- 1Guangdong Guangken Animal Husbandry Group Co., Ltd., Guangzhou, China
- 2Guangdong Provincial Key Laboratory of Animal Nutrition and Regulation, College of Animal Science, South China Agricultural University, Guangzhou, China
- 3Guangdong AIB Polytechnic College, Guangzhou, China
The aim of this study is to evaluate the effects of dietary iron sources on growth performance, iron status and activities of Fe-containing enzymes and gene expression related to iron homeostasis in tissues of weaned pigs. A total of 480 piglets at d 28 (Duroc X Landrace) were allotted to four groups as a factorial arrangement of treatments with 30 pigs/pen (male: female = 1:1) and 4 replicate pens/treatment. The treatments for iron in the diets were: control basal diet (Con); Con + 150 mg Fe/kg as inorganic Fe (iFe); Con + 75 mg Fe/kg as inorganic Fe + 75 mg Fe/kg as organic Fe-peptide complex (iFe+oFe) and Con + 150 mg of Fe/kg as organic Fe-peptide complex (oFe). The feeding trial lasted for 36 days. There were no significant differences in final body weight, ADG, ADFI, and G/F as well as blood hemoglobin and MCHC contents between piglets fed the control and iron-supplemented groups (P > 0.05). The iron supplemented groups exhibited increased iron content in the liver, kidney and spleen as well as the CAT and SDH activities in liver compared to the control group (P < 0.05), while piglets in oFe group experienced greater Fe accumulation and activities of CAT and SDH in the liver than piglets in the iFe group. Compared with the control group, dietary supplementation of iron increased the NCOA4 mRNA expression and decreased the TfR1 mRNA expression in liver of piglets. The TfR1, NCOA4 and Ferritin mRNA expressions of bone marrow in both iFe and iFe+oFe groups were greater than both in the Con and oFe groups. These results suggest that dietary supplementation of iron does not influence the growth performance and hematological parameters in weaned pigs fed a corn-soybean meal basal diet (75.8 mg/kg) from d 28 to d 70, but increased tissue iron status and activities of Fe-containing enzymes at d 70. The addition of organic Fe-peptide complexes presents greater beneficial effects on enhancing tissue Fe accumulation and Fe-containing enzyme activities, which may be involved in different gene expression patterns related to iron intake and transport in tissues of weaned pigs.
Introduction
The phenomenon of piglet anemia in production has been paid more and more attention. Piglets are prone to iron deficiency anemia for several reasons, including low iron stores at birth, inadequate sow milk supply, and rapid growth rate (1, 2). For decades, pigs have been selected for large litters, high birth weights and fast growth, which has resulted in greater body blood volume, red blood cells (RBC) count, and iron demands (3). Additionally, weaned piglets have a limited ability to utilize dietary iron (4). Therefore, various iron supplementation strategies have been shown to be beneficial in reducing anemia, as evaluated by measuring the RBC indices and iron status (5). Several attempts have been made to enhance iron status of piglets by iron injection (dosage of 200 mg of iron dextran) or dietary supplementation (100 mg Fe/kg of a corn-soybean meal basal diet as FeSO4, Fe-Gly or Heme) without significant effect on growth performance and hematologic variables (6, 7). These results may be attributed to the inability of iron sources and dietary supplemental levels in providing sufficient iron uptake to meet iron requirements. For example, the use of inorganic iron sources is known to affect the absorption and utilization of iron, showing lower bioavailability in animal diets. Organic iron, as an alternative to inorganic iron, presents the advantages of good bioavailability, high absorbability, excellent stability and high safety (8, 9). Among the different types of organic iron supplements, Fe-peptide chelating complexes have been received increasing attention in improving iron bioavailability, with regard to biological effects and intestinal iron absorption properties (10). Organic Fe-peptide chelating complexes bind with iron to form soluble chelate that avoids iron precipitation, and are absorbed through the intestinal peptide absorption pathway, while regulating the proliferation and differentiation of enterocytes through the interaction with the intestinal iron absorption pathway. However, data concerning the effects of Fe-peptide chelating complexes dietary supplementation on iron uptake and transport in piglets are relatively limited. Therefore, the objective of the current study is to evaluate the effects of replacing inorganic Fe with organic Fe-peptide complex on growth performance, hematological parameters, Fe status and activities of enzymes and gene expression related to the iron intake and transport in tissues of weaned pigs.
Materials and methods
Animals and diets
The animal care and use protocol was approved by the Animal Care and Use Committee of South China Agricultural University (SCAU-10564), and the study was conducted following the Regulations for the Administration of Affairs Concerning Experimental Animals.
A total of 480 weaned pigs (Duroc X Landrace) at 28 d with an average initial body weight of 8.14 ± 0.71 kg were allotted to four treatments (approximately 0.4 m2 of floor space/pig) as a factorial arrangement of treatments, with 30 pigs/pen (male: female = 1:1) and 4 replicate pens/treatment. Four treatments of Fe in the diet were performed: control basal diet (Con, Table 1); Con + 150 mg Fe/kg as inorganic Fe (iFe); Con + 75 mg Fe/kg as inorganic Fe + 75 mg Fe/kg as organic Fe-peptide complex (iFe+oFe), and Con + 150 mg of Fe/kg as organic Fe-peptide complex(oFe). The corn-soybean meal basal diet was formulated to meet nutrient requirements other than iron according to the recommendation of NRC (11). The supplemental Fe level added to the basal diet was considered industry standards, thus exceeding the NRC (11) requirements. Lysine and methionine, as the first and second limited amino acid (AA) in weaned pig diets, could eliminate the effect of additional AA produced by iron-peptide complexes and were considered to AA content in the diets. Therefore, lysine and methionine levels in the control diet or diets supplemented with inorganic iron were balanced by adding synthetic lysine-HCl and DL-methionine depending on the amount of lysine and methionine supplementation from the source of the Fe-peptide complex. Each pen contained two stainless steel nipple drinkers and a four-hole, stainless steel feeder. The ambient temperatures were initially set at 28°C but were adjusted as needed to remain within pigs' comfort zone. Pigs had ad libitum access to diets and water during the 42 experimental days from d 28 to d 70. The body weight and feed intake of pigs were measured by pens on d 28 and d 70 of the trial, and then average daily gain (ADG), average daily feed intake (ADFI) and the ratio of feed intake to gain (F/G) were calculated as following.
The feed intake was calculated by subtracting the residual feed from the feed provided, after making corrections for dry matter content of the feed ADFI = (Total feed eaten) / (pigs placed × d on-test). F/G is calculated by dividing the kg of feed eaten daily, by the kg of live weight gained daily.
After 12 h of fasting, the same two piglets per replicate pen were bled via cannulation of the ear veins puncture for repeat sampling of small volumes (<4 mL) at birth before dietary iron treatment, and on d 42, 56 and 70, respectively. At d 70, these selected pigs were anesthetized with an intravenous injection of pentobarbital sodium (50 mg/kg, Tc-P8411, Toscience Biotechnology, Shanghai, China) and sacrificed for tissue samples collection. Samples of their liver, kidney, and spleen were collected and a subsample was frozen at −20°C for analyses of iron content and catalase (CAT) and succinate dehydrogenase (SDH) activities. Another subsample of liver and bone marrow tissues were frozen in liquid nitrogen for gene expression assays. At the end of the experiment, the rest of piglets were removed and were fed with a commercial feed to meet the nutrients requirements. Lighting and feeding management were performed according to the instructions of piglets' management guidelines. The recovery performance standards and normal behaviors were done to evaluate the optimum welfare weekly.
Sample collections and analyses
Blood samples (2 to 3 mL) were placed on ice and transported to the laboratory for hematological measurements. Liver, kidney, and spleen samples were homogenized in ice-cold 10% (wt/vol) physiological saline for 1 min using a tissue grinder (T 18 D S25; IKA Group, Staufen, Germany) and then processed for 1 min using an ultrasonic wave cell grinder (JY92-11; Ningbo Scientz Biotechnology Co., Ltd., Ningbo, Jiangsu, P. R. China) for 1 min (1 s, with 2 s interval). Then, the homogenates were centrifuged at 1,000 × g for 15 min at 4°C to harvest the supernatants for immediately analyzing total protein content and CAT and SDH activities.
Iron contents in the diets and tissues were determined by inductively coupled plasma emission spectroscopy after wet digestions with HNO3 and HCIO4 as described by Zhang et al. (12). The iron analysis was validated with bovine liver powder as a standard reference material (GBW [E] 080193; National Institute of Standards and Technology, Beijing, China). Concentrations of Ca and CP in feed ingredient or diet samples were determined as described by the AOAC (13). Lysine and methionine contents of organic iron were analyzed by an automatic amino acid analyzer L-8800 (Hitachi, Tokyo, Japan). Hemoglobin (HGB) and mean corpuscular hemoglobin concentration (MCHC) levels in blood were determined using an automated AVIDIA 2010 analyzer (Siemens, Germany). CAT activities were analyzed by Goth's colorimetric method (14) with a commercial available test kit (A022-1-1; Jiancheng Bioengineering Institute, Nanjing, China). The activities of SDH were analyzed using a colorimetric method with a commercially available test kit (A002; Jiancheng Bioengineering Institute, Nanjing, China). Total protein concentrations in the supernatants of tissue homogenates were assayed by a BCA Protein Assay kit (23225; Thermo Scientific, Rockford, IL, USA) as per the manufacturer's instruction. The activities of these enzymes were expressed on a per mg protein basis.
Total RNA in the liver and marrow were isolated using TRIZOL reagent (15596018; Life Technologies, Carlsbad, CA, USA) according to the manufacturer's instructions. Reverse transcription was performed using the PrimeScript RT reagent Kit with gDNA Eraser (RR047A; Takara Bio Inc., Otsu, Japan) according to the manufacturer's protocols. The obtained cDNA was used to determine the gene mRNA expression of Ferritin, transferrin (Tf), transferrin receptor 1 (TfR1), and nuclear receptor coactivator 4 (NCOA4) by ABI 7500 RT-PCR detection system (Applied Biosystems, Merelbeke, Belgium, USA) using Fast SYBR Green Master Mix. Information of primers was summarized in Table 2. The relative gene expression was quantified by normalizing to the mRNA expression of β-actin as per the 2−ΔΔCt method (15).
Statistical analyses
Data were analyzed by one-way ANOVA using the PROC GLM procedure of the SAS (SAS Inst. Inc., Cary, NC) and all data are presented as mean ± SD. The number of replicate served as the experimental unit. Differences among means were tested by the Fisher's Least Significance Difference test method, and statistical significance was set at P ≤ 0.05.
Results
Growth performance and blood hemoglobin and MCHC levels
No significant differences (P > 0.05) were observed between pigs fed the control diet and those fed the iron-supplemented diets in terms of final body weight, ADG, ADFI, and F/G (Table 3). There were also no significant differences (P > 0.05) in hemoglobin and MCHC contents at all sampling time points between the pigs fed the control diet and those fed Fe-supplemented diets (Table 4).
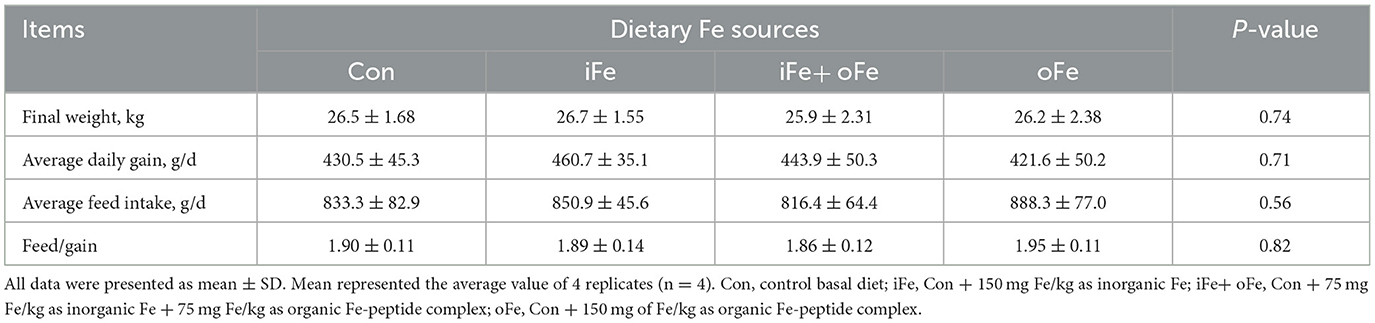
Table 3. Effects of different iron sources on growth performance of weaned piglets from d 28 to d 70.
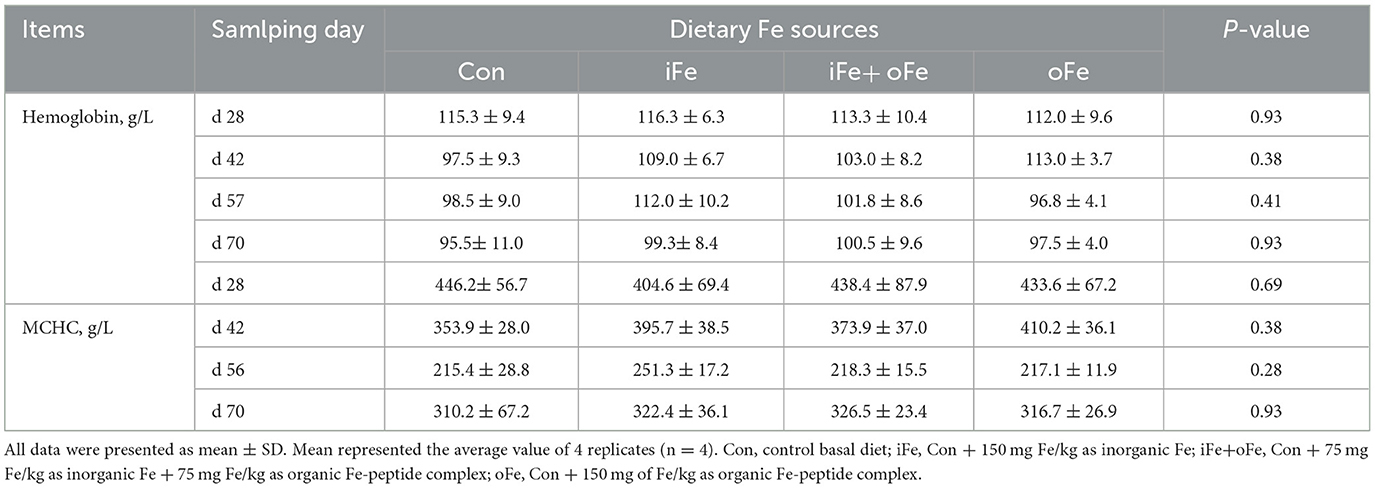
Table 4. Effects of different dietary iron sources on hemoglobin and MCHC levels of weaned piglets from d 28 to d 70.
Tissue iron content and activities of fe-containing enzymes
Compared with the control piglets, piglets in Fe supplemented groups had greater iron concentrations in the liver, kidney and spleen regardless of the iron source (P < 0.05; Table 5), while piglets in oFe group had greater tissue iron concentrations than iFe or iFe+oFe groups (P < 0.05). Piglets in either iFe+oFe or oFe groups showed greater CAT and SDH activities in liver than Con group (P < 0.05; Table 5), while piglets in oFe group had greater CAT and SDH activities in liver than those in iFe group (P < 0.05). There were no significant differences between Con and iFe groups as well as between iFe+oFe and oFe groups. CAT and SDH activities were higher in the oFe than in the Con group in kidney of piglet (P < 0.05), but there were no significant differences between the other groups. There was no difference in the activities of CAT and SDH in spleen among different groups.
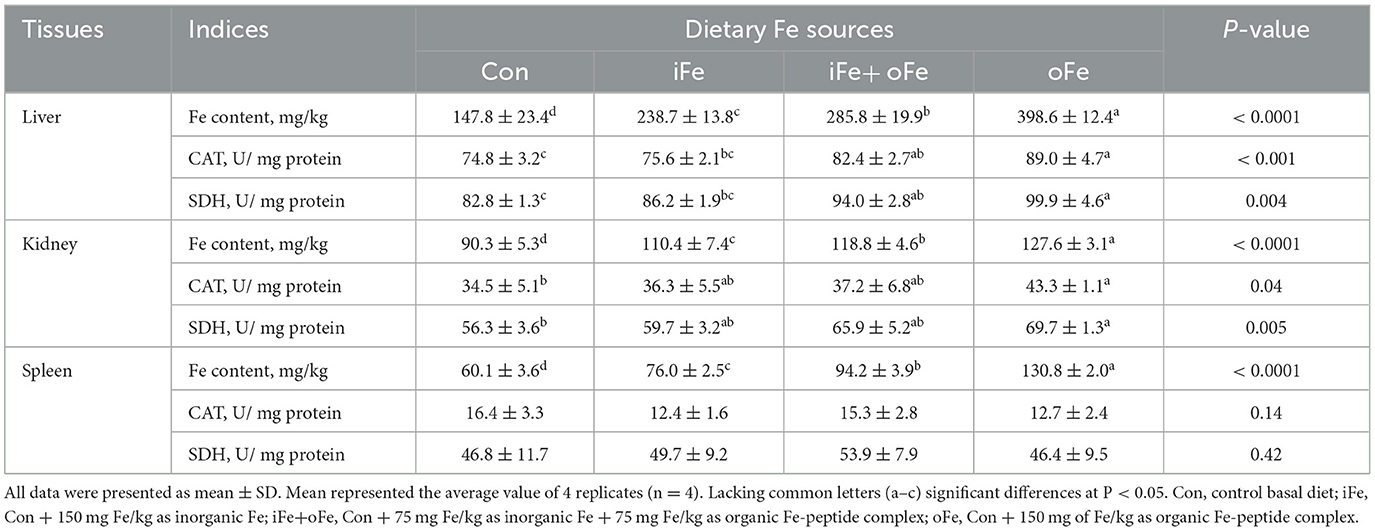
Table 5. Effects of different dietary iron sources on Fe contents and Fe-containing enzyme activities in tissues of weaned piglets at d 70.
Gene expression related to iron homeostasis
Dietary iron supplementation increased (P < 0.05) the expression of NCOA4 mRNA and decreased (P < 0.05) the expression of TfR1 mRNA expression in liver. However, there were no significant differences (P > 0.05) in above gene mRNA expressions in the liver of piglets fed the diets with different iron sources. The TfR1, NCOA4 and Ferritin mRNA expressions of bone marrow were greater (P < 0.05) than that of the control and oFe groups. There were no significant differences (P > 0.05) in the above gene mRNA expressions of bone marrow between the piglets fed the control diet and those fed oFe-supplemented diets.
Discussion
In the present study, no significant effects were observed for growth performance in piglets from d 28 to d 70 after dietary 150 mg Fe/kg supplementation originated by different sources in the corn-soybean meal basal diet. On the one hand, both hepatic iron reserves and the sow's milk are sufficient to maintain growth after weaning. On the other hand, dietary supplementation with no supplementation of iron could provide sufficient iron uptake to meet the iron requirements. As reported previously, the corn-soybean meal basal diet supplemented with 100 mg Fe/kg as FeSO4, Fe-Gly or Heme did not affect the growth performance of weanling pigs aged from d 25 to d 53 (6). However, the inconsistent result showed that the iron-deficient diet (25.8 mg/kg) supplemented with 100 mg/kg ferrous glycine significantly improved growth performance in weanling pigs during the 21-day trial period (16). The discrepancy between the studies may depend on the differences in the supplemental Fe levels, Fe sources, Fe content in basal diets and Fe depletion periods of the piglets. Recently, larger and faster growing pigs have shown signs of anemia earlier because of their increasing tissue growth and the corresponding need for a greater blood volume (3). Therefore, the aged effect of iron sources in hematological parameters was determined in the present study. Several studies have focused on the level of hemoglobin and the cut-off values for anemia in piglets (17, 18). Supplementation of purified or semi-purified diets with different iron contents and sources (~20.2 mg Fe/kg), below requirements, resulted in higher concentrations of hemoglobin and hematocrit in piglets (19) and chick broilers (20). In the present study, there were no significant differences in hematological indices of hemoglobin and MCHC levels between the control diet and iron-supplemented diets during the experimental period, suggesting that hematological indices of piglets may not be sufficient sensitivity required to detect differences of iron status in piglets when a commercial corn-soybean meal diet containing a higher iron content of 75.8 mg Fe/kg is adopted, which are consistent with the results of the previous studies in piglets (7, 17, 21) and broilers (12). Iron depletion using a practical basal diet for 36 days after weaning may be not enough to present a subclinical form of iron deficiency in micro erythrocytes.
Target tissue accumulations of iron have always been considered to be sensitive criteria to assess the iron status and relative bioavailability of iron sources (12, 22). In the present study, supplementation with 150 mg Fe/kg, irrespective of the source of iron deficiency diet, significantly increased iron content in liver, kidney and spleen. In particular, iron retention in tissue increased as the organic iron peptide complexes replaced inorganic iron levels. Iron amino acid complex (23) and iron glycine chelate (24) were more effective in improving iron status of weanling pigs than iron sulfate. Collectively, these results imply that organic Fe-peptide complex may have better bioavailability than inorganic iron salts fed to lactating pigs, due to its high absorption and good stability. Firstly, Organic Fe-peptide complexes are more stable in the digestive tract and less prone to interactions and antagonisms due to binding to organic molecules, and as a result, improve iron efficiency by intestinal iron absorption pathway (25). Zhang et al. (12) and Cao et al. (22) indicated that the relative bioavailability of organic iron sources was closely related to their chelation strength, and organic iron sources with greater chelation strengths showed higher iron bioavailability. Secondly, Fe-peptide complexes have been reported to be absorbed by intestinal peptide absorption pathway (24). Iron is an essential element required for the functions of numerous enzymes, such as CAT, SDH, and so on (26). The activities of enzymes are affected by Fe level (27), and they are used as indices to evaluate iron supplementation efficiency in pigs. In the present experiment, compared with control group, CAT activities in the liver and kidney of pigs increased with dietary Fe supplementation regardless of Fe sources, and the same tendency of SDH activity in liver was observed in broilers (12) and piglets. In addition, piglets fed with oFe diet had greater CAT and SDH activities in kidney than those fed Con diet, with no significant differences observed among other groups. These results indicate the sensitivity of Fe status and the activities of Fe-containing enzymes in tissues over indicators of growth performance and mature erythrocytes in detecting the bioavailability of iron sources in weaned piglets.
Iron uptake, transport and retention are indicators of the efficiency of absorption and bioavailability of supplemental Fe level and sources (4). Hepatic iron stores represent the primary source of iron in response to the metabolic demands of the organism. Ferritin, a ubiquitous and highly conserved iron-chelating protein, is considered as the major iron storage protein that maintains a large iron core in its cavity and has ferroxidase activity (28). However, no significant effect of on hepatic ferritin mRNA expression was observed in pigs between the control and Fe-supplemented groups in the current study, while ferritin mRNA expression in bone marrow was increased in either iFe or iFe+oFe group compared with the control and oFe groups (Table 6). This is because transferrin is saturated, and the highly available free iron form induces ferritin expression. It was speculated that low levels of free iron or high levels of chelated iron were present in bone marrow of piglets fed the control and oFe diets, respectively. In addition, delivery of ferritin to lysosomes required NCOA4 protein to enable cells to use stored iron, which recruits ferritin as a cargo molecule (29). Our results show that there is a decrease in the NCOA4 mRNA expression in liver and marrow under iron depletion. In vitro study revealed that NCOA4-deficient cells were unable to degrade ferritin, leading to a decrease in bioavailable intracellular iron (30). However, NCOA4 mRNA expression was observed to be significantly decreased in the bone marrow of piglet fed oFe diet. This finding could be possibly attributed to the degradation of soluble NCOA4 and ferritin by the macro autophagy pathway in an effort to prevent relative excessive iron storage under prolonged iron repletion by Fe-peptide complex with high bioavailability. Our results suggest that the NCOA4-ferritin axis may be involved in modulating intracellular iron homeostasis in accordance with cellular iron availability. Under physiological circumstances, the major iron uptake route utilized by most cells involves Tf-bound iron, which is bound to TfR1 and then internalized through receptor-mediated endocytosis (31). In the present study, dietary iron supplementation regardless of sources failed to affect Tf mRNA expression in liver and marrow. Translation of TfR1 mRNA in both liver and marrow was increased under iron deficient conditions, which was consistent with the increased tissue Fe accumulation due to the resultant reduction in cellular iron export. The transferring receptor serves as a sensitive indicator of iron deficiency and can be used to provide a reliable index of iron stores.
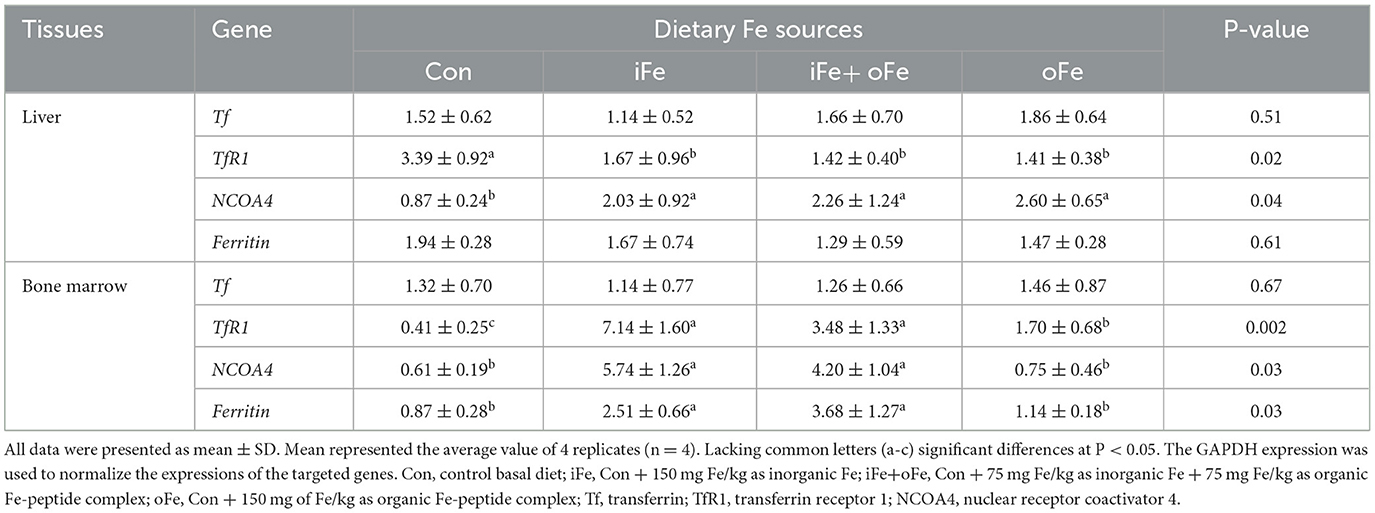
Table 6. Effects of different dietary iron sources on gene expression related to related to iron homeostasis in tissues of weaned piglets at d 70.
Conclusion
In conclusion, the levels and sources of dietary iron supplementation do not influence growth performance and hematological parameters in weaned piglets fed a corn-soybean meal basal diet supplemented with 150 mg Fe/kg from d 28 to d 70. Supplementation of 150 mg Fe/kg in the basal diet regardless of sources increases tissue iron status and activities of Fe-containing enzymes and the addition of organic Fe-peptide complex presents greater beneficial effects on iron bioavailability of weaned pigs at d 70. In addition, the iron homeostasis regulated by iron sources may involve different gene expression patterns related to iron intake and transport in tissues.
Data availability statement
The original contributions presented in the study are included in the article/supplementary material, further inquiries can be directed to the corresponding authors.
Ethics statement
The animal study was reviewed and approved by the Animal Care and Use Committee of South China Agricultural University (SCAU-10564) and the study was conducted following the Regulations for the Administration of Affairs Concerning Experimental Animals.
Author contributions
All authors listed have made a substantial, direct, and intellectual contribution to the work and approved it for publication.
Funding
This study was sponsored by the National Key R&D Program of China (2022YFD1301800 and 1300400), the Key Open Laboratory of Chinese Veterinary Medicine of State Ethnic Affairs Commission and National Local Joint Engineering Research Center for the Separation and Purification Technology of Ethnic Chinese Veterinary Medicine [(2022)09], National Natural Science Foundation of China (31972584), Guangdong Provincial Science and Technology Special Foundation (210723106900762 and 2021020103-2), and China Agriculture Research System (CARS-42-15).
Conflict of interest
JY, JL, Y-HJ, and R-QH were employed by the Guangdong Guangken Animal Husbandry Group Co., Ltd.
The remaining authors declare that the research was conducted in the absence of any commercial or financial relationships that could be construed as a potential conflict of interest.
Publisher's note
All claims expressed in this article are solely those of the authors and do not necessarily represent those of their affiliated organizations, or those of the publisher, the editors and the reviewers. Any product that may be evaluated in this article, or claim that may be made by its manufacturer, is not guaranteed or endorsed by the publisher.
References
1. Szudzik M, Starzyński RR, Jończy A, Mazgaj R, Lenartowicz M, Lipiński P, et al. Iron supplementation in suckling piglets: an ostensibly easy therapy of neonatal iron deficiency anemia. Pharmaceuticals. (2018) 11:128. doi: 10.3390/ph11040128
2. Starzyński RR, Laarakkers CM, Tjalsma H, Swinkels DW, Pieszka M, Styś A, et al. Iron supplementation in suckling piglets: how to correct iron deficiency anemia without affecting plasma hepcidin levels. PLoS ONE. (2013) 8:e64022. doi: 10.1371/journal.pone.0064022
3. Bhattarai S, Framstad T, Nielsen JP. Iron treatment of pregnant sows in a Danish herd without iron deficiency anemia did not improve sow and piglet hematology or stillbirth rate. Acta Vet Scand. (2019) 61:60. doi: 10.1186/s13028-019-0497-6
4. Ding H, Yu X, Feng J. Iron homeostasis disorder in piglet intestine. Metallomics. (2020) 12:1494–507. doi: 10.1039/d0mt00149j
5. Friendship R, Seip V, Amezcua R. A comparison of 4 iron supplementation protocols to protect suckling piglets from anemia. Can Vet J. (2021) 62:55–8. Available online at: https://pubmed.ncbi.nlm.nih.gov/33390600/
6. Zhou Z, Yu X, Li S, Fang S, Feng J. Heme and non-heme iron on growth performances, blood parameters, tissue mineral concentration, and intestinal morphology of weanling pigs. Biol Trace Elem Res. (2019) 187:11–417. doi: 10.1007/s12011-018-1385-z
7. Svoboda M, Ficek R, Drábek J. Reticulocyte indices in the diagnosis of iron deficiency in suckling piglets. B Vet I Pulawy. (2008) 52:125–30. doi: 10.2376/0005-9366-121-78
8. Martin RE, Mahan DC, Hill GM, Link JE, Jolliff JS. Effect of dietary organic microminerals on starter pig performance, tissue mineral concentrations, and liver and plasma enzyme activities. J Anim Sci. (2011) 89:1042–55. doi: 10.2527/jas.2009-2384
9. Bai S, Cao S, Ma X, Li X, Liao X, Zhang L, et al. Organic iron absorption and expression of related transporters in the small intestine of broilers. Poultry Sci. (2021) 100:101182. doi: 10.1016/j.psj.2021.101182
10. Wu W, Yang Y, Sun N, Bao Z, Lin S. Food protein-derived iron-chelating peptides: The binding mode and promotive effects of iron bioavailability. Food Res Int. (2020) 131:108976. doi: 10.1016/j.foodres.2020.108976
12. Zhang LY, Lu L, Luo XG. The chemical characteristics of organic iron sources and their relative bioavailabilities for broilers fed a conventional corn-soybean meal diet. J Anim Sci. (2016) 94:2378–96. doi: 10.2527/jas.2016-0297
13. Association AOAC of Official Analytical Chemists: Official Methods of Analysis. 9th Edn. Arlington, VA: AOAC International. (1990).
14. Góth L. A simple method for determination of serum catalase activity and revision of reference range. Clin Chim Acta. (1991) 196:143–51. doi: 10.1016/0009-8981(91)90067-M
15. Livak KJ, Schmittgen TD. Analysis of relative gene expression data using real-time quantitative PCR and the 2(-Delta Delta C(T)). Methods. (2001) 25:402–8. doi: 10.1006/meth.2001.1262
16. Sun L, Yu B, Luo Y, Zheng P, Huang Z, Yu J, et al. Effects of different sources of iron on growth performance, immunity, and intestinal barrier functions in weaned pigs. Agriculture. (2022) 12:1627. doi: 10.3390/agriculture12101627
17. Wei KQ, Xu ZR, Luo XG, Zeng LL, Chen WR, Timothy MF, et al. Effects of iron from an amino acid complex on the iron status of neonatal and suckling piglets. Asian Austral J Anim. (2005) 18:1485–91. doi: 10.5713/ajas.2005.1485
18. Egeli AK, Framstad T. Evaluation of the efficacy of perorally administered glutamic acid-chelated iron and iron-dextran injected subcutaneously in Duroc and Norwegian Landrace piglets. J Vet Med A. (1998) 45:53–61. doi: 10.1111/j.1439-0442.1998.tb00800.x
19. Ettle T, Schlegel P, Roth FX. Investigations on iron bioavailability of different sources and supply levels in piglets. J Anim Physiol An N. (2008) 92:35–43. doi: 10.1111/j.1439-0396.2007.00707.x
20. Ma XY, Liu SB, Lu L, Li SF, Xie JJ, Zhang LY, et al. Relative bioavailability of iron proteinate for broilers fed a casein-dextrose diet. Poultry Sci. (2014) 93:556–63. doi: 10.3382/ps.2013-03296
21. Cook JD. Diagnosis and management of iron-deficiency anaemia. Best Pract Res Clin Haematol. (2005) 18:319–32. doi: 10.1016/j.beha.2004.08.022
22. Cao J, Luo XG, Henry PR, Ammerman CB, Littell RC, Miles RD, et al. Effect of dietary iron concentration, age, and length of iron feeding on feed intake and tissue iron concentration of broiler chicks for use as a bioassay of supplemental iron sources. Poultry Sci. (1996) 75:495–504. doi: 10.3382/ps.0750495
23. Yu B, Huang WJ, Chiou PWS. Bioavailability of iron from amino acid complex in weanling pigs. Anim Feed Sci Tech. (2000) 86:39–52. doi: 10.1016/S0377-8401(00)00154-1
24. Fang CL, Zhuo Z, Fang SL, Yue M, Feng J. Iron sources on iron status and gene expression of iron related transporters in iron-deficient piglets. Anim Feed Sci Tech. (2013) 182:121–5. doi: 10.1016/j.anifeedsci.2013.03.005
25. Guo L, Harnedy PA, Li B, Hou H, Zhang Z, Zhao X, et al. Food protein-derived chelating peptides: biofunctional ingredients for dietary mineral bioavailability enhancement. Trends Food Sci Tech. (2014) 37:92–105. doi: 10.1016/j.tifs.2014.02.007
26. Pondarré C, Antiochos BB, Campagna DR, Clarke SL, Greer EL, Deck KM, et al. The mitochondrial ATP-binding cassette transporter Abcb7 is essential in mice and participates in cytosolic iron-sulfur cluster biogenesis. Hum Mol Genet. (2006) 15:953–64. doi: 10.1093/hmg/ddl012
27. Feng J, Ma WQ, Xu ZR, He JX, Wang YZ, Liu JX, et al. The effect of iron glycine chelate on tissue mineral levels, fecal mineral concentration, and liver antioxidant enzyme activity in weanling pigs. Anim Feed Sci Tech. (2009) 150:106–13. doi: 10.1016/j.anifeedsci.2008.07.004
28. Torti FM, Torti SV. Regulation of ferritin genes and protein. Blood. (2002) 99:3505–16. doi: 10.1182/blood.V99.10.3505
29. Bogdan AR, Miyazawa M, Hashimoto K, Tsuji Y. Regulators of iron homeostasis: new players in metabolism, cell death, and disease. Trends Biochem Sci. (2016) 41:274–86. doi: 10.1016/j.tibs.2015.11.012
30. Mancias JD, Wang X, Gygi SP, Harper JW, Kimmelman AC. Quantitative proteomics identifies NCOA4 as the cargo receptor mediating ferritinophagy. Nature. (2014) 509:105–9. doi: 10.1038/nature13148
Keywords: weaned pig, iron sources, growth performance, iron status, gene expression
Citation: Huang R-Q, Yang X-J, Xie G-M, Li J, Jian Y-H, Yang J and Zhu Y-W (2023) Effects of dietary iron sources on growth performance, iron status, Fe-containing enzyme activity and gene expression related to iron homeostasis in tissues of weaned pigs. Front. Vet. Sci. 10:1111257. doi: 10.3389/fvets.2023.1111257
Received: 29 November 2022; Accepted: 17 February 2023;
Published: 08 March 2023.
Edited by:
Ilias Giannenas, Aristotle University of Thessaloniki, GreeceReviewed by:
Sabreen Ezzat Fadl, Matrouh University, EgyptJing Wang, Nanjing Agricultural University, China
Panagiotis E. Simitzis, Agricultural University of Athens, Greece
Panagiotis Sakkas, CCPA group, France
Hongkui Wei, Huazhong Agricultural University, China
Copyright © 2023 Huang, Yang, Xie, Li, Jian, Yang and Zhu. This is an open-access article distributed under the terms of the Creative Commons Attribution License (CC BY). The use, distribution or reproduction in other forums is permitted, provided the original author(s) and the copyright owner(s) are credited and that the original publication in this journal is cited, in accordance with accepted academic practice. No use, distribution or reproduction is permitted which does not comply with these terms.
*Correspondence: Jing Yang, 215766253@qq.com; Yong-Wen Zhu, zhuyw0724@scau.edu.cn
†These authors have contributed equally to this work