- 1Departmento de Producción Animal, Facultad de Medicina Veterinaria y Zootecnia, Universidad Autónoma del Estado de México, Toluca, Mexico
- 2División Académica de Ciencias Agropecuarias, Universidad Juárez Autónoma de Tabasco, Villahermosa, Mexico
- 3Institute of Feed Research of Chinese Academy of Agricultural Sciences, Key Laboratory of Feed Biotechnology of the Ministry of Agriculture and Rural Affairs, Beijing, China
- 4Department of Animal Sciences, School of Agriculture, Policy and Development, University of Reading, Reading, United Kingdom
Mexico has many agricultural by-products that can be used for animal feed, and green tomatoes are produced throughout the country and can be an alternative to overcome the high prices of cereal-based feeds. This study determined in vitro fermentation kinetics, production performance, nutrient intake, digestibility, and nitrogen balance from sheep supplemented with whole plant green tomato (GT) on corn silage (CS) based diets. For 21 days, eighteen Suffolk lambs (38 ± 4 kg of live weight) were grouped into three dietary GT inclusion levels to replace CS: a control diet based on 100% CS (GT0, 570 g /kg dry matter, DM), while 100 g/kg DM (GT100) and 200 g/kg DM (GT200) of GT were included as a replacement for CS. A completely randomized design was used to measure in vitro gas production, in vitro rumen fermentation, chemical composition, and in vivo parameters. In vitro gas production, “A” (ml/g DM), fermentation rates “B,” (h−1), and “C” (h−½), were lower for GT200, while DM disappearance (mg/100mg) was lower for GT100 compared with GT0. Compared to GT0, GT100 and GT200 did not affect (P > 0.05) DM and organic matter (OM) intake (g/kgLW0.75). Ether extract intake was higher for GT0 and GT100 (P < 0.001) compared to GT200. Neutral detergent fiber (NDF) intake was higher (P < 0.05) for GT200 compared with GT0. Intake of lignin was higher (P < 0.001) for GT200 than that of GT0 and GT100. Digestibility coefficients for DM, OM, NDF, and Acid detergent fiber (ADF) were lower (P < 0.05) in GT100 than in the rest of the treatments. Nitrogen intake and N excreted in feces and urine were lower (P < 0.001) for GT0. N balance was negative for all treatments, being higher for GT200 (P < 0.05). Overall, the addition of GT at 100 or 200 g/kg DM in sheep diets negatively affects nutrient digestibility and N balance, so their dietary inclusion is not recommended.
Introduction
Mexico is an important producer and exporter of vegetables worldwide and the main vegetables produced are green (Physalis philadelphica) and red (Solanum lycopersicum) tomatoes. Mexico has many agricultural by-products that can be used for animal feeding, and this is an alternative to overcome the high prices of cereal-based feeds (1). Red tomato by-products such as hay or silage have been used in sheep (1, 2) diets. Forages with high water content can be cut and dried in the field until they reach 35% dry matter (DM) and then ensiled to conserve their nutritional content (3). Green tomato is harvested in almost all-Mexican territory, producing around 7,712 thousand tons per year (4). The importance of green tomatoes lies in their culinary use, and their antioxidant and vitamin contents (5), and when tomatoes are harvested, the plant residues are left on the field and used as organic matter for soil improvement. These by-products could be used as ruminant feed, either as a grazing material or cut fresh (6). To date, there are no reports on the use of green tomato silage for ruminant feed. The hypothesis of this study was that green tomato silage can alternate with corn silage in lamb diets, thus two experiments (in vitro and in vivo) were conducted to determine the effect of different inclusion levels of whole plant green tomatoes on in vitro gas production and rumen fermentation kinetics, nutrient intake, digestibility, and nitrogen balance in sheep.
Materials and methods
Green tomatoes and corn crops
Forage samples of whole plant green tomatoes (Physalis philadelphica) with fruits were collected at 2,840 m above sea level, between the coordinates 19° 04' north latitude and 99°32' west longitude. The climate is humid temperate, Cb (w2) (w) (i') (g), with summer rains and little thermal oscillation (7). Green tomatoes (GT) seeds were planted in May 2019. A total area of 100 m2 was previously fertilized with sheep manure. For the study, the vegetative stage of the plant was left to mature until fruits were harvested at 90 days after cropping. Then, GT whole plant was chopped in a hammer mill (size 5 cm Ø) and left to sun dry for 4 days. In another crop, 100 m2 of whole corn plants were harvested when the plant was in a milky stage, and they were chopped using a hammer mill (5 cm Ø) for subsequent corn silage (CS) production. Then, silages from green tomatoes and corn, were placed in layers, compacted, sealed, and ensiled in six hard plastic containers (975 mm in height, 594 mm Ø, and 208 liters of capacity) per treatment (GT Whole Plant or CS Whole Plant), together with the addition of 0.001% fresh Pulque as an inoculant to accelerate the silage fermentation process (8).
In vitro gas production
A buffer solution was prepared according to Menke and Steingass (9), where 200 mg DM of each diet mixture was incubated in glass syringes of 100 mL. Ruminal fluid (300 mL per animal) was obtained from three fistulated male Suffolk sheep ([62 ± 3 kg Live weight (LW) (average ± SD)]), previously fed with the control diet. Ruminal fluid was extracted and filtered through a triple layer of gauze and homogenized with CO2 for 5 min. Glass syringes were filled under anaerobic conditions with 200 mL of the previous mixture (100 mL of rumen inoculum and 900 mL of an incubation solution). In 1 L, this solution consisted of 238 mL/L of buffer solution [14 g NaHCO3 and 1.5 g (NH4)HCO3 per L], 238 mL/L of a macro mineral solution (5.7 g Na2HPO4, 6.2 g KH2PO4 and 0.6 g MgSO4.7H2O per L), 474 mL/L of distilled water, 0.1 mL/L of micro minerals (13.2 g CuCl2.2H2O, 10.0 g MnCl24H2O, 1.0 g CoCl2.6H2O, 8.0 g FeCl2.6H2O and made up to 100 mL with H2O) and 50 mL/L of a reduction solution (47.5 mL distilled water, 2 mL of 1N NaOH and 313 mg HCl-cysteine), and resazurin (phenoxazine dye). Two additional syringes without substrate were also prepared as blanks to account for the presence of other soluble extracts on overall gas production and to correct readings for substrate, including syringes from the self-fermentation of rumen inoculum. Glass syringes per triplicate per treatment, were filled with the incubation solution under a CO2 stream, and incubated for 96 h in a water bath at 39°C. The gas volume was recorded at 3, 6, 9, 12, 24, 36, 48, 60, 72, 84, and 96 h of incubation in three series of incubation.
Animals and diets
All experimental procedures were approved by the Animal Experimental Guidelines of the Universidad Autonoma del Estado de México (project code UAEMex 4974/2020). Eighteen male Suffolk lambs [38 ± 4 kg LW (average ± SD)], were arranged in a completely randomized design for a 21-day period that consisted of 14 d for diet adaptation and 7 d for sample collection.
The control diet was formulated according to the nutritional requirements for growing sheep (10) receiving 105 g crude protein (CP)/d and 10.5 MJ metabolizable energy/kg DM. Three levels of GT inclusion were used to replace CS: a control diet based on 100% CS (GT0), 100 g/kg DM (GT100), and 200 g/kg DM (GT200) of GT were included as replacements for CS. Chemical composition of feedstuffs and treatments is shown in Tables 1, 2 respectively. Before feeding, individual live weights were measured at the beginning and end of the experimental period. Animals were housed in individual metabolic cages (1.20 × 0.80 m), fed individually twice a day (0800 and 1,500 h), with free access to water. Feed intake and refusals were measured daily but only data from the last 7 days were accounted for the statistical analysis. During the last 7 days of the period, samples of feces and urine were collected daily and 10% of the total samples for feces and urine were frozen at −20°C for further analysis.
Chemical analyses
All chemical analyses were performed in triplicate. Feed orts, fecal samples, individual feedstuffs, and diets were dried in a forced air oven (60°C, 48 h), then ground in a mill (Willey, 2 mm Ø Arthur H. Thomas Philadelphia, PA) to determine organic matter (OM; 942.05) (9). Total nitrogen (N; 954.01) was determined by the Kjeldahl method (9) using a conversion factor of 6.25 for crude protein (CP) determination. Neutral detergent fiber (NDF), acid detergent fiber (ADF) and lignin were determined according to Van Soest (11) with the addition of sodium sulfite and alpha-amylase using an ANKOM fiber system. All feedstuffs were analyzed for ether extract (920.39) (12). Composite samples of tomato (n = 6) and corn (n = 6) silages were analyzed for ammonia and volatile fatty acids (VFA) by gas chromatography according to Moon et al. (13). Corn and green tomato silages pH were determined in triplicate. Feces and urine samples were used for nitrogen (N; 991.20) determination (12) to assess nitrogen excretion. Nutrient digestibility coefficients were determined as: digestibility (g/kg) = (nutrient intake – nutrient excreted) / (nutrient intake) × 1,000 (14).
Calculations
The accumulated gas volume of each sample was determined using the model proposed by France et al. (15).
Where: “Y” is the cumulative gas production (mL) “t” is the incubation time (h), A is the asymptote curve (total gas produced, mL), B (h−1), and C (h−½) are the gas production constants, T is the time of delay (h) that colonize the microorganisms to begin the fermentation.
After in vitro incubation periods, samples were filtered and dried (48 h, 60°C) to determine dry matter disappearance (DMd96h), and gas yield production at 24 h (GY24). The volume of gas (mL gas/g DM) produced after 24 h of incubation was calculated by dividing the amount of DMd96h (g):
The gas production (GP) at 96 h was correlated with DM disappearance to produce relative gas yield (RGY; mL gas/g DMd96h) (16).
Intakes (kg/day and g/kg LW0.75) were estimated during the feeding trial.
Statistical analysis
Each in vitro experiment was completed in 4 days, using three replicates per treatment per incubation run (nine replicates per treatment). The analytical replicates were averaged before statistical analysis, so the statistical number of treatment replicates (n = 3) are the true replicates (three incubation runs). The experimental unit was therefore the mean of the three replicates obtained per incubation run forms a statistical replicate. A completely randomized design was used to determine in vitro gas production, and in vitro microbial fermentation (17). Orthogonal contrast was used to test the linear and quadratic responses of each in vitro dependent variable at increasing levels (G0, G100, G200 g/kg DM) of green tomato silage.
A completely randomized design was used to determine the chemical composition and in vivo parameters (17) as follows.
Where Yij = is each observation of treatments it; μ is the general mean; Txi (n = 3) is the treatment effect; and εij is the experimental error.
Data from the in vivo experiment (n = 18) were analyzed using a one-way ANOVA (18). Where treatment (G0, G100, G200 g/kg DM) was the main effect. Digestibility and N balance data also were analyzed using a one-way ANOVA. The Tukey test was used when significant differences were observed between treatments P < 0.05. Orthogonal contrast was used to test the linear and quadratic responses to determine differences among treatments.
Results
In vitro fermentation kinetics
In vitro fermentation parameters ate shown in Table 3. Gas production “A” (mL/g DM), fermentation rates “B” (h−1), and “C” (h−½), were lower for GT200. DMd96h was lower (P < 0.05) for GT100. Gas production at 24 h and RGY were higher (P < 0.05) for GT0 than the rest of the treatments.
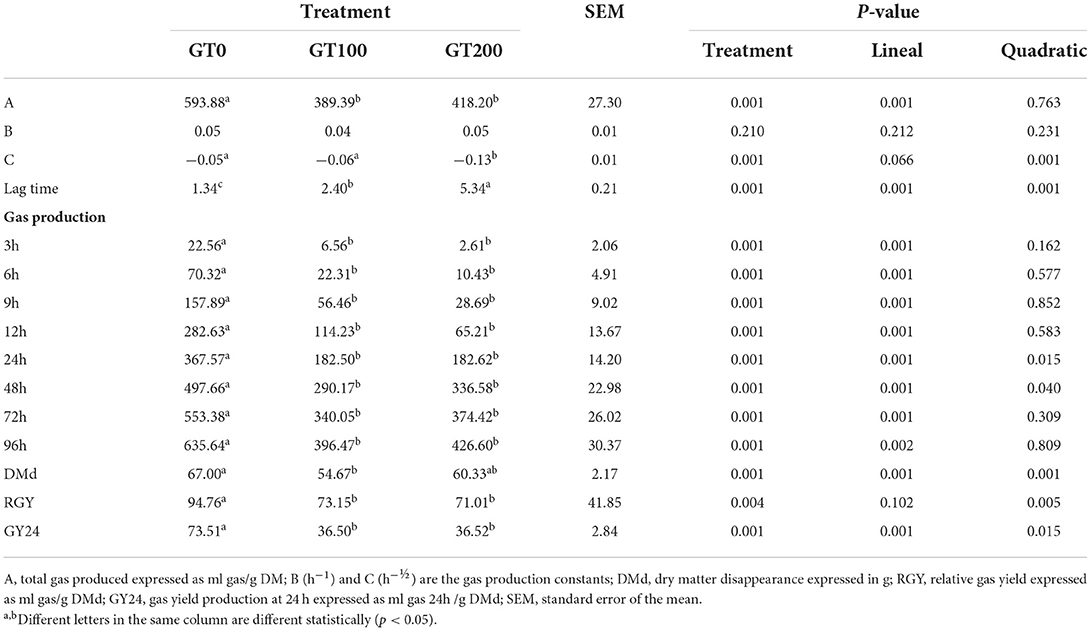
Table 3. In vitro gas production from different inclusion levels of whole green tomato silage (GT) as an alternative to corn silage.
Nutrient intake and digestibility
Nutrient intake and digestibility data are shown in Table 4. Dry matter and OM intake (g/kg LW0.75) were similar (P > 0.05) between treatments. Compared to GT200, ether extract was higher for GT0 and GT100 (P < 0.001). Compared with GT0, NDF intake was higher (P < 0.05) for GT200. Digestibility coefficients for DM, OM, NDF, and ADF were lower (P < 0.05) in GT100 than in the rest of the treatments.
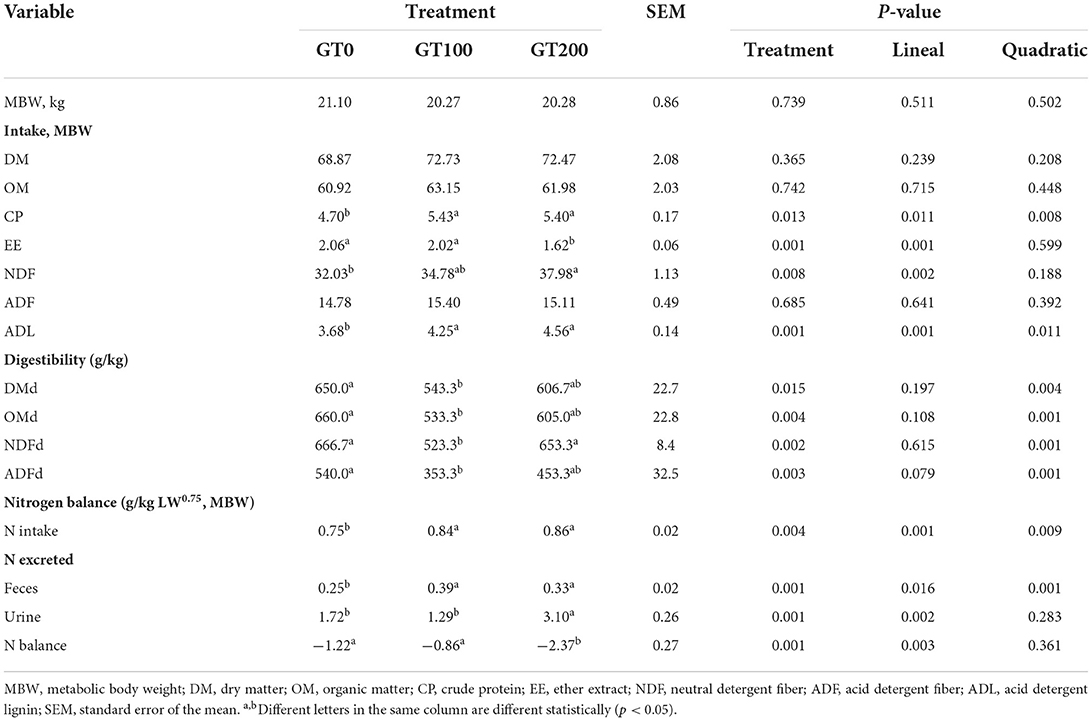
Table 4. Dry matter intake (g/kg LW0.75, MBW) with different inclusion levels of whole green tomato silage (GT) as an alternative to corn silage in lamb diets and nitrogen balance (g/kg LW0.75, MBW).
Nitrogen balance
Nitrogen intake and nitrogen excreted in feces and urine (g/kg LW0.75) (Table 4), were lower (P < 0.001) for GT0. A negative nitrogen balance was also obtained in all treatments, and this had a linear effect (P < 0.001), being GT200 the one that has the most negative nitrogen balance (P < 0.001) compared with the rest of the treatments.
Discussion
Feed quality and diet composition
A proximate analysis of major feed ingredients is shown in Table 1. The DM, CP, NDF, and ADF contents of corn silage, alfalfa hay, soybean meal, canola meal and wheat bran indicated that these feeds are within the expected nutrient levels (10). There are no data related to the chemical composition of whole plant green tomato (as fresh or silage), however Fondevila et al. (19) reported data on tomato pomace (Solanum lycopersicum), which contains 24 % CP which is higher than whole plant green tomato silage (10.1%CP), however, they are similar in NDF content (56%).
Composition of the three diets fed in the study is shown in Table 2. Green tomato silage, which was added at the expense of corn silage, increased from about 6 to 12% of dietary DM (GT100 and GT200 respectively); actual CP concentrations ranged from 103 to 108 g/kg (DM basis). The inclusion of GT had little effect on NDF, ADF and Lignin content compared with the control diet.
In vitro fermentation kinetics
In vitro gas production was lower for GT200. Besharati et al. (20) reported that in a study with red tomato addition, gas yields were lower in the initial incubation times compared to GT0 due to high contents of slowly fermented carbohydrates in tomato, as tomato has a high level of NDF (454 g NDF/ kg DM) and needs more time to adhere to the microorganism (21).
In this study, the decrease in gas production from tomato inclusion (GT100 and GT200) could be explained by flavonoid presence. Until now, not much information is available on the mechanism of action of flavonoids against rumen microbes, but flavonoids generally act against microorganisms by inhibiting cytoplasmic membrane function, inhibiting bacterial cell wall synthesis, or inhibiting nucleic acid synthesis (22, 23). Similarly, secondary compounds are inhibitors of gas production, ruminal microflora, protozoan content of ruminal flow, and proportionate production (24). In general, compared to control (GT0), green tomato treatments decreased fermentation parameters which agrees with studies showing that total gas production is negatively correlated with secondary plant metabolites (25).
The affected rumen fermentation kinetics observed in this study should be further considered in an experimental setup where less amounts of GT are added to diets and rumen microbiome is analyzed. Secondary plant compounds such as flavonoids, have antimicrobial effects and provoke a shift of protozoa and gram-positive bacteria populations in the rumen (26). Thus, using GT could be a nutritional strategy aiming at reducing enteric methane emissions not only in sheep but in other ruminant species. This approach has been recently revised (27–29) and dietary flavonoids have the potential for improving nutrient digestibility and animal performance.
Nutrient intake and digestibility
The observed increase in NDF and lignin intake observed with GT100 and GT200 could be attributed to a higher amount NDF content of tomato plants, compared with CS, which is associated with the phenological stage of the plant, which in this study was in its final growth stage (stem and green tomatoes). A limiting factor that affects the efficiency of energy use is the excess of fiber, which limits the development of the animals. Diets with high amounts of indigestible fiber can lead to low production of volatile fatty acids which eventually affects energy synthesis and availability (30, 31).
Dry matter and OM digestibility were similar among treatments, on the contrary, NDF and ADF digestibility decreased as the GT inclusion increased, contrary to Abdollahzadeh et al. (32) and Gawad et al. (33), who reported that feeding tomato silage as a replacer for alfalfa hay for Holstein dairy cows and buffaloes, led to a significant increase in the digestibility of CP and NDF. The lower digestibility of NDF with respect to the inclusion of GT may be because it has a higher concentration of indigestible NDF and lignin.
The fiber composition of the basal diet used to feed sheep is important as it could lead to different effects on OM digestibility, for example, it has been reported that OM digestibility of dried tomato from 56% (12) in lambs fed on alfalfa hay-based diets while lambs fed on dried tomato in a diet based on barley straw can have an OM digestibility of 90% (3, 34).
Nitrogen balance
In the present study, animals had a negative nitrogen balance, however, sheep fed on GT200 had the highest nitrogen loss compared with GT0 and GT100. This could be due to a higher presence of secondary metabolites including phenolic compounds, phytoalexins, protease inhibitors, and glycoalkaloids in green tomatoes, which could have decreased the efficiency of N utilization, making it difficult to absorb and usage of N in the digestive tract (30, 35, 36), and consequently, the nitrogen content was lost, resulting in the microbial protein in the post-ruminal tract not reflected into sheep growth (37). Also, tomato plants (green or red) synthesize glycoalkaloids dehydrotomatine and alpha-tomatine, as a defense against bacteria, fungi, viruses, and insects (38). Yamashoji and Onoda (39), evaluated the antiobesity effect of immature green tomatoes and reported that they inhibited the accumulation of lipid in adipocytes, a-tomatine from tomatoes interferes with cholesterol absorption and increase sterol excretion by forming a non-absorbable complex with cholesterol in the gastrointestinal tract. Health hazards of a-tomatine have been studied by various animal tests and it is known that the toxicity of α-tomatine depends on the presence of lycotetraose, because removal of one or all four sugar residues renders α-tomatine less toxic (40). In this study, it is possible that tomato secondary compounds disrupted rumen microorganisms' populations with negative effects on nutrient metabolism. Further studies should consider analyzing rumen microbiome to confirm these findings.
The reported apparent digestibility of N from dried tomato pomace was less than that from soybean meal (41). A highly concentrated diet containing dried tomato at 30% of the DM had an apparent CP digestibility of 51% compared with 69% for a diet containing soybean meal (33, 41). Previous reports on the N balance of lambs fed dried tomato pomace are not consistent. In agreement with our data, Ammerman et al. (41) reported a lower N balance when lambs were fed with dried red tomato than when lambs were fed with soybean meal. However, Fondevila et al. (19) reported no difference in N balance between lambs fed dried red tomato and that fed soybean meal. In the present study, urine excretion was highest for GT200 (2,004 mL), followed by GT100 (1,139 mL) and the lowest excretion was for GT0 (569 mL). Increases in urine volume in animals fed green tomatoes increased urinary N excretion, with GT200 having the most negative nitrogen balance (Table 4) with a 94 % increase in total N excretion with respect to GT0. This increase in the excretion of urine and therefore of N, can be seen as a mechanism of excretion of toxic compounds present in tomato (α-tomatine) (42) and consequently, a greater excess of N in urine in the animal by including GT00 and GT200.
This increase in urine excretion was reflected in an increase in water consumption, possibly due to a detoxification response of the animals as it has been observed that the green tomatoes whole plant accumulates a variety of secondary metabolites (38, 43). In this sense, it has been reported that dehydrotomatine and alpha-tomatine contents from tomatoes varied from 42 to 1,498 and 521 to 16,285 mg/g of fresh weight, respectively (42, 43), which represents 120 times more tomatine than red tomatoes (38). Taken together, in this study, it is very possible that this increase in tomatine affected animal welfare, and consequently, one way to eliminate the compound was by increasing the excretion of urine and therefore of N.
Conclusions
In sheep diets, the inclusion of whole plant green tomato silage at 100 or 200 g/kg DM as an alternative to corn silage, does not affect dry matter intake but negatively affects nutrient digestibility and N balance. Therefore, under the conditions of this study, its inclusion in sheep diets is not recommended.
Data availability statement
The raw data supporting the conclusions of this article will be made available by the authors, without undue reservation.
Ethics statement
The animal study was reviewed and approved by all experimental procedures were approved by the Animal Experimental Guidelines of the Universidad Autónoma del Estado de México (Project Code: UAEMex 4974/2020).
Author contributions
LER-J, MG-R, and EV-B-P are the principal investigator of this project. ACN-L, MG-R, and EV-B-P conducted the experiment. ACN-L, AS-R, and AJC-C conducted the data analysis. LER-J, OAC-O, NZ, MG-R, and EV-B-P drafted the manuscript. All authors contributed to the article and approved the submitted version.
Funding
This project was partially supported by Universidad Autónoma del Estado Mexico (Grant Number: UAEMex 4974/2020).
Acknowledgments
LER-J and AS-R were awarded a Conacyt scholarship during their Ph.D. studies in the Ph.D. program (PCARN) at the Universidad Autónoma del Estado de México. During the study, EV-B-P was a visiting scholar also supported by project number 4974/2020CIB from Universidad Autónoma del Estado de México.
Conflict of interest
The authors declare that the research was conducted in the absence of any commercial or financial relationships that could be construed as a potential conflict of interest.
Publisher's note
All claims expressed in this article are solely those of the authors and do not necessarily represent those of their affiliated organizations, or those of the publisher, the editors and the reviewers. Any product that may be evaluated in this article, or claim that may be made by its manufacturer, is not guaranteed or endorsed by the publisher.
References
1. Abbeddou S, Rischkowsky B, Hilali ME, Hess HD, Kreuzer M. Influence of feeding Mediterranean food industry byproducts and forages to Awassi sheep on physicochemical properties of milk, yoghurt and cheese. J Dairy Res. (2011) 78:426–35. doi: 10.1017/S0022029911000665
2. Nudda A, Buffa G, Atzori AS, Cappai MG, Caboni P, Fais G, Pulina G. Small amounts of agro-industrial byproducts in dairy ewes diets affects milk production traits and hematological parameters. Anim Feed Sci Technol. (2019) 251:76 85 doi: 10.1016/j.anifeedsci.2019.02.007
3. Ziaei N, Molaei S. Evaluation of nutrient digestibility of wet tomato pomace ensiled withwheat straw compared to alfalfa hay in Kermani sheep. J Anim Vet Adv. (2010) 9:771–3. doi: 10.3923/javaa.2010.771.773
4. SIAP (2018). Servicio de Información Agroalimentaria y Pesquera 29 de mayo de 2018. Available online at: https://www.gob.mx/siap/es/articulos/tomate-verde-ingrediente-esencial-de-la-comida-mexicana?idiom=es (accessed on 13 August 2020).
5. AOAC. Association of Official Analytical Chemists. Official Methods of Analysis, 15th ed. Washington, DC: Association of Official Analytic Chemist. (1990).
6. Reyes-Muro L, Camacho-Villa TC, Guevara-Hernández F. Rastrojos: manejo, uso y mercado en el centro y sur de México. Instituto Nacional de Investigaciones Forestales, Agrícolas y Pecuarias Libro Técnico Núm 7 Pabellón de Arteaga, Aguascalientes, México i–viii, p 1–242. (2013).
7. CONAGUA (2015). Comision nacional del agua. Informe (2015) Sistema Nacional de Informacion del Agua (SINA). Available online at: http://sina.conagua.gob.mx/sina/index.php?publicaciones=1 (accessed August 3, 2020).
8. Martínez JRPF, Huerta AG, López DdJP, Cuevas RS, Salem AZM, Robles-Jimenez LE, et al. Effect of xylanase, cellulase and natural maguey extract on the chemical composition of corn silage and in vitro rumen gas production. Int J Agric Nat Resour. (2020) 47:23–34. doi: 10.7764/ijanr.v47i1.2128
9. Menke KH, Steingass H. Estimation of the energetic feed value obtained from chemical analysis and in vitro gas production using rumen fluid. Anim Res Develop. (1988) 28:375–86.
10. NRC. National Research Council. Nutrient Requirementsof Small Ruminants: Sheep, Goats, Cervids, and New Worldcamelids. National Academies Press: Washington, DC (2007) p 392.
11. Van Soest PJ, Robertson JB, Lewis BA. Methods of diet-ary, neutral detergent fiber and non-starch polysacchar-ides in relation to animal nutrition. J Dairy Sci. (1991) 74:3583–97. doi: 10.3168/jds.S0022-0302(91)78551-2
12. AOAC. Association of Official Analytical Chemists. Official Methods of Analysis, 16th ed. Arlington, VA: Association of Official Analytical Chemists. (1997).
13. Moon NJ, Ely LO, Sudweeks EM. Fermentation of wheat, corn and alfalfa silages inoculated with Lactobacillus acidophilus and Candida sp. at ensiling J Dairy Sci. (1981) 64:807–13. doi: 10.3168/jds.S0022-0302(81)82651-3
14. Wiseman J. Editorial: Digestibility and degradability in animal nutrition studies. J Agric Sci. (2018) 156:1161–2. doi: 10.1017/S0021859619000121
15. France J, Dhanoa MS, Theodorou MK, Lister SJ, Davies DR, Isac DA. Model to interpret gas accumulation pro-files associated with in vitro degradation of ruminantfeeds. J Theo Biol. (1993) 163:99–111. doi: 10.1006/jtbi.1993.1109
16. Gonzalez Ronquillo M, Fondevila M, Barrios Urdaneta A. Newman Y. In vitro gas production from buffelgrass (Cenchrus ciliaris L.) fermentation in relation to thecutting interval, the level of nitrogen fertilisation and theseason of growth. Anim Feed Sci Tech. (1998) 72:19–32. doi: 10.1016/S0377-8401(97)00181-8
17. Steel RGD, Torrie JH, Dickey DA. Principles and procedures of statistics. In: A biometrical approach, (3rd Ed.). McGraw Hill Book Co: New York, NY. (1997).
18. SAS. Statistical Analysis System Institute. Statistical Analysis System Institute Inc. SAS/STAT User's Guide: Cary, NC. (2010).
19. Fondevila M, Guada JA, Gasa J, Castrillo C. Tomato pomace as a protein supplement for growing lambs. Small Rumin Res. (1994) 13:117. doi: 10.1016/0921-4488(94)90086-8
20. Besharati M, Taghizadeh A, Janmohammadi H, Moghadam GA. Evaluation of some by-products using in situ and in vitro gas production techniques. Am J Anim Vet Sci. (2008) 3:7–12. doi: 10.3844/ajavsp.2008.7.12
21. Ball DM, Collins M, Lacefield GD, Martin NP, Mertens DA, Olson KE, et al. Understanding Forage Quality. American Farm Bureau Federation Publication 1-01: Park Ridge, IL. (2001).
22. Cushnie TPT, Lamb AJ. Recent advances in understanding the antibacterial properties of flavonoids. Int J Antimicrob Agents. (2011) 38:99–107. doi: 10.1016/j.ijantimicag.2011.02.014
23. Oskoueian E, Abdullah N, Oskoueian A. Effects of flavonoids on rumen fermentation activity, methane production, and microbial population. BioMed Res Int. (2013) 2013:1–8. doi: 10.1155/2013/349129
24. Xiao J, Sarker SD, Asakawa Y (eds.) Handbook of Dietary Phytochemicals. Singapore: Springer. (2021). doi: 10.1007/978-981-15-4148-3
25. Kara K, Güçlü BK, Baytok E. Comparison of nutrient composition and anti-methanogenic properties of different Rosaceae species. J Anim Feed Sci. (2015) 24:308–14. doi: 10.22358/jafs/65613/2015
26. Szumacher-Strabel M, Zmora P, Roj E, Stochmal A, Pers-Kamczyc E, Urbańczyk A, et al. The potential of the wild dog rose (Rosa canina) to mitigate in vitro rumen methane production. J Anim Feed Sci. (2011) 20: 285– 299 doi: 10.22358/jafs/66185/2011
27. Yaghoubi M, Gh G, Satari R. Antimicrobial activity of Iranian propolis and its chemical composition. DARU J Pharml Sci. (2007) 15:45–8.
28. Hassan F-u, Arshad MA, Li M, Rehman MS-u, Loor JJ, Huang J. Potential of mulberry leaf biomass and its flavonoids to improve production and health in ruminants: mechanistic insights and prospects. Animals. 2020 10:2076. doi: 10.3390/ani10112076
29. Ku-Vera JC, Jiménez-Ocampo R, Valencia-Salazar SS, Montoya-Flores MD, Molina-Botero IC, Arango J, et al. Role of secondary plant metabolites on enteric methane mitigation in ruminants. Front Vet Sci. (2020) 7:584. 42 doi: 10.3389/fvets.2020.00584
30. Waghorn G. Beneficial and detrimental effects of dietary condensed tannins for sustainable sheep and goat production-Progress and challenges. Anim Feed Sci Technol. (2008) 147:116–39. doi: 10.1016/j.anifeedsci.2007.09.013
31. DAIRY GLOBAL (2020). 4 underutilised feed sources for ruminants. Available online at: https://www.dairyglobal.net/health-and-nutrition/nutrition/4-underutilised-feed-sources-for-ruminants/ (accessed on 1, August 2022).
32. Abdollahzadeh F, Pirmohammadi R, Farhoomand P, Fatehi F, Pazhoh FF. The effect of ensiled mixed tomato and apple pomace on Holstein dairy cow. Ital J Anim Sci. (2010) 9:2. doi: 10.4081/ijas.2010.e41
33. Gawad ARMA, Hanafy MA, Mahmoud AEM, Al-Slibi YH. Effect of tomato pomace, citrus and beet pulp on productive performance and milk quality of Egyptian buffaloes. Pak J Biol Sci. (2020) 23:1210–9. doi: 10.3923/pjbs.2020.1210.1219
34. Abbeddou S, Riwahi S, Iñiguez L, Zaklouta M, Hess HD, Kreuzer M. Ruminal degradability, digestibility, energy content, and influence on nitrogen turnover of various Mediterranean by-products in fat-tailed Awassi sheep. Anim Feed Sci Technol. (2011) 163:99–110. doi: 10.1016/j.anifeedsci.2010.10.011
35. González-Chavira MM, Guzmán-Maldonado SH, Pons-Hernandez JL, Villalobos-Reyes S, González-Pérez E. Caracterización genética, química y agronómica de líneas avanzadas de tomate de cáscara. Agron Mesoam. (2019) 30:101–14. doi: 10.15517/am.v30i1.34402
36. Jerónimo E, Pinheiro C, Lamy E, Dentinho MT, Sales-Baptista E, Lopes O, et al. Tannins in ruminant nutrition: impact on animal performance and quality of edible products. In Combs CA (ed.) Tannins: Biochemistry, Food Sources and Nutritional Properties. New York, NY: Nova Science Publishers Inc. (2016) p 121–168.
37. Soltan Y, Abdalla FA, Abdalla A, Berenchtein B, Schiavinatto P, Costa C. (2021). Replacing maize with low tannin sorghum grains: lamb growth performance, microbial protein synthesis and enteric methane production. Anim Prod Sci. (2021) 61:1348–55. doi: 10.1071/AN20605
38. Friedman M. Tomato Glycoalkaloids: Role in the Plant and in the Diet. J Agr Food Chem. (2002) 50:5751–80. doi: 10.1021/jf020560c
39. Yamashoji S, Onoda E. Detoxification and function of immature tomato. Food Chem. (2016) 209:171–6. doi: 10.1016/j.foodchem.2016.04.042
40. Ökmen B, Etalo DW, Joosten MHAJ, Bouwmeester HJ, de Vos RCH, Collemare J, et al. Detoxification of α-tomatine by Cladosporium fulvumis required for full virulence on tomato. New Phytol. (2013) 198:1203–14. doi: 10.1111/nph.12208
41. Ammerman CB, Arrington LR, Loggins PE, McCall JT, Davis GK. Nutritive value of dried tomato pulp for ruminants. J Agric Food Chem. (1963) 11:347. doi: 10.1021/jf60128a025
42. Xu Y-M, Wijeratne EMK, Brooks AD, Tewary P, Xuan L-J, Wang W-Q, et al. Cytotoxic and other withanolides from aeroponically grown Physalis philadelphica. Phytochem. (2018) 152:174–81. doi: 10.1016/j.phytochem.2018.04.018
Keywords: green tomatoes, corn silage, sheep, fermentation kinetics, crop residues
Citation: Robles-Jimenez LE, Narváez-López AC, Chay-Canul AJ, Sainz-Ramirez A, Castelan-Ortega OA, Zhang N, Gonzalez-Ronquillo M and Vargas-Bello-Pérez E (2022) Effect of different dietary inclusion levels of whole plant green tomato (Physalis philadelphica) silage on nutrient intake and digestibility, and in vitro rumen fermentation kinetics in sheep. Front. Vet. Sci. 9:980619. doi: 10.3389/fvets.2022.980619
Received: 28 June 2022; Accepted: 16 September 2022;
Published: 14 October 2022.
Edited by:
Tugay Ayasan, Osmaniye Korkut Ata University, TurkeyReviewed by:
Mariangela Caroprese, University of Foggia, ItalyYosra Ahmed Soltan, Alexandria University, Egypt
Copyright © 2022 Robles-Jimenez, Narváez-López, Chay-Canul, Sainz-Ramirez, Castelan-Ortega, Zhang, Gonzalez-Ronquillo and Vargas-Bello-Pérez. This is an open-access article distributed under the terms of the Creative Commons Attribution License (CC BY). The use, distribution or reproduction in other forums is permitted, provided the original author(s) and the copyright owner(s) are credited and that the original publication in this journal is cited, in accordance with accepted academic practice. No use, distribution or reproduction is permitted which does not comply with these terms.
*Correspondence: Manuel Gonzalez-Ronquillo, mrg@uaemex.mx; Einar Vargas-Bello-Pérez, e.vargasbelloperez@reading.ac.uk
†ORCID: Lizbeth E. Robles-Jimenez orcid.org/0000-0003-3385-7177
Alondra C. Narváez-López orcid.org/0000-0002-4168-8724
Alfonso J. Chay-Canul orcid.org/0000-0003-4412-4972
Aurora Sainz-Ramirez orcid.org/0000-0002-1916-6559
Octavio A. Castelan-Ortega orcid.org/0000-0003-4693-9673
Naifeng Zhang orcid.org/0000-0001-6272-7553
Manuel Gonzalez-Ronquillo orcid.org/0000-0003-3616-4157
Einar Vargas-Bello-Pérez orcid.org/0000-0001-7105-5752