- 1Department of Biological Sciences, University of Notre Dame, Notre Dame, IN, United States
- 2Wildlife Ecology and Health Group (WE&H), Departament de Ciència Animal, Escola Tècnica Superior d'Enginyeria Agrària (ETSEA), Universitat de Lleida (UdL), Lleida, Spain
- 3Interdisciplinary Laboratory of Clinical Analysis (Interlab-UMU), Regional Campus of International Excellence “Campus Mare Nostrum”, University of Murcia, Murcia, Spain
- 4Innovación en Gestión y Conservación de Ungulados S.L., Cáceres, Spain
- 5Departamento de Medicina Animal, Faculta de Veterinaria, Universidad de Extremadura, Cáceres, Spain
- 6Wildlife Health and Ecology Group (WE&H), Servei d' Ecopatologia de Fauna Salvatge (SEFaS), Departament de Medicina i Cirurgia Animals, Facultat de Veterinària, Universitat Autònoma de Barcelona (UAB), Bellaterra, Barcelona, Spain
- 7Servei d'Hematologia Clínica Veterinaria (SHCV) – Veterinary Clinical Hematology Service, Departament de Medicina i Cirurgia Animals, Facultat de Veterinària, Universitat Autònoma de Barcelona (UAB), Bellaterra, Barcelona, Spain
In recent decades, there has been a fast-growing interest in using biomarkers of oxidative stress (BOS) in conservation programs of many vertebrate species. Biomarkers of oxidative stress can be measured in different biological samples (e.g., body fluids and tissues). However, since comparisons of the same battery of BOS among tissues of the same individual are scarce in the literature, the chosen target tissues regularly rely on arbitrary decisions. Our research aimed to determine if the oxidative status of free-ranging wild boar (Sus scrofa) naturally infected with Mycobacterium spp (etiological agent of tuberculosis, TB), varies depending on the sample where it was quantified. We compared antioxidant p-nitrophenyl esterase activity (EA), glutathione peroxidase (GPX) concentrations, and total oxidative status (TOS) in serum, lung, spleen, kidney, and muscle of 63 wild boar hunter-harvested in central Spain. Biomarkers of oxidative stress in serum had higher concentrations than in other tissues. The poor agreement between serum and other tissues highlights the importance of running complete BOS assessments in the same fluid or tissue. Further, low concentrations of BOS in tissues of TB-affected individuals were observed, and significant differences between healthy and sick boar were only detected in the serum of individuals developing mild TB and in the muscle of individuals with mild or severe disease status. However, all organs from wild boars affected with mild TB were not in oxidative imbalance compared to healthy control animals, suggesting that wild boars may cope well with TB. Our data indicate that serum and other tissues can be used as BOS in field conservation programs to monitor wildlife population health. Still, context-specific validations are needed to determine the most appropriate samples to use.
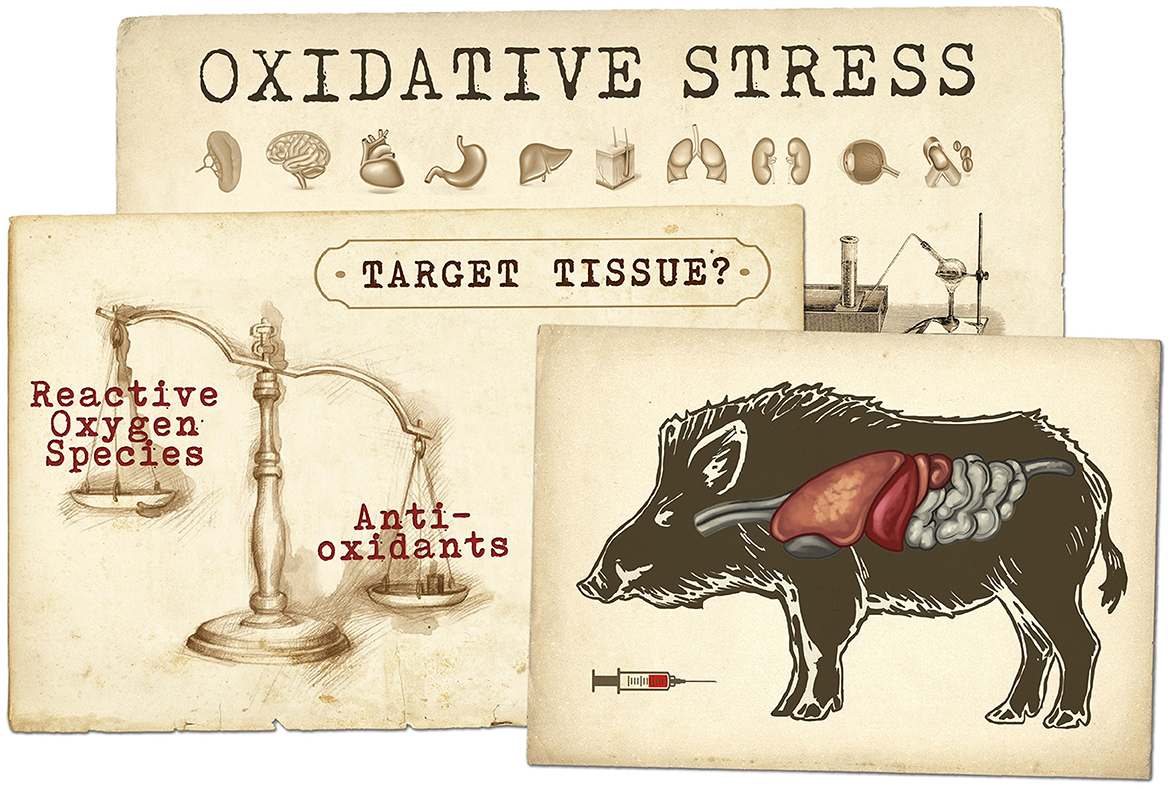
Graphical Abstract. This drawing represents the concept of oxidative stress resulting from an imbalance arising as a consequence of the rate of production of reactive oxygen species exceeding the capacity of the antioxidant defence and repair mechanisms. Oxidative stress then leads to subsequent oxidative damage to biomolecules, tissue dysfunction and disease. Our work is intended to explore how the choice of a biological sample (e.g., muscle, serum, organs) influences oxidative status assessment in wild boar naturally infected with tuberculosis.
1. Introduction
The loss of biodiversity due to anthropogenic disturbances like habitat loss, fragmentation, emerging infectious diseases, and climate change is one of the most critical environmental challenges affecting our planet (1). Assessing the physiological response of animals to these environmental disruptions is an essential component of management, monitoring, and conservation programs in wildlife populations [e.g., (2)]. Biomarkers like stress hormones (glucocorticoids) (3) and immune response indicators (4) have regularly been used for this objective, achieving results with different degrees of robustness [see this review Kalliokoski et al. (5)]. In recent years, there has been a growing interest in incorporating oxidative stress biomarkers (BOS) to assess the physiological response of wildlife to environmental disturbances due to their stability and easy quantification (6, 7). Oxidative stress (OS) is an imbalance between reactive oxygen species (ROS) and antioxidants in living systems. The ROS are unstable molecules (i.e., free radicals) with potential to damage to cell structures, including lipids and membranes, proteins, and DNA (8). There is a rising body of research linking OS to reproduction (9), growth (10), survival (11), immune response (12), and particularly health/disease status (13–15).
Oxidative damage, and in consequence, OS, is generally higher in cells, tissues, and organs suffering from inflammation or local immune response [e.g., erythrocytes, (16); liver (17); intestines (18); kidneys (19) and lungs (20)]. This fact hampers sampling opportunities for researchers working with live animals who prefer to collect sperm (7, 21) or blood serum (22) to avoid animal killing. However, body fluids often fail to detect oxidative damage even in sick individuals (6, 23), making any attempt to monitor OS by non-invasive methods more complicated. Ideally, the choice of specific BOS and the biological samples to properly evaluate OS should be determined on a system-specific basis, considering the particular aims of the study, the experimental design (e.g., access to samples), and the clinical relevance in the selected subjects (24). Unfortunately, very little information is available to inform these decisions, specifically about the agreement between BOS measurements obtained from different tissues (25, 26). Consequently, there is a growing need for experimental and observational studies to evaluate the suitability of specific BOS and biological samples to assess vertebrates' oxidant and antioxidant status. These studies are of significant importance when investigating animals facing conservation issues due to reduced sample availability and in the case of animals infected with zoonotic pathogens, as biosafety measures need to be put in place before sampling occurs.
In this study, we investigated how BOS collected from various body tissues relates to OS in animals with different health statuses. To do this, we compared the concentrations of BOS, antioxidants, and the specific oxidative imbalance in serum, lung, spleen, kidney, and muscle of wild boar (Sus scrofa) hunter-harvested from populations with endemic tuberculosis. The Graphical Abstract at the top of this page summarizes the aims of our work. Tuberculosis (TB) is an infective zoonosis caused by Mycobacterium spp. This bacteria produces chronic inflammation and systemic OS (27, 28), resulting in lung fibrosis and dysfunction (27–29). Wild boar is the main reservoir of TB in the Mediterranean basin (30), showing differential degrees of resistance to disease progression due to environmental factors (31), genetic characteristics (32), and concomitant infections (33). Boars can develop severe TB with gross lesions in lymph nodes (i.e., mild TB) and the lungs, liver, and spleen (33). A more severe TB implies a more significant bacillary load (34) and lower survival probability (31). First, we compared BOS from different tissues and degrees of TB infection (i.e., negative, mild TB, severe TB). Then, we explored whether BOS measurements are interchangeable among different biological samples from the same individual. Finally, to define the most reliable target tissue to assess OS caused by TB in wild boar, we compared BOS concentrations among different organs and the serum of diseased and healthy animals. Recent work has linked TB progression with OS in the serum of wild boar (35), but, as in most vertebrates, there is no previous information about the most reliable biological sample to assess OS in TB-infected animals. Likewise, no information exists regarding the oxidant-antioxidant imbalance in animals showing severe TB.
2. Materials and methods
2.1. Wild boar and study area
A total of 63 wild boar (50 females and 13 males) aged from 5 to 60 months were hunter-harvested in a private 2,000 ha hunting state in Toledo (central Spain; 39°55′ 15.55″N, 5 °10′ 32.33″ E). For each hunted animal, the objective was to cause the least animal welfare harm and the least impact in stress possible. This was done by attempting shoots that cause a short flight distance (i.e., the actual distance traveled by an animal from where it was initially struck by the first bullet to where it fell recumbent, immobile, and regarded as unconscious) and dead. The vegetation in this area is a Mediterranean forest dominated by scattered holm oaks (Quercus ilex) and Mediterranean shrubs, primarily Cistus ladanifer, Erica spp., and Genista anglica. Wild boar density in the study area was about 40 boars per 100 ha (unpublished data). Supplemental feeding (Jabalí Familia, Mercoguadiana, S.A., Spain) is regularly provided during summer when natural resources are scarce. Boars were legally hunted in their habitat by authorized hunters within the framework of an annual hunting plan approved by the regional authority in charge of livestock and wildlife management. No approvals were needed from an animal ethic committee since the wild boars were not sacrificed for research purposes.
2.2. Tissue samples
After animal culling, sex was determined through a visual inspection of the genitalia area, while age was assessed by dental biometry (36). The positivity of wild boars to M. bovis was determined by microbiological cultures from intact submandibular or retropharyngeal lymph nodes and a piece of a caudal lung lobe following the methods described in Gassó et al. (35). Animals were classified into three groups: (i) TB-free boars (negative culture and no signs of TB lesions), (ii) positive culture and localized gross lesions (mild TB), and (iii) positive culture and disseminated gross lesions (severe TB) (33, 37).
Within the first 5 h after culling, blood, lung, spleen, kidney, and muscle samples were collected, placed in cold boxes at 4°C, transported to the laboratory, and stored at −80°C. Blood was collected from the cavernous sinus (38) in plain tubes, and the tubes were centrifugated at 3,500 rpm (1068 g) for 10 min to obtain serum. Before analysis, lung, spleen, kidney, and muscle samples were processed according to Renerre et al. (39). In brief, tissue samples were homogenized in a 50 mM phosphate buffer, pH 7.0, and centrifuged at 1,000 g for 15 min at 4°C. The supernatant was transferred to Eppendorf tubes and used for analyses. Highly hemolytic samples were identified by visual inspection and eliminated from the study due to their potential interference with the analytical methods (40).
2.3. Oxidative stress biomarkers analysis
We used the antioxidant p-nitrophenyl esterase activity (EA), glutathione peroxidase (GPX) concentrations, and total oxidative status (TOS) analysis to describe the overall picture of vertebrates' oxidant (TOS) and antioxidant (EA, GPX) balance. The EA (IU/mL) was analyzed by measuring the hydrolysis of p-nitrophenyl acetate to p-nitrophenol as described elsewhere (41). The reaction was monitored at 405 nm. The non-enzymatic hydrolysis of phenylacetate, based on the hydrolysis rate in the absence of a sample, was subtracted from the total hydrolysis rate. The molar extinction coefficient used to calculate the rate of hydrolysis was 14,000 M−1 cm−1 (41). When EA is measured in serum using this method, it is named paraoxonase 1 (PON1, also in IU/ml) because, in serum, this enzyme has shown activity when p-nitrophenyl-acetate is used as substrate (42). In other tissues, it is not well characterized yet; thus, it is generally called EA. This enzyme has been considered in our study because it protects against bacterial infections and destroys oxidized lipids [wild boar stores large amounts of fat in tissues and organs (37)].
The GPX (IU/L) was determined with a commercial kit (Randox Laboratories, UK). The method of GPX activity determination was based on the reaction by which GPX catalyzes glutathione oxidation by cumene hydroperoxide. In the presence of glutathione reductase and NADPH, the oxidized glutathione was immediately converted to the reduced form with concomitant oxidation of NADPH to NADP+, and the corresponding decrease in absorbance at 340 nm was measured. Previous work showed the changes in GPX due to M. bovis infection in experimentally infected boars (35).
The TOS (μmol/L) was measured as previously described by Erel (43), with some modifications (44). The method is based on the reaction that the ferric ion makes a colored complex with xylenol orange in an acidic medium. The color intensity, measured at 560 nm using 800 nm as the reference, is related to the total amount of oxidant molecules present in the sample. The assay was calibrated with hydrogen peroxide. The EA, GPX, and TOS analyses were performed in an automatic analyzer (AU 600 automated biochemical analyzer, Olympus, Minneapolis, Minn) to minimize pipetting errors and execution time (44). The intra- and inter-variation of coefficients among all the methods were below 15%, and linearity under the dilution coefficient of variation was close to 1 in all cases.
In the present study, we decided not to correct tissue extracts for the total protein concentration of the sample. Such correction could result in situation-related bias (45) and additional expenses not necessarily contributing to accurate result interpretation. Additionally, we performed a preliminary test with corrected and raw values of the BOS measured in lung tissues. We got identical results to those shown throughout this work (the correlation between corrected and raw pairs was over 0.8 in all cases, results not shown).
2.4. Statistical analysis
Descriptive statistics (mean, standard deviation, minimum, and maximum) and Kruskal–Wallis tests were used to compare EA/PON1, GPX, and TOS mean rank concentrations among biological samples. The non-parametric statistic was used due to the skewed distribution shown by our biomarkers. Later, a Bland–Altman (BA) analysis was used to explore the agreement between enzyme concentrations in the lung, spleen, kidney, muscle, and serum samples. This graphical method is the most popular for comparing two measurement techniques (46) using the representation of the mean differences (Y-axis) and magnitude of such measurements (X-axis). The limits of agreement (LoA) at 95% defined as the mean differences ± 1.96 x SD are also estimated and represented. Variables were log-transformed when differences were proportional to means values (47). However, the BA plots do not distinguish between fixed and proportional bias (48). To control this, we explored plots of differences between biomarker values, expressed as percentages of the values on the Y-axis [e.g., (GPX in serum– GPX in Lungs)/mean%], vs. the mean of the two measurements. Finally, a t-test based on 1000 bootstrapped replications was used to compare the mean values of our BOS indicators between health and TB-affected boars (mild and severe TB).
Bland-Altman analysis was performed in the “blandr” package version 0.4.3 (49), whereas bootstrapping in the “boot” package version 1.3–20 (50) of the R software 4.2.2 version (51).
3. Results
3.1. Comparison of BOS values in different tissues
Among all the sample types, the highest concentration of GPX, PON1, and TOS was observed in boar serum. This difference was significantly higher in all the cases except when comparing TOS in serum and lungs (p > 0.05, permutation test). The patterns of biomarkers concentration among different organs and serum were specific for each biomarker (Figure 1).
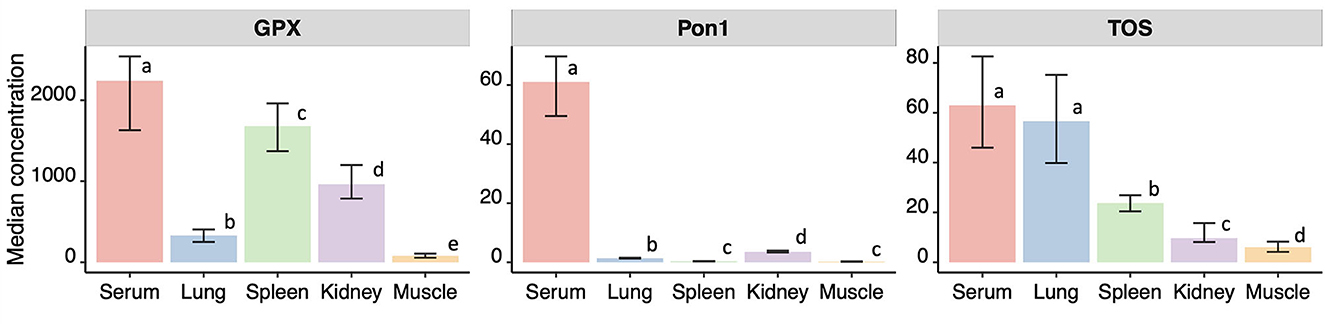
Figure 1. Median and confidence intervals of the median (in brackets) concentrations of p-nitrophenyl esterase activity (EA, in IU/ml), paraoxonase 1 (PON1, in IU/ml) in serum, glutathione peroxidase (GPX, in IU/L), and TOS (total oxidative status, in μmol/L) measured in serum, lung, spleen, kidney and muscle from 63 wild boars hunter-harvested in Toledo, Central Spain. Different letters indicate significant differences in pairwise comparisons at alpha = 0.05.
3.2. Agreement between BOS measurements
There was a poor agreement among BOS measurements in boar serum and organs (Table 1). For example, the variation in the concentration of PON1 in serum differed between 118% in the lower range and 367% in the upper range compared to lung, spleen, kidney, and muscle. Similarly, GPX concentration in serum differed between 80% in the lower range and 1182% in the upper range compared to other tissues. In line with the previous results, the upper and lower limits of agreement for TOS concentrations were above 100%. The large confidence intervals in all three tissues hamper any attempt at an agreement with serum measurements.
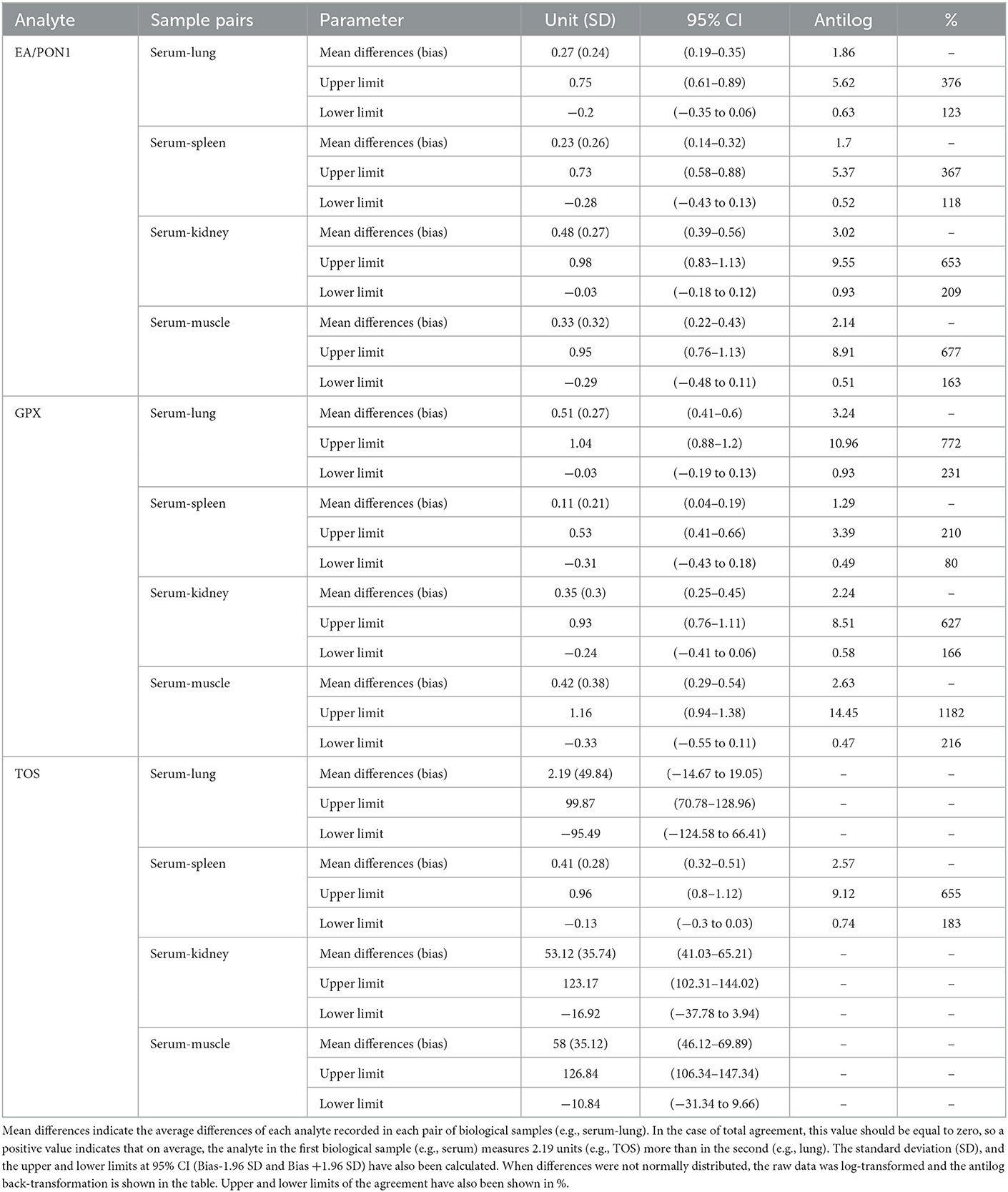
Table 1. Descriptive statistics for the Bland Altman analysis of selected oxidative stress biomarkers: p-nitrophenyl esterase activity (EA)/paraxonase 1 (PON1), glutathione peroxidase (GPX), and total oxidative status (TOS) simultaneously measured in serum, lung, spleen, kidney, and muscle from 63 wild boar hunter-harvested in Toledo, Central Spain.
3.3. Effect of TB on BOS in different tissues
With very few exceptions (i.e., PON1 in serum or GPX in spleen), the BOS concentrations were low in tissues from TB-infected individuals. Significant differences between healthy and diseased boars were only detected in the serum and muscle of individuals developing mild and severe TB disease status. In particular, p-nitrophenyl esterase activity (EA) in the muscle of healthy boar was between 1.8 times to 2.7 times higher than in individuals with mild (t = 2.41, p-value = 0.02) and severe (t = 3.7, p-value = 0.001) TB. Regarding GPX, statistically significant differences between healthy and TB-affected individuals were only detected in the muscle of severely affected boar (t = 3.41, p-value = 0.02). Finally, statistically significant differences in TOS concentrations were only observed in serum, particularly between healthy and mild TB-affected individuals (t = 2.92, p-value = 0.01). Mean and standard deviations of EA (PON1 in serum), GPX, and TOS concentrations in serum, lung, spleen, kidney, and muscle from healthy and sick boars are summarized in Table 2.
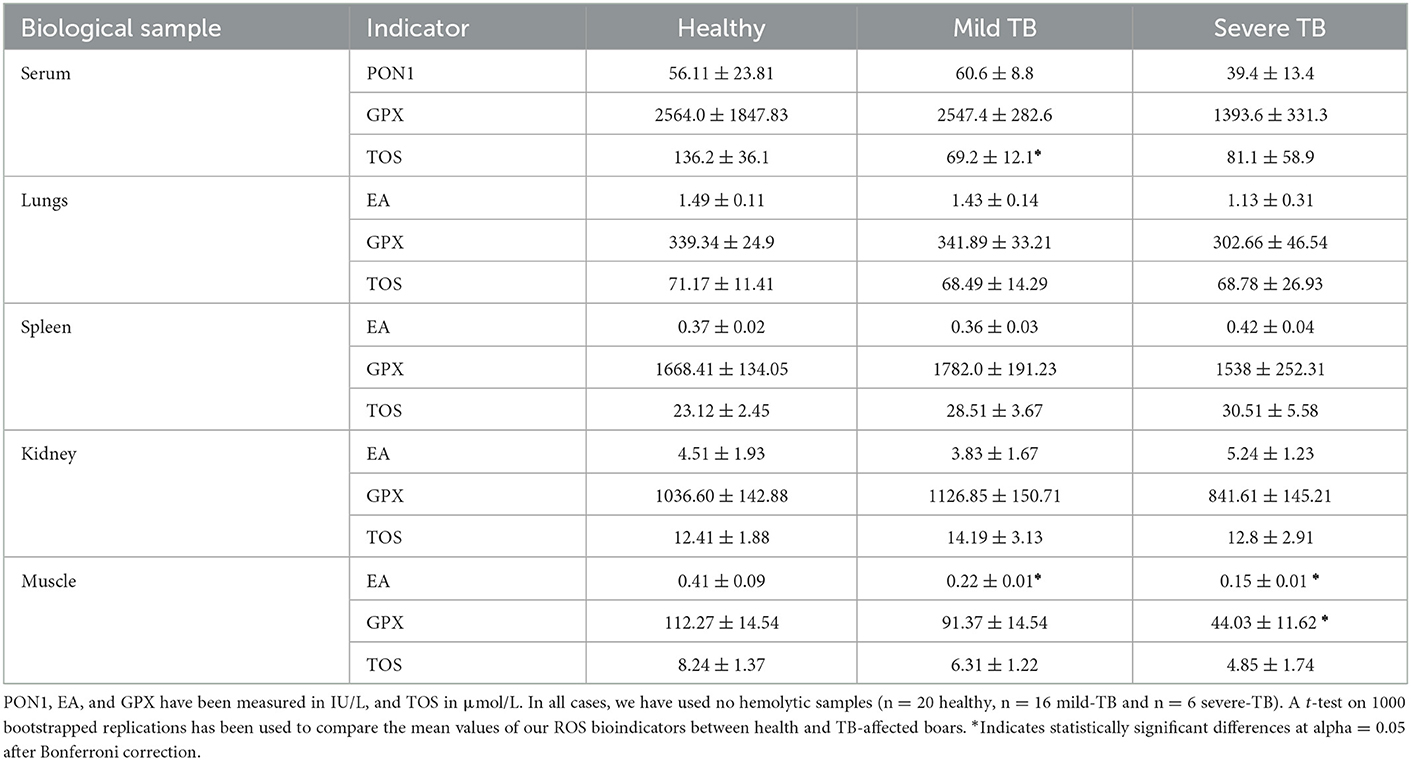
Table 2. Mean ± standard deviation for PON1 (paraoxonase 1), EA (p-nitrophenyl esterase activity), GPX (glutathione peroxidase), and TOS (total oxidative serum) measured in serum, lung, spleen, kidney, and muscle from healthy and TB-infected (mild and severely affected) wild boar, hunter-harvested in central Spain.
4. Discussion
We investigated the most reliable biological sample to assess OS in TB-infected wild boar. The BOS concentrations in serum were significantly higher than in other wild boar tissues such as lungs, spleen, kidney, or muscle. The poor agreement between BOS measures in different samples difficult the assessment of oxidative stress in the wild boar-TB systems. Only some tissues (e.g., TOS in serum and GPX or TOS in muscle) presented statistical differences in BOS concentrations between healthy and TB-affected individuals. These results highlight the importance of refining our understanding of intraindividual differences in OS assessments, particularly, when selecting appropriate samples for assessing specific animal populations' stressors.
Using OS biomarkers to evaluate chronic and acute stressors in wildlife needs to consider potential quantitative intraindividual differences among the biological samples collected. In our study, the higher levels of TOS, EA/ PON1, and GPX observed in serum compared to other tissues might be the additive result of BOS activity from different tissues transported and quantified in serum. More specifically, the activity of GPX varies depending on intra-individual characteristics like the tissues sampled (26, 52, 53), and time of sampling (54) and inter-individual factors like physiological status and sex (54, 55). A similar intra- and inter-individual variation have also been described in the activity of PON1 (42, 56, 57) and a variety of other BOS (24, 58). However, a higher BOS concentration in serum or other specific tissue does not necessarily imply a better sample for OS assessment. For instance, Gassó et al. (35) observed that the variability of OS was correlated with disease status in boars experimentally infected with TB. In the same work, and in line with our results, revealed that TB status was not the main factor explaining the observed variability in OS biomarkers, probably because other intrinsic [e.g., co-infections (33)] and extrinsic factors (e.g., food availability) may hide the TB effects. Our results highlight the need for baseline experiments to confirm the association between specific BOS and a stress source (e.g., disease) before their use as a proxy for animal health (23).
The poor agreement between BOS observed in our study hampers the opportunity of interchange markers between sample origins when assessing OS in wildlife. Variations between conspecifics in BOS at the species and individual scales (e.g., sex, age) are well-documented in different systems (59–61). However, intraindividual differences in BOS, like those observed in our study, have not received much attention despite their significant importance for an adequate sampling plan. This problem was partially addressed in a meta-analysis performed by Isaksson (60) to compare the influence of sampling methods [i.e., invasive sampling (e.g., liver, kidney, and lungs) and less-invasive sampling (blood and urine)] in the OS of vertebrates and invertebrates exposed to environmental pollution. Invasive sampling was more reliable in evaluating OS due to a smaller marker's concentration variation than less-invasive sampling. This result led to the hypothesis that in the case of contaminants and Eco toxicological studies, organs (e.g., the liver) are likely to be a better source of BOS due to their direct role in detoxifying harmful metabolites and pollutants. Tuberculosis in wild boar is characterized by causing a wide variety of pathophysiological consequences, including non-specific inflammatory responses and focalized gross lesions in lymph nodes, lungs, liver, mesenteric lymph nodes, and spleen (33, 34). These multiple potential OS sources might influence the agreement among biological samples highlighting the importance of context-specific consideration when assessing OS in wildlife populations. This includes context-specific extrinsic factors like species and stressor stimulus and context-specific intrinsic factors like biological samples and the physiological mechanisms of the species of interest to deal with the stressor stimulus.
Evidence of altered OS balance in wild boars infected with TB was detected only in animals with mild disease presentation. This can be explained in part due to the ability of wild boars to cope with TB infection (62), supporting the role of wild boar as a TB reservoir in the Mediterranean basin (30), and by the specific role of OS during early stages of TB development in the host. An increase in OS is one of the main physiological mechanisms described in humans and rats to prevent the multiplication of Mycobacterium spp. and TB-like gross lesions (63, 64). This mechanism is likely important in mild TB presentation, maintaining granulomatous lesions in a latent form and directly increasing the OS imbalance. In addition, Gassó et al. (35) reported a similar difficulty detecting altered OS in wild boar naturally infected with TB, hypothesizing that TB alone was not enough to trigger OS imbalance and that host intrinsic factors may also play an important role in OS levels. In this regard, special attention should be directed to the sex and age of the host as they have a direct influence on the immune and stress response of mammals (59–61).
5. Conclusions
The BOS evaluated in this study were detected in higher concentrations in serum compared to samples from other tissues. A poor agreement of BOS among samples was observed, making OS comparison among body fluids and tissue very difficult. In addition, organs from wild boars affected with mild TB (i.e., lung, spleen, kidney, muscle, and serum), were not in oxidative imbalance compared to healthy control animals. This suggests that wild boars may cope well with TB, developing an efficient antioxidant response to avoid OS. Our data indicate that more context-specific research on the association of BOS with stress factors like diseases is needed to allow the use of these parameters in field conservation programs.
Data availability statement
The original contributions presented in the study are included in the article/supplementary material, further inquiries can be directed to the corresponding author/s.
Author contributions
Study conception and design: DG, ES, and RC. Data collection: DG, AT, DR, PG, PF-L, GM, and WG. Analysis and interpretation of results: DG, AT, OA, RV, ES, RC, and GM. Drafted manuscript preparation: OA, DG, and ES. All authors reviewed the results and approved the final version of the manuscript.
Funding
ES is supported by the Spanish Ministerio de Ciencia Innovación y Universidades (MICINN) through a Ramon y Cajal agreement (RYC-2016-21120). GM is a Sierra Hunter Fellow.
Acknowledgments
The authors would like to thank J.R.L.O and members of INGULADOS for their assistance during wild boar sampling.
Conflict of interest
The authors declare that the research was conducted in the absence of any commercial or financial relationships that could be construed as a potential conflict of interest.
Publisher's note
All claims expressed in this article are solely those of the authors and do not necessarily represent those of their affiliated organizations, or those of the publisher, the editors and the reviewers. Any product that may be evaluated in this article, or claim that may be made by its manufacturer, is not guaranteed or endorsed by the publisher.
References
1. Ceballos G, Ehrlich PR, Barnosky AD, García A, Pringle RM, Palmer TM, et al. Accelerated modern human-induced species losses: entering the sixth mass extinction. Sci Adv. (2015) 1:e1400253. doi: 10.1126/sciadv.1400253
2. Hing S, Narayan EJ, Andrew Thompson RC, Godfrey SS. The relationship between physiological stress and wildlife disease: consequences for health and conservation. Wildl Res. (2016) 43:51–60. doi: 10.1071/WR15183
3. Sheriff MJ, Dantzer B, Delehanty B, Palme R, Boonstra R. Measuring stress in wildlife: techniques for quantifying glucocorticoids. Oecologia. (2011) 166:869–87. doi: 10.1007/s00442-011-1943-y
4. Cray C, Zaias J, Altman NH. Acute phase response in animals: a review. Comp Med. (2009) 59:517–26.
5. Kalliokoski O, Jellestad FK, Murison R. A systematic review of studies utilizing hair glucocorticoids as a measure of stress suggests the marker is more appropriate for quantifying short-term stressors. Sci Rep. (2019) 9:11997. doi: 10.1038/s41598-019-48517-2
6. Beaulieu M, Costantini D. Biomarkers of oxidative status: missing tools in conservation physiology. Conserv Physiol. (2014) 2:cou014. doi: 10.1093/conphys/cou014
7. Costantini D, Helfenstein F. Editorial: oxidative Stress and Signal Honesty. Front Ecol Evol. (2017) 5:32. doi: 10.3389/fevo.2017.00032
8. Valko M, Leibfritz D, Moncol J, Cronin MTD, Mazur M, Telser J, et al. Free radicals and antioxidants in normal physiological functions and human disease. Int J Biochem Cell Biol. (2007) 39:44–84. doi: 10.1016/j.biocel.2006.07.001
9. Costantini D. Oxidative stress in ecology and evolution: lessons from avian studies. Ecol Lett. (2008) 11:1238–51. doi: 10.1111/j.1461-0248.2008.01246.x
10. Alonso-Alvarez C, Bertrand S, Faivre B, Sorci G. Increased susceptibility to oxidative damage as a cost of accelerated somatic growth in zebra finches. Funct Ecol. (2007) 21:873–9. doi: 10.1111/j.1365-2435.2007.01300.x
11. Freeman-Gallant CR, Amidon J, Berdy B, Wein S, Taff CC, Haussmann MF, et al. Oxidative damage to DNA related to survivorship and carotenoid-based sexual ornamentation in the common yellowthroat. Biol Lett. (2011) 7:429–32. doi: 10.1098/rsbl.2010.1186
12. Sorci G, Faivre B. Inflammation and oxidative stress in vertebrate host–parasite systems. Philos Trans R Soc Lond B Biol Sci. (2009) 364:71–83. doi: 10.1098/rstb.2008.0151
13. Lykkesfeldt J, Svendsen O. Oxidants and antioxidants in disease: oxidative stress in farm animals. Vet J. (2007) 173:502–11. doi: 10.1016/j.tvjl.2006.06.005
14. Oliver-Guimerá A, Martínez-Carrasco C, Tvarijonaviciute A, Ybáñez MR, Martínez-Guijosa J, López-Olvera JR, et al. The physiological cost of male-biased parasitism in a nearly monomorphic mammal. Parasit Vectors. (2017) 10:200. doi: 10.1186/s13071-017-2060-5
15. Pacios-Palma I, Moreno S, Selman C, Rouco C. Oxidative stress in wild European rabbits naturally infected with myxoma virus and rabbit haemorrhagic disease virus. Eur J Wildl Res. (2018) 64:1–17. doi: 10.1007/s10344-018-1203-0
16. Becker K, Tilley L, Vennerstrom JL, Roberts D, Rogerson S, Ginsburg H, et al. Oxidative stress in malaria parasite-infected erythrocytes: host–parasite interactions. Int J Parasitol. (2004) 34:163–89. doi: 10.1016/j.ijpara.2003.09.011
17. Fatima M, Usmani N, Firdaus F, Zafeer MF, Ahmad S, Akhtar K, et al. In vivo induction of antioxidant response and oxidative stress associated with genotoxicity and histopathological alteration in two commercial fish species due to heavy metals exposure in northern India (Kali) river. Comp Biochem Physiol Toxicol Pharmacol. (2015) 176:17–80. doi: 10.1016/j.cbpc.2015.07.004
18. Circu ML, Aw TY. Intestinal redox biology and oxidative stress. Semin Cell Dev Biol. (2012) 23:729–37. doi: 10.1016/j.semcdb.2012.03.014
19. Gamboa JL, Billings FT, Bojanowski MT, Gilliam LA, Yu C, Roshanravan B. Mitochondrial dysfunction and oxidative stress in patients with chronic kidney disease. Physiol Rep. (2016) 4:12780. doi: 10.14814/phy2.12780
20. Rahman I, MacNee W. Oxidative stress and regulation of glutathione in lung inflammation. Eur Respir J. (2000) 16:534–54. doi: 10.1034/j.1399-3003.2000.016003534.x
21. Helfenstein F, Losdat S, Møller AP, Blount JD, Richner H. Sperm of colourful males are better protected against oxidative stress. Ecol Lett. (2010) 13:213–22. doi: 10.1111/j.1461-0248.2009.01419.x
22. van de Crommenacker J, Richardson DS, Koltz AM, Hutchings K, Komdeur J. Parasitic infection and oxidative status are associated and vary with breeding activity in the Seychelles warbler. Proc Biol Sci. (2012) 279:1466–76. doi: 10.1098/rspb.2011.1865
23. Argüelles S, García S, Maldonado M, Machado A, Ayala A. Do the serum oxidative stress biomarkers provide a reasonable index of the general oxidative stress status? Biochim. Biophys Acta. (2004) 1674:251–9. doi: 10.1016/j.bbagen.2004.06.023
24. Marrocco I, Altieri F, Peluso I. Measurement and clinical significance of biomarkers of oxidative stress in humans. Oxid Med Cell Longev. (2017) 2017:6501046. doi: 10.1155/2017/6501046
25. Margaritelis NV, Veskoukis AS, Paschalis V, Vrabas IS, Dipla K, Zafeiridis A, et al. Blood reflects tissue oxidative stress: a systematic review. Biomarkers. (2015) 20:97–108. doi: 10.3109/1354750X.2014.1002807
26. Tkachenko H, Kurhaluk N, Grudniewska J, Andriichuk A. Tissue-specific responses of oxidative stress biomarkers and antioxidant defenses in rainbow trout Oncorhynchus mykiss during a vaccination against furunculosis. Fish Physiol Biochem. (2014) 40:1289–300. doi: 10.1007/s10695-014-9924-9
27. Jack CI, Jackson MJ, Hind CR. Circulating markers of free radical activity in patients with pulmonary tuberculosis. Tuber Lung Dis. (1994) 75:132–7. doi: 10.1016/0962-8479(94)90042-6
28. Kwiatkowska S, Piasecka G, Zieba M, Piotrowski W, Nowak D. Increased serum concentrations of conjugated diens and malondialdehyde in patients with pulmonary tuberculosis. Respir Med. (1999) 93:272–6. doi: 10.1016/S0954-6111(99)90024-0
29. Palanisamy GS, Kirk NM, Ackart DF, Shanley CA, Orme IM, Basaraba RJ, et al. Evidence for oxidative stress and defective antioxidant response in guinea pigs with tuberculosis. PLoS ONE. (2011) 6:e26254. doi: 10.1371/journal.pone.0026254
30. Naranjo V, Gortazar C, Vicente J, de la Fuente, J. Evidence of the role of European wild boar as a reservoir of Mycobacterium tuberculosis complex. Vet Microbiol. (2008) 127:1–9. doi: 10.1016/j.vetmic.2007.10.002
31. Barasona JA, Acevedo P, Diez-Delgado I, Queiros J, Carrasco-García R, Gortazar C, et al. Tuberculosis-associated death among adult wild boars, Spain, 2009-2014. Emerg Infect Dis. (2016) 22:2178–80. doi: 10.3201/eid2212.160677
32. Acevedo-Whitehouse K, Vicente J, Gortazar C, Höfle U, Fernández-Mera IG, Amos W. Genetic resistance to bovine tuberculosis in the Iberian wild boar. Mol Ecol. (2005) 14:3209–17. doi: 10.1111/j.1365-294X.2005.02656.x
33. Risco D, Serrano E, Fernández-Llario P, Cuesta JM, Gonçalves P, García-Jiménez WL, et al. Severity of bovine tuberculosis is associated with co-infection with common pathogens in wild boar. PLoS ONE. (2014) 9:e110123. doi: 10.1371/journal.pone.0110123
34. García-Jiménez WL, Benítez-Medina JM, Fernández-Llario P, Abecia JA, García-Sánchez A, Martínez R, et al. Comparative pathology of the natural infections by Mycobacterium bovis and by Mycobacterium caprae in wild boar (Sus scrofa). Transbound Emerg Dis. (2013) 60:102–9. doi: 10.1111/j.1865-1682.2012.01321.x
35. Gassó D, Vicente J, Mentaberre G, Soriguer R, Rodríguez RJ, Navarro-González N, et al. Oxidative stress in wild boars naturally and experimentally infected with Mycobacterium bovis. PLoS ONE. (2016) 11:e0163971. doi: 10.1371/journal.pone.0163971
36. Gonçalves P, Risco D, Fernández-Llario P, Barquero-Pérez O, Serrano E, Hermoso-de-Mendoza J. A new method for ageing wild boar using dental measures. Ecol Indic. (2016) 62:328–32. doi: 10.1016/j.ecolind.2015.11.013
37. Risco D, Gonçalves P, Mentaberre G, Navarro-González N, Casas-Díaz E, Gassó D, et al. Biometrical measurements as efficient indicators to assess wild boar body condition. Ecol Indic. (2018) 88:43–50. doi: 10.1016/j.ecolind.2017.12.048
38. Arenas-Montes A, García-Bocanegra I, Paniagua J, Franco JJ, Miró F, Fernández-Morente M, et al. Blood sampling by puncture in the cavernous sinus from hunted wild boar. Eur J Wildl Res. (2013) 59:299–303. doi: 10.1007/s10344-013-0701-3
39. Renerre M, Dumont F, Gatellier P. Antioxidant enzyme activities in beef in relation to oxidation of lipid and myoglobin. Meat Sci. (1996) 43:111–21. doi: 10.1016/0309-1740(96)84583-9
40. Kamodyov N, Banasová L, Janšáková K, Koborová I, Tóthová L, Stanko P, et al. Blood contamination in saliva: impact on the measurement of salivary oxidative stress markers. Dis Markers. (2015) 2015:479251. doi: 10.1155/2015/479251
41. Tvarijonaviciute A, Tecles F, Caldin M, Tasca S, Cerón J. Validation of spectrophotometric assays for serum paraoxonase type-1 measurement in dogs. Am J Vet Res. (2012) 73:34–41. doi: 10.2460/ajvr.73.1.34
42. Ceron JJ, Tecles F, Tvarijonaviciute A. Serum paraoxonase 1 (PON1) measurement: an update. BMC Vet Res. (2014) 10:74. doi: 10.1186/1746-6148-10-74
43. Erel O. A new automated colorimetric method for measuring total oxidant status. Clin Biochem. (2005) 38:1103–11. doi: 10.1016/j.clinbiochem.2005.08.008
44. Franco-Martinez L, Romero D, García-Navarro JA, Tecles F, Teles M, Tvarijonaviciute A, et al. Measurement of p-nitrophenyl acetate esterase activity (EA), total antioxidant capacity (TAC), total oxidant status (TOS) and acetylcholinesterase (AChE) in gills and digestive gland of Mytilus galloprovincialis exposed to binary mixtures of Pb, Cd and Cu. Environ Sci Pollut Res. (2016) 23:25385–92. doi: 10.1007/s11356-016-7677-y
45. Contreras-Aguilar MD, Escribano D, Martínez-Subiela S, Martínez-Miró S, Rubio M, Tvarijonaviciute A, et al. Influence of the way of reporting alpha-Amylase values in saliva in different naturalistic situations: a pilot study. PLoS ONE. (2017) 12:e0180100. doi: 10.1371/journal.pone.0180100
46. Zaki R, Bulgiba A, Nordin N, Azina Ismail N. A systematic review of statistical methods used to test for reliability of medical instruments measuring continuous variables. Iran J Basic Med Sci. (2013) 16:803–7.
47. Bland JM, Altman DG. Statistical methods for assessing agreement between two methods of clinical measurement. Lancet. (1986) 1:307–10. doi: 10.1016/S0140-6736(86)90837-8
48. Ludbrook J. Statistical techniques for comparing measurers and methods of measurement: a critical review. Clin Exp Pharmacol Physiol. (2002) 7:527–36. doi: 10.1046/j.1440-1681.2002.03686.x
49. Datta D. Blandr: A Bland-Altman Method Comparison Package for R (2017). doi: 10.5281/zenodo.824514
51. R Core Team. R: A Language and Environment for Statistical Computing. R Foundation for Statistical Computing Vienna, Austria (2018).
52. Szymonik-Lesiuk S, Czechowska G, Stryjecka-Zimmer M, Słomka M, Madro A, Celiński K, et al. Catalase, superoxide dismutase, and glutathione peroxidase activities in various rat tissues after carbon tetrachloride intoxication. J Hepatobiliary Pancreat Surg. (2003) 10:309–15. doi: 10.1007/s00534-002-0824-5
53. Tappel ME, Chaudiere J, Tappel AL. Glutathione peroxidase activities of animal tissues. Comp Biochem Physiol B. (1982) 73:945–9. doi: 10.1016/0305-0491(82)90341-8
54. Finley JW, Kincaid RL. Effect of sex and time of sampling on selenium and glutathione peroxidase activity in tissues of mature rats. Biol Trace Elem Res. (1991) 29:181–91. doi: 10.1007/BF03032676
55. Capel ID, Smallwood AE. Sex Differences in the Glutathione Peroxidase Activity of Various Tissues of the Rat. J Urol. (1984) 40:367–78.
56. Ferreira CER, Haas CS, Goularte KL, Rovani MT, Cardoso FF, Schneider A, et al. Expression of paraoxonase types 1, 2. and 3 in reproductive tissues and activity of paraoxonase type 1 in the serum and seminal plasma of bulls. Andrologia. (2018) 50:e12923 doi: 10.1111/and.12923
57. Maksoud E, Taha N, Mandour A, Lebda M, El Mmorshedy A. Distribution of paraoxonase-1 enzyme activity in some camels tissues. Alexandria J Vet Sci. (2016) 51:2. doi: 10.5455/ajvs.207546
58. Frijhoff J, Winyard PG, Zarkovic N, Davies SS, Stocker R, Cheng D, et al. Clinical relevance of biomarkers of oxidative stress. Antioxid Redox Signal. (2015) 23:1144–70. doi: 10.1089/ars.2015.6317
59. Costantini D, Czirják GÁ, Melzheimer J, Menges V, Wachter B. Sex and species differences of stress markers in sympatric cheetahs and leopards in Namibia. Comp Biochem Physiol A Mol Integr Physiol. (2019) 227:8–13. doi: 10.1016/j.cbpa.2018.09.002
60. Isaksson C. Pollution and its impact on wild animals: a meta-analysis on oxidative stress. Ecohealth. (2010) 7:342–50. doi: 10.1007/s10393-010-0345-7
61. Monaghan P, Metcalfe NB, Torres R. Oxidative stress as a mediator of life history trade-offs: mechanisms, measurements and interpretation. Ecol Lett. (2009) 12:75–92. doi: 10.1111/j.1461-0248.2008.01258.x
62. Barroso P, Serrano E, Carpio A, Acevedo P, Vicente J, Gortázar C, et al. Low impact of tuberculosis severity on wild boar body condition. Res Vet Sci. (2023) 155:161–7. doi: 10.1016/j.rvsc.2023.01.014
63. Chan J, Tanaka K, Carroll D, Flynn J, Bloom BR. Effects of nitric oxide synthase inhibitors on murine infection with Mycobacterium tuberculosis. Infect Immun. (1995) 63:736–40. doi: 10.1128/iai.63.2.736-740.1995
Keywords: glutathione peroxidase, Mycobacterium tuberculosis, physiological ecology, p-nitrophenyl esterase activity, Sus scrofa, oxidative stress
Citation: Aleuy OA, Gassó D, Tvarijonaviciute A, Risco D, Garcia W, Gonçalves P, Fernández-Llario P, Mentaberre G, Velarde R, Serrano E and Cuenca R (2023) Tissue-specific assessment of oxidative status: Wild boar as a case study. Front. Vet. Sci. 10:1089922. doi: 10.3389/fvets.2023.1089922
Received: 04 November 2022; Accepted: 30 January 2023;
Published: 06 March 2023.
Edited by:
Francesca Ciani, University of Naples Federico II, ItalyReviewed by:
Jiri Pikula, University of Veterinary and Pharmaceutical Sciences Brno, CzechiaRichard Meitern, University of Tartu, Estonia
José Pérez, Universidad de Córdoba, Spain
Copyright © 2023 Aleuy, Gassó, Tvarijonaviciute, Risco, Garcia, Gonçalves, Fernández-Llario, Mentaberre, Velarde, Serrano and Cuenca. This is an open-access article distributed under the terms of the Creative Commons Attribution License (CC BY). The use, distribution or reproduction in other forums is permitted, provided the original author(s) and the copyright owner(s) are credited and that the original publication in this journal is cited, in accordance with accepted academic practice. No use, distribution or reproduction is permitted which does not comply with these terms.
*Correspondence: Emmanuel Serrano, ZW1tYW51ZWwuc2VycmFubyYjeDAwMDQwO3VhYi5jYXQ=
†These authors have contributed equally to this work