- 1Postgraduate Program in Animal Science (PPGCAN), Institute of Veterinary Medicine, Federal University of Para (UFPA), UFRA, Brazilian Agricultural Research Corporation (EMBRAPA), Castanhal, Brazil
- 2Federal Rural University of the Amazon (UFRA), Institute of Animal Health and Production, Belém, Brazil
- 3Federal Rural University of the Amazon (UFRA), Institute of Agricultural Sciences, Capanema, Brazil
- 4Federal University of Western Pará (UFOPA), Institute of Engineering and Geosciences, Santarém, Brazil
- 5Federal University of the South and Southeast of Pará (UNIFESSPA), Institute of Veterinary Medicine, Xinguara, Pará, Brazil
Thermal stress causes severe effects on the wellbeing and reproduction of cattle, including changes in oogenesis and spermatogenesis, generating great concerns, which last for decades. In cattle, the occurrence of thermal stress is associated with a reduction in the production of spermatozoids and ovarian follicles, in addition to the increase of major and minor defects in gametes or in their intermediate stages. In bovine females able to reproduce, a reduction in the rate of estrus manifestation and an increase in embryonic mortality has been observed. Therefore, keeping animals on good welfare conditions, with water supply and in shaded areas can favor the improvement of different reproductive parameters. For all this, the present study aimed to gather, synthesize and argue recent studies related to animal welfare, focusing on the effects of thermal stress on the reproduction of cattle, aiming to support possible strategies to mitigate the harmful effects of thermal stress in this species.
Introduction
Animal welfare (AW) is defined as the physical or mental state of an animal in relation to the environment in which it lives and dies (1). Thus, a good degree of AW means to say that an individual is safe, healthy, comfortable, well-nourished and free to express natural behaviors of the species without suffering from harmful mental states such as pain, frustration and stress.
The behavioral reactions observed in animals can be determined by the way they react to the environment, with animals of other species or the same, as well as humans, which can directly interfere with the change in their behavior, posture and attitude (2). When the animals have behavioral characteristics defined as normal in an unknown environment, it is believed that they have a good degree of AW, for example, the practice of rumination and the waiting period signals tranquility (3).
According to the five domains of animal welfare proposed by Mellor et al. (4), the environment (for example, physical and atmospheric characteristics) impacts the physical and mental health of all species.
In cattle, one of the most mentioned causes capable of reducing their well-being are thermal conditions, that is: temperatures that are too high or too low, due to anatomical reasons or the places where they are raised (5).
Several consequences derived from heat stress can be cited, such as reduced oocyte and sperm quality. Thus, in tropical climate regions these variables express greater influence on the thermal comfort of cattle, a fact that worsens even more in pastures without the presence of trees or shading, because the animals start to receive high solar radiation, and are exposed to critical temperatures and high relative humidity, resulting in thermal stress (4).
Most livestock species, including cattle, define their thermal comfort zone between 16 and 25°C, that is, in this range of ambient temperature the basal metabolism is lower and therefore thermoregulation takes place without evapotranspiration (6).
Several studies have been carried out to measure the effects resulting from microclimates that interfere with the thermal comfort of cattle, the main variables being measurable; temperature, humidity, wind speed and solar radiation that are used to determine the indices of thermal comfort in animals (7–13).
Cattle, being a homeothermic animal, have the ability to control body temperature when exposed to large temperature variations, and the thermoregulation mechanism is responsible for this balance (14). Thus, when the animal is exposed to critical temperatures, sweating, increased water intake, respiratory rate and vasodilation can occur, and when the ambient temperature is higher than the thermoneutrality zone (Figure 1) thermal stress can occur, harming wellbeing, health and reproduction (16).
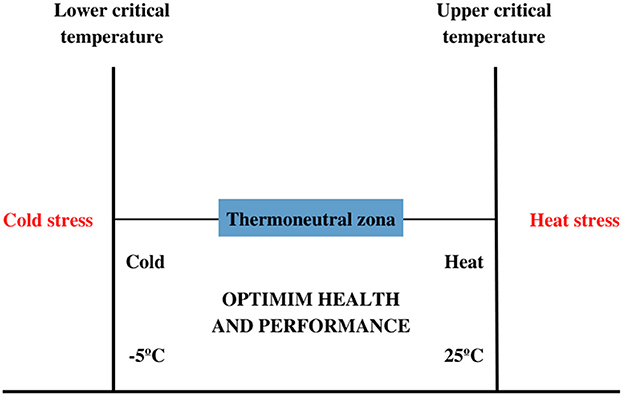
Figure 1. Critical temperatures and thermo-neutral zone in dairy cattle. Adapted from Avendaño-Reyes (15).
For all these reasons, the main objective of this research was to analyze scientific information on welfare, but also to gather, synthesize and discuss recent discoveries, with a focus on heat stress in bovine reproduction, providing information capable of supporting strategies to mitigate its negative effects in this species.
Generalities about animal welfare
The AW theme is widely discussed in animal production. Historically, it began in the United Kingdom in 1964, with different reports published by journalist Ruth Harrison, warning of the need to observe animals as living beings and not mechanically, as inert beings (17).
In 1965, the British Parliament set up a committee coordinated by Professor Rogers Brambell to provide information to livestock farmers on how to raise animals. The guidelines set out in the report were known as: “Brambell's five freedoms”, such as: “turn around”, “lie down”, “get up”, “scan your limbs” and “take care of your own body” (18). After these publications, different views were established about the AW, seeking to create criteria in order to measure this parameter in the animals.
In conceptual terms, the definition of the most accepted AW was established by Broom (19), who described it as the state of an individual during his attempts to balance himself in an environment. Thus, when animals are placed in different environments or subjected to inadequate management, they tend to present stress, which results in negative aspects in production (20).
The five freedoms are presented as an important milestone in the science of AW, where the animal must be: 1. free from hunger and thirst, with free access to water and diet to maintain health and vigor; 2. free from discomfort, with an appropriate environment, with shelter and comfortable waiting area; 3. free from pain, injury and illness, with rapid prevention, diagnosis and treatment; 4. free to express normal and natural behavior, with sufficient space, adequate and species-specific facilities; and 5. freedom from fear and distress, with conditions and treatment that prevent mental suffering (21). However, it is worth noting that the FAWC suggests that the five freedoms should not be adopted as minimum animal welfare standards (22) (Figure 2).
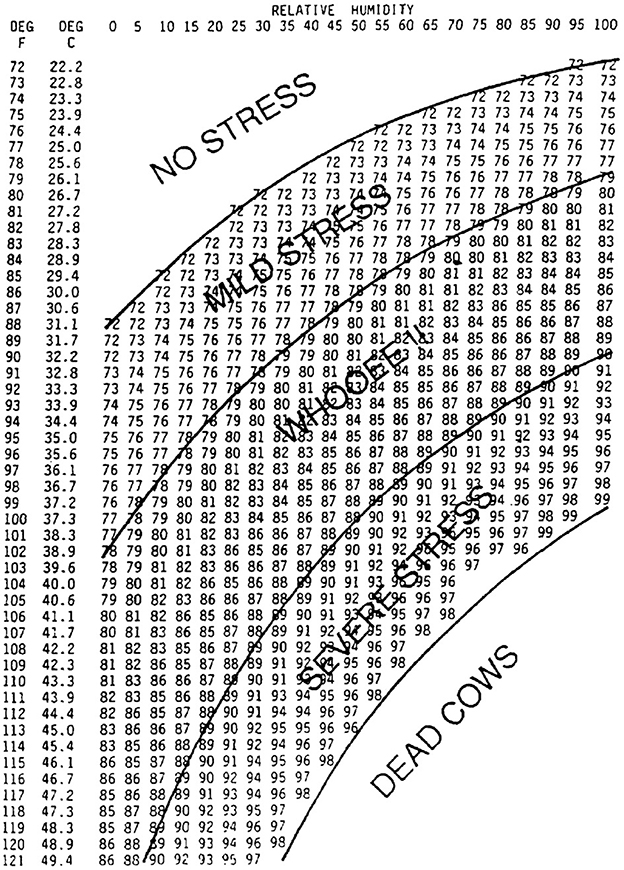
Figure 2. Temperature-humidity index (THI) table for dairy producer to estimate heat stress for dairy cows. Deg, Degrees. Relative humidity expressed as percentage. Adapted from Armstrong (23).
In 2004, the European Union presented the “Welfare Quality® Project”, stimulating integration between researchers about AW from different institutions. Four approaches and 12 criteria were proposed (23), such as: Good nutrition, defined by absence of hunger and prolonged thirst (1 and 2); Good accommodation, for waiting (3) and providing thermal comfort (4), in addition to ease of movement (5); Good health, characterized by the absence of injuries (24), diseases (6), pain induced by handling (7); and present appropriate behavior, such as: the expression of social behavior (8), of other behaviors (9), in addition to having a good interaction between human and animal (10) and positive emotional states (11).
Mellor (25) proposed the model of the five domains, seeking to encompass the negative and positive aspects related to animals, divided into four physical principles and one mental, namely: nutrition, environment, health, behavior and the mental state of animals, considering- whether positive and negative emotions and feelings are presented. Thus, the search for positive AW indicators is essential, combined with measures that aim to maximize the expression of normal behaviors (26).
Key animal welfare indicators
To understand AW, it is necessary to know the species to be studied, considering the behavioral characteristics in the natural habitat, as well as the breeding system of the individuals. When trying to measure AW, attention should be paid to a variety of multidimensional indicators, in addition to different parameters to understand the general state of everyone (27).
AW indicators can be classified into two types: the first is related to animals that cannot adapt to a certain environment, the second represents the effort made by the animal to adapt to the environment (28).
Mismanagement errors during the animal's adaptation process in a given environment can cause problems such as an increase in the mortality rate (29) (Table 1) and a decrease in live-born calves (30). Attempts to adapt to the new environment may present different indicators, such as an increase in the number of diseases (31), a decrease in milk production (32) (Table 2) and in the growth rate of animals (34).
Another important factor is the milk production index, which is also affected when heat stress is evident in dairy cattle (Figure 3).
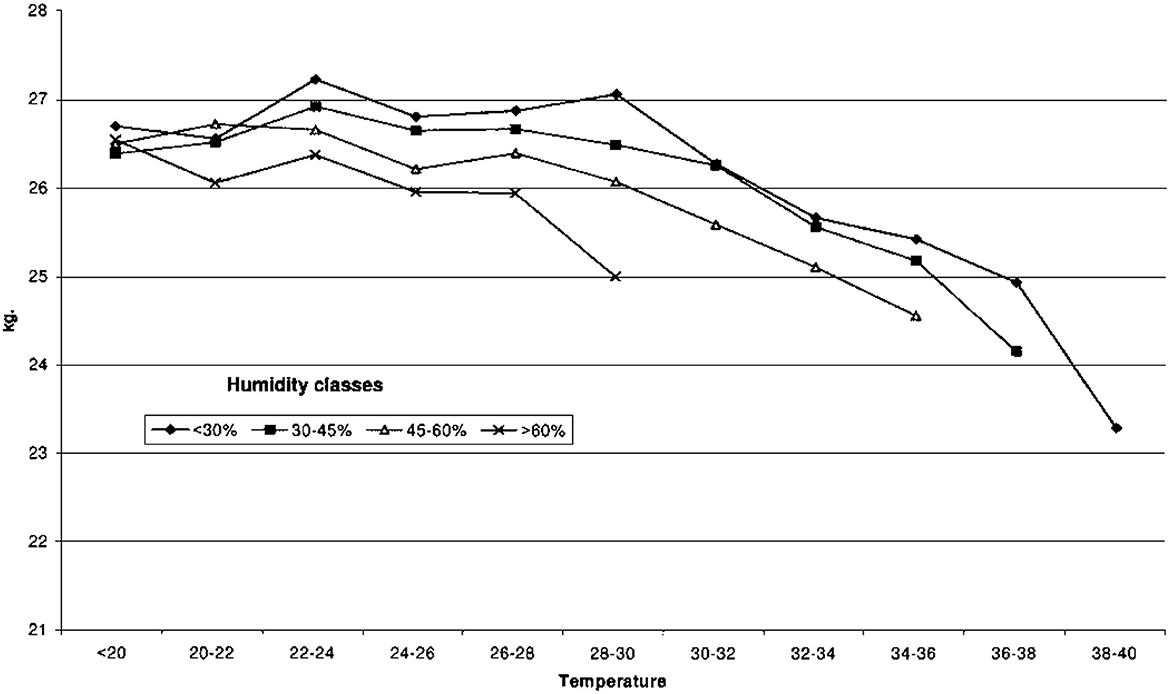
Figure 3. For milk production, the yield was relatively constant up to about 24°C and then began to decline as the temperature increased. Adapted from the study developed by Ravagnolo et al. (35).
Regarding fertility (36), heat stress promotes deleterious effects at all stages (Figures 4–6). Starting from the development of the oocyte (38, 39), continuing through the later stages, as well as its fertilization capacity (40). It is also harmful to the estrous cycle and estrous behavior (41). As well as the development and implantation of the embryo, persisting in the uterine environment, extending to the fetal calf (42).
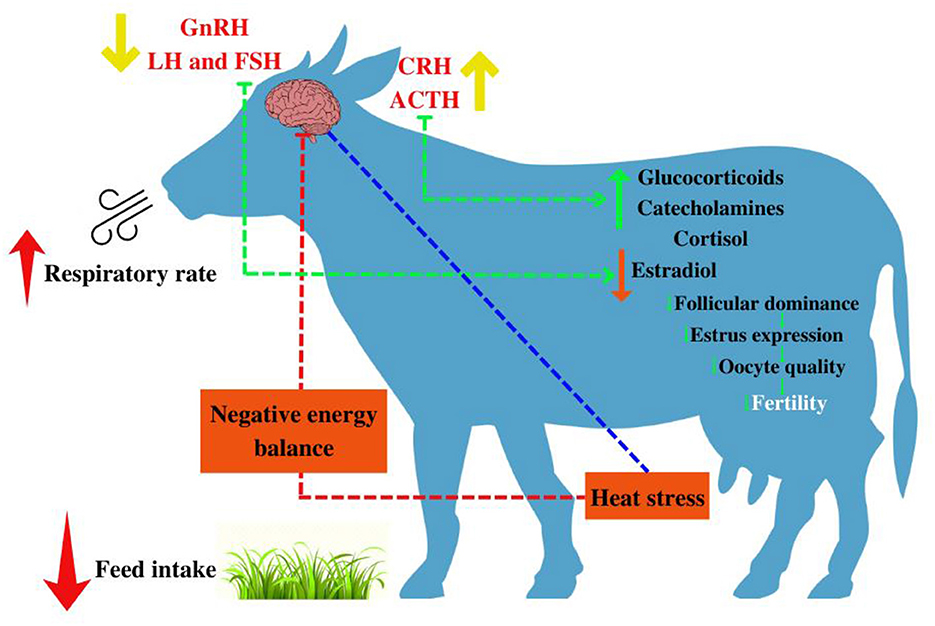
Figure 4. Impact of heat stress on female reproductive performance. Adapted from Krishnan et al. (37). Mitigation of the heat stress impact in livestock reproduction.

Figure 5. Impact of heat stress on pregnancy in livestock. Adapted from Krishnan et al. (37). Mitigation of the heat stress impact in livestock reproduction.
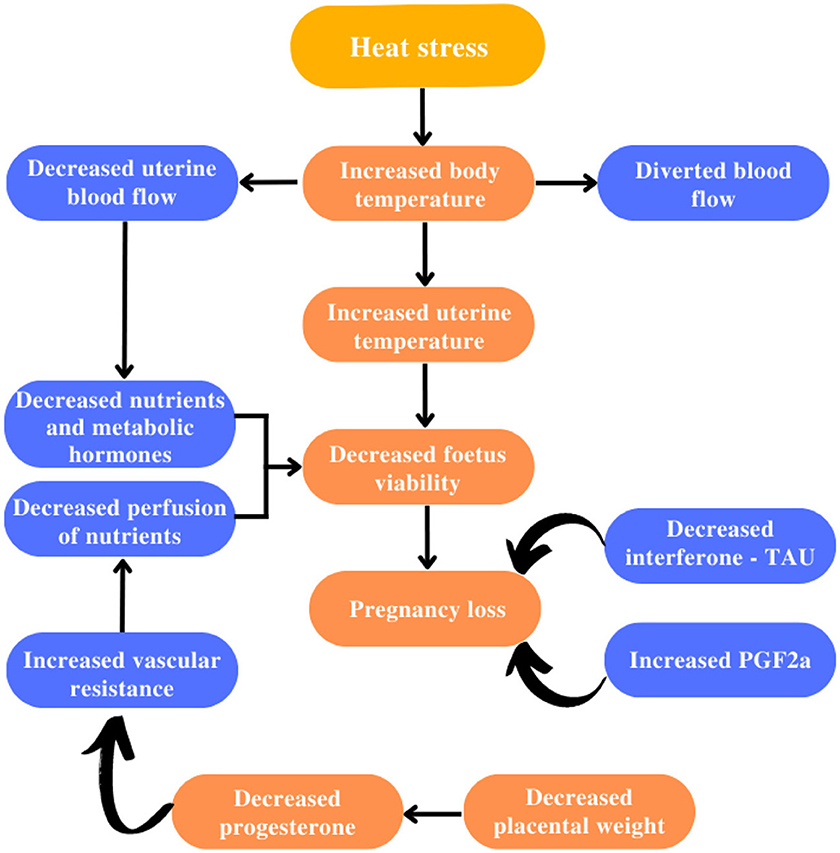
Figure 6. Aspects of the main impacts caused by heat stress during cattle handling. Adapted from Krishnan et al. (37).
The assessment of the degree of AW can be performed under different conditions, such as short-term conditions, for example, in the case of pre-slaughter management, instantaneous indicators such as heart rate, blood cortisol levels and the different behaviors of the animals during the slaughter are used (43), or conditions of prolonged duration, occurring in rearing systems, in which animals have a longer time to adapt (44).
AW is associated with different indicators that arise in response to forms of adaptation of environments, evidenced among animals; therefore, the adoption of a single indicator is an unfeasible alternative (28). In this sense, Botreau et al. (45) provided indicators that can be adopted in the evaluation of AW, such as the principle of good food, good accommodation, good health and expression of appropriate behavior.
In the first principle “good nutrition”, two criteria were defined that address issues related to the absence of hunger and thirst for long periods. In the second, described as “good accommodation”, comfort criteria were formulated related to the animals' waiting place, thermal comfort and mobility within the facilities. The third, “good health”, follows the criteria of absence of injuries, diseases and pain caused during the handling of the animals. Finally, the fourth criterion: “appropriate behavior”, has as its principle the expression of adequate social behavior and other behaviors, a harmonious relationship between the human being and the animal and the positive emotional state of the animals (45, 46).
Thus, in each criterion, we sought to identify specific measures that can be adopted to carry out the AW assessment, giving importance to its validity, repeatability and feasibility (47). It is worth mentioning that the measures established aim to evaluate the welfare of the animals individually, as it allows acquiring information directly linked to the adaptation of the animal to the environment, pointing out its performance in the breeding system, during a given production cycle.
Four principles are intrinsically linked to the reproduction of cattle and can be used when evaluating the AW, they are: nutrition related to the supply of water, food and essential nutrients; the environment, which represents the environmental challenges that are inserted; health linked to diseases, injuries and functional impairment; animal behavior, and, finally, the mental state of animals, which considers the positive and negative emotions and feelings presented (48).
Behavioral indicators of AW in cattle
The behavioral reactions observed in animals can be determined by the way they react to the environment, animals of other species or the same, as well as the presence of human beings, which can directly interfere in the change of their behavior, attitude and posture (2). Communication between cattle is carried out through their senses and their behaviors are linked to their perception ability to relate sensory functions such as vision, hearing, smell and touch (49).
Bovines use visual signals as a strong means of communication, because due to issues related to the evolutionary process, these animals have anatomically large and well-developed eyes, in addition to panoramic vision (320°), which help in their survival. Visual sign language or body movements may relate to body movement or just a part of it (50).
Regarding body movement, the head and tail deserve to be highlighted. They have great mobility and allow different movements in relation to the body, being able to express different types of information, mainly during aggressive or submissive behavior (2), constituting, therefore, a strong mood indicator (51).
The vocalizations of cattle do not prove to be specific to a given situation, but mainly due to the degree of arousal, being reported mainly during situations of stress (Figure 7, Table 3), frustration and pain (50, 53).
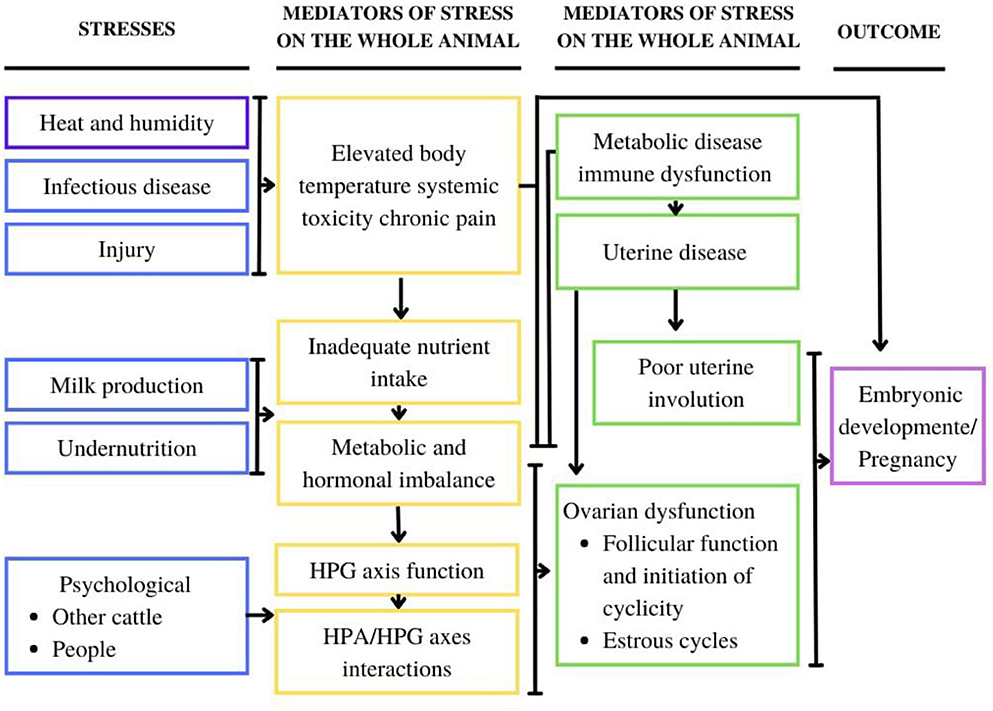
Figure 7. Schematic drawing on the main aspects that affect cattle, as well as the mediators of stress in the animal, and finally the associated strain that affects the pregnancy outcome. HPG, hypothalamic-pituitary-gonadal; HPA, hypothalamic-pituitary-adrenal. Adapted from Matthew (52).
The following parameters are recommended for interpreting vocalization levels: if 1% of the cattle emit sound (excellent), 3% (acceptable), 4–10% (not acceptable) and >10% indicate serious problems related to welfare (54). When an animal continuously and intensely avoids a situation, it externalizes information related to its degree of wellbeing. Thus, an accentuated avoidance reaction, during the presence of a stimulus, can signal a good or bad degree of welfare (55).
When an animal cannot express a natural behavior, even trying to perform it at different times, it has a poor degree of wellbeing when compared to another animal that can perform the behavior. For this reason, knowing the behavior of animals in a natural environment makes it more feasible to evaluate the behavior of individuals in a different condition than the natural one, allowing a more accurate diagnosis of welfare (56).
Stress
Stress can be defined as the set of physiological reactions that occurs in an organism in order to adapt to different situations. However, these reactions can cause imbalance, depending on the intensity and duration (57). The emotional state of the animal, whether positive or negative, can cause stress. However, the adaptation to the new environment will provide the restoration of balance or return to the normal state (58).
The animal organism tends to prioritize homeostasis, however, when subjected to factors that trigger stress, they may respond through a combination of biochemical, physiological and behavioral reactions (2).
The General Adaptation Syndrome (GAS) is divided into three different phases: the first phase, also called alarm or alert, is characterized by the response of the Sympathetic Nervous System (SNS), which signals the activation of the adrenal glands, with secretion of hormones cortisol, adrenaline and noradrenaline. As a consequence, there will be tachycardia, tachypnea and elevated blood glucose levels (59).
The second phase (adaptation or resistance) is characterized by the continuous secretion of glucocorticoid hormones (Figure 8), providing the animal's organism with a considerable improvement in physical and cognitive activity, which aims to nullify the aggressor (59). Finally, the last and third phase begins, called exhaustion, when the animals are subjected to intense and prolonged stress, which thus preserves the body's response. Thus, stress becomes chronic, which promotes physiological reactions and changes in behavior, in addition to causing energy overload and system exhaustion (60).
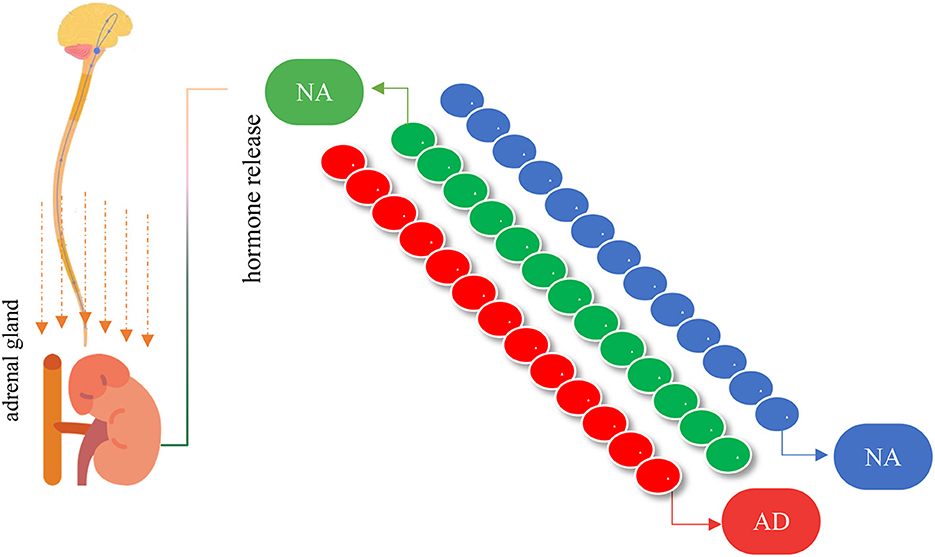
Figure 8. Illustration of the initial response when there is a stressor stimulus in cattle. The sympathetic nervous system stimulates the adrenal gland to release catecholamines [adrenaline (AD) and noradrenaline (NAD)] and cortisol (CT).
Heat stress
The sum of external environmental forces acting on animals is defined as heat stress, which results in an increase in body temperature and causes a physiological response (61). Reduced productivity, decreased wellbeing, increased susceptibility to diseases and decreased fertility are effects of heat stress, which in extreme situations increase the mortality rate (62) and cause negative effects on all the domesticated species (63).
Thus, among the parameters affected by heat stress, the energy reserves of cattle stand out (64, 65), impairing the development of beef cattle and lactating cows, as these animals need good nutrition. Heat dissipation is the animal's main response to heat stress (64) which takes place through behavioral and physiological thermoregulatory mechanisms (65–67).
In this scenario, these mechanisms dissipate heat through sweating and peripheral circulation, increased respiration, wheezing, and decreased rate of food intake to retain metabolic heat (67). Finally, the detailed mechanism of heat dissipation in cattle exposed to heat stress was be reviewed by Berman (68) and Collier et al. (69).
Other studies sought to investigate the consequences caused by heat stress on the physiology of dairy cattle [for example, (70–73)] and confined livestock [for example, (74–81)] with revision limitation in the cow-calf sector. Note that most mechanisms are consistent between beef and dairy cattle. The different visual mechanisms of heat stress in beef cattle can be seen in Figure 9.
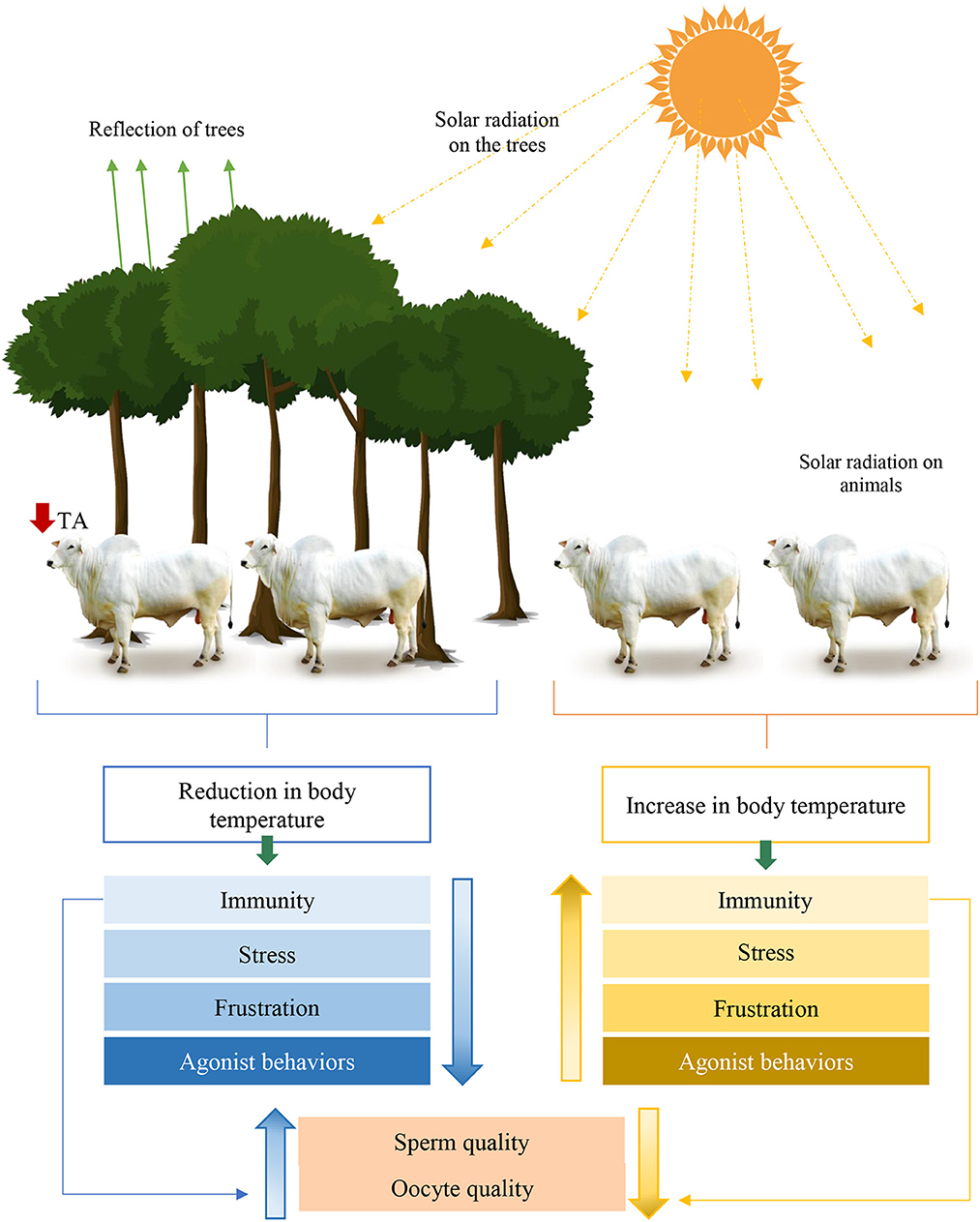
Figure 9. Infographic of environmental effects on physiological, behavioral and stress parameters in male and female cattle conditioned in sun and shade systems. Adapted from Edwards-Callaway et al. (82). TA, Ambient Temperature.
Egg loss and early embryo loss caused by heat stress
Embryonic death is considered one of the main causes of low reproductive efficiency in cattle, reaching 25–40% of cases and can be divided into early and late, and early can record 40% of embryonic losses. In bovids, the occurrence of early embryonic losses begins between the 7th and 16th day of gestation, during the hatching of the blastocyst and its subsequent implantation, without interfering with the extension of the cycles (83).
In addition, it directly harms production and economic investment in the sector. For this reason, some parameters seek to be addressed in order to reduce cases of embryonic loss and ensure pregnancy rates in cattle. Regarding the clinical factors manifested by early embryonic loss, progesterone deficiency, inbreeding, multiple pregnancy, incompatibility, chromosomal aberrations and heat stress, among others, stand out.
Heat stress is related to early embryonic losses, more common in high dairy animals. Due to the presence of greater productive capacity, there is a greater intake of dry matter, requiring an instantaneous metabolic work, causing the animal to produce more heat. Heat stress between the 1st and 3rd days of embryo development decreases embryo viability due to its high sensitivity (42) (Figure 10).
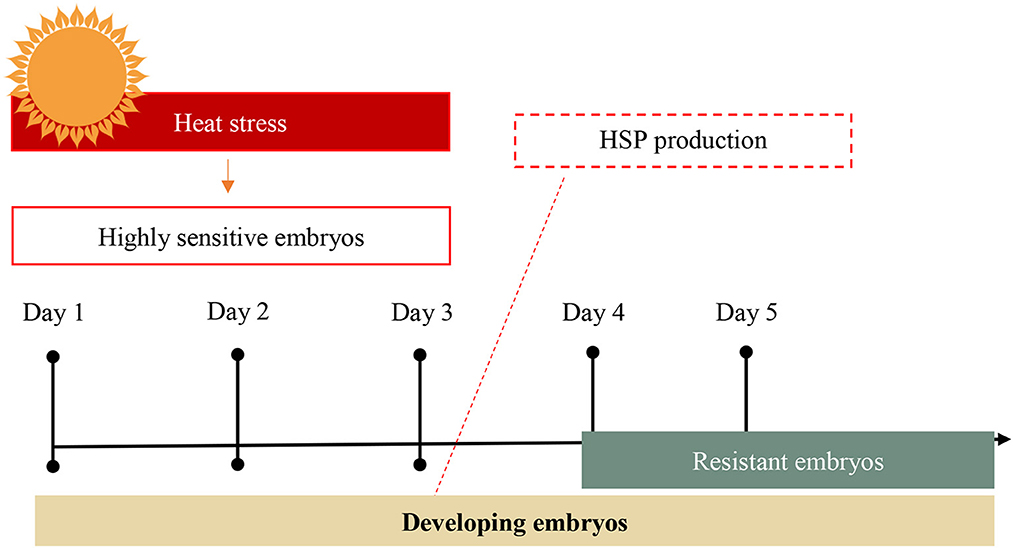
Figure 10. Effects of heat stress on bovine embryo development and heat shock protein (HSP) production (42).
In dairy cows subjected to heat stress, there is a tendency to present fertility problems. This is due to different factors, affecting from the manifestations of estrus signs, to changes in follicular function, ovulation, or even promoting embryonic death (Table 4) (72, 84, 99–103).
Effects of heat stress on female reproduction
Heat stress is defined as one of the limiting and essential factors in the dairy production sector, even though the bovine property is maintained under controlled environmental techniques. Severe heat waves in early summer can be harmful to the animal when it is not in a climate-controlled environment (104, 105). For this reason, heat stress in bovine reproduction has been widely addressed in recent decades (52, 106–114).
In this context, it is observed that heat stress has had negative consequences in terms of reproductive function. Among these effects, oocyte maturation, early embryonic development, fetal and placental growth and lactation stand out (115). It is noteworthy that the unfavorable effects are related to the situation of hyperthermia or physiological changes developed by the animal in a situation of thermal stress, making it impossible for this individual to dissipate heat to regulate body temperature (105).
The impacts of heat stress on reproductive function affect the formation and function of male and female gametes, embryonic development, as well as fetal growth and development. The proportion of these effects is greater in high producing cows than in low producing cows and non-lactating heifers. The result of this process can occur immediately or late in various reproductive tissues and reproduction processes (101, 115–118).
Effects on ovarian follicle development and dynamics
The most evident effect of heat stress that interferes with reproduction occurs through deleterious mechanisms on ovarian follicles. Heat stress tends to alter follicular growth and development in addition to affecting ovarian follicular dynamics during the estrous cycle (119–121). Follicles developed exposed to temperatures close to 40°C can be permanently damaged and become unviable (85). The identification of these damages can be expressed after a short interval or belatedly (122, 123).
Some authors emphasize that heat stress delays follicle selection and, to make matters worse, prolongs the interval of the follicular wave and follicular steroidogenesis. All of this results in suppression of large follicles, as the dominant follicle fails to exert dominance (120, 124, 125).
The effects can be evidenced through the incidence of large follicles and follicular codominance. However, there are no reports of the immediate effect of heat stress on the small, medium and large follicles present in Girolando cows (123). The period of follicular growth can occur before the antral phase (42 days), or in the primary follicle (85 days), or in the period above three estrous cycles (123).
Heat stress interferes with follicular growth and dominance in the pre-ovulatory phase. In heat-stressed cows and heifers, dominant and subordinate follicles are smaller and larger, respectively, resulting in codominance (126–129).
It is noteworthy that the follicular proportion of the first wave is similar, however the secondary wave in thermoneutral cows has a larger diameter when compared to animals under heat stress (120). On the other hand, different results were obtained in Holstein cows, in which heat stress had a permanent negative effect on the antral follicles, especially in the period between 40 and 50 days after growth (130).
Effect of heat stress on oocyte development
Oocytes are temperature-sensitive female germ cells that are subject to heat shock (131–133), remaining vulnerable to heat stress during the pre-ovulatory period (101). According to Paula Lopes et al. (133), the germinal vesicle and maturing oocytes are among the main ones affected by the adverse effects of heat stress. In this way, cows affected by heat stress have the quality of the gamete impaired, since the stressed animal cannot obtain greater production of oocytes.
Heat stress causes cellular damage to the cytoplasm and nucleus of the oocyte. In addition, it can stimulate apoptosis, compromise cytoskeleton and mitochondrial function. All this will end up harming the quality of oocyte development (132, 133).
The cytoplasm is the part most susceptible to heat shock compared to the nucleus (133), this anomaly is closely linked to changes in the amount of progesterone, LH and FSH secreted during the estrous cycle (105, 122, 134).
The reaction to heat stress in Bos taurus taurus and Bos taurus indicus cattle shows negative and late results regarding oocyte competence (123). Thus, the development of small follicles can be impaired by heat stress during the summer and lead to ovulation of immature oocytes (135).
Influence of heat stress on fertilization, embryonic development and early embryonic losses
For Hansen et al. (115), heat stress impairs oocyte quality and fertilization rate, which can result in the formation of poor quality embryos (116, 131, 132).
According to Wolfenson et al. (117), the early embryo is the most susceptible to the effects of heat stress. Thus, the deleterious effect becomes more worrying when it occurs in the first divisions of cleavage, or when part of the embryonic genome is inactive. After that, depending on the progress of its development, the bovine embryo gradually acquires greater resistance to the increase in temperature (105). Paula Lopes et al. (133) emphasize that the consequences of heat stress are less common in heat-tolerant breeds (Bos taurus indicus) when comparing them with heat-sensitive or exotic breeds.
Cows under heat stress tend to lose their embryos before completing 42 days (101, 136, 137). This can be explained by the fact that in less heat-tolerant breeds, there is a decrease in blood flow to the ovaries, causing a reduction in the supply of nutrients and hormones, which will interfere unfavorably with embryonic development. This scenario can lead to increased production of PGF2y promoting premature luteolysis and early embryonic mortality (138).
Heat stress in the male bovine
Consequences of increased testicular temperature due to climate change
The process of transpiration and respiration in cattle has the function of dissipating the heat retained in their body, but these mechanisms are not so favorable to transfer heat to the environment, thus characterizing the limit of ambient temperature as thermal stress (139).
One of the mechanisms responsible for the occurrence of testicular heat stress is the increase in ambient temperature provided by global climate change (140). Given this condition, several studies have reported the negative effects of increased environmental temperatures on male reproduction (141).
It was observed in bulls exposed to high ambient temperatures (40°C), lower sperm quality, in other words, a reduction in the percentage of motile sperm and an increase in the percentage of abnormal sperm (142–144).
In addition, there is a significant reduction in testicular blood flow during the warmer months, causing deleterious effects on seminal plasma enzymatic activity and various aspects of semen quality (145).
In a study carried out with Simmental (Bos taurus) bulls, Koivisto et al. (146) reported higher rates of sperm defects in summer compared to winter, because younger animals are more sensitive to high ambient temperatures.
These studies help to clarify why bulls more resistant to heat tend to have a higher quality of thawed semen, varying throughout the year, due to the occurrence of a high proportion of ejaculates discarded during the summer (147, 148) (Table 5).
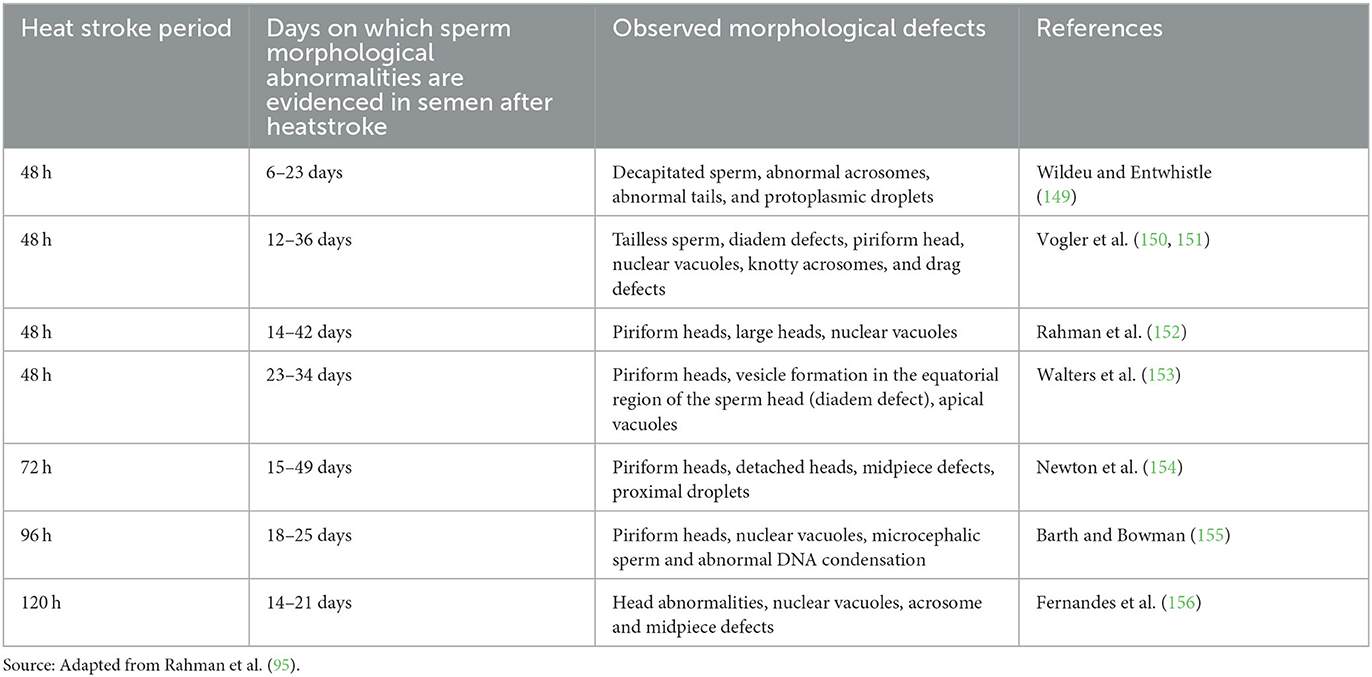
Table 5. Morphological defects observed in studies after heat stress due to scrotal isolation in cattle.
Effects of heat stress on spermatogenesis and semen quality
The direct effects of heat stress on sperm production can also impact sperm quality, through the proportion and duration of testicular heating. Sperm morphology can be altered by an increase in testicular temperature, although they may remain normal for a few days if the epididymal spermatozoa are minimally affected, followed gradually by the emergence of morphologically abnormal sperm (157).
In contrast, elevated testicular temperatures were observed in spermatozoa in the epididymis (150, 151, 158, 159), which after 2 days of scrotal isolation were adversely affected, showing reduced mobility and acrosomal integrity capacity.
As for sperm reproduction, Vogler et al. (151) report several morphological defects (Table 6) that are evidenced by the distal reflexes of the midpiece, by the proximal and distal droplets and by the knotty acrosomes that appeared when reaching the peak between 11 and 18 days post-isolation. The aforementioned team also reports the identification of microcephalic and teratoid spermatozoa, with nuclear vacuoles, pyriform heads, in addition to curled tails.
Scrotal isolation associated with dexamethasone treatment can also be used to relate the effects of testicular heating and the effect of stress on spermatogenesis in bulls, although similar results can be obtained in the types of morphological defects and in the temporal relationships of ascent and descent (155).
Mild testicular heat stress in bulls (8 h of scrotal isolation) has negative effects on the quality of frozen and thawed sperm (169). This was reported by Pérez-Crespo et al. (170) when studying the isolation of the scrotal neck in B. taurus bulls that showed an increase in scrotal-testicular temperature accompanied by an increase in the percentage of abnormalities between the head and the midpiece.
Some bull breeds, especially those crossbred between B. taurus taurus x B. taurus indicus, have no impact on semen quality during isolation from the scrotum. However, total isolation of the scrotum within 4 days is capable of reducing sperm production and quality in crossbred bulls (171).
In Nelore Bulls (B. taurus indicus), there may be a reduction in the amount of normal sperm and an increase in sperm with some type of head deficiency, mainly nuclear vacuoles and chromatin defects, during isolation from 14 to 21 days, after 5 days of total isolation of the bull scrotum (156).
On the other hand, in Brahman bulls (B. taurus indicus), submitted to 48 h of scrotal isolation, there was a tendency to decrease sperm motility. As a consequence, there was an increase in the rates of malformation of the head and cytoplasmic droplets, after 41 days of isolation (173).
In the case of mammals in the process of normal spermatogenesis, the testes need to be at room temperature, that is, in the range of 4–5°C lower than the body's core temperature (174). All this can be evidenced in most mammals, due to the location of the testes in the scrotum, therefore, outside the abdominal cavity (175).
The proportion of the vasculature and the lymphatic arrangement of the testes together with the superficial blood vessels of the scrotum seek to facilitate the removal of heat dissipation from the testes. The action of these neurons by the cold causes a reduction in the constriction of blood vessels while, on the other hand, the heat provides a vasodilation vasodilation. of these arterioles and, therefore, decreases or increases, respectively, the blood supply to the scrotum (176).
Beneath the surface of the scrotal skin is the tunica dartos, which plays an important role in thermoregulation. In this context, cutaneous muscle, specifically, a layer of smooth, thin muscle located under the tonic control of nerves corresponding to the lumbar sympathetic system that positions the scrotum towards the abdomen or further away from the abdomen in response to cold and environmental conditions (cold and hot, respectively) (113). As for the cremaster muscle, one of its contributions is to bring the testicles closer to the abdomen after contraction. Therefore, the striated nature of this muscle means that the muscle cannot sustain contraction for an extended period of time (95).
Other structures also favor the cooling of the testes, such as the vascular system. With regard to the testicular artery, one of its attributes is to transport warm blood from the body's core to the testicles. This artery is intertwined by a venous network known as the pampiniform plexus and this structure formed by the venous network plus the artery is called the testicular vascular cone (177).
Because of this characteristic vasculature, a countercurrent blood circulation system may be evident in the testes. As a result of this circulation system, arterial blood entering the testes is cooled as venous blood is eliminated by the testes (Figures 11A, B).
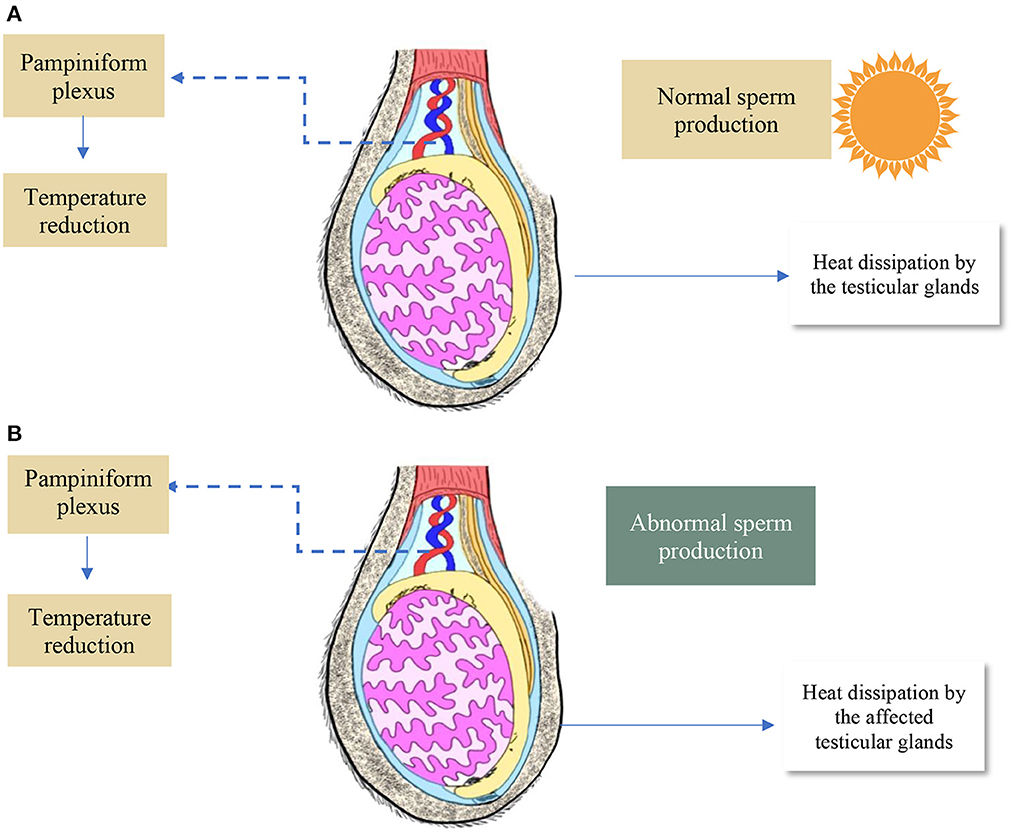
Figure 11. Bovine testis. (A) Morphological characteristics of the testis without heat stress. (B) Morphological characteristics of the testis under heat stress.
According to Makker et al. (178), in bulls, sweat glands are another important factor. They also have attributes related to the control of testicular temperature, since the density of these glands is greater in the scrotal skin than in other regions of the body and for this reason—to a certain extent—bulls that have adequate scrotal circumference may have a greater ability to cope. with heat stress to some extent.
Mechanisms of the effects of heat stress on spermatogenesis and semen quality
The temperature resistance capacity is influenced by the duration of insolation (Figure 12), according to the degeneration of the germ cells that are induced (179). In this case, the exposure of male mice to a high temperature and humidity index compromises the sperm morphology and the integrity of the plasma membrane, disfavoring sperm mortality, and may even lead to infertility (163, 165, 170, 180).
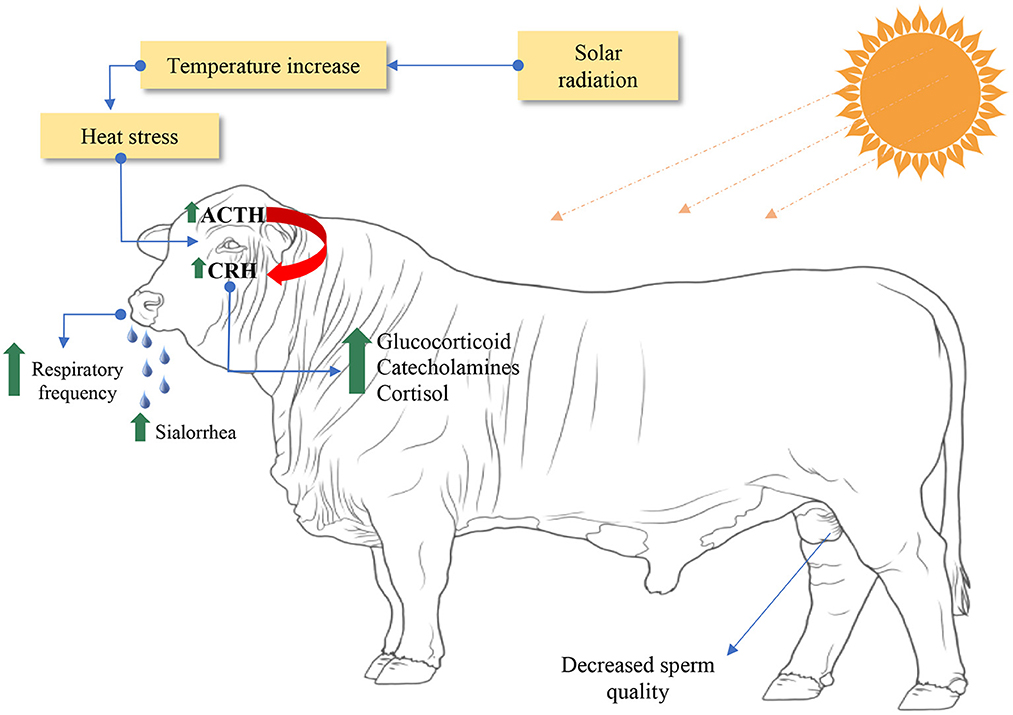
Figure 12. Illustration of the effects of heat stress on male bovine reproduction. ACTH, adrenocorticotropic hormone, or corticotropin; CRH, corticotropin releasing hormone. Adapted from Krishnan et al. (37) and Singh et al. (172).
The impacts caused by heat stress induce an increase in testicular temperature, evolving to sperm abnormalities. The appearance, proportion and severity of sperm abnormalities present in the ejaculate vary due to the intensity and duration of heat stress and the developmental stages of the affected germ cells (181).
It is noteworthy that the abnormalities are predominantly located in the sperm head, with emphasis on the presence of acrosomal defects, piriform heads, micro and macrocephalic heads (163). Sperm motility during 14–21 days can also be compromised by mild or short-term heat stress, resulting in aggravations in the spermatid and spermatocyte developmental stages (182, 183).
Final considerations
Based on the information described in the literature on animal welfare and stress in reproductive aspects, it was possible to observe that the ability of thermal stress to negatively influence ovarian and testicular activity in cattle of different breeds, reared in different environmental and climatic conditions, is evident conditions, causing detrimental effects on spermatogenesis, sperm quality, folliculogenesis, ovulation, manifestation of estrus and embryo survival. In addition, other harmful consequences resulting from heat stress have also been reported, such as the increase in embryonic mortality rates that occurred in several countries, even in temperate climates. It is suggested to condition the animals in environments with shaded areas and the use of places that allow the animals to thermoregulate efficiently.
Author contributions
Experiment design: WS, JS, and JBL-J. Experiment perform and writing—original draft: WS, JS, RC-J, ÉS, MS, RV, AS, CS, and JBL-J. Data curation: WS and JBL-J. All authors edited and approved the final manuscript.
Funding
This study was funded in part by the Federal University of Pará and Coordenação de Aperfei-çoamento de Pessoal de Nível Superior (CAPES) Brasil. This study also received financial support for the publication fee from the Pró-Reitoria de Pesquisa e Pós-Graduação (PROPESP/UFPA–Announcement-02/2023).
Acknowledgments
We would like to thank the Coordination for the Improvement of Higher Education Personnel (CAPES) – Brazil. We also thank the research group on Animal Production in the Amazon and Gaia – Livestock Health and Reproduction Research Group.
Conflict of interest
The authors declare that the research was conducted in the absence of any commercial or financial relationships that could be construed as a potential conflict of interest.
Publisher's note
All claims expressed in this article are solely those of the authors and do not necessarily represent those of their affiliated organizations, or those of the publisher, the editors and the reviewers. Any product that may be evaluated in this article, or claim that may be made by its manufacturer, is not guaranteed or endorsed by the publisher.
References
1. OIE (World Organization for Animal Health). Definição de bem-estar animal. (2019). Available online at: https://www.oie.int/en/what-we-do/standards/codes-and-manuals/terrestrial-manual-online-access/ (accessed August 15, 2022).
2. Costa PT, Silveira IDB, Silveira RF, Vaz RZ, Fernandes TA, Moreira SM, et al. Características comportamentais dos bovinos: Aspectos básicos, processo de aprendizagem e fatores que as afetam. REDVET Revista Electrónica de Veterinaria. (2017) 18:1–16.
3. Marques JA, Maggioni D, Abrahão JJS, Guilherme E, Arruda Bezerra G, Lugão SM. Comportamento de touros jovens em confinamento alojados isoladamente ou em grupo. Archivos Latino americanos de Produccinon. Animal. (2005) 13:97–102.
4. Mellor DJ, Beausoleil NJ, Littlewood KE, McLean AN, McGreevy PD, Jones B, et al. The 2020 five domains model: Including human–animal interactions in assessments of animal welfare. Animals. (2020) 10:1870. doi: 10.3390/ani10101870
5. Lemes AP, Garcia AR, Pezzopane JRM, Brandão FZ, Watanabe YF, Cooke RF, et al. (2021). Silvopastoral system is an alternative to improve animal welfare and productive performance in meat production systems. Scientific Reports. 11:14092.
6. Abdelnour SA, Abd El-Hack ME, Khafaga AF, Arif M, Taha AE, Noreldin AE. Stress biomarkers and proteomics alteration to thermal stress in ruminants: a review. J Therm Biol. (2019) 79:120–34. doi: 10.1016/j.jtherbio.2018.12.013
7. Perissinotto M, De Moura DJ. Determinação do conforto térmico de vacas leiteiras utilizando a mineração de dados. Revista Brasileira de Engenharia de Biossistemas. (2007) 1:117–26. doi: 10.18011/bioeng2007v1n2p117-126
8. Perissinotto M, Moura DJ, Cruz VF, Souza SRLD, Lima KAOD, Mendes AS. Conforto térmico de bovinos leiteiros confinados em clima subtropical e mediterrâneo pela análise de parâmetros fisiológicos utilizando a teoria dos conjuntos fuzzy. Ciência Rural. (2009) 39:1492–8. doi: 10.1590/S0103-84782009005000094
9. Bertoncelli P, Martin T, Ziech MF, Paris W, Cella P. Conforto térmico alterando a produção leiteira. Enciclopédia Biosfera. (2013) 9:17.
10. Silva WC, Silva ÉBR, Santos MRP, Junior RNCC, Barbosa AVC, Silva JAR, et al. Behavior and thermal comfort of light and dark coat dairy cows in the Eastern Amazon. Front Vet Sci. (2022) 9:1006093. doi: 10.3389/fvets.2022.1006093
11. Hooper HB, Salomão DDOS, Ayres GF, Titto CG, dos Santos RM, de Mattos Nascimento MRB. Conforto térmico de vacas leiteiras mestiças durante a inseminação e a relação com a taxa de concepção. Revista Acadêmica Ciência Animal. (2018) 16:1–10. doi: 10.7213/1981-4178.2018.161006
12. Neto OV, Bittar DY. Análise do conforto térmico e sua influência na produção e qualidade do leite em ambiente de domínio de cerrado. Pubvet. (2018) 12:147. doi: 10.22256/pubvet.v12n4a75.1-6
13. Silva JAR, Pantoja MHA, Silva WC, Almeida JCF, Noronha RDPP, Barbosa AVC, et al. Thermoregulatory reactions of female buffaloes raised in the sun and in the shade, in the climatic conditions of the rainy season of the Island of Marajó, Pará, Brazil. Front Vet Sci. (2022) 9:998544. doi: 10.3389/fvets.2022.998544
14. Rashamol V. Adaptabilidade fisiológica da pecuária ao estresse térmico: Uma revisão atualizada. J. Anim Behav Biometeorol. (2018) 6:62–71.
15. Avendaño-Reyes L. Heat stress management for milk production in arid zones. In Milk production-An up-to-date overview of animal nutrition, management and health. IntechOpen. (2012). doi: 10.5772/51299
16. Mata BC, Pires MDFÁ, Marques LCG, Porto BR, de Carvalho Junior IS. Comportamento diurno de vacas Holandesas puras por cruza em ambiente quente. Caderno de Ciências Agrárias. (2016) 8:49–56.
17. Van de Weerd H, Sandilands V. Erratum to “Bringing the issue of animal welfare to the public: A biography of Ruth Harrison (1920–2000)” Appl Anim Behav Sci. (2009) 116:306. doi: 10.1016/j.applanim.2008.10.003
18. Brambell FWR. Technical committee to enquire into the welfare of animals kept under intensive livestock husbandry systems. (1965). In: Report of the Technical Committee... Animals Kept Under Intensive Livestock Husbandry Systems. Richmond, United Kingdom: HM Stationery Office.
19. Broom DM. Indicators of poor welfare. Br Vet J. (1986) 142:524–6. doi: 10.1016/0007-1935(86)90109-0
20. Oliveira CBD, Bortoli ECD, Barcellos JOJ. Diferenciação por qualidade da carne bovina: a ótica do bem-estar animal. Ciência Rural. (2008) 38:2092–6. doi: 10.1590/S0103-84782008000700049
22. FAWC - Farm Animal Welfare Council. Farm animal welfare in Great Britain: Past, present future. London, UK: Farm Animal Welfare Council. (2009). Available online at: https://www.gov.uk/government/uploads/system/uploads/attachment_data/file/319292/Farm_Animal_Welfare_in_Great_Britain_-_Past__Present_and_Future.pdf (accessed 28 March, 2009).
23. Armstrong D. Heat stress interaction with shade and cooling. J Dairy Sci. (1994) 77:2044–50. doi: 10.3168/jds.S0022-0302(94)77149-6
24. Dias TP, Pereira AM, da Silva Rocha J, de Sousa Silva A, Honorato-Sampaio KHS, Costa APR. Efeito da época do ano e período do dia sobre os parâmetros fisiológicos de ovelhas Morada Nova na Microrregião do Alto Médio Gurguéia. J Health Sci. (2013) 15:4.
25. Mellor DJ, Reid CSW. Concepts of Animal Well-Being and Predicting the Impact of Procedures on Experimental Animals. (1994).
26. Anderson C, Yngvesson J, Boissy A, Uvnäs-Moberg K, Lidfors L. Behavioural expression of positive anticipation for food or opportunity to play in lambs. Behav Proc. (2015) 113:152–8. doi: 10.1016/j.beproc.2015.02.003
27. Broom DM. Welfare of animals: behavior as a basis for decisions. In: Breed MD, Moore J (eds). Encyclopedia of Animal Behavior. Oxford: Academic Press. (2010). p. 580–584. doi: 10.1016/B978-0-08-045337-8.00080-2
28. Broom DM. Coping, stress and welfare. In: Coping With Challenge: Welfare in Animals Including Humans. (2001) p. 1–9.
29. Mellor DJ, Stafford KJ. Animal welfare implications of neonatal mortality and morbidity in farm animals. Vet J. (2004) 168:118–33. doi: 10.1016/j.tvjl.2003.08.004
30. Kirkden RD, Broom DM, Andersen IL. Invited review: piglet mortality: management solutions. J Animal Sci. 91:3361–89. doi: 10.2527/jas.2012-5637
31. Broom DM, Corke MJ. Effects of disease on farm animal welfare. Acta Veterinaria Brno. (2002) 71:133–6. doi: 10.2754/avb200271010133
32. Summer A, Lora I, Formaggioni P, Gottardo F. Impact of heat stress on milk and meat production. Animal Front. (2019) 9:39–46. doi: 10.1093/af/vfy026
33. Samal L. Heat stress in dairy cows-reproductive problems and control measures. Int J Livestock Res. (2013) 3:14–23.
34. Mitlöhner FM, Morrow JL, Dailey JW, Wilson SC, Galyean ML, Miller MF, et al. Shade and water misting effects on behavior, physiology, performance, and carcass traits of heat-stressed feedlot cattle. J Animal Sci. (2001) 79:232735. doi: 10.2527/2001.7992327x
35. Ravagnolo O, Misztal I, Hoogenboom G. Genetic component of heat stress in dairy cattle, development of heat index function. J Dairy Sci. (2000) 83:2120–5. doi: 10.3168/jds.S0022-0302(00)75094-6
36. Sammad A, Umer S, Shi R, Zhu H, Zhao X, Wang Y. Dairy cow reproduction under the influence of heat stress. J Anim Physiol Anim Nutr. (2020) 104:978–86. doi: 10.1111/jpn.13257
37. Krishnan G, Bagath M, Pragna P, Vidya MK, Aleena J, Archana PR, et al. Mitigation of the heat stress impact in livestock reproduction. Theriogenology. (2017) 8:8–9. doi: 10.5772/intechopen.69091
38. Roth Z, Aroyo A, Yavin S, Arav A. The antioxidant epigallocatechin gallate (EGCG) moderates the deleterious effects of maternal hyperthermia on follicle-enclosed oocytes in mice. Theriogenology. (2008) 70:887–97. doi: 10.1016/j.theriogenology.2008.05.053
39. Lawrence JL, Payton RR, Godkin JD, Saxton AM, Schrick FN, Edwards JL. Retinol improves development of bovine oocytes compromised by heat stress during maturation. J Dairy Sci. (2004) 87:2449–54. doi: 10.3168/jds.S0022-0302(04)73368-8
40. López-Gatius F. Is fertility declining in dairy cattle?: a retrospective study in northeastern Spain. Theriogenology. (2003) 60:89–99. doi: 10.1016/S0093-691X(02)01359-6
41. López-Gatius F, Santolaria P, Martino A, Delétang F, De Rensis F. The effects of GnRH treatment at the time of AI and 12 days later on reproductive performance of high producing dairy cows during the warm season in northeastern Spain. Theriogenology. (2006) 65:820–30. doi: 10.1016/j.theriogenology.2005.07.002
42. Ealy AD, Drost M, Hansen PJ. Developmental changes in embryonic resistance to adverse effects of maternal heat stress in cows. J Dairy Sci. (1993) 76:2899–905. doi: 10.3168/jds.S0022-0302(93)77629-8
43. Broom DM. Welfare assessment and welfare problem areas during handling and transport. In:Grandin T, , editor, Livestock Handling and Transport. Wallingford: CAB International. (2000). doi: 10.1079/9780851994093.0043
44. Kovács L, Kézér FL, Póti P, Boros N, Nagy K. Upper critical temperature-humidity index for dairy calves based on physiological stress variables. J Dairy Sci. (2020) 103:2707–10. doi: 10.3168/jds.2019-17459
45. Botreau R, Veissier I, Butterworth A, Bracke MB, Keeling LJ. Definition of criteria for overall assessment of animal welfare. Animal Welfare-Potters Bar Then Wheathampstead. (2007) 16:225. doi: 10.1017/S0962728600031390
46. Blokhuis HJ. International cooperation in animal welfare: the Welfare Quality® project. Acta veterinaria scandinavica. (2008) 50:1–5. doi: 10.1186/1751-0147-50-S1-S10
47. Velarde A, Dalmau A. Animal welfare assessment at slaughter in Europe: moving from inputs to outputs. Meat Sci. (2012) 92:244–51. doi: 10.1016/j.meatsci.2012.04.009
48. Mellor DJ. Operational details of the five domains model and its key applications to the assessment and management of animal welfare. Animals. (2017) 7:60. doi: 10.3390/ani7080060
49. Adamczyk K, Pokorska J, Makulska J, Earley B, Mazurek M. Genetic analysis and evaluation of behavioural traits in cattle. Livestock Sci. (2013) 154:1–12. doi: 10.1016/j.livsci.2013.01.016
50. Bouissou MF, Boissy A, Neindre PL, Veissier I. The social behaviour of cattle. In: Social Behaviour in Farm Animals. Wallingford UK: cabi Publishing. (2001) p. 113–145. doi: 10.1079/9780851993973.0113
51. Albright JL, Arave CW. The Behaviour of Cattle. Wallingford, United Kingdom: CAB International. (1997). doi: 10.1079/9780851991962.0000
52. Lucy MC. Stress, strain, and pregnancy outcome in postpartum cows. Animal Reprod. (2019) 16:455–64. doi: 10.21451/1984-3143-AR2019-0063
53. Green AC, Lidfors LM, Lomax S, Favaro L, Clark CE. Vocal production in postpartum dairy cows: temporal organization and association with maternal and stress behaviors. J Dairy Sci. (2021) 104:826–38. doi: 10.3168/jds.2020-18891
54. Ludtke CB, Ciocca JRP, Barbalho PC, Dandim T, Vilela JA, Ferrarini C. Abate humanitários de bovinos. São Paulo: World Animal Protection São Paulo. (2015) p. 136.
55. Keeling LJ, Winckler C, Hintze S, Forkman B. Towards a positive welfare protocol for cattle: a critical review of indicators and suggestion of how we might proceed. Front Animal Sci. (2021) 2:70. doi: 10.3389/fanim.2021.753080
56. Broom DM, Molento CFM. Bem-estar animal: Conceito e Questões relacionadas revisão. Arch Vet Sci. (2004) 9:2. doi: 10.5380/avs.v9i2.4057
57. Godoy LD, Rossignoli MT, Delfino-Pereira P, Garcia-Cairasco N, de Lima Umeoka EH. A comprehensive overview on stress neurobiology: basic concepts and clinical implications. Front Behav Neurosci. (2018) 12:127. doi: 10.3389/fnbeh.2018.00127
58. Terlouw EMC, Arnould C, Aupérin B, Berri C, Le Bihan-Duval E, Deiss V, et al. Pre-slaughter conditions, animal stress and welfare: current status and possible future research. Animal. (2008) 2:1501–17. doi: 10.1017/S1751731108002723
59. Njisane YZ, Muchenje V. Farm to abattoir conditions, animal factors and their subsequent effects on cattle behavioural responses and beef quality—a review. Asian-Australas J Anim Sci. (2017) 30:755. doi: 10.5713/ajas.16.0037
60. Zulkifli I, Goh YM, Norbaiyah B, Sazili AQ, Lotfi M, Soleimani AF, et al. Changes in blood parameters and electroencephalogram of cattle as affected by different stunning and slaughter methods in cattle. Animal Prod Sci. (2013) 54:187–93. doi: 10.1071/AN12128
61. Šímová V, Večerek V, Passantino A, Voslárová E. Pre-transport factors affecting the welfare of cattle during road transport for slaughter–a review. Acta Veterinaria Brno. (2016) 85:303–18. doi: 10.2754/avb201685030303
62. Dikmen S, Hansen PJ. Is the temperature-humidity index the best indicator of heat stress in lactating dairy cows in a subtropical environment? J Dairy Sci. (2009) 92:109–16. doi: 10.3168/jds.2008-1370
63. Godde C, Mason-D'Croz D, Mayberry D, Thornton PK, Herrero M. Risk of climate-related impacts on the livestock sector: A review of the evidence. Global Food Security. (2021) 28:100488. doi: 10.1016/j.gfs.2020.100488
64. Scharf B, Wax LE, Aiken GE, Spiers DE. Regional differences in sweat rate response of steers to short-term heat stress. Int J Biometeorol. (2008) 52:725–32. doi: 10.1007/s00484-008-0165-4
65. Young BA, Hall AB. Heat load in cattle in the Australian environment. Australian Beef . (1993) 143–8.
66. Young BA. Implications of excessive heat load to the welfare of cattle in feedlots. In: Recent Advances in Animal Nutrition in Australia. (1993) p. 45–50.
67. Farooq U, Samad HA, Shehzad F, Qayyum A. Physiological responses of cattle to heat stress. World Appl Sci J. (2010) 8:38–43.
68. Berman A. Estimates of heat stress relief needs for Holstein dairy cows. J Anim Sci. (2005) 83:1377–84. doi: 10.2527/2005.8361377x
69. Collier RJ, Baumgard LH, Zimbelman RB, Xiao Y. Heat stress: physiology of acclimation and adaptation. Animal Front. (2019) 9:12–9. doi: 10.1093/af/vfy031
70. Kadzere CT, Murphy MR, Silanikove N, Maltz E. Heat stress in lactating dairy cows: a review. Livestock Prod Sci. (2002) 77:59–91. doi: 10.1016/S0301-6226(01)00330-X
71. Das R, Sailo L, Verma N, Bharti P, Saikia J. Impact of heat stress on health and performance of dairy animals: a review. Vet World. (2016) 9:260. doi: 10.14202/vetworld.2016.260-268
72. Polsky LB, Madureira AM, Drago Filho EL, Soriano S, Sica AF, Vasconcelos JL, et al. Association between ambient temperature and humidity, vaginal temperature, and automatic activity monitoring on induced estrus in lactating cows. J Dairy Sci. (2017) 100:8590–601. doi: 10.3168/jds.2017-12656
73. Herbut P, Angrecka S, Godyń D, Hoffmann G. The physiological and productivity effects of heat stress in cattle–a review. Ann Anim Sci. (2019) 19:579–93. doi: 10.2478/aoas-2019-0011
74. Hahn GL. Dynamic responses of cattle to thermal heat loads. J Animal Sci. (1999) 77:10–20. doi: 10.2527/1997.77suppl_210x
75. Gates R, Brown-Brandt T, Yanagi T, Xin H, Bucklin R, Ross G. A new telemetry system for measuring core body temperature in livestock and poultry. Appl Eng Agric. (2003) 19:583–9. doi: 10.13031/2013.15316
76. Brown-Brandl TM, Eigenberg RA, Nienaber JA, Hahn GL. Dynamic response indicators of heat stress in shaded and non-shaded feedlot cattle, part 1: analyses of indicators. Biosyst Engineer. (2005) 90:451–62. doi: 10.1016/j.biosystemseng.2004.12.006
77. Brown-Brandl TM, Eigenberg RA, Nienaber JA. Heat stress risk factors of feedlot heifers. Livest Sci. (2006) 105:57–68. doi: 10.1016/j.livsci.2006.04.025
78. Eigenberg RA, Brown-Brandl TM, Nienaber JA, Hahn GL. Dynamic response indicators of heat stress in shaded and non-shaded feedlot cattle, part 2: predictive relationships. Biosyst Engineer. (2005) 91:111–8. doi: 10.1016/j.biosystemseng.2005.02.001
79. Mader TL, Davis MS, Brown-Brandl T. Environmental factors influencing heat stress in feedlot cattle. J Anim Sci. (2006) 84:712–9. doi: 10.2527/2006.843712x
80. Gaughan JB, Mader TL, Holt SM, Sullivan ML, Hahn GL. Assessing the heat tolerance of 17 beef cattle genotypes. Int J Biometeorol. (2010) 54:617–27. doi: 10.1007/s00484-009-0233-4
81. Lees AM, Sejian V, Wallage AL, Steel CC, Mader TL, Lees JC, et al. The impact of heat load on cattle. Animals. (2019) 9:322. doi: 10.3390/ani9060322
82. Edwards-Callaway LN, Cramer MC, Cadaret CN, Bigler EJ, Engle TE, Wagner JJ, et al. Impacts of shade on cattle well-being in the beef supply chain. J Animal Sci. (2021) 99:skaa375 doi: 10.1093/jas/skaa375
84. Collier RJ, Beede DK, Thatcher WW, Israel LA, Wilcox CJ. Influences of environment and its modification on dairy animal health and production. J Dairy Sci. (1982) 65:2213–27. doi: 10.3168/jds.S0022-0302(82)82484-3
85. Wilson SJ, Marion RS, Spain JN, Spiers DE, Keisler DH, Lucy MC. Effects of controlled heat stress on ovarian function of dairy cattle. 1 Lactating cows. J Dairy Sci. (1998) 81:2124–31. doi: 10.3168/jds.S0022-0302(98)75789-3
86. Schüller LK, Michaelis I, Heuwieser W. Impact of heat stress on estrus expression and follicle size in estrus under field conditions in dairy cows. Theriogenology. (2017) 102:48–53. doi: 10.1016/j.theriogenology.2017.07.004
87. Alves BG, Alves KA, Lúcio AC, Martins MC, Silva TH, Alves BG, et al. Ovarian activity and oocyte quality associated with the biochemical profile of serum and follicular fluid from Girolando dairy cows postpartum. Anim Reprod Sci. (2014) 146:117–25. doi: 10.1016/j.anireprosci.2014.02.019
88. Roth Z, Wolfenson D. Comparing the effects of heat stress and mastitis on ovarian function in lactating cows: basic and applied aspects. Domest Anim Endocrinol. (2016) 56:S218–27. doi: 10.1016/j.domaniend.2016.02.013
89. Silva CF, Sartorelli ES, Castilho ACS, Satrapa RA, Puelker RZ, Razza EM, et al. Effects of heat stress on development, quality and survival of Bos indicus and Bos taurus embryos produced in vitro. Theriogenology. (2013) 79:351–7. doi: 10.1016/j.theriogenology.2012.10.003
90. Maya-Soriano MJ, Taberner E, López-Béjar M. Retinol improves in vitro oocyte nuclear maturation under heat stress in heifers. Zygote. (2013) 21:377–84. doi: 10.1017/S0967199412000135
91. Lussier JG, Matton P, Dufour JJ. Growth rates of follicles in the ovary of the cow. Reproduction. (1987) 81:301–7. doi: 10.1530/jrf.0.0810301
92. Roth Z, Arav A, Bor A, Zeron Y, Braw-Tal R, Wolfenson D. Improvement of quality of oocytes collected in the autumn by enhanced removal of impaired follicles from previously heat-stressed cows. Reproduction-Cambridge. (2001) 122:737–44. doi: 10.1530/rep.0.1220737
93. Malama E, Zeron Y, Janett F, Siuda M, Roth Z, Bollwein H. Use of computer-assisted sperm analysis and flow cytometry to detect seasonal variations of bovine semen quality. Theriogenology. (2017) 87:79–90. doi: 10.1016/j.theriogenology.2016.08.002
94. Sabés-Alsina M, Johannisson A, Lundeheim N, Lopez-Bejar M, Morrell JM. Effects of season on bull sperm quality in thawed samples in northern Spain. Vet Record. (2017) 180:251–251. doi: 10.1136/vr.103897
95. Rahman MB, Schellander K, Luceño NL, Van Soom A. Heat stress responses in spermatozoa: Mechanisms and consequences for cattle fertility. Theriogenology. (2018) 113:102–12. doi: 10.1016/j.theriogenology.2018.02.012
96. Lucio AC, Alves BG, Alves KA, Martins MC, Braga LS, Miglio L, et al. Selected sperm traits are simultaneously altered after scrotal heat stress and play specific roles in in vitro fertilization and embryonic development. Theriogenology. (2016) 86:924–33. doi: 10.1016/j.theriogenology.2016.03.015
97. Edwards JL, Hansen PJ. Differential responses of bovine oocytes and preimplantation embryos to heat shock. Mol Reprod Dev. (1997) 46:138–45. doi: 10.1002/(SICI)1098-2795(199702)46:2<138::AID-MRD4>3.0.CO;2-R
98. Negrón-Pérez VM, Fausnacht DW, Rhoads ML. Invited review: management strategies capable of improving the reproductive performance of heat-stressed dairy cattle. J Dairy Sci. (2019) 102:10695–710. doi: 10.3168/jds.2019-16718
99. Putney DJ, Drost M, Thatcher WW. Embryonic development in superovulated dairy cattle exposed to elevated ambient temperatures between days 1 to 7 post insemination. Theriogenology. (1988) 30:195–209. doi: 10.1016/0093-691X(88)90169-0
100. Wolfenson D, Lew BJ, Thatcher WW, Graber Y, Meidan R. Seasonal acute heat stress effects on steroid production by dominant follicles in cows. Anim Reprod Sci. (1997) 47:9–19. doi: 10.1016/S0378-4320(96)01638-7
101. De Rensis F, Scaramuzzi RJ. Heat stress and seasonal effects on reproduction in the dairy cow—a review. Theriogenology. (2003) 60:1139–51. doi: 10.1016/S0093-691X(03)00126-2
102. West JW. Effects of heat-stress on production in dairy cattle. J Dairy Sci. (2003) 86:2131–44. doi: 10.3168/jds.S0022-0302(03)73803-X
103. Schüller LK, Burfeind O, Heuwieser W. Impact of heat stress on conception rate of dairy cows in the moderate climate considering different temperature–humidity index thresholds, periods relative to breeding, and heat load indices. Theriogenology. (2014) 81:1050–7. doi: 10.1016/j.theriogenology.2014.01.029
104. Hansen PJ. Exploitation of genetic and physiological determinants of embryonic resistance to elevated temperature to improve embryonic survival in dairy cattle during heat stress. Theriogenology. (2007) 68:S242–9. doi: 10.1016/j.theriogenology.2007.04.008
105. Hansen PJ. Effects of heat stress on mammalian reproduction. Philos Trans R Soc. (2009) 364:3341–50. doi: 10.1098/rstb.2009.0131
106. Sage TL, Bagha S, Lundsgaard-Nielsen V, Branch HA, Sultmanis S, Sage RF. The effect of high temperature stress on male and female reproduction in plants. Field Crops Res. (2015) 182:30–42. doi: 10.1016/j.fcr.2015.06.011
107. Dash S, Chakravarty AK, Singh A, Upadhyay A, Singh M, Yousuf S. Effect of heat stress on reproductive performances of dairy cattle and buffaloes: a review. Vet World. (2016) 9:235. doi: 10.14202/vetworld.2016.235-244
108. Roth Z. Cooling is the predominant strategy to alleviate the effects of heat stress on dairy cows. Reprod Domest Anim. (2022) 57:16–22. doi: 10.1111/rda.13765
109. Hansen PJ. Reproductive physiology of the heat-stressed dairy cow: implications for fertility and assisted reproduction. Animal Reprod. (2019) 16:497–507. doi: 10.21451/1984-3143-AR2019-0053
110. Wolfenson D, Roth Z. Impact of heat stress on cow reproduction and fertility. Animal Front. (2019) 9:32–8. doi: 10.1093/af/vfy027
111. Gernand E, König S, Kipp C. Influence of on-farm measurements for heat stress indicators on dairy cow productivity, female fertility, and health. J Dairy Sci. (2019) 102:6660–71. doi: 10.3168/jds.2018-16011
112. Fathoni A, Boonkum W, Chankitisakul V, Duangjinda M. An appropriate genetic approach for improving reproductive traits in crossbred thai–holstein cattle under heat stress conditions. Vet Sci. (2022) 9:163. doi: 10.3390/vetsci9040163
113. Morrell JM. Heat stress and bull fertility. Theriogenology. (2020) 153:62–7. doi: 10.1016/j.theriogenology.2020.05.014
114. Gaskins AJ, Mínguez-Alarcón L, VoPham T, Hart JE, Chavarro JE, Schwartz J, et al. Impact of ambient temperature on ovarian reserve. Fertil Steril. (2021) 116:1052–60. doi: 10.1016/j.fertnstert.2021.05.091
115. Hansen PJ, Drost M, Rivera RM, Paula-Lopes FF, Al-Katanani YM, Krininger CE III, et al. Adverse impact of heat stress on embryo production: causes and strategies for mitigation. Theriogenology. (2001) 55:91–103. doi: 10.1016/S0093-691X(00)00448-9
116. Rivera RM, Hansen PJ. Development of cultured bovine embryos after exposure to high temperatures in the physiological range. Reproduction-Cambridge. (2001) 121:107–15. doi: 10.1530/rep.0.1210107
117. Wolfenson D, Roth Z, Meidan R. Impaired reproduction in heat-stressed cattle: basic and applied aspects. Anim Reprod Sci. (2000) 60:535–47. doi: 10.1016/S0378-4320(00)00102-0
118. Barnabás B, Jäger K, Fehér A. The effect of drought and heat stress on reproductive processes in cereals. Plant, Cell Environ. (2008) 31:11–38. doi: 10.1111/j.1365-3040.2007.01727.x
119. Nardone A, Ronchi B, Lacetera N, Ranieri MS, Bernabucci U. Effects of climate changes on animal production and sustainability of livestock systems. Livest Sci. (2010) 130:57–69. doi: 10.1016/j.livsci.2010.02.011
120. Badinga L, Thatcher WW, Diaz T, Drost M, Wolfenson D. Effect of environmental heat stress on follicular development and steroidogenesis in lactating Holstein cows. Theriogenology. (1993) 39:797–810. doi: 10.1016/0093-691X(93)90419-6
121. Wolfenson D, Thatcher WW, Badinga L, Savio JD, Meidan R, Lew BJ, et al. Effect of heat stress on follicular development during the estrous cycle in lactating dairy cattle. Biol Reprod. (1995) 52:1106–13. doi: 10.1095/biolreprod52.5.1106
122. Roth Z, Meidan R, Shaham-Albalancy A, Wolfenson D. Immediate Delayed Effects of Heat Stress on Follicular Development and Function in Lactating Cows Annual Meeting. Nashville: American Society of Animal Science. (1997) p. 367.
123. Roth Z, Meidan R, Braw-Tal R, Wolfenson D. Immediate delayed effects of heat stress on follicular development and its association with plasma FSH and inhibin concentration in cows. J Reprod Fertil. (2000) 120:83–90. doi: 10.1530/reprod/120.1.83
124. Torres-Júnior JDS, De FA Pires M, De Sa WF, Ferreira ADM, Viana JHM, Camargo LSDA, et al. Effect of maternal heat-stress on follicular growth and oocyte competence in Bos indicus cattle. Theriogenology. (2008) 69:155–66. doi: 10.1016/j.theriogenology.2007.06.023
125. Roth Z, Meidan R, Shaham-Albalancy A, Braw-Tal R, Wolfenson D. Delayed effect of heat stress on steroid production in medium-sized and preovulatory bovine follicles. Reproduction-Cambridge. (2001) 121:745–51. doi: 10.1530/rep.0.1210745
126. Roth Z. Heat stress, the follicle, and its enclosed oocyte: mechanisms and potential strategies to improve fertility in dairy cows. Reprod Domest Anim. (2008) 43:238–44. doi: 10.1111/j.1439-0531.2008.01168.x
127. Sartori R, Barros CM. Reproductive cycles in Bos indicus cattle. Anim Reprod Sci. (2011) 124:244–50. doi: 10.1016/j.anireprosci.2011.02.006
128. López-Gatius F, López-Béjar M, Fenech M, Hunter RHF. Ovulation failure and double ovulation in dairy cattle: risk factors and effects. Theriogenology. (2005) 63:1298–307. doi: 10.1016/j.theriogenology.2004.06.010
129. Ahmad Para I, Ahmad Dar P, Ahmad Malla B, Punetha M, Rautela A, Maqbool I, et al. Impact of heat stress on the reproduction of farm animals and strategies to ameliorate it. Biol Rhythm Res. (2020) 51:616–32. doi: 10.1080/09291016.2018.1548870
130. Lopes FFDP, Lima RS, Risolia PHB, Ispada J, Assumpção MEO, Visintin JA. Heat stress induced alteration in bovine oocytes: functional and cellular aspects. Animal Reprod. (2012) 9:395–403.
131. Kadokawa H, Sakatani M, Hansen PJ. Perspectives on improvement of reproduction in cattle during heat stress in a future Japan. Animal Sci J. (2012) 83:439–45. doi: 10.1111/j.1740-0929.2012.01011.x
132. Cushman RA. Physiology endocrinology symposium: the current status of heat shock in early embryonic survival and reproductive efficiency. J Anim Sci. (2013) 91:1141–2. doi: 10.2527/jas.2013-6231
133. Paula-Lopes FF, Lima RSD, Satrapa RA, Barros CM. Physiology endocrinology symposium: Influence of cattle genotype (Bos indicus vs. Bos taurus) on oocyte and preimplantation embryo resistance to increased temperature. J Animal Sci. (2013) 91:1143–53. doi: 10.2527/jas.2012-5802
134. Ronchi B, Stradaioli G, Supplizi AV, Bernabucci U, Lacetera N, Accorsi PA, et al. Influence of heat stress or feed restriction on plasma progesterone, oestradiol-17β, LH, FSH, prolactin and cortisol in Holstein heifers. Livestock Prod Sci. (2001) 68:231–41. doi: 10.1016/S0301-6226(00)00232-3
135. Howell JL, Fuquay JW, Smith AE. Corpus luteum growth and function in lactating Holstein cows during spring and summer. J Dairy Sci. (1994) 77:735–9. doi: 10.3168/jds.S0022-0302(94)77007-7
136. Vasconcelos JLM, Silcox RW, Lacerda JA, Pursley JR, Wiltbank MC. Pregnancy rate, pregnancy loss, and response to head stress after AI at 2 different times from ovulation in dairy cows. Biol Reprod. (1997) 56:230–230.
137. Ambrose JD, Drost M, Monson RL, Rutledge JJ, Leibfried-Rutledge ML, Thatcher MJ, et al. Efficacy of timed embryo transfer with fresh and frozen in vitro produced embryos to increase pregnancy rates in heat-stressed dairy cattle. J Dairy Sci. (1999) 82:2369–76. doi: 10.3168/jds.S0022-0302(99)75487-1
138. Jordan ER. Effects of heat stress on reproduction. J Dairy Sci. (2003) 86:E104–14. doi: 10.3168/jds.S0022-0302(03)74043-0
139. Wettemann RP, Boehmer BH. Influence of heat stress on male fertility. In: Proc Appl Reprod. Strateg. Beef Cattle. Stillwater, OK: K-State Res. Extensio, 257e63. (2014).
140. Shahat AM, Rizzoto G, Kastelic JP. Amelioration of heat stress-induced damage to testes and sperm quality. Theriogenology. (2020) 158:84–96. doi: 10.1016/j.theriogenology.2020.08.034
141. Capela L, Leites I, Romão R, Lopes-da-Costa L, Pereira, R. M. L. N. Impact of Heat Stress on Bovine Sperm Quality and Competence. Animals. (2022) 12:975. doi: 10.3390/ani12080975
142. Gloria A, Candeloro L, Wegher L, Robbe D, Carluccio A, Contri A. Environmental temperature and relative humidity differently affect the sperm characteristics in Brown Swiss and Belgian Blue bulls. Int J Biometeorol. (2021) 65:2189–99. doi: 10.1007/s00484-021-02184-z
143. Skinner JD, Louw GN. Heat stress and spermatogenesis in Bos indicus and Bos taurus cattle. J Appl Physiol. (1966) 21:1784–90. doi: 10.1152/jappl.1966.21.6.1784
144. Meyerhoeffer DC, Wettemann RP, Coleman SW, Wells ME. Reproductive criteria of beef bulls during and after exposure to increased ambient temperature. J Anim Sci. (1985) 60:352–7. doi: 10.2527/jas1985.602352x
145. Hedia MG, El-Belely MS, Ismail ST, El-Maaty AMA. Seasonal changes in testicular ultrasonogram pixel-intensity and their association with semen characteristics in rams. Asian Pacific J Reprod. (2020) 9:49. doi: 10.4103/2305-0500.275635
146. Koivisto MB, Costa MTA, Perri SHV, Vicente WRR. The effect of season on semen characteristics and freezability in Bos indicus and Bos taurus bulls in the southeastern region of Brazil. Reprod Domest Anim. (2009) 44:587–92. doi: 10.1111/j.1439-0531.2008.01023.x
147. Nichi M, Bols PEJ, Züge RM, Barnabe VH, Goovaerts IGF, Barnabe RC, et al. Seasonal variation in semen quality in Bos indicus and Bos taurus bulls raised under tropical conditions. Theriogenology. (2006) 66:822–8. doi: 10.1016/j.theriogenology.2006.01.056
148. Balić IM, Milinković-Tur S, SamardŽija M, Vince S. Effect of age and environmental factors on semen quality, glutathione peroxidase activity and oxidative parameters in simmental bulls. Theriogenology. (2012) 78:423–31. doi: 10.1016/j.theriogenology.2012.02.022
149. Wildeus S, Entwistle KW. A quantitative histological study of testicular and epididymal development in Bos indicus cross bulls. Anim Reprod Sci. (1983) 6:1–10. doi: 10.1016/0378-4320(83)90002-7
150. Vogler CJ, Saacke RG, Bame JH, Dejarnette JM, McGilliard ML. Effects of scrotal insulation on viability characteristics of cryopreserved bovine semen. J Dairy Sci. (1991) 74:3827–35. doi: 10.3168/jds.S0022-0302(91)78575-5
151. Vogler AP, Desalle R, Assmann T, Knisley CB, Schultz TD. Molecular population genetics of the endangered tiger beetle Cicindela dorsalis (Coleoptera: Cicindelidae). Ann Entomol Soc Am. (1993) 86:142–52. doi: 10.1093/aesa/86.2.142
152. Rahman ZU, Khan S, Bhatti SA, Iqbal J, Iqbal A. Factors affecting first lactation performance of Sahiwal cattle in Pakistan. Arch Animal Breed. (2008) 51:305–17. doi: 10.5194/aab-51-305-2008
153. Walters AH, Eyestone WE, Saacke RG, Pearson RE, Gwazdauskas FC. Sperm morphology and preparation method affect bovine embryonic development. J Androl. (2004) 25:554–63. doi: 10.1002/j.1939-4640.2004.tb02826.x
154. Newton LD, Kastelic JP, Wong B, Van der Hoorn F, Thundathil J. Elevated testicular temperature modulates expression patterns of sperm proteins in Holstein bulls. Mol Reprod Dev. (2009) 76:109–18. doi: 10.1002/mrd.20934
155. Barth AD, Bowman PA. The sequential appearance of sperm abnormalities after scrotal insulation or dexamethasone treatment in bulls. Can Vet J. (1994) 35:93.
156. Fernandes CE, Dode MAN, Pereira D, Silva AEDF. Effects of scrotal insulation in Nellore bulls (Bos taurus indicus) on seminal quality and its relationship with in vitro fertilizing ability. Theriogenology. (2008) 70:1560–8. doi: 10.1016/j.theriogenology.2008.07.005
157. Barth AD, Oko RJ. Abnormal Morphology of Bovine Spermatozoa. Iowa: Iowa State University Press. (1989).
158. Mieusset R, Casares PQ, Partida LS, Sowerbutts SF, Zupp JL, Setchell BP. Effects of heating the testes and epididymides of rams by scrotal insulation on fertility and embryonic mortality in ewes inseminated with frozen semen. Reproduction. (1992) 94:337–43. doi: 10.1530/jrf.0.0940337
159. Kastelic JP, Cook RB, Coulter GH. Contribution of the scrotum, testes, and testicular artery to scrotal/testicular thermoregulation in bulls at two ambient temperatures. Anim Reprod Sci. (1997) 45:255–61. doi: 10.1016/S0378-4320(96)01587-4
160. Al-Kanaan A, König S, Brügemann K. Effects of heat stress on semen characteristics of Holstein bulls estimated on a continuous phenotypic and genetic scale. Livest Sci. (2015) 177:15–24. doi: 10.1016/j.livsci.2015.04.003
161. Valeanu S, Johannisson A, Lundeheim N, Morrell JM. Seasonal variation in sperm quality parameters in Swedish red dairy bulls used for artificial insemination. Livest Sci. (2015) 173:111–8. doi: 10.1016/j.livsci.2014.12.005
162. Sharma M, Yaqoob B, Singh A, Sharma N, Rawat S. Effect of temperature humidity index on semen quality of bovine bull. Int J Curr Microb Appl Sci. (2017) 6:1822–30. doi: 10.20546/ijcmas.2017.612.206
163. Sharma M, Bhat Y, Sharma N, Singh A. Comparative study of seasonal variation in semen characteristics of buffalo bull. J Entomol Zool Stud. (2018) 6:52–109.
164. Sabés-Alsina M, Lundeheim N, Johannisson A, López-Béjar M, Morrell JM. Relationships between climate and sperm quality in dairy bull semen: a retrospective analysis. J Dairy Sci. (2019) 102:5623–33. doi: 10.3168/jds.2018-15837
165. Garcia-Oliveros LN, de Arruda RP, Batissaco L, Gonzaga VHG, Nogueira VJM, Florez-Rodriguez SA, et al. Heat stress effects on bovine sperm cells: a chronological approach to early findings. Int J Biometeorol. (2020) 64:1367–78. doi: 10.1007/s00484-020-01917-w
166. Luceño-Moreno L, Talavera-Velasco B, García-Albuerne Y, Martín-García J. Symptoms of posttraumatic stress, anxiety, depression, levels of resilience and burnout in Spanish health personnel during the COVID-19 pandemic. Int J Environ Res Public Health. (2020) 17:5514. doi: 10.3390/ijerph17155514
167. Residiwati G, Tuska HS, Kawai GK, Seifi-Jamadi A, Santoro D, Leemans B, et al. Practical methods to assess the effects of heat stress on the quality of frozen-thawed Belgian Blue semen in field conditions. Anim Reprod Sci. (2020) 221:106572. doi: 10.1016/j.anireprosci.2020.106572
168. Pires BV, Stafuzza NB, de Freitas LA, Mercadante MEZ, Ramos ES, Paz CCP. Expression of candidate genes for residual feed intake in tropically adapted Bos taurus and Bos indicus bulls under thermoneutral and heat stress environmental conditions. J Therm Biol. (2021) 99:102998. doi: 10.1016/j.jtherbio.2021.102998
169. Januskauskas A, Rodriguez-Martinez H. Assessment of sperm viability by measurement of ATP, membrane integrity and motility in frozen/thawed bull semen. Acta Vet Scand. (1995) 36:571–4. doi: 10.1186/BF03547671
170. Pérez-Crespo M, Pintado B, Gutiérrez-Adán A. Scrotal heat stress effects on sperm viability, sperm DNA integrity, and the offspring sex ratio in mice. Mol Reprod Dev. (2008) 75:40–7. doi: 10.1002/mrd.20759
171. Brito LF, Silva AE, Barbosa RT, Kastelic JP. Testicular thermoregulation in Bos indicus, crossbred and Bos taurus bulls: relationship with scrotal, testicular vascular cone and testicular morphology, and effects on semen quality and sperm production. Theriogenology. (2004) 61:511–28. doi: 10.1016/S0093-691X(03)00231-0
172. Singh SP, Kumar A, Sourya N. Effects of heat stress on animal reproduction. Int J Fauna Biol Sci. (2021) 8:16–20. doi: 10.22271/23940522.2021.v8.i2a.806
173. Boe-Hansen GB, Rêgo JPA, Satake N, Venus B, Sadowski P, Nouwens A, et al. Effects of increased scrotal temperature on semen quality and seminal plasma proteins in Brahman bulls. Mol Reprod Dev. (2020) 87:574–97. doi: 10.1002/mrd.23328
174. Zhang P, Gong RH, Yanai Y, Tokura H. Effects of clothing material on thermoregulatory responses. Textile Res J. (2002) 72:83–9.
175. Barnes BM, Kretzmann M, Licht P, Zucker I. The influence of hibernation on testis growth and spermatogenesis in the golden-mantled ground squirrel, Spermophilus lateralis. Biol Reprod. (1986) 35:1289–97. doi: 10.1095/biolreprod35.5.1289
176. Bozkaya F, Atli MO, Guzeloglu A, Kayis SA, Yildirim ME, Kurar E, et al. Effects of long-term heat stress and dietary restriction on the expression of genes of steroidogenic pathway and small heat-shock proteins in rat testicular tissue. Andrologia. (2017) 49:e12668. doi: 10.1111/and.12668
177. Mete F, Kilic E, Somay A, Yilmaz B. Effects of heat stress on endocrine functions and behaviour in the pre-pubertal rat. Indian J Medical Res. (2012) 135:233–9.
178. Makker K, Agarwal A, Sharma R. Oxidative stress and male infertility. Indian J Medical Res. (2009) 129:357e67. doi: 10.1007/978-981-10-4017-7_10
179. Paul C, Murray AA, Spears N, Saunders PT. A single, mild, transient scrotal heat stress causes DNA damage, subfertility and impairs formation of blastocysts in mice. Reproduction. (2008) 136:73. doi: 10.1530/REP-08-0036
180. Rahman MB, Vandaele L, Rijsselaere T, Maes D, Hoogewijs M, Frijters A, et al. Scrotal insulation and its relationship to abnormal morphology, chromatin protamination and nuclear shape of spermatozoa in Holstein-Friesian and Belgian Blue bulls. Theriogenology. (2011) 76:1246–57. doi: 10.1016/j.theriogenology.2011.05.031
181. Romanello N, de Brito Lourenço Junior J, Barioni Junior W, Brandão FZ, Marcondes CR, Pezzopane JRM, et al. Thermoregulatory responses and reproductive traits in composite beef bulls raised in a tropical climate. Int J Biometeorol. (2018) 62:1575–86. doi: 10.1007/s00484-018-1557-8
182. Rizzoto G, Boe-Hansen G, Klein C, Thundathil JC, Kastelic JP. Acute mild heat stress alters gene expression in testes and reduces sperm quality in mice. Theriogenology. (2020) 158:375–81. doi: 10.1016/j.theriogenology.2020.10.002
Keywords: heat load, thermal stress, reproduction, thermotolerance, spermatogenesis
Citation: Silva WC, Silva JAR, Camargo-Júnior RNC, Silva ÉBR, Santos MRP, Viana RB, Silva AGM, Silva CMG and Lourenço-Júnior JB (2023) Animal welfare and effects of per-female stress on male and cattle reproduction—A review. Front. Vet. Sci. 10:1083469. doi: 10.3389/fvets.2023.1083469
Received: 29 October 2022; Accepted: 22 February 2023;
Published: 31 March 2023.
Edited by:
Sebastián Demyda-Peyrás, Universidad Nacional de La Plata, ArgentinaReviewed by:
Muhammad Zahoor, University of Agriculture Dera Ismail Khan, PakistanDaniel Mota-Rojas, Metropolitan Autonomous University, Mexico
Copyright © 2023 Silva, Silva, Camargo-Júnior, Silva, Santos, Viana, Silva, Silva and Lourenço-Júnior. This is an open-access article distributed under the terms of the Creative Commons Attribution License (CC BY). The use, distribution or reproduction in other forums is permitted, provided the original author(s) and the copyright owner(s) are credited and that the original publication in this journal is cited, in accordance with accepted academic practice. No use, distribution or reproduction is permitted which does not comply with these terms.
*Correspondence: Welligton Conceição da Silva, welligton.medvet@gmail.com