- 1Instituto Nacional de Investigación Agropecuaria (INIA), Plataforma de Salud Animal, Estación experimental INIA Tacuarembó, Tacuarembó, Uruguay
- 2Laboratorio Regional Noroeste “Miguel C. Rubino”, División de Laboratorios Veterinarios “Miguel C. Rubino”, Paysandú, Uruguay
- 3Laboratorio de Vectores y enfermedades transmitidas, Departamento de Ciencias Biológicas, CENUR Litoral Norte - Salto, Universidad de la República, Salto, Uruguay
- 4Unidad de Genética y Bioinformática, Departamento de Ciencias Biológicas, CENUR Litoral Norte - Salto, Universidad de la República, Salto, Uruguay
The major surface protein 1a (MSP1a) gene has been used to characterize Anaplasma marginale genetic diversity. This pathogen causes significant productivity and economic losses to the cattle industry. The objective of the present study was to report the first characterization of A. marginale genetic diversity in Uruguay based on MSP1a genotypes and their putative relationship with Rhipicephalus microplus. This cross-sectional study was conducted between 2016 and 2020. The study included whole blood samples from clinical cases of bovine anaplasmosis obtained from 30 outbreaks located in six Uruguay territorial departments. Diagnosis was performed using Giemsa-stained smears and confirmed by nested Polymerase Chance Reaction (nPCR) targeting the A. marginale major surface protein 5 gene. The genetic diversity of A. marginale strains was characterized by analyzing the microsatellite and tandem repeats of MSP1a. Based on the microsatellite structure, four genotypes were identified. Genotype E was the most prevalent. Analysis of MSP1a tandem repeats showed 28 different strains from the combination of 31 repeats, with τ-10-15 and α-β-β-β-Γ being the most common. Repeats Γ, β, α, and γ were associated with the absence of R. microplus with statistical significance (p < 0.05). Molecular observations showed that 46.7% of the strains identified in our samples lacked the ability to bind to tick cells; therefore, they were probably transmitted by other vectors. Strain genetic diversity provides valuable information for understanding the epidemiological behavior of A. marginale and could contribute to the development of effective vaccines for the control of this disease.
Introduction
Bovine anaplasmosis is caused by an obligate intraerythrocytic Anaplasmataceae agents, Anaplasma marginale (1), found within membrane-bound vacuoles (1 μm in size) in the cytoplasm of host cells. This bacterium belongs to the order Rickettsiales and family Anaplasmataceae (2). Bovine anaplasmosis is widely distributed throughout the world, particularly in tropical and subtropical regions (3). It is considered a major economic and production problem for cattle in enzootic tick-infested areas (4).
Different species of Dermacentor and Rhipicephalus can biologically transmit A. marginale. However, some A. marginale strains are not infective or transmissible by ticks (5, 6). In Uruguay, the only tick species related to the transmission of A. marginale to cattle was Rhipicephalus microplus (7).
Anaplasma marginale is often transmitted mechanically to susceptible cattle by blood-contaminated mouthparts of the bloodsucking diptera of the Tabanus and Stomoxys genera, or via fomites (8). In cattle, the only site for the replication is within the erythrocytes, where it develops membrane-bound vacuoles which contain−4–8 A. marginale (9).
The prepatent period ranges from 7 to 60 days (depending on the infective dose), and as many as 70% or more of the erythrocytes may become infected during acute infections and/or during the manifestation of clinical sings (3). The animals most susceptible to developing clinical diseases are bovines older than 1 year. Severe anemia, icterus (without haemoglobinuria), fever, weight loss, lethargy, depression, and abortion were the main clinical signs observed (10). The major postmortem findings include severe haemolytic anemia, icterus, splenomegaly, hepatomegaly, and petechial hemorrhage on the serosa surface over the heart and pericardium. All tissues were pale and blood was thin and watery (11). Cattle that survive acute infections may remain carriers for life (1).
Currently, there are different strains of A. marginale worldwide, with diverse epidemiological behaviors, virulence, pathogenicity, adaptation to ecological niches and induction of the host‘s immune response (12). The major surface proteins (MSP1a, MSP 4, and MSP 5) have been used for the molecular characterization of strains (5), as these are single genes that do not vary antigenically within isolates (3). In particular, the analysis of MSP1a gene sequences provides information regarding genetic diversity, evolution of host-pathogen and vector-pathogen relationships, and transmissibility of phenotypes. Moreover, these sequences can be used to compare strains in a given region (12, 13). Furthermore, using information from the MSP1a tandem repeat, it is possible to design peptide-based vaccines (12).
Therefore, MSP1a is a stable marker of strain and has been widely used to identify different strains of A. marginale based on N-terminal variable tandem repeats (more than 300 strains characterized by tandem repeat structures) and 5′- untranslated region (UTR) microsatellite (described eleven genotypes identified by letters A to L) located in the MSP1a gene (14–16).
There are many studies around the world that report the genetic diversity of A. marginale. This diversity is higher in region where the vector R. microplus is present (14, 15, 17, 18). This situation of wide diversity is frequently reported in South American countries where they have endemic region of R. microplus, both in beef and dairy cattle production (19–22).
Currently, no studies have been carried out in Uruguay to characterize the strains of A. marginale. Therefore, the aim of the present cross-sectional study was to characterize the genetic diversity of A. marginale in clinically sick animals in Uruguay and its possible relationship with R. microplus.
Materials and methods
Study design, geographical area, and sample collection
A cross-sectional study was carried out in Uruguay, a country located in the Southern Hemisphere temperate zone between parallels 30° and 35° of the South latitude and meridians 53° and 58° of the West longitude. The region has subtropical climatic conditions, with an average annual temperature of 17.5°C and an annual average rainfall of 1,200 mm. Convenience sampling was performed between August 2016 and April 2020. Sixty blood samples from tail vein in tube with potassium EDTA3 K anticoagulant (ethylenediaminetetraacetic acid) from clinical cases of anaplasmosis were collected from animals of different ages (cows, calves, steers, and heifers). The samples were obtained from 30 farms (outbreaks) located in six of 19 Uruguayan departments: Artigas, Salto, Paysandú, Rio Negro, Soriano, and Colonia (Figure 1). Of these 30 farms, belonged: 25 beef production cross breed herd (48 samples) and five herd of dairy cattle Holland (12 samples). These samples were sent by veterinary practitioners to the laboratory of the “División Laboratorios Veterinarios” (DILAVE) Northwest region of the “Ministerio de Ganadería, Agricultura y Pesca” (MGAP), Uruguay for diagnosis. The presence or absence of R. microplus has been reported for each outbreak.
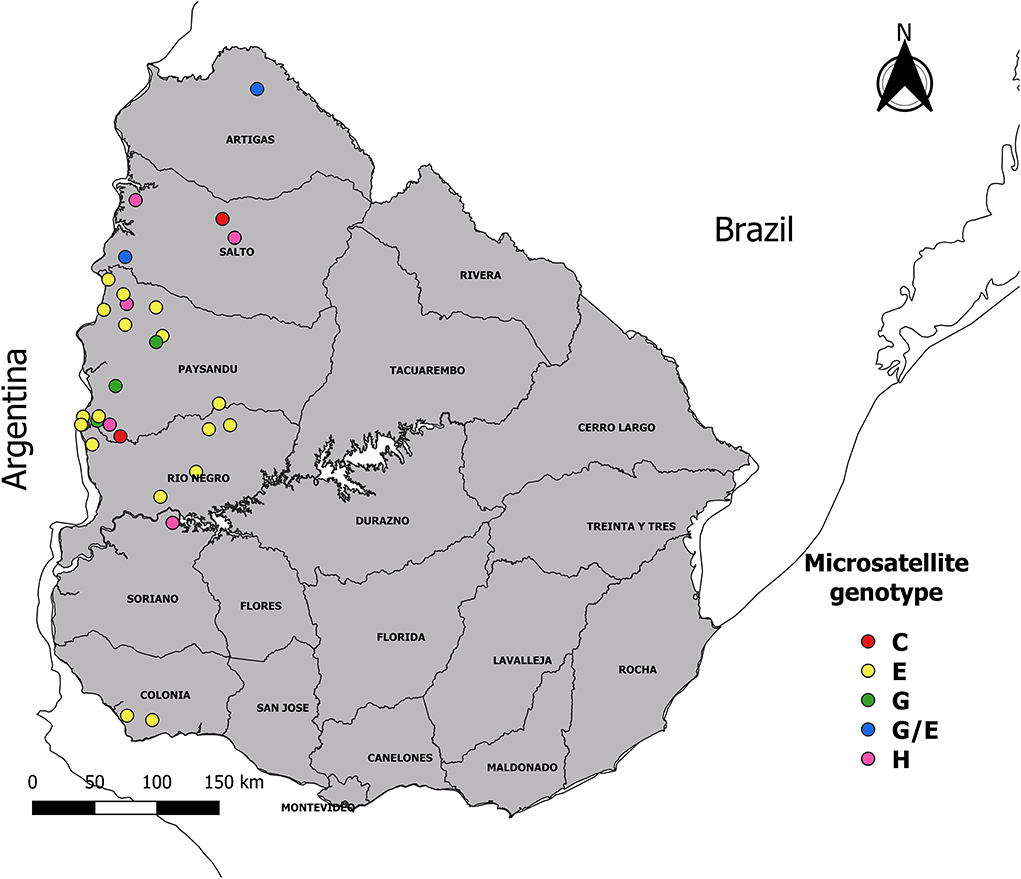
Figure 1. Map showing geographic location of the samples. The 60 samples of infected animals with Anaplasma marginale were obtained from 30 outbreaks of bovine anaplasmosis (dots with different color according to microsatellite genotype), distributed in six departments of Uruguay.
Blood samples were collected from animals with fever (>39.9°C), anemia (anemia was considered when the microhematocrit was <26%; for this microtube with blood was centrifuged at 11,800 × g for 5 min), weakness, jaundice, and pale mucosa. The average morbidity and mortality (minimum–maximum) registered in the farms where samples were collected were 5.4% (0.2%−32%) and 2.8% (0%−25.7%), respectively.
Blood smear and molecular diagnosis
To confirm clinical cases of anaplasmosis, smears and molecular detection using nested PCR (nPCR) were performed. For this, blood extracted from tail vein was used. Blood smears were fixed with methanol for 5 min and stained with Giemsa for 60 min. All smear readings were performed by the same trained technician. For a positive result, at least 5% of the parasitised erythrocytes had to be observed in 100 fields. The smears were viewed under a light microscope at 1,000× magnification. For molecular diagnosis, DNA extraction from 200 μl aliquots of blood was performed using the PureLink Genomic DNA Mini Kit (Invitrogen, USA) following the manufacturer's instructions. DNA was quantified using a NanoDrop 2000 spectrophotometre (Thermo Scientific) and stored at −20°C until further analysis. Molecular detection of A. marginale was carried out by nPCR targeting a 458 bp fragment of the MSP-5 gene, as previously described (23). In each run of the assay, a negative control (ultrapure water) and a positive control (A. marginale Paysandú isolate), previously sequenced and stored, were included.
PCR targeting MSP1a gene and sequence processing
Molecularly confirmed A. marginale samples were used to amplify a fragment of the MSP1a gene. For this purpose, a semi-nested PCR method described by Lew et al. (24) was carried out. The primers used were as follows: 1733F (5′TGTGCTTATGGCAGACATTTCC3′) and 2957R 5′AAACCTTGTAGCCCCAACTTATCC 3′) in the first step, and 1733F and 3134R (5′ TCACGGTCAAAACCTTTGCTTACC 3′) in the second round, only when the first step showed no detectable bands. Reactions were performed in a final 25 μl volume consisting of 12.5 μl of MangoMix (Bioline; Meridian Bioscience), 6.5 μl of ultrapure water, 4 μl of sample DNA solution, and 1 μl (10 pmol) of each primer. The amplification cycling following an initial denaturation at 94°C for 3 min, 30 cycles of 30 s at 94°C, 1 min at 55°C and 2 min at 72°C, followed by a final cycle with a 7 min extension step at 72°C. The amplification protocol was performed as described by Lew et al. (24). The PCR products from each reaction were run on a 1.5% agarose gel stained with GoodView nucleic acid stain (Beijing SBS Genetech) and visualized under a UV transilluminator.
Amplicons of the expected size were purified using a PureLink quick PCR purification kit (Invitrogen) and sequenced (Macrogen, Seoul, South Korea). Sequence identities were confirmed using BLASTN (http://www.ncbi.nlm.nih.gov/BLAST). The raw sequences were assembled with the software MEGA X (25). Each sequence was carefully checked, and manual corrections were performed when necessary.
Molecular characterization of the A. marginale
Anaplasma marginale strains were classified using microsatellite genotyping and tandem repeat (TR) composition (15, 26). A microsatellite is located in the 5′-UTR of the MSP1a gene between the putative Shine-Delgarno (GTAGG) sequence and the translation initiation codon (ATG), SD-ATG (5), containing the sequence structure GTAGG (G/ATTT)m (GT)n T ATG. The Shine-Delgarno and initial codon distances were calculated as (4 × m) + (2 × n) + 1, and the resulting genotypes were identified by the letters A–L (14, 16).
MSP 1a sequences were classified by TR sequence and number following the nomenclature described by de la Fuente et al. (26) and other authors (15, 16, 27). The TRs present in each sequence were classified using RepeatAnalyzer software (28). The amino acid composition of the MSP 1a repeats is described for each sample.
Phylogenetic analysis
Phylogenetic analyses were conducted using the MSP1a amino acid sequences. Theoretical translation of nucleotide sequences into amino acid sequences was performed using the ExPASy translation tool web server (https://web.expasy.org/translate/).
A set of reference sequences for phylogenetic analysis was obtained by a BLAST search of each Uruguayan sequence against the GenBank database. BLAST's top five hits by sequence (E-values < 1.0 × 10–5, >700 bp long, and with available information of country and collection date) were retrieved. The repeated sequences in the dataset were removed. This resulted in a final dataset of 114 sequences from nine countries. The amino acid sequences obtained in this study were aligned with sequences retrieved from GenBank using MAFFT v7.467 program (29) and subjected to maximum likelihood (ML) phylogenetic analysis. The ML tree was inferred with IQ-TREE 1.6.1 software (30) under the JTT+F+I+G4 amino acid replacement model selected by ModelFinder application. Branch support was assessed using the approximate likelihood-ratio test based on a Shimodaira-Hasegawa-like procedure (SH-aLRT) with 1,000 replicates (31).
Statistical analysis
The association between the TRs identified in the sample and the presence or absence of R. microplus was evaluated using the Phi correlation coefficient (ϕ) and Yates Chi2 test (X2Yates), with a 95% confidence level. The test was performed with the statistical software Statistical Package for the Social Sciences (SPSS), v. 28.0.0.0 (International Business Machines –IBM, USA).
Results
All 60 samples were smear-positive, showing more than 15% parasitised erythrocytes. In addition, all samples were molecularly confirmed as A. marginale by MSP five nPCR. Regarding animal age, the positivity distribution was 45 cows, 13 steers, one heifer, and one calf. Sixteen outbreaks were tick-free (35 animals) and 14 outbreaks had ticks (25 animals). All animals showed clinical signs of haemolytic disease.
Molecular characterization of A. marginale strains using MSP 1a
MSP1a sequence analysis showed amplicons between 670 and 1,110 bp. Based on the structure of the MSP1a microsatellite of A. marginale, four genotypes were identified. The most frequent genotype was E (45/60), followed by G (8/60), H (6/60), and C (1/60).
Tandem repeats analysis revealed 28 different genotypes were found in our 60 samples, contained between two and eight TRs.
The strains commonly observed had three (42%), five (28%), and four (10%) tandem repeats. Thirty-one TRs were found, being the most frequently identified 15, τ, 10, Γ, F, β, and α (Table 1 and Supplementary Table SI).
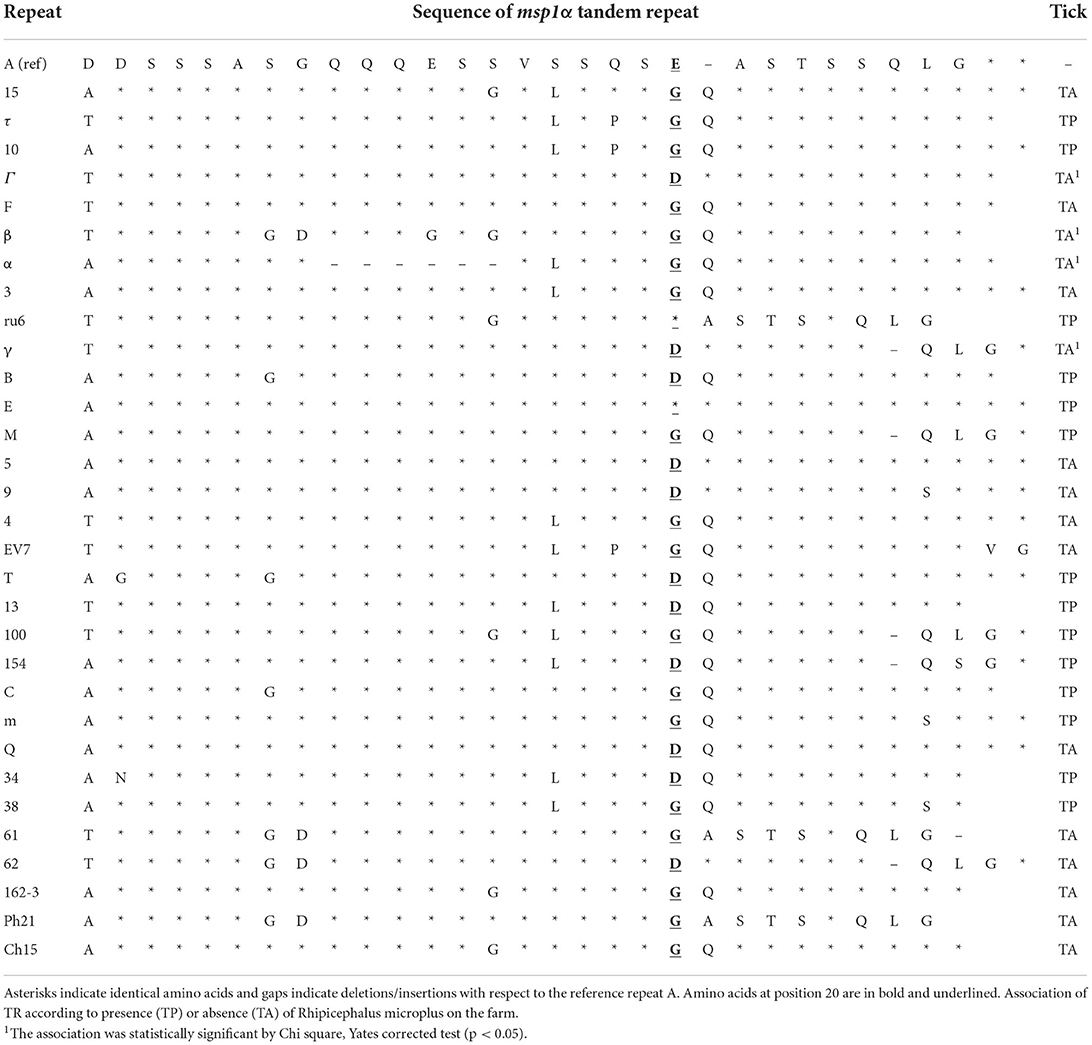
Table 1. Sequence of MSP1a tandem repeats found in the Anaplasma marginale strains of this study, ordered from highest to lowest presence.
Based on the data set provide by Repeat Analyzer we found 22 new strains. The strains τ-10-15 and α-β-β-β-Γ were most prevalent (Table 2). We found circulation of more than one genotype in ten A. marginale outbreaks (10/30).
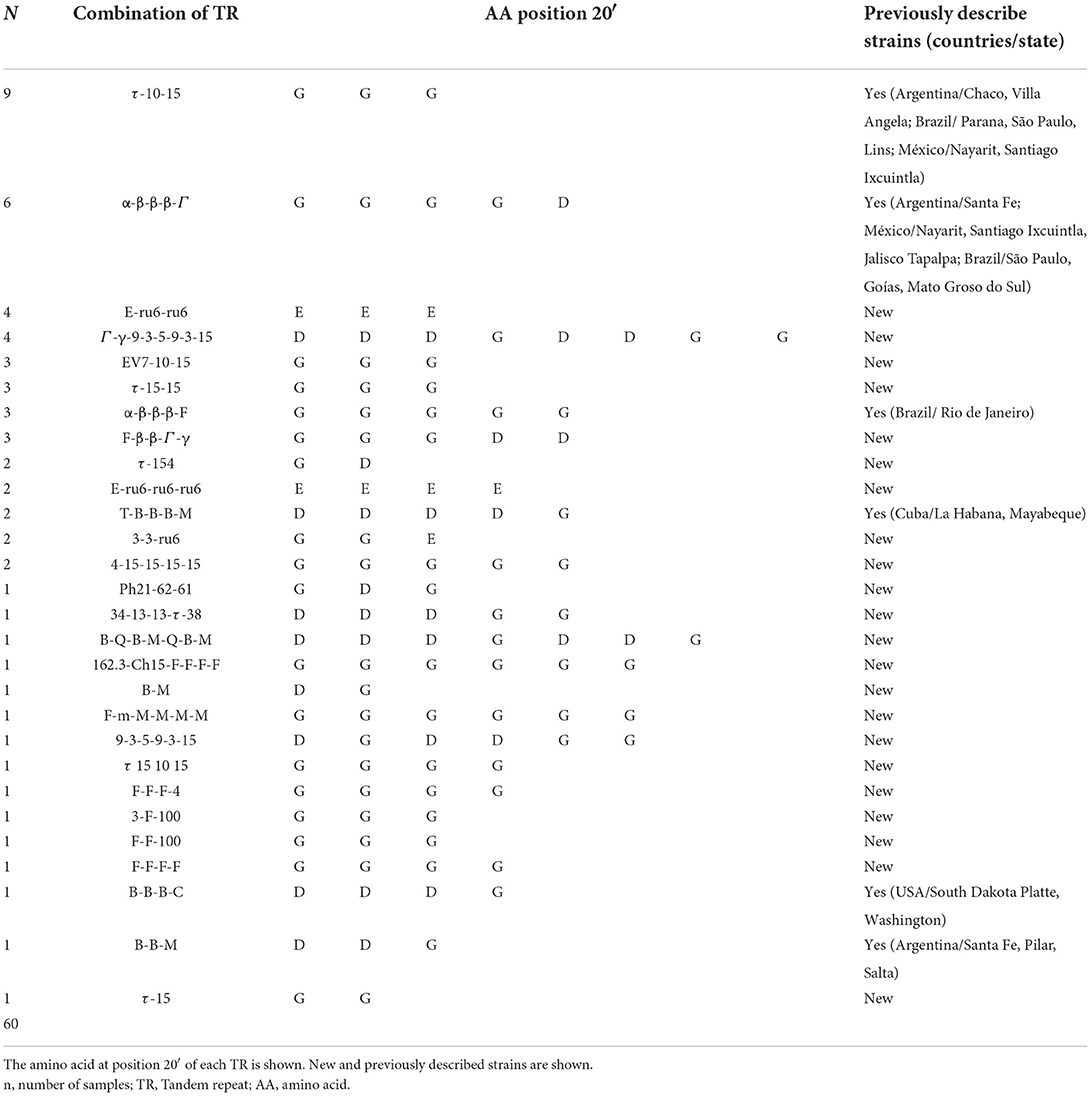
Table 2. Characterization of Anaplasma marginale strains according to the combination of tandem repeat.
In 46.7% (28/60) of our samples, the strains had the amino acid glycine (G) at position 20 of the MSP1a tandem repeat, 40% (24/60) had G and aspartate acid (D), 10% (6/60) had glutamic acid (E), and only 3.3% (2/60) had a mixture of E and G (Table 2). The data presented in the study are deposited in the Genbank repository, accession numbers OP382972-OP383031.
Phylogenetic analysis
The results of phylogenetic analysis using MSP 1a amino acid sequences demonstrated the heterogeneity of the strains found in this study. Most of our sequences were closely related to sequences from Brazil. The phylogenetic tree showed a cluster that agreed with the structure of the tandem repeats. A large part of our sample was grouped into five clusters related to the tandem repeats β, B, τ, Γ, and E. Twelve, 5, 16, 5, and 6 samples were grouped based on the tandem repeats β, B, τ, Γ, and E, respectively, with 93, 97.4, 92.8, 99.7, and 100 support values (Figure 2).
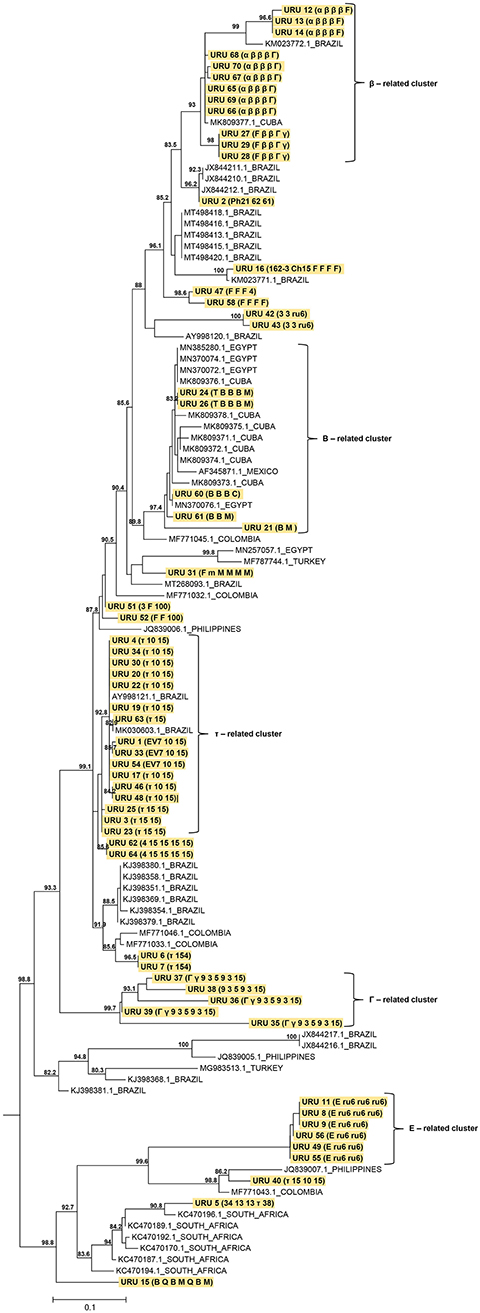
Figure 2. Maximum likelihood (ML) phylogenetic tree of A. marginale strains based in partial MSP1a amino acid sequences. The analysis involved 114 amino acid sequences (strains found in this study were highlighted in yellow). The ML phylogenetic tree was midpoint rooted and only SH-aLRT support values >80 are shown. The branch lengths are drawn to scale with the bar at the bottom indicating amino acid substitution per site. Combinations of tandem repeats are shown in parenthesis.
Statistical analysis
Statistical analysis of tandem repeats using phi correlation was performed considering the presence or absence of R. microplus. We classified the repeats into two classes: TR found in the presence (TP) or absence (TA) of ticks. Only the repeats Γ, β, α, and γ were found in the TA, with a statistically significant correlation (p < 0.05; Table 1).
Discussions
Bovine anaplasmosis caused by A. marginale is one of the main parasitic diseases transmitted biologically or mechanically by vectors, which causes significant losses to the livestock industry. It is highly prevalent in tropical and subtropical regions (7, 27, 32, 33). As observed in this study, the bovine anaplasmosis mainly affects adult animals, which is consistent with several reports (1, 34, 35). In contrast, young cattle often evolve into subclinical diseases, responding more efficiently and developing a rapid immune response (36, 37).
Several studies worldwide have been conducted to characterize A. marginale strains, for which the major surface protein genes are widely used (15). MSP1a is one of the six MSP-reported proteins and has been described as a model molecule for the analysis of A. marginale genetic diversity (14, 26). This protein is involved in the adhesion of A. marginale to bovine erythrocytes and ticks gut cells. It plays an important role in cattle infection, tick transmission, and development of bovine immunity against A. marginale (3). The major surface protein 1a gene has a molecular size between 630 and 1,200 bp (24). This length polymorphism in MSP 1a is due to the variation in the number of TRs (12). The sizes of the sequenced samples were within this range.
During strain genotyping based on the microsatellite structure, we observed that genotype E was the most prevalent. These results are consistent with previous results for strains found mainly in Brazil (19, 20, 38), Argentina (18) and Colombia (39). Other genotypes frequently observed in our work were G and H. Studies carried out in Brazil (São Paulo and Maranhão) reported that genotype H was the most frequently found in beef and dairy cattle (21, 22). However, the genetic diversity of circulating strains in the equatorial zone, comprising the countries of Ecuador, Mexico, Cuba, and subtropical countries such as South Africa and the USA, are different, with G and C being the main genotypes (15, 27, 40).
The work carried out by Estrada-Peña et al., (14) reports that clusters of microsatellite-genotyped strains were observed according to the geographic region. Furthermore, the same author described that SD-ATG length (between 19 and 29 nucleotides) of the MSP1a microsatellite has been correlated with expression of the gene, which affects pathogen infection and transmission of A. marginale. The expression of MSP1a is lower in variants with an SD-ATG distance of 19 nucleotides, while those with a distance of 23 and 29 nucleotides have higher MSP1a gene expression (14). In our study, 88% (53/60) of the strains corresponded to genotypes with a distance of 23 nucleotides, suggesting that these A. marginale strains may have high infectivity.
In addition, in this study, based on the information provided by Repeat Analyzer 22 new strains were found. The τ-10-15 and α-β-β-β-Γ strain was overrepresented, which may be because we worked with clinical cases. It is possible that this strain is more pathogenic than the other strains reported. Theses strains have been previously described as the most prevalent strain in cattle in Brazil, Argentina and México (4, 20, 38). Correlation analysis of the tandem repeats, based on the presence or absence of a tick, revealed that only four repeats (Γ, β, α, and γ) were associated (p < 0.05) with the absence of R. microplus, which suggests that strains containing these repeats may be less adapted to tick transmission. Similar studies carried out in Argentina observed that repeat M was associated (p < 0.05) with the absence of R. microplus (18). Due to the high genetic diversity of the strains found in our region and the small number of samples analyzed, more studies must be carried out to validate the provisional results shown here.
The high genetic diversity of A. marginale in tick enzootic regions facilitated the co-circulation of several strains during the same outbreak. Argentinian studies have reported the presence of more than one Anaplasma strain infecting the same animal (18), as well as Barbosa et al. reported on work carried out in Angus cattle in Brazil (22).
Previous work carried out by de la Fuente et al. (41) demonstrated that tandem repeated peptides containing the amino acids D or E at position 20 of their tandem repeats have the ability to bind to tick cells. G-containing peptides do not bind to tick cell extracts. In this study, we observed that 53.3% of our samples contained D or E at position 20 of their tandem repeats, whereas 46.7% had G. These findings indicate that >50% of the strains that we characterized have the ability to bind to ticks cells and be biologically transmitted, but the remaining proportion does not. More studies are necessary to discover competent vectors of A. marginale strains that do not have the capacity to be biologically transmitted by ticks, as tabanids, Stomoxys calcitrans and other mechanical vector.
A phylogenetic tree revealed the co-circulation of different A. marginale strains in Uruguay. This provides valuable information on the distribution of strains, as reported in other studies (5, 14). A cluster related to TR was observed, in agreement with other reports (19, 42). Several of the strains found in this study are related to strains from Brazil. This could be attributed to Uruguayan cattle entering from southern Brazil and northern Argentina (43), bringing with them R. microplus ticks and different strains of A. marginale. These strains have to adapt to different factors such as climatic conditions, host breed, host immune response, population dynamics of ticks and insects that transmit them, transportation of cattle, and use of insecticides and acaricides (15, 41). This adaptation most likely caused the strains to evolve and generate a wide genetic diversity.
Further genetic studies based on the MSP1a protein are imperative, as it has been described as the reference gene for monitoring genetic diversity and evolution, an important identity marker of Anaplasma marginale strains. It also provides important information regarding the epidemiological behavior of these species. Finally, as it is an immunoreactive protein, the information provided here may be used for future studies on the design of vaccines for the control of this disease.
Conclusion
This is the first study conducted in Uruguay to genetically characterize A. marginale strains. Analysis of MSP1a revealed the co-circulation of different strains identified in clinical diseases in cattle. This study provides valuable epidemiological information for understanding bovine anaplasmosis as well as basic information for the design of potential vaccines for the control and prevention of this disease.
Data availability statement
The data presented in the study are deposited in the Genbank repository, accession numbers OP382972-OP383031.
Ethics statement
This study did not require ethical approval because the samples that we used was provided by animals with natural infections that arrived at the laboratory of the Division of Veterinary Laboratories (DILAVE) of the Ministry of Livestock, Agriculture and Fisheries (MGAP) for diagnosis. It was a convenience sampling. Written informed consent was obtained from the owners for the participation of their animals in this study.
Author contributions
PP, MA-F, RR, and JV contributed to conception, design of the study, and performed the experiments. MS provided the statistical analysis. PP, DM, MB-G, and NR-O characterization and analysis of genetic sequences. PP and JV conducted writing—reviewing. PP, RR, and JV acquired the funding. All authors contributed to the article and approved the submitted version.
Funding
The research that gives rise to the results presented in this publication received funds from the Instituto Nacional de Investigación Agropecuaria (INIA), Uruguay, by the project CL_35: Determination of the current situation of Rhipicephalus microplus and tick fever and integrated control of both diseases, Animal Health Platform.
Acknowledgments
The authors are grateful to Federico Giannitti, Sofia Fernandez, and all veterinarians and farmers who collaborated with this project. Anderson Saravia helped us with the map design.
Conflict of interest
The authors declare that the research was conducted in the absence of any commercial or financial relationships that could be construed as a potential conflict of interest.
Publisher's note
All claims expressed in this article are solely those of the authors and do not necessarily represent those of their affiliated organizations, or those of the publisher, the editors and the reviewers. Any product that may be evaluated in this article, or claim that may be made by its manufacturer, is not guaranteed or endorsed by the publisher.
Supplementary material
The Supplementary Material for this article can be found online at: https://www.frontiersin.org/articles/10.3389/fvets.2022.990228/full#supplementary-material
References
1. Kocan KM, de la Fuente J, Blouin EF, Coetzee JF, Ewing SA. The natural history of Anaplasma marginale. Vet Parasitol. (2010) 167:95–107. doi: 10.1016/j.vetpar.2009.09.012
2. Dumler JS, Barbet AF, Bekker CPJ, Dasch GA, Palmer GH, Ray SC, et al. Reorganization of the genera in the families Rickettsiaceae and Anaplasmataceae in the order Rickettsiales: unification of some species of Ehrlichia with Anaplasma, Cowdria with Ehrlichia and Ehrlichia with Neorickettsia, descriptions of six new species combinations and designation of Ehrlichia equi and “HGE agent” as subjective synonyms of Ehrlichia phagocytophila. Int J Syst Evol Microbiol. (2001) 51:2145–65. doi: 10.1099/00207713-51-6-2145
3. Aubry P, Geale DW. A review of Bovine anaplasmosis. Transbound Emerg Dis. (2011) 58:1–30. doi: 10.1111/j.1865-1682.2010.01173.x
4. Vidotto MC, Kano SF, Gregori F, Headley SA, Vidotto O. Phylogenetic analysis of Anaplasma marginale strains from Paraná State, Brazil, using the msp1-alfa and msp4 genes. J Vet Med B Infect Dis Vet Public Health. (2006) 53:404–11. doi: 10.1111/j.1439-0450.2006.00984.x
5. de la Fuente J, Garcia-Garcia JC, Blouin EF, Rodriguez SD, Garcia MA, Kocan KM. Evolution and function of tandem repeats in the major surface protein 1a of the ehrlichial pathogen Anaplasma marginale. Anim. Health Res Rev. (2001) 2:163–173. doi: 10.1079/AHRR200132
6. Kocan KM, de la Fuente J, Golsteyn Thomas EJ, Van Den Bussche RA, Hamilton RG, Tanaka EE, et al. Recent studies on characterization of Anaplasma marginale isolated from North American Bison. Ann N Y Acad Sci. (2004) 1026:114–7. doi: 10.1196/annals.1307.015
7. Solari MA, Dutra F, Quintana S. Epidemiología y prevención de los hemoparásitos (Babesia sp. y Anaplasma marginale) en el Uruguay. In: Fiel C, Nari A, eds. Enfermedades Parasitarias de Importancia Clínica y Productiva en Rumiantes. Montevideo: Hemisferio Sur (2013), p. 689–714.
8. Scoles GA, Broce AB, Lysyk TJ, Palmer GH. Relative efficiency of biological transmission of Anaplasma marginale (Rickettsiales: Anaplasmataceae) by Dermacentor andersoni (Acari: Ixodidae) compared with mechanical transmission by Stomoxys calcitrans (Diptera: Muscidae). J Med Entomol. (2005) 42:668–75. doi: 10.1093/jmedent/42.4.668
9. Richey EJ. Bovine anaplasmosis. In: Howard RJ, ed. Current Veterinary Therapy Food Animal Practice. Philadelphia, PA: The W.B. Saunders Co. (1981), p. 767–72.
10. Ristic M. Bovine anaplasmosis. In: Kreier J, ed. Parasitic Protozoa, Vol. 4. New York, NY: Academic Press (1977), p. 235–49.
11. Richey EJ. Bovine anaplasmosis. In: Proceedings of the 24th Annual Conference of the American Association of Bovine Practitioners. Orlando, FL (1992), p. 3–11.
12. Cabezas-Cruz A, de la Fuente J. Anaplasma marginale major surface protein 1a: a marker of strain diversity with implications for control of bovine anaplasmosis. Ticks Tick Borne Dis. (2015) 6:205–10. doi: 10.1016/j.ttbdis.2015.03.007
13. de la Fuente J, Garcia-Garcia JC, Barbet AF, Blouin EF, Kocan KM. Adhesion of outer membrane proteins containing tandem repeats of Anaplasma and Ehrlichia species (Rickettsiales: Anaplasmataceae) to tick cells. Vet Microbiol. (2004) 98:313–22. doi: 10.1016/j.vetmic.2003.11.001
14. Estrada-Peña A, Naranjo V, Acevedo-Whitehouse K, Mangold AJ, Kocan KM, de la Fuente J. Phylogeographic analysis reveals association of tick-borne pathogen, Anaplasma marginale, MSP1a sequences with ecological traits affecting tick vector performance. BMC Biol. (2009) 7:1–13. doi: 10.1186/1741-7007-7-57
15. Cabezas-Cruz A, Passos LMF, Lis K, Kenneil R, Valdés JJ, Ferrolho J, et al. Functional and immunological relevance of Anaplasma marginale major surface protein 1a sequence and structural analysis. PLoS ONE. (2013) 8:e65243. doi: 10.1371/journal.pone.0065243
16. Fedorina EA, Arkhipova AL, Kosovskiy GY, Kovalchuk SN. Molecular survey and genetic characterization of Anaplasma marginale isolates in cattle from two regions of Russia. Ticks Tick Borne Dis. (2018) 10:251–7. doi: 10.1016/j.ttbdis.2018.10.011
17. Almazán C, Medrano C, Ortiz M, de la Fuente J. Genetic diversity of Anaplasma marginale strains from an outbreak of bovine anaplasmosis in anendemic area. Vet Parasitol. (2008) 158:103–9. doi: 10.1016/j.vetpar.2008.08.015
18. Ruybal P, Moretta R, Perez A, Petrigh R, Zimmer P, Alcaraz E, et al. Genetic diversity of Anaplasma marginale in Argentina. Vet Parasitol. (2009) 162:176–80. doi: 10.1016/j.vetpar.2009.02.006
19. Machado RZ, Silva JB, André MR, Gonçalves LR, Matos CA, Obregón D. Outbreak of anaplasmosis associated with the presence of different Anaplasma marginale strains in dairy cattle in the states of São Paulo and Goiás, Brazil. Rev Bras Parasitol Vet. (2015) 24:438–46. doi: 10.1590/S1984-29612015078
20. de Souza Ramos IA, Herrera HM, Fernandes SJ, do Amaral RB, Zanatto DCS, da Silva TMV, et al. Genetic diversity of Anaplasma marginale in beef cattle in the Brazilian Pantanal. Ticks Tick Borne Dis. (2019) 10:805–14. doi: 10.1016/j.ttbdis.2019.03.015
21. Pereira JG, Garcia AB, Gonçalves LR, Ramos IAS, Braga MDSCO, Santos LSD, et al. High genetic diversity of Anaplasma marginale infecting dairy cattle in northeastern Brazil. Rev Bras Parasitol Vet. (2021) 30:e014321. doi: 10.1590/s1984-29612021097
22. Barbosa AG, Gomes MM, Freschi CR, Souza Ramos IA, Mendes NS, do Amaral RB, et al. High genetic diversity and superinfection by Anaplasma marginale strains in naturally infected Angus beef cattle during a clinical anaplasmosis outbreak in Southeastern Brazil. Ticks Tick Borne Dis. (2022) 13:101829. doi: 10.1016/j.ttbdis.2021.101829
23. Torioni S, Knowles DP, McGuire TC, Palmer GH, Suarez CE, McElwain TF. Detection of cattle naturally infected with Anaplasma marginale in a region of endemicity by nested PCR and a competitive enzyme-linked immunosorbent assay using recombinant major surface protein 5. J Clin Microbiol. (1998) 36:777–82. doi: 10.1128/JCM.36.3.777-782.1998
24. Lew AE, Bock RE, Minchin CM, Masaka S. A msp1a polymerase chain reaction assay for specific detection and differentiation of Anaplasma marginale isolates. Vet Microbiol. (2002) 86:325–35. doi: 10.1016/S0378-1135(02)00017-2
25. Kumar S, Stecher G, Li M, Knyaz C, Tamura K. MEGAX: molecular evolutionary genetics analysis across computing plataforms. Mol Biol Evol. (2018) 35:1547–9. doi: 10.1093/molbev/msy096
26. de la Fuente J, Lew A, Lutz H, Meli ML, Hofmann-Lehmann R, Shkap V, et al. Genetic diversity of Anaplasma species major surface proteins and implications for anaplasmosis serodiagnosis and vaccine development. Anim Health Res Rev. (2007) 6:75–89. doi: 10.1079/AHR2005104
27. Guarnizo TRM, Alvarez DO, Díaz-Sánchez AA, Cabezas-Cruz A, Gutiérrez LZ, Marrero SM, et al. Epidemiology and genetic diversity of Anaplasma marginale in Zamora-Chinchipe, Ecuador. Ticks Tick Borne Dis. (2020) 11:101308. doi: 10.1016/j.ttbdis.2020.101380
28. Catanese HN, Brayton KA, Gebremedhin AH. Repeatanalyzer: a tool for analysing and managing short-sequence repeat data. BMC Genomics. (2016) 17:1–13. doi: 10.1186/s12864-016-2686-2
29. Katoh K, Misawa K, Kuma K, Miyata T. MAFFT: a novel method for rapid multiple sequence alignment based on fast Fourier transform. Nucleic Acids Res. (2002) 30:3059–66. doi: 10.1093/nar/gkf436
30. Nguyen LT, Schmidt HA, von Haeseler A, Minh BQ. IQ-TREE: a fast and effective stochastic algorithm for estimating maximum likelihood phylogenies. Mol Biol Evol. (2015) 32:268–74. doi: 10.1093/molbev/msu300
31. Anisimova M, Gascuel O. Approximate likehood-ratio test for branch: a fast, accurate, and powerful alternative. Syst Biol. (2006) 55:539–52. doi: 10.1080/10635150600755453
32. Guglielmone AA. Epidemiology of babesiosis and anaplasmosis in South and Central America. Vet Parasitol. (1995) 57:109–19. doi: 10.1016/0304-4017(94)03115-D
33. Vidotto MC, Vidotto O, Andrade GM, Palmer G, McElwain T, Knowles DP. Seroprevalence of Anaplasma marginale in cattle in Paraná State, Brazil, by MSP-5 competitive ELISA. Ann N Y Acad Sci. (1998) 849:424–6. doi: 10.1111/j.1749-6632.1998.tb11087.x
34. Amorim LS, Wenceslau AA, Carvalho FS, Carneiro PL, Albuquerque GR. Bovine babesiosis and anaplasmosis complex: diagnosis and evaluation of the risk factors from Bahia, Brazil. Rev Bras Parasitol Vet. (2014) 23:328–36. doi: 10.1590/S1984-29612014064
35. Parodi P, Armúa-Fernandez M, Corbellini LG, Rivero R, Miraballes C, Riet-Correa F, et al. Description of bovine babesiosis and anaplasmosis outbreaks in northern Uruguay between 2016 and 2018. Vet Parasitol Reg Stud. (2022). doi: 10.1016/j.vprsr.2022.100700
36. Kieser ST, Eriks IE, Palmer GH. Cyclic rickettsemia during persistent Anaplasma marginale infection in cattle. Infect Immun. (1990) 58:1117–9. doi: 10.1128/iai.58.4.1117-1119.1990
37. Palmer GH, Rurangirwa FR, Kocan KM, Brown WC. Molecular basis for vaccine development against the ehrlichial pathogen Anaplasma marginale. Parasitol. (1999) 15:253–300. doi: 10.1016/S0169-4758(99)01469-6
38. Silva JB, Cabezas-Cruz A, Fonseca AH, Barbosa JD, de la Fuente J. Infection of water buffalo in Rio de Janeiro Brazil with Anaplasma marginale strains also reported in cattle. Vet Parasitol. (2014) 205:730–4. doi: 10.1016/j.vetpar.2014.09.009
39. Jaimes-Dueñez J, Triana-Chávez O, Mejía-Jaramillo AM. Genetic, host and environmental factors associated with a high prevalence of Anaplasma marginale. Ticks Tick Dis. (2018) 9:1286–95. doi: 10.1016/j.ttbdis.2018.05.009
40. Obregón D, Corona BG, de la Fuente J, Cabezas-Cruz A, Gonçalves LR, Matos CA, et al. Molecular evidence of the reservoir competence of water buffalo (Bubalus bubalis) for Anaplasma marginale in Cuba. Vet Parasitol Reg Stud Reports. (2018) 13:180–7. doi: 10.1016/j.vprsr.2018.06.007
41. de la Fuente J, Van Den Bussche RA, Prado TM, Kocan KM. Anaplasma marginale msp1α genotypes evolved under positive selection pressure but are not markers for geographic isolates. J Clin Microbiol. (2003) 41:1609–16. doi: 10.1128/JCM.41.4.1609-1616.2003
42. Baêta BA, Ribeiro CCDU, Teixeira RC, Cabezas-Cruz A, Passos LMF, Zweygarth E, et al. Characterization of two strains of Anaplasma marginale isolated from cattle in Rio de Janeiro, Brazil, after propagation in tick cell culture. Ticks Tick Borne Dis. (2015) 6:141–5. doi: 10.1016/j.ttbdis.2014.11.003
Keywords: bovine, Anaplasma marginale, genotyping, MSP1a, Uruguay
Citation: Parodi P, Armúa-Fernández MT, Schanzembach M, Mir D, Benítez-Galeano MJ, Rodríguez-Osorio N, Rivero R and Venzal JM (2022) Characterization of strains of Anaplasma marginale from clinical cases in bovine using major surface protein 1a in Uruguay. Front. Vet. Sci. 9:990228. doi: 10.3389/fvets.2022.990228
Received: 09 July 2022; Accepted: 30 August 2022;
Published: 20 September 2022.
Edited by:
Sergio D. Rodríguez Camarillo, INIFAP, MexicoReviewed by:
Marcos Rogério André, São Paulo State University, BrazilAbdulaziz Alouffi, King Abdulaziz City for Science and Technology, Saudi Arabia
Copyright © 2022 Parodi, Armúa-Fernández, Schanzembach, Mir, Benítez-Galeano, Rodríguez-Osorio, Rivero and Venzal. This is an open-access article distributed under the terms of the Creative Commons Attribution License (CC BY). The use, distribution or reproduction in other forums is permitted, provided the original author(s) and the copyright owner(s) are credited and that the original publication in this journal is cited, in accordance with accepted academic practice. No use, distribution or reproduction is permitted which does not comply with these terms.
*Correspondence: Pablo Parodi, cHBhcm9kaUBpbmlhLm9yZy51eQ==