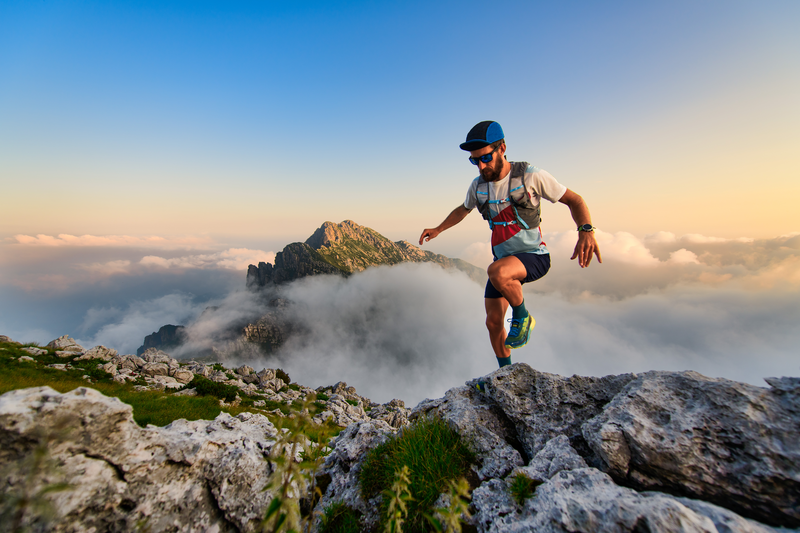
95% of researchers rate our articles as excellent or good
Learn more about the work of our research integrity team to safeguard the quality of each article we publish.
Find out more
ORIGINAL RESEARCH article
Front. Vet. Sci. , 24 February 2023
Sec. Animal Nutrition and Metabolism
Volume 10 - 2023 | https://doi.org/10.3389/fvets.2023.1039323
This article is part of the Research Topic Feedlot Cattle Nutrition and Metabolism View all 10 articles
Evaluation of the effects of feeding NutraGen supplement (NutraGen, NTG; Phibro Animal Health, Teaneck, NJ, USA) on growth performance, energetic efficiency, carcass characteristcs, and characteristics of digestion in calf-fed Holstein steers fed a conventional growing-finishing diet. Trial 1 evaluated growth performance, dietary energetics and carcass characteristics. Two hundred Holstein steer calves (134 ± 5 kg) were blocked by initial body weight (BW) and randomly assigned to 40 pens (5 steers/pen). Dietary treatments consisted of a steam-flaked corn-based growing-finishing diet supplemented with 0, 0.2, 0.4, or 0.6% NTG (DM basis). In trial 2, four Holstein steers (170 ±6 kg) with cannulas in the rumen and proximal duodenum were used in a 4 × 4 Latin square experiment to evaluate digestibility and ruminal characteristics using the treatments from trial 1. Compared to non-supplemented cattle, NTG increased BW (2.0%, P = 0.02) and tended to increase ADG (3.6%, P = 0.07) during the initial 56 d period. However, there were no treatment effects on overall growth performance and efficiency of dietary energy utilization after the first 56 days (P > 0.10). Supplementation of NTG increased (linear effect; P ≤ 0.03) longissimus muscle area and kidney, pelvic, and heart fat. There was no effect (P ≥ 0.05) of NTG supplementation on other carcass characteristics, liver abscess incidence, or liver abscess scars. Supplementation decreased the molar proportion of ruminal propionate (P = 0.05) and tended to increase acetate:propionate molar ratio (P = 0.09). However, there was no effect of NTG supplementation on ruminal and total tract diet digestion. NTG increased performance of Holstein steers during the first 56 d on feed in the feedlot. In addition, the steers had an increase in KPH fat and LM area, indicating that the additive induced change in metabolism of the steers.
Recently, there has been an increased concern about the over-routine use of supplemental antibiotics, which has increased the investigation of potential non-antibiotic alternatives in cattle diets (1). NutraGen (NTG; Phibro Animal Health, Teaneck, NJ, USA) contains similar properties to OmniGen (OMG; Phibro Animal Health, Teaneck, NJ, USA), which is used in the dairy industry (2). Previous research has reported that the use of this blend in dairy cows' diets includes improved metabolic response to a glucose tolerance test (2), modulation of inflammatory responses (3), increased milk production (4), and enhanced immunomodulatory response to heat stress (5, 6). The latter may be particularly relevant for enhancing growth performance responses in feedlot cattle during extreme ambient temperatures. The NTG is a blend of silicon dioxide, calcium aluminosilicate, sodium aluminosilicate, dehydrated brewer's yeast, mineral oil, calcium carbonate, rice hulls, niacin, biotin, d-calcium pantothenate, choline chloride, thiamine mononitrate, pyridoxine hydrochloride, riboflavin-5-phosphate, and folic acid.
Although some of these compounds have been studied in the beef industry individually, to the authors' knowledge, the mechanism of action of NTG and OMG on heat stress is still unknown. Wang et al. (7) observed that OMG supplementation increased the expression of neutrophil associated genes in peri-parturient dairy cows, such as interleukin-1b converting enzyme, interleukin-4 receptor, interleukin-8 receptor and thymopoeitin-α (7). Feeding OMG for 2 weeks increased mammary inflammatory response and antigen presentation to mammary infections caused by a single strain of mastitis pathogens (Streptococcus uberis, Escherichia coli, and Staphylococcus aureus) in a mouse model of infection, suggesting OMG could decrease the incidence of infectious diseases in ruminants (8). These reports together indicated that OMG might reduce the incidence of infectious diseases in ruminants. However, no information has been published on the effects of supplemental NTG on cattle growth performance and digestive function when cattle are fed a common steam-flaked corn-based diet.
Moreover, there is no information on the potential benefits of feeding NTG as a heat load mitigator for calf-fed Holstein steers in the southern desert region of the United States. A region characterized by its extreme heat conditions during the summer months. Therefore, the objective of the current study was to evaluate the effects of NTG supplementation on growth performance, energetic efficiency, carcass characteristics, and digestive function in calf-fed Holstein steers.
Procedures for animal care and management were conducted under a protocol (#20548) approved by the University of California, Animal Use and Care Advisory Committee.
Two hundred Holstein steer calves (130.9 ± 4 kg) were utilized to evaluate the influence of NTG supplementation on growth performance, dietary energetics, and carcass characteristics. The trial was initiated on January 14, 2020, and completed following a 322-d feeding period (December 1, 2020). Calves were purchased from a commercial calf ranch (CalfTech, Tulare, CA). Upon arrival at the University of California Desert Research and Extension Center (Holtville, CA), calves were vaccinated for IBR, BVD, PI3, and BRSV (Bovi-shield® Gold One Shot, Zoetis Animal Health, New York, NY), clostridials (Ultrabac® 7, Zoetis Animal Health, New York, NY), treated against internal and external parasites (Dectomax, Zoetis Animal Health, New York, NY), injected with 1,500 IU vitamin E (as d-alpha-tocopherol) 500,000 IU vitamin A (as retinyl-palmitate) and 50,000 I.U. vitamin D3 (Vital E-AD, Stuart Products, Bedford, TX), and 300 mg tulathromycin (Draxxin, Zoetis Animal Health, New York, NY). Calves were blocked by initial shrunk (off truck) weight and randomly assigned within weight groupings to 40 pens (5 steers/pen, 10 pens/treatment). On day 28, calves received the Ultrabac® 7 booster vaccination and were vaccinated with Endovac-Beef (Endovac Animal Health, Columbia, MO). On d 56 calves received the Endovac-Beef booster vaccination. Pens were 62 m2 with 25 m2 overhead shade, automatic waterers, and 2.4 m fence-line feed bunks. Steers were allowed ad libitum access to feed and water. Fresh feed was provided daily.
Dietary treatments are shown in Table 1, consisting of a steam-flaked corn-based diet supplemented with (DM basis): (1) no feed additive; (2) 0.2% of NTG; (3) 0.4% of NTG; (4) 0.6% of NTG. Nutragen doses were based on a previous study on dairy cows, and no feed additive was included in the diet. Diets were prepared weekly and stored in plywood boxes in front of each pen. On d 112 and 224, all steers were reinjected subcutaneously with 500,000 IU vitamin A (Vital E-A + D, Stuart Products, Bedford, TX) and implanted with Revalor-S (Intervet, Millsboro, DE). The health status of cattle was checked daily. Cattle were monitored daily by trained personnel for signs of illness or pinkeye. Cattle with signs of illness were pulled out, classified as morbid, and treated with an antimicrobial if the rectal temperature was ≥ 39.5°C. Antimicrobial treatments were conducted following a veterinarian's recommendation. A post-treatment interval of 3 days was implemented after the first and second treatments. If cattle remained morbid after the third treatment and the prognosis of a full recovery was unlikely, cattle were removed from the study (two animals died during the experiment for causes unrelated to dietary treatments).
Steer full body weight (BW) was recorded every 28 days until the end of the experiment to monitor live weight changes. Steers were not denied feed or water before weighing. In determining ADG, interim and final weights were reduced by 4% to account for digestive tract fill (9). From March 9, 2020, to the end of the experiment, SmaX-tec intraruminal boluses (SmaX-tec animal care technology®, Graz, Austria) were orally inserted into the rumen (1 steer per pen) to monitor the ruminal temperature. Continuous real-time temperature data was retrieved using a monitoring device located in the proximity of cattle pens.
Hot carcass weights (HCW) and liver abscess incidence [based on size and number, scaled as 0, A–, A, and A+; (10)], as well as liver scarring measures, were obtained at the time of slaughter. After carcasses were chilled for 24 h, the following measurements were obtained: Longissimus muscle (LM) area (cm2) by direct grid reading of the muscle at the 12th rib; subcutaneous fat (cm) over the LM at the 12th rib taken at a location 3/4 the lateral length from the chine bone end (adjusted for unusual fat distribution); kidney, pelvic and heart fat (KPH) as a percentage of HCW; marbling score [(11); using 3.0 as minimum slight, 4.0 as minimum small, 5.0 as minimum modest, 6.0 as minimum moderate, etc.], and preliminary as well as estimated retail yield of boneless, closely trimmed retail cuts from the round, loin, rib and chuck as a percentage of HCW [Yield, % = 52.56–1.95 × subcutaneous fat−1.06 × KPH + 0.106 × LM area−0.018 × HCW; (12)].
Daily energy gain (EG; Mcal/d) was calculated by the equation: EG = ADG1.097 0.0557W0.75, where W is the mean shrunk B.W. [kg; (13)] Maintenance energy (EM) was calculated by the equation: EM = 0.086W 0.75. Dietary NEg was derived from NEm by the equation: NEg = 0.877 NEm−0.41 (14). Dry matter intake is related to energy requirements and dietary NEm according to the equation: DMI = (EM/NEm) + (EG/(0.877NEm−0.41). From this relationship, dietary NE can be resolved by means of the quadratic formula: x = (–b – √ b2 – 4ac)/ 2c, where: x = NEm, a = – 0.42 EM, b = 0.887 EM + 0.41 DMI + EG, and c = – 0.887 DMI (15).
Climatic variables (ambient temperature and relative humidity) were obtained every hour from an on-site weather station (California Irrigation Management Information System; Meloland Station) throughout the experimental period. The temperature humidity index was calculated using the following formula THI = (0.8 × Ta) + [(H/100) × (Ta – 14.4)] + 46.4, where Ta is air temperature (°C) and H is relative humidity (16, 17); Min = minimum; Max = maximum.
Pens were used as experimental units. The experimental data were analyzed as a randomized complete design experiment according to the following statistical model: Yij = μ + Bi + Tj + Eij, where μ is the common experimental effect, Bi represents the initial weight group effect (df = 6), Tj represents the dietary treatment effect (df = 3), and Eij represents the residual error (df = 18). Treatment effects were tested using the following contrasts: 0 vs. NTG and linear and quadratic polynomials to assess the effect of dosage (Stastix 10, Analytical Software, Tallahassee, FL).
Four Holstein steers (170 ±6 kg) with cannulas in the rumen and proximal duodenum were used in a 4 × 4 Latin square experiment. Treatments are the same as in Trial 1 (Table 1). A single basal diet was prepared with 0.3% chromic oxide as a digesta marker. Corresponding amounts of NTG (0, 0.2, 0.4, and 0.6% of diet DM) were top-dressed on the basal diet at the time of feeding. Dry matter intake was restricted to 2.2% of live weight to avoid feed refusals. Diets were fed at 0,800 and 2,000 daily. Experimental periods consisted of a 17-d diet adjustment period followed by a 4-d collection period. During the collection period, duodenal and fecal samples were obtained from all steers twice daily: d 1, 0750, and 1,350; d 2, 0,900, and 1,500; d 3, 1,050, and 1,650; and d 4, 1,200 and 1,800. Individual samples consisted of approximately 700 ml duodenal chyme and 200 g (wet basis) fecal material. Additionally, on d4 of the collection period at 1,200 h, 100 mL of ruminal fluid was obtained from each steer via the ruminal cannula. Ruminal fluid pH was determined on freshly collected samples. Ruminal fluid was then strained through 4 layers of cheesecloth. Freshly prepared 25% (wt/vol) m-phosphoric acid (2 mL) was added to 8 mL of the strained ruminal fluid, centrifuged (17,000 × g for 10 min) and supernatant fluid stored at −20° C for VFA analysis [direct injection gas chromatography, using HP 5890A gas chromatograph, Hewlett Packard, Palo Alto, CA; DB-FFAP column, J&W Instruments, New Brighton, MN; WSFA-2 VFA standards, Supelco Analytical, Bellefonte, PA; 3-methyvaleric acid internal standard, TCI America, Portland, OR; (18)]. Duodenal and fecal samples from each steer within each collection period were composited for analysis. Upon completion of the experiment, ruminal fluid was obtained via the ruminal cannula from all steers and composited for the isolation of ruminal bacteria by differential centrifugation (19). Feed, duodenal fluid, and fecal samples were subjected to the following analysis: DM [oven drying at 105°C until no further weight loss; method 930.15; (20)]; ash [method 942.05; (20)], Kjeldahl N [method 984.13; (20)]; aNDFom (21), corrected for NDF-ash, incorporating heat stable α-amylase (Ankom FAA, Ankom Technology, Macedon, NY) at 1 mL per 100 mL of NDF solution]; chromic oxide (22); and starch (23). Duodenal samples were also analyzed for ammonia N (method 941.04, 20) and purines (24). Duodenal flow and fecal excretion of DM were determined based on marker ratio using chromic oxide. Microbial organic matter (MOM) and nitrogen (MN) leaving the abomasum were estimated using purines as microbial markers (24). Organic matter (OM) fermented in the rumen is considered equal to OM intake minus the difference between the amount of total OM reaching the duodenum and MOM reaching the duodenum. Feed N escape to the small intestine is considered equal to total N leaving the abomasum minus ammonia-N, MN, and endogenous N [0.195 × BW0.75; (25)]. Methane production was estimated based on the theoretical fermentation balance for observed molar distribution of VFA and OM fermented in the rumen (26).
The trial was analyzed as a balanced 4 × 4 Latin square according to the following statistical model: Yijk = μ+ Ai + Pj + Tk + Eijk, where Yijk is the response variable, μ is the common experimental effect, Ai is the steer effect, Pj is the period effect, Tk is the treatment effect, and Eijk is the residual error. Treatment main effects were assessed by means of orthogonal polynomials.
In the present study, NTG was supplemented at 0, 0.2, 0.4, and 0.6% of diet DM. During the first 56 days of the study, steers consumed 0, 59, 119, and 178 mg per kg of live BW per day, respectively. However, the observed intake from 112 to the end of the experiment averaged 0, 39, 79, and 117 mg per kg of live BW per day, respectively. Treatment effects on health, growth performance, and estimated dietary NE are shown in Table 2. Morbidity and mortality were low and not affected (P > 0.20) by dietary treatments, averaging 5.5 and 0.5%, respectively. Supplementation of NTG increased (quadratic effect; P = 0.05) shrunk live weight on d 56 of the trial, with cattle supplemented with 0.2% NTG being the heaviest. Moreover, NTG supplementation tended to increase (P = 0.07) ADG from d 1–56 and decrease (P = 0.06) ADG from d 112–322. There was no effect of NTG on growth at any other time and no effect on DMI, feed efficiency, or efficiency of energy utilization (P > 0.10) (Table 2).
Table 2. Effects of feeding NutraGen supplement on health, growth performance and dietary net energy utilization.
Treatment effects on carcass characteristics, liver abscess incidence, liver scars measures, and morbidity are shown in Table 3. Dietary supplementation of NTG increased LM area (linear effect; P = 0.03) and KPH fat (linear effect; P = 0.01). However, there was no effect (P ≥ 0.12) of NTG supplementation on other carcass characteristics, liver abscess incidence, or liver abscess scars.
Table 3. Effects of feeding NutraGen supplement on carcass characteristics, liver abscess, and cattle morbidity.
Treatment effects on minimum, average, and maximum ruminal temperature during the summer months (June, July, August, and September) are presented in Table 4. There was no effect (P ≥ 0.18) of NTG supplementation on minimum, average, or maximum ruminal temperature during summer months when cattle were under high ambient temperature conditions (Figure 1).
Figure 1. Temperature-humidity index (THI) during the 286-d feeding period: THI = (0.8 × Ta) + [(H/100) × (Ta – 14.4)] + 46.4, where Ta is air temperature (°C), and H is relative humidity (16, 17); Min = minimum; Max = maximum.
Treatment effects on ruminal and total tract digestion and ruminal pH and VFA are shown in Tables 5, 6, respectively. There was no effect (P ≥ 0.12) of NTG supplementation on ruminal and total tract digestion. The supplementation of NTG decreased (P = 0.05) propionate concentration in the rumen by 16.5% compared to the non-supplemented diet; therefore, the ruminal acetate/propionate ratio tended (P = 0.09) to increase linearly with NTG supplementation. There was a quadratic effect (P < 0.01) on isobutyrate concentration in the rumen, with isobutyrate minimal concentration being observed when cattle were supplemented with 0.2% of NTG in the diet. Moreover, cattle supplemented with NTG had a linear increase (P = 0.05) in the methane/mol glucose ratio compared to non-supplemented cattle. There was no effect (P ≥ 0.10) of NTG supplementation on ruminal pH and other VFA concentrations.
Table 5. Effects of feeding NutraGen supplement on characteristics of ruminal and total tract digestion.
Although this product had not been previously tested in cattle under the conditions of the present study, we hypothesized that NTG supplementation might enhance growth performance, dry matter intake, and apparent digestibility of nutrients, ruminal parameters, and carcass characteristics of calf-fed Holstein steers. According to the manufacturer, NTG combines natural components with immunostimulant function, especially under stressful circumstances. Previous research reported that most respiratory diseases in the feedlot occurred within the first 30 days after arrival (27). Therefore, the greater growth performance observed in calves supplemented with NTG during the first weeks of the experiment may be associated with a greater immune response to challenges being faced during the receiving period, as has been suggested in previous studies (1–4, 6). However, in agreement with the current study, Colombo et al. (6) did not observe an overall effect on growth performance when crossbred yearling cattle were fed a similar immunomodulatory supplement in the feedlot. Sanchez et al. (2) observed an increase in the final BW of beef heifers supplemented with OMG, a similar product used in the current experiment. The positive effects of OMG (mainly in dairy studies; 2–4, 6) have been attributed to the enhanced immune system, growth performance responses to OMG may largely depend on the stress conditions that animals are experiencing. For comparison, no prior studies evaluate growth performance responses to NTG supplementation of calf-fed Holstein steers.
In the present study, the dosage of NTG was supplied in the percentage of DMI (0, 0.2, 0.4, and 0.6% of DM), representing an increase in the dosage consumed according to BW. However, the intake in the first 56 days (0, 59, 119, and 178 mg of NTG per kg of live BW per day) was greater compared from 112 to the end of the experiment (0, 39, 79, and 117 mg of NTG per kg of live BW per day). However, the effect of NTG on growth performance at the beginning of the experiment was not dose-dependent. Sanchez et al. (2) and Moriel et al. (28) reported an enhancement in the metabolism of beef cattle supplemented with 100 and 88 mg of OMG per kg of BW, respectively.
Brown-Brandl et al. (29) stated that under ambient conditions where THI is ≥ 78, cattle are experiencing heat stress and “danger” conditions. In the present study, cattle were experiencing THI greater than 70 for almost half of their time on feed. Colombo et al. (6) fed 111 mg of OMG per kg of live BW per day and observed that this supplementation ameliorated hyperthermia in finishing beef cattle exposed to heat stress conditions. Although supplemental OMG has been shown to decrease the negative effect of heat-stress conditions on the performance of heifers and steers in feedlot and pasture (2, 6, 28), these extreme heat conditions faced by steers in the present study may have reduced the potential benefits of feeding an immunomodulatory supplement (NTG). The weather conditions that calf-fed Holstein steers were exposed to in this study were unfavorable from a growth performance standpoint (Figure 1). Nevertheless, no death loss occurred during the period of elevated THI. Historically, very low or no mortality during the early growing phase of calf-fed Holstein has been observed at the Research Center used in this study.
The percentage of KPH fat and LM area increased linearly with increasing levels of NTG supplementation, indicating that the metabolic effect that effects of NTG on carcass characteristics were dose-dependent. The acetate and glucose are used in ruminant metabolism as substrates for fatty acid synthesis. Sanchez et al. (2) observed that heifers supplemented with OMG were more sensitive to changes in blood glucose. Moreover, Buntyn et al. (30) observed an increase in blood glucose in steers fed OMG without affecting the growth performance of feedlot steers or carcass characteristics. These findings indicate that OMG (or NTG) supplementation may affect the storage and redistribution of energy deposits: expressed by changes in KPH fat and LM area. However, research is needed to provide more information on how NTG supplementation may have brought about the observed changes.
Very little information is available that evaluates the effects of NTG on ruminal parameters digestibility. Prior research in dairy cows supplemented with OMG reported no effects of supplementation on ruminal pH and VFA concentrations (31). In the current study, NTG decreased ruminal propionate molar proportion, leading to increased estimated methane energy loss. According to these results appears that NTG may affect microorganisms that enhance methanogens. Nevertheless, components of NTG, such as dehydrated brewer's yeast, have been observed to increase ruminal propionate molar concentration in dairy cattle (32). However, as previously stated, to the authors' knowledge, there are no prior studies evaluating the effects of NTG (or OMG) on diet digestibility or ruminal kinetics in cattle fed a grain-based feedlot finishing diet.
Supplementing calf-fed Holstein steers with NTG increased the live weight of steers during the initial 56 days on feed. However, despite decreasing propionate concentration, treatment effects were not appreciable on overall cattle growth performance. Calf-fed Holstein steers supplemented with NTG had a greater percentage of KPH fat and LM area. However, NTG supplementation had no major effect on diet digestibility and ruminal temperature.
The raw data supporting the conclusions of this article will be made available by the authors, without undue reservation.
The animal study was reviewed and approved by Procedures for animal care and management were conducted under protocol (#20548) approved by the University of California, Animal Use and Care Advisory Committee.
All authors listed have made a substantial, direct, and intellectual contribution to the work and approved it for publication.
This project was supported through the University of California Agricultural Experiment Station with Hatch funding from the USDA National Institute of Food and Agriculture (CA-D-ASC-6578-H).
The authors declare that the research was conducted in the absence of any commercial or financial relationships that could be construed as a potential conflict of interest.
All claims expressed in this article are solely those of the authors and do not necessarily represent those of their affiliated organizations, or those of the publisher, the editors and the reviewers. Any product that may be evaluated in this article, or claim that may be made by its manufacturer, is not guaranteed or endorsed by the publisher.
1. Broadway P, Carroll J, Callaway T. Alternative antimicrobial supplements that positively impact animal health and food safety. Agri Food Anal Bacteriol. (2014) 4:109–21.
2. Sanchez NCB, Carroll JA, Broadway PR, Schell TH, Puntenney SB, McLean DJ. Supplementation of OmniGen-AF improves the metabolic response to a glucose tolerance test in beef heifers. Transl Anim Sci. (2019) 3:1521–9. doi: 10.1093/tas/txz130
3. Wu Z, Alugongo GM, Xiao J, Li J, Yu Y, Li Y, et al. Effects of an immunomodulatory feed additive on body weight, production parameters, blood metabolites, and health in multiparous transition Holstein cows. J Anim Sci. (2019) 90:167–77. doi: 10.1111/asj.13066
4. Brandão AP, Cooke RF, Correa FN, Piccolo MB, Gennari R, Leiva T, Vasconcelos JLM. Physiologic, health, and production responses of dairy cows supplemented with an immunomodulatory feed ingredient during the transition period. J Dairy Sci. (2016) 99:5562–5572. doi: 10.3168/jds.2015-10621
5. Leiva T, Cooke RF, Brandao AP, Schubach KM, Batista LFD, Miranda MF, et al. Supplementing an immunomodulatory feed ingredient to modulate thermoregulation, physiological, and production responses in lactating dairy cows under heat stress conditions. J Dairy Sci. (2017) 100:4829–38. doi: 10.3168/jds.2016-12258
6. Colombo EA, Cooke RF, Millican AA, Schubach KM, Scatolin GN, Rett B, et al. Supplementing an immunomodulatory feed ingredient to improve thermoregulation and performance of finishing beef cattle under heat stress conditions. J Anim Sci. (2019) 97:4085–92. doi: 10.1093/jas/skz266
7. Wang YQ, Puntenney SB, Burton JL, Forsberg NE. Use of gene profiling to evaluate the effects of a feed additive on immune function in periparturient dairy cattle. J Anim Physiol Anim Nutr. (2009) 93:66–75. doi: 10.1111/j.1439-0396.2007.00780.x
8. Rowson AD, Wang YQ, Aalseth E, Forsberg NE, Puntenney SB. Effects of an immunomodulatory feed additive on the development of mastitis in a mouse infection model using four bovine-origin isolates. Animal. (2011) 5:220–9. doi: 10.1017/S1751731110001850
10. Brown H, Bing RF, Grueter HP, McAskill JW, Cooley CO, Rathmacher RP. Tylosin and Chlortetracycline for the prevention of liver abscesses, improved weight gains, and feed efficiency in feedlot cattle. J Anim Sci. (1975) 40:207–13. doi: 10.2527/jas1975.402207x
11. USDA. United States Standards for Grading of Carcass Beef. Washington, DC: Agricultural Marketing Service, USDA (1997).
12. Murphey CE, Hallett DK, Tyler WE, Pierce JC. Estimating Yields of Retail Cuts from Beef Carcasses. Presented at the 62nd Meet Am Soc Anim Prod. Chicago, IL (1960).
13. NASEM. Nutrient Requirements of Beef Cattle (6th Ed.). Washington, DC: National Academy Press (1984)
14. Zinn RA. Influence of lasalocid and monensin plus Tylosin on comparative feeding value of steam-flaked versus dry-rolled corn diets for feedlot cattle. J Anim Sci. (1987) 65:256–66. doi: 10.2527/jas1987.651256x
15. Zinn RA. Shen Y. An evaluation of ruminally degradable intake protein and metabolizable amino acid requirements of feed lot calves. J Anim Sci. (1998) 76:1280–9. doi: 10.2527/1998.7651280x
17. NOAA (National Oceanic and Atmospheric Administration). Livestock Hot Weather Stress. Regional Operations Manual Letter C-31–76. Central Region, Kansas City, MO (1976) US Dept Commerce, Natl Weather Serv.
18. Zinn RA. Crude protein and amino acid requirements of growing-finishing Holstein steers gaining 1.43 kilograms per day. J Anim Sci. (1988) 66:1755–63. doi: 10.2527/jas1988.6671755x
19. Bergen WG, Purser DB, Cline JH. Effect of ration on the nutritive quality of rumen microbial protein. J Anim Sci. (1968) 27:1497–501. doi: 10.2527/jas1968.2751497x
20. AOAC. Official Methods of Analysis of AOAC International. 17th ed. Gaithersburg, MD: Assoc Off Anal Chem (2000).
21. Van Soest PJ, Robertson B, Lewis BA. Methods for dietary fiber, neutral detergent fiber, and nonstarch polysaccharides in relation to animal nutrition. J Dairy Sci. (1991) 74:3583–3597. doi: 10.3168/jds.S0022-0302(91)78551-2
22. Hill FW, Anderson DL. Comparison of metabolizables energy and productive energy determinations with growing chicks. J Nutr. (1958) 64:587–603. doi: 10.1093/jn/64.4.587
23. Zinn RA. Influence of flake density on the comparative feeding value of steam-flaked corn for feedlot cattle. J Anim Sci. (1990) 68:767–75. doi: 10.2527/1990.683767x
24. Zinn RA, Owens FN. A rapid procedure for purine measurement and its use for estimating net ruminal protein synthesis. Can J Anim Sci. (1986) 66:157–66. doi: 10.4141/cjas86-017
25. Ørskov ER, MacLeod NA, Kyle DJ. Flow of nitrogen from the rumen and abomasum in cattle and sheep given protein-free nutrients by intragastric infusion. Br J Nutr. (1986) 56:241–8. doi: 10.1079/BJN19860103
26. Wolin, MJ. A theoretical rumen fermentation balance. J Dairy Sci. (1960) 43:1452–1459. doi: 10.3168/jds.S0022-0302(60)90348-9
27. Hollis LC. Investigating feedlot respiratory disease outbreaks, chapter 128 - food animal practice (Fifth Edition), W.B. Saunders (2009). p. 673–76. doi: 10.1016/B978-141603591-6.10128-9
28. Moriel P, Palmer EA, Oliveira RA, Vedovatto M, Izquierdo VS, Silva HM, et al. Stair-step strategy and immunomodulatory feed ingredient supplementation for grazing heat-stressed Bos indicus-influenced beef heifers. J Anim Sci. (2022) 100:1–13. doi: 10.1093/jas/skac107
29. Brown-Brandl TM, Eigenberg RA, Nienaber JA, Hahn GL. Dynamic response indicators of heat stress in shaded and non-shaded feedlot cattle, Part 1: analyses of indicators. Biosyst Eng. (2005) 90:451–462. doi: 10.1016/j.biosystemseng.2004.12.006
30. Buntyn JO, Sieren SE, Curtis JBB, Dirk B, Galen EE, Sanchez NCB, et al. Effects of Feeding OmniGen- AF® on Immune Function, Performance, and Carcass Characteristics during the Feeding Period. Nebraska Beef Cattle Reports 864 (2016).
31. Mezzetti M, Minuti A, Piccioli-Cappelli F, Gabai G, Trevisi E. Administration of an immune stimulant during the transition period improved lipid metabolism and rumination without affecting inflammatory status. Animals. (2019) 28:619. doi: 10.3390/ani9090619
32. Baker LM, Kraft J, Karnezos TP, Greenwood SL. Review: the effects of dietary yeast and yeast-derived extracts on rumen microbiota and their function. Animal Feed Sci Technol. (2022) 294:115476. doi: 10.1016/j.anifeedsci.2022.115476
Keywords: Holstein, feedlot, performance, heat-stress, cattle
Citation: Carvalho PHV, Latack BC, Ferraz Junior MVC, Flores R, Sanchez-Cruz G, Montaño MF and Zinn RA (2023) The effects of NutraGen supplement on cattle growth performance, energetic efficiency, carcass characteristics, and characteristics of digestion in calf-fed Holstein steers. Front. Vet. Sci. 10:1039323. doi: 10.3389/fvets.2023.1039323
Received: 08 September 2022; Accepted: 07 February 2023;
Published: 24 February 2023.
Edited by:
Bangyuan Wu, China West Normal University, ChinaReviewed by:
Ilias Giannenas, Aristotle University of Thessaloniki, GreeceCopyright © 2023 Carvalho, Latack, Ferraz Junior, Flores, Sanchez-Cruz, Montaño and Zinn. This is an open-access article distributed under the terms of the Creative Commons Attribution License (CC BY). The use, distribution or reproduction in other forums is permitted, provided the original author(s) and the copyright owner(s) are credited and that the original publication in this journal is cited, in accordance with accepted academic practice. No use, distribution or reproduction is permitted which does not comply with these terms.
*Correspondence: Richard A. Zinn, cmF6aW5uQHVjZGF2aXMuZWR1
Disclaimer: All claims expressed in this article are solely those of the authors and do not necessarily represent those of their affiliated organizations, or those of the publisher, the editors and the reviewers. Any product that may be evaluated in this article or claim that may be made by its manufacturer is not guaranteed or endorsed by the publisher.
Research integrity at Frontiers
Learn more about the work of our research integrity team to safeguard the quality of each article we publish.