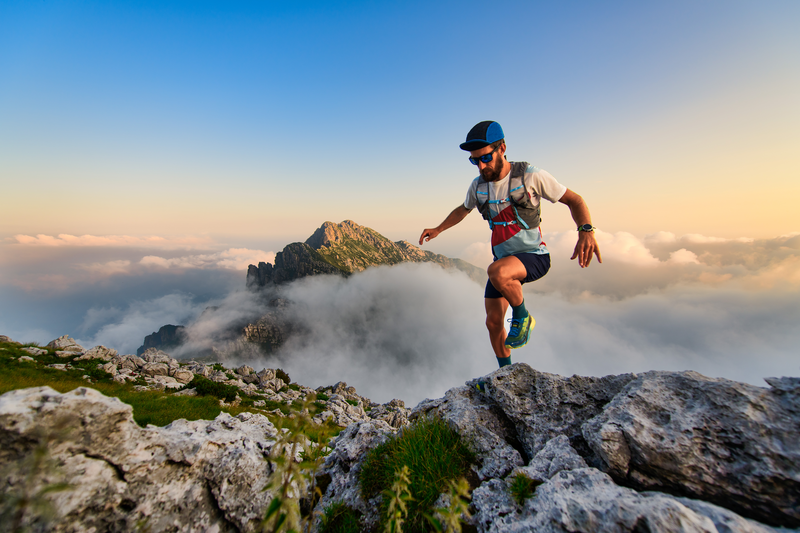
94% of researchers rate our articles as excellent or good
Learn more about the work of our research integrity team to safeguard the quality of each article we publish.
Find out more
ORIGINAL RESEARCH article
Front. Vet. Sci. , 27 January 2023
Sec. Animal Nutrition and Metabolism
Volume 10 - 2023 | https://doi.org/10.3389/fvets.2023.1107149
This article is part of the Research Topic Gut Microbiota: Allied with Livestock Nutrition, Health, And Welfare View all 19 articles
Introduction: The objective of this study was to investigate the effects of dietary supplementation of tributyrin and anise mixture (TA) on growth performance, apparent nutrient digestibility, fecal noxious gas emission, fecal score, jejunal villus height, hematology parameters, and fecal microbiota of weaned pigs.
Methods: A total of 150 21-day-old crossbred weaned pigs [(Landrace × Yorkshire) × Duroc] were used in a randomized complete block design experiment. All pigs were randomly assigned to 3 groups based on the initial body weight (6.19 ± 0.29 kg). Each group had 10 replicate pens with 5 pigs (three barrows and two gilts) per pen. The experimental period was 42 days and consisted of 3 phases (phase 1, days 1–7; phase 2, days 8–21; phase 3, days 22–42). Dietary treatments were based on a corn-soybean meal-basal diet and supplemented with 0.000, 0.075, or 0.150% TA.
Results and discussion: We found that dietary supplementation of graded levels of TA linearly improved body weight, body weight gain, average daily feed intake, and feed efficiency (P < 0.05). TA supplementation also had positive effects on apparent dry matter, crude protein, and energy digestibility (P < 0.05) and jejunal villus height (P < 0.05). The emission of ammonia from feces decreased linearly with the dose of TA increased (P < 0.05). Moreover, TA supplementation was capable to regulate the fecal microbiota diversity, manifesting in a linearly increased Chao1 index and observed species and a linearly decreased Pielou's index (P < 0.05). The abundance of Lactobacillus reuteri, Lactobacillus amylovorus, Clostridium butyricum were increased, while the abundance of Prevotella copri was decreased, by treatment (P < 0.05). Therefore, we speculated that TA supplementation would improve growth performance and reduce fecal ammonia emission through improving nutrient digestibility, which was attributed to the increase of jejunal villus height and the regulation of fecal microbiota.
Post-weaning is a critical phase in swine husbandry. During this period, weaned pigs will encounter challenges in intestinal microflora disorder and/or gastrointestinal tract dysfunction, which will limit their digestion and absorption capacity, resulting in growth retardation and/or diarrhea (1, 2). For decades, antibiotics have been used to cope with the post-weaning challenges in swine husbandry (3). However, due to antibiotic resistance, the use of antibiotics in livestock has been considered as a threat to the safety in animal production and human health (4). For this reason, seeking suitable alternatives to antibiotics has always been the direction in animal husbandry research.
Tributyrin, also known as tributyrate glyceride, is a triglyceride containing 3 butyrate molecules, which is regarded as a precursor of butyrate (5, 6). When taken orally, it can directly release into the hindgut (7), thus exerting several biological effects such as regulating intestinal microflora (8, 9), improving intestinal health (2, 6), enhancing muscle development (10, 11), alleviating antioxidant stress (12, 13), promoting nutrients absorption (14), and ameliorating growth retardation (13, 15).
When feeding animals with a mixture of tributyrin and herb-derived extract, an increase in growth performance and a decrease in intestinal harmful bacteria were observed (16). Chen et al. (17) found that tributyrin and herb-derived extract mixture supplementation was capable to improve growth performance, maintain intestinal mucosal integrity, and regulate intestinal microbiota in weaned pigs.
Anise has long been used as an aromatic for mammals in order to induce imprinting effects (18, 19). Anise is an important traditional Chinese medicine. Bioactive compounds presented in anise, such as anethole, estragole, limonene, pinene, β-phellandrene, and α-terpineol allows it anti-inflammatory (20, 21), bacteriostatic (22, 23), antioxidant (22, 23), resist pathogenicity bacterial infection (21), and growth promotion (24, 25) characteristics. Therefore, it is reasonable to believe that the combination of tributyrin and anise has positive effects on the performance of weaned pigs.
However, no study has investigated the effects of tributyrin and anise mixture (TA) supplementation on the productive performance of pigs.
The technique of 16S rRNA high-throughput sequencing provides a reliable method for bacterial identification. The gene of 16S rRNA is a conserved sequence region that exists in all bacteria and can be targeted by broad-range polymerase chain reaction (PCR) primers (26). The 16S rRNA sequencing has been used to describe the species composition of various communities in the study of bacterial diversity (27). Therefore, we use the technique of 16S rRNA high-throughput sequencing to investigate the effects of TA supplementation on intestinal microbiota of weaned pigs. We hypothesized that feeding weaned pigs with TA containing diet had positive effects on jejunal villus height and the abundance of beneficial bacteria in intestinal microbiota, so as to improve growth performance by increasing nutrient digestibility, limiting fecal noxious gas emission, reducing fecal score.
A total of 150 21-day-old crossbred weaned pigs [(Landrace × Yorkshire) × Duroc] with an average initial body weight of 6.19 ± 0.29 kg were used in a completely randomized block design experiment. The protocol (DK-1-2034) of this study was approved by the Animal Care and Use Committee of Dankook University (Cheonan, South Korea).
Based on the initial body weight, all pigs were randomly assigned to three groups. Each group had 10 replicate pens with five pigs (three barrows and two gilts) per pen. The experimental period was 42 days and divided into 3 phases (phase 1, days 1–7; phase 2, days 8–21; phase 3, days 22–42). Dietary treatments were based on a corn-soybean meal-basal diet, which were formulated to meet the nutrient requirements of the NRC (28), and supplemented with 0.000, 0.075, or 0.150% TA to form control, TRT1, and TRT2 groups. The chosen dose was determined in a preliminary study. Feed ingredients and analyzed nutrient composition of the basal diet were shown in Table 1. The commercial TA (ElanPlus® TB50) used in this study was obtained from Olus Plus BV (8 Randweg, Hasselt, The Netherlands). The additive was composed of 50% tributyrin, 5% anise coated by potato starch (10% w/v), and 45% Vehicle q.s. (Silica).
All pigs were housed in an environmentally controlled room. The temperature during week 1 was maintained at 30°C and then gradually reduced by 1°C every week to maintain in 24°C. The relative humidity within the room was 60%. The room was equipped with a mechanical ventilation system and the floor was slatted plastic. A nipple drinker was installed in each pen to ensure that pigs could drink freely. In addition, stainless steel self-feeders were installed on one-side of the pens to ensure that pigs had free access to feed. All pigs were allowed ad libitum access to feed and water.
On days 7, 21, and 24, representative feed samples were taken to analyze feed composition. Feed samples were dried in an oven with 70°C for 72 h, and later they were ground to pass through a 1-mm sieve and collected. Powder feed samples were analyzed for dry matter (DM; method 930.15), crude protein (CP; nitrogen × 6.25; method 968.06), calcium (method 984.01), phosphorus (method 965.17), and crude fat (method 954.02) following the procedures established by AOAC (29). The lysine and methionine contents in the diet were measured using an AA analyzer (Beckman 6300; Beckman Coulter, Inc., Fillerton, CA). The combustion heat was measured by a bomb calorimeter (Parr 6100; Parr Instrument Co., Moline, IL, USA) to determine the energy content in the feed sample.
Individual body weight of pigs was measured on days 1, 7, 21, and 42. Data of body weight was pooled on a pen basis to determine average daily gain (ADG) during days 1–7, 8–21, 22–42, and 1–42. Pen-based feed intake was measured daily to calculate the average daily feed intake (ADFI) during days 1–7, 8–21, 22–42, and 1–42. The feed efficiency (gain to feed ratio) during days 1–7, 8–21, 22–42, and 1–42 was calculated using ADG and ADFI values.
During days 1–7, 14–21, and 35–42, 0.20% chromium oxide as an indigestible marker was added to the diet of each group for measuring apparent nutrient digestibility. On day 7, 21, and 42, two pigs (one barrow and one gilt) were randomly selected from each replicate pen for fecal taking (about 250 g) by the rectal massage method. Fecal samples were dried in an oven with 70°C for 72 h. After that, samples were ground into powder, which can pass through a 1-mm sieve, and be collected in duplicate. Fecal samples were analyzed for DM (method 930.15) and CP (nitrogen × 6.25; method 968.06) following the procedures established by AOAC (29). The combustion heat was measured by a bomb calorimeter (Parr 6100; Parr Instrument Co., Moline, IL, USA) to determine the energy in feces. The chromium levels were analyzed via UV absorption spectrophotometry (UV-1201, Shimadzu, Kyoto, Japan). The apparent total tract digestibility was calculated according to the equation provided by Liu and Kim (30).
Two pigs per pen (one barrow and one gilt) were selected randomly and euthanized with an intravenous injection of 3 mg/kg body weight of chlorpromazine hydrochloride injection on day 42. The entire intestine of euthanized pigs was then removed and dissected free of mesenteric attachments and placed on a smooth and cold surface. The jejunum was separated. The isolated intestinal segments were immediately opened lengthwise following the mesentery line and flushed with ice-cold saline. Approximately 2 cm segments of the jejunum at consistent locations were collected immediately, fixed in 10% formalin, then subsequently embedded, sectioned and stained with hematoxylin and eosin by routine methods. Villus height of the jejunum was measured in ~10 microscopic fields using an image analysis system by a blinded investigator.
Fecal scores of pigs were recorded daily using the scoring system proposed by Hashem and Shehata (31) in the first week post-weaning.
On the last day of the experiment, before euthanizing the pigs used above, they were bled for collecting blood samples via anterior vena cava puncture into non-heparinized vacuum tubes (Becton Dickinson Vacutainer Systems, Franklin Lakes, NJ, USA). The blood samples were collected during 11:00 to 12:00 h in order to exclude the circadiurnal fluctuations in hormone concentrations. Pigs did not receive any feed before sampling. Blood samples were centrifuged (3,000 × g) for 15 min at 4°C to obtain serum samples and then stored at −20°C until analysis. The concentrations of total protein and albumin were assayed using colorimetric methods. Additionally, globulin levels were evaluated through the difference between total protein and albumin. Serum total cholesterol, triglyceride, and high-density lipoprotein cholesterol (HDL-C) concentrations were determined enzymatically using reagent kits (Wako Pure Chemical Industries Ltd., Tokyo, Japan).
On day 7, 21, and 42, the method of rectal massage was used to take fecal samples (about 300 g) from two randomly selected pigs (one barrow and one gilt) in each pen for measuring fecal ammonia (NH3), hydrogen sulfide (H2S), acetic acid, carbon dioxide (CO2), and total mercaptans (R-SH) emission by the method provided by Dang et al. (32). The fecal samples from the same pen were pooled, mixed, and transported to lab for further analysis of fecal noxious gas emission.
Fresh stool samples were taken from 15 pigs (five pigs/group) after feeding phytogenic TA additives for 6 weeks. The specimens were kept in liquid nitrogen until they arrived at the laboratory. Metagenomic DNA (mDNA) from 15 fecal samples was extracted using QIAamp Power Fecal Kit (Qiagen, Germany). The mDNA extraction experimental steps were performed according to the manufacturer's instructions with the inclusion of a homogenization step in which 100 mg fecal samples were pooled in 1.4 ml lysis buffer. When the samples were thoroughly homogenized, tissue lysis stages were implemented for 6 min at 30 Hz, followed by the ending process at 95°C for 5 min. The extracted mDNA was eluted in 100 μl with buffer provided in the kit. Thereafter, the quality check of all mDNA samples was conducted using NanoDrop One (ThermoFisher Scientific, USA), and all samples were stored at 4°C until the next process. All PCR steps were performed with 2X KAPA HiFi Hot Start Ready Mix (Roche, Germany). A primer pair suitable for the 16S V3–V4 amplification was used, and the sequences were as follows: 341F forward primer is 5′-TCGTCGGCAGCGTCAGATGTGTATAAGAGACAG-3′. 806R reverse primer is 5′-GTCTCGTGGG CTCGGAGATGTG TATAAGAGACAG-3′. After PCR amplification, all amplicons were purified with the AMPure XP beads (Beckman Coulter, USA). The second PCR amplification was then conducted at lower cycles to add the Illumina adapter and multiplexing indices included in Nextera XD Index (Illumina, USA). All second PCR products were purified with AMPure XP beads once again. The final amplicon products were pooled with normalized concentration, and the library size was checked using the TapeStation system (Agilent, CA, USA). Finally, high-throughput amplicon sequencing was carried out using the Illumina Miseq™ paired-end (2 × 300) platform (Illumina, USA).
Sequencing reads created from microbial 16S V3–V4 regions were demultiplexed by the split_libraries_fastq.py function of QIIME 2, a next-generation microbiome bioinformatics platform (33). The sequences were then trimmed using the Divisive Amplicon Denoising Algorithm 2 (DADA2) plugin which detects and corrects amplicon errors. Also, sequence quality was controlled with DADA2 by filtering out PhiX chimeric sequences. Sequences including ambiguous base calls and <100 bp were trimmed to minimize the random errors. After the denoising step, the data was specified with pre-trained Naïve Bayes classifier artifact using the machine learning Python library scikit-learn in the QIIME2 pipeline. The classifier artifact was trained on SILVA database v138 which is trimmed to include only V3–V4 regions, pre-clustered with 99% sequence identity, and classified bacterial taxonomy using a 70% confidence threshold (default).
For all samples, alpha and beta diversity analyses were performed with the “diversity” QIIME2 algorithm to measure changes or differences in microorganisms. The alpha-diversity was estimated by observed ASVs, Chao1, Shannon's abundance, and Pielou's evenness indices, indicating microbial richness and evenness measures within a single sample. The Kruskal–Wallis non-parametric test was then calculated to identify statistical differences among the three groups. The beta-diversity measures were estimated using principal coordinate analysis (PCoA) of both Unweighted UniFrac and Bray-Curtis abundance dissimilarity metrics with the non-parametric permutational multivariate analysis of variance (PERMANOVA) test which is used to compare the differences between the groups. All non-parametric statistical analyses were conducted by GraphPad PRISM v8 (GraphPad Software. Inc., CA, USA), and the P-value < 0.05 was considered statistically significant. All visualization data was created using R bioinformatics packages.
A relative abundance analysis was carried out to determine the relative frequency of bacteria from phylum to species level of each group. The Kruskal–Wallis non-parametric test was also calculated to confirm statistical differences among three groups. Furthermore, we visualized the statistically significant species of the three groups with the Venn diagram (P < 0.05). The heatmap visualization was performed on 15 species among the shared species shown in the Venn diagram except for the unclassification and uncultured bacteria using R studio version 4.1.2. Finally, we compared the relative frequency of the representative four species to analyze the linear effects of the phytogenic additive on the weaning pigs.
Before the analysis, all the percentage data were transformed by arcsine transformations. Data were then subjected to statistical analysis in a randomized complete block design using the General Linear Models procedures (SAS Institute, Cary, NC, USA). The normality of data was examined by the Shapiro–Wilk test and QQ plots. The replicate pen was used as the experimental unit. Orthogonal polynomials were used to assess the linear and quadratic effects of storage duration. Differences among groups were evaluated by the one-way ANOVA for multiple comparisons. Variability in the data was expressed as the pooled standard error of means (SEM). P < 0.05 was considered statistically significant.
In comparison to control group, feeding weaned pigs with 0.15% TA containing diet led to higher body weight on day 42 (P = 0.038), ADG during days 8–21 (P = 0.012), 22–42 (P = 0.009), and 1–42 (P = 0.006), ADFI during days 22–42 (P = 0.040) and 1–42 (P = 0.028), and feed efficiency during days 1–42 (P = 0.034). Above growth performance parameters were increased linearly with the dose of TA increased. Moreover, no significant difference in fecal score has been observed among groups (Table 2).
Table 2. Effects of tributyrin and anise mixture (TA) supplementation on growth performance and fecal score of weaned pigs1.
Apparent DM digestibility on day 7 (P = 0.008), day 21 (P = 0.043), and 42 (P = 0.029), apparent CP digestibility on day 42 (P = 0.033), and apparent energy digestibility on day 7 (P = 0.002), day 21 (P = 0.028), and 42 (P = 0.010) were the highest in the group of TRT2 in comparison to other groups and they were increased linearly with the dose of TA increased in the diet. Additionally, feeding weaned pigs with graded levels of TA containing diet increased jejunal villus height (Figure 1) in a dose-dependent manner (linearly; P = 0.043), of which the highest value was presented in TRT2 group (Table 3).
Figure 1. Slice of the jejunum in weaned piglets fed the diet supplemented 0.000 (A), 0.075 (B), or 0.150 (C) % tributyrin and anise mixture.
Table 3. Effects of tributyrin and anise mixture (TA) supplementation on apparent nutrient digestibility and jejunal villus height of weaned pigs1.
The concentrations of serum total protein, albumin, globulin, cholesterol, triglyceride, and HDL-C did not differ among groups (Table 4).
Table 4. Effects of tributyrin and anise mixture (TA) supplementation on hematology parameters of weaned pigsa.
Weaned pigs fed the diet supplemented with TA linearly decreased fecal NH3 emission on day 42 (P = 0.040), while did not affect the emissions of H2S, R-SH, acetic acid, and CO2. Moreover, the emission of NH3 in control group was lower than that in TRT2 group (Table 5).
Table 5. Effects of tributyrin and anise mixture (TA) supplementation on fecal noxious gas emission of weaned pigs1.
The average number of total raw reads for 16S metagenome sequencing was 189,215 and the average number of filtered reads, denoised reads, merged reads, and non-chimeric reads were 39,551, 35,450, 23,394, and 14,794, respectively. We confirmed that non-chimeric read data was sufficient enough to analyze the microbiome and the number of ASVs taxonomy classified by SILVA v138 reference database with a >70% confidence threshold was 1,045.
We performed the alpha-diversity analysis to analyze in abundance and evenness differences of each group. The alpha-diversity was measured using Chao1 index, Observed species, Shannon index, and Pielou's index (Table 6). As a result of diversity estimation, observed Chao1 index (P = 0.042) and observed species (P = 0.044) measures linearly increased when feeding weaned pigs with graded levels of TA containing diet. In contrast, Pielou's evenness estimated scores linearly decreased with the dose of TA increased, showing a significant statistical value (P = 0.020). Additionally, the highest value of Chao1 index and Observed species were presented in TRT2 group, whereas the lowest value of Pielou's index was presented in TRT1 and TRT2 groups, in comparison to other groups.
Table 6. Effects of tributyrin and anise mixture (TA) supplementation on diversity and abundance indexes in fecal microbiota of weaned pigs1.
We analyzed beta-diversity to affirm the change of species in each group by coordinates analysis (PCoA) using phylogenetic qualitative Unweighted UniFrac (A) and Bray-Curtis (B) abundance dissimilarity distance (Figure 2). We found that both indices showed statistical importance (P = 0.001) based on the PERMANOVA test. In addition, pseudo-F, which indicates the ratio of the between-cluster variations to the within-cluster variation, showed higher scores in Bray-Curtis than Unweighted UniFrac (Table 7).
Figure 2. Beta-diversity analysis of the three groups between each weaning pigs (n = 5). Microbial beta-diversity analysis of the three groups is measured by phylogenetic qualitative Unweighted UniFrac (A) and Bray-Curtis (B) abundance dissimilarity distance matrix for all 15 samples. The colored circular clusters denote the control (red), TRT1 (blue), and TRT2 (green). Control was defined as the weaned pigs fed the basal diet. TRT1 was defined as the weaned pigs fed the diet supplemented with 0.075% tributyrin and anise mixture. TRT2 was defined as the weaned pigs fed the diet supplemented with 0.150% tributyrin and anise mixture.
We confirmed a relative bacterial frequency at the genus level based on the taxonomy classification results using SILVA v138 16S rRNA gene database (Figure 3). The 10 most abundant bacteria among groups were mainly involved in Subdoligranulum, Muribaculaceae, Megasphaera, Faecalibacterium, Prevotellaceae_NK3B31_group, Blautia, Agathobacter, Clostridium_sensu_stricto_1, Lactobacillus, and Prevotella.
Figure 3. Relative bacterial abundance of the groups at the genus level (n = 5). The relative abundance box plots indicate the composition of the three groups at the genus levels which are classified based on the 16S V3–V4 metagenome profiling. Each graph bar is ordered from the highest proportion. Control was defined as the weaned pigs fed the basal diet. TRT1 was defined as the weaned pigs fed the diet supplemented with 0.075% tributyrin and anise mixture. TRT2 was defined as the weaned pigs fed the diet supplemented with 0.150% tributyrin and anise mixture.
In order to accurately determine the effects of the TA diets on the gut microbiome, we first selected the species showing statistical significance, and 106 species were identified in the three groups (Supplementary Table 1). Then, we identified the species of each group using the Venn diagram and classified three species, five species, and 11 unique species in the control, TRT1, and TRT2 groups, respectively. There were also three species shared between the control and TRT1 groups, common two species between the control and the TRT2, and five species between the TRT1 and TRT2 groups. In line with the purpose of the study, we focused on 64 common species in the three groups (Figure 4). Finally, we compared the relative abundance of the 15 species, which was converted into a z-score using a heatmap, excluding ambiguous species (e.g., unclassification or uncultured_bacterium; Figure 5A). Among the 15 species, we picked representative four species to investigate the effects of the dietary additive. The abundance of Lactobacillus reuteri in TRT2 group was higher than that in control and TRT1 groups (P < 0.05). The abundance of Lactobacillus amylovorus and Clostridium butyricum in TRT1 and TRT2 groups were higher than that in control group (P < 0.05). Moreover, the abundance of Prevotella copri was significantly reduced by treatment, of which higher value was observed in TRT2 group in compared to that in TRT1 group (Figure 5B).
Figure 4. Venn diagram visualization at the species level. The Venn diagram represents the species with statistical significance in the three groups. Among the 106 species, we select 64 species shared by all three groups to analyze the linear effect of the additive. Control was defined as the weaned pigs fed the basal diet. TRT1 was defined as the weaned pigs fed the diet supplemented with 0.075% tributyrin and anise mixture. TRT2 was defined as the weaned pigs fed the diet supplemented with 0.150% tributyrin and anise mixture.
Figure 5. Analysis of bacterial relative abundance at the species level (n = 5). (A) Heatmap plot shows the changes in the relative abundance of the shared 15 species of each weaning pig except for ambiguous species. The relative frequency of the shared bacteria is converted to z-score value. (B) A bar chart presents the four species' relative frequency. Control was defined as the weaned pigs fed the basal diet. TRT1 was defined as the weaned pigs fed the diet supplemented with 0.075% tributyrin and anise mixture. TRT2 was defined as the weaned pigs fed the diet supplemented with 0.150% tributyrin and anise mixture. a − cMeans in the same figure with different superscript differ significantly (P < 0.05).
In the present study, feeding weaned pigs with graded levels of TA containing diet had positive effects on growth performance. Body weight and ADG as important economic parameters are closely related to ADFI and feed efficiency. A high ADFI ensures a good nutrition supply. A high feed efficiency allows good absorption of nutrients from the feed. It is reported that tributyrin supplementation was capable to regulate appetite (8, 13, 34). Gu et al. (8) reported that feeding lipopolysaccharide-challenged weaned pigs with 0.6 g/kg tributyrin containing diet prevented growth retardation by stimulating appetite. Wang et al. (13) noted that dietary supplementation of 0.75 g/kg tributyrin increased ADFI and ADG in diquat-challenged weaned pigs. However, the mechanism of tributyrin promoting feed intake is still unclear, which is probably related to butyrate systemic circulation mediated by tributyrin (7, 35, 36). In addition, dietary supplementation of aromatic substances has been reported to increase the appetite, thus promoting voluntary feed intake and further improving growth performance (25, 37). The flavor of trans-anethole is described as sweet (38), and its chemosensory features could improve palatability and feed preferences of weaned pigs by exerting pleasant sensations (37). Charal (23) reported that feeding weaned pigs with 50 mg/kg anise oil containing diet had positive effects on feed intake and growth performance. Dang et al. (19) noted that the ADG of weaned pigs increased by anise-containing mixture supplementation, which was attributed to the promotion of feed intake. Therefore, as the components of TA, the tributyrin and anise were capable to regulate appetite, which partially contributed to the improvement of growth performance. Additionally, Hou et al. (14) reported that feeding 5 g/kg tributyrin containing diet to weaned pigs was capable to increase feed efficiency and then improve their body weight. Sotira et al. (39) noted that weaned pigs fed the diet supplemented with 2 g/kg tributyrin increased feed efficiency, thus improving ADG. In addition, the supplementation of biochemical substances from anise also has been reported to improve the feed efficiency of weaned pigs (21, 23). Charal (23) reported that the supplementation of anise oil increased the growth of weaned pigs in nursery stages, and had a positive effect on feed efficiency. Yi et al. (21) noted that supplementing 300 mg/kg anethole to Enterotoxigenic Escherichia coli-infected weaned pigs increased feed efficiency. The improvement of digestibility, utilization rate, and retention rate of energy and nutrients partly explain the improvement of feed efficiency in pigs (40, 41). In this study, weaned pigs fed the diet supplemented with TA had positive effects on apparent nutrient digestibility. Similarly, some studies have reported that the supplementation of tributyrin was beneficial to improve nutrient digestibility in weaned pigs (42–44). However, no study has investigated the effects of anise supplementation on nutrient digestibility in weaned pigs. Therefore, we considered that dietary supplementation of TA had positive effects on nutrient digestibility, feed efficiency, and ADFI, and therefore contributed to the improvement in body weight and ADG.
It is reported that manipulating intestinal microbiota communities is a strategy to improve nutrient digestibility and/or feed efficiency (45, 46). Our diversity (alpha and beta) results demonstrated that the TA treatment explicitly influenced the composition of the weaning pigs' gut microbiome, especially in the aspects of evenness and abundance. The classification results at the genus level showed that the microbiome transition was related to additive treatments to some extent. However, species-level classification was further necessary because the genera have functions in both beneficial and pathogenic ways depending on the specific species. Therefore, we first selected 64 species shared by the three groups and selected 15 species showing statistical significance except for unclassified and uncultured bacteria. Finally, four representative species were analyzed to measure the effects of the additive. We found that the abundance of L. reuteri, L. amylovorus, and C. butyricum were positively, whereas P. copri was negatively, affected by treatment. A substantial number of studies have shown that L. reuteri and L. amylovorus in the intestine of pigs had positive effects on growth performance and intestinal health (e.g., gut integrity, nutrient digestion) (47–49). In particular, L. reuteri is crucial commensal bacteria in pigs that produce exopolysaccharides to increase intestinal adhesion and inhibit the proliferation of E. coli, thereby promoting growth performance (50, 51). Further, reutericyclin, produced by L. reuteri, is capable to prevent Clostridium difficile infection (52). Shen et al. (49) also reported that L. amylovorus showed the characteristics of probiotics in livestock production. Based on these previous studies, we speculated that the additive had a positive effect on weaned pigs by increasing Lactobacillus species. In addition to the two species, we also found a more than 20-fold increase in C. butyricum and an approximately half decrease in P. copri in the treatment groups. Studies have examined the positive effects of C. butyricum on intestinal morphology, intestinal microflora balance, and growth performance (53, 54). Moreover, Clostridium butyiricum, a butyric acid producer, is capable to provide energy to intestinal epithelium (55) and beneficial to improve nutrient digestibility (56). Regarding P. copri, it is capable to induce dysbiosis, reduce short-chain fatty acids, and increase the risk of colitis (57–61). Additionally, research indicated that the P. copri is a potential pathogen that causes a series of metabolic diseases (62). Therefore, the increase of beneficial bacteria such as L. reuteri, L. amylovorus, and C. butyricum as well as the decrease of harmful bacteria such as P. copri caused by TA supplementation was beneficial to the improvement of nutrient digestibility and/or feed efficiency, and therefore contributed to the improvement of growth performance.
On the other hand, the physiology of the intestinal tract is the cornerstone for ensuring high nutrient digestibility and/or feed efficiency (63, 64). The villus height condition is related to the ability of nutrient absorption (42, 65). The supplementation of tributyrin to the diet has been reported to increase the villus height in the intestine of weaned pigs (2, 8, 13, 66, 67). Tributyrin is a strong mitosis promoter and a differentiation agent in the growth of villi in gastrointestinal tract (7, 68), which is considered to be the mechanism by which the height of intestinal villus increases (65). In addition, feeding E. coli K88-challenged weaned pigs with 300 mg/kg anethole containing diet has been reported to increase the villus height in the duodenum, thus increasing feed efficiency (21). Taken together, these data indicated that both tributyrin and anise bioactive components have the ability to enhance the intestinal absorption of nutrients by increasing villus height.
Feeding manipulation to alleviate post-weaning diarrhea is also a strategy to improve growth performance (69). It has been reported that dietary supplementation of tributyrin decreased fecal score during the post-weaning (67). However, Zhang et al. (70) noted that feeding weaned pigs with 1 g/kg tributyrin containing diet did not affect the diarrhea rate. In this study, pigs were healthy and showed no signs of diarrhea. In addition, results of 16S rRNA analysis indicated that pig feces were rich in probiotics, such as Prevotella (71), Lactobacillus (72), Agathobacter (73), Blautia (71), Prevotellaceae_NK3B31_group (74), Faecalibacterium (75), Megasphaera
(76), and Muribaculaceae (77). Indeed, the efficacy of tributyrin varies according to the health status of animals (15). In addition, Hamer et al. (78) reported the dual effect of butyrate on intestinal epithelial permeability, that is, low dose of butyrate enhanced tight junction and decreased intestinal permeability in vitro and in vivo in pigs, while high concentration of butyrate increased permeability both in some intestinal cell lines and in murine models. Therefore, we considered that the effect of tributyrin on fecal score was variable. Its supplementation may not be useful for pigs with no diarrhea symptoms. Studies that evaluated the effects of anise supplementation on fecal score in weaned pigs are still limited. In this study, we considered that the supplementation of TA did not affect the fecal score in weaned pigs, which was probably due to the lack of diarrhea symptoms in pigs.
The noxious gas from feces is produced by the unabsorbed nutrients fermented by intestinal microbiota (79). Decrease the fermentation substrate by improving nutrient digestibility and regulate the fermentation process by regulating intestinal microbiota are strategies to reduce fecal noxious gas emission (32, 80). In this study, we observed that feeding weaned pigs with TA containing diet improved apparent nutrient digestibility and upregulated the abundance of beneficial microbiota in feces. We speculated that the reduction of fecal NH3 emission can be explained by the improvement in nutrient digestibility and the increase in beneficial microbiota abundance.
The total protein, albumin, and globulin concentrations in vivo are always linked to nutritional status (81, 82). In this study, dietary supplementation of TA had no significant effects on serum total protein, albumin, and globulin concentrations. Similarly, several studies reported that feeding weaned pigs with tributyrin containing diet did not affect the concentrations of total protein, globulin, and albumin in serum (2, 39, 70, 83). The effect of anise on serum total protein, albumin, and globulin concentrations are not yet known, but at least, it does not generate any damaging effect.
Tributyrin supplementation has been reported to regulate blood lipids (39, 84). Butyrate could promote the secretion of the main proteins that make up HDL-C, thus promoting the synthesis of HDL-C (85, 86). Xiong et al. (87) noted that feeding lipopolysaccharide-challenged broiler chicks with tributyrin containing diet increased serum HDL-C concentrations. In addition, feeding weaned pigs with anise containing herbal mixture also has been reported to increase the serum HDL-C concentrations, whereas decrease the serum total cholesterol concentrations. However, in this study, weaned pigs fed the diet supplemented with TA had no effects on serum total cholesterol, triglyceride, and HDL-C concentrations, which was affirmed by the studies of Dell'Anno et al. (83) and Weber et al. (88). Therefore, as far as the results obtained in this study were concerned, the contribution of TA to the regulation of serum lipid metabolism parameters was limited.
Our findings confirmed that TA supplementation would improve growth performance and reduce fecal ammonia emission through improving nutrient digestibility, which was attributed to the increase of jejunal villus height and the regulation of fecal microbiota. Dose of TA at 0.15% seems suitable to be used in the diet of weaned pigs.
The data presented in this study are deposited in the figshare repository, accession number https://doi.org/10.6084/m9.figshare.21626027.v3.
The protocol (DK-1-2034) of this study was approved by the Animal Care and Use Committee of Dankook University (Cheonan, South Korea).
IK, KL, and JS: conceptualization and methodology. DD and SL: formal analysis. DD, HL, SM, and KH: writing—original draft preparation. DD and JS: investigation. IK: supervision. DD, HL, SM, KH, and IK: writing—reviewing and editing. All authors reviewed the manuscript.
This research was supported by Basic Science Research Capacity Enhancement Project through Korea Basic Science Institute (National research Facilities and Equipment Center) grant funded by the Ministry of Education (Grant No. 2019R1A6C1010033) and the Department of Animal Resource & Science was supported through the Research-Focused Department Promotion & Interdisciplinary Convergence Research Projects as a part of the University Innovation Support Program for Dankook University in 2022.
The authors gratefully acknowledge the Center for Bio-Medical Engineering Core Facility at Dankook University for providing critical reagents and equipment and OlusPlus Co., Ltd., for providing the additive used in this research.
KL was employed by Semi Feed Tech Co., Ltd.
The remaining authors declare that the research was conducted in the absence of any commercial or financial relationships that could be construed as a potential conflict of interest.
All claims expressed in this article are solely those of the authors and do not necessarily represent those of their affiliated organizations, or those of the publisher, the editors and the reviewers. Any product that may be evaluated in this article, or claim that may be made by its manufacturer, is not guaranteed or endorsed by the publisher.
The Supplementary Material for this article can be found online at: https://www.frontiersin.org/articles/10.3389/fvets.2023.1107149/full#supplementary-material
1. Xiong X, Tan B, Song M, Ji P, Kim K, Yin Y, et al. Nutritional intervention for the intestinal development and health of weaned pigs. Front Vet Sci. (2019) 6:46. doi: 10.3389/fvets.2019.00046
2. Zhang WX, Zhang Y, Zhang XW, Deng ZX, Liu JX, He ML, et al. Effects of dietary supplementation with combination of tributyrin and essential oil on gut health and microbiota of weaned piglets. Animals. (2020) 10:180. doi: 10.3390/ani10020180
3. Allen HK, Levine UY, Looft T, Bandrick M, Casey BT. Treatment, promotion, commotion: antibiotic alternatives in food-producing animals. Trends Microbiol. (2013) 21:114–9. doi: 10.1016/j.tim.2012.11.001
4. Byarugaba DK. Antimicrobial resistance in developing countries and responsible risk factors. Int J Antimicrob Ag. (2004) 24:105–10. doi: 10.1016/j.ijantimicag.2004.02.015
5. Donovan JD. Microencapsulation of Tributyrin to Improve Sensory Qualities and Intestinal Delivery. [PhD thesis]. Urbana and ?Champaign?, IL: University of Illinois at Urbana-Champaign (2015).
6. Tugnoli B, Piva A, Sarli G, Grilli E. Tributyrin differentially regulates inflammatory markers and modulates goblet cells number along the intestinal tract segments of weaning pigs. Livest Sci. (2020) 234:103996. doi: 10.1016/j.livsci.2020.103996
7. Guilloteau P, Martin L, Eeckhaut V, Ducatelle R, Zabielski R, Van Immerseel F. From the gut to the peripheral tissues: the multiple effects of butyrate. Nutr Res Rev. (2010) 23:366–84. doi: 10.1017/S0954422410000247
8. Gu Y, Song Y, Yin H, Lin S, Zhang X, Che L, et al. Dietary supplementation with tributyrin prevented weaned pigs from growth retardation and lethal infection via modulation of inflammatory cytokines production, ileal FGF19 expression, and intestinal acetate fermentation. J Anim Sci. (2017) 95:226–38. doi: 10.2527/jas.2016.0911
9. Yang Z, Yang C, Li X, Li G. Effects of ginger root, star anise and salvia root on growth performance, antioxidant status and serum metabolites in growth pigs. J Anim Sci. (2018) 96:324–5. doi: 10.1093/jas/sky404.712
10. Murray RL, Zhang W, Iwaniuk M, Grilli E, Stahl CH. Dietary tributyrin, an HDAC inhibitor, promotes muscle growth through enhanced terminal differentiation of satellite cells. Physiol Rep. (2018) 6:e13706. doi: 10.14814/phy2.13706
11. Gonzalez ML, Jacobs RD, Ely KM, Johnson SE. Dietary tributyrin supplementation and submaximal exercise promote activation of equine satellite cells. J Anim Sci. (2019) 97:4951–6. doi: 10.1093/jas/skz330
12. Glueck B, Han Y, Cresci GAM. Tributyrin supplementation protects immune responses and vasculature and reduces oxidative stress in the proximal colon of mice exposed to chronic-binge ethanol feeding. J Immunol Res. (2018) 2018:9671919. doi: 10.1155/2018/9671919
13. Wang C, Cao S, Zhang Q, Shen Z, Feng J, Hong Q, et al. Dietary tributyrin attenuates intestinal inflammation, enhances mitochondrial function, and induces mitophagy in piglets challenged with diquat. J Agric Food Chem. (2019) 67:1409–17. doi: 10.1021/acs.jafc.8b06208
14. Hou YQ, Liu YL, Hu J, Shen WH. Effects of lactitol and tributyrin on growth performance, small intestinal morphology and enzyme activity in weaned pigs. Asian Australas J Anim Sci. (2006) 19:1470–7. doi: 10.5713/ajas.2006.1470
15. Miragoli F, Patrone V, Prandini A, Sigolo S, Dell'Anno M, Rossi L, et al. Implications of tributyrin on gut microbiota shifts related to performances of weaning piglets. Microorganisms. (2021) 9:584. doi: 10.3390/microorganisms9030584
16. Bonos E, Skoufos I, Giannenas I, Sidiropoulou E, Fotou K, Stylianaki I, et al. Effect of an herbal mixture of oregano, garlic, sage and rock samphire extracts in combination with tributyrin on growth performance, intestinal microbiota and morphology, and meat quality in broilers. Sustainability. (2022) 14:13565. doi: 10.3390/su142013565
17. Chen G, Zhuo R, Ding H, Yang K, Xue J, Zhang S, et al. Effects of dietary tributyrin and physterol ester supplementation on growth performance, intestinal morphology, microbiota and metabolites in weaned piglets. J Appl Microbiol. (2022) 132:2293–305. doi: 10.1111/jam.15321
18. Figueroa J, Solà-Oriol D, Vinokurovas L, Manteca X, Pérez JF. Prenatal flavour exposure through maternal diets influences flavour preference in piglets before and after weaning. Anim Feed Sci Tech. (2013) 183:160–7. doi: 10.1016/j.anifeedsci.2013.04.023
19. Dang DX, Han KD, Kim IH. Supplementing volatile-flavour herbal-extract mixture to the diet of sows and their weaned offspring improves the growth performance of weaned piglets. J Appl Anim Nutr. (2021) 2021:1–6. doi: 10.3920/JAAN2021.0009
20. Windisch W, Schedle K, Plitzner C, Kroismayr A. Use of phytogenic products as feed additives for swine and poultry. J Anim Sci. (2008) 86:E140–8. doi: 10.2527/jas.2007-0459
21. Yi Q, Liu J, Zhang Y, Qiao H, Chen F, Zhang S, et al. Anethole attenuates enterotoxigenic Escherichia coli-induced intestinal barrier disruption and intestinal inflammation via modification of TLR signaling and intestinal microbiota. Front Microbiol. (2021) 12:647242. doi: 10.3389/fmicb.2021.647242
22. Wang GW, Hu WT, Huang BK, Qin LP. Illicium verum: a review on its botany, traditional use, chemistry and pharmacology. J Ethnopharmacol. (2011) 136:10–20. doi: 10.1016/j.jep.2011.04.051
23. Charal JW. Influence of Feeding Anise Oil to Piglets and Broilers. [PhD thesis]. Baton Rouge, AK: Louisiana State University (2014).
24. Zeng Z, Zhang S, Wang H, Piao X. Essential oil and aromatic plants as feed additives in non-ruminant nutrition: a review. J Anim Sci Biotechnol. (2015) 6:1–10. doi: 10.1186/s40104-015-0004-5
25. Charal JW, Bidner TD, Southern LL, Lavergne PASTA. Effect of anise oil fed to lactating sows and nursery pigs on sow feed intake, piglet performance, and weanling pig feed intake and growth performance. Prof Anim Sci. (2016) 32:99–105. doi: 10.15232/pas.2015-01433
26. Hou D, Zhou X, Zhong X, Settles ML, Herring J, Wang L, et al. Microbiota of the seminal fluid from healthy and infertile men. Fertil Steril. (2013) 100:1261–9. doi: 10.1016/j.fertnstert.2013.07.1991
27. Hou DS, Long WM, Shen J, Zhao LP, Pang XY, Xu C. Characterisation of the bacterial community in expressed prostatic secretions from patients with chronic prostatitis/chronic pelvic pain syndrome and infertile men: a preliminary investigation. Asian J Androl. (2012) 14:566–73. doi: 10.1038/aja.2012.30
29. AOAC. Official Methods of Analysis of AOAC International. Washington, DC: AOAC International (2000).
30. Liu X, Kim IH. Effects of long-term feeding of Achyranthes japonica Nakai extract as a supplement to diets with different protein levels diets on the growth performance and meat quality characteristics of growing-fattening pigs. Anim Feed Sci Tech. (2021) 279:115030. doi: 10.1016/j.anifeedsci.2021.115030
31. Hashem NM, Shehata MG. Antioxidant and antimicrobial activity of Cleome droserifolia (Forssk) Del and its biological effects on redox status, immunity, and gut microflora. Animals. (2021) 11:1929). doi: 10.3390/ani11071929
32. Dang DX, Kim YM, Kim IH. Effects of a root extract from Achyranthes japonica Nakai on the growth performance, blood profile, fecal microbial community, fecal gas emission, and meat quality of finishing pigs. Livest Sci. (2020) 239:104160. doi: 10.1016/j.livsci.2020.104160
33. Bolyen E, Rideout JR, Dillon MR, Bokulich NA, Abnet CC, Al-Ghalith GA, et al. Reproducible, interactive, scalable and extensible microbiome data science using QIIME 2. Nat Biotechnol. (2019) 37:852–7.doi: 10.1038/s41587-019-0209-9
34. Le Gall M, Gallois Seve B, Louveau I, Holst JJ, Oswald IP, Lallès JP, et al. Comparative effect of orally administered sodium butyrate before or after weaning on growth and several indices of gastrointestinal biology of piglets. Br J Nutr. (2009) 102:1285–96. doi: 10.1017/S0007114509990213
35. Manzanilla EG, Nofrarias M, Anguita M, Castillo M, Perez JF, Mart-Orúe SM, et al. Effects of butyrate, avilamycin, and a plant extract combination on the intestinal equilibrium of early-weaned pigs. J Anim Sci. (2006) 84:2743–51. doi: 10.2527/jas.2005-509
36. Chen TH, Chen WM, Hsu KH, Kuo CD, Hung SC. Sodium butyrate activates ERK to regulate differentiation of mesenchymal stem cells. Biochem Biophys Res Commun. (2007) 355:913–8. doi: 10.1016/j.bbrc.2007.02.057
37. Reyes-Camacho D, Pérez JF, Vinyeta E, Aumiller T, Van der Klis JD, Solà-Oriol D. Prenatal exposure to innately preferred d-limonene and trans-anethole does not overcome innate aversion to eucalyptol, affecting growth performance of weanling piglets. Animals. (2021) 11:2062. doi: 10.3390/ani11072062
38. Marinov V, Valcheva-Kuzmanova S. Review on the pharmacological activities of anethole. Scr Sci Pharm. (2015) 2:14–9. doi: 10.14748/ssp.v2i2.1141
39. Sotira S, Dell'Anno M, Caprarulo V, Hejna M, Pirrone F, Callegari ML, et al. Effects of tributyrin supplementation on growth performance, insulin, blood metabolites and gut microbiota in weaned piglets. Animals. (2020) 10:726. doi: 10.3390/ani10040726
40. Harris AJ, Patience JF, Lonergan SM, Dekkers CJM, Gabler NK. Improved nutrient digestibility and retention partially explains feed efficiency gains in pigs selected for low residual feed intake. J Anim Sci. (2012) 90:164–6. doi: 10.2527/jas.53855
41. Verschuren LMG, Schokker D, Bergsma R, van Milgen J, Molist F, Calus MPL, et al. Variation in faecal digestibility values related to feed efficiency traits of grower-finisher pigs. Animal. (2021) 15:100211. doi: 10.1016/j.animal.2021.100211
42. Dong L, Zhong X, He J, Zhang L, Bai K, Xu W, et al. Supplementation of tributyrin improves the growth and intestinal digestive and barrier functions in intrauterine growth-restricted piglets. Clin Nutr. (2016) 35:399–407. doi: 10.1016/j.clnu.2015.03.002
43. Sakdee J, Poeikhampha T, Rakangthong C, Poungpong K, Bunchasak C. Effect of tributyrin supplementation in diet on production performance and gastrointestinal tract of healthy nursery pigs. Pak J Nutr. (2016) 15:954–62. doi: 10.3923/pjn.2016.954.962
44. Wang C, Cao S, Shen Z, Hong Q, Feng J, Peng Y, et al. Effects of dietary tributyrin on intestinal mucosa development, mitochondrial function and AMPK-mTOR pathway in weaned pigs. J Animal Sci Biotechnol. (2019) 10:93. doi: 10.1186/s40104-019-0394-x
45. Gardiner GE, Metzler-Zebeli BU, Lawlor PG. Impact of intestinal microbiota on growth and feed efficiency in pigs: a review. Microorganisms. (2020) 8:1886. doi: 10.3390/microorganisms8121886
46. Reyer H, Oster M, McCormack UM, Muráni E, Gardiner GE, Ponsuksili S, et al. Host-microbiota interactions in ileum and caecum of pigs divergent in feed efficiency contribute to nutrient utilization. Microorganisms. (2020) 8:563. doi: 10.3390/microorganisms8040563
47. Frese SA, Benson AK, Tannock GW, Loach DM, Kim J, Zhang M, et al. The evolution of host specialization in the vertebrate gut symbiont Lactobacillus reuteri. PLoS Genet. (2011) 7:e1001314. doi: 10.1371/journal.pgen.1001314
48. Hou C, Zeng X, Yang F, Liu H, Qiao S. Study and use of the probiotic Lactobacillus reuteri in pigs: a review. J Anim Sci Biotechnol. (2015) 6:1–8. doi: 10.1186/s40104-015-0014-3
49. Shen J, Zhang J, Zhao Y, Lin Z, Ji L, Ma X. Tibetan pig-derived probiotic Lactobacillus amylovorus SLZX20-1 improved intestinal function via producing enzymes and regulating intestinal microflora. Front Nutr. (2022) 9:846991. doi: 10.3389/fnut.2022.846991
50. Yang Y, Zhao X, Le MH, Zijlstra RT, Gänzle MG. Reutericyclin producing Lactobacillus reuteri modulates development of fecal microbiota in weanling pigs. Frontiers Microbiol. (2015) 6:762. doi: 10.3389/fmicb.2015.00762
51. Tian Z, Cui Y, Lu H, Ma X. Effects of long-term feeding diets supplemented with Lactobacillus reuteri 1 on growth performance, digestive and absorptive function of the small intestine in pigs. J Funct Foods. (2020) 71:104010. doi: 10.1016/j.jff.2020.104010
52. Hurdle JG, Heathcott AE, Yang L, Yan B, Lee RE. Reutericyclin and related analogues kill stationary phase Clostridium difficile at achievable colonic concentrations. J Antimicrob Chemother. (2011) 66:1773–6. doi: 10.1093/jac/dkr201
53. Chen L, Li S, Zheng J, Li W, Jiang X, Zhao X, et al. Effects of dietary Clostridium butyricum supplementation on growth performance, intestinal development, and immune response of weaned piglets challenged with lipopolysaccharide. J Anim Sci Biotechnol. (2018) 9:1–14. doi: 10.1186/s40104-018-0275-8
54. Casas GA, Blavi L, Cross TWL, Lee AH, Swanson KS, Stein HH. Inclusion of the direct-fed microbial Clostridium butyricum in diets for weanling pigs increases growth performance and tends to increase villus height and crypt depth, but does not change intestinal microbial abundance. J Anim Sci. (2020) 98:skz372. doi: 10.1093/jas/skz372
55. Meimandipour A, Shuhaimi M, Soleimani AF, Azhar K, Hair-Bejo M, Kabeir BM, et al. Selected microbial groups and short-chain fatty acids profile in a simulated chicken cecum supplemented with two strains of Lactobacillus. Poult Sci. (2010) 89:470–6. doi: 10.3382/ps.2009-00495
56. Hashem NM, Soltan YA, El-Desoky NI, Morsy AS, Sallam SMA. Effects of Moringa oleifera extracts and monensin on performance of growing rabbits. Livest Sci. (2019) 228:136–43. doi: 10.1016/j.livsci.2019.08.012
57. Scher JU, Sczesnak A, Longman RS, Segata N, Ubeda C, Bielski C, et al. Expansion of intestinal Prevotella copri correlates with enhanced susceptibility to arthritis. Elife. (2013) 2:e01202. doi: 10.7554/eLife.01202
58. Holman DB, Brunelle BW, Trachsel J, Allen HK. Meta-analysis to define a core microbiota in the swine gut. MSystems. (2017) 2:e00004–17. doi: 10.1128/mSystems.00004-17
59. Alpizar-Rodriguez D, Lesker TR, Gronow A, Gilbert B, Raemy E, Lamacchia C, et al. Prevotella copri in individuals at risk for rheumatoid arthritis. Ann Rheum Dis. (2019) 78:590–3. doi: 10.1136/annrheumdis-2018-214514
60. Amat S, Lantz H, Munyaka PM, Willing BP. Prevotella in pigs: the positive and negative associations with production and health. Microorganisms. (2020) 8:1584. doi: 10.3390/microorganisms8101584
61. Iljazovic A, Roy U, Gálvez EJ, Lesker TR, Zhao B, Gronow A, et al. Perturbation of the gut microbiome by Prevotella spp. enhances host susceptibility to mucosal inflammation. Mucosal Immunol. (2021) 14:113–24. doi: 10.1038/s41385-020-0296-4
62. Pedersen HK, Gudmundsdottir V, Nielsen HB, Hyotylainen T, Nielsen T, Jensen BA, et al. Human gut microbes impact host serum metabolome and insulin sensitivity. Nature. (2016) 535:376–81. doi: 10.1038/nature18646
63. Pluske JR. Physiology of feed efficiency in the pig: emphasis on the gastrointestinal tract and specific dietary examples. In:Patience JF, , editor. Feed Efficiency in Swine. Wageningen: Wageningen Academic Publishers (2012), p. 239–57. doi: 10.3920/978-90-8686-756-1_12
64. Khan J, Islam MN. Morphology of the intestinal barrier in different physiological and pathological conditions. In:Martinez EP, , editor. The Histopathology-Reviews and Recent Advances. Rijeka: Intech Publishers (2012), p. 133–52. doi: 10.5772/50659
65. Kotunia A, Wolinski J, Laubitz D, Jurkowska M, Rome V, Guilloteau P, et al. Effect of sodium butyrate on the small intestine. J Physiol Pharmacol. (2004) 55:59–68.
66. Piva A, Grilli E, Fabbri L, Pizzamiglio V, Gatta PP, Galvano F, et al. Intestinal metabolism of weaned piglets fed a typical United States or European diet with or without supplementation of tributyrin and lactitol. J Anim Sci. (2008) 86:2952–61. doi: 10.2527/jas.2007-0402
67. Wang C, Shen Z, Cao S, Zhang Q, Peng Y, Hong Q, et al. Effects of tributyrin on growth performance, intestinal microflora and barrier function of weaned pigs. Anim Feed Sci Tech. (2019) 258:114311. doi: 10.1016/j.anifeedsci.2019.114311
68. Piva A, Prandini A, Fiorentini L, Morlacchini M, Galvano F, Luchansky JB. Tributyrin and lactitol synergistically enhanced the trophic status of the intestinal mucosa and reduced histamine levels in the gut of nursery pigs. J Anim Sci. (2002) 80:670–80. doi: 10.2527/2002.803670x
69. Zhu C, Lv H, Chen Z, Wang L, Wu X, Chen Z, et al. Dietary zinc oxide modulates antioxidant capacity, small intestine development, and jejunal gene expression in weaned piglets. Biol Trace Elem Res. (2017) 175:331–8. doi: 10.1007/s12011-016-0767-3
70. Zhang Y, Wang M, Li FF, Zhu YJ, Huang TJ, He ML, et al. Effects of tributyrin and oregano essential oil on growth performance, serum biochemical indices and nutrient apparent digestibility of weaning piglets. Anim Nutr. (2016) 28:2786–94.
71. Yang H, Xiao Y, Wang J, Xiang Y, Gong Y, Wen X, et al. Core gut microbiota in Jinhua pigs and its correlation with strain, farm and weaning age. J Microbiol. (2018) 56:346–55. doi: 10.1007/s12275-018-7486-8
72. Zhang Z, Lv J, Pan L, Zhang Y. Roles and applications of probiotic Lactobacillus strains. Appl Microbiol Biotechnol. (2018) 102:8135–43. doi: 10.1007/s00253-018-9217-9
73. Horvath A, Bausys A, Sabaliauskaite R, Stratilatovas E, Jarmalaite S, Schuetz B, et al. Distal gastrectomy with Billroth II reconstruction is associated with oralization of gut microbiome and intestinal inflammation: a proof-of-concept study. Ann Surg Oncol. (2021) 28:1198–208. doi: 10.1245/s10434-020-08678-1
74. Shang Q, Liu S, Liu H, Mahfuz S, Piao X. Impact of sugar beet pulp and wheat bran on serum biochemical profile, inflammatory responses and gut microbiota in sows during late gestation and lactation. J Anim Sci Biotechnol. (2021) 12:1–14. doi: 10.1186/s40104-021-00573-3
75. Zhao H, Xu H, Chen S, He J, Zhou Y, Nie Y. Systematic review and meta-analysis of the role of Faecalibacterium prausnitzii alteration in inflammatory bowel disease. J Gastroenterol Hepatol. (2021) 36:320–8. doi: 10.1111/jgh.15222
76. Yohe TT, Enger BD, Wang L, Tucker HLM, Ceh CA, Parsons CLM, et al. Does early-life administration of a Megasphaera elsdenii probiotic affect long-term establishment of the organism in the rumen and alter rumen metabolism in the dairy calf? J Dairy Sci. (2018) 101:1747–51. doi: 10.3168/jds.2017-12551
77. Smith BJ, Miller RA, Schmidt TM. Muribaculaceae genomes assembled from metagenomes suggest genetic drivers of differential response to acarbose treatment in mice. Msphere. (2021) 6:e00851–21. doi: 10.1128/msphere.00851-21
78. Hamer HM, Jonkers DMAE, Venema K, Vanhoutvin SALW, Troost FJ, Brummer RJ. The role of butyrate on colonic function. Aliment Pharmacol Ther. (2008) 27:104–19. doi: 10.1111/j.1365-2036.2007.03562.x
79. Ferket PR, van Heugten E, van Kempen TATG, Angel R. Nutritional strategies to reduce environmental emissions from nonruminants. J Anim Sci. (2002) 80:E168–82. doi: 10.2527/animalsci2002.80E-Suppl_2E168x
80. Liu X, Lee SI, Kim IH. Achyranthes japonica extracts supplementation to growing pigs positively influences growth performance, nutrient digestibility, fecal microbial shedding, and fecal gas emission. Asian-Australas J Anim Sci. (2020) 34:427–33. doi: 10.5713/ajas.20.0012
81. Dvorák M. Changes in blood protein levels in piglets during development and during stress. Vet Med. (1986) 31:403–14.
82. Fuhrman MP, Charney P, Mueller CM. Hepatic proteins and nutrition assessment. J Am Diet Assoc. (2004) 104:1258–64. doi: 10.1016/j.jada.2004.05.213
83. Dell'Anno M, Reggi S, Caprarulo V, Hejna M, Sgoifo Rossi CA, Callegari ML, et al. Evaluation of tannin extracts, leonardite and tributyrin supplementation on diarrhoea incidence and gut microbiota of weaned piglets. Animals. (2021) 11:1693. doi: 10.3390/ani11061693
84. He J, Dong L, Xu W, Bai K, Lu C, Wu Y, et al. Dietary tributyrin supplementation attenuates insulin resistance and abnormal lipid metabolism in suckling piglets with intrauterine growth retardation. PLoS ONE. (2015) 10:e0136848. doi: 10.1371/journal.pone.0136848
85. Nazih H, Nazih-Sanderson F, Krempf M, Michel Huvelin J, Mercier S, Marie Bard J. Butyrate stimulates ApoA-IV-containing lipoprotein secretion in differentiated Caco-2 cells: role in cholesterol efflux. J Cell Biochem. (2001) 83:230–8. doi: 10.1002/jcb.1221
86. Duka A, Fotakis P, Georgiadou D, Kateifides A, Tzavlaki K, von Eckardstein L, et al. ApoA-IV promotes the biogenesis of apoA-IV-containing HDL particles with the participation of ABCA1 and LCAT [S]. J Lipid Res. (2013) 54:107–15. doi: 10.1194/jlr.M030114
87. Xiong J, Qiu H, Bi Y, Zhou HL, Guo S, Ding B. Effects of dietary supplementation with tributyrin and coated sodium butyrate on intestinal morphology, disaccharidase activity and intramuscular fat of lipopolysaccharide-challenged broilers. Braz J Poult Sci. (2018) 40:707–16. doi: 10.1590/1806-9061-2018-0787
Keywords: tributyrin, anise, weaned pig, villus height, growth performance, nutrient digestibility
Citation: Dang DX, Lee H, Lee SJ, Song JH, Mun S, Lee KY, Han K and Kim IH (2023) Tributyrin and anise mixture supplementation improves growth performance, nutrient digestibility, jejunal villus height, and fecal microbiota in weaned pigs. Front. Vet. Sci. 10:1107149. doi: 10.3389/fvets.2023.1107149
Received: 24 November 2022; Accepted: 05 January 2023;
Published: 27 January 2023.
Edited by:
Jie Yu, Sichuan Agricultural University, ChinaReviewed by:
Nesrein M. Hashem, Alexandria University, EgyptCopyright © 2023 Dang, Lee, Lee, Song, Mun, Lee, Han and Kim. This is an open-access article distributed under the terms of the Creative Commons Attribution License (CC BY). The use, distribution or reproduction in other forums is permitted, provided the original author(s) and the copyright owner(s) are credited and that the original publication in this journal is cited, in accordance with accepted academic practice. No use, distribution or reproduction is permitted which does not comply with these terms.
*Correspondence: Kyudong Han, a3l1ZG9uZy5oYW5AZ21haWwuY29t; In Ho Kim,
aW5ob2tpbUBkYW5rb29rLmFjLmty
†These authors have contributed equally to this work and share first authorship
Disclaimer: All claims expressed in this article are solely those of the authors and do not necessarily represent those of their affiliated organizations, or those of the publisher, the editors and the reviewers. Any product that may be evaluated in this article or claim that may be made by its manufacturer is not guaranteed or endorsed by the publisher.
Research integrity at Frontiers
Learn more about the work of our research integrity team to safeguard the quality of each article we publish.