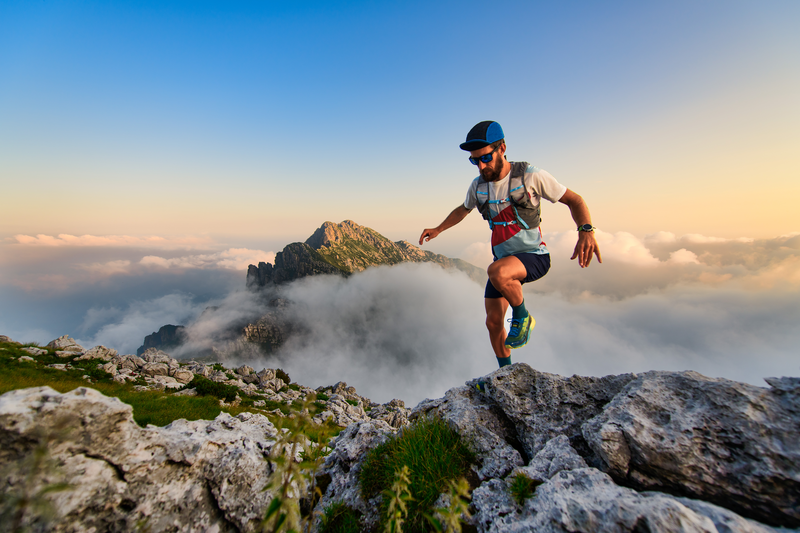
94% of researchers rate our articles as excellent or good
Learn more about the work of our research integrity team to safeguard the quality of each article we publish.
Find out more
ORIGINAL RESEARCH article
Front. Vet. Sci. , 21 September 2022
Sec. Animal Nutrition and Metabolism
Volume 9 - 2022 | https://doi.org/10.3389/fvets.2022.997709
This article is part of the Research Topic Nutritional Management and Organelle Dysfunction View all 6 articles
Leukemia inhibitory factor (LIF) is a multipotent cytokine of the IL-6 family which plays a critical role in the maturation and development of oocytes. This study evaluated the influence of LIF on the maturation and development ability of yak oocytes, and the quality of subsequent blastocysts under in vitro culture settings. Different concentrations of LIF (0, 25, 50, and 100 ng/mL) were added during the in vitro culture of oocytes to detect the maturation rate of oocytes, levels of mitochondria, reactive oxygen species (ROS), actin, and apoptosis in oocytes, mRNA transcription levels of apoptosis and antioxidant-related genes in oocytes, and total cell number and apoptosis levels in subsequent blastocysts. The findings revealed that 50 ng/mL LIF could significantly increase the maturation rate (p < 0.01), levels of mitochondria (p < 0.01) and actin (p < 0.01), and mRNA transcription levels of anti-apoptotic and antioxidant-related genes in yak oocytes. Also, 50 ng/mL LIF could significantly lower the generation of ROS (p < 0.01) and apoptosis levels of oocytes (p < 0.01). In addition, blastocysts formed from 50 ng/mL LIF-treated oocytes showed significantly larger total cell numbers (p < 0.01) and lower apoptosis rates (p < 0.01) than the control group. In conclusion, the addition of LIF during the in vitro maturation of yak oocytes improved the quality and the competence of maturation and development in oocytes, as well as the quality of subsequent blastocysts. The result of this study provided some insights into the role and function of LIF in vitro yak oocytes maturation, as well as provided fundamental knowledge for assisted reproductive technologies in the yak.
The yak (Bos grunniens) is an ancient species of the Tibetan plateau that lives year-round at high altitudes (1) and provides important subsistence and economic resources such as meat, milk, hide, hair, and dung for Tibetans and other nomadic people (2). Yaks are seasonal estrus animals, usually coming into estrus between July and October (3, 4). Because of their low reproductive performance (one litter in 2 years or 2 L in 3 years) (1), yak populations are gradually decreasing year by year. This affects the living standards of Tibetan people and is not conducive to species diversity in China. The employment of assisted reproductive techniques to enhance the yak population is an excellent alternative. The in vitro maturation of cultured yak oocytes and the in vitro development of early embryos are essential to assisted reproductive technologies, but numerous obstacles hinder their growth. Therefore, it is highly vital to discover the appropriate mechanism of in vitro maturation of yak oocytes and the in vitro development of early embryos.
Oocyte quality is a vital element for effective oocyte fertilization and preimplantation embryo development. Following ovulation, whether in vivo or in vitro, oocytes produce reactive oxygen species (ROS) due to a lack of adenosine triphosphate (ATP), which down-regulates anti-apoptotic proteins such as Bcl-2 and induces apoptosis (5, 6). There are various crucial actors such as mitochondria, ROS, and actin that affect oocyte maturation and development. Mitochondria are organelles in mammalian cells that play a crucial role in oocyte growth and function, and their activity may be utilized as a key signal to identify oocyte quality (7). Mitochondria play a key role in cellular metabolism and epigenetics (8, 9), where they interact with the nucleus to impact oocyte maturation and preimplantation embryonic development (10, 11). Furthermore, mitochondrial malfunction may raise the quantity of ROS, which can play a significant role in the apoptotic process by controlling apoptotic signaling factors such as ROS (5, 12). ROS are a class of short-lived, highly reactive small molecules (13). They are produced as a byproduct of aerobic metabolism and contain hydrogen peroxide (H2O2), superoxide anion (O), and hydroxyl radicals (OH·) (14). The intracellular ROS content is at a stable level through regulation. It has been shown that low to moderate doses of ROS are considered essential in the regulation of normal physiological functions involved in cell cycle processes such as proliferation, differentiation, migration and cell death (15). Excessive ROS are produced in the cell when the antioxidant detoxification system fails to regulate intracellular ROS levels at a low level, causing damage to proteins, nucleic acids, lipids, cell membranes, and organelles (e.g., mitochondria) (13, 16), exacerbating oocyte apoptosis (17), and reducing oocyte fertility (18). Actin is one of the numerous and highly conserved protein groups found in most eukaryotic cells which plays an important role in many cellular functions (19). Actin alters various states involved in important processes such as nuclear localization, germinal vesicle transit and rupture, spindle migration and anchoring, spindle rotation, chromosomal segregation, and polar body extrusion during meiosis in mammalian oocytes (20, 21).
Leukemia inhibitory factor (LIF) is a potent cytokine that belongs to the IL-6 family of cytokines (22). LIF is involved in several crucial phases of mammalian reproduction. LIF has been found to have a key function in mammalian follicle growth, ovulation, oocyte meiosis, maturation, and embryo development and implantation (23–26). However, there are few studies on the ability of LIF to influence oocyte development and maturation through the modulation of oocyte mitochondria, ROS, and actin levels. The study sought to determine the regulatory effects of LIF on mitochondria, ROS, actin, and apoptosis in yak oocytes, which consequently alters the developmental ability of yak oocytes and the quality of subsequent blastocysts.
Unless otherwise stated, all chemicals and reagents were purchased from Sigma Aldrich (St. Louis, MO, USA).
Ovaries were collected from a commercial slaughterhouse (Xining, Qinghai, China) and stored at 30°C in sterile saline (0.9% NaCl) with two antibiotics (100 IU/mL penicillin and 0.05 mg/mL streptomycin), and sent to the laboratory within 4 h (4, 27). Upon arrival at the laboratory, ovarian samples were cleaned three times with sterile saline containing two antibiotics at 35°C to minimize external contamination. Follicular fluid was collected by extracting follicles at a diameter of 3–8 mm from the surface of the ovary using an 18G needle. The cumulus-oocyte complexes (COCs) were washed with an oocyte picker under a microscope to eliminate leftover contaminants. At the same time, unexpanded cumulus-oocyte complexes with homogenous cytoplasm surrounded by three or more layers of cumulus cells were chosen for the subsequent analysis.
The immature cumulus-oocyte complexes were put in maturation media and cultivated in a 38°C, 5% CO2 humidified incubator for 24 h. The maturation fluid consisted of TCM-199 (Gibco, Grand Island, NY, USA), 10% FBS, 10 μg/mL follicle-stimulating hormone (FSH), 10 μg/mL Luteinizing hormone (LH) and 1 μg/mL β-Estradiol (E2) (28). The final concentrations of LIF (LIF1050, Sigma Aldrich, USA) were 0 ng/mL, 25 ng/mL, 50 ng/mL and 100 ng/mL by adding leukemia inhibitory factor to the maturation medium. The maturation solution was applied to a four-well plate, 400 μL per well, and incubated in a CO2 incubator.
After 24 h of culture, the cumulus cells enclosing the oocytes were digested with 0.1% hyaluronidase to get bare oocytes. The oocyte samples were put in 5 μM ionomycin and incubated in the incubator for 5 min. After washing, they were put in 2 mM 6-dimethylaminopurine and incubated in the incubator for 4 h (29, 30). The activated oocytes were washed three times before being transferred to synthetic oviduct fluid and cultured in a humidified incubator at 38.5°C and 5% CO2. After 8 d, blastocysts of each experimental group were taken for further apoptosis detection assays to count the total cell number of blastocysts.
Oocytes mitochondrial distribution was detected using MitoTracker® Mitochondrion-Selective Probes (M22426, Invitrogen, CA). According to the manufacturer's instructions, the probe was adjusted to the working solution concentration of 200 nM and then incubated. The oocyte samples were transferred to the working solution and incubated at 37°C for 30 min in the dark. After washing 3 times, it was transferred to a glass slide and images were taken using a fluorescence inverted microscope.
Oocytes ROS levels were measured using a reactive oxygen species assay kit (S0033, Beyotime, China). The trial procedure was carried out exactly in line with the manufacturer's instructions. Briefly, the cleaned oocytes were incubated in DCFA-DA (1:5,000) in the dark at 37°C for 20 min. After washing 3 times, it was transferred to a glass slide and images were taken using a fluorescence inverted microscope.
Actin was detected using the Phalloidin-iFluor™ 594 Conjugate Kit (23122, AAT Bioquest). According to the manufacturer's instructions, the oocyte samples were immersed in 4% paraformaldehyde for 30 min and then rinsed 3 times for 5 min each. The working solution was formed into microdroplets, and the oocytes were transferred to the microdroplets and incubated at room temperature for 45 min in the dark. After washing three times, the oocytes were placed on a glass slide, covered with a coverslip, and photographed using a fluorescent inverted microscope.
The early apoptosis level of oocytes was measured using the Annexin V-FITC apoptosis kit (C1062L, Beyotime, China). According to the instructions, the Annexin V-FITC working solution was prepared by mixing 2.5 μL Annexin V-FITC with 97.5 μL Annexin V-FITC binding solution. The oocyte samples were transferred to the Annexin V-FITC working solution and incubated at room temperature for 20 min in the dark. The oocytes were then put on glass plates and images were taken using a fluorescent inverted microscope.
Yak sperm was purchased from the Centre of Livestock Reproductive and Developmental in Qinghai province of China, which was frozen in 0.25-ml straws. The method was optimized according to a procedure previously reported (31, 32). Briefly, yak frozen sperm from a single straw were extracted, thawed in 37°C water for 1 min, cleaned 3 times in DPBS, and centrifuged at 1,000 g for 5 min. The sperm at the bottom of the centrifuge tube were transferred to the capacitation solution prepared in advance, kept at the bottom of the centrifuge tube, and the sperm capacitated for 1 h using the swim-up approach. The sperm density was increased to 1 × 106/mL, and 400 μL of each well was inserted into a four-well plate. Mature COCs were put in a four-well plate, 40 per well, and incubated in a 38°C, 5% CO2 incubator for 1.5 h. After the sperm and eggs had combined, they were preserved in 4% paraformaldehyde for 30 min. The bound sperm surrounding the oocytes were stained with DAPI and quantified by using a fluorescent inverted microscope.
The apoptosis level of blastocysts before implantation was detected using the In Situ Cell Death Detection Kit (11684795910, Roche). According to the manufacturer's instructions, briefly, the cultivated blastocysts were washed, deposited in 4% paraformaldehyde for 30 min, and permeabilized in permeabilization solution for 30 min. The embryos were transferred to a TUNEL working solution (Enzyme Solution: Label Solution = 1:9 mix) and kept in a 37°C, 5% CO2 humidified incubator for 1 h in the dark. DAPI was used to stain the nuclei and incubated at room temperature for 5 min in the dark. The blastocysts were placed on glass slides, covered with coverslips, observed under a fluorescence microscope, and photographed. The total number of cells and the number of apoptotic cells in the samples were counted and used to calculate the apoptotic rate.
Samples for immunofluorescence staining experiments were imaged using an Olympus IX81 microscope. The excitation wavelength of the fluorescence excitation block contains three sections: the ultraviolet region (340–380 nm), the blue light region (460–500 nm), and the green light region (500–570 nm). The configuration settings of the inverted microscope and the fluorescent excitation block under the same detection were consistent. Quantitative analysis was done by evaluating the fluorescence intensity of the samples using Image J (NIH, Bethesda, MD, US).
Total RNA was extracted using the Micro Elute Total RNA Kit (Omega, US). cDNA was obtained via reverse transcription using the GoScript™ Reverse Transcription Kit (Promega, US). The operational procedures are carried out in full conformity with the manufacturer's instructions. Quantitative real-time PCR (qRT-PCR) were conducted in 96-well plates using TB Green® Premix Ex Taq™ II (TaKaRa, Tokyo, Japan) and the LightCycler® 96 System (Roche, Basel, Switzerland). Reaction volume: 2 μL of cDNA, 0.8 μL (10 μM) of upstream and downstream primers, 10 μL of the enzyme, and 6.4 μL of water to make a 20 μL system. Reaction conditions: pre-deformation at 95°C for 30 s, denaturation at 95°C for 5 s, annealing at 60°C for 34 s, extension at 72°C for 30 s, 45 cycles, and chilling at 4°C. Genes mRNA levels were normalized to glyceraldehyde 3-phosphate dehydrogenase (GAPDH), and the relative mRNA expression was calculated using the 2−ΔΔCt method. All primer sequences are listed in Table 1.
Data were analyzed using SPSS 26.0 (Statistical Product and Service Solutions, US) using one-way ANOVA. Each experiment was performed at least 3 independent times. Data are presented as mean ± standard error of the mean (Mean ± SEM). Unless otherwise stated, *p < 0.05 and **p < 0.01.
After the immature COCs were cultured in the maturation solution for 24 h, the mature status of COCs was evaluated under the microscope. The COCs cultured for 24 h were digested with hyaluronidase, and after gently pipetting, naked oocytes were obtained. The exposure of the first polar body could be observed under a microscope (Figure 1A), which signifies that the oocytes had matured. The results showed that adding LIF to the maturation fluid could enhance the oocytes maturation rate (Figure 1B). The first polar body rate of oocytes in the 50 ng/mL LIF-treated group was significantly higher than that in the other three groups (control group, 25 ng/mL LIF-treated group, and 100 ng/mL LIF-treated group, p < 0.01), meanwhile which in the 25 ng/mL LIF-treated group was significantly higher than that in the control group (p < 0.05). The first polar body rate of oocytes in the 100 ng/mL LIF-treated group was not significantly different from that in the control group (p > 0.05).
Figure 1. Effects of different concentrations of LIF (0, 25, 50, and 100 ng/mL) on yak oocytes in vitro maturation. (A) Yak oocytes growth status. Yak cumulus oocyte complexes were matured and cultured for 24 h in vitro, and the cumulus cells were digested to reveal the growth state of naked oocytes. Bar = 100 μm. (B) Statistics of yak oocyte maturation rate. The first polar body burst rate of oocytes in each experimental group was recorded and counted under the microscope, and the data was expressed as Mean ± SEM. * or ** on the bar graph indicates a significant difference (*p < 0.05 and **p < 0.01).
By examining the distribution of mitochondria in oocytes, the results showed that LIF could improve the distribution of oocytes mitochondria (Figure 2A). The fluorescence intensity of oocytes mitochondria in the LIF-treated group was significantly higher than that in the control group (p < 0.01, Figure 2B), and the fluorescence intensity of oocytes mitochondria in the 50 ng/mL LIF-treated group was also significantly higher than that in the 25 ng/mL LIF-treated group and the 100 ng/mL LIF-treated group (p < 0.01, Figure 2B).
Figure 2. Effects of different concentrations of LIF on the distribution and expression of mitochondria in yak oocytes. (A) Effects of LIF on mitochondrial distribution in oocytes. Bar = 50 μm. (B) Analysis of the intensity of mitochondrial fluorescence in oocytes in each experimental group. Data results are presented as Mean ± SEM. **On the histogram indicates a significant difference (**p < 0.01).
The results revealed that adding varying amounts of LIF to the COCs maturation medium could diminish the production of ROS in oocytes (Figure 3A). The production of ROS in oocytes in the LIF-treated group was significantly lower than that in the control group (p < 0.01), and which in the 50 ng/mL LIF-treated group was also significantly lower than that in the 25 ng/mL LIF-treated group and the 100 ng/mL LIF-treated group (p < 0.01, Figure 3B).
Figure 3. Effects of varying doses of LIF on ROS in yak oocytes. (A) Effect of LIF on ROS in oocytes. Bar = 50 μm. (B) Examination of the intensity of ROS fluorescence in oocytes in each experimental group. Data results are presented as Mean ± SEM. **On the histogram shows a significant difference (**p < 0.01).
Using the Phalloidin-iFluor™ 594 Conjugate Kit, the results showed that LIF could improve oocytes actin integrity after adding various amounts of LIF to the COCs mature culture media (Figure 4A). The expression of actin in oocytes in the LIF-treated group was significantly higher than that in the control group (p < 0.01), and the expression of actin in oocytes in the 50 ng/mL LIF-treated group was significantly higher than that in the 25 ng/mL LIF treatment group and 100 ng/mL LIF-treated group (p < 0.01, Figure 4B).
Figure 4. Effects of various doses of LIF on oocytes actin in yak oocytes. (A) Effects of LIF on oocytes actin integrity. Bar = 50 μm. (B) Analysis of oocytes actin fluorescence intensity in each experimental group. Data findings are reported as Mean ± SEM. **On the histogram shows a significant difference (**p < 0.01).
Using Annexin V-FITC apoptosis detection kit, the presence of fluorescent signals on the cell membrane of oocytes is considered to that the early apoptosis occurs in oocytes (Figure 5A). The findings demonstrated that the addition of various quantities of LIF may lower the apoptosis level of oocytes (Figure 5B). The apoptosis levels of the oocytes in the 25 ng/mL LIF-treated group and the 100 ng/mL LIF-treated group were significantly lower than those in the control group (p < 0.05, Figure 5B). The apoptosis level of oocytes in the 50 ng/mL LIF treatment group was significantly lower than that in the control group (p < 0.01, Figure 5B), and also significantly lower than that in the 25 ng/mL LIF-treated and the 100 ng/mL LIF-treated groups (p < 0.05, Figure 5B).
Figure 5. Effects of different concentrations of LIF on yak oocytes early apoptosis. (A) Effects of LIF on oocyte membrane apoptosis. Bar = 50 μm. (B) Analysis of the oocyte membrane's apoptosis fluorescence intensity in each experimental group. Data results are presented as Mean ± SEM. * or ** on the bar graph indicates a significant difference (*p < 0.05 and **p < 0.01).
Through the in vitro COCs-sperm binding experiment, after adding various doses of LIF to the COCs mature culture medium, the binding capacity of oocytes and sperm was enhanced (Figure 6A). The number of sperm bound to oocytes in the 25 ng/mL LIF-treated group was significantly higher than that in the control group (p < 0.01, Figure 6B), and the number of sperm bound to oocytes in the 100 ng/mL LIF-treated group was also significantly higher than that in the control group (p < 0.05, Figure 6B). The number of spermatozoa binding to oocytes in the 50 ng/mL LIF-treated group was significantly higher than that in the control, 25 ng/mL LIF-treated and 100 ng/mL LIF-treated groups (p < 0.01, Figure 6B).
Figure 6. Effects of different concentrations of LIF on the binding capacity of yak oocytes and sperm. (A) Effects of LIF on oocytes and sperm binding capacity. Bar = 50 μm. (B) Analysis of the number of oocytes-bound sperm in each experimental group. Data results are presented as Mean ± SEM. * or ** on the bar graph indicates significant difference (*p < 0.05 and **p < 0.01).
Using the In Situ Cell Death Detection Kit, the presence of fluorescent signals on the nucleus of the blastocyst is considered to that apoptosis occurs in the blastocyst.. Oocytes treated with different concentrations of LIF could reduce the apoptosis level of subsequent blastocysts (Figure 7A). The blastocysts apoptosis rate of each experimental group was counted and the results showed that the blastocyst apoptotic rates in the 25 ng/mL LIF-treated group and the 100 ng/mL LIF-treated group were significantly lower than those in the control group (p < 0.01, Figure 7B). The apoptotic rates of blastocysts in the 50 ng/mL LIF-treated group were significantly lower than those in the control, 25 ng/mL LIF-treated and 100 ng/mL LIF-treated groups (p < 0.01, Figure 7B).
Figure 7. Effects of LIF addition during IVM on the level of apoptosis and total cell number in parthenogenetically activated blastocysts. (A) Effects of LIF on blastocysts. Bar = 50 μm. (B) Analysis of blastocysts apoptosis rate after parthenogenetic activation of oocytes in each experimental group. (C) Statistics of the total number of blastocysts after parthenogenetic activation of oocytes in each experimental group. Data results are expressed as Mean ± SEM. **On the histogram indicates significant difference (**p < 0.01).
The total cell number of blastocysts in each experimental group was counted and the results revealed that the total cell number of blastocysts in the 25 ng/mL LIF-treated and the 50 ng/mL LIF-treated groups were significantly higher than that in the control group (p < 0.01, Figure 7C). However, the total cell number of blastocysts in the 100 ng/mL LIF treatment group was not significantly different from that in the control group (p > 0.05, Figure 7C). The total cell number of blastocysts in the 50 ng/mL LIF-treated group was extremely higher than that in the 25 ng/mL LIF-treated (p < 0.01) and the 100 ng/mL LIF-treated groups (p < 0.01, Figure 7C).
In this study, qRT-PCR was used to assess the mRNA expression levels of oocytes apoptosis-related genes and antioxidant-related genes (Figures 8A–G). The results showed that the mRNA expression of Bax in oocytes in the 25 ng/mL LIF-treated and the 50 ng/mL LIF-treated groups were not significantly different from that in the control group (p > 0.05), but the mRNA expression of Bax in oocytes in the 100 ng/mL LIF-treated group was significantly higher than that in the control group (p < 0.01). The mRNA expression of Bax in oocytes in the 50 ng/mL LIF-treated group was significantly lower than that in the 25 ng/mL LIF-treated group (p < 0.05). The expression of Bcl-2 mRNA in oocytes in the 50 ng/mL LIF-treated group was substantially greater than that in the control group (p < 0.01), while the expression of Bcl-2 mRNA in oocytes in the 100 ng/mL LIF-treated group was significantly higher than that in the control group (p < 0.05), whereas the expression of Bcl-2 mRNA in oocytes in the 25 ng/mL LIF treatment group was not statistically different from that in the control group (p > 0.05). The Bax/Bcl-2 mRNA expression ratio of oocytes in the 50 ng/mL LIF-treated group was significantly lower than that in the control group (p < 0.01), and the Bax/Bcl-2 mRNA expression ratio in oocytes in the 25 ng/mL LIF-treated and 100 ng/mL LIF-treated groups was not significantly different from the control group (p > 0.05). The expression of Caspase-3 mRNA in oocytes of the 25 ng/mL LIF-treated and the 50 ng/mL LIF-treated groups was significantly lower than that of the control group (p < 0.01, p < 0.05), but the expression of Caspase-3 mRNA of oocytes in the 100 ng/mL LIF-treated group was significantly higher than that of the control group (p < 0.01). The mRNA expression of Survivin in oocytes in the 50 ng/mL LIF-treated and the 100 ng/mL LIF-treated groups was significantly higher than that in the control group (p < 0.01), while the mRNA expression of Survivin in oocytes in the 25 ng/mL LIF-treated group was significantly higher than that in the control group (p < 0.05). The expression of SOD2 mRNA in oocytes in the 50 ng/mL LIF-treated and the 100 ng/mL LIF-treated groups was significantly higher than that in the control group (p < 0.01, p < 0.05), but the expression of SOD2 mRNA in oocytes in the 25 ng/mL LIF-treated group was not significantly different from the control group (p > 0.05). The expression of Gpx4 mRNA in oocytes of the 25 ng/mL LIF-treated group, 50 ng/mL LIF-treated group, and 100 ng/mL LIF-treated group was significantly higher than that of the control group (p < 0.01).
Figure 8. Effects of varying doses of LIF on the mRNA expression levels of yak oocyte-related genes. (A–E) Apoptosis-related gene expression [(A) Bax, (B) Bcl-2, (C) Bax/Bcl-2, (D) Caspase-3, (E) Survivin]. (F,G) Antioxidant-related gene expression [(F) SOD2, (G) Gpx4]. Data results are reported as Mean ± SEM. * or ** on the bar graph shows that the findings are substantially different (*p < 0.05 and **p < 0.01).
Previous studies have demonstrated that LIF may enhance the developing competence of oocytes in mammals such as mice, pigs, cattle and sheep (24, 26, 33, 34). Therefore, we hypothesized that LIF could improve the development and maturation of yak oocytes. To prove this hypothesis, we examined the rate of the first polar body of oocytes and the ability of sperm-egg binding, which are important indicators of oocyte quality and viability and the key steps of fertilization (35). The results showed that LIF could improve the quality of yak oocytes in vitro maturation. Meanwhile, the effect of 50 ng/mL LIF on oocytes was significantly higher than that of 25 ng/mL LIF and 100 ng/mL LIF. Therefore, we concluded that yak oocytes have different sensitivity to different concentrations of LIF. We then examined the quality of subsequent blastocysts, another indicator of oocytes quality. The results showed that the blastocysts after parthenogenetic activation of oocytes treated with 50 ng/mL LIF had higher total cell number and lower apoptosis rate than those treated with 25 ng/mL and 100 ng/mL LIF, which proved the previous conclusion.
To explore the potential regulatory mechanism, we examined the effects of LIF on oocytes early apoptosis, genes, mitochondria, ROS and actin. The findings of this experiment revealed that LIF could lower the apoptosis level of oocytes membranes to promote the maturation and development of oocytes. In addition, the gene expression data showed that LIF might regulate the mRNA expression of apoptosis-related genes (Bax, Bcl-2, Survivin, Caspase-3) to reduce the early apoptosis level of oocytes. Moreover, LIF could raise the mRNA expression levels of oocyte antioxidant genes (SOD2, Gpx4) to resist oxidative stress damage to oocytes. Therefore, LIF could regulate genes expression to improve oocytes maturation and development in vitro.
The findings of this experiment demonstrated that LIF could modify the distribution of mitochondria in yak oocytes and increase their amount. Oocytes are the biggest cells in mammals. Mitochondria are key organelles producing energy inside the cell (8), occupy a large fraction of the cytoplasm of the oocyte (36) and affect the developmental potential of the oocytes (37). Therefore, impaired mitochondrial activity lowers oocytes developmental ability (38). Mitochondrial supplementation in human, mouse, porcine, and bovine oocytes is found to increase egg quality and in vitro fertilization success (39–41), and restores preimplantation embryonic developmental competence (38). The quantity of mitochondrial storage directly impacts the amount of ATP. Numerous events such as transcription, translation, and spindle assembly during oocytes maturation, fertilization, and preimplantation embryos development require considerable quantities of ATP (36, 42). Decreased ATP generation may impair processes such as cell cycle regulation, spindle formation, fertilization, and preimplantation embryos development (36). Furthermore, mitochondria may mediate the endogenous apoptotic pathway in mammalian oocytes (43). Mitochondria play a crucial role in apoptosis by modulating signaling molecules such as calcium Ca2+, cyclic adenosine monophosphate (cAMP), and ROS (5, 12). Calcium and Bcl-2 family proteins are involved in the process of apoptosis (44). When calpain is activated, intracellular Bcl-2 protein expression drops, which in turn stimulates mitochondrial apoptosis (13). Therefore, we concluded that LIF can improve the quality and developmental ability of oocytes by regulating the function of mitochondria, and then affect the quality of subsequent blastocysts in vitro.
The findings of this experiment demonstrated that LIF could lower the production of ROS in yak oocytes and maintain a reasonably low level of ROS. ROS is a product of oocyte metabolism, and excess ROS may contribute to oxidative stress (5). ROS are also crucial signal transduction molecules that play a critical role in regulating cellular metabolic processes by oxidizing protein cysteine residues to promote proliferation, differentiation, and migration (36). Oxidative stress may oxidize RNA, DNA, and proteins, altering the structure of cell membranes and consequently affecting oocyte development (45). In addition, ROS are very directly associated with the activation of mitochondrial pathways (44). Most of the intracellular ROS is created owing to leakage of the respiratory electron transport chain (46). ROS damage mitochondrial DNA, which in turn impairs the transcription of mtRNA proteins involved in the electron transport chain, leading to respiratory chain dysfunction and further production of ROS, resulting in loss of mitochondrial membrane potential and impairment of ATP synthesis (44), which in turn affects oocyte and embryonic developmental capacity (47, 48). Therefore, we concluded that LIF can reduce the level of ROS and resist the damage of oxidative stress to mitochondria, RNA, DNA and proteins in oocytes, thereby promoting the maturation and development of oocytes in vitro.
The findings of this experiment revealed that LIF could enhance the integrity of actin and raise the amount of actin. During mammalian oocytes maturation, it is required for the oocytes to undergo two asymmetric divisions (49). This asymmetric division protects the cytoplasm, comprising essential resources such as mRNA, proteins, and mitochondria, to promote early embryonic development (21). Actin plays a crucial function in this asymmetric division. Firstly, actin plays a vital function in spindle movement and cortical polarization during oocyte meiosis (50). Secondly, in a mouse model, dynamic actin filaments around the meiotic spindle push mitochondria, thus producing a counterforce to spindle migration in oocytes (51). Therefore, we concluded that LIF can affect actin to ensure the normal meiosis of oocytes, thereby promoting the development of oocytes in vitro.
In summary, the results of this study showed that adding LIF (50 ng/mL) treatment during the in vitro maturation of yak oocytes could enhance mitochondrial and actin levels in oocytes, reduce ROS and apoptosis levels and regulate the mRNA levels of related genes, thereby promoting the maturation rate of oocytes and their ability to combine with sperm under in vitro culture conditions. In addition, LIF-treated oocytes could lower the apoptosis rate of subsequent blastocysts and increase the total cell number of blastocysts.
This study explored the effect of LIF on the in vitro maturation of yak oocytes. LIF decreased oocyte apoptosis by modulating mitochondria, oxidative stress and actin levels in yak oocytes, consequently enhancing the quality of yak oocytes, promoting the in vitro maturation and development of yak oocytes and the quality of subsequent blastocysts. The result of this study provided some supportive insights into the role and function of LIF in vitro yak oocytes maturation, as well as provided fundamental knowledge for assisted reproductive technologies in the yak.
The original contributions presented in the study are included in the article/supplementary material, further inquiries can be directed to the corresponding author/s.
The animal study was reviewed and approved by the Experimental Animal Ethics Committee of Gansu Agricultural University (Ethic approval file No. GSAU-Eth-VMC-2022-23).
TZhao, YP, YC, and SY came up with and designed the study. JW and TZhan collected samples. TZhao, QL, and TD performed the experiments. TZhao, YW, LZ, and XH performed the statistical analysis. TZhao wrote the manuscript. YP, RN, AB, and SY revised the manuscript. All authors contributed to the article and approved the submitted version.
This research was funded by the National Natural Science Foundation of China (Grant Nos. 31972760 and 32160850), the Fund for Distinguished Young Scholars of Gansu Province (Grant No. 20JR10RA561), the Science and Technology Major Project of Gansu Province (Grant No. 21ZD10NA001), the Seed Industry Research Project of Gansu Province (Grant No. GZGG-2021-1), and the Key Talent Project of Gansu Province (2022-0623-RCC-0307).
The authors declare that the research was conducted in the absence of any commercial or financial relationships that could be construed as a potential conflict of interest.
All claims expressed in this article are solely those of the authors and do not necessarily represent those of their affiliated organizations, or those of the publisher, the editors and the reviewers. Any product that may be evaluated in this article, or claim that may be made by its manufacturer, is not guaranteed or endorsed by the publisher.
1. Zi XD. Reproduction in female yaks (Bos Grunniens) and opportunities for improvement. Theriogenology. (2003) 59:1303–12. doi: 10.1016/S0093-691X(02)01172-X
2. Qiu Q, Zhang G, Ma T, Qian W, Wang J, Ye Z, et al. The yak genome and adaptation to life at high altitude. Nat Genet. (2012) 44:946–9. doi: 10.1038/ng.2343
3. Yu SJ, Li FD. Profiles of plasma progesterone before and at the onset of puberty in yak heifers. Anim Reprod Sci. (2001) 65:67–73. doi: 10.1016/S0378-4320(00)00216-5
4. Pan Y, Cui Y, Baloch AR, Fan J, He J, Li G, et al. Insulinlike growth factor i improves yak (bos grunniens) spermatozoa motility and the oocyte cleavage rate by modulating the expression of bax and Bcl-2. Theriogenology. (2015) 84:756–62. doi: 10.1016/j.theriogenology.2015.05.007
5. Tiwari M, Prasad S, Tripathi A, Pandey AN, Ali I, Singh AK, et al. Apoptosis in mammalian oocytes: a review. Apoptosis. (2015) 20:1019–25. doi: 10.1007/s10495-015-1136-y
6. Lord T, Aitken RJ. Oxidative stress and ageing of the post-ovulatory oocyte. Reproduction. (2013) 146:R217–27. doi: 10.1530/REP-13-0111
7. Schatten H, Sun QY, Prather R. The impact of mitochondrial function/dysfunction on Ivf and new treatment possibilities for infertility. Reprod Biol Endocrinol. (2014) 12:111. doi: 10.1186/1477-7827-12-111
8. Spinelli JB, Haigis MC. The multifaceted contributions of mitochondria to cellular metabolism. Nat Cell Biol. (2018) 20:745–54. doi: 10.1038/s41556-018-0124-1
9. D'Aquila P, Bellizzi D, Passarino G. Mitochondria in health, aging and diseases: the epigenetic perspective. Biogerontology. (2015) 16:569–85. doi: 10.1007/s10522-015-9562-3
10. Matilainen O, Quirós PM, Auwerx J. Mitochondria and epigenetics- crosstalk in homeostasis and stress. Trends Cell Biol. (2017) 27:453–63. doi: 10.1016/j.tcb.2017.02.004
11. Ge ZJ, Schatten H, Zhang CL, Sun QY. Oocyte ageing and epigenetics. Reproduction. (2015) 149:R103–14. doi: 10.1530/REP-14-0242
12. Qi L, Chen X, Wang J, Lv B, Zhang J, Ni B, et al. Mitochondria: the panacea to improve oocyte quality? Ann Transl Med. (2019) 7:789. doi: 10.21037/atm.2019.12.02
13. Redza-Dutordoir M, Averill-Bates DA. Activation of apoptosis signalling pathways by reactive oxygen species. Biochim Biophys Acta. (2016) 1863:2977–92. doi: 10.1016/j.bbamcr.2016.09.012
14. Schieber M, Chandel NS. Ros function in redox signaling and oxidative stress. Curr Biol. (2014) 24:R453–62. doi: 10.1016/j.cub.2014.03.034
15. Covarrubias L, Hernández-García D, Schnabel D, Salas-Vidal E, Castro-Obregón S. Function of reactive oxygen species during animal development: passive or active? Dev Biol. (2008) 320:1–11. doi: 10.1016/j.ydbio.2008.04.041
16. Halliwell B. Free radicals and antioxidants - quo vadis? Trends Pharmacol Sci. (2011) 32:125–30. doi: 10.1016/j.tips.2010.12.002
17. Chaube SK, Prasad PV, Thakur SC, Shrivastav TG. Hydrogen peroxide modulates meiotic cell cycle and induces morphological features characteristic of apoptosis in rat oocytes cultured in vitro. Apoptosis. (2005) 10:863–74. doi: 10.1007/s10495-005-0367-8
18. Takahashi T, Takahashi E, Igarashi H, Tezuka N, Kurachi H. Impact of oxidative stress in aged mouse oocytes on calcium oscillations at fertilization. Mol Reprod Dev. (2003) 66:143–52. doi: 10.1002/mrd.10341
19. Dominguez R, Holmes KC. Actin structure and function. Annu Rev Biophys. (2011) 40:169–86. doi: 10.1146/annurev-biophys-042910-155359
20. Duan X, Sun SC. Actin cytoskeleton dynamics in mammalian oocyte meiosis. Biol Reprod. (2019) 100:15–24. doi: 10.1093/biolre/ioy163
21. Uraji J, Scheffler K, Schuh M. Functions of actin in mouse oocytes at a glance. J Cell Sci. (2018) 131:218099. doi: 10.1242/jcs.218099
22. Rose-John S. Interleukin-6 family cytokines. Cold Spring Harb Perspect Biol. (2018) 10:a028415. doi: 10.1101/cshperspect.a028415
23. Nilsson EE, Kezele P, Skinner MK. Leukemia inhibitory factor (Lif) promotes the primordial to primary follicle transition in rat ovaries. Mol Cell Endocrinol. (2002) 188:65–73. doi: 10.1016/S0303-7207(01)00746-8
24. Cadoret V, Jarrier-Gaillard P, Papillier P, Monniaux D, Guérif F, Dalbies-Tran R. Leukaemia inhibitory factor modulates the differentiation of granulosa cells during sheep in vitro preantral to antral follicle development and improves oocyte meiotic competence. Mol Hum Reprod. (2021) 27:gaab051. doi: 10.1093/molehr/gaab051
25. Mo X, Wu G, Yuan D, Jia B, Liu C, Zhu S, et al. Leukemia inhibitory factor enhances bovine oocyte maturation and early embryo development. Mol Reprod Dev. (2014) 81:608–18. doi: 10.1002/mrd.22327
26. Vendrell-Flotats M, García-Martínez T, Martínez-Rodero I, Lopez-Bejar M, LaMarre J, Yeste M, et al. In vitro maturation with leukemia inhibitory factor prior to the vitrification of bovine oocytes improves their embryo developmental potential and gene expression in oocytes and embryos. Int J Mol Sci. (2020) 21:7067. doi: 10.3390/ijms21197067
27. He H, Zhang H, Li Q, Fan J, Pan Y, Zhang T, et al. Low oxygen concentrations improve yak oocyte maturation and enhance the developmental competence of preimplantation embryos. Theriogenology. (2020) 156:46–58. doi: 10.1016/j.theriogenology.2020.06.022
28. Li Q, Pan Y, He H, Hu X, Zhao T, Jiang J, et al. DNA methylation regulated by ascorbic acids in yak preimplantation embryo helps to improve blastocyst quality. Mol Reprod Dev. (2019) 86:1138–48. doi: 10.1002/mrd.23230
29. Szymanska KJ, Ortiz-Escribano N, Van den Abbeel E, Van Soom A, Leybaert L. Connexin hemichannels and cell death as measures of bovine coc vitrification success. Reproduction. (2019) 157:87–99. doi: 10.1530/REP-18-0387
30. Ortiz-Escribano N, Smits K, Piepers S, Van den Abbeel E, Woelders H, Van Soom A. Role of cumulus cells during vitrification and fertilization of mature bovine oocytes: effects on survival, fertilization, and blastocyst development. Theriogenology. (2016) 86:635–41. doi: 10.1016/j.theriogenology.2016.02.015
31. Miao Y, Zhou C, Bai Q, Cui Z, ShiYang X, Lu Y, et al. The protective role of melatonin in porcine oocyte meiotic failure caused by the exposure to Benzo(a)Pyrene. Hum Reprod. (2018) 33:116–27. doi: 10.1093/humrep/dex331
32. Li WD, Yu S, Luo SM, Shen W, Yin S, Sun QY. Melatonin defends mouse oocyte quality from benzo[ghi]perylene-induced deterioration. J Cell Physiol. (2019) 234:6220–9. doi: 10.1002/jcp.27351
33. De Matos DG, Miller K, Scott R, Tran CA, Kagan D, Nataraja SG, et al. Leukemia inhibitory factor induces cumulus expansion in immature human and mouse oocytes and improves mouse two-cell rate and delivery rates when it is present during mouse in vitro oocyte maturation. Fertil Steril. (2008) 90:2367–75. doi: 10.1016/j.fertnstert.2007.10.061
34. Wasielak M, Wiesak T, Bogacka I, Jalali BM, Bogacki M. Maternal effect gene expression in porcine metaphase ii oocytes and embryos in vitro: effect of epidermal growth factor, interleukin-1β and leukemia inhibitory factor. Zygote. (2017) 25:120–30. doi: 10.1017/S0967199416000332
35. Aydin H, Sultana A, Li S, Thavalingam A, Lee JE. Molecular architecture of the human sperm izumo1 and egg juno fertilization complex. Nature. (2016) 534:562–5. doi: 10.1038/nature18595
36. van der Reest J, Nardini Cecchino G, Haigis MC, Kordowitzki P. Mitochondria: their relevance during oocyte ageing. Ageing Res Rev. (2021) 70:101378. doi: 10.1016/j.arr.2021.101378
37. Cecchino GN, Seli E, Alves da Motta EL, García-Velasco JA. The role of mitochondrial activity in female fertility and assisted reproductive technologies: overview and current insights. Reprod Biomed Online. (2018) 36:686–97. doi: 10.1016/j.rbmo.2018.02.007
38. Chiaratti MR, Ferreira CR, Perecin F, Méo SC, Sangalli JR, Mesquita LG, et al. Ooplast-Mediated developmental rescue of bovine oocytes exposed to ethidium bromide. Reprod Biomed Online. (2011) 22:172–83. doi: 10.1016/j.rbmo.2010.10.011
39. Yi YC, Chen MJ, Ho JY, Guu HF, Ho ES. Mitochondria transfer can enhance the murine embryo development. J Assist Reprod Genet. (2007) 24:445–9. doi: 10.1007/s10815-007-9161-6
40. El Shourbagy SH, Spikings EC, Freitas M, St John JC. Mitochondria directly influence fertilisation outcome in the pig. Reproduction. (2006) 131:233–45. doi: 10.1530/rep.1.00551
41. Labarta E, de Los Santos MJ, Escribá MJ, Pellicer A, Herraiz S. Mitochondria as a tool for oocyte rejuvenation. Fertil Steril. (2019) 111:219–26. doi: 10.1016/j.fertnstert.2018.10.036
42. Eichenlaub-Ritter U, Staubach N, Trapphoff T. Chromosomal and cytoplasmic context determines predisposition to maternal age-related aneuploidy: brief overview and update on mcak in mammalian oocytes. Biochem Soc Trans. (2010) 38:1681–6. doi: 10.1042/BST0381681
43. Aitken RJ, Findlay JK, Hutt KJ, Kerr JB. Apoptosis in the germ line. Reproduction. (2011) 141:139–50. doi: 10.1530/REP-10-0232
44. Orrenius S, Gogvadze V, Zhivotovsky B. Calcium and mitochondria in the regulation of cell death. Biochem Biophys Res Commun. (2015) 460:72–81. doi: 10.1016/j.bbrc.2015.01.137
45. Rodríguez-Varela C, Labarta E. Clinical application of antioxidants to improve human oocyte mitochondrial function: a review. Antioxidants (Basel). (2020) 9:1197. doi: 10.3390/antiox9121197
46. Dickinson BC, Chang CJ. Chemistry and biology of reactive oxygen species in signaling or stress responses. Nat Chem Biol. (2011) 7:504–11. doi: 10.1038/nchembio.607
47. Van Blerkom J, Davis P. Mitochondrial signaling and fertilization. Mol Hum Reprod. (2007) 13:759–70. doi: 10.1093/molehr/gam068
48. Van Blerkom J. Mitochondrial function in the human oocyte and embryo and their role in developmental competence. Mitochondrion. (2011) 11:797–813. doi: 10.1016/j.mito.2010.09.012
49. Sun SC, Kim NH. Molecular mechanisms of asymmetric division in oocytes. Microsc Microanal. (2013) 19:883–97. doi: 10.1017/S1431927613001566
50. Sun QY, Schatten H. Regulation of dynamic events by microfilaments during oocyte maturation and fertilization. Reproduction. (2006) 131:193–205. doi: 10.1530/rep.1.00847
Keywords: leukemia inhibitory factor, oocyte, mitochondria, reactive oxygen species, actin, apoptosis, blastocyst quality
Citation: Zhao T, Pan Y, Li Q, Ding T, Niayale R, Zhang T, Wang J, Wang Y, Zhao L, Han X, Baloch AR, Cui Y and Yu S (2022) Leukemia inhibitory factor enhances the development and subsequent blastocysts quality of yak oocytes in vitro. Front. Vet. Sci. 9:997709. doi: 10.3389/fvets.2022.997709
Received: 19 July 2022; Accepted: 22 August 2022;
Published: 21 September 2022.
Edited by:
Fan Yang, Jiangxi Agricultural University, ChinaCopyright © 2022 Zhao, Pan, Li, Ding, Niayale, Zhang, Wang, Wang, Zhao, Han, Baloch, Cui and Yu. This is an open-access article distributed under the terms of the Creative Commons Attribution License (CC BY). The use, distribution or reproduction in other forums is permitted, provided the original author(s) and the copyright owner(s) are credited and that the original publication in this journal is cited, in accordance with accepted academic practice. No use, distribution or reproduction is permitted which does not comply with these terms.
*Correspondence: Sijiu Yu, c2lqaXV5QDEyNi5jb20=
Disclaimer: All claims expressed in this article are solely those of the authors and do not necessarily represent those of their affiliated organizations, or those of the publisher, the editors and the reviewers. Any product that may be evaluated in this article or claim that may be made by its manufacturer is not guaranteed or endorsed by the publisher.
Research integrity at Frontiers
Learn more about the work of our research integrity team to safeguard the quality of each article we publish.