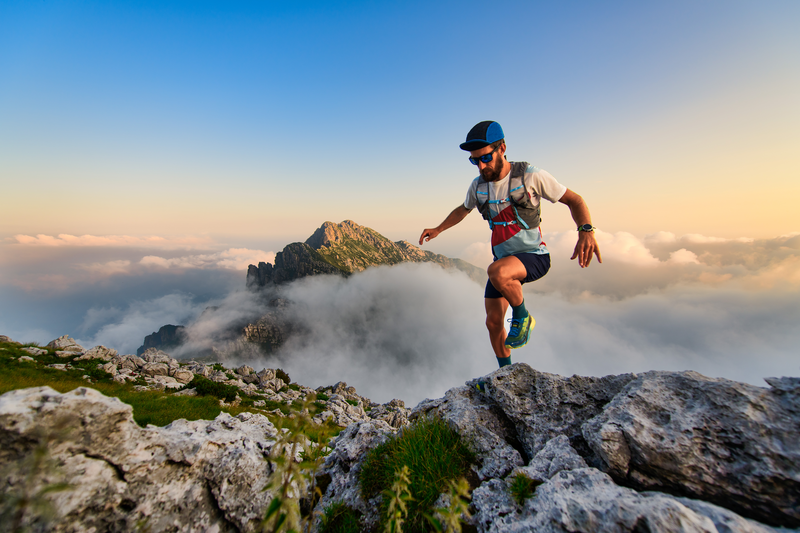
95% of researchers rate our articles as excellent or good
Learn more about the work of our research integrity team to safeguard the quality of each article we publish.
Find out more
ORIGINAL RESEARCH article
Front. Vet. Sci. , 20 March 2023
Sec. Animal Nutrition and Metabolism
Volume 10 - 2023 | https://doi.org/10.3389/fvets.2023.1090107
This article is part of the Research Topic Feedlot Cattle Nutrition and Metabolism View all 10 articles
This study was designed to evaluate a spray-dried multivalent polyclonal antibody preparation (PAP) against lactate-producing bacteria as an alternative to monensin (MON) to control ruminal acidification. Holstein cows (677 ± 98 kg) fitted with ruminal cannulas were allocated in an incomplete Latin square design with two 20 days period. Cows were randomly assigned to control (CTL), PAP, or MON treatments. For each period, cows were fed a forage diet in the first 5 days (d−5 to d−1), composed of sugarcane, urea and a mineral supplement, followed by a 74% concentrate diet for 15 days (d 0 to d 14). There were no treatment main effects (P > 0.05) on dry matter intake (DMI) and microbial protein synthesis. However, there was a large peak (P < 0.01) of intake on d 0 (18.29 kg), followed by a large decline on d 1 (3.67 kg). From d2, DMI showed an increasing pattern (8.34 kg) and stabilized around d 8 (12.96 kg). Higher mean pH was measured (P < 0.01) in cattle-fed MON (6.06 vs. PAP = 5.89 and CTL = 5.91). The ruminal NH3-N concentration of CTL-fed cows was lower (P < 0.01) compared to those fed MON or PAP. The molar concentration of acetate and lactate was not affected (P > 0.23) by treatments, but feeding MON increased (P = 0.01) propionate during the first 4 days after the challenge. Feeding MON and PAP reduced (P = 0.01) the molar proportion of butyrate. MON was effective in controlling pH and improved ruminal fermentation of acidosis-induced cows. However, PAP was not effective in controlling acidosis. The acidosis induced by the challenge was caused by the accumulation of SCFAs. Therefore, the real conditions for evaluation of this feed additive were not reached in this experiment, since this PAP was proposed to work against lactate-producing bacteria.
Intensive cattle management systems often include increased amounts of cereal grains in the diet to increase energy input and improve performance (1). Thus, energy-dense diets typically fed to highly productive ruminants can lead to digestive disorders, such as ruminal acidosis, in response to the rapid ruminal fermentation of starch and sugars (2). Ruminal acidosis is the most economically important metabolic disorder in intensive cattle production systems, and that exists in both acute and subacute forms (3). Ruminal acidosis has been reported as the second most common health problem affecting feedlot cattle in Brazil (1).
Sub-acute ruminal acidosis (SARA) is an ongoing problem in the dairy and feedlot sector, responsible for the onset of different pathologies, such as rumenitis, parakeratosis, laminitis, and metabolic acidosis. Streptococcus bovis was the major initiators of ruminal acidosis by producing lactate as the major fermentation product under low ruminal pH (4). This results in consistent economic losses in both beef and dairy industries primarily due to decreased efficiency of milk production, premature culling, and reduction in milk yield and milk fat (5, 6) as well as a reduction on efficiency and performance of beef cattle (6, 7).
In this context, ionophore antibiotics, such as monensin (MON), are widely used in feedlot diets for acidosis control by modulating rate the of ruminal fermentation reducing dry matter intake (8, 9). Moreover, this ionophore modify rumen fermentation dynamics by inhibiting growth of Gram-positive bacteria, including lactate-producing rumen bacteria such as Streptococcus bovis (10), reducing ruminal lactate production and the risk of acidosis. Moreover, studies reported that monensin reduces ruminal protein degradation and decreased microbial protein synthesis (11, 12), due to inhibitory effects on hyper-ammonia-producing bacteria (13). Despite the beneficial effects of monensin, the use of antibiotics and growth promoters has raised the concern about the risk of these products in increasing bacterial resistance to antibiotics and, consequently, possible risks to human health (14). However, these mechanisms of resistance are not fully understood, since the genes responsible for ionophore resistance in ruminal bacteria have not been identified (15).
As a result, there is a search for antibiotic replacements to modulate ruminal fermentation to control acidosis and increase animal performance (16). An alternative to antibiotics is passive immunization with polyclonal antibodies preparations (PAP) against specific groups of ruminal bacteria, such as lactate-producing ruminal bacteria (Streptococcus bovis) and bacteria related to liver abscesses (Fusobacterium necrophorum). In this context, the use of PAP against lactate-producing ruminal bacteria have led to a reduction in the concentration of rumen lactate (17–19), and also in the control of acidosis in animals during the rapid transition to a high-concentrate diet (16, 20).
Recent ruminal metabolism studies with PAP were conducted under the most extreme condition of abrupt transition to high-concentrate diets (18, 20). However, most experiments carried out with acidosis induction for the evaluation of additives were designed to monitor rumen fermentation in short periods (between 1 and 2 d; 3.5–7 g PAP/d (18, 20). In this context, there is a lack of information on the persistence of action of feed additives, as well as the administration time necessary to reduce the negative effects of this nutritional disorder on ruminal fermentation. Furthermore, this allows the comparison of PAP with other additives, such as MON, under these conditions of the longer evaluation period.
Therefore, it was hypothesized that, in a situation of induction of ruminal acidosis, MON reduces the flow of microbial protein to the intestine. Furthermore, PAP would be effective in preventing lactic acidosis after an abrupt change to high-concentrate diet. Thus, the present study aimed to evaluate the spray-dried multivalent polyclonal antibody preparation against lactate-producing bacteria (Streptococcus bovis, Fusobacterium necrophorum, and Lactobacillus ssp.) as an alternative to monensin to control ruminal acidification in cow fed high concentrate diets.
All protocols and procedures followed in this study were approved by the São Paulo State University Ethical Committee for Animal Research (CONCEA, 2013), São Paulo State University, Botucatu campus, Brazil.
Polyclonal antibodies were produced by CAMAS Inc. (Le Center, MN, USA). The commercial product contains 39.5% immunoglobulins against Streptococcus bovis (ATCC 9809), 17.6% against Lactobacillus ssp. (ATCC 4356; 14917; 9649 and 7469), 13.20% against Fusobacterium necrophorum (ATCC 27852), 17.6% against E. coli O157:H7, and endotoxins. For PAP preparation, the procedures were similar to those described by DiLorenzo et al. (17), with the exception that a multivalent PAP was tested, rather than antibodies to specific organisms. Streptococcus bovis and Lactobacillus ssp. are the main lactic acid-producing bacteria during the process of acidosis; Fusobacterium necrophorum is strongly associated with liver abscess in cattle; while E. coli is a commensal in the rumen and can be opportunistically pathogenic in humans. Endotoxins are the lipopolysaccharides that are present in the walls of gram-negative ruminal bacteria, being released in situations of bacterial death. The PAP in solid form was obtained by spray drying and was maintained in hermetically sealed packages Protected from heat light during the experimental period.
The experiment was conducted at the School of Veterinary Medicine and Animal Science at the University of São Paulo (USP), Campus of Pirassununga, São Paulo, Brazil. Nine non-pregnant and non-lactating Holstein cows with an average live weight of 677 ± 98 kg previously fitted with ruminal cannulas were used. The animals were housed in individual stalls (Stall size: 1.5 m bunk space per 9 m. 13.5 m2 per animal) with sand bedding, feed bunk, and access to drinking water. The facility had fans suspended from the ceiling that was turned on automatically during the hottest hours of the day, to mitigate the effects of ambient temperature.
An incomplete Latin square design was used, divided into two experimental periods of 20 d each (d−5 to d 14). The choice for only two experimental periods is attributed to the attempt to avoid diluting the challenge effect of high-concentrate diets by potential metabolic memory in an attempt to avoid large intakes of concentrate. Cattle were submitted to the following treatments: (1) Control (CTL); (2) Monensin (MON); (3) Polyclonal Antibody Preparations (PAP) against Streptococcus bovis, Fusobacterium necrophorum, and Lactobacillus ssp. The PAP and Monensin were inserted through the ruminal cannula twice a day, before each meal and inside envelopes made of absorbent paper, between days 0 and 14 of each experimental period. The MON was administered at a dose of 300 mg/d, which corresponds to 3 g/d of the commercial product Rumensin (Elanco Animal Health, Indianapolis, IN). This commercial product contains 10% sodium monensin per kilogram of product. The PAP (CAMAS Inc, Le Centre, MN, USA) was administered at a dose of 3 g/d (corresponding to 10 mL of liquid product).
The experiment had a total duration of 55 d, divided into two periods of 20 d each (d−5 to d 14), with an interval of readaptation to the roughage diet of 15 d between periods. The washout period was used to reestablish normal ruminal pH conditions. During d−5 to−1, the cows received only a roughage diet, composed of sugarcane, urea (1.4% DM), and a mineral supplement to supply the following levels of crude protein (CP), NDF, and ADF (DM basis): 14.05, 43.98, and 22.18%, respectively. From d 0 to d 14, a 74% concentrate diet was offered (Table 1), composed of sugarcane, high-moisture corn silage, soybean meal, and vitamin and mineral premix.
Between d−5 to d 14, the following variables were measured: individual dry matter intake; ruminal pH; ruminal concentration of total lactate and short-chain fatty acids (SCFA); NH3-N concentrations, and microbial protein synthesis.
Before the two experimental periods, ~20 kg of rumen content was extracted from each animal and these portions were mixed. After this procedure, the same amount of rumen content, removed and already mixed, was returned to each animal. This procedure aimed to homogenize the ruminal microbial population before the application of the experimental treatment.
Diets were offered twice a day, at 800 h and 1,600 h. The experimental diet was administered as a total mixed ration (TMR), with a roughage: concentrate ratio of 26:74, in which the roughage source used was fresh sugarcane (2.9% CP, 47.48% NDF, and 25.68% ADF, DM basis) chopped with a theoretical mean particle size of 1.14 cm (21). The concentrate was composed of soybean meal (44.12% CP, 20.57% NDF, and 7.30% ADF, DM basis), and high-moisture corn silage (7.91% CP, 6.22% NDF, and 3.31% ADF, DM basis; Table 1). The DM, mineral matter (MM), CP, ether extract (EE), calcium, and phosphorus analyzes were performed according to AOAC (22), while the NDF corrected for ash and ADF were performed according to Van Soest et al. (23). For NDF analysis, α-amylase and urea were added. The starch concentration was carried out according to Pereira and Rossi Jr. (24), in which extraction of carbohydrates was performed according to Hendrix (25). The diet was formulated according to the NRC (26) and evaluated in the Cornell Net Carbohydrate and Protein System (CNCPS program, version 5.0.40) (27).
To evaluate dry matter intake in kg (DMI), DMI expressed as % of BW (DMI % BW), DMI expressed as g/kg of metabolic weight (DMI g/kg BW0,75), the amount of diet offered and refused were collected and weighed daily, from d−5 to d 15. All feed bunks were examined every morning. If there was no feed remaining, the amount offered was raised by 10%. If up to 10% remained, the amount of feed offered was not changed and if the surplus was >10%, the feed offered was reduced by 10%. Additionally, the fluctuation of dry matter intake (DMIF) was calculated for each animal, as the difference in dry matter intake between consecutive days, according to the methodology proposed by Bevans et al. (28), as follows:
The DMIF was performed between days−1 and 3 of each experimental period.
At each daily collection, at least 500 mL of rumen content was removed at three different points of the rumen (through an electric vacuum pump), which were returned to the rumen-reticulum after collecting the appropriate aliquots for determination of lactate, NH3-N, and SCFA molar concentrations. The collections were carried out daily, from d−5 to d 14 at 1,100 h (3 h after the morning feeding, carried out at 800 h) (29). Immediately after collection, 100 mL of rumen fluid was used for pH determination in a portable digital potentiometer (HANNA instruments HI8424), calibrated with pH 4.0 and pH 7.0 buffer solutions. Regarding the determination of the days on which the animals presented acidosis (DEA), according to definitions created by several authors (6, 9, 30), sub-acute acidosis was considered to occur when the pH was ≤ 5.6. So, the number of days in which the ruminal pH of each animal was pH < 5.66 was counted. The adoption of the second decimal place is due to the sensitivity of the pH measuring device. Thus, pH values starting at 5.66 were considered pH 5.7 and discarded as acidotic pH. Only the post-challenge experimental phase was accounted for (d 0 to d 14), and the results were expressed as a percentage of this phase.
To determine the ruminal total lactate, 2 mL of rumen fluid was placed in test tubes and subsequently measured by the colorimetric technique according to Pryce (31). For short-chain fatty acid (SCFA) analyses that included acetate, propionate, and butyrate, a fraction of ~100 mL of rumen content was centrifuged at 2000 x g for 20 min; 2 mL of the supernatant was added to 0.4 mL of formic acid and frozen at−20 °C for further analyses, according to Erwin et al. (32). The SCFA were measured by gas chromatography (Thermo Scientific®, model Focus GC) with an automatic sample injector (Thermo Electron Corporation®, model AS-3000) equipped with a 2 m long, and 1/5” diameter glass column was used, packed with Carbopack B-DA/4% Carbowax® 20M 80-120 (Supelco®) and flame ionization detector (FID) maintained at 270°C. The gas chromatograph oven was maintained at 190°C during the analysis and the injector temperature was 220°C. The carrier gas was high-purity H2, maintained in a flow of 30 mL/min. The number of repetitions per sample was the one necessary for the difference between readings to be <5%.
To determine the concentration of NH3-N, fractions of 2 mL of rumen fluid were placed in test tubes containing 1 mL of 1N sulfuric acid solution and stored under refrigeration until the analysis by colorimetry (Kjeltec 2300 Analyzer Unit, Tecator, Hoganas, Sweden), according to the method described by Kulasek (33) and adapted by Foldager (34).
Analyses to determine microbial protein synthesis were performed at the Laboratory of Animal Biochemistry and Physiology of the VNP-FMVZ/USP, based on the quantification of urinary purine derivatives (PD), according to the methodology described by Valadares et al. (35) and Rennó (36), considering the absorption of purines from the formula suggested by Verbic et al. (37).
Urine samples (50 mL, spot sample) were collected from all animals on d−3; 3, and 14 of each experimental period, ~3 h after feeding. The urine was filtered and 10 ml aliquots were immediately diluted in 40 mL of 0.018M sulfuric acid to avoid bacterial destruction of purine derivatives and uric acid precipitation, then stored at −15 °C for further analysis of allantoin and acid. uric. A pure urine sample was stored for the determination of total nitrogen compounds, urea and creatinine.
Creatinine concentrations were determined by commercial kits (Laborlab®), using an enzymatic reaction in a spectrophotometer (SBA-200 Celm®). The total daily urinary volume was estimated by dividing the daily urinary excretions of creatinine by the observed values of creatinine concentration in the urine of the spot samples according to Oliveira et al. (38).
The daily urinary excretion of creatinine was estimated from the established mean daily excretion of 24.05 mg/kg body weight for dairy cows (39). Thus, with the average daily excretion of creatinine and the concentration of creatinine (mg/dL) in the spot urine sample, the total daily volume of urine, in liters per cow, was estimated. The levels of allantoin and uric acid in the urine were determined by the colorimetric method, according to the methodology of Fujihara et al. (40), described by Chen and Gomes (41).
The total excretion of PD was calculated as the sum of allantoin and uric acid excreted in the urine, expressed in mmol/day. Absorbed microbial purines (AP, mM/day) were calculated from the urinary excretion of purine derivatives (PD, mM/day), using the equation:
where 0.84 is the recovery of purines absorbed as purine derivatives and 0.236 is the endogenous excretion of PD (42).
Absorbed microbial purines were also assessed, considering the endogenous excretion of 0.512*BW0.75 and the recovery of 0.70 found by Gonzalez-Ronquillo et al. (43). Microbial protein synthesis (Pmic, g of N/day) was calculated based on the AP (absorbed microbial purines, mM/day), using the Equation (41):
where 70 is the N content in the purines (mgN/mol); 0.134, the purine N: total N ratio in bacteria (35); and 0.83, the intestinal digestibility of microbial purines. To obtain the microbial crude protein synthesis, the Pmic data were multiplied by the Kjeldahl factor of 6.25.
The experimental design was an incomplete Latin square. Data were analyzed by Statistical Analysis System software (SAS version 9.2; SAS Inst., Inc., Cary, NC, USA), in which the model included the effects of treatments as fixed, and period and animal as random. Before the analysis of variance, the normality of the residuals was verified by the SHAPIRO-WILK Test (PROC UNIVARIATE), and the variances were compared by the “F” Test. Data (dependent variable) that did not meet these premises were submitted to logarithmic [Log (X+1)] or square root [SR (X+1/2)] transformation. The original or transformed data, when the latter procedure was necessary, were subjected to analysis of variance that separated the effects of treatments and period as sources of variation, plus the factor repeated measures over time, referring to the different sampling days. Such analysis was performed using the MIXED procedure of SAS. The effect of time analysis was only reported when the interaction between time and treatment effects was significant. The differences between means were performed using the Tukey test. Effects were considered significant at P < 0.05.
No interactions were found between day and treatment for DMI (P = 1.00), DMI % BW (P = 0.99), and DMI g/kg BW0,75 (P = 0.99). The administration of feed additives via ruminal cannula did not result in changes (P > 0.11) in DMI when compared to the control (Tables 2, 3). However, a day effect (P < 0.01) was found for all dry matter intake variables mentioned above. There was a large peak of intake on d 0 (18.29 kg or 2.60% of BW), the day when the experimental diet was started, followed by a large decline on d 1 (3.67 kg or 0.54 % of BW; Figure 1). From d 2, DMI showed an increasing pattern (8.34 kg or 1.23 % of BW) and stabilized around d 8 (12.96 kg or 1.86 % of BW) for all treatments. The mean difference in intake between the forage diet on d−1 and the experimental diet on d 0 was 10.61 kg. On the other hand, cows ingested 14.63 kg less feed on d 1, compared to d 0. Additionally, a decrease in the variation of DMI was observed from d 2 (4.67 kg) and d 3 (1.29 kg), which corroborates the increasing pattern found in DMI and DMI % BW.
Table 2. Dry matter intake in kg (DMI, kg/d), DMI as a percentage of body weight (DMI, %BW), and DMI based on metabolic BW (DMI, g/kg BW0.75) of cows induced to ruminal acidosis receiving polyclonal antibody preparations (PAP) or monensin (MON).
Table 3. Fluctuation in dry matter intake (DMIF, kg) of cows induced to ruminal acidosis receiving polyclonal antibody preparations (PAP) or monensin (MON).
Figure 1. Dry matter intake expressed in kg [DMI, (A)] and as a percentage of body weight [DMI %BW, (B)] of cows induced to ruminal acidosis. The bold arrow represents the change from the 100% forage diet to the 74% concentrate diet containing the treatments.
No interaction was observed between day and treatment (P = 0.19) for rumen pH 3 h after feeding. Intraruminal addition of MON resulted in higher pH (P < 0.01) at the third postprandial hour compared to the other treatments (MON = 6.06 vs. CTL = 5.91 and PAP = 5.89; Table 4). Additionally, a day effect was found (P < 0.01) for rumen pH, where the decline in pH commenced on day 0 and the maximum decline was on day 1 (Figure 2A) regardless of treatments.
Table 4. Ruminal fermentation variables of cows induced to acidosis and receiving polyclonal antibodies preparations (PAP) or monensin (MON).
Figure 2. Ruminal pH (A), acetate [% molar proportion, (B)], propionate [% molar proportion, (C)], acetate: propionate ratio (D), and N-NH3 concentration (E) of cows induced to ruminal acidosis receiving polyclonal antibodies (PAP) or monensin (MON). The bold arrow represents the change from the 100% forage diet to the 74% concentrate diet containing the treatments.
There was no interaction between day and treatment (P = 0.78) for rumen lactate. The molar concentration of lactate remained low throughout the experimental period (0.23 mM). The molar concentration of SCFA was higher in cows treated with PAP (P = 0.02) but there was no interaction between day and treatment (P = 0.20). Furthermore, a treatment effect was observed (P = 0.02), in which cows receiving PAP presented the greatest concentration. Similarly, a day effect was detected (P < 0.01), in which the total SCFA increased from ~90 mM in the pre-challenge period to a maximum value of 135 mM on d 1, stabilizing around 129 mM from d 8 (data not shown).
The interaction between day and treatment was not significant (P = 0.38) for the molar concentration of acetate. Additionally, neither feed additive influenced acetate concentrations (P = 0.33; Table 4). There was a time effect (P < 0.01) for the molar proportion of this SCFA (Figure 2B), where the molar proportions of acetate went from ~60% to around 55% on d 1, after the abrupt change to the experimental diet.
There was an interaction between day and treatment (P < 0.01; Figure 2C), in which an increase in the molar proportions of propionate was observed in cows receiving MON during the first 4 days following the challenge, an increase of 78% on d 4 when compared to control (MON = 30.42 vs. PAP = 21.63 and CTL = 17.07 mM on d 4). An interaction between day and treatment (P = 0.01) was also observed for the acetate/propionate ratio, in which MON reduced this proportion in the three first days following the challenge (Figure 2D).
There was no interaction between day and treatment (P = 0.25) for the molar concentration of butyrate, but both feed additives were effective (P < 0.01; Table 4) in decreasing ruminal butyrate (MON = 15.42 and PAP = 16.35 vs. CTL = 18.43).
The interaction between day and treatment was not significant (P = 0.90) for NH3-N concentration. However, a treatment effect was found (<0.01), in which animals treated with additives had higher concentrations than CTL animals (MON = 14.74 and PAP = 13.64 vs. CTL = 11.20). Moreover, the day effect was significant (P < 0.0001), where the animals went from a concentration of around 19 mg/dL when fed a forage diet, to 27 mg/dL on d 0, 3.56 on d 1, and remaining around 12 mg/dL (with large variations) until the end of the experiment (Figure 2E).
No interactions were observed between day and treatment for any of the evaluated experimental variables (P > 0.05). Moreover, the treatments with feed additives did not result in significant differences (P > 0.05, Table 5) for any experimental variable related to microbial protein synthesis (Figure 3).
Table 5. Microbial protein synthesis in cattle induced to ruminal acidosis receiving polyclonal antibodies (PAP) or monensin (MON).
Figure 3. Total urine excreted (A), urinary allantoin (B), total purines (C), and absorbed microbial purines (D), microbial-N (E) and microbial crude protein (F) of cows induced to ruminal acidosis. The bold arrow represents the change from the 100% forage diet to the 74% concentrate diet containing the treatments.
The results of the current study indicate that monensin was effective in controlling subacute ruminal acidosis in cattle abruptly shifted to a high-concentrate diet, and also in improving rumen fermentation by altering SCFA molar proportions. In addition, the acidosis challenge induced by the abrupt increase in diet energy levels was caused by the accumulation of SCFAs. As a result, PAP was not effective in controlling acidosis, which can be explained by the fact that this product targets the control of lactate-producing bacteria. However, it is worth mentioning that the experimental design, as well as the protocol used in the present study, which included a simultaneous increase in diet density and different treatments inserted through the rumen cannulae, may have influenced the results. Since cows get used to consecutive acidosis challenges, an incomplete Latin square was adopted. Despite of these limitations, and considering the complexity of acidosis induction studies, the experimental protocol used in the study was efficient for inducing acidosis.
The reduction in DMI and increases in feed efficiency in animals fed high-concentrate diets in response to MON supplementation are well documented in the literature (8, 9). Similarly, reductions in intake in animals receiving MON have been found in comparative performance studies with PAP (44, 45). However, in the present study, MON did not reduce the DMI after an abrupt transition to high-concentrate diets, and this corroborates the absence of difference in DMI reported by Rodrigues et al. (46) where they found no reduction in dry matter intake in animals that received MON, also compared to PAP. From a metabolic point of view, the evaluation of the intake behavior of animals fed high-concentrate diets with MON may provide better information than the simple quantification of the total daily intake, because this negative effect of MON on DMI is related to a lower DMI per meal (47, 48), which consequently leads to an increase in the number of meals without changing the total amount of dry matter ingested daily (49, 50). In this context, this is the best mode of action for a feed additive to prevent subacute acidosis, associated with increased concentrations of SCFA (9), and, consequently, the control of dry matter intake prevents excessive fermentation of high starch content. These facts may explain the faster recovery of pH to normal rumen conditions in animals treated with MON in the present study.
However, the low DMI observed on day 1 may be explained by the increase in rumen osmolarity, as a result of reduced absorption and an increase in substances that contribute to an increase in osmolarity, such as glucose, SCFAs, and lactate, may lead to an influx of fluid from the blood into the rumen and, consequently, decrease DMI (51). In the present study, the greatest fluctuations in DMI occurred between day−1 and day 1, where the lowest average daily pH (5.43) was observed on day 1. Increases in DMI variation have been identified as an indicator of subacute acidosis (52, 53). However, the fluctuations decreased over the days, and instead of a cyclic pattern, as would be expected in animals that experienced ruminal acidosis, an increase in DMI was observed from day 2 onwards. The large amount of feed consumed by cows on the day of the challenge (d 0) can be explained by the fact that ruminants show a preference for feeds or diets that compensate for nutrient deficiencies (54). During the pre-challenge phase, cows were fed a high-forage diet. This may have increased the avidity for the concentrate after the challenge, increasing the DMI from day 2.
Furthermore, by evaluating pH and lactate concentration, it is possible to classify the acidosis caused by the challenge in this study as subacute (9). However, it is worth noting that in the present study, a single sample was collected 3 h after feeding on each day of the experimental period (29), but a recent study reported that rumenocentesis should be performed in the late afternoon or evening to maximize the probability of detecting animals with pH values below the threshold level (55, 56). Subacute ruminal acidosis (SARA) is characterized by a decline in ruminal pH below 5.8 or 5.6 (57). However, the diagnosis of SARA should not be made based on rumen pH alone, but in combination with symptoms to make SARA identification more accurate and feasible, including fecal consistency, rumen motility, and inflammatory markers (57). Nevertheless, there is not complete agreement on the etiology and symptoms of SARA (57, 58). In recent years, the development of sequencing technologies has enriched the study of SARA by expanding the understanding of the rumen microbiota (59). Changes in the structure and function of the rumen microbiota have been reported during SARA, including decreased bacterial richness and diversity, decreased relative abundance of fibrolytic bacteria and increased levels of amylolytic bacteria, and increased levels of propionate and total SCFA (59, 60). In the present study, feeding MON was more effective in minimizing reductions in rumen pH when compared to PAP and CTL. It is well documented in the literature that MON improve feed efficiency by reducing dry matter intake (47), reducing ruminal lactate production because this ionophore shifts the rumen microbial population by inhibiting growth of Gram-positive bacteria, including lactate-producing rumen bacteria such as Streptococcus bovis (10). However, considering only the average pH from day 0 to day 14, it was observed that cattle fed MON had a minimized risk of ruminal acidosis (pH 5.84), whereas cows from the other two treatments presented, on average, acidotic pH (pH 5.60).
In addition, the high-grain diet challenge resulted in some clinical manifestations, which are indicative of SARA, such as diarrhea in all animals on day 1. The change in feces could be due to the large flow of readily fermentable carbohydrates from the rumen to the intestine, causing excessive fermentation in these organs (61). Also, the high osmolarity promoted by the experimental diet described in animals with subacute ruminal acidosis, may retain fluid in the lumen and alter fecal consistency (62). The increase in total SCFA concentration in the rumen of cows fed PAP was not sufficient to reduce ruminal pH to a level below that of cows fed no feed additive. Therefore, feeding PAP may play a role in controlling rumen acidification during an abrupt change from a high-forage to a high-concentrate diets.
In this context, the effect of MON in modulating the lactate-producing bacteria population in vivo and in vitro in acute acidosis situations is well described in the literature (51). The microbiological changes in acute and lactic acidosis are also well documented in the literature (9), but very little is known about the changes that occur in ruminants with subacute acidosis (63). This may be because MON is a feed additive with a broad-spectrum of activity as it reduces the rumen fermentation rate, which may be explained by the effect of treatment on the total SCFA concentration. It is worth mentioning that the lactate concentration was low in the present study. Based on this fact, the lactate levels in the ruminal fluid of cattle with subacute ruminal acidosis are usually not increased, which shows that the total concentration of SCFA is more important in subacute acidosis and the lactate concentration is more important in acute acidosis (6, 57, 64). Also, SARA may promote a gene expression change on rumen epithelium, due to an accumulation of intracellular cholesterol and its metabolites in response to a higher substrate supply (total SCFA). This could stimulate cell proliferation, increase membrane permeability, and induce epithelial inflammation, that eventually disrupts rumen homeostasis and negatively affects cow health (59).
In contrast, the lack of effect of PAP in controlling rumen pH may have been due to the high specificity of these antibodies. Since SARA was induced by high concentrations of SCFA, the favorable conditions for a true evaluation of this additive were not presented because the target microorganisms were mostly acid-tolerant bacteria. In clinical and sub-clinical acidosis, the rumen pH decreases to a point where cellulolytic bacteria are inhibited and lactate-producing bacteria predominate, particularly Streptococcus bovis and Lactobacillus sp. (65). However, the observation of the lack of results in studies performed with PAP in solid form (66) raises doubts about whether the loss of antibody activity occurs during the conversion of the liquid to solid phase. Cassiano et al. (18) reported that neither liquid nor powdered forms of PAP altered rumen acidosis variables in adapted or unadapted animals. In this context, further comparative studies between the two forms of product presentation and new drying techniques are recommended.
The effects of MON in manipulating rumen fermentation are due to changes in rumen microbial ecology (67, 68). The increase in the molar proportion of propionate, the decrease in the acetate/propionate ratio, and the decrease in the molar proportions of butyrate in animals treated with MON can be explained by the inhibition of the growth of the population of Gram-positive bacteria, which are sensitive to ionophores and produce mainly acetate, butyrate, H2, and formate (67). However, when the above changes are verified, a decrease in the molar proportion of acetate would be expected, an effect confirmed by others studies (67–69). Probably, the decrease in the molar proportions of acetate occurs in experimental situations where rumen fermentation is already stabilized, which was not the case in this study. Thus, given the abrupt change in diet and the start of administration of the treatments on the day of challenge, it may have taken some time for rumen bacterial community to change. In addition, low acetate/propionate ratios are desirable to some extent in cattle, to maintain the necessary daily weight gain (48, 70). However, low acetate/propionate ratios can lead to a decrease in DMI and weight gain in unstable situations (53). In dairy cattle, low acetate/propionate ratios may decrease milk fat and an increase in body condition scores (71).
Furthermore, SARA has been characterized as a condition of elevated SCFA concentration that can lead to a critical rumen pH because of the imbalance between the production and absorption of these acids (57, 72), causing reduced microbial protein synthesis (73). In the present study, no changes in microbial protein synthesis were observed in response to the treatments. Studies have reported a reduction in microbial protein flux into the gut and decreased efficiency of microbial protein synthesis in response to MON (74). However, these studies show results in situations in which fermentation would theoretically be stabilized, in contrast to the present study in which animals were induced to acidosis. A possible explanation for the lack of effect of the treatments would be that abrupt changes, promoted by both diet and additives, contributed to a greater growth of the ruminal microbial population, and shortened the time for MON to contain bacterial growth. As a result, a linear increase in purine derivatives excretion and an increase in microbial protein were observed during the experimental period, explained by the increase in energy density of the diet and the greater supply of rapidly fermentable carbohydrates (75).
Contrary to expectations, animals treated with both MON and PAP had higher concentrations of ruminal NH3-N. Both additives affect the population of Streptococcus bovis, a highly proteolytic gram-positive rumen bacterium (76) and therefore may indirectly affect rumen ammonia concentrations. In addition, MON may act on the partitioning of protein metabolism by decreasing the production of NH3-N in the rumen and increasing escape of dietary protein from ruminal degradation [increasing the passage rate of protein from 22 to 55%; (77) and (78), respectively]. However, despite decades of widespread use of this ionophore, the protein partitioning effect has never been fully explained (79), largely because of the observation that most isolated ammonia-producing rumen bacteria are Gram-negative (80). Chen and Russell (79, 81) were able to obtain three isolates of gram-positive bacteria (Peptostreptococcus anaerobius, Clostridium sticklandii and Clostridium aminophilum) with highly specific activities for the production of ammonia, which are also sensitive to MON in in vitro studies. However, further studies are needed to explain the increase in rumen NH3-N concentration in animals. However, it is noteworthy to mention that the protocol used in the present study, which involved a simultaneous increase in diet density and different treatments, may have influenced the results. Ammonia is the predominant base in the rumen (51); therefore the sharp decrease in the concentration of NH3-N observed on day 1 could be due to the low rumen pH, along with the decrease in DMI on that day. In addition, much of the NH3-N may have been incorporated by the bacteria, which may have had a high growth rate during this experimental period.
Monensin was effective in controlling subacute acidosis in cattle challenged with the abrupt transition to a high-concentrate diet and improved rumen fermentation by altering SCFA molar proportions. The PAP was not effective in controlling acidosis, which can be explained by the fact that this product targets the control of lactate-producing bacteria. However, the acidosis induced by the challenge was caused by the accumulation of SCFAs. Therefore, the real conditions for evaluation of this feed additive were not reached in this experiment, which opens the possibility of new studies under the conditions of lactic acidosis. It is worth mentioning that the protocol used in the present study, which included a simultaneous increase in diet density and different treatments, may have influenced the results.
The raw data supporting the conclusions of this article will be made available by the authors, without undue reservation.
The animal study was reviewed and approved by São Paulo State University Ethical Committee for Animal Research (CONCEA, 2013), São Paulo State University, Botucatu campus, Brazil.
RP and DM: conceived and designed study, collected and complied, and analyzed data. CTM, CLM, JB, MA, and PR: provided intellectual input. RP, JS, and DM: provided intellectual input and drafted and edited manuscript. All authors contributed to the article and approved the submitted version.
This project was financially supported by Fapesp, The São Paulo Research Foundation (Grant Number: 09/01968-3).
We wish to acknowledge the FAPESP (São Paulo Research Foundation) for the scholarship provided to conduct this study.
CTM is a Post Doctoral Researcher at Embrapa Beef Cattle under a scholarship.
The remaining authors declare that the research was conducted in the absence of any commercial or financial relationships that could be construed as a potential conflict of interest.
All claims expressed in this article are solely those of the authors and do not necessarily represent those of their affiliated organizations, or those of the publisher, the editors and the reviewers. Any product that may be evaluated in this article, or claim that may be made by its manufacturer, is not guaranteed or endorsed by the publisher.
1. Silvestre AM, Millen DD. The 2019 brazilian survey on nutritional practices provided by feedlot cattle consulting nutritionists. Revista Brasileira de Zootecnia. (2021) 50:e20200189. doi: 10.37496/rbz5020200189
2. McCarthy MM, Yasui T, Ryan CM, Mechor GD, Overton TR. Performance of early-lactation dairy cows as affected by dietary starch and monensin supplementation. J Dairy Sci. (2015) 98:3335–50. doi: 10.3168/jds.2014-8820
3. Aikman PC, Henning PH, Humphries DJ, Horn CH. Rumen Ph and fermentation characteristics in dairy cows supplemented with Megasphaera Elsdenii Ncimb 41125 in early lactation. J Dairy Sci. (2011) 94:2840–9. doi: 10.3168/jds.2010-3783
4. Kim H, Park T, Kwon I, Seo J. Specific inhibition of streptococcus Bovis by Endolysin Lyjh307 supplementation shifts the rumen microbiota and metabolic pathways related to carbohydrate metabolism. J Anim Sci Biotechnol. (2021) 12:93. doi: 10.1186/s40104-021-00614-x
5. Abdela N. Sub-acute ruminal acidosis (Sara) and its consequence in dairy cattle: a review of past and recent research at global prospective. Achiev Life Sci. (2016) 10:187–96. doi: 10.1016/j.als.2016.11.006
6. Krause KM, Oetzel GR. Understanding and preventing subacute ruminal acidosis in dairy herds: a review. Anim Feed Sci Technol. (2006) 126:215–36. doi: 10.1016/j.anifeedsci.2005.08.004
7. Schwartzkopf-Genswein KS, Beauchemin KA, Gibb DJ, Crews DH Jr, Hickman DD, Streeter M, et al. Effect of bunk management on feeding behavior, ruminal acidosis and performance of feedlot cattle: a review. J Anim Sci. (2003) 81:E149–E58. doi: 10.2527/2003.8114_suppl_2E149x
8. Goodrich RD, Garrett JE, Gast DR, Kirick MA, Larson DA, Meiske JC. Influence of monensin on the performance of cattle. J Anim Sci. (1984) 58:1484–98. doi: 10.2527/jas1984.5861484x
9. Nagaraja TG, Titgemeyer EC. Ruminal acidosis in beef cattle: the current microbiological and nutritional outlook. J Dairy Sci. (2007) 90 (Suppl. 1):E17–38. doi: 10.3168/jds.2006-478
10. Weimer PJ, Stevenson DM, Mertens DR, Thomas EE. Effect of monensin feeding and withdrawal on populations of individual bacterial species in the rumen of lactating dairy cows fed high-starch rations. Appl Microbiol Biotechnol. (2008) 80:135–45. doi: 10.1007/s00253-008-1528-9
11. Andrade-Montemayor H, García Gasca T, Kawas J. Ruminal fermentation modification of protein and carbohydrate by means of roasted and estimation of microbial protein synthesis. Revista Brasileira de Zootecnia. (2009) 38:277–91. doi: 10.1590/S1516-35982009001300028
12. Yang CM, Russell JB. The effect of monensin supplementation on ruminal ammonia accumulation in vivo and the numbers of amino acid-fermenting bacteria. J Anim Sci. (1993) 71:3470–6. doi: 10.2527/1993.71123470x
13. Eschenlauer SC, McKain N, Walker ND, McEwan NR, Newbold CJ, Wallace RJ. Ammonia production by ruminal microorganisms and enumeration, isolation, and characterization of bacteria capable of growth on peptides and amino acids from the sheep rumen. Appl Environ Microbiol. (2002) 68:4925–31. doi: 10.1128/AEM.68.10.4925-4931.2002
14. rd CoS. [Decision No. 1786/Ec of the European Parliament and of the Council of September 23rd, concerning the adoption of a program of community action in the field of public health (2003-2008)]. An Sist Sanit Navar. (2003) 26:109-19. doi: 10.23938/ASSN.0466
15. Russell JB, Houlihan AJ. Ionophore resistance of ruminal bacteria and its potential impact on human health. FEMS Microbiol Rev. (2003) 27:65–74. doi: 10.1016/S0168-6445(03)00019-6
16. Blanch M, Calsamiglia S, DiLorenzo N, DiCostanzo A, Muetzel S, Wallace RJ. Physiological changes in rumen fermentation during acidosis induction and its control using a multivalent polyclonal antibody preparation in heifers. J Anim Sci. (2009) 87:1722–30. doi: 10.2527/jas.2008-1184
17. DiLorenzo N, Diez-Gonzalez F, DiCostanzo A. Effects of feeding polyclonal antibody preparations on ruminal bacterial populations and ruminal ph of steers fed high-grain diets. J Anim Sci. (2006) 84:2178–85. doi: 10.2527/jas.2005-489
18. Cassiano ECO, Junior FP, Barros TA, Marino CT, Pacheco RDL, Ferreira FA, et al. Evaluation of liquid and powdered forms of polyclonal antibody preparation against streptococcus bovis and fusobacterium necrophorum in cattle adapted or not adapted to highly fermentable carbohydrate diets. Anim Biosci. (2021) 34:74–84. doi: 10.5713/ajas.19.0761
19. Millen DD, Pacheco RD, DiLorenzo N, Martins CL, Marino CT, Bastos JP, et al. Effects of feeding a spray-dried multivalent polyclonal antibody preparation on feedlot performance, feeding behavior, carcass characteristics, rumenitis, and blood gas profile of brangus and nellore yearling bulls. J Anim Sci. (2015) 93:4387–400. doi: 10.2527/jas.2015-9227
20. Barros TA, Cassiano ECO, Marino CT, Pacheco RDL, Ferreira FA, Junior FP, et al. Polyclonal antibodies as a feed additive for cattle adapted or not adapted to highly fermentable carbohydrate diets. J Appl Anim Res. (2019) 47:565–72. doi: 10.1080/09712119.2019.1691561
21. Lammers BP, Buckmaster DR, Heinrichs AJ. A simple method for the analysis of particle sizes of forage and total mixed rations. J Dairy Sci. (1996) 79:922–8. doi: 10.3168/jds.S0022-0302(96)76442-1
22. Chemists AoOA. Official Methods of Analysis of Aoac. 18 ed. Maryland, MD: Association of Official Analytical Chemists (2005).
23. Van Soest PJ. Nutritional Ecology of the Ruminant. 2nd ed. Ithaca, NY: Cornell Univ Press (1994).
24. Pereira JRA, Rossi Jr P. Practical Manual of Feedstuffs Nutritional Evaluation. 1st ed. Piracicaba, SP: FEALQ (1995).
25. Hendrix DL. Rapid extraction and analysis of nonstructural carbohydrates in plant tissues. Crop Sci. (1993) 33:1306–11. doi: 10.2135/cropsci1993.0011183X003300060037x
26. NRC. Nutrient Requirements of Dairy Cattle. 7th rev. ed. Washington, DC: Natl. Acad. Press (2001).
27. Fox DG, Sniffen CJ, O'Connor JD, Russell JB, Van Soest PJ, A. Net carbohydrate and protein system for evaluating cattle diets: III. Cattle requirements and diet adequacy. J Anim Sci. (1992) 70:3578–96. doi: 10.2527/1992.70113578x
28. Bevans DW, Beauchemin KA, Schwartzkopf-Genswein KS, McKinnon JJ, McAllister TA. Effect of rapid or gradual grain adaptation on subacute acidosis and feed intake by feedlot cattle. J Anim Sci. (2005) 83:1116–32. doi: 10.2527/2005.8351116x
29. Nordlund KG EF. Rumenocentesis: a technique for collecting rumen fluid for the diagnosis of subacute rumen acidosis in dairy herds. Bovine Pract. (1994) 28:109–12.
30. Owens FN, Secrist DS, Hill WJ, Gill DR. Acidosis in cattle: a review. J Anim Sci. (1998) 76:275–86. doi: 10.2527/1998.761275x
31. Pryce JD. A Modification of the barker-summerson method for the determination of lactic acid. Analyst. (1969) 94:1151–2. doi: 10.1039/an9699401151
32. Erwin ES, Marco GJ, Emery EM. Volatile fatty acids analyses of blood and rumen fluid by gas chromatography. J Dairy Sci. (1961) 44:1768–71. doi: 10.3168/jds.S0022-0302(61)89956-6
33. Kulasek GA. A micromethod for determination of urea in plasma, whole blood cells using urease and phenol reagent. Pol Arch Weter. (1972) 15:801–10.
34. Foldager J. Protein Requirement and Non Protein Nitrogen for High Producing Cow in Early Lactation. East Lasing, MI: PhD Diss. Michigan State University (1977).
35. Valadares RFD, Broderick GA, Filho SCV, Clayton MK. Effect of replacing alfalfa silage with high moisture corn on ruminal protein synthesis estimated from excretion of total purine derivatives. J Dairy Sci. (1999) 82:2686–96. doi: 10.3168/jds.S0022-0302(99)75525-6
36. Rennó LN. Consumo, Digestibilidade Total E Parcial, Produção Microbiana, Parâmetros Ruminais E Excreções De Uréia E Creatinina Em Novilhos Alimentados Com Dietas Contendo Quatro Níveis De Uréia Ou Dois De Proteína. Viçosa: Universidade Federal de Viçosa (2003).
37. Verbic J, Chen XB, MacLeod NA, Ørskov ER. Excretion of purine derivatives by ruminants. Effect of microbial nucleic acid infusion on purine derivative excretion by steers. J Agri Sci. (2009) 114:243–8. doi: 10.1017/S0021859600072610
38. Oliveira AS, Valadares RFD., Valadares Filho SdC, Cecon PR, Rennó LN, Queiroz ACd, et al. Produção De Proteína Microbiana E Estimativas Das Excreções De Derivados De Purinas E De Uréia Em Vacas Lactantes Alimentadas Com Rações Isoprotéicas Contendo Diferentes Níveis De Compostos Nitrogenados Não-Protéicos. Revista Brasileira de Zootecnia. (2001) 30:1621–9. doi: 10.1590/S1516-35982001000600032
39. Chizzoti ML, Valadares Filho SC, Valadares RFD, Marcondes MI, Fonseca MA, Pina DS, et al. Excreção De Creatinina Em Novilhos E Novilhas Anais da 41o Reunião Anual da Sociedade Brasileira de Zootecnia. Campo Grande (2004).
40. Fujihara T, Ørskov ER, Reeds PJ, Kyle DJ. The effect of protein infusion on urinary excretion of purine derivatives in ruminants nourished by intragastric nutrition. J Agric Sci. (2009) 109:7–12. doi: 10.1017/S0021859600080916
41. Chen XB, Gomes MJ. Estimation of Microbial Protein Supply to Sheep and Cattle Based on Urinary Excretion of Purine Derivatives – an Overview of Technical Details: Rowett Research Institute. Bucksburnd: International Feed Resources Unit (1992).
42. Orellana Boero P, Balcells J, Martin-Orúe SM, Liang JB, Guada JA. Excretion of purine derivatives in cows: endogenous contribution and recovery of exogenous purine bases. Livestock Prod Sci. (2001) 68:243–50. doi: 10.1016/S0301-6226(00)00231-1
43. Gonzalez-Ronquillo M, Balcells J, Guada JA, Vicente F. Purine derivative excretion in dairy cows: endogenous excretion and the effect of exogenous nucleic acid supply. J Dairy Sci. (2003) 86:1282–91. doi: 10.3168/jds.S0022-0302(03)73712-6
44. Millen DD, Pacheco RD, Arrigoni MD, Galyean ML, Vasconcelos JT, A. Snapshot of management practices and nutritional recommendations used by feedlot nutritionists in Brazil. J Anim Sci. (2009) 87:3427–39. doi: 10.2527/jas.2009-1880
45. Millen DD, Pacheco RDL, Arrigoni MB, Parrili M, Matsuhara SA, Fossa MV, et al. Feedlot performance and rumen parakeratosis incidence in bos indicus type bullocks fed high grain diets and monensin or polyclonal antibodies preparations against rumen bacteria. In:2007 Joint Annual Meeting, , editor. Asas – American Society of Animal Science, Vol. 85. San Antonio, TX: Journal of Animal Science (2007). p. 552.
46. Rodrigues M, Deschk M, Santos GGF, Perri SHV, Merenda VR, Hussni CA, et al. Avaliação Das Características Do Líquido Ruminal, Hemogasometria, Atividade Pedométrica E Diagnóstico De Laminite Subclínica Em Vacas Leiteiras. Pesquisa Veterinária Brasileira. (2013) 33:99–106. doi: 10.1590/S0100-736X2013001300016
47. Duffield TF, Merrill JK, Bagg RN. Meta-analysis of the effects of monensin in beef cattle on feed efficiency, body weight gain, and dry matter intake. J Anim Sci. (2012) 90:4583–92. doi: 10.2527/jas.2011-5018
48. Rigueiro ALN, Pereira MCS, Squizatti MM, Ferreira MM, Dondé SC, Luiz FP, et al. Different combinations of sodium monensin and virginiamycin during feedlot finishing of nellore cattle. Anim Prod Sci. (2020) 60:1061–72. doi: 10.1071/AN18657
49. Erickson GE, Milton CT, Fanning KC, Cooper RJ, Swingle RS, Parrott JC, et al. Interaction between bunk management and monensin concentration on finishing performance, feeding behavior, and ruminal metabolism during an acidosis challenge with feedlot cattle. J Anim Sci. (2003) 81:2869–79. doi: 10.2527/2003.81112869x
50. Mariani TM, Millen DD, Pacheco RDL, Lauritano RD, Arrigoni MDB, Martins CL, et al. Feeding behavior of nellore and brangus cattle fed monensin or polyclonal antibody preparation against lactate-producing rumen bacteria. In: ADSA-CSAS-ASAS, editor. 2009 Joint Adsa-Csas-Asas Annual Meeting, Vol. 87. Montreal, QC (2009). p. 283.
51. Owens FDG. AL Ruminal Fermentation. The Ruminant Animal: Digestive Physiology and Nutritio. Wavelend: Long Grove (1988). p. 145–71.
52. Bauer ML, Herold DW, Britton RA, Stock RA, Klopfenstein TJ, Yates DA. Efficacy of laidlomycin propionate to reduce ruminal acidosis in cattle. J Anim Sci. (1995) 73:3445–54. doi: 10.2527/1995.73113445x
53. Stock R. Acidosis in cattle: an overview. In: 33rd Annual Convention of the American Association of Bovine Practitioners Proceedings. Rapid City, SD (2000). p. 30–7.
54. Forbes JM, Provenza F. D. Integration of learning and metabolic signals into a theory of dietary choice and food intake. In:Cp B, , editor. Ruminant Physiology Digestion, Metabolism, Growth and Reproduction. New York, NY: CABI Publishing (2000). p. 3–20.
55. Jonsson NN, Kleen JL, Wallace RJ, Andonovic I, Michie C, Farish M, et al. Evaluation of reticuloruminal Ph measurements from individual cattle: sampling strategies for the assessment of herd status. Vet J. (2019) 243:26–32. doi: 10.1016/j.tvjl.2018.11.006
56. Denwood MJ, Kleen JL, Jensen DB, Jonsson NN. Describing temporal variation in reticuloruminal Ph using continuous monitoring data. J Dairy Sci. (2018) 101:233–45. doi: 10.3168/jds.2017-12828
57. Plaizier JC, Mulligan FJ, Neville EW, Guan LL, Steele MA, Penner GB. Invited review: effect of subacute ruminal acidosis on gut health of dairy cows. J Dairy Sci. (2022) 105:7141–60. doi: 10.3168/jds.2022-21960
58. Plaizier JC, Danesh Mesgaran M, Derakhshani H, Golder H, Khafipour E, Kleen JL, et al. Review: enhancing gastrointestinal health in dairy cows. Animal. (2018) 12:s399–418. doi: 10.1017/S1751731118001921
59. Mu Y, Qi W, Zhang T, Zhang J, Mao S. Multi-omics analysis revealed coordinated responses of rumen microbiome and epithelium to high-grain-induced subacute rumen acidosis in lactating dairy cows. mSystems. (2022) 7:e0149021. doi: 10.1128/msystems.01490-21
60. Mu YY Qi WP, Zhang T, Zhang JY, Mao SY. Gene function adjustment for carbohydrate metabolism and enrichment of rumen microbiota with antibiotic resistance genes during subacute rumen acidosis induced by a high-grain diet in lactating dairy cows. J Dairy Sci. (2021) 104:2087–105. doi: 10.3168/jds.2020-19118
61. Oetzel GR, editor. Clinical Aspects of Ruminal Acidosis in Dairy Cattle. In: Proceedings of the 33rd Annual Convention of the American Association of Bovine Practitioner. Rapid City, SD (2000).
62. Garry FB. Indigestion in ruminants. In:Smith BP, , editor. Large Animal Internal Medicine St Louis, MO: Mosby (2002). p. 722–47.
63. Goad DW, Goad CL, Nagaraja TG. Ruminal microbial and fermentative changes associated with experimentally induced subacute acidosis in steers. J Anim Sci. (1998) 76:234–41. doi: 10.2527/1998.761234x
64. Meyer NF, Bryant TC. Diagnosis and management of rumen acidosis and bloat in feedlots. Vet Clin North Am Food Anim Pract. (2017) 33:481–98. doi: 10.1016/j.cvfa.2017.06.005
65. Khafipour E, Li S, Plaizier JC, Krause DO. Rumen microbiome composition determined using two nutritional models of subacute ruminal acidosis. Appl Environ Microbiol. (2009) 75:7115–24. doi: 10.1128/AEM.00739-09
66. Barducci RS, Arrigoni MDB, Pacheco RDL, Millen DD, Martins CL, Baldin PFS, et al. Feedlot performance of brangus cattle fed monensin or polyclonal antibody preparation against lactate- producing rumen bacteria. In: ADSA-CSAS-ASAS, editor. 2009 Joint Adsa-Csas-Asas Annual Meeting, Vol. 87. Montreal, QC (2009). p. 283–4.
67. Bergen WG, Bates DB. Ionophores: their effect on production efficiency and mode of action. J Anim Sci. (1984) 58:1465–83. doi: 10.2527/jas1984.5861465x
68. Bell NL, Anderson RC, Callaway TR, Franco MO, Sawyer JE, Wickersham TA. Effect of monensin inclusion on intake, digestion, and ruminal fermentation parameters by bos taurus indicus and bos taurus taurus steers consuming bermudagrass hay. J Anim Sci. (2017) 95:2736–46. doi: 10.2527/jas.2016.1011
69. Ruiz R, Albrecht GL, Tedeschi LO, Jarvis G, Russell JB, Fox DG. Effect of monensin on the performance and nitrogen utilization of lactating dairy cows consuming fresh forage. J Dairy Sci. (2001) 84:1717–27. doi: 10.3168/jds.S0022-0302(01)74607-3
70. Kleen JL, Hooijer GA, Rehage J, Noordhuizen JP. Subacute ruminal acidosis (Sara): a review. J Vet Med A Physiol Pathol Clin Med. (2003) 50:406–14. doi: 10.1046/j.1439-0442.2003.00569.x
71. Urrutia NL, Harvatine KJ. Acetate dose-dependently stimulates milk fat synthesis in lactating dairy cows. J Nutr. (2017) 147:763–9. doi: 10.3945/jn.116.245001
72. Allen MS, Voelker JA, Oba M. Physically effective fiber and regulation of ruminal Ph: more than just chewing. In:NP Joshi TH, , editor. Production Diseases in Farm Animals. Wageningen: Wageningen Academic Publishers (2006). p. 270–8.
73. Kolver ES, de Veth MJ. Prediction of ruminal ph from pasture-based diets. J Dairy Sci. (2002) 85:1255–66. doi: 10.3168/jds.S0022-0302(02)74190-8
74. Fereli F, Branco AF, Jobim CC, Coneglian SM, Granzotto F, Barreto JC. Monensina Sódica E Saccharomyces Cerevisiae Em Dietas Para Bovinos: Fermentação Ruminal, Digestibilidade Dos Nutrientes E Eficiência De Síntese Microbiana. Revista Brasileira de Zootecnia. (2010) 39:183–90. doi: 10.1590/S1516-35982010000100024
75. Pina DS, Valadares Filho SC, Tedeschi LO, Barbosa AM, Valadares RF. Influence of different levels of concentrate and ruminally undegraded protein on digestive variables in beef heifers. J Anim Sci. (2009) 87:1058–67. doi: 10.2527/jas.2008-1069
76. Russell JB, Bottje WG, Cotta MA. Degradation of protein by mixed cultures of rumen bacteria: identification of streptococcus bovis as an actively proteolytic rumen bacterium. J Anim Sci. (1981) 53:242–52. doi: 10.2527/jas1981.531242x
77. Owens FN, Lusby KS, Mizwicki K, Forero O. Slow ammonia release from urea: rumen and metabolism studies. J Anim Sci. (1980) 50:527–31. doi: 10.2527/jas1980.503527x
78. Poos MI, Hanson TL, Klopfenstein TJ. Monensin effects on diet digestibility, ruminal protein bypass and microbial protein synthesis. J Anim Sci. (1979) 48:1516–24. doi: 10.2527/jas1979.4861516x
79. Chen G, Russell JB. More monensin-sensitive, ammonia-producing bacteria from the rumen. Appl Environ Microbiol. (1989) 55:1052–7. doi: 10.1128/aem.55.5.1052-1057.1989
80. Bladen HA, Bryant MP, Doetsch RN. A study of bacterial species from the rumen which produce ammonia from protein hydrolyzate. Appl Microbiol. (1961) 9:175–80. doi: 10.1128/am.9.2.175-180.1961
Keywords: additives, rumen, Streptococcus bovis, Fusobacterium necrophorum, Lactobacillus ssp
Citation: Pacheco RDL, Souza JM, Marino CT, Bastos JPST, Martins CL, Rodrigues PHM, Arrigoni MDB and Millen DD (2023) Ruminal fermentation pattern of acidosis-induced cows fed either monensin or polyclonal antibodies preparation against several ruminal bacteria. Front. Vet. Sci. 10:1090107. doi: 10.3389/fvets.2023.1090107
Received: 04 November 2022; Accepted: 28 February 2023;
Published: 20 March 2023.
Edited by:
Abdelfattah Zeidan Mohamed Salem, Universidad Autónoma del Estado de México, MexicoReviewed by:
Ian John Lean, Scibus, AustraliaCopyright © 2023 Pacheco, Souza, Marino, Bastos, Martins, Rodrigues, Arrigoni and Millen. This is an open-access article distributed under the terms of the Creative Commons Attribution License (CC BY). The use, distribution or reproduction in other forums is permitted, provided the original author(s) and the copyright owner(s) are credited and that the original publication in this journal is cited, in accordance with accepted academic practice. No use, distribution or reproduction is permitted which does not comply with these terms.
*Correspondence: Danilo D. Millen, ZGFuaWxvLm1pbGxlbkB1bmVzcC5icg==
Disclaimer: All claims expressed in this article are solely those of the authors and do not necessarily represent those of their affiliated organizations, or those of the publisher, the editors and the reviewers. Any product that may be evaluated in this article or claim that may be made by its manufacturer is not guaranteed or endorsed by the publisher.
Research integrity at Frontiers
Learn more about the work of our research integrity team to safeguard the quality of each article we publish.