- 1Department of Production Animal Studies, Faculty of Veterinary Science, University of Pretoria, Pretoria, South Africa
- 2Agricultural Research Council–Bacteriology and Zoonotic Diseases Diagnostic Laboratory, Onderstepoort Veterinary Research, Pretoria, South Africa
- 3Agricultural Research Council–Transboundary Animal Diseases Programme, Onderstepoort Veterinary Research, Onderstepoort, South Africa
- 4Faculty of Medical Sciences, School of Veterinary Medicine, University of the West Indies, St. Augustine, Trinidad and Tobago
Q fever in animals and humans and its economic and public health significance has been widely reported worldwide but in South Africa. There are few studies on the prevalence of this zoonosis and its associated risk factors in South African livestock. Therefore, a cross-sectional study was conducted to determine the seroprevalence, molecular prevalence, and risk factors associated with C. burnetii in cattle on farms in South Africa’s Limpopo province. Out of 383 cattle tested for antibodies, the overall seroprevalence was 24.28%. Herd size of >150 (OR: 9.88; 95%CI: 3.92–24.89; p < 0.01) remained associated with C. burnetii seropositivity in cattle. For PCR detection, targeting IS1111 fragment, cattle with no abortion history (OR: 0.37; 95%CI: 0.18–0.77; p < 0.01) and herd size of >150 (OR: 3.52; 95%CI: 1.34–9.24; p < 0.01) remained associated with C. burnetii positivity. The molecular prevalence in sheath scrapings and vaginal swabs by IS1111 PCR was 15.67%. Cohen’s kappa agreement test revealed a fair agreement between the PCR and ELISA results (k = 0.40). Sequence analysis revealed that the amplicons had similarities to the C. burnetii transposase gene fragment, confirming the presence of the pathogen. The higher seroprevalence than molecular prevalence indicated a past C. burnetii infection, no bacterial shedding through vaginal mucus in cows, or preputial discharge in bulls. Similarly, the detection of C. burnetii by PCR in the absence of antibodies could be partly explained by recent infections in which antibodies have not yet been produced against the bacteria, or the level of these antibodies was below the detectability threshold. The presence of the pathogen in cattle and the evidence of exposure, as shown by both PCR and ELISA suggests an active circulation of the pathogen. This study demonstrated that C. burnetii is widespread in the study area and that a herd size of >150 is associated with C. burnetii seroprevalence and molecular prevalence.
1. Introduction
Coxiella burnetii is the etiology of Q fever or coxiellosis in animals and humans. The bacterium is Gram-negative coccobacillus which is obligately intracellular (1, 2). The bacterium belongs to the Gama-subdivision of Proteobacteria within the Legionellales order and family Coxiellaceae, according to 16S rRNA sequence analysis (1). It has been observed that the bacterium can survive in dairy, meat products, aborted foetuses, manure, clothes, and animal feed for a long period (3).
Coxiella burnetii infects a wide range of hosts, including humans, cattle, sheep, goats, and wild animals such as deer, buffaloes, squirrels, and rabbits (4). The main reservoirs for the bacterium are mostly cattle, sheep, and goats; ticks and rodents are considered the natural reservoirs (5, 6). Coxiella burnetii is shed in milk and vaginal secretions by goats and cows and in large numbers in faeces by sheep (7). Coxiella burnetii infection in domestic ruminants is mainly asymptomatic, and as such, the infection may remain unnoticed (3). The disease in these animals may result in huge economic losses as the disease causes reproductive disorders such as late abortions, weak or dead offspring in sheep and goats as well as infertility, abortion, metritis, and mastitis, in cattle which occur during later stages of infection (8, 9).
The disease has been reported worldwide except in New Zealand, and there are a few reports of C. burnetii infection in South African livestock (10, 11). Q fever seroprevalence in cattle has been reported in Zimbabwe (12), Kenya (13), Algeria (14) and in Nigeria (15). In the Netherlands the economic costs of the Q fever outbreak (2007–2010) in about 4,000 human cases acquired from infected dairy goat farms were estimated to be ~0.307 billion EUR (16). Human-to-human spread is rare, transmission to humans is mainly from contact with livestock or domestic animals, including consumption of raw milk, making the disease of public health significance (17, 18).
Coxiella burnetii seroprevalence in cattle has been reported in South Africa’s former Transvaal province, now, Gauteng province, where 7.80% of cattle were seropositive for the pathogen (19). Also, there has been a report on C. burnetii infections where 38.90% of cattle in Bushbuckridge municipality, Mpumalanga province, were positive (20). A recent abattoir-based study by Mangena et al. (10) in the Gauteng province of South Africa reported higher Q fever seroprevalence in cattle than in sheep and pigs. Q fever is of economical and occupational importance, and there is currently no data on seroprevalence and associated risk factors for transmission in the Limpopo Province of South Africa. There is limited data on the molecular prevalence of C. burnetii in cattle from South Africa. As such, we investigated the seroprevalence, molecular prevalence, associated risk factors, and the agreement of molecular and serological diagnostic of C. burnetii in cattle on farms in Limpopo province, South Africa.
2. Materials and methods
2.1. Ethical statement
Permission to conduct the study was obtained from the Animal Ethics Committee and the Research Committee of the Faculty of Veterinary Science (University of Pretoria). In addition, the Section 20 Ethical Approval Committee Certificate was granted for the study by the Department of Agriculture, Forestry, and Fisheries (DAFF). All the samples were collected with the assistance of the state animal health technicians. All the experiments were conducted under biosafety level III conditions at the Agricultural research council – Onderstepoort veterinary research.
2.2. Study area
This cross-sectional study was conducted in Limpopo province, South Africa’s northernmost, with a total area of 125,755 km2 and five district municipalities. Limpopo province is one of South Africa’s warmest regions, with an average daily high temperature of 26°C. For several month, temperatures are consistently above 25°C, sometimes reaching 29°C. The sampled district municipalities are Capricorn which is a stopover between Gauteng province and the northern areas of the country and between the north-western areas and the Kruger National Park and has a total area of 21,705 km2. Sekhukhune district lies in south-eastern part of the province with a total area of 13,528 km2. Lastly, Waterberg district is bordered to the north by Capricorn district municipality and to the south by Sekhukhune district municipality in the east. The Waterberg district has a total area of 44,913 km2.1 These district municipalities represent 50% of the districts in the province as other areas are foot and mouth disease (FMD) restricted areas. The study was conducted in the local municipalities that fall under each district municipality, namely Blouberg, Molemole, Polokwane, Lepelle Nkumpi (Capricorn), Lephalale, Bela-Bela, Mogalakwena and Modimolle (Waterberg) and Greater Tubatse, Ephraim Mogale, Elias Motsoaledi (Sekhukhune) (see text footnote 1; Figure 1).
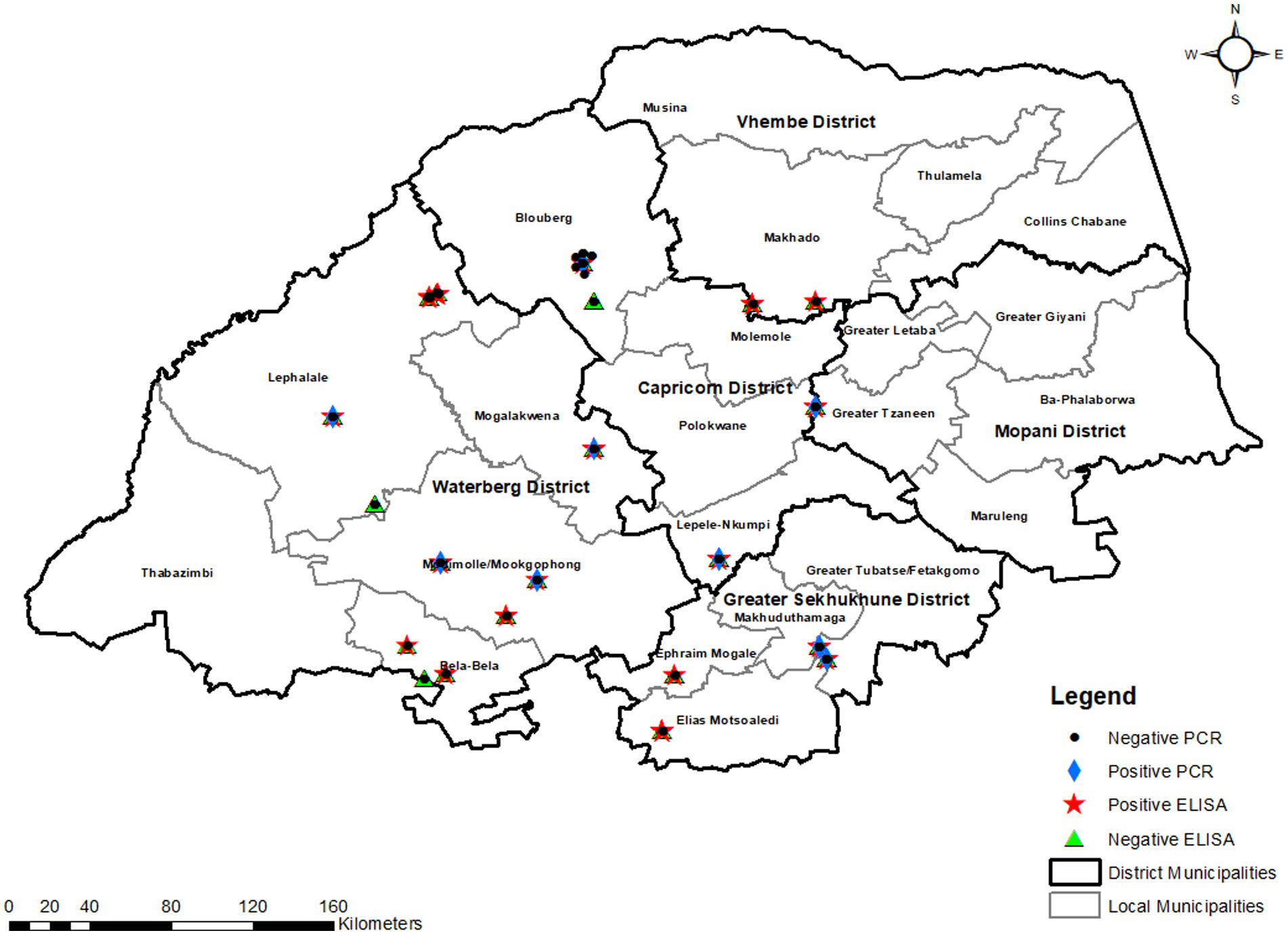
Figure 1. ELISA and PCR-based prevalence in the selected districts and local municipalities of Limpopo province.
2.3. Sample size and study population
The sample size for the study was calculated using the formula by Thrusfield (21) using an expected prevalence ( of 50% because no information on the prevalence of Coxiella burnetii in the study population was available. The desired precision (d) was set to 5%.
In this study, convenience sampling was used because only those farmers willing to participate in the research were included. Only adult and healthy cattle (bulls and cows) on the farms were included in the survey. A minimum of 128 cattle were to be sampled from each of the three districts in Limpopo province. A vast majority of the farms were communal in operation, but commercial farms were available and included in the study. A list of cattle farms, approximate cattle populations, and locations (GPS coordinates) or districts, and contact numbers of the owners were obtained from the Veterinary Division in the Limpopo province. Samples were collected from 22 cattle farms. The samples from each district were distributed according to the following criteria:
a. Farms with <5 cattle: all animals were sampled.
b. Farms with 5–20 cattle: a maximum of 8 were sampled.
c. Farms with 21–30 cattle: a maximum of 12 were sampled.
d. Farms with 31–50 cattle: a maximum of 18 were sampled.
e. Farms with >50 cattle: maximum of 20 were sampled.
Samples were collected from nine farms with 1–50 cattle and four farms with 51–100 cattle. We also collected samples from eight farms with 101–150 cattle and only one farm with more than 150 cattle. A total of 383 cattle were sampled from all the local municipalities of the three district municipalities except two local municipalities that were excluded due to financial constraints.
2.4. Questionnaire survey
A consent form was issued to the farm owner or the attendant after explaining the study’s objectives and background on Q fever in Sepedi/English language to solicit their participation in the study. Prior to this sampling, the farmers and farm workers were unaware of Q fever. Also, a structured questionnaire survey was administered to the farmers in their local language (Sepedi) and in English to those that speak and understand the English language to collect demographic data and potential risk factors for exposure of animals and humans to C. burnetii. The information obtained from the questionnaire included the location of the farm or a dip tank in the province using GPS coordinates. Factors tested include district municipality, type of farm (commercial, communal), herd size (cattle number), sex, and tick infestation or presence of rodents in the farms, abortion history, infertility history, weak offspring history and if manure is used.
2.5. Sample collection
Samples were collected only from healthy adult cattle (bulls and cows) since no consent was given to sample calves or sick cattle. Blood samples were collected from the selected cattle from the coccygeal vein into tubes. Blood samples (10 ml) were collected using BD-Vacutainer® SST™ II Advance 10 ml serum collection tubes. Sera were harvested by centrifuging the clotted blood in collection tubes at 1,000g for 10 min. The harvested sera were stored at −20°C until analysis. Vaginal swabs were collected from cows, including heifers using dry swabs dipped in 10 ml phosphate-buffered saline (PBS) (0.01 M, pH 7.4) and stored in sterile plastic bags. To collect sheath scrapings from bulls, scraping and simultaneous aspiration were performed with Uterine Infusion Pipettes (Kyron Laboratories, Johannesburg, South Africa) connected to a sterile disposable 10 ml hypodermic syringe with a silicon-rubber tube. Bulls were held in a sturdy crush with a neck clamp during sheath collection. Additional restraints included tying one back leg or using a Rau animal Immobilizer placed in the rectum to deliver low-level electrical stimulation. The samples were then transferred to plastic tubes containing 10 ml of PBS (0.01 M, pH 7.4).
2.6. Serological testing
Indirect enzyme-linked immunosorbent assay (iELISA) was used to screen serum samples for Q fever because of its high sensitivity and specificity (22). The IDEXX Q-FEVER 2/strip ELISA kit (IDDEX Laboratories, Liebelfld-Bern, Switzerland) was used to detect IgG antibodies to C. burnetii infection following the manufacturer’s instructions. Before use, all reagents (IDEXX Q Fever 2/strip antibody test kit reagents, as well as the frozen serum samples) were allowed to reach room temperature. Before use, the wash concentrate (10×) was diluted 1/10 with distilled water. All samples and positive and negative controls were prediluted (1/400) using the wash solution. Following the predilution, 100 μl of the positive and negative controls and samples were pipetted into antigen-coated wells. The plates were then tightly sealed and incubated at 37°C for 1 h. The plates were washed three times with the wash solution, and 100 μl of the conjugate was added to each well and incubated for 1 h at 37°C. Subsequently, 100 μl of TMB substrate N.12 was added to each well after washing three times with the wash solution. The plates were then incubated at 26°C for 15 min. After adding 100 μl of the stop solution N.3 into each well, the wavelength was read at 450 nm using a Thermo Lab systems Multiskan MS Original microplate reader (Thermo Fischer Scientific, Waltham, MA, United States). To validate the assay, the following procedures were used: The average optical density value of the two negative controls (NCx) at 450 nanometers (A450) must be equal to and not (≤) 0.500 for the assay to be valid. At 450 nanometers (A450), the two positive controls’ (PCx) average value should be less than or equal to (≤) 2.500. PCx-NCx (A450) must thus be greater than or equal to (≥) 0.300. The sample-to-positive control (S/P) ratio was calculated according to the formula:
In terms of results interpretation, S/P % < 30% denoted a negative result, 30% ≤ S/P% < 40% denoted a suspect, while S/P% ≥40% denoted a positive result.
2.7. Molecular detection
2.7.1. DNA extraction and PCR for detection of Coxiella burnetii
For extraction of DNA from sheath scrapings and vaginal swabs, the High Pure PCR template preparation kit (Roche Diagnostics GmbH, Mannheim, Germany) was used according to the manufacturer’s instructions. A sample volume of 1 ml from sheath scrapings/vaginal swabs in PBS was transferred to a 2 ml centrifuge tube and centrifuged at 1,467g for 10 min. A volume of the supernatant was discarded, leaving ~200 μl to re-suspend the pellet. Briefly, 40 μl of proteinase K and 200 μl of the binding buffer were added to 200 μl of the sample. Following incubation at 70°C for 10 min, 100 μl of isopropanol was added, and the mixture was then centrifuged at 8,000g for 1 min, and the flow-through and collection tube was discarded. A total volume of 500 μl of inhibitor removal buffer was added, the mixture was centrifuged at 8,000g for 1 min, and the flow-through and collection tube was discarded. This was followed by adding 500 μl of the wash buffer and centrifugation at 8,000g for 1 min and the flow-through and collection tube was discarded. Following the addition of 500 μl of wash buffer, the mixture was centrifuged at 8,000g for 1 min, and the flow-through was discarded. After centrifuging for 10 s at 13,000g, the collection tube was discarded, and a new tube was added, along with 200 μl of elution buffer, before centrifuging for 1 min at 8,000g and storing at −20°C. The extracted DNA was amplified using Coxiella IS1111 PCR.
2.7.2. Detection of Coxiella burnetii by PCR
The Coxiella IS1111 PCR was conducted as previously described by Mangena et al. (10). The reactions for C. burnetti detection in seropositive and seronegative samples were performed in a 50 μl reaction targeting the multi-copy transposase gene in insertion element; IS1111 (23) with primers IS1111F 5′CGCAGCACGTCAAACCG3′ and IS1111R 5′TATCTTTA ACAGCGCTTGAACGTC3′. The positive control used was an in-house DNA sample from Mangena et al. (10), which has been sequenced, and the negative control used was distilled water. The reaction mixture contained 2 μl of each 10 μM primer (IS1111F and IS1111R), 25 μl of the Amplicon 2X Taq DNA polymerase Master Mix Red (Amplicon A/S, Odense, Denmark), and 10 μl of the extracted DNA. For C. burnetii DNA amplification, a 146 bp sequence of the IS1111 gene was amplified using BIO-RAD T100™ thermal cycler (BIO-RAD, Singapore) in which the first denaturation was at 95°C for 15 min, 35 cycles were carried out with denaturation at 95°C for the 30s, primer annealing for 30s at 60°C, followed by 72°C for 60 s and the final extension step at 72°C for 10 min.
2.7.3. Gel electrophoresis
The amplicons from all the PCR assays were electrophoresed on a 1.5% ethidium bromide-stained agarose gel at 100 V for 1:30 h or until the separation of the DNA bands was observed. The size of the amplicons was determined using a 100 bp molecular weight marker. Gels were visualized under ultraviolet light using a gel documentation system (Chemi XRQ, Vacutec, United Kingdom).
2.8. Sequence verification
The PCR products were sent to Inqaba Biotechnologies, South Africa, for sequencing of both forward and reverse strands of the IS1111 gene using an ABI sequencer. Due to financial constraints, only seven amplicons were sequenced. Sequences were edited manually, and pairwise alignments were undertaken using the BioEdit Sequence alignment editor (version 7.1.9) and the sequences were analyzed on the NCBI BLAST platform for species identification2 by megablast.
2.9. Data analysis
A univariable analysis using Chi-square test was applied to screen the variables, district municipality (Capricorn, Sekhukhune, Waterberg), type of farm (commercial, communal), herd size (1–50, 51–100, 101–150, >150), sex (female or male) and tick infestation or presence of rodents in the farms (yes or no), abortion history (yes or no), infertility history (yes or no), weak offspring history (yes or no) and manure uses (fertilizer or not used) against disease exposure at the individual level. In the initial analysis, all the variables were tested individually for their unconditional association with outcome using the Chi-square test (p ≤ 0.30). The variable that generated the highest p > 0.3 during univariate logistic regression analysis was excluded. Variables with a p ≤ 0.3 in the univariate analysis were used to develop a multilevel logistic regression model for each exposure. The logistic model was reduced by stepwise elimination removing variables with p > 0.05. Farm type and manure use were excluded from the ELISA multilevel logistic regression model analysis, and tick infestation or presence of rodents in the farms was excluded from the PCR multilevel logistic regression model analysis because they were confounding factors. This process was repeated until the model with the lowest Akaike’s second-order information criterion (AIC) was identified. The odds ratios with 95% confidence interval results were noted. Cohen’s Kappa agreement test between the PCR assay and ELISA was done. Data was captured and cleaned in a Microsoft Excel spreadsheet. All analysis was done using StataCorp. 2015. Stata Statistical Software: Release 14.2 College Station, TX: StataCorp LP.
3. Results
3.1. Seroprevalence and associated risk factors
In the univariable analyses, there were significant differences in seropositivity to C. burnetii antibodies between farms that use manure and those that do not use (p = 0.03). There were also significant associations between Q fever seroprevalence in cattle and herd size (p < 0.01), and farm type (communal and commercial; p = 0.02). There were no significant differences in seropositivity to C. burnetii antibodies in infertility history (p = 0.13) and abortion history (p = 0.20; Table 1). The final multivariable logistic regression model showed that for herd size, the odds of ELISA positivity were higher in farms that had a herd size of >150 (OR: 9.88; 95%CI: 3.92–24.89; p < 0.01) as compared to those that had cattle <50. Of the 383 cattle tested for antibodies against C. burnetii, the overall seroprevalence was 24.28% (93/383) (Table 2).
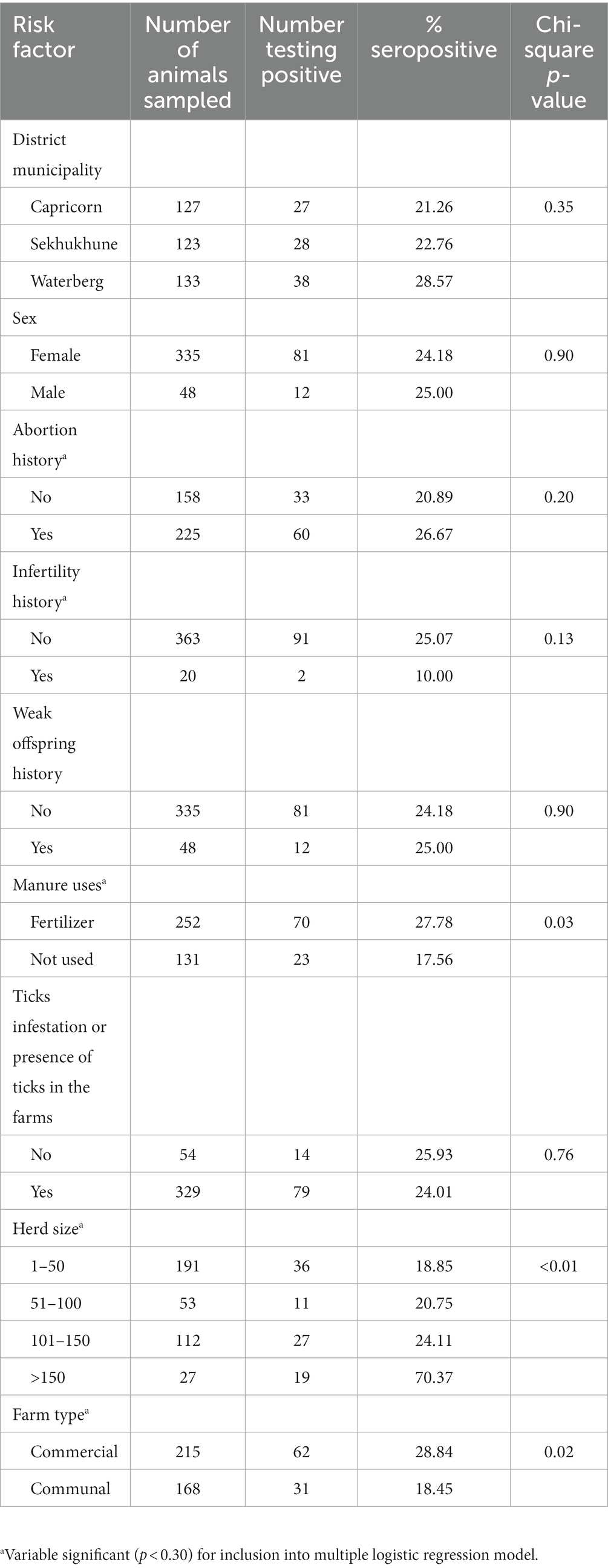
Table 1. Seroprevalence of Coxiella burnetii (Q fever) in cattle on farms in Limpopo province, South Africa, and univariable analysis of possible risk variables in cattle for Q fever seropositivity.
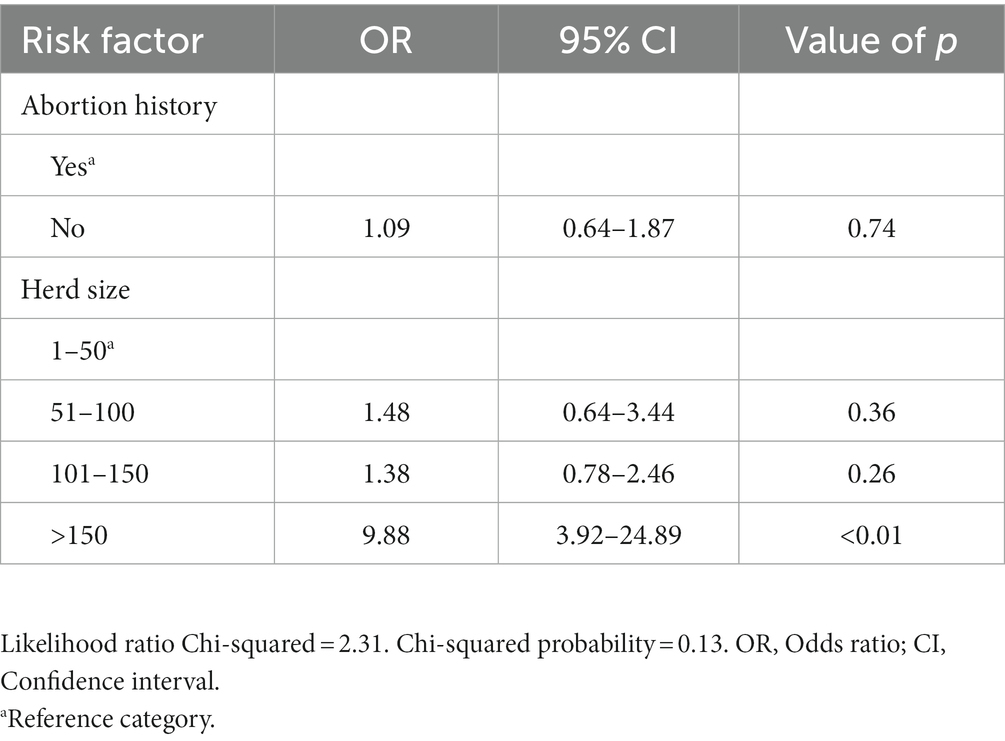
Table 2. Multivariable analysis of risk factors associated with seropositivity to C. burnetii in cattle farms in Limpopo province, South Africa.
3.2. Molecular prevalence and associated risk factors
In the univariable analyses, we observed significant differences between PCR positivity and abortion history (p < 0.01) and herd size (p < 0.01). There were no significant differences in PCR positivity to tick infestation/presence of ticks (p = 0.07), manure uses (p = 0.18), sex (p = 0.14) and farm type (p = 0.13; Table 3). The odds of PCR positivity were higher in farms that had no abortion history compared to those that had abortion history. We also observed that the odds of PCR positivity were higher in farms with cattle >150 than farms with cattle <50 and between 50 and 150 (Table 4). The farms with no abortion history (OR: 0.37; 95%CI: 0.18–0.77; p < 0.01) and herd size of >150 (OR: 3.52; 95%CI: 1.34–9.24; p < 0.01) remained associated with C. burnetii positivity by PCR. Of the 383 cattle samples tested by IS1111 PCR, 60 (15.67%) cattle were positive by PCR. Molecular detection of C. burnetii from sheath scrapings and vaginal swabs by PCR targeting the 262 IS1111 is illustrated as an example in Figure 2.
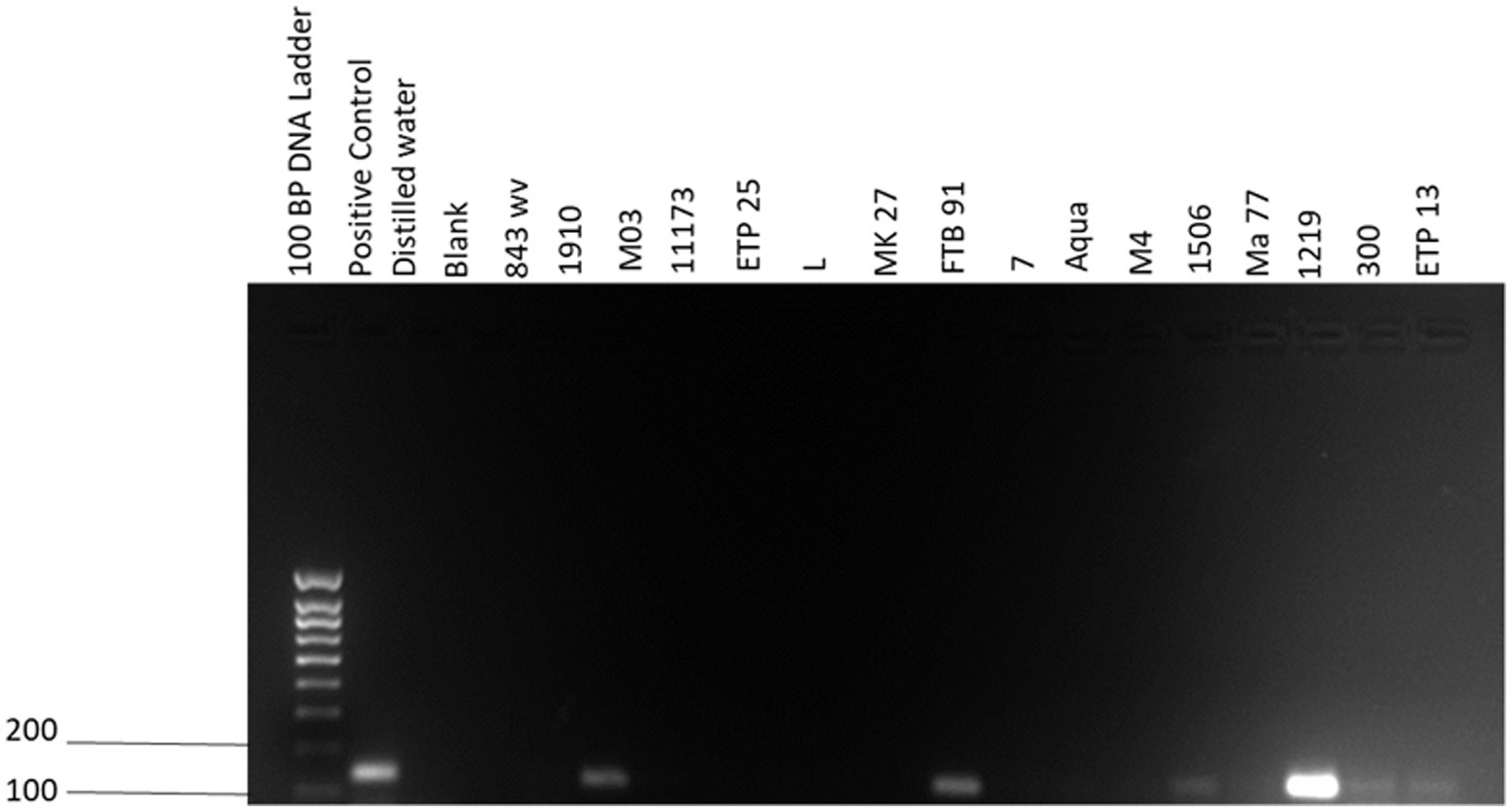
Figure 2. IS1111 gel electrophoresis of PCR amplicons. Quick-Load® 100 bp DNA ladder was used in the first lane (New England Biolabs, Ipswich, MA, United States). The positive control is an in-house positive control from Mangena et al. (10), the negative control is distilled water, and the blank is an empty lane. 843 wv, 1910, M03, 11173, ETP 25, L, MK27, FTB 91, 7, Aqua, M4, 1506, Ma 77, 1219, 300 and ETP 13 are cattle vaginal swab samples.
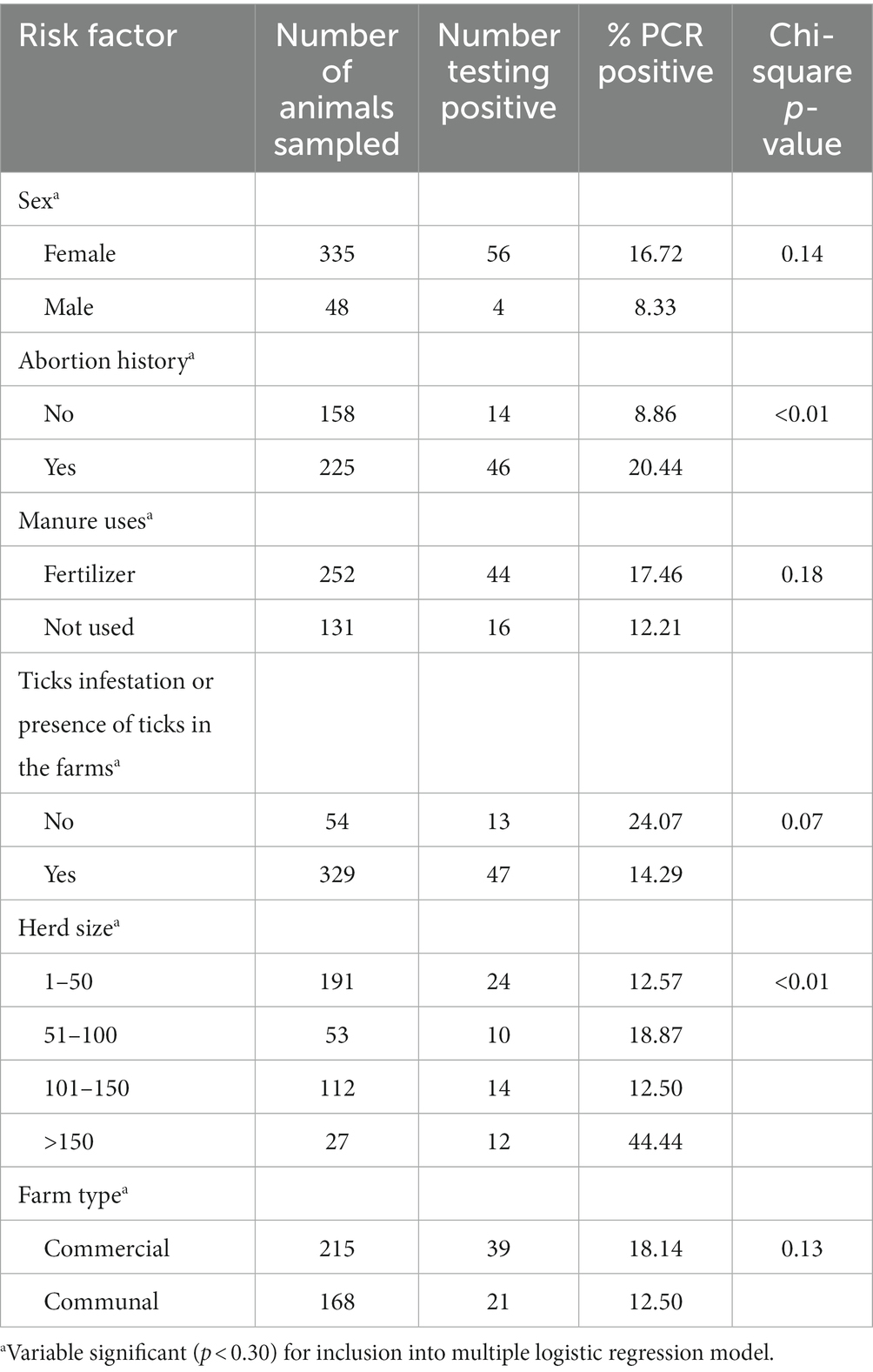
Table 3. Molecular prevalence of Q fever and univariable analysis of risk factors associated with PCR positivity to C. burnetii in cattle on farms in Limpopo province, South Africa.
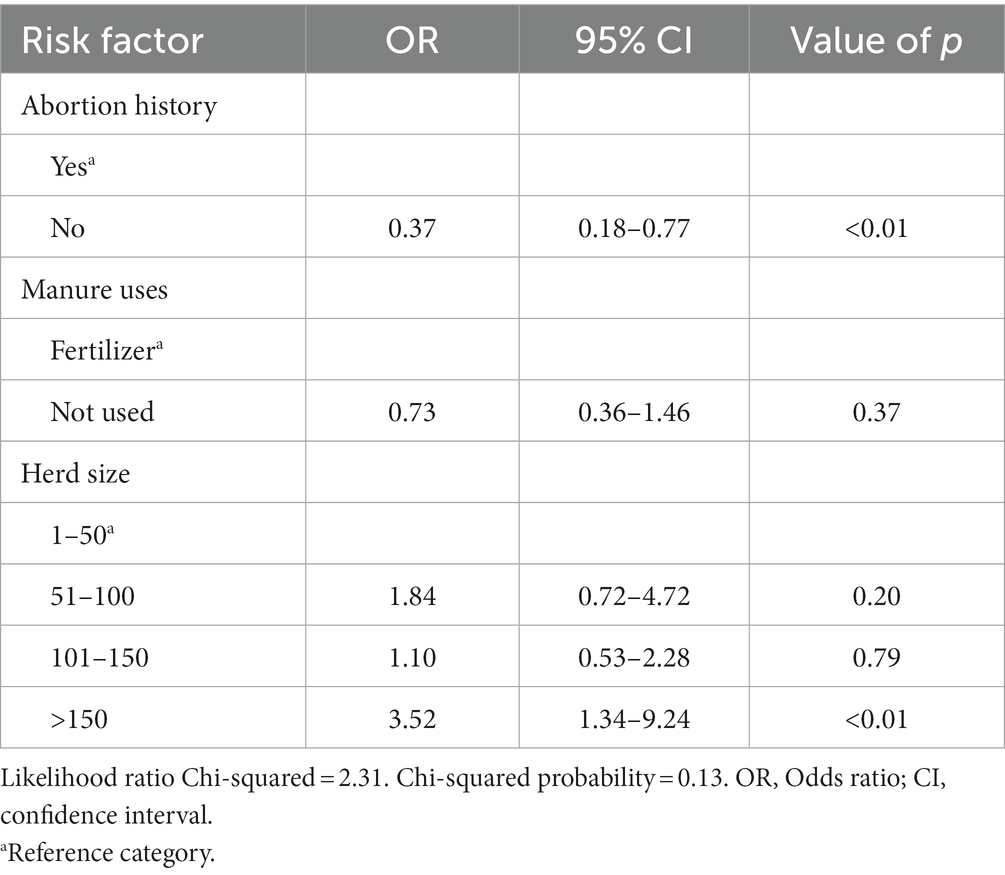
Table 4. Multivariable analysis of risk factors associated with PCR positivity to C. burnetii in cattle farms in Limpopo province, South Africa.
The Cohen’s kappa agreement test was done to assess if there is agreement between the PCR and ELISA results which revealed a kappa value of 0.40. Relatively fewer cattle, (60, 15.67%) were positive by PCR than by ELISA (93, 24.28%). Of the total ELISA-negative samples, (22, 7.59%) were positive by PCR and we observed a correlation of 93.41%. Of the overall ELISA-positive samples, there was an agreement of (38, 40.86%) positive by PCR. Out of all the 323 samples that were negative by PCR, (55, 20.52%) were positive by ELISA and a correlation of 79.48% was observed. Of the overall PCR-positive samples there was an agreement of (38, 63.33%) positive by ELISA assay.
4. Discussion and conclusions
A seroprevalence of 24.28% observed for Q fever in this study using indirect ELISA is the first evidence of Coxiella burnetii presence in cattle on farms in Limpopo province, South Africa. The seroprevalence observed in this study differs from other surveys conducted in the country. A survey conducted in the abattoirs in Gauteng province of South Africa by Mangena et al. (10) reported a lower seroprevalence of 9.40% than in the current study in cattle using an indirect ELISA. The seroprevalence noted in this study is lower than that in a survey by Adesiyun et al. (20), which reported a significantly higher prevalence of 38.9% in cattle in Bushbuckridge municipality, Mpumalanga province of South Africa, using an indirect ELISA. Gummow et al. (19) reported a seroprevalence of 7.8% using CFT in the Transvaal (Gauteng) province of South Africa, which is much lower than what was obtained in this study. The differences in the seroprevalence obtained in all these studies can be ascribed to factors such as study locations (Limpopo, Mpumalanga, Gauteng provinces of South Africa) and the years in which samples were collected (November 2020 to September 2021, April to September 2013, March 1985 to July 1986, respectively). These variables potentially influence exposure and detection of C. burnetii infections (24–26). The difference in the seroprevalence noted in all these studies may also be due to geographical variability. Previous studies have shown that, geographic region may influence seroprevalence (27–29). Mpumalanga has a subtropical climate with mild to cool winters that transition to cold and frosty conditions in the Highveld and an average temperature of 21°C which favors the development of pests such as ticks (30, 31). In Gauteng province, only the months of July and August experience the coldest weather, with an average daily temperature of 24°C. Limpopo has an average daily high temperature of 26°C. The majority of Limpopo province is warm all year, with little cold experienced during winter (30).
In comparison to other countries in Africa, the seroprevalence reported in the current study is comparable and falls within the range noted in other African countries. A study in Egypt revealed a seroprevalence of 19.3% in cattle using an indirect ELISA (32), which is slightly lower than the findings in the current study. Seroprevalences of 6.5% using complement fixation test and 32% using commercial ELISA kit were reported in Malawi and Cameroon’s Adamawa region cattle by Staley et al. (33) and Scolamacchia et al. (31), respectively. Mwololo et al. (13) reported a seroprevalence of 3.00% in cattle in Kenya’s Tana River and Garissa counties using iELISA.
Since we only sampled cattle in our study, we could not determine prevalence variation by animal species, and we also did not document any cattle housing system; however, the seroprevalence data inthe current study shows that the cattle in the study area are exposed to Coxiella burnetii with prevalence comparable to other studies (34). However, other studies, have shown that prevalence varies according to animal species (35, 36).
Farms with herd size >150 (p < 0.01) had a significantly higher risk of being seropositive to C.burnetii infection compared to farms with herd size between 1 and 50. The findings agree with the report of Cadmus et al. (15), who reported a higher seroprevalence in the herd size category of above 50 but a lower seroprevalence in smaller herds (10–30). Adamu et al. (37) demonstrated that the larger the herd size, the greater the risk of disease transmission through interaction with other infected animals or herds while grazing on contaminated pasture or sharing water stations.
This is the first study in South Africa to determine the molecular prevalence of C.burnetii in cattle. Other studies have reported molecular prevalence somewhere else. In the current study, we detected a lower molecular prevalence (15.67%) by PCR. Studies by Cardinale et al. (38) and Knobel et al. (6) reported lower molecular prevalences of 0.81 and 2.1% in cattle vaginal swabs samples in Reunion Island and western Kenya, respectively. A study conducted in India also reported a lower molecular prevalence of 1.81% in cattle vaginal swabs samples (39). Anastácio et al. (40) reported a higher prevalence (20.0%) in bulk tank milk samples in Portugal. This can be attributed to the type of sampling, the detection method used, and the types of samples since these could affect detection rates (10, 40, 41).
This current study demonstrated a significant association (p < 0.01) between C. burnetii positivity by PCR and cattle with no abortion history on the farms compared to those with history of abortion and this can be explained, in part, by the fact that infected ruminants can either be asymptomatic or symptomatic showing symptoms such as metritis, and mastitis (3). To reduce Q fever infection in cattle, vaccination with a phase 1 vaccine has been shown to reduce the occurrence of abortions and other reproductive problems in livestock (42, 43). However, South Africa has no policy plan for a cattle vaccination program.
The association between herd size and an increased risk of C. burnetii infection has been well-established (44, 45). In our study, we observed a high frequency (44.44%) of cattle shedding C. burnetii in the herd size category of >150. Also, we also discovered that farms with more than 150 cattle were more likely to be PCR positive (p < 0.01). This association can be explained, in part, by a larger population at risk, an increased risk of pathogen introduction and transmission within and between herds, such as increased feed and animal supply, and more professionals working at or visiting the farm (46). Larger herds are more likely to contract and develop Q fever, and the number of animals must be considered a risk factor for C. burnetii spread (40).
A comparison of the positivity detected by ELISA and PCR assay revealed a kappa value of 0.40 which is defined as a fair agreement by McHugh (47). In the current study, we observed a lower molecular prevalence detected by PCR compared to the seroprevalence of Q fever assayed by iELISA. This finding could be attributed to some of the cattle shedding C. burnetii through other routes (faecal or milk) (48), which were not assayed by PCR in this current study. These data also suggest that C. burnetii is widespread within the sampled areas. In this current study, ELISA positivity and PCR negativity indicated a past C. burnetii infection with no bacterial shedding through vaginal mucus in cows or preputial discharge in bulls. Infected animals shed C. burnetii in their faeces, vaginal discharges, and milk for several days or months after parturition (49–52). In contrast, the presence of PCR-positive samples and ELISA-negative samples could be explained by a recent C. burnetii infection in cattle in which antibodies have not yet been produced against the bacteria, or the level of these antibodies is below the detectability threshold (14, 40, 53). The presence of the pathogen in cattle and the evidence of exposure, as shown by both PCR and ELISA positivity in the current study suggests an active circulation of the pathogen, and possibly past exposure reflected by the ELISA results, as earlier reported (54).
5. Conclusion
Finally, the study examined the seroprevalence, PCR prevalence, and risk factors for C. burnetii in cattle on farms in South Africa’s Limpopo province. This study determined that C. burnetii is present in the study areas (Capricorn, Waterberg, and Sekhukhune). Herd size with cattle >150, was significantly associated with Q fever seroprevalence. Cattle or farms with no abortion history and herd size with cattle >150 were found to be significantly associated with Q fever molecular prevalence as detected by PCR. Coxiella burnetii should be considered a possible source of human Q fever in the study areas and widespread in cattle.
6. Limitations
The present study had limitations, including the number of samples collected lower than the calculated sample size for the survey. Failure to sample two local municipalities due to financial constraints, resulted in a smaller sample size and two districts municipalities that are FMD-restricted areas. Some farmers in the sampled district municipalities were unwilling to participate in the study and were thus excluded.
7. Recommendations
Conduct large-scale studies in South Africa in animals and people to determine the prevalence of C. burnetii and the risk factors of C. burnetii infection in humans. Management measures should be developed based on the identified risk factors for exposure to Q fever. Future studies should investigate the seroprevalence and PCR prevalence of C. burnetii infection to differentiate between past and current exposure and conduct a molecular characterization of the pathogen to determine the strains circulating in the country. Other studies should be conducted on ticks and Coxiella-like bacteria. A long-term plan for animal vaccination should be developed to reduce the number of infections and animal-to-human transmission. Awareness campaigns should also be launched to educate farmers about the risks of Q fever and proper hygiene practices. It is recommended that a surveillance system for Q fever control and prevention be developed in South Africa.
Data availability statement
The original contributions presented in the study are included in the article/supplementary material, further inquiries can be directed to the corresponding author.
Ethics statement
The animal study was reviewed and approved by Faculty of Veterinary Science (University of Pretoria).
Author contributions
VS conducted the experiments, wrote, and edited the manuscript. NG, MM, and AA equally conceptualized, wrote, edited, and reviewed the manuscript while YN conducted statistical analysis of the data and co-authored the manuscript. All authors contributed to the article and approved the submitted version.
Funding
Financial support to conduct the study was obtained from the Red Meat Research and Development in South Africa (RMRD-SA) and the Department of Trade, Industry, and Competition (DTIC), South Africa – THRIP (THRIP/23/30/11/2017).
Acknowledgments
The authors would like to thank the cattle farmers in various districts of Limpopo province, South Africa and State veterinarians from the Department of Agriculture and Rural development, Limpopo province, for their assistance with sampling and Kgaogelo Mogano for her assistance in plotting a map.
Conflict of interest
The authors declare that the research was conducted in the absence of any commercial or financial relationships that could be construed as a potential conflict of interest.
Publisher’s note
All claims expressed in this article are solely those of the authors and do not necessarily represent those of their affiliated organizations, or those of the publisher, the editors and the reviewers. Any product that may be evaluated in this article, or claim that may be made by its manufacturer, is not guaranteed or endorsed by the publisher.
Footnotes
References
1. Eldin, C, Mélenotte, C, Mediannikov, O, Ghigo, E, Million, M, Edouard, S, et al. From Q fever to Coxiella burnetii infection: a paradigm change. Clin Mmicrobiol Rev. (2017) 30:115–90. doi: 10.1128/CMR.00045-16
2. Mohabati Mobarez, A, Khalili, M, Mostafavi, E, and Esmaeili, S. Molecular detection of Coxiella burnetii infection in aborted samples of domestic ruminants in Iran. PLoS One. (2021) 16:e0250116:e0250116. doi: 10.1371/journal.pone.0250116
3. Pexara, A, Solomakos, N, and Govaris, A. Q fever and prevalence of Coxiella burnetii in Milk. Trends Food Sci Technol. (2018) 71:65–72. doi: 10.1016/j.tifs.2017.11.004L508
4. Marrie, T, Williams, J, Schlech, WIII, and Yates, L. Q fever pneumonia associated with exposure to wild rabbits. Lancet. (1986) 327:427–9. doi: 10.1016/S0140-6736(86)92380-9
5. Galiero, A, Fratini, F, Cammà, C, Di Domenico, M, Curini, V, Baronti, I, et al. Occurrence of Coxiella burnetii in goat and ewe unpasteurized cheeses: screening and genotyping. Int J Food Microbiol. (2016) 237:47–54. doi: 10.1016/j.ijfoodmicro.2016.08.008
6. Knobel, DL, Maina, AN, Cutler, SJ, Ogola, E, Feikin, DR, Junghae, M, et al. Coxiella burnetii in humans, domestic ruminants, and ticks in rural western Kenya. J Natl Malaria Soc. (2013) 88:513–8. doi: 10.4269/ajtmh.12-0169
7. Bauer, B, Prüfer, L, Walter, M, Ganter, I, Frangoulidis, D, Runge, M, et al. Comparison of Coxiella burnetii excretion between sheep and goats naturally infected with one cattle-associated genotype. Pathogens. (2020) 9:652. doi: 10.3390/pathogens9080652
8. Benaissa, MH, Ansel, S, Mohamed-Cherif, A, Rachid, R, Benfodil, K, Khelef, D, et al. Seroprevalence and risk factors for Coxiella burnetii, the causative agent of Q fever in the dromedary camel (Camelus dromedarius) population in Algeria. Onderstepoort J Vet Res. (2017) 84:1–7. doi: 10.4102/ojvr.v84i1.1461
9. Roest, HI, Bossers, A, van Zijderveld, FG, and Rebel, JM. Clinical microbiology of Coxiella burnetii and relevant aspects for the diagnosis and control of the zoonotic disease Q fever. Vet Q. (2013) 33:148–60. doi: 10.1080/01652176.2013.843809
10. Mangena, M, Gcebe, N, Pierneef, R, Thompson, PN, and Adesiyun, AA. Q fever: Seroprevalence, risk factors in slaughter livestock and genotypes of Coxiella burnetii in South Africa. Pathogens. (2021) 10:258. doi: 10.3390/pathogens10030258
11. Tissot-Dupont, H, and Raoult, D. Q fever. Infect Dis Clin North Am. (2008) 22:505–14. doi: 10.1016/j.idc.2008.03.002
12. Kelly, PJ, Matihewman, LA, Mason, PR, and Raoult, D. A review of the disease and the results of a serosurvey of humans, cattle, goats and dogs. SAMJ, S. Afr. Med. (1993) 83:21–25.
13. Mwololo, D, Nthiwa, D, Kitala, P, Abuom, T, Wainaina, M, Kairu-Wanyoike, S, et al. Sero-epidemiological survey of Coxiella burnetii in livestock and humans in Tana River and Garissa counties in Kenya. PLOS Negl. Trop. Dis. (2022) 16:e0010214. doi: 10.1371/journal.pntd.0010214
14. Menadi, SE, Chisu, V, Santucciu, C, Di Domenico, M, Curini, V, and Masala, G. Serological, molecular prevalence and genotyping of Coxiella burnetii in dairy cattle herds in northeastern Algeria. Veterinary Sciences. (2022) 9:40. doi: 10.3390/vetsci9020040
15. Cadmus, SI, Akporube, KA, Ola-Daniel, F, Adelakun, OD, and Akinseye, VO. Seroprevalence and associated factors of brucellosis and Q-fever in cattle from Ibarapa area, Oyo state, South-western Nigeria. Pan Afr Med J. (2020) 36:370. doi: 10.11604/pamj.2020.36.370.24925
16. Ullah, Q, Jamil, T, Saqib, M, Iqbal, M, and Neubauer, H. Q fever—a neglected zoonosis. Microorganisms. (2022) 10:1530. doi: 10.3390/microorganisms10081530
17. Crump, JA, Morrissey, AB, Nicholson, WL, Massung, RF, Stoddard, RA, Galloway, RL, et al. Etiology of severe non-malaria febrile illness in northern Tanzania: a prospective cohort study. PLoS Negl Trop Dis. (2013) 7:e2324. doi: 10.1371/journal.pntd.0002324
18. World Organization for Animal Health (OIE). (2019) Manual of diagnostic tests and vaccines for terrestrial animals 2019 chapter 3.1.16 Q fever. Available at: https://www.oie.int/index.php?id=169&L=0&htmfile=sommaire.htm.L535
19. Gummow, B, Poerstamper, N, and Herr, S. The incidence of Coxiella burnetii antibodies in cattle in the Transvaal. Onderstepoort J Vet Res. (1987) 54:569–71.
20. Adesiyun, AA, Knobel, DL, Thompson, PN, Wentzel, J, Kolo, FB, Kolo, AO, et al. Sero-epidemiological study of selected zoonotic and Abortifacient pathogens in cattle at a wildlife-livestock Interface in South Africa. Vector-Borne Zoonotic Dis. (2020) 20:258–67. doi: 10.1089/vbz.2019.2519
21. Thrusfield, M. Veterinary epidemiology 3rd ed. London: Blackwell Science Ltd., John Wiley & Sons (2007). 227–247.
22. Peter, O, Dupuis, G, Bee, D, Lüthy, R, Nicolet, J, and Burgdorfer, W. Enzyme-linked immunosorbent assay for diagnosis of chronic Q fever. J. Clin. Microbiol. (1988) 26:1978–1982. doi: 10.1128/jcm.26.10.1978-1982.1988
23. De Bruin, A, van Alphen, PT, van der Plaats, RQ, ND de Heer, L, Reusken, CB, van Rotterdam, BJ, et al. Molecular typing of Coxiella burnetii from animal and environmental matrices during Q fever epidemics in the Netherlands. BMC Vet. Res. (2012) 8:1–9. doi: 10.1186/1746-6148-8-165
24. Larson, PS, Espira, L, Grabow, C, Wang, CA, Muloi, D, Browne, AS, et al. The sero-epidemiology of Coxiella burnetii (Q fever) across livestock species and herding contexts in Laikipia County, Kenya. Zoonoses Public Health. (2019) 66:316–24. doi: 10.1111/zph.12567
25. Muleme, M, Stenos, J, Vincent, G, Campbell, A, Graves, S, Warner, S, et al. Bayesian validation of the indirect immunofluorescence assay and its superiority to the enzyme-linked immunosorbent assay and the complement fixation test for detecting antibodies against Coxiella burnetii in goat serum. Clin Vaccine Immunol. (2016) 23:507–14. doi: 10.1128/CVI.00724-15
26. Plummer, PJ, McClure, JT, Menzies, P, Morley, PS, Van den Brom, R, and Van Metre, DC. Management of Coxiella burnetii infection in livestock populations and the associated zoonotic risk: a consensus statement. J Vet Intern Med. (2018) 32:1481–94. doi: 10.1111/jvim.15229
27. Georgiev, M, Afonso, A, Neubauer, H, Needham, H, Thiéry, R, Rodolakis, A, et al. Q fever in humans and farm animals in four European countries, 1982 to 2010. Eur Secur. (2013) 18:20407. doi: 10.2807/ese.18.08.20407-en
28. Hilbert, A, Blaha, I, Froehlich, A, Hensler, E, Reith, P, Henning, K, et al. Aspects seroepidemiological studies on Q fever in unvaccinated dairy cattle herds. Berl Munch Tierarztl Wochenschr. (2014) 127:149–57.
29. Wittenbrink, MM, Gefäller, S, Failing, K, and Bisping, W. The effect of herd and animal factors on the detection of complement-binding antibodies against Coxiella burnetii in cattle. Berl Munch Tierarztl Wochenschr. (1994) 107:185–91.
30. Municipality. Spatial development framework. Bloemfontein: Mangaung Metropolitan Municipality. (2012) Available at: https://municipalities.co.za/
31. Scolamacchia, F, Handel, IG, Fèvre, EM, Morgan, KL, Tanya, VN, and Bronsvoort, BM. Serological patterns of brucellosis, leptospirosis and Q fever in Bos indicus cattle in Cameroon. PLoS One. (2010) 5:8223. doi: 10.1371/journal.pone.0008623
32. Klemmer, J, Njeru, J, Emam, A, El-Sayed, A, Moawad, AA, Henning, K, et al. Q fever in Egypt: epidemiological survey of Coxiella burnetii specific antibodies in cattle, buffaloes, sheep, goats and camels. PLoS One. (2018) 13:e0192188. doi: 10.1371/journal.pone.0192188
34. Zangue, CT, Kouamo, J, Ngoula, F, Tawali, LPMB, Talla, MM, Mbeba, LYK, et al. Seroprevalence and risks factors associated with Coxiella burnetii infection in slaughterhouse zebu cattle (Bos indicus) from northern regions of Cameroon. Epidemiologia. (2022) 3:434–42. doi: 10.3390/epidemiologia3040033
35. Johnson, SA, Kaneene, JB, Asare-Dompreh, K, Tasiame, W, Mensah, IG, Afakye, K, et al. Seroprevalence of Q fever in cattle, sheep and goats in the Volta region of Ghana. Vet Med Sci. (2019) 5:402–11. doi: 10.1002/vms3.160
36. Vanderburg, S, Rubach, MP, Halliday, JE, Cleaveland, S, Reddy, EA, and Crump, JA. Epidemiology of Coxiella burnetii infection in Africa: a OneHealth systematic review. PLoS Negl Trop Dis. (2014) 8:E2787. doi: 10.1371/journal.pntd.0002787
37. Adamu, SG, Kabir, J, Umoh, JU, and Raji, MA. Seroprevalence of brucellosis and Q fever (Coxiellosis) in cattle herds in Maigana and Birnin Gwari agro-ecological zone of Kaduna state, Nigeria. Trop Anim Health Prod. (2018) 50:1583–9. doi: 10.1007/s11250-018-1598-3
38. Cardinale, E, Esnault, O, Beral, M, Naze, F, and Michault, A. Emergence of Coxiella burnetii in ruminants on Reunion Island? Prevalence and risk factors. PLoS Negl Trop Dis. (2014) 8:e3055. doi: 10.1371/journal.pntd.0003055
39. Yadav, JP, Magotra, A, Bangar, YC, Kumar, A, Yadav, AS, Garg, AR, et al. Coxiella burnetii in cattle and their human contacts in a gaushala (cattle shelter) from India and its partial com 1 gene sequence-based phylogenetic analysis. Anim Biotechnol. (2021):1–10. doi: 10.1080/10495398.2021.1955701
40. Anastácio, S, Carolino, N, Sidi-Boumedine, K, and Da Silva, GJ. Q fever dairy herd status determination based on serological and molecular analysis of bulk tank milk. Transbound Emerg Dis. (2016) 63:e293–300. doi: 10.1111/tbed.12275
41. Barkallah, M, Gharbi, Y, Hassena, AB, Slima, AB, Mallek, Z, Gautier, M, et al. Survey of infectious etiologies of bovine abortion during mid-to late gestation in dairy herds. PLoS One. (2014) 9:e91549. doi: 10.1371/journal.pone.0091549
42. Arricau-Bouvery, N, Souriau, A, Bodier, C, Dufour, P, Rousset, E, and Rodolakis, A. Effect of vaccination with phase I and phase II Coxiella burnetii vaccines in pregnant goats. Vaccine. (2005) 23:4392–402. doi: 10.1016/j.vaccine.2005.04.010
43. Hogerwerf, L, Van Den Brom, R, Roest, HI, Bouma, A, Vellema, P, Pieterse, M, et al. Reduction of Coxiella burnetii prevalence by vaccination of goats and sheep, the Netherlands. Emerg Iinfect Dis. (2011) 17:379–86. doi: 10.3201/eid1703.101157
44. Agger, JF, and Paul, S. Increasing prevalence of Coxiella burnetii seropositive Danish dairy cattle herds. Acta Vet Scand. (2014) 56:1–4. doi: 10.1186/s13028-014-0046-2
45. Nusinovici, S, Frössling, J, Widgren, S, Beaudeau, F, and Lindberg, A. Q fever infection in dairy cattle herds: increased risk with high wind speed and low precipitation. J Hyg. (2015) 143:3316–26. doi: 10.1017/S0950268814003926
46. Schimmer, B, Luttikholt, S, Hautvast, JL, Graat, EA, Vellema, P, and van Duynhoven, YT. Seroprevalence and risk factors of Q fever in goats on commercial dairy goat farms in the Netherlands, 2009-2010. BMC Vet Res. (2011) 7:1–14. doi: 10.1186/1746-6148-7-81
47. McHugh, ML. Interrater reliability: the kappa statistic. Biochem Med. (2012) 22:276–82. doi: 10.11613/BM.2012.031L488
48. Guatteo, R, Beaudeau, F, Berri, M, Rodolakis, A, Joly, A, and Seegers, H. Shedding routes of Coxiella burnetii in dairy cows: implications for detection and control. Vet Res. (2006) 37:827–33. doi: 10.1051/vetres:2006038
49. Berri, M, Souriau, A, Crosby, M, Crochet, D, Lechopier, P, and Rodolakis, A. Relationships between the shedding of Coxiella burnetii, clinical signs and serological responses of 34 sheep. Vet Rec. (2001) 148:502–5. doi: 10.1136/vr.148.16.502
50. Hatchette, TF, Hudson, RC, Schlech, WF, Campbell, NA, Hatchette, JE, Ratnam, S, et al. Goat-associated Q fever: a new disease in Newfoundland. Int Conf Emerg Infect Dis. (2001) 7:413–9. doi: 10.3201/eid0703.017308
51. Ho, T, Htwe, KK, Yamasaki, N, Zhang, GQ, Ogawa, M, Yamaguchi, T, et al. Isolation of Coxiella burnetii from dairy cattle and ticks, and some characteristics of the isolates in Japan. Jpn J Microbiol. (1995) 39:663–71. doi: 10.1111/j.1348-0421.1995.tb03254.x
53. Muskens, J, Van Engelen, E, Van Maanen, C, Bartels, C, and Lam, TJGM. Prevalence of Coxiella burnetii infection in Dutch dairy herds based on testing bulk tank milk and individual samples by PCR and ELISA. Vet Rec. (2011) 168:79:79–9. doi: 10.1136/vr.c6106
Keywords: IS1111, PCR, South Africa, cattle, Q fever, Coxiella burnetii, ELISA
Citation: Sadiki V, Gcebe N, Mangena ML, Ngoshe YB and Adesiyun AA (2023) Prevalence and risk factors of Q fever (Coxiella burnetii) in cattle on farms of Limpopo province, South Africa. Front. Vet. Sci. 10:1101988. doi: 10.3389/fvets.2023.1101988
Edited by:
Maureen T. Long, University of Florida, United StatesReviewed by:
Sara Savic, Scientific Veterinary Institute Novi Sad, SerbiaGerardo Acosta-Jamett, Austral University of Chile, Chile
Copyright © 2023 Sadiki, Gcebe, Mangena, Ngoshe and Adesiyun. This is an open-access article distributed under the terms of the Creative Commons Attribution License (CC BY). The use, distribution or reproduction in other forums is permitted, provided the original author(s) and the copyright owner(s) are credited and that the original publication in this journal is cited, in accordance with accepted academic practice. No use, distribution or reproduction is permitted which does not comply with these terms.
*Correspondence: Vhahangwele Sadiki, VmhhaGFuZ3dlbGVfc2FkaWtpQHlhaG9vLmNvbQ==
†ORCID: Abiodun A. Adesiyun, https://orcid.org/0000-0001-9470-9421