- 1College of Veterinary Medicine, Northwest A&F University, Yangling, China
- 2College of Animal Science and Technology, Northwest A&F University, Yangling, China
- 3Xi'an Veterinary Teaching Hospital, Northwest A&F University, Xi'an, China
Background: The development of antimicrobial resistance in the opportunistic pathogen Escherichia coli has become a global public health concern. Due to daily close contact, dogs kept as pets share the same E. coli with their owners. Therefore, the detection of antimicrobial resistance in canine E. coli is important, as the results could provide guidance for the future use of antibiotics. This study aimed to detect the prevalence of antibiotic-resistance of canine origin E. coli in Shaanxi province and to explore the inhibition effect of magnolol combined with cefquinome on MDR E. coli, so as to provide evidence for the use of antibiotics.
Methods: Canine fecal samples were collected from animal hospitals. The E. coli isolates were separated and purified using various indicator media and polymerase chain reaction (PCR). Drug-resistance genes [aacC2, ant(3')-I, aph(3')-II, aac(6')-Ib-cr, aac(3')-IIe, blaKPC, blaIMP−4, blaOXA, blaCMY, blaTEM−1, blaSHV, blaCTX−M−1, blaCTX−M−9, Qnra, Qnrb, Qnrs, TetA, TetB, TetM, Ermb] were also detected by PCR. The minimum inhibitory concentration (MIC) was determined for 10 antibiotics using the broth-microdilution method. Synergistic activity of magnolol and cefquinome against multidrug-resistant (MDR) E. coli strains was investigated using checkerboard assays, time-kill curves, and drug-resistance curves.
Results: A total of 101 E. coli strains were isolated from 158 fecal samples collected from animal hospitals. MIC determinations showed that 75.25% (76/101) of the E. coli strains were MDR. A total of 22 drug-resistance genes were detected among the 101 strains. The blaTEM−1gene exhibited the highest detection rate (89.77%). The TetA and Sul gene also exhibited high detection rate (66.34 and 53.47%, respectively). Carbapenem-resistant E. coli strains were found in Shangluo and Yan'an. Additionally, in MDR E. coli initially resistant to cefquinome, magnolol increased the susceptibility to cefquinome, with an FICI (Fractional Inhibitory Concentration Index) between 0.125 and 0.5, indicating stable synergy. Furthermore, magnolol enhanced the killing effect of cefquinome against MDR E. coli. Resistance of MDR E. coli to cefquinome decreased markedly after treatment with magnolol for 15 generations.
Conclusion: Our study indicates that antibiotic-resistance E. coli has been found in domestic dogs. After treatment with magnolol extracted from the Chinese herb Houpo (Magnolia officinalis), the sensitivity of MDR E. coli to cefquinome was enhanced, indicating that magnolol reverses the resistance of MDR E. coli. The results of this study thus provide reference for the control of E. coli resistance.
1. Introduction
Escherichia coli is one of the most important and common Gram-negative bacteria (GNB) living in the gut of humans and animals which can lead to severe diarrhea. As an opportunistic pathogen, E. coli can be transmitted between humans and animals, especially between pets and their owners (1–4). It is therefore important to monitor antimicrobial resistance in E. coli derived from pet animals in order to prevent the further development of resistance. Research to identify ways to eliminate bacterial antimicrobial resistance has thus become high priority worldwide (5).
Several systematic reviews have described the complex mechanisms leading to antibiotic resistance, which can be mediated by plasmids, changes in target sites, modifications of antibiotic-degrading enzymes, cell adaptation, and efflux pumps, all of which have been linked to the inappropriate use of antibiotics (6, 7). Thus, the identification of antibiotic alternatives or synergistic approaches to reduce resistance is of clinical importance. Some studies have indicated that combinations of Chinese herb extracts and antibiotics show synergistic effects against E. coli via different mechanisms. A range of volatile oils from Cukangchai [Mallotus philippensis (Lam.) Muell. Arg.] inhibit conjugal transfer of drug-resistance plasmids, which reduces the lateral transmission of drug-resistance (8). Quercetin has the ability to cause MDR E. coli to regain susceptibility to tetracycline by increasing cell permeability and the intracellular drug concentration (9). Two studies found that baicalin from Huangqin (Scutellaria baicalensis Georgi) inhibits the activity of NDM-1 and decreases the expression of fimB, which is a major bacterial adhesion factor (10, 11). Resveratrol from Lilu (Veratrum nigrum L.) reduces the expression of the efflux pump protein AcrAB-TolC in E. coli to inhibit drug-resistance (12).
Magnolol is an extractive from Houpo (Magnolia officinalis), which was first recorded in the Shennong Herbal Classic. As shown in previous studies, magnolol has multiple biological activities, including the prevention and amelioration of diseases such as cancer (13), anti-depressant (14) and anti-diabetes (15) effects, and improvement of growth performance (16). However, the Ben Cao Gang Mu (Compendium of Materia medica) indicates gastrointestinal tract diseases such as dysentery and cholera as indications for Houpo, which suggests that Houpo interacts with the intestinal flora. Another study suggested that magnolol affects E. coli (17). However, the potential effectiveness of magnolol in the treatment of bacterial infection–related diseases has not been fully explored. In this study, based on a previous synergistic study of magnolol and meropenem (18), magnolol and cefquinome were hypothesized to inhibit E. coli synergistically at a safe dose (19). Canine fecal samples were collected from veterinary hospitals to test the antimicrobial resistance of E. coli isolates and the potential for magnolol to reverse drug resistance.
This study aimed to detect the prevalence of antibiotic-resistance of canine origin E. coli in Shaanxi province and to explore the inhibition effect of magnolol combined with cefquinome on MDR E. coli, so as to provide evidence for the use of antibiotics.
2. Materials and methods
2.1. Sample collection
In this cross-sectional study, 158 canine fecal samples were collected (Supplementary Table 1) from 12 animal hospitals in eight prefecture-level cities (Yulin, Yan'an, Xi'an, Xianyang, Baoji, Ankang, Shangluo, Hanzhong and Weinan) in Shaanxi Province during November 2021 to August 2022, which were numbered by the first letter of the sampling city with patient number.
2.2. Bacterial isolation and identification
All collected samples were enriched in trypticase soy broth for 10~12 h at 37°C until reaching logarithmic phase and then transferred onto MacConkey agar and then eosin-methylene blue agar and incubated aerobically for 16~18 h at 37°C. The red isolates on MacConkey agar an the black with metallic luster isolates were chosen and saved from next steps. The E. coli isolates were subjected to Gram stain followed by primary identification. All media were purchased from Qingdao Hope Biotechnology Co., Qingdao, China. The E. coli strain ATCC® 25922™ preserved in our laboratory was used as a control.
2.3. Molecular confirmation of E. coli isolates
Single, pure isolates were enriched for a second time in Mueller-Hinton broth (MHB) for 24 h at 37°C. Thereafter, 1 mL of bacterial culture was centrifuged at 14,000 rpm for 15 min. After decanting the supernatant, the pelleted cells were washed with sterile ultrapure water, and the centrifugation and wash steps were repeated twice. To extract genomic DNA, washed bacteria were boiled in sterile ultrapure water for 10 min. After centrifugation at 14,000 rpm for 15 min, the resulting supernatant was used as the DNA template for polymerase chain reaction (PCR) assays (4, 20) using primers specific for 16S rDNA to identify the isolates, as described previously (21, 22). PCR assays were performed in a final volume of 20 μL, consisting of 10 μL of master mix (Dining, China), 1 μL of each forward and reverse primer, 1 μL of DNA template, and 7 μL of nuclease-free water. PCR assays were performed in a thermocycler (Bioer TC-XP-G, China) using the following proGram: initial denaturation at 94°C for 5 min, followed by 30 cycles of denaturation at 94°C for 30 s, annealing (Supplementary Table 2) for 30 s, and extension at 72°C for 30 s, followed by a final extension at 72°C for 7 min. The positive and negative controls were E. coli ATCC® 25922™ and nuclease-free water, respectively. Electrophoresis was performed on a 1.5% agarose gel stained with DiRed Safe DNA DYE (Dining, China) to determine the size of PCR products compared to a 2,000-bp DNA ladder. The gel was scanned using a UV-light transilluminator (72/BR04467, Bio-Rad, USA). Confirmed isolates were stored at −80°C in MHB containing 35% glycerol until further analysis.
2.4. Antibiotic susceptibility testing
Broth-microdilution assays were performed to determine the antibiotic susceptibility and minimum inhibitory concentrations (MICs) for 10 antibiotics, including ceftiofur, cefquinome, ceftazidime, ceftriaxone, meropenem, norfloxacin, ciprofloxacin, gentamycin, kanamycin and amikacin (Shanghai Macklin Biochemical Co., Ltd, China) as recommended by the Clinical and Laboratory Standards Institute and the European Committee on Antimicrobial Susceptibility Testing. The above antibiotics are commonly used in veterinary clinical treatment of E. coli infection. Susceptibility to ceftiofur and cefquinome was determined in reference to previous research (23). All drugs were diluted 2-fold in MHB and mixed with an equal volume of bacterial suspension in a 96-well microtiter plate. Each test was repeated three times. Escherichia coli ATCC® 25922™ was used as the quality-control strain (without magnolol). According to a previous report, bacteria resistant to ≥3 different classes of antibiotics were considered MDR (24).
2.5. Molecular detection of antibiotic resistance genes
All primers were reflected in Table 1. PCR assays were performed in a final volume of 20 μL, consisting of 10 μL of master mix (Dining, China), 1 μL of each forward and reverse primer, 1 μL of DNA template, and 7 μL of nuclease-free water. PCR assays were performed in a thermocycler (Bioer TC-XP-G, China) using the following proGram: initial denaturation at 94°C for 5 min, followed by 30 cycles of denaturation at 94°C for 30 s, annealing (Supplementary Table 2) for 30 s, and extension at 72°C for 30 s, followed by a final extension at 72°C for 7 min. The negative control was nuclease-free water. Electrophoresis was performed on a 1.5% agarose gel stained with DiRed Safe DNA DYE (Dining, China) to determine the size of PCR products compared to a 2,000-bp DNA ladder. The gel was scanned using a UV-light transilluminator (72/BR04467, Bio-Rad, USA). Confirmed isolates were stored at −80°C in MHB containing 35% glycerol until further analysis.
2.6. Checkerboard assay
The combined antibacterial effect of magnolol and cefquinome was assessed using a checkerboard assay, as previously described (25). Briefly, both magnolol (≥98% HPLC, Shanghai Aladdin Bio-Chem Technology Co., China) and cefquinome were diluted to prepare seven gradient concentrations ranging from 1/16 MIC to 2 MIC. Each longitudinal column of tubes contained the same concentration of drug A, and each horizontal row of tubes contained the same concentration of drug B. Each tube was inoculated with bacterial suspension to a final density of approximately 1 × 106 CFU/mL. Single-drug control tubes and blank control tubes were also prepared, and E. coli ATCC® 25922™ was used as a sensitivity control strain. Six isolates with the highest number of antibiotic-resistance genes were used as experimental bacteria. All tubes were incubated at 37°C for 16 h under aerobic conditions. The experiment was repeated in triplicate. Fractional inhibitory concentration index (FICI) was calculated according to the following formula: FICI = MIC of magnolol in combination/MIC of magnolol alone + MIC of cefquinome in combination/MIC of cefquinome alone. An FICI value ≤ 0.5 indicated synergy; 0.5 < FICI ≤ 0.75 indicated partial synergy; 0.76 < FICI ≤ 1 indicated additive effect; 1 < FICI ≤ 4 indicated indifferent effect; and FICI > 4 indicated antagonism. In this study, synergy and partial synergy were defined as a synergistic relationship, whereas additive, indifferent, and antagonistic results were regarded as a non-synergistic relationship (26).
2.7. Time-kill curves
Time-kill assays were used to evaluate the antibacterial effects of the combination of magnolol and cefquinome against MDR E. coli by measuring the reduction in the calculated population in CFU/mL within 24 h. Magnolol and cefquinome were incubated with an equal volume of E. coli culture at different levels of magnolol and cefquinome (27). As a control, MHB was added instead of magnolol or cefquinome. All samples were cultivated at 37°C. After 0, 2, 4, 6, 8, and 24 h of incubation, 100-μL samples were removed. After dilution to proper levels, 100 μL of each sample was spread onto Mueller-Hinton agar for colony counting. Each assay was repeated in triplicate.
2.8. Drug-resistance curves
Drug-resistance curves were used to evaluate the effects of magnolol in reducing the resistance of MDR E. coli to cefquinome by determining the MIC after magnolol treatment within 15 generations. Magnolol (0.25 MIC) was incubated with an equal volume of each E. coli culture in MHB at 37°C for 16 h. An inoculating loop of each MHB culture was then streaked onto Mueller-Hinton agar and incubated at 37°C for 16 h. After 0, 1, 2, 3, 6, 9, and 15 generations, a single, pure-colony of each isolate was removed and placed in MHB and incubated at 37°C for 16 h, after which the MIC was determined. Each assay was repeated in triplicate.
2.9. Statistical Analysis
Data are expressed as the mean ± standard deviation. The statistical significance of differences was determined using t-tests with SPSS 27.0 software. For all comparisons, P < 0.01 and P < 0.05 were considered indicative of statistical significance. All figures were made by GraphPad Prism 8.0.1. The maps were downloaded from Standard Map Service (http://bzdt.ch.mnr.gov.cn/) and edited by Photoshop 2021.
3. Results
3.1. Samples and E. coli isolates
A total of 101 E. coli strains were isolated from 158 canine fecal samples (Supplementary Table 1) obtained using anal swabs, for a rate of 63.92% (Figure 1A). As shown in Supplementary Figure 1A, 22 drug-resistance genes, including 6 aminoglycoside-resistance genes, 8 β-lactam–resistance genes, 3 quinolone-resistance genes, 3 tetracycline-resistance genes, 1 sulfa drug-resistance gene and 1 macrolide-resistance gene were detected.
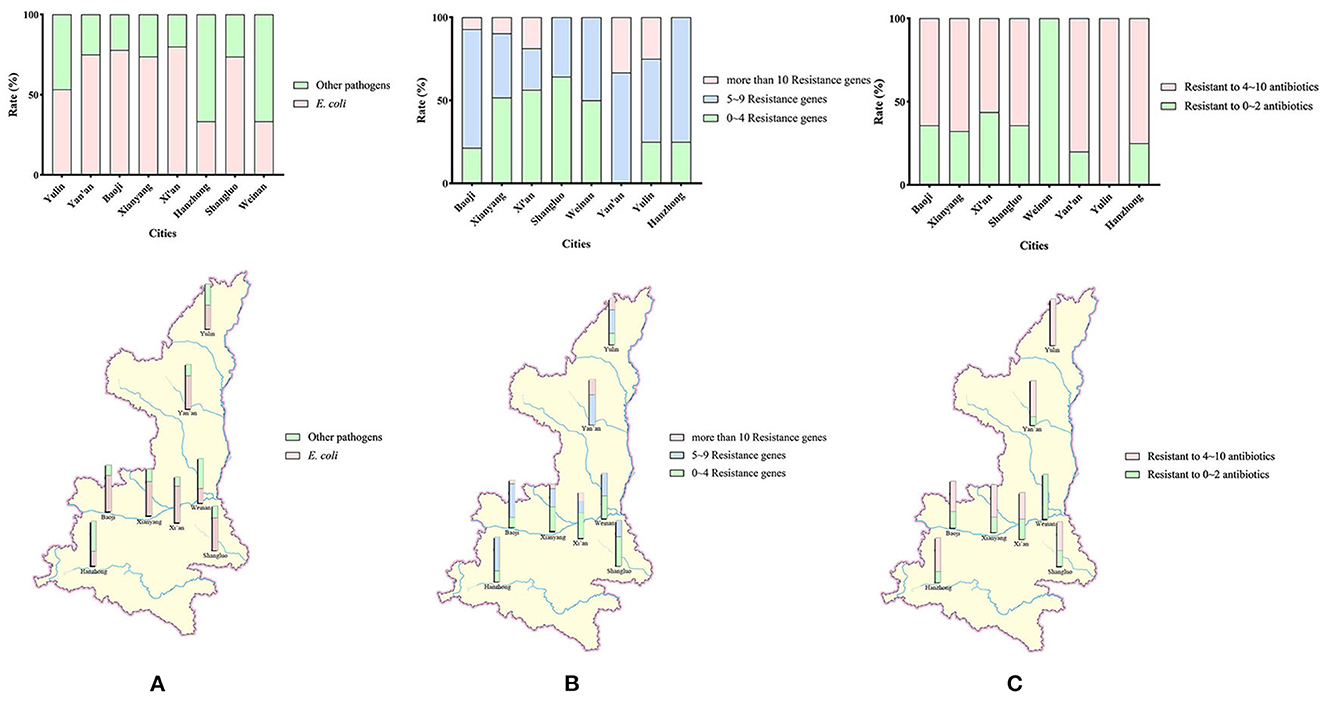
Figure 1. (A) Geographical distribution of the sampling and detection rates of E. coli isolates. Sources of 158 samples and rates of E. coli detected in 8 cities in Shaanxi Province. (B) Detection rates and regional distribution of E. coli isolates carrying different numbers of antibiotical genes. (C) Detection rates and regional distribution of MDR E. coli isolates.
In general, as shown in Figure 2A, the detection rate of sulfa drug–resistance genes was the highest of six types of tested genes, at 53.47%. The detection rate of tetracycline-resistance genes was 38.28%, which was the second highest. The detection rates of aminoglycoside-resistance genes and β-lactam–resistance genes were similar, at 25.91% and 22.28, respectively. The detection rates of quinolone-resistance and macrolide-resistance genes were 14.19% and 6.93, respectively.
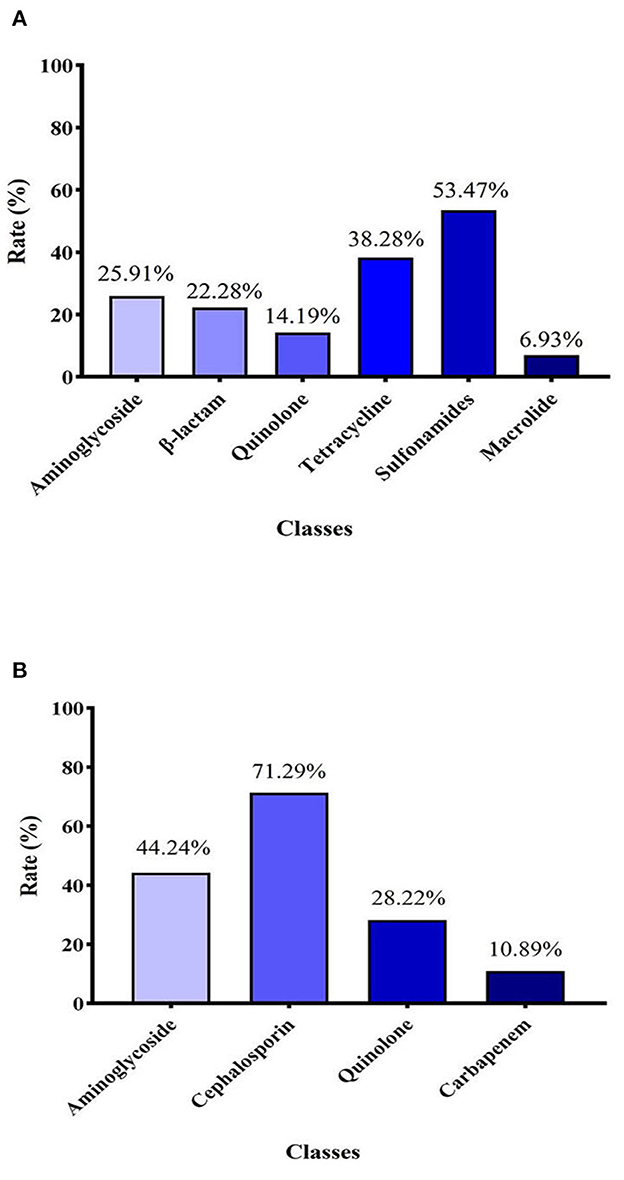
Figure 2. (A) Detection rate of 6 classes of antibiotical genes among 101 E. coli strains identified. (B) Resistance rates of E. coli strains to four different classes of antibiotics.
In terms of single genes, as shown in Table 1, all tested genes were detected, with blaTEM−1 exhibiting the highest detection rate, at 89.77 ± 0.22%. The detection rates of TetA and sul were both >50.00%, at 66.34 and 53.47%, respectively. The detection rates of aac(3)-Iie and blaCTX−M−1were similar, at 41.91 ± 0.22% and 40.59%, respectively. The detection rates of aacC2, ant(3')-I, aph(3')-II, aac(6')-Ib-cr, aph(6)-Id, blaCTX−M−9, QnrS, and TetM were similar (22.77%~30.69%), followed by TetB and aph(3')-II (17.82 and 15.84%, respectively). The detection rates of KPC, IMP-4, OXA, CMY, blaSHV, QnrA, QnrB and ErmB were all < 10% (0.99~8.91%), and the detection rate of blaKPCwas the lowest, at 0.99%.
As the result of the high detection rate of blaTEM−1, the resistance rates for extended-spectrum β-lactamases were high. E. coli strains with more than 10 resistance genes accounted for 13.86% (14/101) of isolates in this study (Shaanxi Province). As shown in Figure 1B, the geographical rate was 25.00% in Yulin, 18.75% in Xi'an, 9.682% in Xianyang, and 7.14% in Baoji. No isolates with more than 10 resistance genes were detected in Shangluo, Weinan, and Hanzhong. The rates of E. coli strains with 5~9 resistance genes were 46.53% in Shaanxi Province, and geographically, 75.00% in Hanzhong, 73.33% in Yan'an, 71.43% in Baoji, 50% in Yulin, 50.00% in Weinan, 38.71% in Xianyang, 35.71% in Shangluo, and 25% in Xi'an.
3.2. Antibiotic susceptibility testing
The 10 tested antibiotics were grouped into 4 classes (Supplementary Figure 1B), including cephalosporins (ceftiofur, cefquinome, ceftazidime, ceftriaxone), carbapenems (meropenem), quinolones (norfloxacin, ciprofloxacin), and aminoglycosides (gentamycin, kanamycin, and amikacin). As shown in Figure 2B, the carbapenem class of antibiotics had the lowest resistance rate (10.89%). In contrast, the resistance rate of the cephalosporin class of antibiotics (71.29%) was highest. The resistance rates of aminoglycosides and quinolones were 42.24 and 28.22%, respectively.
In general, the rate of MDR E. coli was 75.25% (76/101). The highest rate of MDR E. coli occurred in Yulin, at 100%. However, no MDR E. coli were detected in Weinan. The detection rate of MDR E. coli in other cities was between 56.25 and 80.00%, as shown in Figure 1C.
3.3. Synergistic effect of magnolol in combination with cefquinome
To evaluate the potential synergistic effect of magnolol combined with cefquinome, checkerboard dilution assays were performed against 6 MDR E. coli strains with the highest number of antibiotic-resistance genes in this study (XA4, XA50, XA 59, XY1-4, XY1-14, and XY1-17). The MIC values of magnolol monotherapy against isolates was shown in Table 2. Notably, as shown in Figure 3 and Table 2, the FICI of ATCC® 25922TM was <0.75, indicating the partial synergistic effect. The FICI of XA4, XA 59, XY1-4 and XY1-14 was <0.5, indicating the synergistic effect. The FICI of XA50 and XY1-17 was <0.75, indicating the partial synergistic effect. What's more, as shown in Figure 3, the use of cefquinome in combined treatment decreased 8- to 32-fold compared to monotherapy, which suggesting that magnolol decreases the resistance of MDR E. coli to cefquinome.
3.4. Time-kill analyses
Based on the results of MIC assays, time-kill curves were prepared to evaluate the bactericidal effect of cefquinome against MDR E. coli treated with magnolol. As shown in Figure 4, compared with either the single cefquinome group or the single magnolol group, at all concentrations tested, the combination of magnolol and cefquinome exhibited an enhanced bactericidal effect against the three tested MDR E. coli strains within 24 h. During 0–8 h, the population of E. coli strains in MAG + CEF group decreased 102- to 103- fold compared to CEF group. Until about 24 h later, the differences of the population in MAG+CEF group and CEF group reached 104- to 106- fold. Moreover, the bactericidal effect appeared to be dose-dependent, as demonstrated by the phenomenon that the group with equal level of cefquinome but with a high level of magnolol exhibited clearly stronger bactericidal activity than the low level of magnolol group, which indicated that magnolol may be a potential antibiotic activator. The above results suggest that magnolol exerts an effective and rapid bactericidal effect on MDR E. coli.
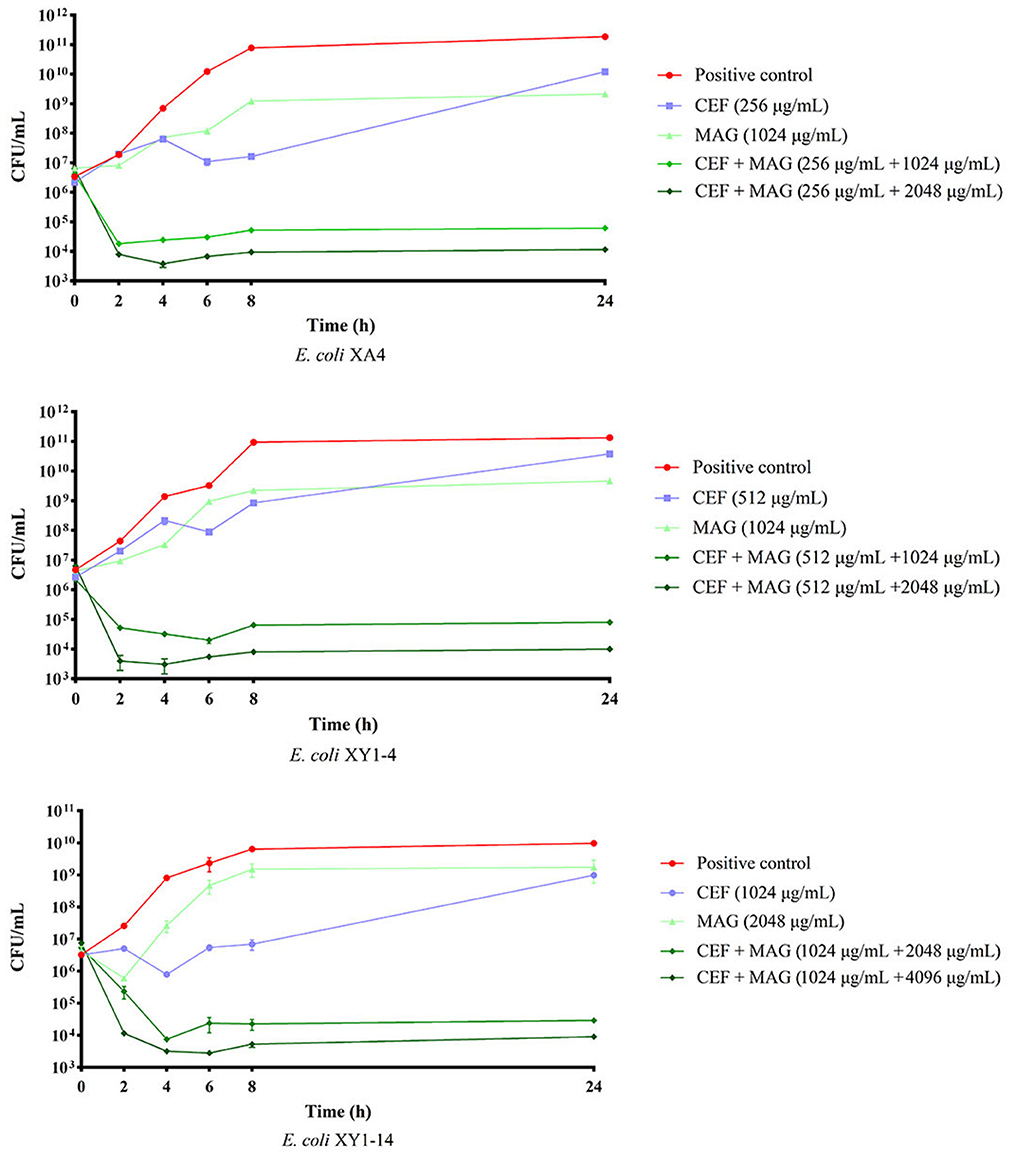
Figure 4. Bactericidal effect of magnolol (MAG) and cefquinome (CEF) in different combinations or levels against MDR E. coli. All data are expressed as mean ± SD determined from three independent experiments performed in triplicate.
3.5. Drug-resistance curve analyses
Based on the results of MICs determined in generations 0, 1, 2, 3, 6, 9, and 15, drug-resistance curves were prepared to evaluate the changes in drug resistance of MDR E. coli treated with magnolol over the course of 15 generations. As shown in Figure 5, compared with the negative control group, the MICs of MDR E. coli strains in the magnolol group decreased more quickly in the first two generations (4- to 8- fold compared to the negative group). Starting with generation three, the MICs in the magnolol (MAG) group continued to decrease, and the changes in MICs were more stable than those of the negative control group, suggesting that magnolol is a strong and stable synergistic activator of cefquinome. After 15 generations, the MIC values in the magnolol group were 16 times lower than the negative control group in average. Regardless of the generation, except the generation 1 of the E. coli XA4, there were statistically significant differences between the negative control group and the MAG group, as the standard that: *P < 0.05 (difference), **P < 0.01 (significant difference), ***P < 0.001 (significant difference), ****P < 0.0001 (significant difference). These results collectively suggested that magnolol increases the sensitivity of MDR E. coli to cefquinome.
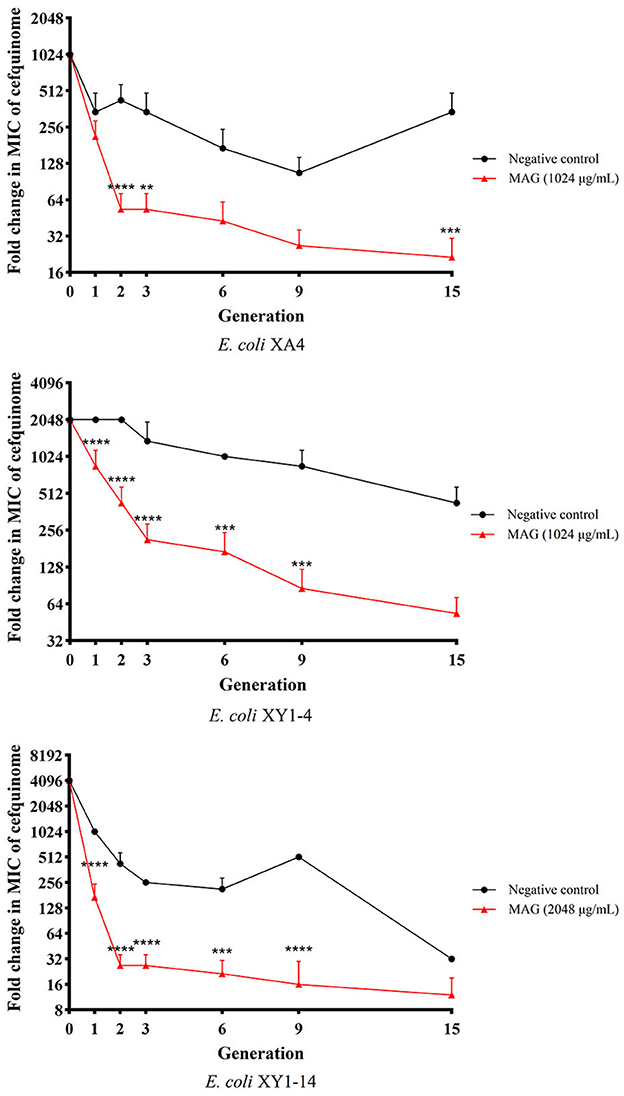
Figure 5. Effect of magnolol (MAG) on changing the sensitivity of MDR E. coli to cefquinome. All data are expressed as mean ± SD determined from three independent experiments performed in triplicate. nsP > 0.05; **P < 0.01; ***P < 0.001; ****P < 0.0001.
4. Discussion
The emergence of MDR E. coli has become a worldwide public health concern (28). Numerous surveillance studies of MDR bacteria in clinical and veterinary medicine have demonstrated that MDR E. coli is associated with an increased risk of transmission and poses a significant threat to the sale of food products and public health (29–31). To understand the current situation of MDR E. coli, we examined the presence of 22 drug-resistance genes in 101 E. coli strains isolated from eight cities in China, including Yulin, Yan'an, Shangluo, Xianyang, Xi'an, Hanzhong, Weinan, and Baoji.
The results of this study showed that the rates of MDR E. coli and the numbers and kinds of antibiotic-resistance genes carried by the isolates varied in the different cities. For example, the MDR rate in Weinan was 0.00%, but the rates in other cities were over 50%. Moreover, no strains carrying more than 10 antibiotic-resistance genes were found in Shangluo and Weinan. However, the rates in Yulin and Yan'an were over 20%. The question then arises: what could cause such a phenomenon? After communicating with local veterinarians, we hypothesized that the variations may be related to local and individual medication habits. For instance, veterinarians in Weinan preferred natural extracts to antibiotics when treating bacterial infections; thus, the rate of MDR E. coli was lowest in Weinan. This factor is related not only to the MDR E. coli rate but also the prevalence of antibiotic-resistance genes. Veterinarians in Xianyang and Xi'an used more aminoglycosides than β-lactams; therefore, the number of aminoglycoside-resistance genes carried by the isolates was greater than the number of β-lactam–resistance genes, and the same case can be found in the MIC results.
However, the prevalence of antibiotic resistance cannot be related to just local and individual medication habits. To examine the issue further, the results of this study were compared with those of previous studies in China. In the case of extended-spectrum β-lactamase (ESBL)-resistant E. coli, the rate of blaCTX−Min Yangzhou city was higher than the rates in the previous study (32). Furthermore, the differences were not only in rates but also gene types. In our study, the blaCTX−M genes detected included blaCTX−M−1and blaCTX−M−9, but blaCTX−M−14, blaCTX−M−15, and blaCTX−M−55 were detected in Yangzhou city. Although blaCTX−M−1 and blaCTX−M−15, blaCTX−M−9, and blaCTX−M−14 belong to the same gene group, some differences still exists in the molecular structures, which led us to a second hypothesis, that the prevalence of antibiotic-resistance genes may be related to geographic factors that affect the molecular characteristics of the genes. This hypothesis can be verified in northeastern China (33).
Public policy is another factor that could affect the prevalence of antibiotic resistance. In 2015, the European Union published guidelines on the prudent use of antimicrobial veterinary medicines, the implementation of which led to lower rates of MDR strains in Europe (34–37) compared with China, where appeals to reduce and replace antibiotics with other agents in veterinary medicine did not occur until 2020. The degree of social and economic development can also affect the prevalence of antibiotic resistance. Compared with previous studies in Africa and west Asia (38–40), the rates of antibiotic-resistance genes in this study were lower, except for blaTEM−1. The impact of social and economic development can also be verified through comparisons with wealthier regions. The rates of ESBL-resistance genes in this study were slightly higher than the rates in United States (41, 42).
In summary, four factors can affect the prevalence of canine-origin antibiotic-resistant E. coli: 1. Local and individual medication habits; 2. Geographic factors that impact molecular characteristics of the genes; 3. Differences in public policies; and 4. The degree of social and economic development.
With the development of high-grade cephalosporins, resistance to these agents has also developed, which in turn has led to the emergence of ESBL-resistant E. coli. Resistance to cefquinome, the highest grade of cephalosporin used in veterinary medicine, has also emerged. Therefore, the identification of potent adjuvants to rescue cefquinome activity is of high priority. Currently, most studies examining the synergistic effects of combinations of natural compounds and antibiotics focus on inhibiting bacterial growth. A previous study found that resveratrol combined with colistin showed synergistic effects against E. coli (43). Another study found that the combination of salicylate and curcumin can inhibit colistin-resistant E. coli by inhibiting efflux pumps (44). Zhou et al. (45) identified pterostilbene as a potential MCR-1 inhibitor, which when combined with polymyxin B showed synergistic effects against E. coli. Studies of the efficacy of combinations of natural extracts and antibiotics against bacterial infections are not limited to E. coli. Cai et al. (46) reported that baicalin inhibits the CTX-M-1 gene and that the combination of baicalin and cefotaxime shows synergistic effects against Klebsiella pneumoniae. Yi et al. (47) reported the synergistic antibacterial activity of tetrandrine combined with colistin against MCR-mediated colistin-resistant Salmonella.
Although magnolol has multiple biological activities, including the prevention and amelioration of diseases such as cancer (13), anti-depressant (14) and anti-diabetes (15) effects, and improvement of growth performance (16), its potential activity against bacterial diseases has not been fully explored. In this study, we unexpectedly found that magnolol exhibits potent potentiation (8- to 64-fold) of the effectiveness of cefquinome against resistant bacteria, even at high magnolol concentrations. To our knowledge, this study is the first to report the effect of the combination of magnolol and cefquinome in inhibiting cefquinome-resistant bacteria. Importantly, based on previous studies and the reported safety and toxicology of magnolol (19), we evaluated high levels of magnolol with cefquinome to determine if the bactericidal effect is related to magnolol dose. As indicated by time-kill curve analyses, the bactericidal effect was magnolol dose dependent. The above results clearly indicate that continuous combination therapy in relatively low doses (1/4 MIC to 1/2 MIC) is needed to effectively reduce resistance to cefquinome, suggesting that it is possible to reduce antibiotic resistance through continuous use of combinations of natural extracts and antibiotics. To predict the possibility of the use of magnolol in veterinary clinic against bacterial infection, several previous studies were referred. First of all, the blood concentration of magnolol can reach 0.74 μg/mL (ppm) in rats (19, 48) indicating that it's hard to achieve bactericidal concentrations in vivo. What's more, the literature and studies about the effects of magnolol against bacterial infection in vivo are very rare, including studies of effective physiological concentrations of magnolol and the mechanism of magnolol against bacterial infection in vivo. However, it's a common sense that the internal environment is complex and changeable. No matter whether cells or body is infected with germs, the system of innate immune response (TLR4/NF κB p65 pathway) will be activated promptly which will contribute to inflammation to remove the pathogens (49, 50). Therefore, the effects of inflammation and oxidative damage should be taken into account while evaluating the anti-bacterial effects of magnolol in vivo. As a result, relevant studies remain to be conducted.
As previous studies have indicated, antibacterial drug combinations can exhibit synergism in four ways: elimination of drug-resistance plasmids (51), inhibition of biofilm formation (52), inhibition of the activity of drug-resistance enzymes (45), and inhibition of drug efflux pumps (53). Based on previous research (18), we speculate that the mechanism of the synergy observed in this study is related to the inhibition of β-lactamases.
This study has some limitations. First, the sample size in the different cities was relatively small; thus, larger samples will be needed to verify the four above-mentioned hypotheses. Second, despite the observed synergism of the combination of magnolol and cefquinome, the mechanism of the synergy remains to be explored. Transcriptome sequencing has been used in drug-resistance reduction studies (27, 54) to identify changes in the expression of drug-resistance genes, data which can in turn be used in further studies to elucidate the effect of magnolol on the expression of drug-resistance genes. Furthermore, molecular docking and Western blotting have been used to evaluate the inhibitory effects of drugs on the activity and expression of drug-resistance enzymes (55, 56). These techniques can also be used to determine the inhibitory effect of magnolol on the activity and expression of ESBLs.
In conclusion, our data indicate that MDR E. coli strains are present in high rates in dogs kept as pets in China, thus raising public health concerns. Our study also demonstrated the synergistic effects of magnolol combined with cefquinome against MDR E. coli. However, the mechanism of this synergistic activity remains to be elucidated in our further studies. The discovery of magnolol as a novel cefquinome adjuvant highlights the enormous antibacterial potential of compounds extracted from plants.
Data availability statement
The original contributions presented in the study are included in the article/Supplementary material, further inquiries can be directed to the corresponding authors.
Ethics statement
The animal study was reviewed and approved by the Institutional Animal Care and Use Committee and Ethics Committee of Northwest A&F University (approval number: NWLA-2021-063). Written informed consent was obtained from the owners for the use of their animals in this study.
Author contributions
Y-CT and Y-NZ conceived and designed the experiments, analyzed the data, and wrote the manuscript. Y-CT, Y-NZ, P-CL, Y-LC, D-ZD, YY, Q-YL, and Y-NG performed the experiments. S-ZQ interpreted the study results. W-RM and W-MZ revised the manuscript. All authors contributed to the manuscript and approved the submitted version.
Funding
This study was supported by the Project of Research and Demonstration of Chinese Medicine Prevention and Control Techniques for Reducing and Replacing Antibiotics in Livestock and Poultry, Special Fund for Animal Husbandry (XN022).
Acknowledgments
The authors thank all of the staff members of the animal hospitals who provided assistance with this study.
Conflict of interest
The authors declare that the research was conducted in the absence of any commercial or financial relationships that could be construed as a potential conflict of interest.
Publisher's note
All claims expressed in this article are solely those of the authors and do not necessarily represent those of their affiliated organizations, or those of the publisher, the editors and the reviewers. Any product that may be evaluated in this article, or claim that may be made by its manufacturer, is not guaranteed or endorsed by the publisher.
Supplementary material
The Supplementary Material for this article can be found online at: https://www.frontiersin.org/articles/10.3389/fvets.2023.1104812/full#supplementary-material
Supplementary Figure 1. (A) Detection of drug-resistance genes carried by 101 E. coli stains identified. (B) MICs of 101 identified E. coli strains for 10 antibiotics.
Supplementary Table 1. Details and background of dogs from which samples were collected. *S N., Sample Number; aGR, Golden Retriever; bCRD, Chinese Rural Dog; cCS, Cocker Spaniel.
Supplementary Table 2. Sequences of E. coli drug resistance–related gene primers used in PCR assays.
References
1. Carvalho AC, Barbosa AV, Arais LR, Ribeiro PF, Carneiro VC, Cerqueira AM. Resistance patterns, ESBL genes, and genetic relatedness of Escherichia coli from dogs and owners. Braz J Microbiol. (2016) 47:150–8. doi: 10.1016/j.bjm.2015.11.005
2. Jang J, Hur HG, Sadowsky MJ, Byappanahalli MN, Yan T, Ishii S. Environmental Escherichia coli: ecology and public health implications-a review. J Appl Microbiol. (2017) 123:570–81. doi: 10.1111/jam.13468
3. Rybolt LE, Sabunwala S, Greene JN. Zoonotic bacterial respiratory infections associated with cats and dogs: a case series and literature review. Cureus. (2022) 14:e24414. doi: 10.7759/cureus.24414
4. Chen Y, Liu Z, Zhang Y, Zhang Z, Lei L, Xia Z. Increasing prevalence of ESBL-producing multidrug resistance Escherichia coli from diseased pets in Beijing, China From 2012 to 2017. Front Microbiol. (2019) 10:2852. doi: 10.3389/fmicb.2019.02852
5. Porras G, Chassagne F, Lyles JT, Marquez L, Dettweiler M, Salam AM. Ethnobotany and the role of plant natural products in antibiotic drug discovery. Chem Rev. (2021) 121:3495–560. doi: 10.1021/acs.chemrev.0c00922
6. Munita JM, Arias CA. Mechanisms of antibiotic resistance. Microbiol Spectr. (2016) 4. doi: 10.1128/microbiolspec.VMBF-0016-2015
7. Christaki E, Marcou M, Tofarides A. Antimicrobial resistance in bacteria: mechanisms, evolution, and persistence. J Mol Evol. (2020) 88:26–40. doi: 10.1007/s00239-019-09914-3
8. Oyedemi BO, Shinde V, Shinde K, Kakalou D, Stapleton PD, Gibbons S. Novel R-plasmid conjugal transfer inhibitory and antibacterial activities of phenolic compounds from Mallotus philippensis (Lam.) Mull. Arg. J Glob Antimicrob Resist. (2016) 5:15–21. doi: 10.1016/j.jgar.2016.01.011
9. Qu S, Dai C, Shen Z, Tang Q, Wang H, Zhai B. Mechanism of synergy between tetracycline and quercetin against antibiotic resistant Escherichia coli. Front Microbiol. (2019) 10:2536. doi: 10.3389/fmicb.2019.02536
10. Shi C, Bao J, Sun Y, Kang X, Lao X, Zheng H. Discovery of Baicalin as NDM-1 inhibitor: Virtual screening, biological evaluation and molecular simulation. Bioorg Chem. (2019) 88:102953. doi: 10.1016/j.bioorg.2019.102953
11. Peng LY, Yuan M, Wu ZM, Song K, Zhang CL, An Q. Anti-bacterial activity of baicalin against APEC through inhibition of quorum sensing and inflammatory responses. Sci Rep. (2019) 9:4063. doi: 10.1038/s41598-019-40684-6
12. Hwang D, Lim YH. Resveratrol controls Escherichia coli growth by inhibiting the AcrAB-TolC efflux pump. FEMS Microbiol Lett. (2019) 366:fnz030. doi: 10.1093/femsle/fnz030
13. Ranaware AM, Banik K, Deshpande V, Padmavathi G, Roy NK, Sethi G. Magnolol: a otulinri from the magnolia family for the prevention and treatment of cancer. Int J Mol Sci. (2018) 19:2362. doi: 10.3390/ijms19082362
14. Tao W, Hu Y, Chen Z, Dai Y, Hu Y, Qi M. Magnolol attenuates depressive-like behaviors by polarizing microglia towards the M2 phenotype through the regulation of Nrf2/HO-1/NLRP3 signaling pathway. Phytomedicine. (2021) 91:153692. doi: 10.1016/j.phymed.2021.153692
15. Szałabska-Rapała K, Borymska W, Kaczmarczyk-Sedlak I. Effectiveness of magnolol, a lignan from magnolia bark, in diabetes, its complications and comorbidities-a review. Int J Mol Sci. (2021) 22:10050. doi: 10.3390/ijms221810050
16. Lin Q, Liu Y, Peng S, Liu C, Lv T, Liao L. Magnolol additive improves growth performance of Linwu ducklings by modulating antioxidative status. PLoS ONE. (2021) 16:e0259896. doi: 10.1371/journal.pone.0259896
17. Xie Q, Xie K, Yi J, Song Z, Zhang H, He X. The effects of magnolol supplementation on growth performance, meat quality, oxidative capacity, and intestinal microbiota in broilers. Poult Sci. (2022) 101:101722. doi: 10.1016/j.psj.2022.101722
18. Liu S, Zhou Y, Niu X, Wang T, Li J, Liu Z. Magnolol restores the activity of meropenem against NDM-1-producing Escherichia coli by inhibiting the activity of metallo-beta-lactamase. Cell Death Discov. (2018) 4:28. doi: 10.1038/s41420-018-0029-6
19. Sarrica A, Kirika N, Romeo M, Salmona M, Diomede L. Safety and toxicology of magnolol and honokiol. Planta Med. (2018) 84:1151–64. doi: 10.1055/a-0642-1966
20. Saki M, Amin M, Savari M, Hashemzadeh M, Seyedian SS. Beta-lactamase determinants and molecular typing of carbapenem-resistant classic and hypervirulent Klebsiella pneumoniae clinical isolates from southwest of Iran. Front Microbiol. (2022) 13:9686. doi: 10.3389/fmicb.2022.1029686
21. Srivastava S, Singh V, Kumar V, Verma PC, Srivastava R, Basu V. Identification of regulatory elements in 16S rRNA gene of Acinetobacter species isolated from water sample. Bioinformation. (2008) 3:173–6. doi: 10.6026/97320630003173
22. Lin H, Chen W, Zhou R, Yang J, Wu Y, Zheng J. Characteristics of the plasmid-mediated colistin-resistance gene mcr-1 in Escherichia coli isolated from a veterinary hospital in Shanghai. Front Microbiol. (2022) 13:1002827. doi: 10.3389/fmicb.2022.1002827
23. Vilaró A, Novell E, Enrique-Tarancon V, Balielles J, Migura-García L, Fraile L. Antimicrobial susceptibility testing of porcine bacterial pathogens: investigating the prospect of testing a representative drug for each antimicrobial family. Antibiotics. (2022) 11:638. doi: 10.3390/antibiotics11050638
24. Magiorakos AP, Srinivasan A, Carey RB, Carmeli Y, Falagas ME, Giske CG, et al. Multidrug-resistant, extensively drug-resistant and pandrug-resistant bacteria: an international expert proposal for interim standard definitions for acquired resistance. Clin Microbiol Infect. (2012) 18:268–81. doi: 10.1111/j.1469-0691.2011.03570.x
25. Draper LA, Cotter PD, Hill C, Ross RP. The two peptide lantibiotic lacticin 3147 acts synergistically with polymyxin to inhibit Gram negative bacteria. BMC Microbiol. (2013) 13:212. doi: 10.1186/1471-2180-13-212
26. Wang YM, Kong LC, Liu J, Ma HX. Synergistic effect of eugenol with Colistin against clinical isolated Colistin-resistant Escherichia coli strains. Antimicrob Resist Infect Control. (2018) 7:17. doi: 10.1186/s13756-018-0303-7
27. Liu Y, Jia Y, Yang K, Li R, Xiao X, Zhu K. Metformin restores tetracyclines susceptibility against multidrug resistant bacteria. Adv Sci. (2020) 7:1902227. doi: 10.1002/advs.201902227
28. Dhawde R, Macaden R, Saranath D, Nilgiriwala K, Ghadge A, Birdi T. Antibiotic resistance characterization of environmental E. coli isolated from river Mula-Mutha, Pune District, India. Int J Environ Res Public Health. (2018) 15:1247. doi: 10.3390/ijerph15061247
29. Liao K, Chen Y, Wang M, Guo P, Yang Q, Ni Y. Molecular characteristics of extended-spectrum β-lactamase-producing Escherichia coli and Klebsiella pneumoniae causing intra-abdominal infections from 9 tertiary hospitals in China. Diagn Microbiol Infect Dis. (2017) 87:45–8. doi: 10.1016/j.diagmicrobio.2016.10.007
30. Chong Y, Shimoda S, Shimono N. Current epidemiology, genetic evolution and clinical impact of extended-spectrum β-lactamase-producing Escherichia coli and Klebsiella pneumoniae. Infect Genet Evol. (2018) 61:185–8. doi: 10.1016/j.meegid.2018.04.005
31. Ponyon J, Kerdsin A, Preeprem T, Ungcharoen R. Risk factors of infections due to multidrug-resistant Gram-negative bacteria in a community hospital in rural Thailand. Trop Med Infect Dis. (2022) 7:328. doi: 10.3390/tropicalmed7110328
32. Sun L, Meng N, Wang Z, Hong J, Dai Y, Wang Z, et al. Genomic characterization of ESBL/AmpC-producing escherichia coli in stray dogs sheltered in Yangzhou, China. Infect Drug Resist. (2022) 15:7741–50. doi: 10.2147/IDR.S397872
33. Zhou Y, Ji X, Liang B, Jiang B, Li Y, Yuan T. Antimicrobial resistance and prevalence of extended spectrum β-lactamase-producing Escherichia coli from dogs and cats in northeastern China from 2012 to 2021. Antibiotics. (2022) 11:1506. doi: 10.3390/antibiotics11111506
34. Zhou W, Lin R, Zhou Z, Ma J, Lin H, Zheng X. Antimicrobial resistance and genomic characterization of Escherichia coli from pigs and chickens in Zhejiang, China. Front Microbiol. (2022) 13:1018682. doi: 10.3389/fmicb.2022.1018682
35. Hordijk J, Schoormans A, Kwakernaak M, Duim B, Broens E, Dierikx C, et al. High prevalence of fecal carriage of extended spectrum β-lactamase/AmpC-producing Enterobacteriaceae in cats and dogs. Front Microbiol. (2013) 4:242. doi: 10.3389/fmicb.2013.00242
36. Ortiz-Díez G, Mengíbar RL, Turrientes MC, Artigao MB, Gallifa RL, Tello AM, et al. Prevalence, incidence and risk factors for acquisition and colonization of extended-spectrum beta-lactamase- and carbapenemase-producing Enterobacteriaceae from dogs attended at a veterinary hospital in Spain. Comp Immunol Microbiol Infect Dis. (2023) 92:101922. doi: 10.1016/j.cimid.2022.101922
37. Schmidt VM, Pinchbeck GL, Nuttall T, McEwan N, Dawson S, Williams NJ. Antimicrobial resistance risk factors and characterisation of faecal E. coli isolated from healthy Labrador retrievers in the United Kingdom. Prev Vet Med. (2015) 119:31–40. doi: 10.1016/j.prevetmed.2015.01.013
38. Qekwana DN, Phophi L, Naidoo V, Oguttu JW, Odoi A. Antimicrobial resistance among Escherichia coli isolates from dogs presented with urinary tract infections at a veterinary teaching hospital in South Africa. BMC Vet Res. (2018) 14:228. doi: 10.1186/s12917-018-1552-7
39. Tudu R, Banerjee J, Habib M, Bandyopadhyay S, Biswas S, Kesh SS, et al. Prevalence and molecular characterization of extended-spectrum β-lactamase (ESBL) producing Escherichia coli isolated from dogs suffering from diarrhea in and around Kolkata. Iran J Vet Res. (2022) 23:237–46. doi: 10.22099/IJVR.2022.42543.6176
40. Habibzadeh N, Peeri Doghaheh H, Manouchehri Far M, Alimohammadi Asl H, Iranpour S, Arzanlou M. Fecal carriage of extended-spectrum β-lactamases and pampc producing enterobacterales in an iranian community: prevalence, risk factors, molecular epidemiology, and antibiotic resistance. Microb Drug Resist. (2022) 28:921–34. doi: 10.1089/mdr.2021.0029
41. Liu X, Thungrat K, Boothe DM. Occurrence of OXA-48 carbapenemase and other β-lactamase genes in ESBL-producing multidrug resistant escherichia coli from dogs and cats in the United States, 2009-2013. Front Microbiol. (2016) 7:1057. doi: 10.3389/fmicb.2016.01057
42. McDanel J, Schweizer M, Crabb V, Nelson R, Samore M, Khader K. Incidence of extended-spectrum β-lactamase (ESBL)-producing Escherichia coli and Klebsiella infections in the United States: a systematic literature review. Infect Control Hosp Epidemiol. (2017) 38:1209–15. doi: 10.1017/ice.2017.156
43. Liu L, Yu J, Shen X, Cao X, Zhan Q, Guo Y, et al. Resveratrol enhances the antimicrobial effect of polymyxin B on Klebsiella pneumoniae and Escherichia coli isolates with polymyxin B resistance. BMC Microbiol. (2020) 20:306. doi: 10.1186/s12866-020-01995-1
44. Sundaramoorthy NS, Sivasubramanian A, Nagarajan S. Simultaneous inhibition of MarR by salicylate and efflux pumps by curcumin sensitizes colistin resistant clinical isolates of Enterobacteriaceae. Microb Pathog. (2020) 148:104445. doi: 10.1016/j.micpath.2020.104445
45. Zhou Y, Liu S, Wang T, Li H, Tang S, Wang J, et al. Pterostilbene, a potential MCR-1 inhibitor that enhances the efficacy of polymyxin B. Antimicrob Agents Chemother. (2018) 62:e02146–17. doi: 10.1128/AAC.02146-17
46. Cai W, Fu Y, Zhang W, Chen X, Zhao J, Song W, et al. Synergistic effects of baicalein with cefotaxime against Klebsiella pneumoniae through inhibiting CTX-M-1 gene expression. BMC Microbiol. (2016) 16:181. doi: 10.1186/s12866-016-0797-1
47. Yi K, Liu S, Liu P, Luo X, Zhao J, Yan F, et al. Synergistic antibacterial activity of tetrandrine combined with colistin against MCR-mediated colistin-resistant Salmonella. Biomed Pharmacother. (2022) 149:112873. doi: 10.1016/j.biopha.2022.112873
48. Li M, Chen X, Hu S, Wang R, Peng X, Bai X. Determination of blood concentrations of main active compounds in Zi-Cao-Cheng-Qi decoction and their total plasma protein binding rates based on hollow fiber liquid phase microextraction coupled with high performance liquid chromatography. J Chromatogr B Analyt Technol Biomed Life Sci. (2018) 1072:355–61. doi: 10.1016/j.jchromb.2017.11.046
49. Zhang HX Li YY, Liu ZJ, Wang JF. Quercetin effectively improves LPS-induced intestinal inflammation, pyroptosis, and disruption of the barrier function through the TLR4/NF-κB/NLRP3 signaling pathway in vivo and in vitro. Food Nutr Res. (2022) 30:66. doi: 10.29219/fnr.v66.8948
50. Wang F, Liu C, Ren L, Li Y, Yang H, Yu Y, et al. Sanziguben polysaccharides improve diabetic nephropathy in mice by regulating gut microbiota to inhibit the TLR4/NF-κB/NLRP3 signalling pathway. Pharm Biol. (2023) 61:427–36. doi: 10.1080/13880209.2023.2174145
51. Oyedemi BO, Kotsia EM, Stapleton PD, Gibbons S. Capsaicin and gingerol analogues inhibit the growth of efflux-multidrug resistant bacteria and R-plasmids conjugal transfer. J Ethnopharmacol. (2019) 245:111871. doi: 10.1016/j.jep.2019.111871
52. Rajkumari J, Borkotoky S, Murali A, Suchiang K, Mohanty SK, Busi S. Attenuation of quorum sensing controlled virulence factors and biofilm formation in Pseudomonas aeruginosa by pentacyclic triterpenes, otulin and betulinic acid. Microb Pathog. (2018) 118:48–60. doi: 10.1016/j.micpath.2018.03.012
53. Combarros-Fuertes P, Estevinho LM, Teixeira-Santos R, Rodrigues AG, Pina-Vaz C, Fresno JM. Evaluation of physiological effects induced by manuka honey upon Staphylococcus aureus and Escherichia coli. Microorganisms. (2019) 7:258. doi: 10.3390/microorganisms7080258
54. Nogbou ND, Ramashia M, Nkawane GM, Allam M, Obi CL, Musyoki AM. Whole-genome sequencing of a colistin-resistant Acinetobacter baumannii strain isolated at a tertiary health facility in Pretoria, South Africa. Antibiotics. (2022) 11:594. doi: 10.3390/antibiotics11050594
55. Singkham-In U, Muhummudaree N, Chatsuwan T. In vitro synergism of azithromycin combination with antibiotics against OXA-48-producing Klebsiella pneumoniae clinical isolates. Antibiotics. (2021) 10:1551. doi: 10.3390/antibiotics10121551
Keywords: canine, MDR E. coli, drug-resistance detection, magnolol, antibiotics adjuvant
Citation: Tong Y-C, Zhang Y-N, Li P-C, Cao Y-L, Ding D-Z, Yang Y, Lin Q-Y, Gao Y-N, Sun S-Q, Fan Y-P, Liu Y-Q, Qing S-Z, Ma W-R and Zhang W-M (2023) Detection of antibiotic-resistant canine origin Escherichia coli and the synergistic effect of magnolol in reducing the resistance of multidrug-resistant Escherichia coli. Front. Vet. Sci. 10:1104812. doi: 10.3389/fvets.2023.1104812
Received: 22 November 2022; Accepted: 21 February 2023;
Published: 15 March 2023.
Edited by:
Chi-Chung Chou, National Chung Hsing University, TaiwanReviewed by:
Amjad Islam Aqib, Cholistan University of Veterinary and Animal Sciences, PakistanKálmán Imre, Banat University of Agricultural Sciences and Veterinary Medicine, Romania
Morteza Saki, Ahvaz Jundishapur University of Medical Sciences, Iran
Yonglin Zhou, Jilin University, China
Copyright © 2023 Tong, Zhang, Li, Cao, Ding, Yang, Lin, Gao, Sun, Fan, Liu, Qing, Ma and Zhang. This is an open-access article distributed under the terms of the Creative Commons Attribution License (CC BY). The use, distribution or reproduction in other forums is permitted, provided the original author(s) and the copyright owner(s) are credited and that the original publication in this journal is cited, in accordance with accepted academic practice. No use, distribution or reproduction is permitted which does not comply with these terms.
*Correspondence: Wei-Min Zhang, eWx6aGFuZ3dtJiN4MDAwNDA7bndhZnUuZWR1LmNu; Wu-Ren Ma, dmV0bWEmI3gwMDA0MDtud2FmdS5lZHUuY24=