- 1Secció de Parasitologia, Departament de Biologia, Sanitat i Medi Ambient, Facultat de Farmàcia i Ciències de l'Alimentació, Universitat de Barcelona, Barcelona, Spain
- 2Section of Parasitology, Department of Public Health and Infectious Diseases, Sapienza-University of Rome, Rome, Italy
- 3Department of Environmental Sciences, Faculty of Sciences, University of Girona, Girona, Spain
- 4Department of Ecological and Biological Sciences, Tuscia University, Viterbo, Italy
Sibling species of the Contracaecum rudolphii (s.l.) complex are habitual endoparasites of cormorants of the Phalacrocoracidae family, worldwide. In Europe, the two species, C. rudolphii sp. A and C. rudolphii sp. B, have been identified. However, information regarding the occurrence and distribution of these anisakids in cormorants from Spain is scarce. In the present study, 20 specimens of the European Shag, Ph. aristotelis desmarestii, from the western Mediterranean Spanish marine coast were parasitologically analyzed for the presence of nematodes. All hosts were found parasitized with Contracaecum specimens (n = 1,517). A representative subsample was genetically identified as C. rudolphii sp. A by sequence analysis of the mtDNA cox2 gene and the ITS1 and ITS2 regions of the rDNA. This represents the first report of C. rudolphii sp. A from the Spanish Mediterranean waters. Population genetic analysis was performed including other C. rudolphii sp. A specimens from the west Sardinian and the Tyrrhenian Sea. At the intraspecific level, a significant genetic differentiation (Fst ≈ 0.08, p < 0.00001) between the metapopulation from the Spanish Mediterranean coast and that from the Sardinian waters was observed; whereas, no differentiation was found between metapopulations of the parasite from the Spanish and the Tyrrhenian Italian coast. The findings highly support the hypothesis of the adaptation of the life cycle of C. rudolphii sp. A in brackish and marine ecosystems. Furthermore, the results on the population genetics of C. rudolphii sp. A suggest the possible role of the migration routes of wintering populations of cormorants in the Mediterranean Sea in influencing the parasite genetic structure.
1. Introduction
The genus Contracaecum Raillet and Henry, 1912 includes species maturing in pinnipeds and waterbirds worldwide, representing the most diverse group of the family Anisakidae (1, 2). Considering only the species of the genus maturing in fish-eating birds, 19 species have been so far described as infecting cormorants and pelicans (1, 3–10). Moreover, several morphospecies of the genus have been demonstrated to include sibling species (1, 2, 8, 11–13).
Among them, the Contracaecum rudolphii (s.l.) species complex has its most habitual definitive hosts in cormorants belonging to the genus Phalacrocorax (Family Phalacrocoracidae) (4, 7, 8, 12, 14, 15). The two cryptic species of the complex parasitizing cormorants belonging to the Family Phalacrocoracidae identified in Europe are C. rudolphii sp. A and C. rudolphii sp. B (7, 15–17). They are mainly parasites in the Great Cormorant, Ph. carbo sinensis. Regarding other sibling species so far included in the C. rudolphii (s.l.) complex, C. rudolphii sp. C has been detected in Ph. auritus from Florida (3). In Australia, the two cryptic species, i.e., C. rudolphii sp. D and C. rudolphii sp. E, have been found as adult parasites in Ph. carbo and Ph. varius (8). Moreover, another species indicated as C. rudolphii sp. F has been identified as parasitizing the Great Pelican, Pelecanus occidentalis, from the Gulf of Mexico (4). Other Contracaecum species identified in cormorants are as follows: C. microcephalum detected in Ph. melanoleucos and Ph. pygmaeus from Australia and Montenegro, respectively (3, 9); C. chubutensis described in Ph. atriceps from the north Patagonian coast (5); C. australe discovered in Ph. brasilianus from Chile and Argentina (18); and C. jorgei observed in Nannopterum brasilianus from Argentina (10).
The European Shag, Phalacrocorax aristotelis, is a cormorant species commonly found along European marine coasts, mainly observed on rocky seasides and islands. Its nesting habitat in Spain includes a wide range of inaccessible sites on the coast, such as cavities, fissures, and cliff ledges. It is generally sedentary, being the individuals faithful to their first breeding area (19, 20). It comprises three subspecies: Ph. aristotelis aristotelis, which inhabits the north-east Atlantic coast, from Iceland to the Iberian Peninsula, including Norway and the Kola Peninsula in Russia; Ph. aristotelis riggenbachi, which breeds in the Atlantic coast of Morocco; and finally, Ph. aristotelis desmarestii, which is endemic of the Mediterranean and the Black Sea (21). Its diet includes a wide range of fish species, mainly demersal and/or benthic, and could be influenced by their habitat characteristics or the stage of their breeding cycle (22). Studies regarding nematodes' presence in the European Shag are scarce. In Spain, a previous analysis (23) reported the presence of C. rudolphii sp. A and C. septentrionale in Ph. a. aristotelis hosts from the north-west Atlantic coast (Galician coast). In the Mediterranean Sea, C. rudolphii sp. A has been identified in Ph. aristotelis from the east coast of Sardinia (24), while C. septentrionale has been reported in the same hosts from Iceland (25, 26).
The aim of the present study was to (1) identify genetically nematodes of the genus Contracaecum parasitizing the European Shag, Ph. aristotelis desmarestii from the north-western Mediterranean Sea (coast of Spain); and (2) investigate the population genetic structure of the parasite species in the western Mediterranean Sea areas.
2. Materials and methods
2.1. Sampling and parasite collection
A parasitological survey was performed on 20 individuals of Ph. aristotelis desmarestii collected during the years 2019–2021 from the Catalan-Spanish waters, in the north-western Mediterranean Sea. The collecting sites of the cormorants are grouped in three different areas, corresponding to the northern (Costa Brava), the central (Maresme coast), and the southern (Costa Daurada) regions of the Catalan coast, as shown in Figure 1. Cormorants were found dead and subsequently frozen until their parasitological analysis. Stomach dissection was carried out at the laboratory for the presence of larval and/or adult nematodes. All the recovered specimens were washed in saline solution, morphologically referred following Berland (27) and Hartwich (14) criteria, and subsequently fixed in 70% ethanol.
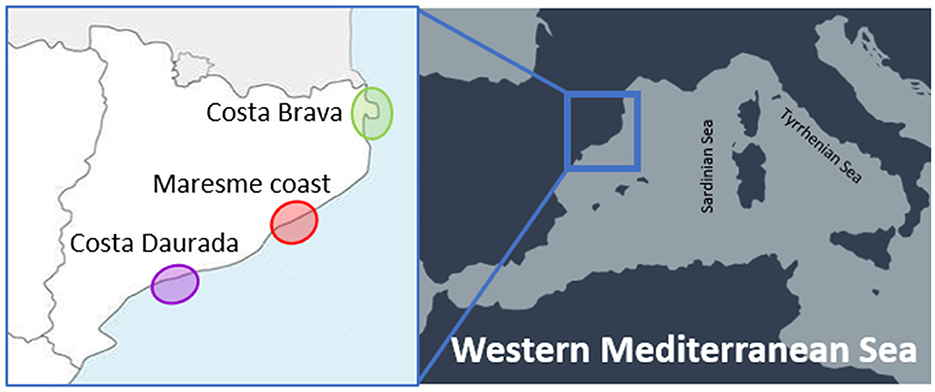
Figure 1. Sampling localities of cormorants from the western Mediterranean Spanish coast (Catalan region).
2.2. Genetic-molecular analysis
Identification at the species level of the recovered nematodes was performed on a subsample of 75 specimens of Contracaecum, 28 from the Costa Brava, 26 from the Costa Daurada, and 21 from the Maresme coast. A minimum of at least two specimens from each cormorant individual was selected for DNA extraction, which was performed using the Quick-DNA Midiprep Plus Kit (Zymo Research, Irvine, USA). Direct sequence analysis of the two genes, i.e., the mtDNA cox2 and the ITS region of the rDNA, was carried out on each nematode specimen. The mitochondrial cox2 gene was amplified using 211F and 210R primers, with a hybridization temperature of 46°C (13, 17). ITS region of the rDNA was amplified using NC5 and NC2 primers at 55°C (28). For both regions, the PCR products were sequenced by Bio-Fab Research (Roma, Italy) using the same primers.
2.3. Sequences analysis
Sequences were aligned using the Clustal X software and adjusted manually (29). Phylogenetic analysis of mtDNA cox2 and ITS rDNA regions of the present study, along with other Contracaecum species previously deposited in GenBank, was carried out by Bayesian analysis (BI) using MrBayes software (30). The sequences used for the analysis are reported in Table 1. Because the entire ITS sequence was not available in GenBank, ITS1 and ITS2 sequences were used to carry out the concatenated sequence analysis using Sequence Matrix software (36). The best-fit substitution models were determined by JModeltest software using the Akaike Information Criterion (AIC) (37). The Bayesian posterior probability analysis was carried out with 1,000,000 generations and a subsampling frequency of 100. Posterior probabilities were estimated and used to assess support for each branch, considering a well-supported value of 0.90.
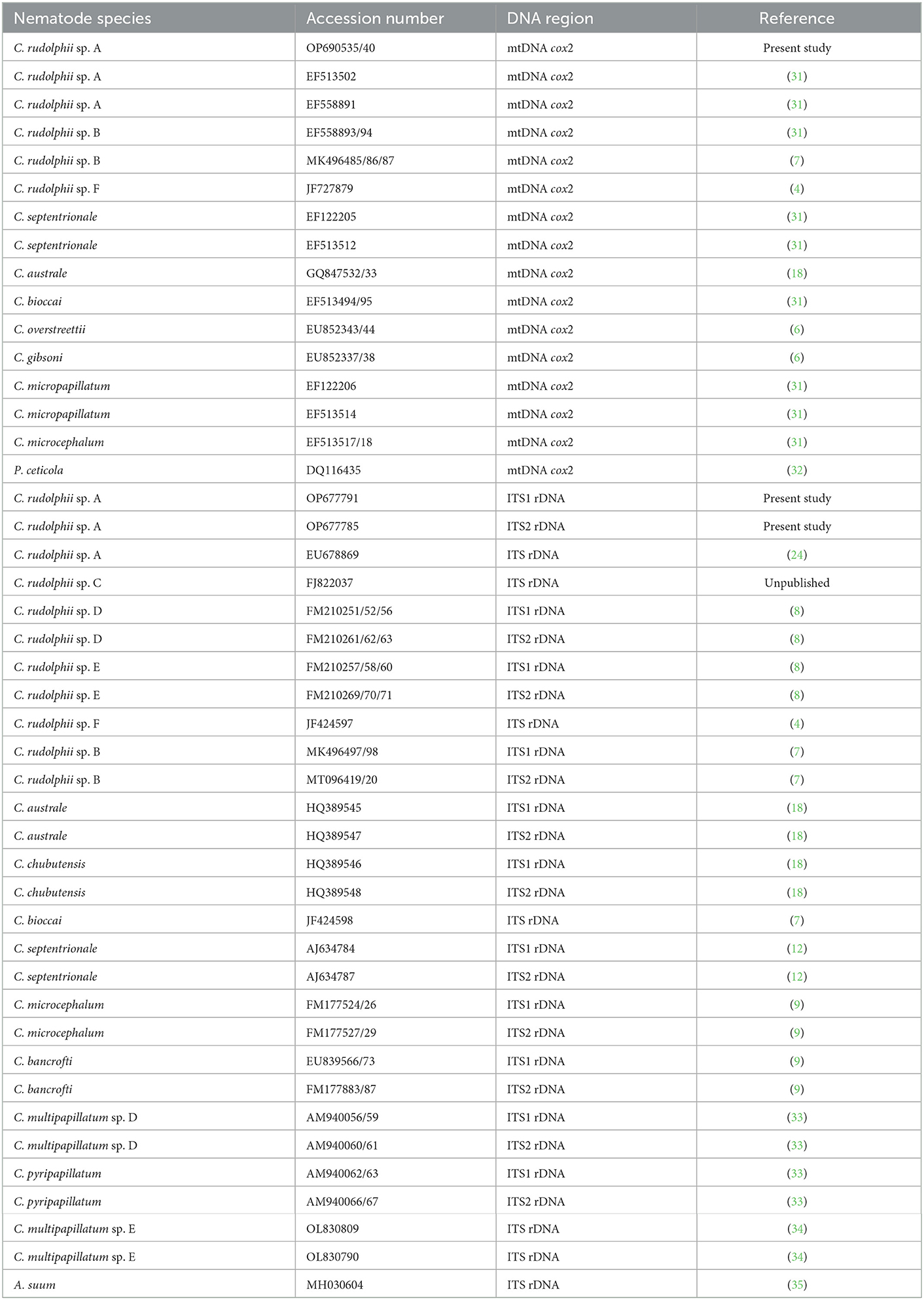
Table 1. List of mtDNA cox2 and ITS rDNA sequences used for the Bayesian inference phylogenetic analysis.
2.4. Intraspecific genetic diversity
Intraspecific genetic diversity of C. rudolphii sp. A population from the Spanish Mediterranean coast identified in the present study, inferred from the mtDNA cox2 sequences analysis, was compared with other conspecific populations previously analyzed by the same genetic markers (7) from cormorants (Ph. carbo sinensis) of several marine localities of the Tyrrhenian coast of Italy. In particular, they were from the Orbetello lagoon, the estuary of the Fiora and Marta rivers, and the Tarquinia salterns. In addition, sequences of the mtDNA cox2 of the same parasite species, available in GenBank, reported from Ph. c. sinensis of the western Sardinian coast [see Amor et al. (38)] were also considered. Population genetic analysis was performed by using DnaSP V5.10.01 (39), estimating the genetic diversity on the following standard statistical parameters: number of haplotypes (Nh), number of private haplotypes (Nuh) nucleotide diversity (π), haplotype diversity (Hd), average number of differences (K), and number of polymorphic sites (S). Spatial analysis of molecular variance (AMOVA) and analysis of the estimation of Fst values were conducted on the genetic datasets inferred from the populations of C. rudolphii sp. A from the distinct sampling areas with ARLEQUIN version 3.5 with 1,000 permutations, establishing the level of significance to a P < 0.05 (40, 41). A haplotype parsimony network was constructed using PopART (42). The analysis was performed using the statistical parsimony procedure (95% parsimony connection limit), implemented in TCS (43). Circle sizes are proportional to the corresponding haplotype frequencies.
3. Results
The parasitological analysis of the Ph. aristotelis desmarestii individuals allowed the identification of 1,517 nematode specimens which were morphologically referred to as third-stage larvae (L3), preadults, and adults of C. rudolphii (s.l.). All cormorants were found to be parasitized with nematode specimens (Prevalence, P = 100%), being the parasites found in the stomach and along the esophagus of the hosts.
Considering the obtained sequences, the best-fit substitution models were GTR+I+G (I+G = 0.5482) and TVM+G (G = 0.3353) for mtDNA cox2 and ITS rDNA regions, respectively. The mtDNA cox2 and the ITS1 and ITS2 rDNA sequences analysis of the C. rudolphii (s.l.) specimens allowed us to identify all the nematode specimens as C. rudolphii sp. A, including 33 adults, 21 preadults, and 21 larvae. DNA sequences from mtDNA cox2 and ITS1 and ITS2 rDNA regions were deposited in GenBank under the accession numbers OP690535-40 (mtDNA cox2), OP677791-96 (ITS1 region of rDNA), and OP677785-90 (ITS2 region of rDNA). Indeed, the Bayesian inference (BI) phylogenetic tree, based on mtDNA cox2 sequences (Figure 2), indicated that all the C. rudolphii sp. A sequences here obtained from specimens parasitizing Ph. a. desmarestii, clustered together, with high probability values in a well-supported clade, with other C. rudolphii sp. A sequences previously deposited in GenBank from specimens parasitizing Ph. carbo sinensis (accession numbers EF513502 and EF558891). This clade was clearly distinct from the other two clades obtained for the other sibling species, C. rudolphii sp. B and C. rudolphii sp. F, reported in Ph. carbo and P. occidentalis, respectively. Similarly, sequences of the other Contracaecum species included in the tree, parasitizing fish-eating bird hosts of the genera Phalacrocorax and Pelicanus, were grouped into distinct and well-supported clades (Figure 2). In the same vein, the BI phylogenetic tree based on rDNA ITS1 and ITS2 sequences (Figure 3) indicated that all the sequences obtained from Contracaecum spp., previously sequenced at the mtDNA cox2 locus, were grouped in a well-supported clade with a reference sequence of C. rudolphii sp. A from the same host (Ph. aristotelis) (accession number EU678869). This clade was phylogenetically distinct in comparison with the other six clades obtained for each sibling species, i.e., C. rudolphii sp. B, C. rudolphii sp. C, C. rudolphii sp. D, C. rudolphii sp. E, and C. rudolphii sp. F, as well as for the other Contracaecum species, from hosts of the Phalacrocorax and the Pelicanus genera (Figure 3).
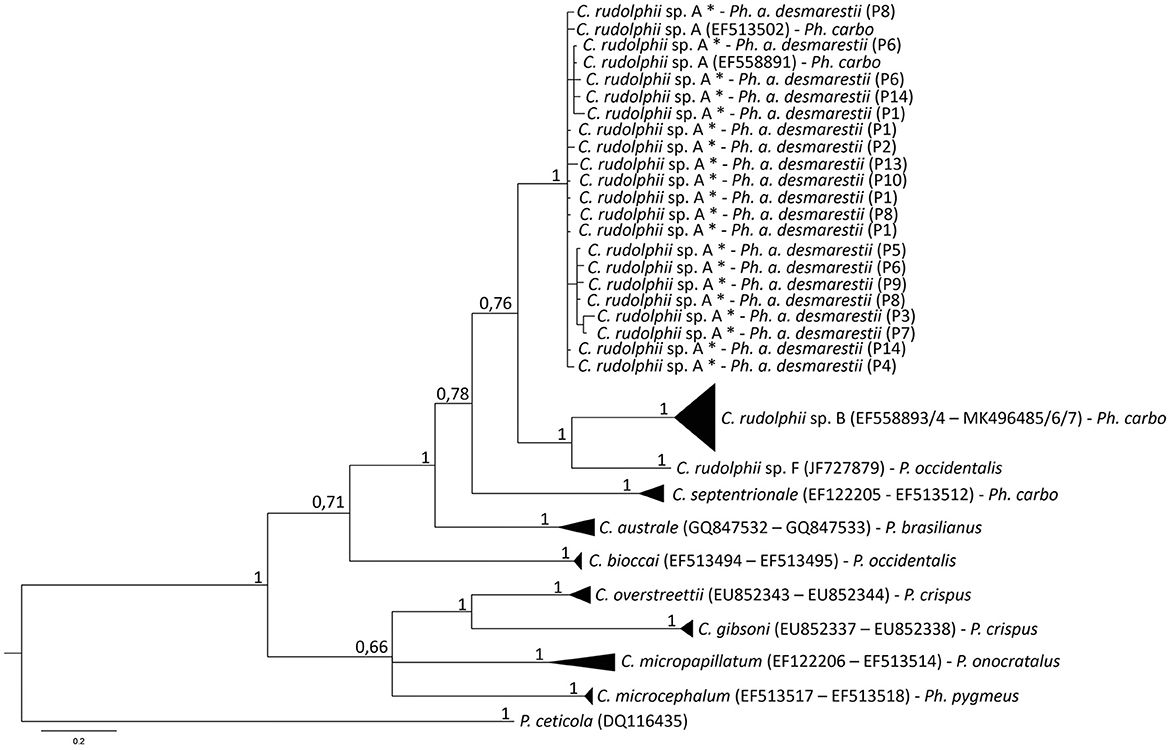
Figure 2. Bayesian inference phylogenetic tree based on sequences of mtDNA cox2 gene of specimens of C. rudolphii sp. A obtained in the present study with respect to the other Contracaecum spp. sequenced at the same gene locus previously deposited in GenBank. Definitive host species of each Contracaecum species sequence are indicated. Specimens obtained in the present study are marked with an asterisk including in brackets the definitive host specimen code. The analysis was performed by MrBayes software (30) using the GTR+I+G (I+G = 0.5482) substitution model as determined by JModeltest software (37). P. ceticola was used as an outgroup.
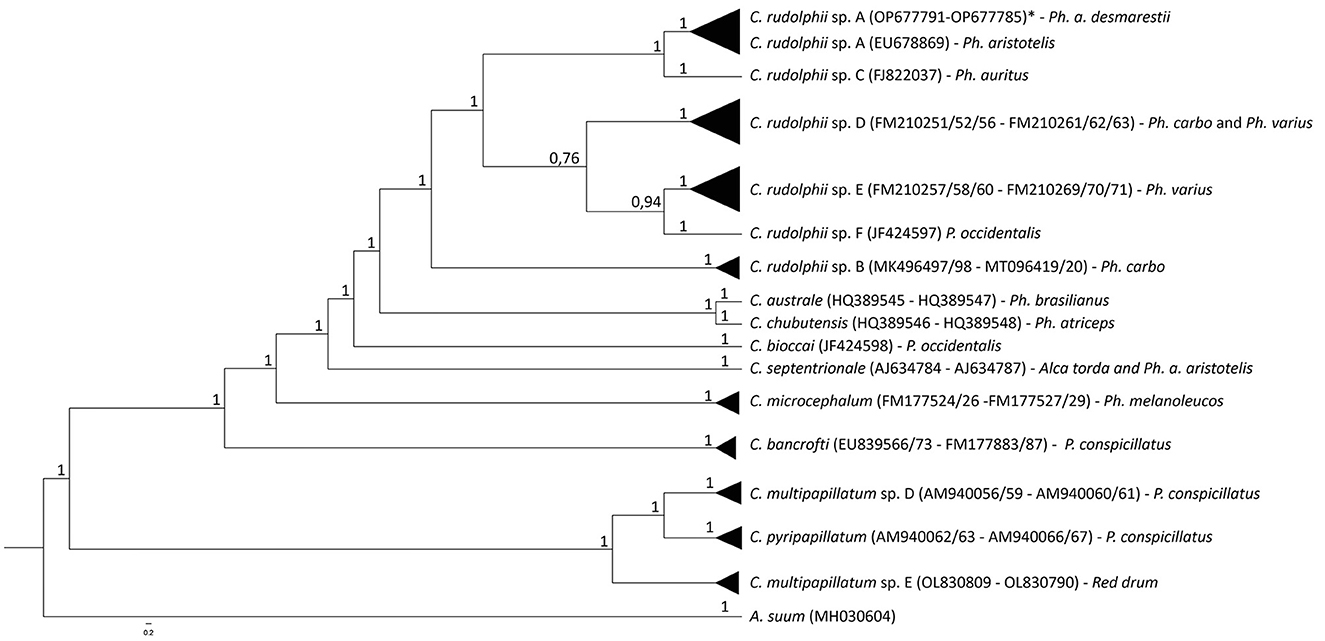
Figure 3. Bayesian inference phylogenetic tree based on sequences of ITS1 and ITS2 rDNA regions of specimens of C. rudolphii sp. A obtained in the present study with respect to the other Contracaecum spp. sequenced at the same locus previously deposited in GenBank. Definitive host species of each Contracaecum species sequence are indicated. The analysis was performed by MrBayes software (30) using TVM+G (G = 0.3353) substitution model as determined by JModeltest software (37). A. suum was used as an outgroup.
The hierarchical gene diversity analysis by AMOVA indicated that 3.69% of the genetic variation of C. rudolphii sp. A from the western Mediterranean localities here considered was expressed among populations while 96.31% was observed within populations. Because no significant intraspecific genetic variation was found among specimens from the Catalan-Spanish coast analyzed in the present work, they were considered as a single population. Estimates of genetic differentiation among C. rudolphii sp. A population of the three analyzed areas from the west Mediterranean, i.e., the Spanish Catalan, the Tyrrhenian, and the Sardinian coasts, inferred from the Fst value and their P-values, are shown in Table 2. Significant genetic differentiation was found among C. rudolphii sp. A metapopulations from the Sardinian coast compared to those from the Spanish region examined here (Fst ≈ 0.08, p < 0.00001). Similarly, significant Fst values were found between C. rudolphii sp. A populations from the Sardinian and Tyrrhenian coasts (Fst ≈ 0.06, p < 0.00001). In contrast, no significant genetic differentiation was observed between the populations from the Mediterranean Spanish coast (Catalan region) and the Tyrrhenian one (Fst ≈ 0.005, p > 0.05). The haplotype parsimony network (TCS) analysis, inferred from cox2 mtDNA of C. rudolphii sp. A, revealed a star-like tree as shown in Figure 4. In the Spanish coast population of the parasite species, 40 haplotypes were detected, while 80 and 28 haplotypes were identified from the Tyrrhenian and Sardinian populations, respectively (Table 3). The Hap7 was the most frequent haplotype shared by all three metapopulations. Hap4 and Hap15 were also frequently observed, shared by several individuals from all geographical areas. Whereas, the Hap3 was mainly shared by individuals from the Spanish coast. However, in these four major haplotypes, the relative frequency of individuals from the Sardinian waters was very low, comprising mainly Spanish and Tyrrhenian individuals. In addition, specimens from the Sardinian coast presented a high frequency of unique haplotypes. As shown in Table 3, the metapopulation from the Sardinian area showed the highest values of nucleotide diversity (per site) (π = 0.112) and the average number of nucleotide differences (K = 5.807). The haplotype diversity (Hd) was high in all three populations, with values ranging between 0.969 and 0.986.

Table 2. Population pairwise Fst estimates inferred from mtDNA cox2 gene sequences analysis among C. rudolphii sp. A from the different western Mediterranean marine waters studied.
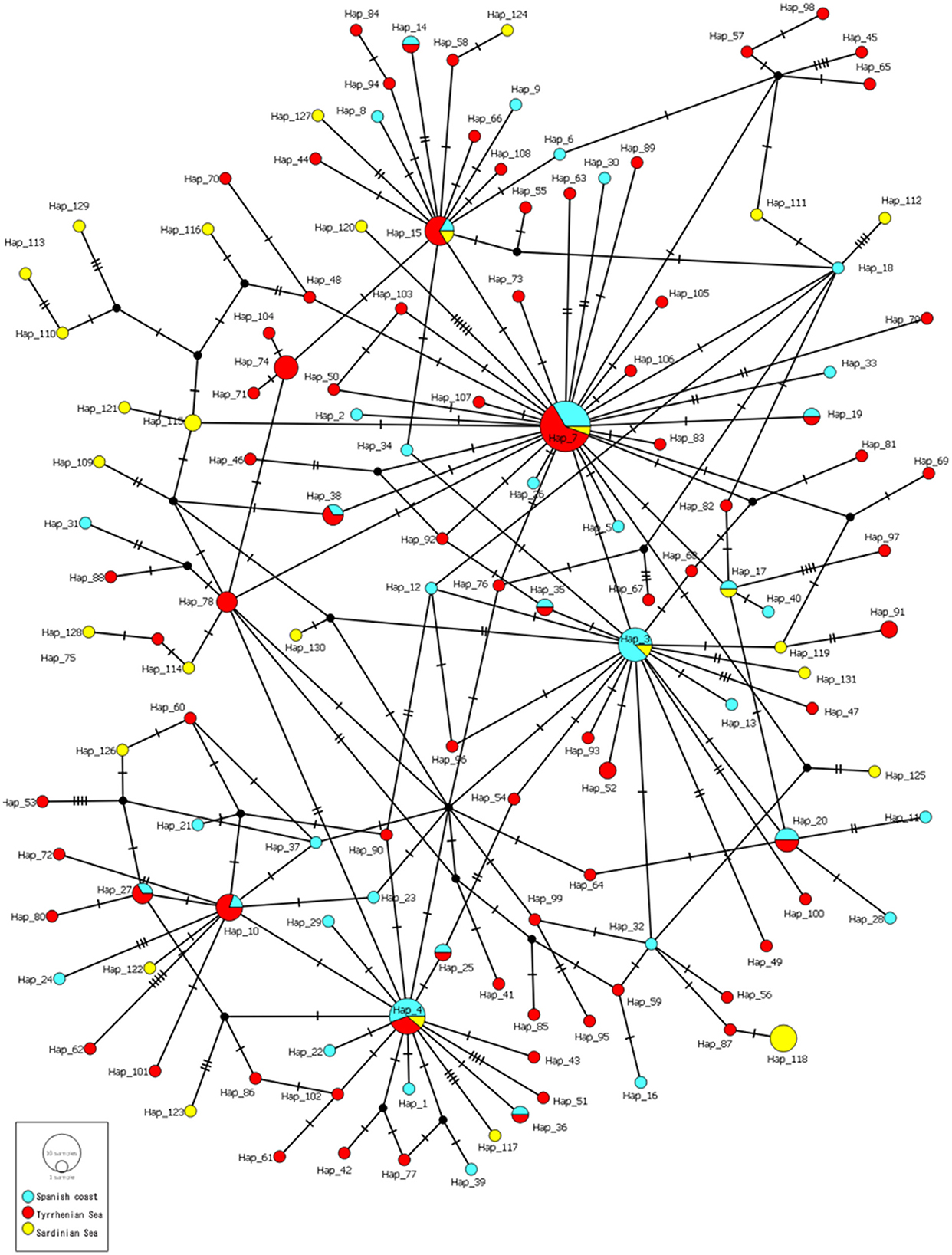
Figure 4. TCS network of C. rudolphii sp. A sequences of mtDNA cox2 dataset from Ph. a. desmarestii obtained in the present study and Ph. c. sinensis from Mattiucci et al. (7) and Amor et al. (38). Hatch marks indicate mutations. Circle sizes are proportional to the number of shared individuals per haplotype.

Table 3. Genetic diversity of C. rudolphii sp. A, inferred from the mtDNA cox2 gene, from the cormorants' definitive host, of the different western Mediterranean marine waters studied.
4. Discussion
Several Contracaecum species included in the C. rudolphii (s.l.) complex have been identified as parasitizing cormorants, worldwide. In the present study, we report the first genetic identification of C. rudolphii sp. A in the European Shag, Ph. aristotelis desmarestii, from the western Mediterranean Spanish coasts.
The multi-marker genetic approach using the mtDNA cox2 gene and the ITS rDNA region allowed the specific identification of all the nematode samples studied. The genetic identification of the sibling species of the C. rudolphii (s.l.) complex is important in order to understand their ecology and geographical distribution, but also the phylogenetic relationship among them. Both BI phylogenetic trees, based on the mtDNA cox2 gene locus and the ITS1 and ITS2 of the rDNA region, respectively, have shown that the C. rudolphii sp. A specimens were genetically distinct from the other species of the C. rudolphii (s.l.) complex, including sequences of C. rudolphii sp. B, C. rudolphii sp. C, C. rudolphii sp. D, C. rudolphii sp. E, and C. rudolphii sp. F (3, 4, 8, 13, 44). As shown in Figure 3, and in accordance with previous studies, a closer genetic relationship was observed between C. rudolphii sp. A with the other sibling species rather than with C. rudolphii sp. B. (7, 9). In this sense, D'Amelio et al. (3), when analyzing the phylogenetic tree inferred from the rrnS rDNA region, found the closest relationship between C. rudolphii sp. A and C. rudolphii sp. C, rather than between C. rudolphii sp. A and C. rudolphii sp. B. Although heterozygote genotypes between C. rudolphii sp. A and C rudolphii sp. B have been reported, inferred from the ITS rDNA region (38, 45), their genetic analysis should be assessed by using a multi-nuclear diagnostic loci approach, as shown between sibling species of other anisakid nematodes, i.e., the species of the Anisakis simplex (s.l.) complex (46–49). Discovering novel nuclear diagnostic markers between the two species would also improve the investigation of the possible existence of hybridization/introgression events between them.
Cormorants of the Ph. a. desmarestii subspecies are sedentary animals that habit strictly marine ecosystems and are endemic to the Mediterranean Sea (21). Data regarding the nematode presence in this host are scarce. In the Mediterranean waters, C. rudolphii sp. A has been identified parasitizing European Shag specimens on the Sardinian coasts (24). In the north-western Spanish waters (Atlantic Galician coast), the presence of C. rudolphii sp. A was reported at high prevalence values parasitizing cormorants of the subspecies Ph. aristotelis aristotelis (23). Those records, combined with the present finding of C. rudolphii sp. A in Ph. aristotelis desmarestii from the western Mediterranean Spanish coast and the absence of the sibling species C. rudolphii sp. B, confirm the adaptation of the former anisakid species to marine and brackish-water ecosystems, as suggested by Mattiucci et al. (7, 15). This hypothesis was supported by the high relative proportion of C. rudolphii sp. A found in Ph. carbo sinensis habiting in brackish environments of Italy, whereas the sibling species C. rudolphii sp. B infecting the same cormorant species was mainly found living in freshwater environments, such as rivers and lakes of Italy (7). Similar proportions of infection, with the species C. rudolphii sp. A from brackish cormorants (38) and with C. rudolphii sp. B in cormorants from freshwater environments (50) have been reported later. It has been hypothesized that the observed differential spatial distribution of C. rudolphii sp. A and C. rudolphii sp. B in distinct aquatic ecosystems could also be related to the differential feeding ecology and the behavior of distinct wintering populations of Ph. carbo sinensis in European areas (7). In this sense, the sedentary pattern and the feeding behavior of the Ph. a. desmarestii, the definitive host herein studied, would be responsible for the maintenance of the life cycle of C. rudolphii sp. A along the Catalan marine coastal ecosystem. The low mobility of the Ph. a. desmarestii could also help to maintain the genetic differentiation of the C. rudolphii sp. A population observed in the present study, justifying the relatively high number of private haplotypes (n = 26) shown by the parasite species in this area (Figure 4). On the other hand, the migration pattern of the Great Cormorant, Ph. carbo, which acts as the main definitive host of C. rudolphii sp. A in European waters might explain the population genetic differentiation here found between the metapopulation of C. rudolphii sp. A from Sardinia in comparison with those conspecific ones from the Catalan and Tyrrhenian areas (Table 2). This fish-eating bird presents its high migration pattern along the brackish and freshwater environments of Europe, inhabiting, among the others, the Catalan and the Tyrrhenian Mediterranean waters during its non-breeding season (51, 52). Although the low number of samples from the Sardinian Sea herein studied (n = 33) should be considered, the existence of a population of Ph. carbo living during the winter season in Sardinia, which could have a different breeding area in comparison with those populations of Ph. carbo wintering in the Spanish and Tyrrhenian areas, might explain the parasite genetic differentiation observed in the present study. In support of this hypothesis, a higher presence through Sardinia has been observed in some Ph. carbo populations during its migration routes (53). Moreover, Marion and Gentil (54) detected the presence of two genetically distinct cormorant populations of Ph. carbo, one along the Sardinia coast and the other one along the Italic Peninsula, as inferred from the mtDNA cox2 gene, which could also explain the present finding of a certain genetic sub-structuring of C. rudolphii sp. A, harbored by cormorants analyzed from those areas, i.e., Sardinia (38) and Latium region (7). Indeed, C. rudolphii sp. A from cormorants of the Sardinian coast also showed a high number of private haplotypes (n = 23), not shared with the other populations, despite the lower number of specimens analyzed, which is in accordance with the estimation of Fst values herein obtained. In addition, the most frequent haplotypes observed in Figure 4 seemed to be shared mainly by specimens from the Spanish and the Tyrrhenian coasts. The influence of the population structure and the migration route of the definitive hosts in shaping the genetic structure of anisakid nematodes has also been observed in Anisakis spp. (55) and Uncinaria lucasi (56). In this sense, it is suggested that the interaction among definitive, intermediate, and paratenic hosts influences the genetic structure of parasite populations, being the geographic origin of the host species a major driver (55). However, the analysis of a high number of specimens of C. rudolphii sp. A from cormorants collected from different brackish and marine ecosystems of European countries, as well as knowledge on the migration patterns of different populations of its phalacrocoracid definitive host, is necessary in order to shed light on the population genetic structure of this waterbird anisakid species.
The high prevalence of C. rudolphii sp. A (P = 100%) observed in the present study from the analyzed cormorant specimens is in accordance with that previously reported by Abollo et al. (23) in Ph. a. aristotelis from the Spanish Atlantic coast and with those recorded in Ph. c. sinensis from the Tyrrhenian Sea (7, 38). Comparison with C. rudolphii sp. A from the population of the European Shag of Sardinia is not possible because of the lack of epidemiological data (24). However, despite the high prevalence values herein observed, the life cycle of C. rudolphii sp. A in the Catalan Spanish coasts is not clear. Various studies analyzing several intermediate/paratenic hosts from this area, such as Micromesistius poutassou (57), Sardina pilchardus (58), Phycis blennoides (59), and Merluccius merluccius (60), among others, have not detected Contracaecum spp. larvae in these fish. Nevertheless, along the Spanish Mediterranean coast, some studies have identified Contracaecum spp. larvae parasitizing other fish species, including Anguilla anguilla (61), Scomber scombrus, Trachurus trachurus (62), Pagellus erythrinus, Trisopterus minutus, and Engraulis encrasicolus (59). Still, specimens were identified only at the genus level, using morphological criteria and without performing the molecular identification of the parasites. In other areas of the Mediterranean, such as Sardinian waters and the Tyrrhenian Sea, specific identification of C. rudolphii sp. A parasitizing various intermediate/paratenic hosts, including Sparus aurata (63), Trachurus sp., Mullus sp., Illex coindettii (64), A. anguilla, Dicentrarchus labrax (7, 65), Gobius niger, G. paganellus, and Solea solea (65) has been reported. Studies regarding the genetic identification of Contracaecum larvae in fish species, likely included in the prey items list of the Ph. a. desmarestii, from the western Mediterranean Spanish coast, should be accomplished for a better understanding of the C. rudolphii sp. A life cycle in the studied area.
Data availability statement
The datasets presented in this study can be found in online repositories. The names of the repository/repositories and accession number(s) can be found in the article/ supplementary material.
Ethics statement
Ethical review and approval was not required for the animal study because the cormorants used in the present study were found dead and no permit and/or ethical consideration were needed.
Author contributions
MC, CT, and JB performed a cormorant necropsy and the collection and morphological identification of parasites. XR-G, MP, and SM contributed to the design of the study, performed molecular and phylogenetic analyses of selected parasites, and wrote the manuscript. All authors contributed to the manuscript revision, and read and approved the submitted version.
Funding
This study was supported by the Grandi Progetti di Ricerca Ateneo of “Sapienza (2020)-University of Rome”; the Programa Pleamar of the Fundación Biodiversidad under the European Maritime and Fisheries Fund (EMFF) in the DESMARES III project of the University of Girona (UdG); the Generalitat de Catalunya Project 2017 SGR 1008; and the Margarita Salas grant for the training of young doctoral graduates under the call for the Requalification of the Spanish University system.
Acknowledgments
We thank Torreferrussa and Aiguamolls de l'Empordà Fauna Recovery Centre for allowing us access to the dead birds. Permits were provided by the Departament de Territori i Sostenibilitat of the Generalitat de Catalunya. We also want to thank Irene Canal Serrano for her help in the dissection of the stomachs and the separation and counting of the parasites.
Conflict of interest
The authors declare that the research was conducted in the absence of any commercial or financial relationships that could be construed as a potential conflict of interest.
Publisher's note
All claims expressed in this article are solely those of the authors and do not necessarily represent those of their affiliated organizations, or those of the publisher, the editors and the reviewers. Any product that may be evaluated in this article, or claim that may be made by its manufacturer, is not guaranteed or endorsed by the publisher.
References
1. Mattiucci S, Nascetti G. Advances and trends in the molecular systematics of Anisakid nematodes, with implications for their evolutionary ecology and host-parasite co-evolutionary processes. Adv Parasitol. (2008) 66:47–148. doi: 10.1016/S0065-308X(08)00202-9
2. Shamsi S. Parasite loss or parasite gain? Story of Contracaecum nematodes in antipodean waters. Parasite Epidemiol Control. (2019) 4:e0087. doi: 10.1016/j.parepi.2019.e00087
3. D'Amelio S, Barros NB, Ingrosso S, Fauquier DA, Russo R, Paggi L. Genetic characterization of members of the genus Contracaecum (Nematoda: Anisakidae) from fish-eating birds from west-central Florida, USA, with evidence of new species. Parasitology. (2007) 134:1041–51. doi: 10.1017/S003118200700251X
4. D'Amelio S, Cavallero S, Dronen NO, Barros NB, Paggi L. Two new species of Contracaecum Railliet & Henry, 1912 (Nematoda: Anisakidae), C. fagerholmi n sp and C. rudolphii F from the brown pelican Pelecanus occidentalis in the northern Gulf of Mexico. Syst Parasitol. (2012) 81:1–16. doi: 10.1007/s11230-011-9323-x
5. Garbin LE, Diaz JI, Cremonte F, Navone GT. A new aniskid species parasitizing the imperial cormorant Phalacrocorax atriceps from the north patagonian coast, Argentina. J Parasitol. (2008) 94:852–9. doi: 10.1645/GE-1369.1
6. Mattiucci S, Paoletti M, Solorzano AC, Nascetti G. Contracaecum gibsoni n. sp. and C. overstreeti n. sp. (Nematoda: Anisakidae) from the Dalmatian pelican Pelecanus crispus (L.) in Greek waters: genetic and morphological evidence. Syst Parasitol. (2010) 75:207–24. doi: 10.1007/s11230-009-9220-8
7. Mattiucci S, Sbaraglia GL, Palomba M, Filippi S, Paoletti M, Cipriani P, et al. Genetic identification and insights into the ecology of Contracaecum rudolphii A and C. rudolphii B (Nematoda: Anisakidae) from cormorants and fish of aquatic ecosystems of Central Italy. Parasitol Res. (2020) 119:1243–57. doi: 10.1007/s00436-020-06658-8
8. Shamsi S, Norman R, Gasser R. Genetic and morphological evidences for the existence of sibling species within Contracaecum rudolphii (Hartwich, 1964) (Nematoda: Anisakidae) in Australia. Parasitol Res. (2009) 529–38. doi: 10.1007/s00436-009-1424-y
9. Shamsi S, Norman R, Gasser R. Redescription and genetic characterization of selected Contracaecum spp. (Nematoda: Anisakidae) from various hosts in Australia. Parasitol Res. (2009) 104:1507–25. doi: 10.1007/s00436-009-1357-5
10. Sardella CJ, Mancini M, Salinas V, Simões RO, Luque JL. A new species of Contracaecum (Nematoda: Anisakidae) found parasitizing Nannopterum brasilianus (Suliformes: Phalacrocoracidae) and Hoplias argentinensis (Characiformes: Erythrinidae) in South America: Morphological and molecular characterization of larval and adult stages. J Helminthol. (2020) 94:1–11. doi: 10.1017/S0022149X20000644
11. Nascetti G, Cianchi R, Mattiucci S. D'amelio S, Orecchia P, Paggi L, et al. Three sibling species within Contracaecum osculatum (Nematoda, Ascaridida, Ascaridoidea) from the Atlantic arctic-boreal region: Reproductive isolation and host preferences. Int J Parasitol. (1993) 23:105–20. doi: 10.1016/0020-7519(93)90103-6
12. Li AX, D'Amelio S, Paggi L, He F, Gasser RB, Lun ZR, et al. Genetic evidence for the existence of sibling species within Contracaecum rudolphii (Hartwich, 1964) and the validity of Contracaecum septentrionale (Kreis, 1955) (Nematoda: Anisakidae). Parasitol Res. (2005) 96:361–6. doi: 10.1007/s00436-005-1366-y
13. Mattiucci S, Paoletti M, Webb SC, Sardella N, Timi JT, Berland B, et al. Genetic relationships among species of Contracaecum Railliet and Henry, 1912 and Phocascaris Höst, 1932 (Nematoda: Anisakidae) from pinnipeds inferred from mitochondrial cox2 sequences, and congruence with allozyme data. Parasite. (2008) 15:408–19. doi: 10.1051/parasite/2008153408
14. Hartwich G. Revision der Vogelparasitischen Nematoden Mitteleuropas II. Die Gattung Contracaecum Railliet and Henry, 1912 (Ascaridoidea). Mitt Zool Mus Berl. (1964) 40:15–53. doi: 10.1002/mmnz.4830400104
15. Mattiucci S, Turchetto M, Bragantini F, Nascetti G. On the occurrence of the sibling species of Contracaecum rudolphii complex (Nematoda: Anisakidae) in cormorants (Phalacrocorax carbo sinensis) from Venice and Caorle lagoons: genetic markers and ecological studies. Parassitologia. (2002) 44:105.
16. Bullini L, Nascetti G, Paggi L, Orecchia P, Mattiucci S, Berland B. Genetic variation of ascarid worms with different life cycle. Evolution. (1986) 40:437–40. doi: 10.2307/2408826
17. Nadler SA, Hudspeth DS. Phylogeny of the Ascaridoidea (Nematoda: Ascaridida) based on three genes and morphology: hypotheses of structural and sequence evolution. J Parasitol. (2000) 86:380–93. doi: 10.1645/0022-3395(2000)086[0380:POTANA]2.0.CO;2
18. Garbin L, Mattiucci S, Paoletti M, González-Acuña D, Nascetti G. Genetic and morphological evidences for the existence of a new species of Contracaecum (Nematoda: Anisakidae) parasite of Phalacrocorax brasilianus (Gmelin) from Chile and its genetic relationships with congeners from fish-eating birds. J Parasitol. (2011) 97:476–92. doi: 10.1645/GE-2450.1
19. Barros Á, Alvarez D, Velando A. Cormorán moñudo-Phalacrocorax aristotelis (Linnaeus, 1761). In:Salvador A, Morales MB, , editors. Enciclopedia virtual de los vertebrados españoles. Madrid: Museo Nacional de Ciencias Naturales (2013).
20. Sponza S, Cosolo M, Kralj J. Migration patterns of the Mediterranean Shag Phalacrocorax aristotelis desmarestii (Aves: Pelecaniformes) within the northern Adriatic Sea. Ital J Zool. (2013) 80:380–91. doi: 10.1080/11250003.2013.775365
21. Nelson JB. Pelicans, Cormorants and their Relatives: The Pelecaniformes. Nelson JB, editor. Oxford: Oxford University Press (2005).
22. Xirouchakis SM, Kasapidis P, Christidis A, Andreou G, Kontogeorgos I, Lymberakis P. Status and diet of the European Shag (Mediterranean subspecies) Phalacrocorax aristotelis desmarestii in the Libyan Sea (South Crete) during the breeding season. Mar Ornithol. (2017) 45:1–9.
23. Abollo E, Gestal C, Pascual S. Anisakid infection in the European shag Phalacrocorax aristotelis aristotelis. J Helminthol. (2001) 75:209–14. doi: 10.1079/JOH200051
24. Farjallah S, Merella P, Ingrosso S, Rotta A, Slimane B, Garippa G, et al. Molecular evidence for the occurrence of Contracaecum rudolphii A (Nematoda: Anisakidae) in shag Phalacrocorax aristotelis (Linnaeus) (Aves: Phalacrocoracidae) from Sardinia (western Mediterranean Sea). Parasitol Int. (2008) 57:437–40. doi: 10.1016/j.parint.2008.05.003
25. Kreis HA. Contracaecum septentrionale, ein neuer Parasit aus dem Kormoran: sein Lebenslauf, sowie Angaben über die Entwicklung der Anisakinae. Z Parasitenkd. (1955) 17:106–21. doi: 10.1007/BF00260308
26. Nascetti G, Mattiucci S, Cianchi R, Berland B, Bullini L, Paggi L. Genetic relationship among Contracaecum spp. (Nematoda: Anisakidae), parasites of cormorants and pelecans of the Boreal region. Acta Parasitol. (2000) 45:153.
27. Berland B. Nematodes from some Norwegian marine fishes. Sarsia. (1961) 2:1–50. doi: 10.1080/00364827.1961.10410245
28. Zhu X, Gasser RB, Podolska M, Chilton NB. Characterisation of Anisakid nematodes with zoonotic potential by nuclear ribosomal DNA sequences. Int J Parasitol. (1998) 28:1911–21. doi: 10.1016/S0020-7519(98)00150-7
29. Thompson JD, Gibson TJ, Plewniak F, Jeanmougin F, Higgins DG. The CLUSTAL X windows interface: flexible strategies for multiple sequence alignment aided by quality analysis tools. Nucleic Acids Res. (1997) 25:4876–82. doi: 10.1093/nar/25.24.4876
30. Ronquist F, Huelsenbeck JP. MrBayes 3: Bayesian phylogenetic inference under mixed models. Bioinformatics. (2003) 19:1572–4. doi: 10.1093/bioinformatics/btg180
31. Mattiucci S, Paoletti M, Olivero-Verbel J, Baldiris R, Arroyo-Salgado B, Garbin L, et al. Contracaecum bioccai n. sp. from the brown pelican Pelecanus occidentalis (L.) in Colombia (Nematoda: Anisakidae): morphology, molecular evidence and its genetic relationship with congeners from fish-eating birds. Syst Parasitol. (2008) 69:101–21. doi: 10.1007/s11230-007-9116-4
32. Valentini A, Mattiucci S, Bondanelli P, Webb SC, Mignucci-Giannone AA, Colom-Llavina MM, et al. Genetic relationships among Anisakis species (Nematoda: Anisakidae) inferred from mitochondrial cox2 sequences, and comparison with allozyme data. J Parasitol. (2006) 92:156–66. doi: 10.1645/GE-3504.1
33. Shamsi S, Gasser R, Beveridge I, Shabani AA. Contracaecum pyripapillatum n. sp. (Nematoda: Anisakidae) and a description of C. multipapillatum (von Drasche, 1882) from the Australian pelican, Pelecanus conspicillatus. Parasitol Res. (2008) 103:1031–9. doi: 10.1007/s00436-008-1088-z
34. Davidovich N, Tedesco P, Caffara M, Yasur-Landau D, Gustinelli A, Drabkin V, et al. Morphological description and molecular characterization of Contracaecum larvae (Nematoda: Anisakidae) parasitizing market-size hybrid tilapia (Oreochromis aureus x Oreochromis niloticus) and red drum (Sciaenops ocellatus) farmed in Israel. Food Waterborne Parasitol. (2022) 26:e00147. doi: 10.1016/j.fawpar.2022.e00147
35. Hoberg EP, Burek-Huntington K, Beckmen K, Camp LE, Nadler SA. Transuterine infection by Baylisascaris transfuga: neurological migration and fatal debilitation in sibling moose calves (Alces alces gigas) from Alaska. Int J Parasitol Parasites Wildl. (2018) 7:280–8. doi: 10.1016/j.ijppaw.2018.07.005
36. Vaidya G, Lohman DJ, Meier R. SequenceMatrix: concatenation software for the fast assembly of multi-gene datasets with character set and codon information. Cladistics. (2011) 27:171–80. doi: 10.1111/j.1096-0031.2010.00329.x
37. Posada D, Crandall KA. MODELTEST testing the model of DNA substitution. Bioinformatics. (1998) 14:817–8. doi: 10.1093/bioinformatics/14.9.817
38. Amor N, Farjallah S, Piras MC, Burreddu C, Garippa G, Merella P. New insights into the coexistence of Contracaecum rudolphii A and Contracaecum rudolphii B (Nematoda: Anisakidae) in Phalacrocorax carbo sinensis from Sardinia: genetic variability and phylogenetic analysis. Parasitology. (2020) 147:1538–51. doi: 10.1017/S0031182020001341
39. Librado P, Rozas J. DnaSP v5: a software for comprehensive analysis of DNA polymorphism data. Bioinformatics. (2009) 25:1451–2. doi: 10.1093/bioinformatics/btp187
40. Weir BS, Cockerham CC. Estimating F-statistics for the analysis of population structure. Evolution. (1984) 38:1358–70. doi: 10.1111/j.1558-5646.1984.tb05657.x
41. Excoffier L. Lischer HEL. Arlequin suite ver 35: A new series of programs to perform population genetics analyses under Linux and Windows. Mol Ecol Resour. (2010) 10:564–7. doi: 10.1111/j.1755-0998.2010.02847.x
42. Bandelt HJ, Forster P, Röhl A. Median-joining networks for inferring intraspecific phylogenies. Mol Biol Evol. (1999) 16:37–48. doi: 10.1093/oxfordjournals.molbev.a026036
43. Clement M, Posada D, Crandall KA. TCS a computer program to estimate gene genealogies. Mol Ecol. (2000) 9:1657–9. doi: 10.1046/j.1365-294x.2000.01020.x
44. Molnár K, Székely C, Baska F, Müller T, Zuo S, Kania PW, et al. Differential survival of 3rd stage larvae of Contracaecum rudolphii type B infecting common bream (Abramis brama) and common carp (Cyprinus carpio). Parasitol Res. (2019) 118:2811–7. doi: 10.1007/s00436-019-06441-4
45. Szostakowska B, Fagerholm HP. Coexistence and genetic variability of Contracaecum rudolphii A and Contracaecum rudolphii B (Nematoda: Anisakidae) in cormorants, Phalacrocorax carbo sinensis, in the baltic region. J Parasitol. (2012) 98:472–8. doi: 10.1645/GE-2884.1
46. Mattiucci S, Acerra V, Paoletti M, Cipriani P, Levsen A, Webb SC, et al. No more time to stay ‘single' in the detection of Anisakis pegreffii, A. simplex (s. s.) and hybridization events between them: a multi-marker nuclear genotyping approach. Parasitology. (2016) 143:998–1011. doi: 10.1017/S0031182016000330
47. Mattiucci S, Bello E, Paoletti M, Webb SC, Timi JT, Levsen A, et al. Novel polymorphic microsatellite loci in Anisakis pegreffii and A. simplex (s. s.) (Nematoda: Anisakidae): implications for species recognition and population genetic analysis. Parasitology. (2019) 146:1387–403. doi: 10.1017/S003118201900074X
48. Bello E, Paoletti M, Webb SC, Nascetti G, Mattiucci S. Cross-species utility of microsatellite loci for the genetic characterisation of Anisakis berlandi (Nematoda: Anisakidae). Parasite. (2020) 27:9. doi: 10.1051/parasite/2020004
49. Roca-Geronès X, Alcover MM, Godínez-González C, Montoliu I. Fisa R. Hybrid genotype of Anisakis simplex (s.s.) and A. pegreffii identified in third- and fourth-stage larvae from sympatric and allopatric Spanish marine waters. Animals. (2021) 11:2458. doi: 10.3390/ani11082458
50. Carmeno C, Rusconi A, Castelli M, Prati P, Bragoni R, Santoro A, et al. Molecular identification of Contracaecum rudolphii A and B (Nematoda: Anisakidae) from cormorants collected in a freshwater ecosystem of the pre-alpine area in Northern Italy. Vet Parasitol Reg Stud Rep. (2022) 27:100674. doi: 10.1016/j.vprsr.2021.100674
51. Frederiksen M, Korner-Nievergelt F, Marion L, Bregnballe T. Where do wintering cormorants come from? Long-term changes in the geographical origin of a migratory bird on a continental scale. J Appl Ecol. (2018) 55:2019–32. doi: 10.1111/1365-2664.13106
52. van Eerden MR, Parz-Gollner R, Marion L, Bregnballe T, Paquet JY, Volponi S, et al. Numbers of Great Cormorants Phalacrocorax carbo wintering in the western Palaearctic in January 2013. Ardea. (2021) 109:271–84. doi: 10.5253/arde.v109i2.a2
53. Franks S, Fiedler, W, Arizaga, J, Jiguet, F, Nikolov, B, van der Jeugd, H,. Online Atlas of the Movements of Eurasian African Bird Populations. EURING/CMS. (2022). Available online at: https://migrationatlas.org/node/1773 (accessed December 6, 2022).
54. Marion L, le Gentil J. Ecological segregation and population structuring of the Cormorant Phalacrocorax carbo in Europe, in relation to the recent introgression of continental and marine subspecies. Evol Ecol. (2006) 20:193–216. doi: 10.1007/s10682-005-5828-6
55. Cipriani P, Palomba M, Giulietti L, Marcer F, Mazzariol S, Santoro M, et al. Distribution and genetic diversity of Anisakis spp. in cetaceans from the Northeast Atlantic Ocean and the Mediterranean Sea. Sci Rep. (2022) 12:13664. doi: 10.1038/s41598-022-17710-1
56. Davies K, Pagan C, Nadler SA. Host population expansion and the genetic architecture of the pinniped hookworm Uncinaria lucasi. J Parasitol. (2020) 106:383–91. doi: 10.1645/19-172
57. Roca-Geronès X, Segovia M, Godínez-González C, Fisa R, Montoliu I. Anisakis and Hysterothylacium species in Mediterranean and North-East Atlantic fishes commonly consumed in Spain: epidemiological, molecular and morphometric discriminant analysis. Int J Food Microbiol. (2020) 325:108642. doi: 10.1016/j.ijfoodmicro.2020.108642
58. Rello FJ, Adroher FJ, Valero A. Hysterothylacium aduncum, the only anisakid parasite of sardines (Sardina pilchardus) from the southern and eastern coasts of Spain. Parasitol Res. (2008) 104:117–21. doi: 10.1007/s00436-008-1168-0
59. Pulleiro-Potel L, Barcala E, Mayo-Hernández E, Muñoz P. Survey of anisakids in commercial teleosts from the western Mediterranean Sea: infection rates and possible effects of environmental and ecological factors. Food Control. (2015) 55:12–7. doi: 10.1016/j.foodcont.2015.02.020
60. Ferrer-Maza D, Lloret J, Muñoz M, Faliex E, Vila S, Sasal P. Parasitism, condition and reproduction of the European hake (Merluccius merluccius) in the northwestern Mediterranean Sea. ICES J Mar Sci. (2014) 71:1088–99. doi: 10.1093/icesjms/fst217
61. Mayo-Hernández E, Peñalver J, García-Ayala A, Serrano E, Muñoz P, Ruiz de Ybáñez R. Richness and diversity of helminth species in eels from a hypersaline coastal lagoon, Mar Menor, south-east Spain. J Helminthol. (2015) 89:345–51. doi: 10.1017/S0022149X14000145
62. Gutiérrez-Galindo JF, Osanz-Mur AC, Mora-Ventura MT. Occurrence and infection dynamics of anisakid larvae in Scomber scombrus, Trachurus trachurus, Sardina pilchardus, and Engraulis encrasicolus from Tarragona (NE Spain). Food Control. (2010) 21:1550–5. doi: 10.1016/j.foodcont.2010.03.019
63. Guardone L, Susini F, Castiglione D, Ricci E, Corradini C, Guidi A, et al. Ascaridoid nematode larvae in wild gilthead seabream (Sparus aurata) and European seabass (Dicentrarchus labrax) caught in the Tyrrhenian Sea (Western Mediterranean Sea): a contribute towards the parasitological risk assessment on two commercially important fish species. Food Control. (2020) 118:107377. doi: 10.1016/j.foodcont.2020.107377
64. Salati F, Meloni M, Cau M, Angelucci G. Presence of Contracaecum spp. in teleosts cultured and fished in Sardinia. Vet Parasitol. (2013) 196:382–7. doi: 10.1016/j.vetpar.2013.03.014
Keywords: Contracaecum rudolphii sp. A, European Shag, Western Mediterranean, molecular identification, population genetics
Citation: Roca-Geronès X, Fisa R, Montoliu I, Casadevall M, Tobella C, Bas JM, Palomba M and Mattiucci S (2023) Genetic diversity of Contracaecum rudolphii sp. A (Nematoda: Anisakidae) parasitizing the European Shag Phalacrocorax aristotelis desmarestii from the Spanish Mediterranean coast. Front. Vet. Sci. 10:1122291. doi: 10.3389/fvets.2023.1122291
Received: 12 December 2022; Accepted: 10 January 2023;
Published: 02 February 2023.
Edited by:
Paolo Merella, University of Sassari, ItalyReviewed by:
Nabil Amor, King Saud University, Saudi ArabiaMonica Caffara, University of Bologna, Italy
Copyright © 2023 Roca-Geronès, Fisa, Montoliu, Casadevall, Tobella, Bas, Palomba and Mattiucci. This is an open-access article distributed under the terms of the Creative Commons Attribution License (CC BY). The use, distribution or reproduction in other forums is permitted, provided the original author(s) and the copyright owner(s) are credited and that the original publication in this journal is cited, in accordance with accepted academic practice. No use, distribution or reproduction is permitted which does not comply with these terms.
*Correspondence: Xavier Roca-Geronès, eGF2aWVycm9jYUB1Yi5lZHU=; Simonetta Mattiucci,
c2ltb25ldHRhLm1hdHRpdWNjaUB1bmlyb21hMS5pdA==