- 1Dairy Science Department, National Research Centre, Giza, Egypt
- 2Department of Animal Sciences, School of Agriculture, Policy and Development, University of Reading, Reading, United Kingdom
The objective of this study was to determine the ability of an isolated strain (EGY_NRC1) or commercial (NCIMB 11181) Enterococcus faecium as a probiotic for lactating cows. Two experiments were conducted: In Experiment 1, the effects of three levels (1, 2, and 3 g/kg diet, DM basis) of isolated and commercial E. faecium on in vitro ruminal fermentation kinetics, gas, methane (CH4) and nutrient degradability were determined. In Experiment 2, thirty multiparous Holstein cows (633 ± 25.4 kg body weight) with 7 days in milk, were randomly assigned to 3 treatments in a completely randomized design in a 60-day experiment. Cows were fed without any additives (control treatment) or supplemented with 2 g/kg feed daily of E. faecium EGY_NRC1 (contain 1.1 × 109 CFU/g) or commercial E. faecium NCIMB 11181 (contain 2 × 1012 CFU/g). Diets were prepared to meet cow's nutrient requirements according to NRC recommendations. Probiotic doses were based on the in vitro Experiment 1. Feed intake, digestibility, blood parameters and lactation performance were evaluated. In Experiment 1, the isolated E. faecium linearly and quadratically increased (P < 0.001) in vitro total gas production (TGP), the degradability of dry matter (dDM) and organic matter (dOM) while decreased (P < 0.05) methane (CH4) percent of TGP, NH3CH4 production, and pH. The commercial E. faecium increased TGP and decreased (P < 0.01) CH4 production, pH and increased the dDM and dOM, short chain fatty acids and ruminal NH3-N concentration. In Experiment 2, the isolated E. faecium increased (P < 0.01) total tract digestibility of DM, neutral and acid detergent fiber, daily milk production and feed efficiency compared to the control treatment without affecting feed intake and milk composition. Moreover, the isolated E. faecium increased (P < 0.05) the proportion of C18:1 trans-9, C18:2 cis-9-12 and C18:2 trans-10 cis-12. Both isolated and commercial E. faecium improved (P < 0.01) organic matter, crude protein and nonstructural carbohydrates digestibility, increased serum glucose (P = 0.002) and decreased serum cholesterol (P = 0.002). Additionally, both E. faecium strains decreased C23:0 (P = 0.005) in milk. In conclusion, the use of E. faecium (isolated and commercial) at 2 g/kg DM of feed improved feed efficiency and production performance, with superior effects on animal performance from isolated E. faecium compared to the commercial one.
Introduction
Probiotics and prebiotics have been administered to animals for several years to enhance their health and production. In animal production, probiotics are now widely accepted as safe and sustainable alternatives to antibiotics (1, 2). For many years, lactic acid bacteria (LAB) have been used in livestock production, as probiotic supplements or as silage preservative by inhibiting pathogenic microorganisms (e.g., fungal and clostridial growth) and increasing lactic acid formation (3, 4). Normally, LAB are predominantly found in the gastrointestinal tract of animals and humans and are also found in dairy products (5).
In livestock, different bacterial and fungal species (i.e., Bacillus, Bifidobacterium, Enterococcus, Lactobacillus, Propionibacterium, Megasphaera elsdenii and Prevotella bryantii) have been used as probiotics as well as strains of Aspergillus and Saccharomyces (1, 6). Strains of LAB including Lactobacillus, Bifidobacterium and Streptococcus are commonly used as probiotics in ruminant feed (1, 7). LAB can alter ruminal fermentation and enhance nutrient digestibility and productive performance (8). LAB reduce oxygen from the rumen environment and prevent excess of ruminal lactate production, inhibit ruminal pathogens, and modulate the microbial balance (9). Previous studies have reported the effect of LAB in ruminant diets, including the increased yield of microbial biomass (10), reduced methane (11) and increased dry matter (DM) digestibility (12). Recently, it has been reported that feeding dairy cows with L. casei TH14 can improve feed utilization, rumen fermentation parameters and milk production (13).
The use of commercial LAB can increase total mixed rations costs and finding alternatives that keep similar efficiency to that from commercial products is of great interest for production improvement. Also, research on novel strains of microorganisms with different origins and properties is still needed. Therefore, the objective of this study was to determine the ability of an isolated strain (EGY_NRC1) or commercial (NCIMB 11181) Enterococcus faecium as a probiotic for lactating cows. The hypothesis of this study was that the in-feed supplementation of commercial or isolated Enterococcus faecium would lead to similar effects on feed utilization, milk production, milk composition and milk fatty acid profile in early lactating dairy Holstein cows.
Materials and methods
Isolation and identification of E. faecium
Lactic acid bacterial strains were isolated from 15 samples of fresh dairy products (homemade 8 samples of yogurt and 5 samples soft white cheese). For each sample, 10 g were added to 90 ml sterile saline solution and homogenized by vortex for 10 min. Decimal dilutions were placed on double layered M17 agar plates then incubated for 48 h at 30°C. Well defined round colonies were selected randomly and only Gram-positive catalase-negative cocci were retained and stored in M17 broth for further experiments.
To characterize the selected isolate, the carbohydrate fermentation pattern (14) of the selected isolate (that possessed antibacterial activity) and its ability to produce ammonia (NH3) from arginine (15) was examined. After that, the strain was identified by matrix-assisted laser desorption ionization-time-of-flight mass spectrometry (MALDI-TOF MS) and 16S ribosomal DNA (rDNA) sequencing. The isolate was identified (Figure 1) via the analysis of its total proteome in which a score with more than 1.7 indicates genus identification and a score with more than 2 is the confidence value at the species level (16).
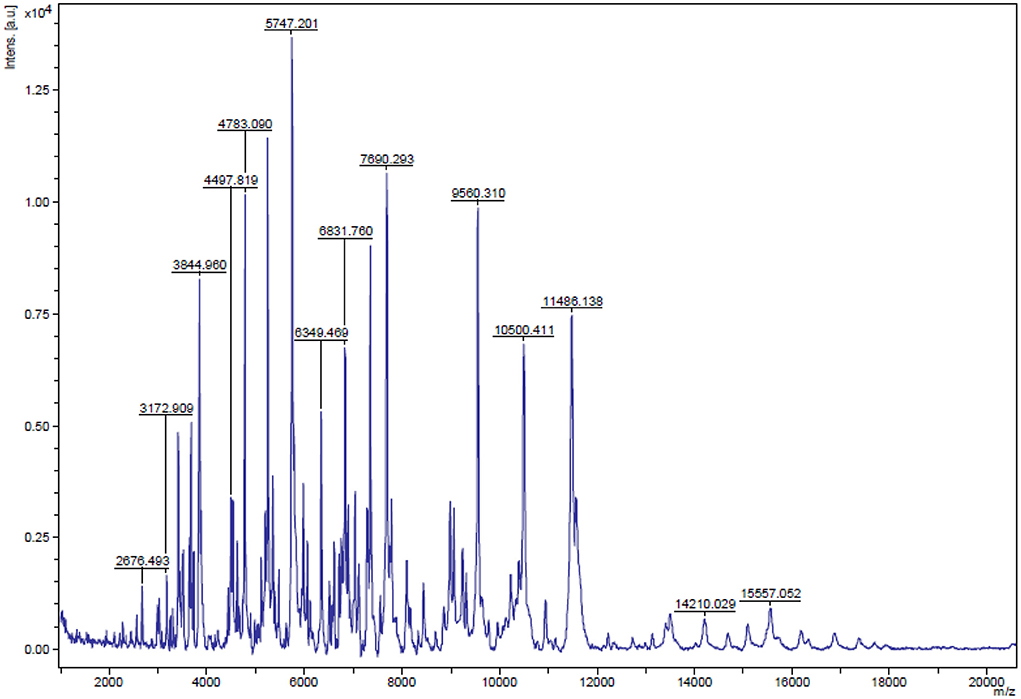
Figure 1. Result of the matrix-assisted laser desorption ionization time-of-flight mass spectrometry (MALDI-TOF MS) analysis. The MALDI-TOF MS showed that the isolated strain of E. faecium that possess antimicrobial activity was initially identified with a high confidence value of 2.31 that indicated a reliable identification of the isolate up to species level.
For DNA extraction, genomic DNA was used from the selected isolate that was cultivated at 37°C for 24 h. Freshly prepared culture was subjected to16S rDNA PCR partial amplification by use of Qiagene genomic DNA purification kit. The genomic DNA was used as a template for PCR amplification of a segment of its 16S rRNA gene. The two primers used were previously described by Liu et al. (17), 8f (5_AGAGTTTGATCCTGGCTCAG-3) and 1495 R (5_CTACGGCTACCTTGTTACGA-3). The PCR products yielded were analyzed on a 1% (w/v) agarose gel after staining with ethidium bromide. The PCR products were separated on an agarose gel, followed by ethidium bromide staining to check for the presence of a unique amplicon. When a gene from a particular species was amplified using a primer initially designed for a different species, the corresponding amplicon was purified by Promega Wizard Genomic DNA Purification Kit and sequenced by HVD life science, Germany.
For phylogenetic analysis (data not shown), the 16S rDNA sequence obtained was added to publicly available bacterial 16srRNA sequences and integrated into the database with the automatic alignment tool. Phylogenetic analysis was inferred using neighbor joining method and the phylogenetic tree was constructed (18). The isolated LAB was identified as E. faecium EGY_NRC1 with NCBI accession number MW856655.
The biomass production of the isolated LAB was done on whey permeate media with the following composition (/L permeate): 5 g yeast extract, 5 g peptone, 0.5 g magnesium sulfate, 3 g ammonium chloride and 2.5 g ascorbic acid. The medium was inoculated individually with 5% (v/v) of the isolated LAB culture (24 h old age activated M17 broth culture) and then incubated at 37°C for 48 h. The cultured biomasses were separated by centrifugation at 6,000 rpm for 15 min at 4°C then added to dry permeate as a carrier agent, and dried in oven at 37°C. The un-inoculated media were used as control. Unpublished data showed that the viable count of the strain after pre-incubation at 60°C for 30 min was enumerated and the result indicated that the remaining count was 3.274 log CFU/mL in compared to 5.477 log CFU/mL without pre-incubation. The reported viable count was determined after drying.
Experiment 1
In vitro evaluation
Using a stomach tube, rumen liquor was obtained from three adult Barki sheep (51 ± 2.6 kg of body weight) fed a fixed amount of concentrate (500 g) and ad libitum berseem hay daily. The rumen contents (liquid and solid contents 1:1 v/v) were collected before morning feeding, kept in pre-warmed thermo containers at 39°C under anaerobic conditions. About 500 mL of ruminal fluid was collected from all ewes of each treatment. To avoid saliva contamination, the first 50 mL of the rumen fluid samples were discarded. The rumen fluid was mixed for 10 s, squeezed through four layers of cheese cloth, and maintained in a water bath at 39°C under continuous CO2 flushing until inoculation (19). Three incubation runs were performed in three different weeks. Rumen contents obtained from the three sheep were combined for each run. Animal use for this trial was approved by the technical committee of the Science, Technology & Innovation Funding Authority (STDF), Egypt (project STDF 33413).
A total mixed ration composed of (per kg DM) 300 g berseem clover, 300 g corn silage, 150 g soybean meal, and 250 g yellow corn was used as a substrate. The nutrient contents of feed ingredients and basal diet are shown in Table 1. The in vitro total gas production (TGP) assay was conducted as described by Theodorou et al. (19) and adapted to the semi-automatic system of Mauricio et al. (20). Ground substrate samples (500 mg of DM) were incubated in 120 mL serum bottles (5 bottles per dose of LAB at each time). LAB (isolated and commercial) was included at 0, 1, 2, and 3 g/kg DM substrate. The isolated bacteria contained 1.1 × 109 CFU/g. Levels of inclusion were based on initial screening of many doses of E. faecium on ruminal fermentation (2, 21). After dispensing, bottles were closed with rubber stoppers, shaken manually, and incubated at 39°C in a forced-air oven for 48 h. The bottles were shaken at 1 h intervals during incubation. The amount of TGP was calculated according to the regression equation {V = 4.974 12 × p + 0.171; n = 500, r2 = 0.98; [V is gas volume (mL); p is measured pressure (psi)]} obtained in our laboratory under our conditions according to the gas volume vs. pressure. Bottles containing only buffered rumen fluid without substrate were considered as blanks. At each incubation time, 5 mL of headspace gas was taken from each bottle and infused into a Gas-Pro detector (Gas Analyzer CROWCON Model Tetra3, Abingdon, UK) to measure the concentration of CH4 and carbon dioxide. The control and experimental treatments were tested in 6 bottles (analytical replicates) and three incubation runs in three consecutive weeks with 3 bottles containing inoculum and buffer but no feed (blanks).
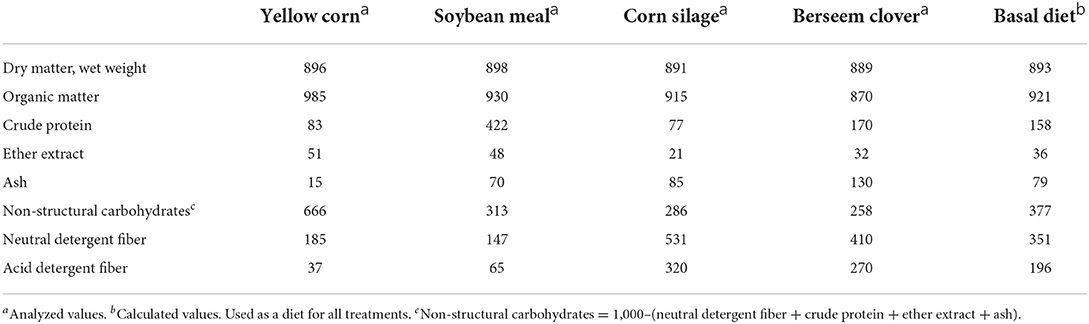
Table 1. Chemical composition of ingredients and control diet used in the in vitro and in vivo experiments (g/kg of dry matter).
At 48 h (the end of incubation), fermentation was terminated by immersing the bottles in ice. For each LAB level, 3 bottles were used to measure the pH, NH3-N (22) and short chain fatty acids (SCFA) by steam distillation and titration (23), whereas the other 3 bottles were filtered in pre-weighed crucibles and washed with hot water then acetone, and the residual DM and ash were estimated to determine true DM, organic matter (OM), neutral detergent fiber (NDF) and acid detergent fiber (ADF) degradability (dDM, dOM, dNDF, and dADF, respectively).
Experiment 2
Animals
Cows were managed in accordance with the Guide for the Care and Use of Agricultural Animals in Agricultural Research and Teaching, 3rd edition, 2010 (Federation of Animal Science Societies, Champaign, IL, USA). The protocol was approved by the technical committee of the Science, Technology & Innovation Funding Authority (STDF), Egypt (project STDF 33413).
During the first week of lactation, thirty lactating Holstein cows (633 ± 25.4 kg body weight, 3 ± 1 parity, 7 ± 1 days in milk, with a previous milk production of 24 ± 2.2 kg/d, were assigned randomly to one of three experimental treatments in a complete randomized designed with 10 cows per treatment for 60 days.
Cows were divided into three barns in soil-surfaced free stalls (122 × 175 cm2/cows), under shade, without any bedding and with free access to water. Cows were fed ad libitum a diet containing [per kg DM] 300 g berseem clover, 300 g corn silage, 150 g soybean meal and 250 g grounded corn grain, to meet their nutrient requirements according to NRC (24) recommendations for 650 kg cow with 20 kg DM intake and 35 kg daily milk production. Animals were fed 10% more of the expected dry matter intake to ensure collection of orts. The diet fed to cows was the same for the in vitro experiment.
Cows were fed their diets without any additives (control treatment) or supplemented with 2 g/kg feed daily of E. faecium EGY_NRC1 (isolated to contain 1.1 × 109 CFU/g) or commercial E. faecium NCIMB 11181 (isolated to contain 2 × 1012 CFU/g; ADM Protexin Limited, Lopen Head, Somerset, TA13 5JH UK). The doses of probiotics were based on the results obtained from Experiment 1 (in vitro experiment). Diets were offered twice a day at 08:00 and 16:00 h. The additives were mixed with all feedstuffs using a feeding wagon. Before use, probiotics were kept at 4°C. Samples of feed ingredients were taken daily, composited weekly, dried at 60°C in a forced-air oven for 48 h (method 930.15) (22) and stored for chemical analyses.
The total mixed ration was prepared and distributed using a horizontal mixer system (DeLaval, Ontario, Canada) after mixing for 20 min. Samples of feed were taken daily, composited weekly, dried at 60°C in a forced-air oven for 48 h (22) and stored for chemical analysis. The nutrient contents of the feed ingredients are shown in Table 1. Cows were weighed on a digital multi-purpose platform scale at the beginning and at the end of the experiment.
Nutrient intake and digestibility
Feed intake was recorded daily by weighing the total daily amount of feed offered to each cow and the total daily amounts of weighed orts. On the 4th and 8th week, nutrient digestibility trials were conducted, in which acid insoluble ash was used as an internal indigestibility marker (25). Acid-insoluble ash contents of feeds and feces were determined gravimetrically after drying, burning, boiling of ash in hydrochloric acid, filtering and washing of the hot hydrolysate, and re-burning. Coefficients of digestion were calculated according to Ferret et al. (26). For the digestibility trial, fecal samples were collected from all cows twice daily at 09:00 and 16:00 h, dried at 60°C for 48 h in a forced-air oven and pooled by cow. The nutritive value of diets expressed as total digestible nutrients (TDN), digestible energy (DE), metabolizable energy (ME), net energy for lactation (NEL) were calculated according to NRC (24) equation, while the net energy requirements for lactation equivalent of 1 kg of standard air-dry barley (UFL) was calculated according to INRA (27) equation.
The dried feed, orts and fecal samples were ground to pass a 1-mm screen using a Wiley mill and analyzed for DM (method ID 930.15), ash (method ID 942.05), nitrogen (method ID 954.01) and ether extract (EE; method ID 920.39) according to the AOAC (22) official methods. Neutral detergent fiber was determined by the procedure of Van Soest et al. (28) with the use of alpha amylase and sodium sulphite. Acid detergent fiber was analyzed according to the AOAC (22). Lignin was analyzed according to Van Soest et al. (28).
Sampling and analysis of blood serum
On the last day of the 4th and 8th weeks of the experiment, individual blood samples (10 mL) were taken before morning feeding at 08:00 h from the jugular vein. Blood samples were centrifuged at 4,000 ×g for 20 min at 4°C. The serum was separated into 2 mL Eppendorf tubes and frozen at −20°C until analysis. By using specific kits (Spinreact, Ctra. Santa Coloma, Girona, Spain) and following the manufacturers' instructions, blood serum samples were analyzed for total protein, albumin, globulin, urea-N, glucose, cholesterol, triglycerides, aspartate aminotransferase (AST), and alanine aminotransferase (ALT). The globulin concentration was calculated by subtracting the albumin values from their corresponding total protein values.
Milk sampling, and milk composition
Cows were milked (DeLaval parallel parlor P2100, SE-147 21 Tumba, Sweden) three times daily at 04:00, 12:00 and 20:00 h, and individual milk samples (30 g/kg of milk yield) were collected at each milking. A mixed sample of milk (proportional to amounts isolated in each milking time) was taken daily every 2 weeks to determine milk composition. Milk samples were analyzed using infrared spectrophotometry (Ekomilk-M ultrasonic milk analyzer, EON Trading 2000, INC, Bulgaria).
The gross energy content was calculated according to Tyrrell and Reid (29). The milk energy output (MJ/d) was calculated as milk energy (MJ/kg) × milk yield (kg/d). The energy corrected milk (ECM) and 4% fat corrected milk (FCM) were calculated according to Sjaunja et al. (30) and Tyrrell and Reid (29), respectively.
Statistical analyses
Data from in vitro measurements were analyzed using the GLM procedure of SAS (SAS Inst. Inc. Cary, NC, USA) in a completely randomized design using the following model: Yij = μ + Di + Eij, where Yij represents the measured variable, μ is the overall mean, Di is the LAB dose, and Eij is the experimental error. Data from each of the three runs within the same sample were averaged prior to the statistical analysis. Polynomial (linear and quadratic) contrasts were used to examine dose responses for increasing levels of LAB.
Data from in vivo measurements were analyzed as a completely randomized design with repeated measures using the PROC MIXED procedure of SAS (SAS Institute, Cary, NC, USA), considering sampling time as repeated measures and individual cow as the experimental unit.
Data for variables measured daily for each week were averaged before statistical analyses. The statistical model included the treatment effect, week effect and the treatment × week interaction. Animal nested within treatment was considered the random effect, while treatment was the fixed effect. Two covariance structures were considered in the REPEATED statement in PROC MIXED: compound symmetry (cs) and auto-regressive [AR(1)]. The error structure, with the lowest Akaike information criteria, that fits the statistics was selected for the model. When the F-test was significant at P < 0.05, means were compared by applying the probability of difference option of the least squares means statement. Significance was declared at P < 0.05.
Results
Experiment 1 (in vitro experiment)
Results of TGP and CH4 proportions differed between the isolated and commercial strains of E. faecium (Table 2). The inclusion of isolated E. faecium linearly and quadratically increased (P < 0.001) TGP, while linearly and quadratically decreased (P < 0.05) proportional CH4, CH4 production (per g dDM, g dOM, g dNDF, g dADF) and pH, and increased SCFA (linear and quadratic effects, P < 0.001). Linear increases in dDM and dOM were observed with the isolated E. faecium, with no effect on dNDF or dADF. The inclusion of isolated E. faecium did not affect the concentration of ruminal NH3-N.
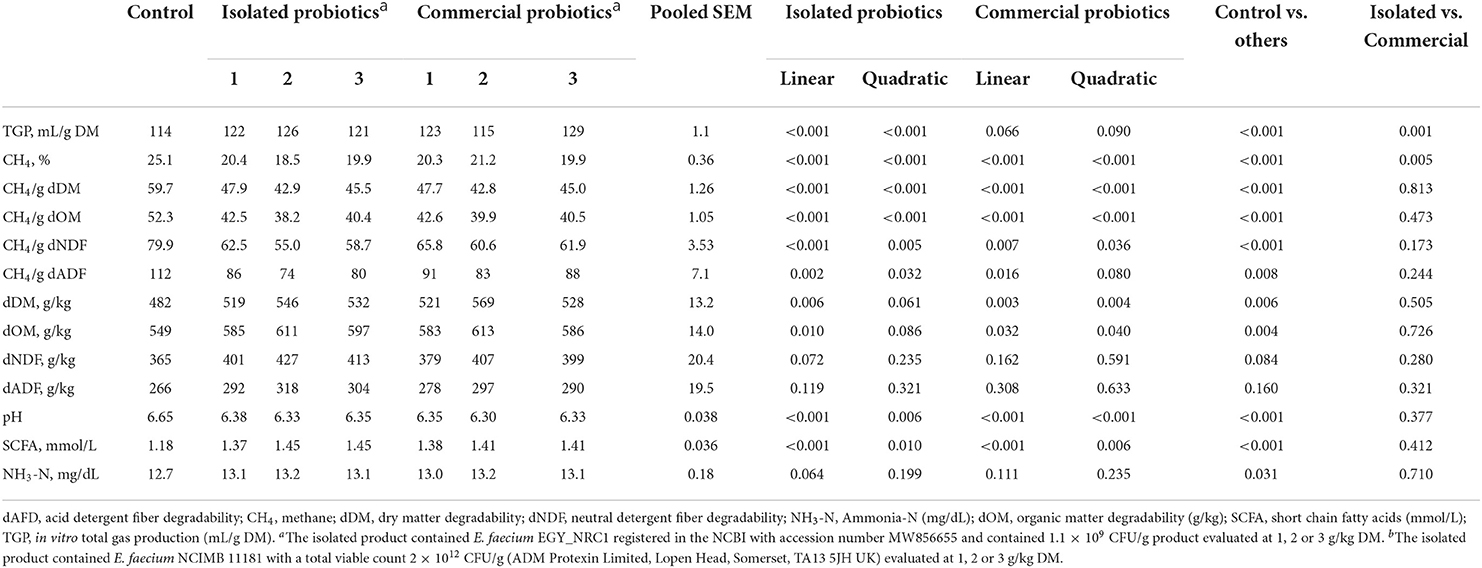
Table 2. In vitro fermentation from Experiment 1 (mean values), where a basal diet was supplemented with isolated or commercial E. faecium as probiotics at 1, 2 or 3 g/kg DM.
Increased TGP and decreased proportional CH4 (linear and quadratic effects, P < 0.01) were observed with the inclusion of the commercial E. faecium strain; however, CH4 production (per g dDM, g dOM, g dNDF, g dADF) and pH linearly decreased (P < 0.01), and dDM, dOM, SCFA and ruminal NH3-N concentration increased, without affecting dNDF and dADF.
Experiment 2 (lactation experiment)
Feed intake, nutrient digestibility, and blood measurements
Feeding E. faecium diets did not affect total feed intake (Table 3). Compared to the control, the highest (P < 0.01) DM, NDF and ADF degradabilities were observed with the isolated E. faecium followed by the commercial E. faecium, while both isolated and commercial E. faecium improved (P < 0.01) OM, CP, EE and NSC digestibility. The isolated E. faecium followed by the commercial E. faecium showed higher (P < 0.01) diet's nutritive value calculated as TDN, DE, ME, NEL, and UFL compared to the control treatment. Both the probiotic strains influenced an increased (P < 0.05) intake of digestible OM, TDN, ME and digestible CP compared to control, while between the strains the improvement in TDN, ME intake was superior (P < 0.05) in isolated than the commercial strain.
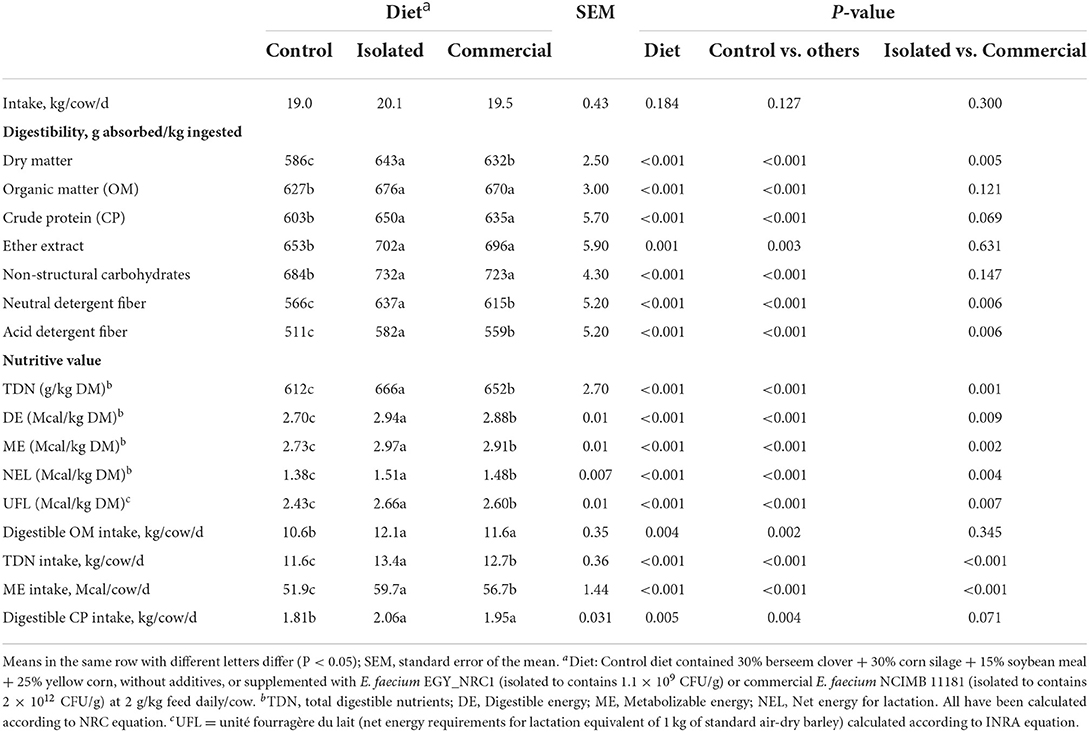
Table 3. Experiment 2: Intake, nutrient digestibility and nutritive value of diet supplemented with isolated and commercial E. faecium as probiotics and fed to lactating Holstein cows.
Isolated or commercial E. faecium did not affect concentrations of serum total protein, albumin, globulin, albumin: globulin ratio and urea-N (Table 4). Both isolated and commercial E. faecium increased serum glucose (P = 0.002) and decreased serum cholesterol (P = 0.002). The commercial E. faecium decreased (P = 0.002) serum triglycerides and ALT (P = 0.038), while the isolated E. faecium decreased serum AST (P = 0.023).
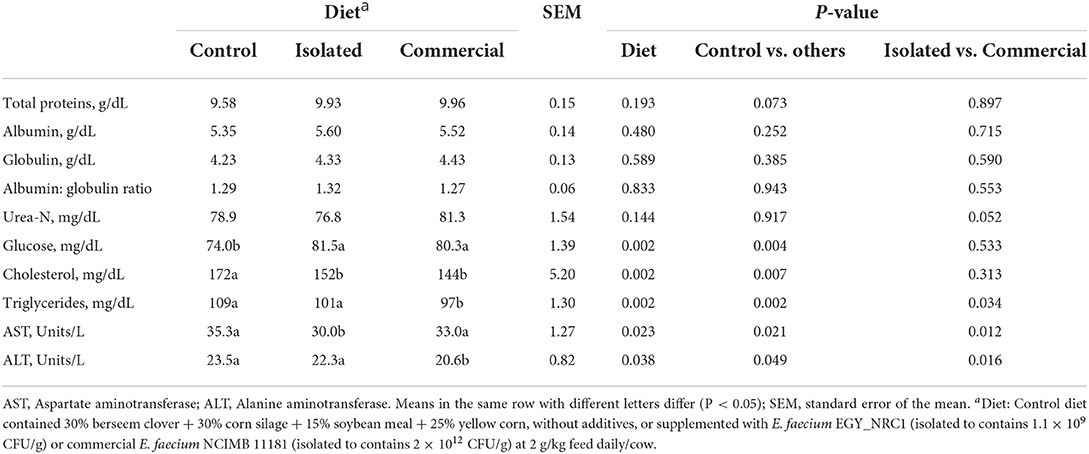
Table 4. Experiment 2: Blood parameters of Holstein cows fed diets supplemented with isolated or commercial E. faecium as probiotics.
Milk production, milk composition, and milk fatty acids
Compared to the control, the isolated E. faecium followed by the commercial E. faecium increase (P < 0.001) daily milk production (actual, ECM and FCM), daily milk component yields and milk energy output (Table 5). Moreover, the isolated E. faecium followed by the commercial E. faecium improved feed efficiency compared to the control treatment. Treatments did not affect the concentrations of milk components.
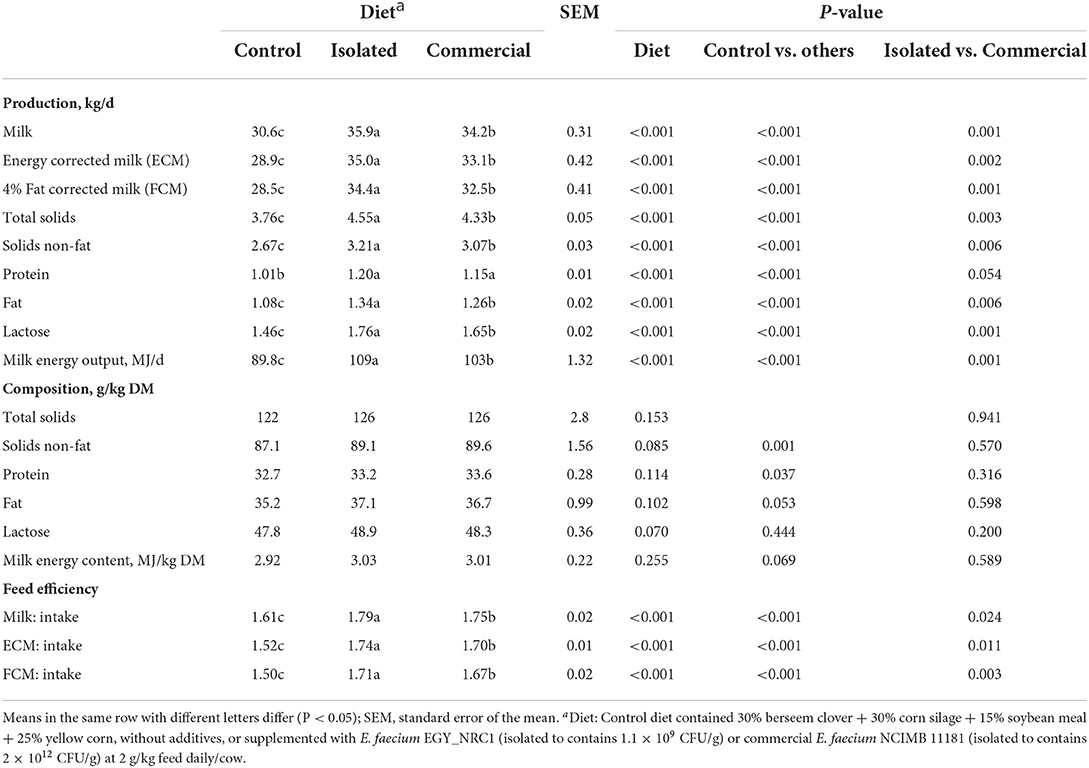
Table 5. Experiment 2: Milk production and composition, and feed efficiency of Holstein cows fed diets supplemented with isolated or commercial E. faecium as probiotics.
Both E. faecium strains decreased the proportion of C23:0 (P = 0.005) and increased (P = 0.017) C18:1 trans-9 in milk (Table 6). Compared to the control treatment, the isolated E. faecium increased (P < 0.05) the proportion of C18:1 trans-9, C18:2 cis-9-12 and C18:2 trans-10 cis-12, while the commercial E. faecium did not affect them.
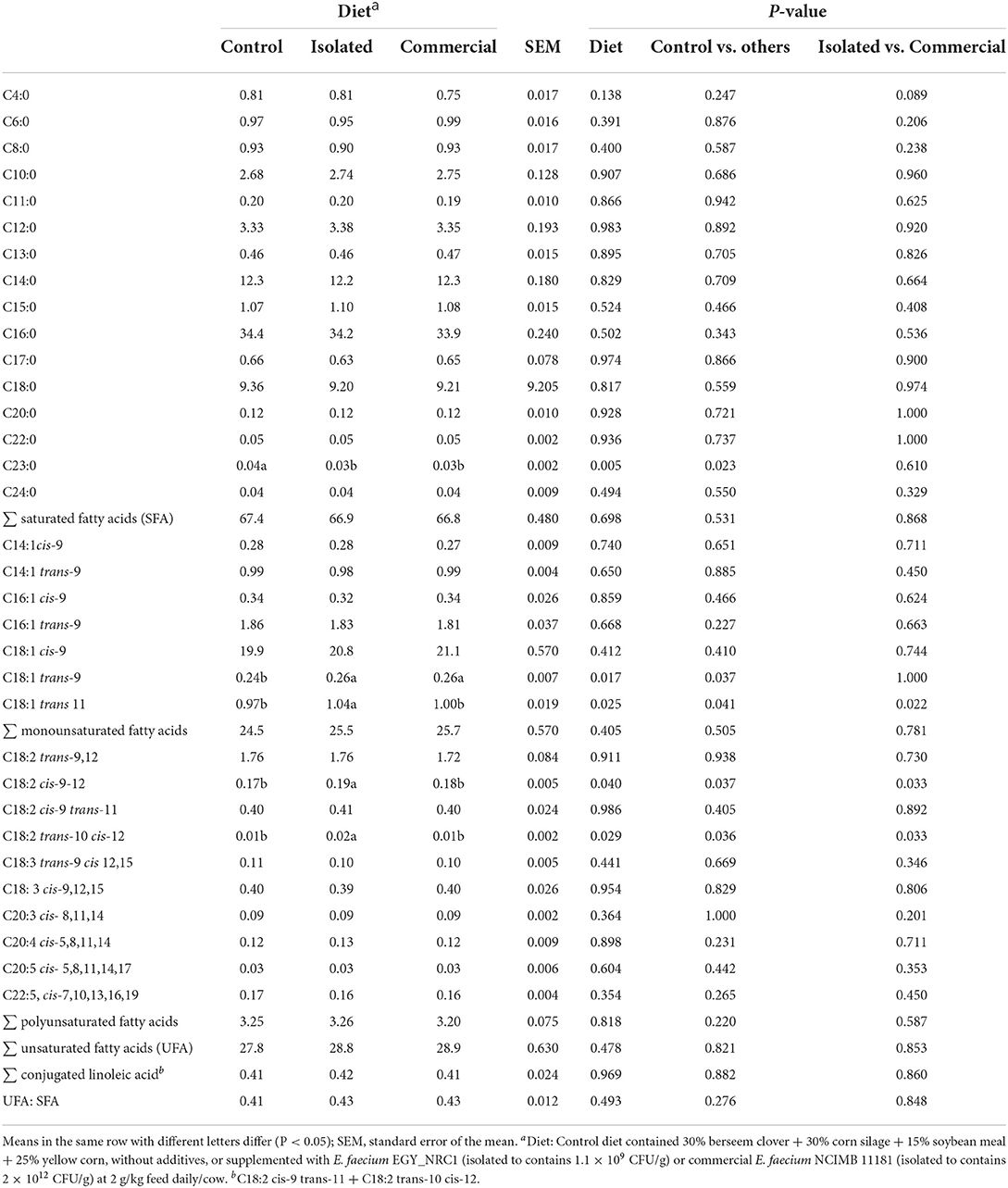
Table 6. Experiment 2: Milk fatty acid profile (g/100 g fatty acids) of lactating Holstein cows fed diets supplemented with isolated or commercial E. faecium as probiotics.
Discussion
As shown in Figure 1, the MALDI-TOF MS showed that the isolated strain of E. faecium possess antimicrobial activity which was initially identified with a high confidence value of 2.31 that indicated a reliable identification of the isolate up to species level. This result agreed with that of 16S rDNA sequencing data (31). The phylogenetic analysis and the 16S rDNA sequencing assigned all the E. faecium EGY NRC1 isolates belonged to E. faecium.
Experiment 1 (in vitro experiment)
The inclusion of isolated E. faecium (both strains) increased TGP. Generally, production of gases depends mainly on the composition and degradability of the incubated substrate and the concentration of the soluble components in the incubated substrates (32–34). In the present experiment, the composition of the diet and soluble components are the same between treatments indicating that the differences are mainly due to the strains of E. faecium. Jiao et al. (35) stated that specific LAB strains interact with rumen microorganisms to alter rumen fermentation with different modes of action in the rumen.
One promising area of research for the use of LAB in ruminant nutrition is its potential for reducing CH4 emissions (36). The isolated and commercial E. faecium decreased proportional CH4 and CH4 production per unit of degraded DM, OM, NDF and ADF which may be related to the reduced methanogenesis by stimulating the growth of lactate-utilizing bacteria such as Selenomonas ruminantium, Megasphaera elsdenii, and Veillonella parvula, which promotes H2 and CO2 sinks during the conversion of lactate to propionate (37). Moreover, LAB stimulates scavenging of hydrogen atoms to form propionate causing a lack of hydrogen as the main substrate for methanogenic bacteria (36). Cao et al. (11) observed a lowered in vitro CH4 production with LAB supplementation to a silage-based diet prepared with whole crop rice. To confirm our findings, further studies should consider analyzing rumen microbiome. The isolated strain of E. faecium increased in vitro TGP and decreased CH4 production compared to the commercial strain, with no clear reason indicating the need for experiments on genome sequence and their ability to produce bacteriocins and non-ribosomal synthesized peptides for explaining such effects (36). Such possible differences between strains or their metabolites will produce different abilities to shift rumen fermentation patterns, and to inhibit specific rumen bacteria that produce H2 or methyl-containing compounds that are the substrates for methanogenesis (36). Increasing TGP is not always advantageous but concurrent reduction in CH4 is definitely advantageous (38). The improved fiber digestion is the most probable reason for the lowered CH4 production (39).
The isolated and commercial strains increased dDM and dOM without affecting dNDF or dADF due to the high fermentative activities of LAB-probiotics. LAB can enhance the whole digestive process, the metabolic utilization of nutrients, and improve the feed efficiency by producing digestive enzymes (e.g., amylases, chitinases, lipases, phytases, proteases) or by just generating volatile fatty acids and B-vitamins: riboflavin, biotin, B12 vitamin (40). Cao et al. (11) observed increased in vitro DM degradability with LAB administration to total mixed ration silage containing whole crop rice.
The commercial E. faecium increased ruminal NH3-N concentration; however, the observed concentrations were greater than the optimum level (8.5 to over 30 mg NH3-N/dL) for rumen microbial proliferation (41). Basso et al. (10) observed no effects on ruminal pH when lambs were fed a diet treated with LAB. However, the isolated and commercial E. faecium decreased fermentation pH, which was somehow mirrored by the obtained SCFA. The isolated and commercial E. faecium increased SCFA concentrations, and this may be related to the improved DM and OM digestibility. So et al. (13) reported increased total SCFA in cows fed diets supplemented with LAB. The observed fermentation pH values in all treatments were greater than the optimum level (5.6) for ruminal fiber degrading and microbial growth (42), without changing ruminal fibrolytic and amylolytic microbial communities (43).
The quadratic effects of treatments (levels of E. faecium) on some parameters are important to emphasize the importance of defining the optimal dose of E. faecium that may improve animal performance. Therefore, the medium dose of E. faecium (2 g/kg feed) was recommended for the in vivo experiment on lactating cows, as this dose showed better effects compared to the low and high doses.
Experiment 2 (lactation experiment)
It was not possible to obtain ruminal contents from cows because the experiment was done in a commercial farm without access to rumen-fistulated lactating cows. Therefore, the in vitro approach shows results that may partially explain and/or support the outcomes of the lactation experiment.
Feed intake, nutrient digestibility, and blood measurements
E. faecium supplementation did not affect feed intake which partly indicates unchanged feed palatability or acceptability. Other studies reported minor effects on feed intake in lambs and ewes fed with probiotics (8, 44), while So et al. (13) observed increased feed intake with LAB supplementation to lactating cows.
The isolated E. faecium improved the digestibility of DM, NDF and ADF compared to the commercial E. faecium; however, both isolated and commercial strains improved OM, CP, EE and NSC digestibility revealing the potential of E. faecium for improving nutrient digestibility. Similarly, So et al. (13) observed improved nutrient digestibility with LAB supplementation in lactating cows. Fiber degradability results are not consistent with those of the in vitro experiment (Experiment 1), which may be due to the conditions of both experiments (in vitro vs. in vivo conditions) and the fact that feeding bacterial direct fed microbial to livestock is based primarily on potential postruminal effects which is not available in the in vitro experiments (35). In this regard, probiotics change rumen fermentation rates and patterns (45), with beneficial effects on the gastrointestinal tract and rumen (46). Additionally, the supplement contains LAB which has a strong inhibitory effect on gastrointestinal infection by pathogens via the production of antimicrobial agents (46). It is expected that E. faecium, especially, the isolated strain, improved growth or activity of ruminal cellulolytic microbial populations and stabilizes the rumen pH (47), leading to improved nutrient digestion (45) and synthesis of microbial proteins (48). As previously noted, the isolated strain improved digestibility of DM, NDF, and ADF, and diet's nutritive value compared to the commercial strain, which confirm our assumption that the genome of both strains differs. The possible differed production of metabolites and bacteriocins may affect the composition of rumen microbiome, especially in those involved in fiber digestion (36). As observed in this study, previous experiments on lambs (49, 50), and lactating ewes (8) reported that probiotics improved nutrient digestibility.
All the measured serum biochemical parameters were within the standard physiological ranges for healthy cows (51). Treatments did not affect the concentrations of serum total protein, albumin, globulin, albumin: globulin ratio and urea-N indicating minimal effects on cow's nutritional status, muscle protein catabolism and kidney function (52). Both E. faecium strains increased serum glucose because of improved apparent OM and NSC. The levels of serum glucose were above the range (40–60 mg/dL), indicating an adequate energy supply for cows without risk of negative energy balance occurring (53). Further studies should follow on these findings as E. faecium supplementation could be helpful during the transition period.
Both E. faecium strains decreased serum cholesterol while commercial E. faecium decrease serum triglycerides showing the ability of E. faecium bacteria to deconjugate bile salts by a specific hydrolase causing a reduction in cholesterol and triglycerides absorption at the intestinal level (54). Additionally, the commercial E. faecium decreased serum ALT, while isolated E. faecium decreased serum AST showing its potential to improve liver activity, function, and health in cows (55).
Milk production, milk composition, and milk fatty acids
In this study, both isolated and commercial E. faecium increased daily milk production by 17.1 and 11.7%, ECM by 21.4 and 14.8% and FCM 20.9 and 14.2%, respectively which is similar to other studies (8, 13, 44, 56) that reported a positive relationship between supplementation of ruminant diets with probiotics and animal performance. The improved nutrient digestibility and increased blood glucose with probiotics supplementation can be considered the main reasons for increasing milk production (44). An improvement in digestibility and intake of nutrients (ME and TDN) supported release of important nutrients required for milk components synthesis (41). Moreover, the improved feed efficiency with the additives is another probable reason for the improved performance (41). As previously noted in Experiment 1, E. faecium decreased CH4 production indicating that a possible suppression in CH4 production would have redistributed energy for improved milk production (57). In this study, the antagonism of pathogenic organisms via antimicrobial effects, competition for adhesion sites or nutrients, stimulation of host defines mechanisms and inhibition of bacterial toxins can partially explain the improved milk production (9, 45).
Moreover, LAB increases the release of different endogenous substances, including antibacterial substances, nutrients, antioxidants, growth factors and coagulating agents, enhances performance and reduces the incidence of diarrhea by increasing the number of beneficial microorganisms in the rumen (45, 58) and enhancing animal health (59), which could also explain the increased milk production. As a result of unchanged feed intake and increased daily milk production, the isolated and commercial E. faecium improved feed efficiency by 11.2 and 8.7% (milk: intake ratio), 14.5 and 11.8 (ECM: intake ratio), and 14 and 11.3% (FCM: intake ratio), respectively. Frizzo et al. (60) observed that supplementing diets of lactating cows with LAB including E. faecium improved feed efficiency.
The weak effect of treatment on the concentrations of milk components is inconsistent with other experiments (8) that reported some changes in the concentrations of total n-3, n-6 fatty acids, polyunsaturated fatty acids and conjugated linoleic acids components when sheep were fed diet supplemented with LAB. However, more experiments are required to explore these effects.
Plasma uptake of fatty acids is responsible for about half of milk fatty acids and the rest amount is synthesized in the mammary gland (61, 62). The improved fiber digestion with the supplementation might be associated with altered milk fatty acid profiles. Both E. faecium strains increased the proportion of C18:1 trans-9, while the isolated E. faecium increased the proportion of C18:1 trans-9, C18:2 cis-9-12 and C18:2 trans-10 cis-12. The observed changes in milk fatty acids are a result of biohydrogenation of dietary PUFA (63). Further attention should be paid to the use of E. faecium on cow diets as they may increase the formation of bioactive fatty acids such as C18:1 trans-11 and C18:2 cis-9-12.
Conclusion
Daily supplementation of cows with E. faecium (isolated and commercial) at 2 g/kg DM feed improved in vitro nutrient degradation and cows feed digestion, milk production and feed efficiency. The isolated strain of E. faecium showed better results compared to the commercial strain. Minimal effects were observed with E. faecium supplementation on milk fatty acid profile. Our data could be useful for producers looking for probiotics generated from byproducts for improving feed utilization and milk production.
Data availability statement
The raw data supporting the conclusions of this article will be made available by the authors, without undue reservation. The names of the repository/repositories and accession number(s) can be found below: NCBI accession number MW856655.
Ethics statement
The animal study was reviewed and approved by the Technical Committee of the Science, Technology & Innovation Funding Authority (STDF), Egypt (project STDF 33413).
Author contributions
HA, AK, and HM: conceptualization, methodology, validation, formal analysis, visualization, and supervision. AK and EV-B-P: writing—original draft preparation and writing—review and editing. HA and AK: investigation and data curation. All authors have read and agreed to the published version of the manuscript.
Conflict of interest
The authors declare that the research was conducted in the absence of any commercial or financial relationships that could be construed as a potential conflict of interest.
Publisher's note
All claims expressed in this article are solely those of the authors and do not necessarily represent those of their affiliated organizations, or those of the publisher, the editors and the reviewers. Any product that may be evaluated in this article, or claim that may be made by its manufacturer, is not guaranteed or endorsed by the publisher.
References
1. Reuben RC, Elghandour MMY, Alqaisi O, Cone JW, Márquez O, Salem AZM. Influence of microbial probiotics on ruminant health and nutrition: sources, mode of action and implications. J Sci Food Agric. (2022) 102:1319–40. doi: 10.1002/jsfa.11643
2. Sun WT, Huang Y, Wu CR, Peng C, Zheng YL, Chen C, et al. Addition of lactic acid bacteria can promote the quality and feeding value of broussonetia papyrifera (paper mulberry) silage. Fermentation. (2022) 8:25. doi: 10.3390/fermentation8010025
3. Kholif AE, Gouda GA, Patra AK. The sustainable mitigation of in vitro ruminal biogas emissions by ensiling date palm leaves and rice straw with lactic acid bacteria and Pleurotus ostreatus for cleaner livestock production. J Appl Microbiol. (2022) 132:2925–39. doi: 10.1111/jam.15432
4. Kaewpila C, Gunun P, Kesorn P, Subepang S, Thip-uten S, Cai Y, et al. Improving ensiling characteristics by adding lactic acid bacteria modifies in vitro digestibility and methane production of forage-sorghum mixture silage. Sci Rep. (2021) 11:1968. doi: 10.1038/s41598-021-81505-z
5. Miranda C, Contente D, Igrejas G, Câmara SPA, Dapkevicius M de LE, Poeta P. Role of exposure to lactic acid bacteria from foods of animal origin in human health. Foods. (2021) 10:2092. doi: 10.3390/foods10092092
6. Ezema. Probiotics in animal production: a review. J Vet Med Anim Health. (2013) 5:308–16. doi: 10.5897/JVMAH2013.0201
7. Vibhute VM, Shelke RR, Chavan SD, Nage SP. Effect of probiotics supplementation on the performance of lactating crossbred cows. Vet World. (2011) 4:557–61. doi: 10.5455/vetworld.2011.557-561
8. Kholif AE, Hamdon HA, Gouda GA, Kassab AY, Morsy TA, Patra AK. Feeding date-palm leaves ensiled with fibrolytic enzymes or multi-species probiotics to Farafra ewes: intake, digestibility, ruminal fermentation, blood chemistry, milk production and milk fatty acid profile. Animals. (2022) 12:1107. doi: 10.3390/ani12091107
9. Elghandour MMY, Salem AZM, Castañeda JSM, Camacho LM, Kholif AE, Chagoyán JCV. Direct-fed microbes: a tool for improving the utilization of low quality roughages in ruminants. J Integr Agric. (2015) 14:526–33. doi: 10.1016/S2095-3119(14)60834-0
10. Basso FC, Adesogan AT, Lara EC, Rabelo CHS, Berchielli TT, Teixeira IAMA, et al. Effects of feeding corn silage inoculated with microbial additives on the ruminal fermentation, microbial protein yield, and growth performance of lambs. J Anim Sci. (2014) 92:5640–50. doi: 10.2527/jas.2014-8258
11. Cao Y, Takahashi T, Horiguchi KI, Yoshida N. Effect of adding lactic acid bacteria and molasses on fermentation quality and in vitro ruminal digestion of total mixed ration silage prepared with whole crop rice. Grassl Sci. (2010) 56:19–25. doi: 10.1111/j.1744-697X.2009.00168.x
12. Xing L, Chen LJ, Han LJ. The effect of an inoculant and enzymes on fermentation and nutritive value of sorghum straw silages. Bioresour Technol. (2009) 100:488–91. doi: 10.1016/j.biortech.2008.06.017
13. So S, Wanapat M, Cherdthong A. Effect of sugarcane bagasse as industrial by-products treated with Lactobacillus casei TH14, cellulase and molasses on feed utilization, ruminal ecology and milk production of mid-lactating Holstein Friesian cows. J Sci Food Agric. (2021) 101:4481–9. doi: 10.1002/jsfa.11087
14. Mac Faddin JF. Biochemical Tests for Identification of Medical Bacteria. 3rd ed. Philadelphia, PA: Lippincott Williams & Wilkins (2000).
15. Niven CF, Smiley KL, Sherman JM. The hydrolysis of arginine by Streptococci. J Bacteriol. (1942) 43:651–60. doi: 10.1128/jb.43.6.651-660.1942
16. Kanak EK, Yilmaz SÖ. Maldi-tof mass spectrometry for the identification and detection of antimicrobial activity of lactic acid bacteria isolated from local cheeses. Food Sci Technol. (2019) 39:462–9. doi: 10.1590/fst.19418
17. Liu W, Sun Z, Zhang J, Gao W, Wang W, Wu L, et al. Analysis of microbial composition in acid whey for dairy fan making in yunnan by conventional method and 16S rRNA sequencing. Curr Microbiol. (2009) 59:199–205. doi: 10.1007/s00284-009-9423-x
18. Tamura K, Stecher G, Peterson D, Filipski A, Kumar S. MEGA6: molecular evolutionary genetics analysis version 60. Mol Biol Evol. (2013) 30:2725–9. doi: 10.1093/molbev/mst197
19. Theodorou MK, Williams BA, Dhanoa MS, McAllan AB, France J. A simple gas production method using a pressure transducer to determine the fermentation kinetics of ruminant feeds. Anim Feed Sci Technol. (1994) 48:185–97. doi: 10.1016/0377-8401(94)90171-6
20. Mauricio RM, Mould FL, Dhanoa MS, Owen E, Channa KS, Theodorou MK, et al. semi-automated in vitro gas production technique for ruminant feedstuff evaluation. Anim Feed Sci Technol. (1999) 79:321–30. doi: 10.1016/S0377-8401(99)00033-4
21. Huo W, Wang X, Wei Z, Zhang H, Liu Q, Zhang S, et al. Effect of lactic acid bacteria on the ensiling characteristics and in vitro ruminal fermentation parameters of alfalfa silage. Ital J Anim Sci. (2021) 20:623–31. doi: 10.1080/1828051X.2021.1906167
22. AOAC. Official Methods of Analysis of AOAC International. 18th ed. Washington, DC: AOAC International (2005).
23. Warner AC. Production of volatile fatty acids in the rumen: methods of measurement. Nutr Abstr Rev. (1964) 34:339–52.
24. NRC. Nutrient Requirements of Dairy Cattle. 7th ed. Washington, DC: National Academies Press (2001).
25. Sales J, Janssens GPJ. Acid-insoluble ash as a marker in digestibility studies: a review. J Anim Feed Sci. (2003) 12:383–401. doi: 10.22358/jafs/67718/2003
26. Ferret A, Plaixats J, Caja G, Gasa J, Prió P. Using markers to estimate apparent dry matter digestibility, faecal output and dry matter intake in dairy ewes fed Italian ryegrass hay or alfalfa hay. Small Ruminant Research. (1999) 33:145–52. doi: 10.1016/S0921-4488(99)00015-2
27. INRA. INRA Feeding System for Ruminants. The Netherlands: Wageningen Academic Publishers (2018).
28. Van Soest PJ, Robertson JB, Lewis BA. Methods for dietary fiber, neutral detergent fiber, and nonstarch polysaccharides in relation to animal nutrition. J Dairy Sci. (1991) 74:3583–97. doi: 10.3168/jds.S0022-0302(91)78551-2
29. Tyrrell HF, Reid JT. Prediction of the energy value of cow's milk. J Dairy Sci. (1965) 48:1215–23. doi: 10.3168/jds.S0022-0302(65)88430-2
30. Sjaunja LO, Baevre L, Junkkarinen L, Pedersen J, Setala J. A nordic proposal for an energy corrected milk (ECM) formula: performance recording of animals. State Art EAAP Publication. (1991) 50:156–7.
31. Murad HA, Hosseany EN, Abd Elhamid SM, Abu-El Khair AG, Zahran MO, Azzaz HH. Yeast biomass production from milk permeate with enrichment application of dairy animal's diets. Inter J Dairy Sci. (2020) 15:134–141. doi: 10.3923/ijds.2020.134.141
32. Elghandour MMY, Kholif AE, Hernández A, Salem AZM, Mellado M, Odongo NE. Effects of organic acid salts on ruminal biogas production and fermentation kinetics of total mixed rations with different maize silage to concentrate ratios. J Clean Prod. (2017) 147:523–30. doi: 10.1016/j.jclepro.2017.01.078
33. Blümmel M, Makkar HPS, Becker K. In vitro gas production: a technique revisited. J Anim Physiol Anim Nutr. (1997) 77:24–34. doi: 10.1111/j.1439-0396.1997.tb00734.x
34. Pal K, Patra AK, Sahoo A, Kumawat PK. Evaluation of several tropical tree leaves for methane production potential, degradability and rumen fermentation in vitro. Livest Sci. (2015) 180:98–105. doi: 10.1016/j.livsci.2015.07.011
35. Jiao PX, Liu FZ, Beauchemin KA, Yang WZ. Impact of strain and dose of lactic acid bacteria on in vitro ruminal fermentation with varying media pH levels and feed substrates. Anim Feed Sci Technol. (2017) 224:1–13. doi: 10.1016/j.anifeedsci.2016.11.005
36. Doyle N, Mbandlwa P, Kelly WJ, Attwood G, Li Y, Ross RP, et al. Use of lactic acid bacteria to reduce methane production in ruminants, a critical review. Front Microbiol. (2019) 10:2207. doi: 10.3389/fmicb.2019.02207
37. Cao Y, Cai Y, Takahashi T, Yoshida N, Tohno M, Uegaki R, et al. Effect of lactic acid bacteria inoculant and beet pulp addition on fermentation characteristics and in vitro ruminal digestion of vegetable residue silage. J Dairy Sci. (2011) 94:3902–12. doi: 10.3168/jds.2010-3623
38. Elghandour MMY, Kholif AE, Salem AZM, Olafadehan OA, Kholif AM. Sustainable anaerobic rumen methane and carbon dioxide productions from prickly pear cactus flour by organic acid salts addition. J Clean Prod. (2016) 139:1362–9. doi: 10.1016/j.jclepro.2016.08.075
39. Elghandour MMY, Kholif AE, Salem AZM, Montes de Oca R, Barbabosa A, Mariezcurrena M, et al. Addressing sustainable ruminal methane and carbon dioxide emissions of soybean hulls by organic acid salts. J Clean Prod. (2016) 135:194–200. doi: 10.1016/j.jclepro.2016.06.081
40. Vieco-Saiz N, Belguesmia Y, Raspoet R, Auclair E, Gancel F, Kempf I, et al. Benefits and inputs from lactic acid bacteria and their bacteriocins as alternatives to antibiotic growth promoters during food-animal production. Front Microbiol. (2019) 10:57. doi: 10.3389/fmicb.2019.00057
41. Jones M, Jones G. Animal Nutrition. 7th ed. UK: Pearson Education Limited (2012). p. 64–83. doi: 10.1017/CBO9780511862793.008
42. Ryle M, Ørskov ER. Energy Nutrition in Ruminants. Dordrecht: Springer Netherlands (1990). p. 149. doi: 10.1007/978-94-009-0751-5
43. Tajima K, Aminov RI, Nagamine T, Matsui H, Nakamura M, Benno Y. Diet-dependent shifts in the bacterial population of the rumen revealed with real-time PCR. Appl Environ Microbiol. (2001) 67:2766–74. doi: 10.1128/AEM.67.6.2766-2774.2001
44. Sallam SMA, Kholif AE, Amin KA, El-Din ANMN, Attia MFA, Matloup OH, et al. Effects of microbial feed additives on feed utilization and growth performance in growing Barki lambs fed diet based on peanut hay. Anim Biotechnol. (2020) 31:447–54. doi: 10.1080/10495398.2019.1616554
45. Kassa SR. Role of probiotics in rumen fermentation and animal performance: a review. Int J Livest Prod. (2016) 7:24–32. doi: 10.5897/IJLP2016.0285
46. Seo JK, Kim SW, Kim MH, Upadhaya SD, Kam DK, Ha JK. Direct-fed microbials for ruminant animals. Asian-Australas J Anim Sci. (2010) 23:1657–67. doi: 10.5713/ajas.2010.r.08
47. Ghazanfar S, Anjum MI, Azim A, Ahmed I. Effects of dietary supplementation of yeast (Saccharomyces cerevisiae) culture on growth performance, blood parameters, nutrient digestibility and fecal flora of dairy heifers. J Anim Plant Sci. (2015) 25:53–9.
48. Uyeno Y, Shigemori S, Shimosato T. Effect of probiotics/prebiotics on cattle health and productivity. Microbes Environ. (2015) 30:126–32. doi: 10.1264/jsme2.ME14176
49. Saleem AM, Zanouny AI, Singer AM. Growth performance, nutrients digestibility, and blood metabolites of lambs fed diets supplemented with probiotics during pre- and post-weaning period. Asian-Australas J Anim Sci. (2017) 30:523–30. doi: 10.5713/ajas.16.0691
50. Hamdon HA, Kholif AE, Mahmoud GB, Khalifa AMA, Abdel Ati MNM. Enhancing the utilization of palm leaf hay using Bacillus subtilis and Phanerochaete chrysosporium in the diet of lambs under desert conditions. Ann Anim Sci. (2020) 20:1395–409. doi: 10.2478/aoas-2020-0052
51. Jerry Kaneko J, Harvey JJ, Bruss ML. Clinical Biochemistry of Domestic Animals. Elsevier (2008). doi: 10.1016/B978-0-12-370491-7.X0001-3
52. Hosten AO. BUN and Creatinine. In:Walker HK, Hall WD, Hurst JW, , editors. Clinical Methods: The History, Physical, and Laboratory Examinations. Boston, MA: Butterworths (1990). p. 874–8.
53. Mair B, Drillich M, Klein-Jöbstl D, Kanz P, Borchardt S, Meyer L, et al. Glucose concentration in capillary blood of dairy cows obtained by a minimally invasive lancet technique and determined with three different hand-held devices. BMC Vet Res. (2016) 12: Article number: 34. doi: 10.1186/s12917-016-0662-3
54. Kumar M, Nagpal R, Kumar R, Hemalatha R, Verma V, et al. Cholesterol-lowering probiotics as potential biotherapeutics for metabolic diseases. Exp Diabetes Res. (2012). 2012:902917. doi: 10.1155/2012/902917
55. Pettersson J, Hindorf U, Persson P, Bengtsson T, Malmqvist U, Werkström V, et al. Muscular exercise can cause highly pathological liver function tests in healthy men. Br J Clin Pharmacol. (2008) 65:253–9. doi: 10.1111/j.1365-2125.2007.03001.x
56. Abd El Tawab AM, Kholif AE, Hassan AM, Matloup OH, El-Nor SAA, Olafadehan OA, et al. Feed utilization and lactational performance of Friesian cows fed beet tops silage treated with lactic acid bacteria as a replacement for corn silage. Anim Biotechnol. (2020) 31:473–82. doi: 10.1080/10495398.2019.1622556
57. Tseten T, Sanjorjo RA, Kwon M, Kim SW. Strategies to Mitigate Enteric Methane Emissions from Ruminant Animals. J Microbiol Biotechnol. (2022) 32:269–277. doi: 10.4014/jmb.2202.02019
58. Pant B, Lohani V, Mishra A, Trakroo MD, Tewari H. Effect of Probiotic Supplementation on Growth of Carp Fingerlings. National Academy Science Letters. (2019) 42:215–220. doi: 10.1007/s40009-018-0733-z
59. Bielke LR, Elwood AL, Donoghue DJ, Donoghue AM, Newberry LA, Neighbor NK, Hargis BM. Approach for selection of individual enteric bacteria for competitive exclusion in Turkey poults. Poult Sci. (2003) 82:1378–1382. doi: 10.1093/ps/82.9.1378
60. Frizzo LS, Zbrun M V, Soto LP, Signorini ML. Effects of probiotics on growth performance in young calves: A meta-analysis of randomized controlled trials. Anim Feed Sci Technol. (2011) 169:147–156. doi: 10.1016/j.anifeedsci.2011.06.009
61. Chilliard Y, Ferlay A. Dietary lipids and forages interactions on cow and goat milk fatty acid composition and sensory properties. Reprod Nutr Dev. (2004) 44:467–492. doi: 10.1051/rnd:2004052
62. Bionaz M, Vargas-Bello-Pérez E, Busato S. Advances in fatty acids nutrition in dairy cows: from gut to cells and effects on performance. J Anim Sci Biotechnol. (2020) 11. doi: 10.1186/s40104-020-00512-8
Keywords: Enterococcus faecium, digestion, feed utilization, lactic acid bacteria, milk production
Citation: Azzaz HH, Kholif AE, Murad HA and Vargas-Bello-Pérez E (2022) A newly developed strain of Enterococcus faecium isolated from fresh dairy products to be used as a probiotic in lactating Holstein cows. Front. Vet. Sci. 9:989606. doi: 10.3389/fvets.2022.989606
Received: 08 July 2022; Accepted: 15 September 2022;
Published: 13 October 2022.
Edited by:
Muhammad Saeed, Northwest A&F University, ChinaReviewed by:
Pablo Jesús Marín-García, Universidad CEU Cardenal Herrera, SpainNeeta Agarwal, Indian Veterinary Research Institute (IVRI), India
Artabandhu Sahoo, National Research Centre on Camel (ICAR), India
Copyright © 2022 Azzaz, Kholif, Murad and Vargas-Bello-Pérez. This is an open-access article distributed under the terms of the Creative Commons Attribution License (CC BY). The use, distribution or reproduction in other forums is permitted, provided the original author(s) and the copyright owner(s) are credited and that the original publication in this journal is cited, in accordance with accepted academic practice. No use, distribution or reproduction is permitted which does not comply with these terms.
*Correspondence: Ahmed E. Kholif, YWVfa2hvbGlmJiN4MDAwNDA7bGl2ZS5jb20=; Einar Vargas-Bello-Pérez, ZS52YXJnYXNiZWxsb3BlcmV6JiN4MDAwNDA7cmVhZGluZy5hYy51aw==