- 1College of Animal Science and Veterinary Medicine of Henan Institute of Science and Technology, Xinxiang, China
- 2School of Agricultural Sciences, Zhengzhou University, Zhengzhou, China
Introduction: The rapid emergence and widespread spread of multidrug-resistant bacteria is a serious threat to the health of humans and animals. The pharmacokinetic/pharmacodynamic (PK/PD) integration model based on mutant selection window (MSW) theory is an important method to optimize the dosage regimen to prevent the emergence and spread of drug-resistant bacteria. Actinobacillus pleuropneumoniae (AP) is a pathogen that can cause pleuropneumonia in pigs.
Methods: We employed an in vitro dynamic infection model (DIM) to study the prevention of drug-resistant mutations of danofloxacin against AP. A peristaltic pump was applied to establish an in vitro DIM to simulate the PK of danofloxacin in plasma, and to study the MSW of danofloxacin against AP. A peristaltic-pump in vitro infection model was established to simulate dynamic changes in the danofloxacin concentration in pig plasma. PK and PD data were obtained. Then, the relationship between PK/PD parameters and antibacterial activity was analyzed by the sigmoid Emax model.
Results and discussion: The area under the curve during 24 h/ the minimum concentration that inhibits colony formation by 99% (AUC24h/MIC99) had the best-fitting relationship with antibacterial activity. The AUC24h/MIC99 values for a bacteriostatic effect, bactericidal effect, and eradication effect were 2.68, 33.67, and 71.58 h, respectively. We hope these results can provide valuable guidance when using danofloxacin to treat AP infection.
Introduction
Actinobacillus pleuropneumoniae (AP) is a pathogen that can cause pleuropneumonia in pigs. The clinical symptoms are fibrinous hemorrhagic pneumonia and necrotizing pneumonia (1–3). AP infection seriously affects development of the pig industry, and can result in considerable economic losses for farmers (4–7). The common prevention and treatment methods for AP infection are vaccination and drug therapy.
Vaccination is an efficacious means for preventing AP infection (8–10). However, the number of serotypes is large and the cross-protection of each serotype against AP is poor. Hence, developing a universal, stable vaccine that works on all serotypes is very difficult (11, 12). Antimicrobial therapy remains an efficacious way to treat AP infection.
The antibiotics used most commonly to treat AP infection in pigs are ceftiofur, tiamulin, danofloxacin, florfenicol, tilmicosin, and cefquinome. However, non-rational use of antimicrobial agents can result in the emergence and spread of drug-resistant bacteria, which leads to treatment failure (13–16). Development of new antibiotics and optimization of dosage regimens can be employed to address drug-resistance issues. The development of new drugs is time-consuming and cannot keep pace with the rate of bacterial mutations. Hence, optimization of dosage regimens can help to prevent the emergence of drug-resistant bacteria. The pharmacokinetics/pharmacodynamics (PK/PD) integration model based on mutant selection window (MSW) theory is an effective method to optimize dosage regimens to prevent drug resistance.
Dong et al. (17) were the first to propose that the mutant prevention concentration (MPC) is a limitation of the MSW theory. The MPC is defined as the lowest drug concentration that inhibits the growth of insensitive bacterial subpopulations at high bacterial concentrations (bacterial number ≥109 CFU/mL). The minimal inhibitory concentration (MIC) is located in the lower part of the MSW. If the drug concentration is within the MSW (particularly in the lower–middle part of the MSW) and subject to multiple selective pressures, then resistant bacteria are selected over susceptible bacteria (18, 19).
The in vitro dynamic infection model (DIM) is convenient, economic, easy to operate, and can simulate PK and PD in infected target organs. It has important application value in optimizing drug-administration regimens for preventing drug-resistant mutations (20–24). The peristaltic pump is a commonly used in vitro model that can simulate the dynamic changes of drug concentrations and bacteria counts in vivo. This model can be employed to obtain the real-time and continuous antibacterial effect between a drug and bacteria.
Danofloxacin is a third-generation fluoroquinolone used only in animals. PK/PD studies have been carried out in vivo and ex vivo using danofloxacin. However, danofloxacin has not been studied in vitro to obtain real-time and continuous antibacterial concentrations.
Here, a peristaltic-pump model was employed to establish an in vitro infection model to study the prevention of drug-resistant mutations based on the MSW. Our results could provide valuable guidance for formulating dosage regimens if using danofloxacin to treat AP infection in clinical settings to prevent the emergence of drug-resistant mutations.
Materials and methods
Strains, drugs, and instruments
AP (CVCC259) was purchased from Chinese Veterinary Culture Collection Center (Qingdao, China). Danofloxacin mesylate powder (content >99%) was provided by Guangdong Dahuanong Biotechnology (Guangdong, China). Tryptic soy broth (TSB) and Mueller–Hinton Agar (MHA) were obtained from Guangdong Huankai Microbiology Technology (Guangdong, China). Nicotinamide adenine dinucleotide (NAD) was sourced from Beijing Puboxin Biotechnology (Beijing, China). Newborn bovine serum was provided by Guangzhou Ruite (Guangzhou, China).
A peristaltic pump (BT100-1F), pump head (DG-2-B/D; 10 roller), and rubber hose (inner diameter ≤3.17 mm; wall thickness = 0.8–1 mm) were purchased from Longer Precision Pump (Baoding, NC, USA). Fiber dialysis tubes (Float-A-Lyzer® 1,000 kD; 10 mL) were sourced from MilliporeSigma (Burlington, MA, USA).
Determination of the MIC, MIC99 and MPC
MHA and TSB were supplemented with 4% newborn bovine serum and NAD (1 mg/mL).
The MIC was tested by an agar-dilution method according to criteria set by the Clinical and Laboratory Standards Institute (25). Briefly, after being cultured for 8 h in a constant-temperature shaker (180–200 rpm, 37°C), the bacterial suspension was diluted to 106 CFU/mL by TSB. Then, the bacterial suspension (100 μL) was added to an MHA plate containing danofloxacin (0.016–1 μg/mL after twofold dilution). After drying, the MHA plates were placed in an incubator in an atmosphere of 5% CO2 for 18–20 h at 37°C. The MIC was determined as the minimum concentration of drug that did not result in bacterial growth.
Next, we determined MIC99. Briefly, a series of MHA plates containing drugs were prepared based on the MIC (90% × MIC, 80% × MIC, 70% × MIC, 60% × MIC, 50% × MIC). After the logarithmic-phase bacterial suspension had been diluted tenfold (10−1, 10−2, 10−3, 10−4, 10−5, 10−6), the dilutions were inoculated to MHA and cultured as described for determination of the MIC. Then, the bacterial populations were counted and compared between drug-containing plates and the blank plate. Percent recovery growth of bacteria was obtained, and a linear formula between the drug concentration and percent recovery was obtained. MIC99 was determined as the value which inhibited the growth of bacteria by 99% (1% recovery).
We also tested the MPC. Briefly, after being cultured for 8 h, a logarithmic-phase bacterial suspension (100 mL) was centrifuged (5,000 × g, 20 min, 4°C). Then, the supernatant was removed and blank TSB (1 mL) was added for a bacterial population of 1.5 × 1011 CFU/mL. Then, the bacterial solution (100 μL) was inoculated on MHA plates (1 × MIC, 2 × MIC, 4 × MIC, 8 × MIC, 16 × MIC, 32 × MIC, 64 × MIC) and incubation allowed to proceed for 72 h. The minimum concentration of danofloxacin that did not elicit bacterial growth was defined as MPCpr. Then, based on MPCpr, the drug concentration was reduced linearly from 10%MPCpr to 50% × MPCpr, and the procedure repeated as described for measurement of MPCpr. The MPC was defined as the lowest concentration of danofloxacin that could inhibit the growth of bacteria. All tests were repeated thrice.
Establishment of an in vitro DIM
The peristaltic pump that we employed has been described in detail previously (26). A storage chamber, central chamber, and elimination chamber were connected through the peristaltic pump and rubber tube. The storage chamber consisted of a blue-cap bottle (500–5,000 mL) for storage of blank TSB broth. The central chamber comprised a modified three-necked flask containing blank TSB broth (290 mL), a dialysis tube, and magnetic rotor. The three-necked flask consisted of an inlet tube, sampling tube, and outlet tube with rubber stoppers. The sampling tube comprised an elongated syringe needle and nylon filters (0.22 μm) for collection of the TSB sample and contamination prevention. The central chamber was placed in a large beaker with water at a constant temperature (37°C) and magnetic-stirring apparatus (100 rpm). The elimination chamber consisted of a blue-cap bottle (500–5,000 mL) for collection of waste liquid. The dialysis tube contained a bacterial suspension (10 mL) and “floated” in blank TSB and 1-cm above TSB thanks to a foam gasket.
The PK parameter of danofloxacin in pigs was in reference to the work of Yang et al. (27). We set the elimination half-life (t1/2) of danofloxacin at 7 h. The elimination rate constant (Kel) was calculated to be 0.693/t1/2. The flow rate of the peristaltic pump (Q) was calculated as Kel × VC (broth volume in the central chamber and dialysis tube). After the flow rate had been set, the device was run for 2 h to enable stabilization. Then, logarithmic-phase AP (108 CFU/mL) was added to the central chamber. The in vitro DIM was established if the bacterial population stabilized at ~108 CFU/mL.
Kill curves and changes in the MIC
We wished to study the antibacterial effect in different parts of the MSW. Hence, seven dosage groups (0 × MIC99, 1/2 × MIC99, 1 × MIC99, 2 × MIC99, 4 × MIC99, 8 × MIC99, 16 × MIC99) were set up and administrated thrice every 24 h. To balance the drug concentration between the dialysis tube and peripheral chambers rapidly, both compartments were administered drugs to ensure that the drug concentration was identical upon experiment initiation. The bacterial suspension (0.1 mL) was collected from the dialysis chamber with a 1-mL sterile syringe. Then, it was diluted and dropped onto a blank MHA plate for bacterial counting at 0, 3, 6, 9, 12, and 24 h after each dose as well as at 48 and 72 h after the final dose. The limit of detection of the bacterial count was 50 CFU/mL. Each dose was repeated thrice. The kill curve of danofloxacin against AP was drawn as the logarithmic value of the bacterial population at different times.
To detect AP mutants, each sample was plated in MHA containing 1 × MIC of danofloxacin 24 h after each dose as well as 48 and 72 h after the final dose. AP with increasing MICs was passed through five generations in MHA to monitor the stability of the mutant. Then, the MIC of mutant AP was tested as described above.
PK/PD fitting and analysis
The concentration of danofloxacin at different time points was tested by high-performance liquid chromatography, but the data were lost because of damage to software. Therefore, the PK of drugs in the model were simulated using a first-order elimination rate and calculated using Equation 1:
C: drug concentration at time t,
C0: initial concentration of danofloxacin,
K: constant of elimination rate,
t: time of sample collection after drug administration.
The drug concentration at each time point after each dose administration was calculated, and drug concentration–time curves were drawn. Values of area under concentration-time curve (AUC24h) and maximum concentration (Cmax) during 24 h were obtained based on a non-compartment model using WinNonlin (version 5.2.1, Pharsight, MO, USA).
The antibacterial effect (E) was defined as the maximum change in the number pf bacteria during the interval of each administration. The antibacterial effect was split into a bacteriostatic effect (0 log10 CFU/mL), bactericidal effect (3 log10 CFU/mL), and eradication effect (4 log10 CFU/mL).
AUC24h/MIC99 and Cmax/MIC99 were obtained directly by the values of AUC24h and Cmax divided by MIC99. The percentage of time that the drug concentration was above MIC99 during the dosing interval of 24 h (i.e., %T >MIC99) was calculated by PD models using WinNonlin.
The relationship between PK/PD parameters and the antibacterial effect was fitted by an inhibitory sigmoid Emax model by WinNonlin using Equation 2:
E: change in the bacterial count in different drug concentrations after administration of each dose,
Emax: change in the bacterial count in the control group after administration of each dose,
E0: maximum change in the bacterial count in the treatment group after administration of each dose,
Ce: PK/PD parameters, AUC24h/MIC99, Cmax/MIC99, %T >MIC99,
EC50: value of the PK/PD parameter to reach half of Emax,
N: Hill coefficient, the slope of the PK/PD parameter, and E curves.
The fitting relationships between PK/PD parameters and E were expressed by the correlation coefficient (R2). The greater the value, the better was the fitting. PK/PD parameters were calculated to make the bacterial population decrease by 0 log10 CFU/mL, 3 log10 CFU/mL, and 4 log10 CFU/mL.
Results
MIC, MIC99, and MPC
The MIC, MIC99, and MPC were 0.0625, 0.05, and 0.4 μg/mL, respectively.
PK
According to Equation 1, the danofloxacin concentration at each time point was obtained by extrapolation. Concentration–time curves were drawn (Figure 1). The values of Cmax and AUC24h after administration of each dose were obtained using WinNonlin.
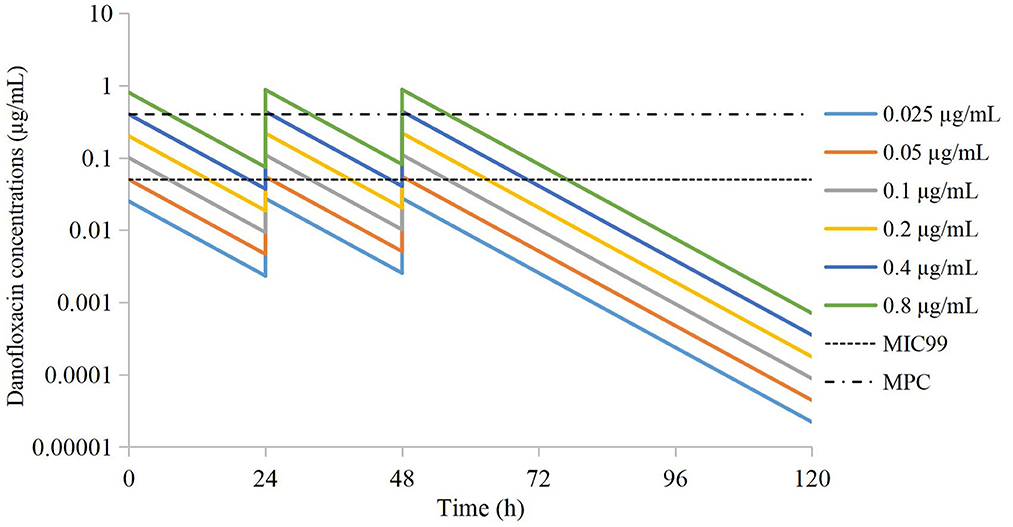
Figure 1. Simulated time-concentration curves of danofloxacin in the peristaltic pump after extrapolation.
Drug concentrations were distributed in different parts of the MSW. The groups of 0.025 μg/mL and 0.05 μg/mL were located outside the MSW. The groups of 0.1 μg/mL and 0.2 μg/mL were located in the lower part of the MSW. The group of 0.4 μg/mL was located in the middle of the MSW. The group of 0.8 μg/mL group was located in the middle and upper parts of the MSW.
In vitro dynamic kill curves
Kill curves at different dosing concentrations are shown in Figure 2. The antibacterial effect after each dose is shown in Table 1. The groups of 0.025 μg/mL and 0.05 μg/mL could produce a bacteriostatic effect. The group of 0.1 μg/mL could reach a bactericidal effect. The group of 0.2 μg/mL could reach an eradication effect.
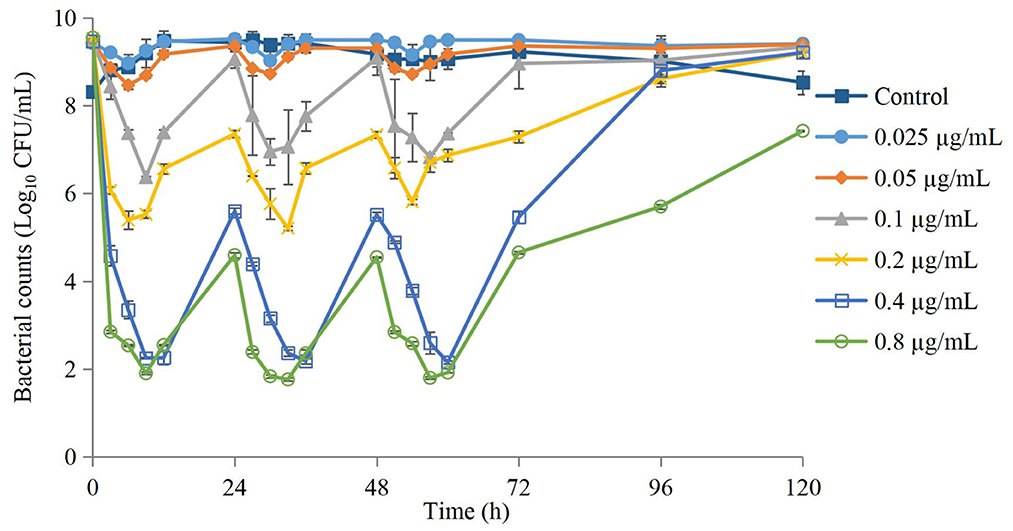
Figure 2. Time-kill curves of danofloxacin against Actinobacillus pleuropneumoniae after administration of different dosages. Values are the mean ±standard deviation (n = 3).
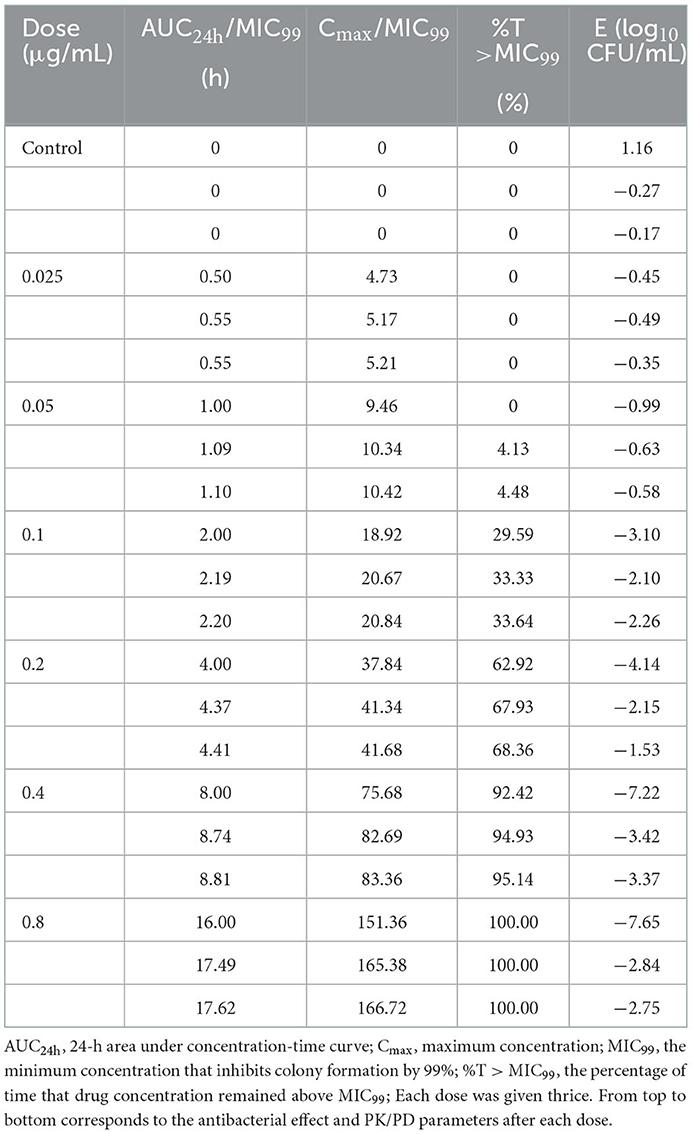
Table 1. Values of the antibacterial effect (E) and PK/PD parameters of danofloxacin against Actinobacillus pleuropneumoniae after three-times administration.
The antimicrobial effect of three-times administration was not significantly different for the group of 0.025 μg/mL compared with that of the control group (Table 1). If the dose >0.025 μg/mL, then the reduction in the bacterial count after the first-time dose was significantly greater than that after the second-time dose and third-time dose. The higher the dose, the greater was the difference, but the difference between the second-time dose and third-time dose was not significant. Changes in the MIC at each dose are shown in Figure 3. The MIC of AP did not change significantly if the drug concentration was lower than MIC99 and higher than the MPC. If the drug concentration was in the middle of the MSW, then the MIC of AP was increased significantly if the frequency of drug administration increased (eightfold increase for the groups of 0.1, 0.2, and 0.4 μg/mL) and recovery to the initial value was observed in the group of 0.05 μg/mL after the final administration.
PK/PD analysis
Using the sigmoid Emax model, AUC24h/MIC99 had the highest correlation with E (R2 = 0.7992) (Figure 4). R2 of %T >MIC99 with E was 0.7935 (Figure 5). The values of AUC24h/MIC99, Cmax/MIC99, %T > MIC99, and E are shown in Table 1. Therefore, AUC24h/MIC99 was selected as the PK/PD parameter to predict the corresponding E. The PK/PD parameters and AUC24h/MIC99 values for different antibacterial effects were obtained (Table 2). The predicted values of AUC24h/MIC99 to produce a bacteriostatic effect, bactericidal effect, and eradication effect were 2.68 h, 33.67 h, and 71.58 h, respectively.
Discussion
Danofloxacin is a third-generation fluoroquinolone used solely in animals. It has a wide range of antibacterial activities. The ex vivo PK/PD of danofloxacin have been studied in ruminants (e.g., sheep, goats, cattle, camels) using tissue-cage infection models, but reports for bacteria that infect pigs are scarce. Although those ex vivo studies reflected the interaction between the host, drugs, and bacteria comprehensively, the drug concentrations were constant. Therefore, a new model is needed to ascertain the influence of dynamic drug concentrations on pathogens.
Previously, we studied the in vivo PK/PD integration of danofloxacin against AP using a tissue-cage infection model. However, the targets of AP are the lungs and blood, so differences exist between tissue fluid and lungs. Considering the high cost and fatality rate using an animal-infection model, establishment of an in vitro infection model to simulate infection of target organs is necessary and valuable.
The peristaltic-pump model can be employed to simulate the dynamic changes in drug concentration and bacterial population in the host in real-time. This strategy provides important support for simulating in vivo PK/PD integration (especially for simulation of difficult-to-obtain target organs). Therefore, we established a peristaltic-pump infection model to study the MSW-based PK/PD integration of danofloxacin against AP in vitro for preventing the emergence and spread of drug-resistant mutant bacteria. In the present study, the PK parameters of danofloxacin in pig blood were referenced with results reported previously (t1/2 = 7.28 ± 1.10 h) (27). We set t1/2 at 7 h after comprehensive consideration of the deviation of different dosing methods and reagents.
Kill curves revealed a marked difference in the antibacterial effect among three-times administration at an identical dosing concentration. In particular, the antibacterial effect of one-time administration was obviously higher than that for two-times and three-times administration. Three main reasons could explain these results. First, the growth rate of mutant AP may be reduced to add its persistence against drugs. Second, a sub-inhibitory concentration of danofloxacin could inhibit the growth of bacteria. Third, the drugs in the bacterial body have antibacterial activity. Therefore, bacteria need a long time to pump-out drugs. Hence, the rate of bacterial growth is reduced and bacterial counts cannot recover to that in the initial population.
The sensitivity of AP decreased if the danofloxacin concentration was between MIC99 and the MPC. These experimental results are consistent with those reported by other investigators (28–31). The main reason is that sensitive bacteria were the main subpopulation in the original population, but a few resistant subpopulations were present. These sensitive bacteria were killed gradually if the drug concentration was between MIC99 and the MPC. After multiple dosing, the resistant subpopulations grew gradually and became the main population that exhibited a higher MIC compared with that of the bacteria in the original population. Therefore, drug concentrations located in the lower part of the MSW should be avoided if dosage regimens are being designed.
If selecting drugs for the treatment of bacterial infections, PK/PD parameters are used often to evaluate the clinical efficacy of antimicrobial agents to prevent the emergence and spread of drug-resistant bacteria (32). For fluoroquinolones, the best-fitting PK/PD parameter related to the antibacterial effect is AUC24h/MIC (33). We also analyzed the relationship between Cmax/MIC99, %T >MIC99, and AUC24h/MIC99, and the antibacterial effect. We discovered that AUC24h/MIC99 and Cmax/MIC99 were correlated more strongly with antibacterial activity (R2 = 0.7992 and 0.7991, respectively) compared with %T >MIC99 (R2 = 0.7935). Hence, we applied AUC24h/MIC99 to analyze the PK/PD parameters between E and calculate the required values of AUC24h/MIC99 to achieve different antibacterial efficacy. The predicted values of AUC24h/MIC99 to produce a bacteriostatic effect, bactericidal effect, and eradication effect were 2.68, 33.67, and 71.58 h, respectively.
Few PK/PD studies of danofloxacin against pathogenic bacteria in pigs have been conducted. The ex vivo PK/PD of danofloxacin against Pasteurella multocida and Haemophilus parasuis in piglet serum were studied by Li et al. (34). The mean values of AUC24h/MIC to produce a bacteriostatic effect and bactericidal effect were 32 h and 49.8 h for P. multocida, whereas they were 14.6 h and 37.8 h for H. parasuis, respectively. Yang et al. (35) studied the ex vivo PK/PD integration of danofloxacin against Escherichia coli in piglet ileum using ultrafiltration probes. The mean values of AUC24h/MIC for ileum ultrafiltrates that achieved a bacteriostatic effect, bactericidal effect, and eradication effect were 99.85, 155.57, and 218.02 h, respectively.
Conclusions and recommendations
We established an in vitro peristaltic-pump infection model to simulate the dynamic changes in danofloxacin concentrations in pig plasma. We obtained real-time and continuous PK data and PD data simultaneously. AUC24h/MIC99 was the best-fitting PK/PD index for the antibacterial effect. The predicted values of AUC24h/MIC99 to produce a bacteriostatic effect, bactericidal effect, and eradication effect were 2.68, 33.67, and 71.58 h, respectively. These results may provide a valuable reference for application of danofloxacin in the treatment of AP infection.
Data availability statement
The raw data supporting the conclusions of this article will be made available by the authors, without undue reservation.
Author contributions
LZ and HW contributed to the methodology, software use, validation, data analysis, writing, and project administration. YiB, YuB, and LW contributed to study supervision, manuscript revision, and funding acquisition. All authors read and approved the final version of manuscript.
Funding
This work was supported by grants from the National Natural Science Foundation of China (grant number 32172862), the National Key R&D Program of China (grant number 2021YFD1301200), the Leading Talents of Scientific and Technological Innovation in the Central Plains (grant number 224200510024), the Outstanding Youth Foundation of Henan Scientific Committee (grant number 222300420043), the Youth Backbone Teacher Project of Colleges and Universities of Henan Province (grant number 2020GGJS162), and the Program for Innovative Research Team (in Science and Technology) in University of Henan Province (grant number 22IRTSTHN026).
Conflict of interest
The authors declare that the research was conducted in the absence of any commercial or financial relationships that could be construed as a potential conflict of interest.
Publisher's note
All claims expressed in this article are solely those of the authors and do not necessarily represent those of their affiliated organizations, or those of the publisher, the editors and the reviewers. Any product that may be evaluated in this article, or claim that may be made by its manufacturer, is not guaranteed or endorsed by the publisher.
References
1. Tonpitak W, Rohde J, Gerlach GF. Prevalence of “Actinobacillus porcitonsillarum” in Porcine tonsils and development of a diagnosis duplex PCR differentiating between “Actinobacillus porcitonsillarum” and Actinobacillus pleuropneumoniae. Vet Microbiol. (2007) 122:157–65. doi: 10.1016/j.vetmic.2007.01.017
2. Stringer OW, Li Y, Bossé JT, Langford PR. JMM Profile: Actinobacillus pleuropneumoniae: a major cause of lung disease in pigs but difficult to control and eradicate. J Med Microbiol. (2022) 71:001483. doi: 10.1099/jmm.0.001483
3. Gale C, Velazquez E. Actinobacillus pleuropneumoniae: a review of an economically important pathogen. Livest. (2020) 25:308–14. doi: 10.12968/live.2020.25.6.308
4. Sassu EL, Bossé JT, Tobias TJ, Gottschalk M, Langford PR, Hennig-Pauka I. Update on Actinobacillus pleuropneumoniae—knowledge, gaps and challenges. Transbound Emerg Dis. (2018) 65:72–90. doi: 10.1111/tbed.12739
5. Tobias TJ, Bouma A, Daemen AJ, Wagenaar JA, Stegeman A, Klinkenberg D. Association between transmission rate and disease severity for Actinobacillus pleuropneumoniae infection in pigs. Vet Res. (2013) 44:1–10. doi: 10.1186/1297-9716-44-2
6. Stringer OW Li Y, Bossé JT, Forrest MS, Hernandez-Garcia J, Tucker AW, et al. Rapid detection of Actinobacillus pleuropneumoniae from clinical samples using recombinase polymerase amplification. Front Vet Sci. (2022) 9:805382. doi: 10.3389/fvets.2022.805382
7. Zhu R, Jiang H, Wang J, Bao C, Liu H, Li F, et al. Dynamic immune response characteristics of piglets infected with Actinobacillus pleuropneumoniae through omic. AMB Express. (2021) 11:1–14. doi: 10.1186/s13568-021-01336-z
8. Loera-Muro A, Angulo C. New trends in innovative vaccine development against Actinobacillus pleuropneumoniae. Vet Microbiol. (2018) 217:66–75. doi: 10.1016/j.vetmic.2018.02.028
9. Jung M, Won H, Shin MK, Oh MW, Shim S, Yoon I, et al. Development of Actinobacillus pleuropneumoniae ApxI, ApxII, and ApxIII-specific ELISA methods for evaluation of vaccine efficiency. J Vet Sci. (2019) 20:e2. doi: 10.4142/jvs.2019.20.e2
10. Dao HT, Shin WS, Do VT, Choi JY, Hahn TW. A Multivalent Vaccine Containing Actinobacillus pleuropneumoniae and Mycoplasma hyopneumoniae Antigens Elicits Strong Immune Responses and Promising Protection in Pigs. J Pure Appl Microbiol. (2021) 15:164–74. doi: 10.22207/JPAM.15.1.11
11. Bosse JT, Li Y, Sarkozi R, Gottschalk M, Angen O, Nedbalcova K, et al. A unique capsule locus in the newly designated Actinobacillus pleuropneumoniae serovar 16 and development of a diagnostic PCR assay. J Clin Microbiol. (2017) 55:902–7. doi: 10.1128/JCM.02166-16
12. Stringer OW, Bosse JT, Lacouture S, Gottschalk M, Fodor L, Angen O, et al. Proposal of Actinobacillus pleuropneumoniae serovar 19, and reformulation of previous multiplex PCRs for capsule-specific typing of all known serovars. Vet Microbiol. (2021) 255:109021. doi: 10.1016/j.vetmic.2021.109021
13. Vanni M, Merenda M, Barigazzi G, Garbarino C, Luppi A, Tognetti R, et al. Antimicrobial resistance of Actinobacillus pleuropneumoniae isolated from swine. Vet Microbiol. (2012) 156:172–7. doi: 10.1016/j.vetmic.2011.10.022
14. Bosse JT Li Y, Rogers J, Crespo RF Li Y, Chaudhuri RR, Holden MTG, Maskell DJ, et al. Whole genome sequencing for surveillance of antimicrobial resistance in Actinobacillus pleuropneumoniae. Front Microbiol. (2017) 8:311. doi: 10.3389/fmicb.2017.00311
15. Bosse JT Li Y, Atherton TG, Walker S, Williamson SM, Rogers J, Chaudhuri RR, et al. Characterisation of a mobilisable plasmid conferring florfenicol and chloramphenicol resistance in Actinobacillus pleuropneumoniae. Vet Microbiol. (2015) 178:279–82. doi: 10.1016/j.vetmic.2015.05.020
16. Da SG, Rossi CC, Santana MF, Langford PR, Bosse JT, Bazzolli DMS. p518, a small floR plasmid from a South American isolate of Actinobacillus pleuropneumoniae. Vet Microbiol. (2017) 204:129–32. doi: 10.1016/j.vetmic.2017.04.019
17. Dong Y, Zhao X, Domagala J, Drlica K. Effect of fluoroquinolone concentration on selection of resistant mutants of Mycobacterium bovis BCG and Staphylococcus aureus. Antimicrob Agents and Ch. (1999) 43:1756–8. doi: 10.1128/AAC.43.7.1756
18. Blondeau JM. New concepts in antimicrobial susceptibility testing: the mutant prevention concentration and mutant selection window approach. Vet Dermatol. (2009) 20:383–96. doi: 10.1111/j.1365-3164.2009.00856.x
19. Zhang L, Xie H, Wang Y, Wang HJ, Hu JH, Zhang GP. Pharmacodynamic parameters of pharmacokinetic/pharmacodynamic (PK/PD) integration models. Front Vet Sci. (2022) 9:860472. doi: 10.3389/fvets.2022.860472
20. Fedrigo NH, Shinohara DR, Mazucheli J, Nishiyama SAB, Carrara-Marroni FE, Martins FS, et al. Pharmacodynamic evaluation of suppression of in vitro resistance in Acinetobacter baumannii strains using polymyxin B-based combination therapy. Sci Rep-UK. (2021) 11:1–12. doi: 10.1038/s41598-021-90709-2
21. Allen GP, Deao KM, Hill SA, Schipelliti SM, Tran T. In vitro evaluation of antimicrobial resistance selection in Neisseria gonorrhoeae. Int J Antimicrob Ag. (2021) 58:106417. doi: 10.1016/j.ijantimicag.2021.106417
22. Pan A, Mei Q, Ye Y, Li HR, Liu B, Li JB. Validation of the mutant selection window hypothesis with fosfomycin against Escherichia coli and Pseudomonas aeruginosa: an in vitro and in vivo comparative study. J Antibiot. (2017) 70:166–73. doi: 10.1038/ja.2016.124
23. Li Y, Feng B, Gu X, Yang DW, Zeng ZL, Zhang BX, et al. Correlation of PK/PD indices with resistance selection for cefquinome against Staphylococcus aureus in an in vitro model. Front Microbiol. (2016) 7:466. doi: 10.3389/fmicb.2016.00466
24. Alieva KN, Strukova EN, Golikova MV, Portnoy YA, Zinner SH, Firsov AA. Time inside the mutant selection window as a predictor of staphylococcal resistance to linezolid. J Antibiot. (2018) 71:514–21. doi: 10.1038/s41429-017-0016-9
25. Clinical and Laboratory Standards Institute. Performance Standards for Antimicrobial Susceptibility Testing; 25th Informational Supplement. Wayne, PA: CLSI Document M100-S25, Clinical and Laboratory Standards Institute (2015).
26. Zhang N, Gu X, Ye X, Wu X, Zhang BX, Zhang LF, et al. The PK/PD Interactions of Doxycycline against Mycoplasma gallisepticum. Front Microbiol. (2016) 7:e44158. doi: 10.3389/fmicb.2016.00653
27. Yang Y, Zhang Y, Li J, Cheng P, Xiao TS, Muhammad I, et al. Susceptibility breakpoint for Danofloxacin against swine Escherichia coli. BMC Vet Res. (2019) 15:1–9. doi: 10.1186/s12917-019-1783-2
28. Cui J, Liu Y, Wang R, Tong W, Drlica K, Zhao X. The mutant selection window in rabbits infected with Staphylococcus aureus. J Infect Dis. (2006) 194:1601–8. doi: 10.1086/508752
29. Xiong M, Wu X, Ye X, Zhang L, Zeng S, Huang Z, et al. Relationship between cefquinome PK/PD parameters and emergence of resistance of Staphylococcus aureus in rabbit tissue-cage infection model. Front Microbiol. (2016) 7:874. doi: 10.3389/fmicb.2016.00874
30. Zhang B, Gu X, Li Y, Li X, Gu M, Zhang N, et al. In vivo evaluation of mutant selection window of cefquinome against Escherichia coli in piglet tissue-cage model. BMC Vet Res. (2014) 10:297. doi: 10.1186/s12917-014-0297-1
31. Zhu Y, Hu L, Mei Q, Cheng J, Liu Y, Ye Y, et al. Testing the mutant selection window in rabbits infected with methicillin-resistant Staphylococcus aureus exposed to vancomycin. J Antimicrob Chemoth. (2012) 67:2700–6. doi: 10.1093/jac/dks280
32. Leroy B, Uhart M, Maire P, Bourguignon L. Evaluation of fluoroquinolone reduced dosage regimens in elderly patients by using pharmacokinetic modelling and Monte Carlo simulations. J Antimicrob Chemoth. (2012) 67:2207–12. doi: 10.1093/jac/dks195
33. Preston SL, Drusano GL, Berman AL, Fowler CL, Chow AT, Dornseif B, et al. Pharmacodynamics of levofloxacin: a new paradigm for early clinical trials. JAMA. (1998) 279:125–9. doi: 10.1001/jama.279.2.125
34. Li J, Niu C, Li X, Feng Y, Sun J, Liu Y, et al. Comparison of PK/PD targets and cutoff values for danofloxacin against Pasteurella multocida and Haemophilus parasuis in piglets. Front Vet Sci. (2022) 9:811967. doi: 10.3389/fvets.2022.811967
Keywords: multidrug resistance, peristaltic pump, PK/PD, mutation selection window, Actinobacillus pleuropneumoniae
Citation: Zhang L, Wang H, Bai Y, Wang L, Bai Y and Hu J (2023) Evaluation of the mutant selection window of danofloxacin against Actinobacillus pleuropneumoniae in an in vitro dynamic model. Front. Vet. Sci. 10:1107608. doi: 10.3389/fvets.2023.1107608
Received: 25 November 2022; Accepted: 11 January 2023;
Published: 30 January 2023.
Edited by:
Nora Mestorino, National University of La Plata, ArgentinaReviewed by:
Yongtao Liu, Chinese Academy of Fishery Sciences (CAFS), ChinaAude A. Ferran, Ecole Nationale Vétérinaire de Toulouse (ENVT), France
Copyright © 2023 Zhang, Wang, Bai, Wang, Bai and Hu. This is an open-access article distributed under the terms of the Creative Commons Attribution License (CC BY). The use, distribution or reproduction in other forums is permitted, provided the original author(s) and the copyright owner(s) are credited and that the original publication in this journal is cited, in accordance with accepted academic practice. No use, distribution or reproduction is permitted which does not comply with these terms.
*Correspondence: Lei Wang, d2xlaV8wMDcmI3gwMDA0MDsxNjMuY29t; Yueyu Bai,
cG9zdGJhaSYjeDAwMDQwO3NvdWh1LmNvbQ==; Jianhe Hu,
amlhbmhlaHUmI3gwMDA0MDsxMjYuY29t
†These authors have contributed equally to this work and share first authorship