighed 2 mg more than the original male adults (Table 4).
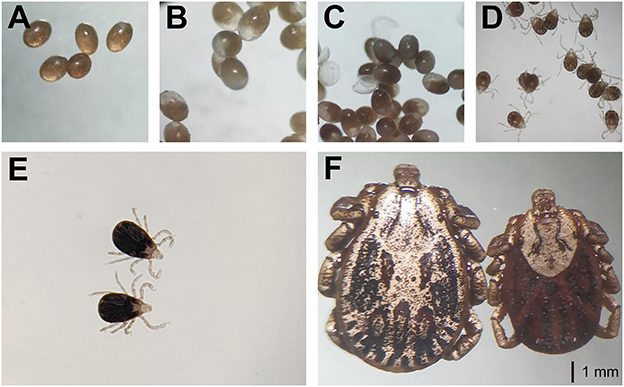
Figure 4. The laboratory-rearing of D. nuttalli. All figures used the same ruler (1 mm). (A) Prophase of eggs. (B) Metaphase of eggs. (C) Anaphase of eggs. (D) Larva. (E) Nymphs. (F) Female and male adults.
Identification of SFG Rickettsia in the midgut and salivary glands in field-collected and first-laboratory generation D. nuttalli males and females
In this study, 100 field-collected D. nuttalli males and 100 females collected were chosen randomly to determine Rickettsia colonization. For first laboratory-generation ticks, 100 males and 116 females were screened by randomly selecting one or two males or females for each female mother tick. SFG Rickettsia in the midgut and salivary glands of the adult D. nuttalli males and females was determined using a PCR assay based on the gltA gene. As shown in Table 5, high colonization rates of Rickettsia were found in both the midgut and the salivary glands of both field-collected D. nuttalli males and females, and no positivity was found in single tissue colonization. However, low rates (4.0–6.0%) of Rickettsia colonization in the midgut or salivary glands of first-laboratory generation D. nuttalli were detected (Table 5). The positivity rates of SFG Rickettsia in the same generation of ticks showed no significant difference depending on either sex and organ.

Table 5. Infection rate (%) of SFG Rickettsia spp. in field-collected and first-laboratory generation adults of D. nuttalli in the QTPA.
All positive samples for the gltA gene were confirmed by PCR of the ompA, ompB and sca4 genes, and the GenBank numbers of these sequences with lengths ranging from 209 to 813 bp are listed in Table 6. BLASTn analysis of the four genes showed that the Rickettsia spp. detected in this study had 98.6 to 100% identities with either R. slovaca or R. raoultii sequences from Dermacentor spp. from China. The phylogenetic analysis of Rickettsia sequences based on the gltA, ompA, ompB and sca4 genes revealed that the sequences obtained from D. nuttalli in this study grouped with the R. slovaca and R. raoultii clades of isolates from Dermacentor spp., Homo sapiens, animals and other tick species from China, Russia, France, Pakistan, the United States, the Netherlands, Turkey, Romania, Austria, and Italy (Figure 5).
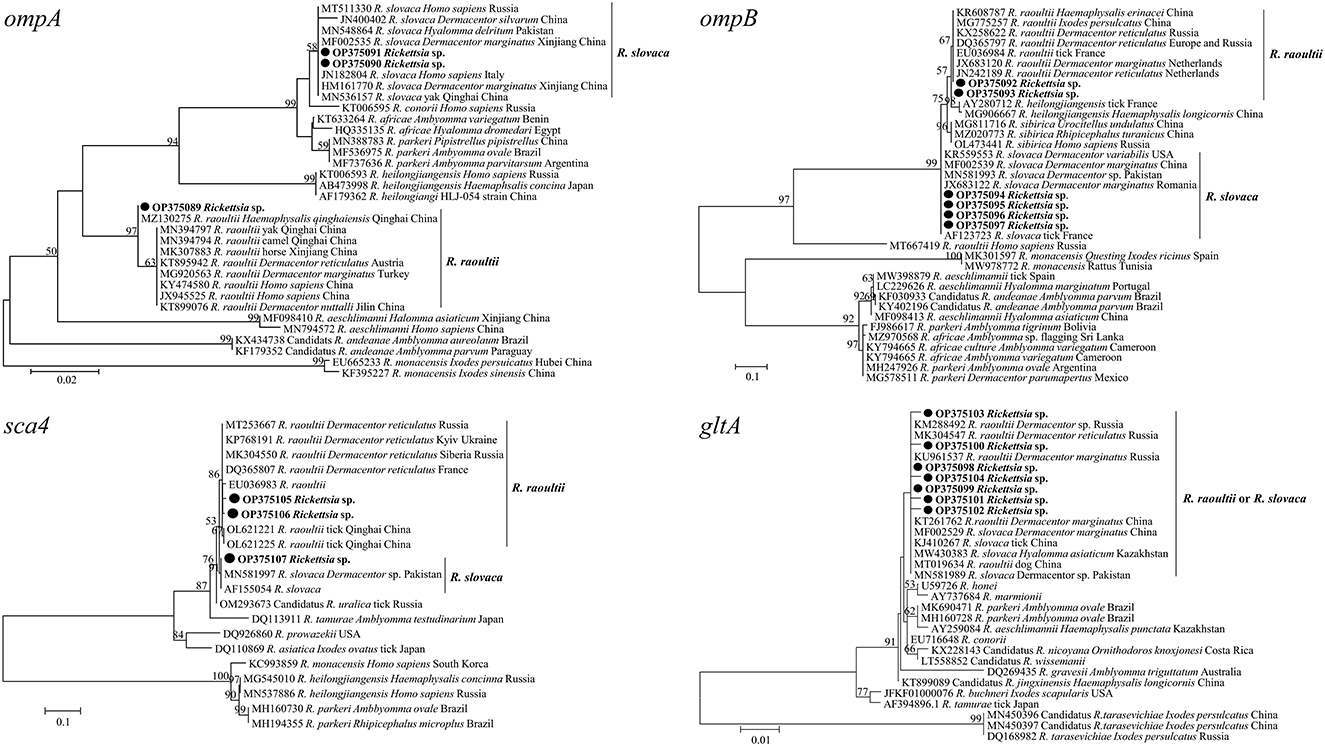
Figure 5. Molecular Phylogenetic analysis by maximum likelihood method based on the gltA, ompA, ompB, and sca4 genes of spotted fever group Rickettsia. The sequences obtained in this study are shown in bold font. The numbers at the nodes represent bootstrap support values.
Discussion
The current sampling sites located in Qinghai Province on the Qinghai-Tibetan Plateau are over 2,500 m above sea level and have a unique natural ecosystem with a cold climate and oxygen deficiency. Some organisms, such as the yak (Bos grunniens) and tick vectors, can survive under the extreme environmental conditions of Qinghai Province. Chen et al. (52) reported that at least 25 species of six tick genera have been recorded in Qinghai Province, and the most common species were Haemaphysalis qinghaiensis, D. nuttalli and D. silvarum (24). In this study, the collected D. nuttalli ticks in this province were molecularly confirmed, and found to have a life cycle with an average of 86.1 days under laboratory conditions. Furthermore, the present study detected two species of SFG Rickettsia that grouped with R. slovaca and R. raoultii in both the salivary glands and midgut of field-collected and first-laboratory generation males and females.
The life-cycle of several tick species including D. everestianus, Ha. qinghaiensis, Hyalomma scupense, Hy. asiaticum and Hy. rufipes under laboratory conditions and D. silvarum, Ha. tibetensis and Ha. concinna under field conditions, has been characterized in China (53–60). These studies showed that the average period of one female tick life-cycle of under laboratory conditions was ranged from 110.2 to 179.0 days, while it ranged from 121.0 to 177.8 days under field conditions (53–60). However, the average periods showed seasonal differences (53–60). D. nuttalli is widely distributed in northern China, including the sampling sites in Qinghai Province in this study (5, 6, 8). Here, an average life cycle of 86.1 days (ranging from 65.0 to 107.0 days) of D. nuttalli under laboratory conditions was found, indicating a shorter length of time than previously reported (53–60). This may be because the ticks were collected in March and April which is the blood-feeding period in the natural cycle, and ticks may retain wild habits during the first cycle in the laboratory. These results suggest the specificity of the life cycle of D. nuttalli compared with other hard ticks, especially in terms of the duration and the morphology of different life stages.
The ubiquity and high proportions of pathogens in the salivary glands and midgut of ticks collected in the field were determined by the species of pathogens and the presence of infectious host blood. The present study detected SFG Rickettsia in both the salivary glands and midgut of males and females, with high infection rates in the original D. nuttalli adults. This suggests that this tick-borne pathogen could be well adapted to the different environmental conditions of both the salivary glands and the midgut in D. nuttalli males and females, which is consistent with the finding of previous studies that SFG Rickettsia was most frequent in D. nuttalli ticks in Qinghai Province, northwestern China (24). Subsequently, the identification of Rickettsia was performed in the first-laboratory generation adults of D. nuttalli. However, the result in contrast with that in the original ticks, in which SFG Rickettsia was detected in either the salivary glands or the midgut in males and females, with low positivity rates. This indicates that the acquisition, maintenance, colonization, and transmission of SFG Rickettsia by D. nuttalli ticks cannot be separated from the blood contributions of an effective host. Moreover, although our study simulated the natural environment of ticks, it is possible that subtle environmental changes could influence the life-cycle of ticks, as well as the colonization and exchange of pathogens in tick salivary glands or midguts (53–60). Furthermore, the positivity rates of SFG Rickettsia in the same generation of ticks showed no significant difference between sexes or organs, suggesting that this pathogen strongly colonizes in both the salivary glands and the midgut in D. nuttalli males and females.
Furthermore, our study provides new evidence of DNA-based detection of SFG Rickettsia pathogens in D. nuttalli ticks in the sampling areas. The sequencing and phylogenetic analysis of Rickettsia spp. based on the gltA, ompA, ompB and sca4 genes revealed that the sequences obtained could be classified into two groups, representing two different rickettsial species, R. slovaca and R. raoultii. Previous studies reported that SFG rickettsiae including R. aeschlimannii, R. conorii, R. sibirica, R. massiliae, Candidatus R. barbariae, R. raoultii, and R. slovaca, were detected in field-collected Ha. qinghaiensis, D. marginatus, D. abaensis, D. silvarum, Ixodes crenulatus, Rhipicephalus turanicus, and D. nuttalli in northwestern China (23, 24). Consistent with our findings in this study, it is common to detect R. raoultii and R. slovaca in D. nuttalli ticks in previous studies (8, 23, 24). The two Rickettsia species were identified as pathogenic factors of human rickettsiosis in tick vectors and humans (39, 40, 61), and importantly, R. raoultii has been detected in humans in China (39, 40). These results suggest that humans and animals are susceptible to the pathogens due to exposure to D. nuttalli tick vectors in the sampling areas.
In conclusion, this study is the first to characterize the life cycle of collected D. nuttalli in the field from the Qinghai-Tibetan Plateau under laboratory conditions, and two species of SFG Rickettsia were identified in the midgut and salivary glands of males and females of both field-collected and first-laboratory generation adults. However, there are differences between laboratory conditions and natural conditions that impact tick survival and may influence the life cycle of laboratory-reared ticks. Moreover, the seasonal diapause of ticks must be considered because it may affect their life cycle and pathogen colonization under laboratory conditions. Our study provides new insights into the pathogen colonization of ticks on the Qinghai-Tibetan Plateau, and the relationships among hosts, ticks and pathogens.
Data availability statement
The datasets presented in this study can be found in online repositories. The names of the repository/repositories and accession number(s) can be found in the article/supplementary material.
Author contributions
YS: conceptualization, data curation, formal analysis, funding acquisition, resources, writing-original draft, and writing-review and editing. HM and JA: data curation, formal analysis, and investigation. MK: resources and writing-review and editing. JL: conceptualization, writing-original draft, and writing-review and editing. All authors contributed to the article and approved the submitted version.
Funding
This study was supported by the Natural Science Foundation of Qinghai Province of China (Grant Number 2022-ZJ-940Q).
Conflict of interest
The authors declare that the research was conducted in the absence of any commercial or financial relationships that could be construed as a potential conflict of interest.
Publisher's note
All claims expressed in this article are solely those of the authors and do not necessarily represent those of their affiliated organizations, or those of the publisher, the editors and the reviewers. Any product that may be evaluated in this article, or claim that may be made by its manufacturer, is not guaranteed or endorsed by the publisher.
References
1. Madison-Antenucci S, Kramer LD, Gebhardt LL, Kauffman E. Emerging tick-borne diseases. Clin Microbiol Rev. (2020) 33:e00083–18. doi: 10.1128/CMR.00083-18
2. Zhao GP, Wang YX, Fan ZW Ji Y, Liu MJ, Zhang WH, et al. Mapping ticks and tick-borne pathogens in China. Nat Commun. (2021) 12:1075. doi: 10.1038/s41467-021-21375-1
3. Kulakova NV, Khasnatinov MA, Sidorova EA, Adel'shin RV, Belikov SI. Molecular identification and phylogeny of Dermacentor nuttalli (Acari: Ixodidae). Parasitol Res. (2014) 113:1787–93. doi: 10.1007/s00436-014-3824-x
4. Wang F, Wang D, Guo G, Hu Y, Wei J, Liu J. Species delimitation of the Dermacentor ticks based on phylogenetic clustering and niche modeling. PeerJ. (2019) 7:e6911. doi: 10.7717/peerj.6911
5. Wang Y, Liu Z, Yang J, Chen Z, Liu J, Li Y, et al. Rickettsia raoultii-like bacteria in Dermacentor spp. ticks, Tibet, China. Emerg Infect Dis. (2012) 18:1532–4. doi: 10.3201/eid1809.120644
6. Liu S, Yuan C, Cui YF Li BX, Wu LJ, Liu Y. Investigation of Borrelia spp. in ticks (Acari: Ixodidae) at the border crossings between China and Russia in Heilongjiang province. China Asian Pac J Trop Med. (2012) 5:459–64. doi: 10.1016/S1995-7645(12)60078-9
7. Igolkina Y, Rar V, Vysochina N, Ivanov L, Tikunov A, Pukhovskaya N, et al. Genetic variability of Rickettsia spp. in dermacentor and haemaphysalis ticks from the Russian far east ticks. Tick Borne Dis. (2018) 9:1594–603. doi: 10.1016/j.ttbdis.2018.07.015
8. Li Y, Wen X, Li M, Moumouni PFA, Galon EM, Guo Q, et al. Molecular detection of tick-borne pathogens harbored by ticks collected from livestock in the Xinjiang Uygur Autonomous Region, China. Ticks Tick Borne Dis. (2020) 11:101478. doi: 10.1016/j.ttbdis.2020.101478
9. von Fricken ME, Qurollo BA, Boldbaatar B, Wang YW, Jiang RR, Lkhagvatseren S, et al. Genetic diversity of Anaplasma and Ehrlichia bacteria found in Dermacentor and Ixodes ticks in Mongolia. Ticks Tick Borne Dis. (2020) 11:101316. doi: 10.1016/j.ttbdis.2019.101316
10. Yaxue Z, Hongtao J, Qiuyue W, Zhixin F, Hongwei G, Pengpeng L, et al. Molecular detection of Anaplasma phagocytophilum in Ixodid ticks in Hebei province, China. Vector Borne Zoonotic Dis. (2011) 11:1323–27. doi: 10.1089/vbz.2010.0253
11. Khasnatinov MA, Liapunov AV, Manzarova EL, Kulakova NV, Petrova IV, Danchinova GA. The diversity and prevalence of hard ticks attacking human hosts in Eastern Siberia (Russian Federation) with first description of invasion of non-endemic tick species. Parasitol Res. (2016) 115:501–10. doi: 10.1007/s00436-015-4766-7
12. Pukhovskaya NM, Morozova OV, Vysochina NP, Belozerova NB, Bakhmetyeva SV, Zdanovskaya NI, et al. Tick-borne encephalitis virus in arthropod vectors in the Far East of Russia. Ticks Tick Borne Dis. (2018) 9:824–33. doi: 10.1016/j.ttbdis.2018.01.020
13. Kholodilov I, Belova O, Burenkova L, Korotkov Y, Romanova L, Morozova L, et al. Ixodid ticks and tick-borne encephalitis virus prevalence in the South Asian part of Russia (Republic of Tuva). Ticks Tick Borne Dis. (2019) 10:959–69. doi: 10.1016/j.ttbdis.2019.04.019
14. Cerný J, Buyannemekh B, Needham T, Gankhuyag G, Oyuntsetseg D. Hard ticks and tick-borne pathogens in Mongolia-A review. Ticks Tick Borne Dis. (2019) 10:101268. doi: 10.1016/j.ttbdis.2019.101268
15. Ni J, Lin H, Xu X, Ren Q, Aizezi M, Luo J, et al. Coxiella burnetii is widespread in ticks (Ixodidae) in the Xinjiang areas of China. BMC Vet Res. (2020) 16:317. doi: 10.1186/s12917-020-02538-6
16. Jiao J, Lu Z, Yu Y, Ou Y, Fu M, Zhao Y, et al. Identification of tick-borne pathogens by metagenomic next-generation sequencing in Dermacentor nuttalli and Ixodes persulcatus in Inner Mongolia, China. Parasit Vectors. (2021) 14:287. doi: 10.1186/s13071-021-04740-3
17. Fan MY, Wang JG, Jiang YX, Zong DG, Lenz B, Walker DH. Isolation of a spotted fever group rickettsia from a patient and related ecologic investigations in Xinjiang Uygur Autonomous Region of China. J Clin Microbiol. (1987) 25:628–32. doi: 10.1128/jcm.25.4.628-632.1987
18. Fan MY, Walker DH, Liu QH, Han L, Bai HC, Zhang JK, et al. Rickettsial and serologic evidence for prevalent spotted fever rickettsiosis in inner Mongolia. Am J Trop Med Hyg. (1987) 36:615–20. doi: 10.4269/ajtmh.1987.36.615
19. Zou Y, Wang Q, Fu Z, Liu P, Jin H, Yang H, et al. Detection of spotted fever group Rickettsia in Haemaphysalis longicornis from Hebei province, China. J Parasitol. (2011) 97:960–2. doi: 10.1645/GE-2751.1
20. Kang YJ, Diao XN, Zhao GY, Chen MH, Xiong Y, Shi M, et al. Extensive diversity of Rickettsiales bacteria in two species of ticks from China and the evolution of the Rickettsiales. BMC Evol Biol. (2014) 14:167. doi: 10.1186/s12862-014-0167-2
21. Liu H, Li Q, Zhang X, Li Z, Wang Z, Song M, et al. Characterization of rickettsiae in ticks in northeastern China. Parasit Vectors. (2016) 9:498. doi: 10.1186/s13071-016-1764-2
22. Yin X, Guo S, Ding C, Cao M, Kawabata H, Sato K, et al. Spotted Fever Group Rickettsiae in Inner Mongolia, China, 2015-2016. Emerg Infect Dis. (2018) 24:2105–7. doi: 10.3201/eid2411.162094
23. Song S, Chen C, Yang M, Zhao S, Wang B, Hornok S, et al. Diversity of Rickettsia species in border regions of northwestern China. Parasit Vectors. (2018) 11:634. doi: 10.1186/s13071-018-3233-6
24. Han R, Yang J, Niu Q, Liu Z, Chen Z, Kan W, et al. Molecular prevalence of spotted fever group rickettsiae in ticks from Qinghai province, northwestern China. Infect Genet Evol. (2018) 57:1–7. doi: 10.1016/j.meegid.2017.10.025
25. Guo WP, Wang YH, Lu Q, Xu G, Luo Y, Ni X, et al. Molecular detection of spotted fever group rickettsiae in hard ticks, northern China. Transbound Emerg Dis. (2019) 66:1587–96. doi: 10.1111/tbed.13184
26. Liu H, Liang X, Wang H, Sun X, Bai X, Hu B, et al. Molecular evidence of the spotted fever group Rickettsiae in ticks from Yunnan province, southwest China. Exp Appl Acarol. (2020) 80:339–48. doi: 10.1007/s10493-020-00467-5
27. Shao JW, Zhang XL Li WJ, Huang HL, Yan J. Distribution and molecular characterization of rickettsiae in ticks in Harbin area of northeastern China. PLoS Negl Trop Dis. (2020) 14:e0008342. doi: 10.1371/journal.pntd.0008342
28. Wang Q, Pan YS, Jiang BG, Ye RZ, Chang QC, Shao HZ, et al. Prevalence of multiple tick-borne pathogens in various tick vectors in northeastern China. Vector Borne Zoonotic Dis. (2021) 21:162–71. doi: 10.1089/vbz.2020.2712
29. Gui Z, Cai H, Qi DD, Zhang S, Fu SY Yu JF, et al. Identification and genetic diversity analysis of Rickettsia in Dermacentor nuttalli within inner Mongolia, China. Parasit Vectors. (2022) 15:286. doi: 10.1186/s13071-022-05387-4
30. Parola P, Raoult D. Ticks and tickborne bacterial diseases in humans: an emerging infectious threat. Clin Infect Dis. (2001) 32:897–928. doi: 10.1086/319347
31. Parola P, Paddock CD, Socolovschi C, Labruna MB, Mediannikov O, Kernif T, et al. Update on tick-borne rickettsioses around the world: a geographic approach. Clin Microbiol Rev. (2013) 26:657–702. doi: 10.1128/CMR.00032-13
32. Robinson MT, Satjanadumrong J, Hughes T, Stenos J, Blacksell SD. Diagnosis of spotted fever group Rickettsia infections: the Asian perspective. Epidemiol Infect. (2019) 147:e286. doi: 10.1017/S0950268819001390
33. Zhang J, Lu G, Kelly P, Zhang Z, Wei L, Yu D, et al. First report of Rickettsia felis in China. BMC Infect Dis. (2014) 14:682. doi: 10.1186/s12879-014-0682-1
34. Sun J, Lin J, Gong Z, Chang Y, Ye X, Gu S, et al. Detection of spotted fever group Rickettsiae in ticks from Zhejiang province, China. Exp Appl Acarol. (2015) 65:403–11. doi: 10.1007/s10493-015-9880-9
35. Wei QQ, Guo LP, Wang AD, Mu LM, Zhang K, Chen CF, et al. The first detection of Rickettsia aeschlimannii and Rickettsia massiliae in Rhipicephalus turanicus ticks, in northwest China. Parasit Vectors. (2015) 8:631. doi: 10.1186/s13071-015-1242-2
36. Zhang J, John Kelly P, Lu G, Cruz-Martinez L, Wang C. Rickettsia in mosquitoes, Yangzhou, China. Emerg Microbes Infect. (2016) 5:e108. doi: 10.1038/emi.2016.107
37. Guo WP, Huang B, Zhao Q, Xu G, Liu B, Wang YH, et al. Human-pathogenic Anaplasma spp, and Rickettsia spp in animals in Xi'an, China. PLoS Negl Trop Dis. (2018) 12:e0006916. doi: 10.1371/journal.pntd.0006916
38. Zhao S, Yang M, Jiang M, Yan B, Zhao S, Yuan W, et al. Rickettsia raoultii and Rickettsia sibirica in ticks from the long-tailed ground squirrel near the China-Kazakhstan border. Exp Appl Acarol. (2019) 77:425–33. doi: 10.1007/s10493-019-00349-5
39. Jia N, Zheng YC, Ma L, Huo QB Ni XB, Jiang BG, et al. Human infections with Rickettsia raoultii, China. Emerg Infect Dis. (2014) 20:866–8. doi: 10.3201/eid2005.130995
40. Li H, Zhang PH, Huang Y, Du J, Cui N, Yang ZD, et al. Isolation and identification of Rickettsia raoultii in human cases: a surveillance study in 3 medical centers in China. Clin Infect Dis. (2018) 66:1109–15. doi: 10.1093/cid/cix917
41. Duan C, Tong Y, Huang Y, Wang X, Xiong X, Wen B. Complete genome sequence of Rickettsia heilongjiangensis, an emerging tick-transmitted human pathogen. J Bacteriol. (2011) 193:5564–5. doi: 10.1128/JB.05852-11
42. Lejal E, Moutailler S, Šimo L, Vayssier-Taussat M, Pollet T. Tick-borne pathogen detection in midgut and salivary glands of adult Ixodes ricinus. Parasit Vectors. (2019) 12:152. doi: 10.1186/s13071-019-3418-7
43. Santos AS, Bacellar F, Santos-Silva M, Formosinho P, Grácio AJ, Franca S. Ultrastructural study of the infection process of Rickettsia conorii in the salivary glands of the vector tick Rhipicephalus sanguineus. Vector Borne Zoonotic Dis. (2002) 2:165–77. doi: 10.1089/15303660260613738
44. Popov VL, Korenberg EI, Nefedova VV, Han VC, Wen JW, Kovalevskii YV, et al. Ultrastructural evidence of the ehrlichial developmental cycle in naturally infected Ixodes persulcatus ticks in the course of coinfection with Rickettsia, Borrelia, and a flavivirus. Vector Borne Zoonotic Dis. (2007) 7:699–716. doi: 10.1089/vbz.2007.0148
45. Narasimhan S, Rajeevan N, Liu L, Zhao YO, Heisig J, Pan J, et al. Gut microbiota of the tick vector Ixodes scapularis modulate colonization of the Lyme disease spirochete. Cell Host Microbe. (2014) 15:58–71. doi: 10.1016/j.chom.2013.12.001
46. Teng KF, Jiang ZJ. Economic insect fauna of China. Fasc 39 Acari: Ixodidae. Science Press, Beijing. (1991)
47. Guo H, Adjou Moumouni PF, Thekisoe O, Gao Y, Liu M, Li J, et al. Genetic characterization of tick-borne pathogens in ticks infesting cattle and sheep from three south African provinces. Ticks Tick Borne Dis. (2019) 10:875–82. doi: 10.1016/j.ttbdis.2019.04.008
48. Kidd L, Maggi R, Diniz PP, Hegarty B, Tucker M, Breitschwerdt E. Evaluation of conventional and real-time PCR assays for detection and differentiation of spotted fever group Rickettsia in dog blood. Vet Microbiol. (2008) 129:294–303. doi: 10.1016/j.vetmic.2007.11.035
49. Hazihan W, Dong Z, Guo L, Rizabek K, Askar D, Gulzhan K, et al. Molecular detection of spotted fever group rickettsiae in ticks parasitizing pet dogs in Shihezi city, northwestern China. Exp Appl Acarol. (2019) 77:73–81. doi: 10.1007/s10493-018-00337-1
50. Zheng W, Liu M, Moumouni PF, Liu X, Efstratiou A, Liu Z, et al. First molecular detection of tick-borne pathogens in dogs from Jiangxi, China. J Vet Med Sci. (2017) 79:248–54. doi: 10.1292/jvms.16-0484
51. Kumar S, Stecher G. Tamura K. MEGA7: molecular evolutionary genetics analysis version 70 for bigger datasets. Mol Biol Evol. (2016) 33:1870–4. doi: 10.1093/molbev/msw054
52. Chen Z, Yang X, Bu F, Yang X, Yang X, Liu J. Ticks (acari: ixodoidea: argasidae, ixodidae) of China. Exp Appl Acarol. (2010) 51:393–404. doi: 10.1007/s10493-010-9335-2
53. Chen Z, Yu Z, Yang X, Zheng H, Liu J. The life cycle of Hyalomma asiaticum kozlovi Olenev, 1931 (Acari: Ixodidae) under laboratory conditions. Vet Parasitol. (2009) 160:134–7. doi: 10.1016/j.vetpar.2008.10.028
54. Yu Z, Zheng H, Chen Z, Zheng B, Ma H, Liu J. The life cycle and biological characteristics of Dermacentor silvarum Olenev (Acari: Ixodidae) under field conditions. Vet Parasitol. (2010) 168:323–8. doi: 10.1016/j.vetpar.2009.11.010
55. Jin S, Wang T, Li T, Liu M, Jia Q, Yang X, et al. Life cycle of Dermacentor everestianus hirst, 1926 (Acari: Ixodidae) under laboratory conditions. Korean J Parasitol. (2017) 55:193–6. doi: 10.3347/kjp.2017.55.2.193
56. Chen Z, Li Y, Liu Z, Yang J, Yin H. The life cycle of Hyalomma rufipes (Acari: Ixodidae) under laboratory conditions. Exp Appl Acarol. (2012) 56:85–92. doi: 10.1007/s10493-011-9490-0
57. Meng H, Xu S, Yu Z, Liu Z, Liu J, Yang X, et al. The life cycle and occurrence of Haemaphysalis concinna (Acari: Ixodidae) under field conditions. Ticks Tick Borne Dis. (2014) 5:887–91. doi: 10.1016/j.ttbdis.2014.07.007
58. Liu M, Li T, Yu ZJ, Gao XH, Zuo CW, Wang RR, et al. Characterization of the life cycle of the tick Haemaphysalis tibetensis under field conditions in Qinghai-Tibet plateau. Exp Appl Acarol. (2016) 69:107–15. doi: 10.1007/s10493-016-0020-y
59. Ma M, Guan G, Chen Z, Liu Z, Liu A, Gou H, et al. The life cycle of Haemaphysalis qinghaiensis (Acari: Ixodidae) ticks under laboratory conditions. Exp Appl Acarol. (2013) 59:493–500. doi: 10.1007/s10493-012-9617-y
60. Yang X, Zhao Y, Chuai X, Cao Q, Meng H, Liu J, et al. The life cycle of Hyalomma scupense (Acari: Ixodidae) under laboratory conditions. Ticks Tick Borne Dis. (2022) 13:102019. doi: 10.1016/j.ttbdis.2022.102019
Keywords: Dermacentor nuttalli, life cycle, Rickettsia, molecular identification, Qinghai-Tibetan Plateau
Citation: Ma H, Ai J, Kang M, Li J and Sun Y (2023) The life cycle of Dermacentor nuttalli from the Qinghai-Tibetan Plateau under laboratory conditions and detection of spotted fever group Rickettsia spp.. Front. Vet. Sci. 10:1126266. doi: 10.3389/fvets.2023.1126266
Received: 17 December 2022; Accepted: 08 February 2023;
Published: 24 February 2023.
Edited by:
Calin Mircea Gherman, University of Agricultural Sciences and Veterinary Medicine of Cluj-Napoca, RomaniaReviewed by:
Abdulaziz Alouffi, King Abdulaziz City for Science and Technology, Saudi ArabiaRemil Galay, University of the Philippines Los Baños, Philippines
Copyright © 2023 Ma, Ai, Kang, Li and Sun. This is an open-access article distributed under the terms of the Creative Commons Attribution License (CC BY). The use, distribution or reproduction in other forums is permitted, provided the original author(s) and the copyright owner(s) are credited and that the original publication in this journal is cited, in accordance with accepted academic practice. No use, distribution or reproduction is permitted which does not comply with these terms.
*Correspondence: Yali Sun, yalisun@qhu.edu.cn