- Guangdong Key Laboratory for Veterinary Drug Development and Safety Evaluation, College of Veterinary Medicine, South China Agricultural University, Guangzhou, China
Riemerella anatipestifer (RA) is an important pathogen found in poultry. RA infection can kill ducks and lead to significant economic losses. Seven RA strains with different susceptibility phenotypes were chosen to study the pharmacokinetic/pharmacodynamic (PK/PD) integration of florfenicol (FF) alone and in combination with doxycycline (DOX). The checkerboard assay indicated that synergy [fractional inhibitory concentration index (FICI) ≤ 0.5] was detected in the CVCC3952 strain of RA and that additivity (FICI >0.5 to ≤ 1) was observed in other strains. Static time–kill curves showed that the bactericidal effect of FF against RA was produced at a FF concentration ≥4 MIC, and the antibacterial activity of FF against RA was enhanced from the aspects of efficacy and efficacy in combination with DOX. Dynamic time–kill curves indicated that FF elicited bactericidal activity against the CVCC3857 strain with a reduction ≥4.88 log10CFU/ml when the dose was ≥8 mg/L. However, a bactericidal effect was not achieved at the maximum administered dose of FF monotherapy (20 mg/L) for isolates with a MIC ≥4 μg/ml. The effect of FF against RA was enhanced upon combination with DOX. The combination of FF with DOX reduced the bacterial burden ≥4.53 log10CFU/ml for all strains with a MIC ≥4 μg/ml. Data were fitted to a sigmoidal Emax model. The PK/PD parameters of AUC24h/MIC (the area under the concentration–time curve over 24 h divided by the MIC) and %T >MIC (the cumulative percentage of time over a 24-h period at which the concentration exceeded the MIC) of FF for eliciting a reduction of 3 log10CFU/ml was 40.10 h and 58.71, respectively. For strains with a MIC ≤ 16 μg/ml, the magnitude of the AUC24h/MIC and Cmax/MIC required for a 3 log10CFU/ml of bacterial killing was 34.84 h and 4.74 in the presence of DOX at 0.5 MIC, respectively. These data suggest that combination of FF with DOX enhanced the activity against RA strains with various susceptibilities to FF and DOX.
Introduction
Riemerella anatipestifer (RA) infects ducks, geese, turkeys, and other types of poultry. RA infection occurs in goslings and ducklings aged 2–8 weeks, and is characterized by septicemia and infectious serositis (1). The gross lesions of necropsy include fibrinous pericarditis, perihepatitis, airsacculitis, catarrhal enteritis, and neurological symptoms (2). RA infection can kill ducks and lead to significant economic losses. More than 21 serotypes of RA have been identified (3). Use of a vaccine alone has limited effects on the high genetic diversity and serotype variation of bacterial strains, so use of pharmacological agents is an important method to control RA. Animal experiments have shown that ceftiofur, cefuroxime, and enrofloxacin are efficacious agents against RA infection (4–6).
Overuse of antimicrobial agents has led to the development of bacterial resistance. RA is resistant to cephalosporins, aminoglycosides, tetracyclines, and fluoroquinolones (1, 7, 8). Gyuris and colleagues showed that 97.9% of RA strains isolated in Hungary were susceptible to florfenicol (FF). However, there are remarkable differences in resistance depending on the use of antibiotics on different farms. The resistance of RA to FF increased from 11 to 43% from 2006 to 2009 in Taiwan (9). Also, 55.26% of RA isolates collected between 2016 and 2020 were FF-positive to the FF-resistant gene floR, and showed a phenotype of resistance or low susceptibility to FF (10). The increasing resistance of RA to common antibiotics can compromise treatment. Studies have indicated that combined use of doxycycline (DOX) and FF has synergistic or additive interactions against Actinobacillus pleuropneumoniae and Pasteurella multocida (11). The combination of DOX and FF has a partial synergistic action against resistant Escherichia coli isolates (12).
FF is a structural analog of thiamphenicol, and is approved for veterinary use only. FF binds irreversibly to the 50S ribosomal subunit of a bacterium, which leads to inhibition of peptidyl transferase activity and prevents subsequent protein formation. FF has stronger antibacterial activity than chloramphenicol. Substitution of the hydroxyl group at C-3 with fluorine enables FF to overcome chloramphenicol-resistant bacteria expressing chloramphenicol acetyl transferases. FF has been approved by the US Food and Drug Administration for treatment of respiratory diseases in cattle and pigs. FF is also used to treat infections caused by E. coli, Salmonella species, and Pasteurella species in chickens in China (12). FF possesses a long elimination half-life (t1/2β) in calves (27.54 h) and pigs (14.46 h), and has a relatively short t1/2β in chickens (6.01 h), geese (2.91 h), and ducks (445 min) (13–17).
DOX is a second-generation tetracycline with broad-spectrum antibacterial activity. It binds to the decoding center of the 30S small ribosomal subunit of microorganisms and inhibits protein synthesis. DOX has been approved by the European Medicines Agency for the prevention and treatment of respiratory and gastrointestinal infections in poultry. Studies on the pharmacokinetics (PK) of DOX in pigs, calves, goats, sheep, chickens, geese, and ducks have been plentiful. DOX possesses good tissue penetration, good oral bioavailability, and a long half-life in the serum of animals. The t1/2β of DOX has been reported to be 21.21 h in 6-week-old healthy Muscovy ducks following intravenous administration (18).
Pharmacokinetic/pharmacodynamic (PK/PD) modeling is an important tool for optimizing the dosage schedule to achieve clinical cure and minimizing the emergence of drug resistance (19). Furthermore, the PK/PD relationship is considered important for development of new antimicrobial compounds by the US Food and Drug Administration and European Medicines Agency (20). Limited information is available on the PK/PD interactions of antibacterial agents against RA.
We undertook four main tasks in the present study. First, the minimum inhibitory concentration (MIC) of FF and DOX against RA was determined. Second, the fractional inhibitory concentration index (FICI) was calculated to measure the effects of a combination of antimicrobial drugs using the checkerboard assay. Third, static time–kill curves in a defined artificial medium were established, and the relationship between the kill rate and drug concentration was fitted to the maximum value of the kill rate (Emax) model. Fourth, the relationship between PK/PD indices and the effect of FF alone and in combination with DOX against RA was investigated in an in vitro dynamic PK/PD model. Our study is important for dosing guidance for treatment of RA infections.
Materials and methods
Materials
The RA strains of CVCC3952 and CVCC3857 were obtained from the Chinese Veterinary Microorganism Culture Collection Center (Beijing, China). Clinical isolates of RA were supplied by the Laboratory of Veterinary Pharmacology within South China Agricultural University (Guangzhou, China). The isolates were obtained originally from the brains of sick ducks and dead ducks in Guangdong Province (China), and identified by polymerase chain reaction targeting the 16S rRNA gene with Sanger sequencing and matrix-assisted laser desorption ionization time-of-flight mass spectrometry. FF (purity ≥98%) and DOX (purity ≥98%) were purchased from Aladdin Industrial Corporation (Ontario, CA, USA). Tryptic soy broth and tryptic soy agar were purchased from Guangdong Huankai Microbial Technology (Guangzhou City, China). Newborn bovine serum was provided by Guangzhou Ruite Biotechnology (Guangzhou, China).
MIC determination
The MIC of FF and DOX for RA strains was determined using a broth-microdilution method with Mueller–Hinton broth supplemented with 5% calf serum (21). A series of concentrations of FF and DOX was prepared by twofold dilution with media. To each of the first-to-ninth column of a 96-well plate (Costar 3599; Corning, Corning, NY, USA) was added 100 μl of drug of different concentrations. Then, 100 μl of an exponential-phase culture of RA diluted with medium was added to ensure the final inoculum size was 105 CFU/ml. A growth control (RA inoculum in the absence of antimicrobial agents) and a sterility control were included. The E. coli ATCC 25922 strain was used as the quality control. The plate was capped and allowed to incubate for 18 h at 37°C. Then, the absorbance was measured using a spectrophotometer (NanoDrop 2000; Thermo Fisher Scientific, San Jose, CA, USA). The MIC measurement for each drug was repeated thrice.
Checkerboard assay
The interactions between FF and DOX were evaluated by the checkerboard assay. The concentration range of each antibiotic was from four-fold to one/16-fold of the MIC. The final concentration of bacteria in each well was 5 × 105 CFU/ml. Turbidity was checked after 18 h of incubation at 37°C. The FICI was calculated using the following formula:
The FICI was interpreted as follows: FICI ≤ 0.5 denotes “synergy”; FICI >0.5 to ≤ 1 denotes “additivity”; FICI >1 to ≤ 4 denotes “no interaction”; FICI >4 denotes “antagonism.” For each strain, the checkerboard assay was repeated thrice (22).
Exposure to a static concentration of antibiotic
Four milliliters of blank medium, 0.5 ml of 10-times the final drug concentration, and 0.5 ml of logarithmic-phase RA were added to a bottle in turn and then mixed. Tubes containing 5-ml cultures were exposed to FF alone (0, 0.25, 0.5, 1, 2, 4, 6, 8, 16, and 32-times the MIC) or FF + DOX (0, 0.25, 0.5, 1, 2, 4, 6, 8, 16, and 32 times the MIC concentration of FF in combination with DOX at 0.5 MIC, respectively). Cultures were incubated at 37°C with shaking. Then, aliquots were taken from each bottle at 0, 3, 6, 9, 12, and 24 h for colony counts. Each sample was serially diluted 10-fold. Then, 20-μl dilutions were transferred to agar plates and incubation allowed for 24 h. The limit of detection was 50 CFU/ml. Bactericidal activity was defined as >3-log10 reduction in CFU/ml. Bacteriostatic activity was defined as >2-log10 reduction in CFU/ml (23).
The kill rate can reflect the efficiency of an antibacterial drug against a pathogen. In the present study, because the maximum antibacterial effect had been reached in 9 h at high concentration, the kill rate from 0 to 9 h was selected to fit different drug concentrations. The Emax model can be presented as:
where E is the kill rate; Emax is the maximum value of the kill rate; Ce is the drug concentration; EC50 is the concentration at which 50% of the maximum kill rate is reached; N is the Hill coefficient (which describes the steepness of the kill rate–concentration curve).
In vitro dynamic PK/PD model
An in vitro one-compartment PK/PD model of infection was employed to examine the antimicrobial efficacy of FF alone and in combination with DOX against RA over 24 h, as described by Xiao et al. (24). The model contained a reserve chamber for fresh tryptic soy broth, a central chamber for drug–bacteria interactions, and a waste storage chamber. The central chamber comprised 200 ml of sterile medium. An inverted 15-ml centrifuge tube with a cellulose-ester membrane (pore size = 0.2 μm) covering the top was placed to prevent the loss of bacteria. The flow rate of the peristaltic pump was set at 0.462 ml/min to simulate the PK of FF (t1/2β = 5 h) in duck plasma. According to the maximum concentration of FF in duck plasma (Cmax), six dose groups (2, 4, 8, 12, 16, and 20 mg/L) were designed for FF in the dynamic modeling for each strain. With respect to combination therapy, DOX was administered as a continuous infusion to simulate its long t1/2β in this model. A series of studies was conducted using different doses of FF in combination with DOX at 0.5 MIC to evaluate the PK/PD target of FF in the presence of DOX against RA. Study arms for bacteria alone and control with FF alone or DOX alone were included for each strain.
Before treatment initiation, 10 ml of a log-phase bacterial suspension and 190 ml of culture medium were introduced into the central chamber of the model and allowed to grow for 2 h. Doses of FF were administered into the central compartment, and the time was regarded as the initial time of the experiment. With regard to combination, a dose of DOX was added to the reserve chamber and central chamber to hold a drug concentration of 0.5 MIC in the whole process. We sampled 0.1 ml before administration (0 h) as well as 0.5, 1, 2, 4, 6, 8, 12, and 24 h after administration for bacterial counting, and 1 ml for determination of the drug concentration. Samples were stored at −20°C until analyses.
Quantification of FF in the medium
The FF concentration in the medium was measured by high-performance liquid chromatography-tandem mass spectrometry using a system from Agilent Technologies (Santa Clara, CA, USA). Separation was achieved on a C18 column (150 mm × 2.0 mm, 5 μm; Phenomenex, Torrance, CA, USA). A mixture of ethyl acetate and ammonia (98:2, v/v) was used as the extractant. The mobile phase consisted of solution A (water) and solution B (acetonitrile) at a flow rate of 0.25 ml/min. The elution gradient was: 0 min, 10% B; 0–2 min, 65% B; 2–6 min, 65% B; 6–8 min, 10% B; and 13 min, 10% B. The injection volume was 2 μl. A calibration curve was established with six FF concentrations (0.001–0.2 μg/ml). PK parameters were calculated using WinNonlin 6.1 (Pharsight, Mountain View, CA, USA).
Fitting and analyses of dynamic time–kill curves
The results of dynamic modeling studies were analyzed using the inhibitory sigmoidal maximum effect (Emax) PD model. The PK/PD parameters of the area under the concentration–time curve over 24 h (AUC24h), Cmax, and the cumulative percentage of time over a 24-h period in which the concentration exceeded the MIC (%T >MIC) were calculated according to a one-compartmental analysis using WinNonlin. The inhibitory sigmoidal Emax model was:
where E is the antibacterial effect; Emax is the change in the control group (log10 CFU/ml) from 0 h to 24 h; E0 is the maximum value of the antibacterial effect; Ce is the value of a PK/PD index (Cmax/MIC, AUC24h/MIC, and %T >MIC); EC50 is the corresponding PK/PD index that produces a 50% reduction of the maximum antibacterial effect; N is the Hill coefficient.
Results
Susceptibility testing
The results of MIC determination and the checkerboard assay are summarized in Table 1. To evaluate the activity of FF and DOX against RA (including phenotypic resistant strains), the MIC of seven RA strains selected ranged from 0.5 to 16 μg/ml. The MIC of DOX on RA ranged from 0.125 to 4 μg/ml. According to the FICI, a synergistic effect was observed when FF was combined with DOX against the CVCC3952 strain of RA, and an additive effect was noted for other strains. The MIC of FF against RA in the presence of DOX at 0.5 MIC in the checkerboard assay is shown in Table 1.
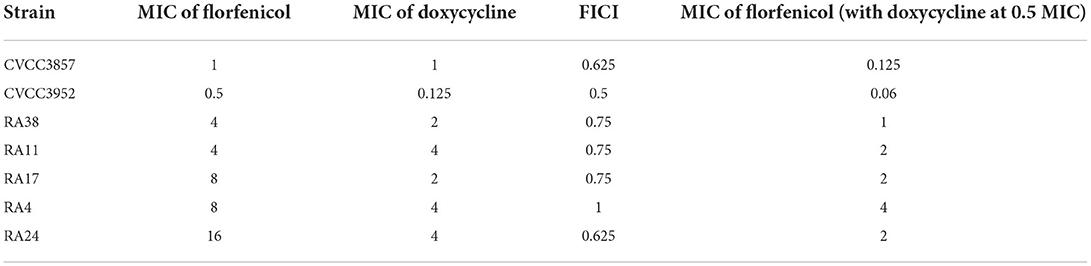
Table 1. Minimum inhibitory concentration (MIC) and fractional inhibitory concentration index (FICI) in in vitro susceptibility testing.
Static time–kill curves
Florfenicol elicited a reduction of 4.25 log10CFU/ml for the CVCC3952 strain and 5.86 log10CFU/ml for the CVCC3857 strain of RA at 32 MIC concentration within 24 h (Figure 1). When the FF concentration was <1 MIC, RA showed a slow increase relative to the initial inoculum size. The bactericidal effect of FF against RA was achieved within 24 h when the drug concentration was ≥4 MIC. To determine the impact of DOX on the antibacterial activity of FF, we created static time–kill curves to FF in the presence of DOX. DOX shows activity against RA, so a fixed subinhibitory concentration of 0.5 MIC was selected for DOX. In the presence of DOX at 0.5 MIC, a bacteriostatic effect was observed at FF concentrations <1 MIC, and a bactericidal effect was observed at FF concentrations ≥1 MIC.
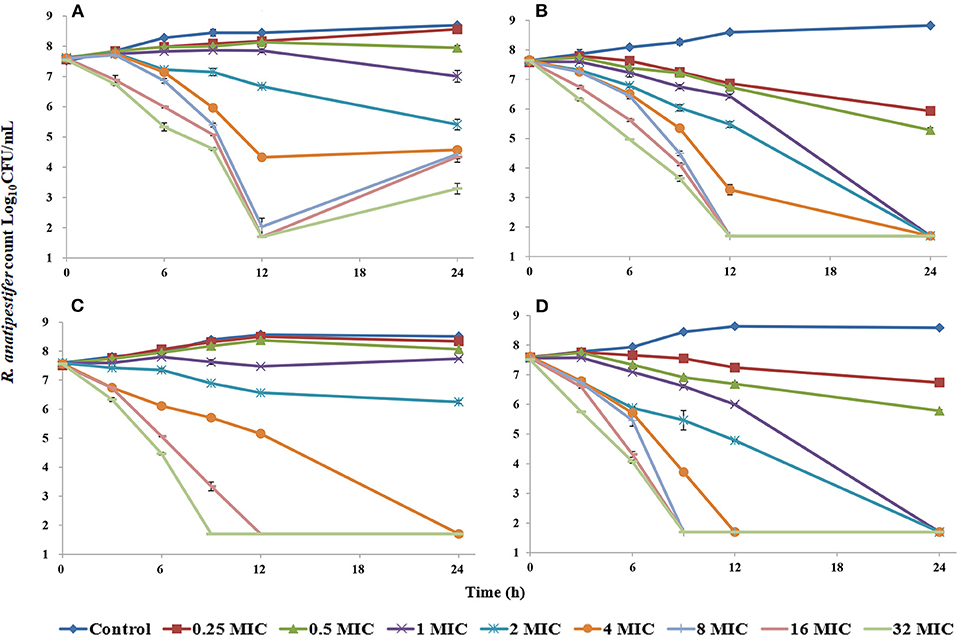
Figure 1. Time–kill studies of florfenicol alone and in combination with doxycycline against Riemerella anatipestifer at constant concentrations [FF alone: (A), CVCC3952; (B), CVCC3857; FF in combination with DOX: (C), CVCC3952; (D), CVCC3857].
The relationship between the kill rate and concentration is presented in Figure 2 (time interval was 0–9 h): the kill rate increased with increasing concentration. The relationship between the concentration and kill rate was fitted by the Emax model, and the obtained parameters of Emax, EC50, and the Hill coefficient are presented in Table 2.
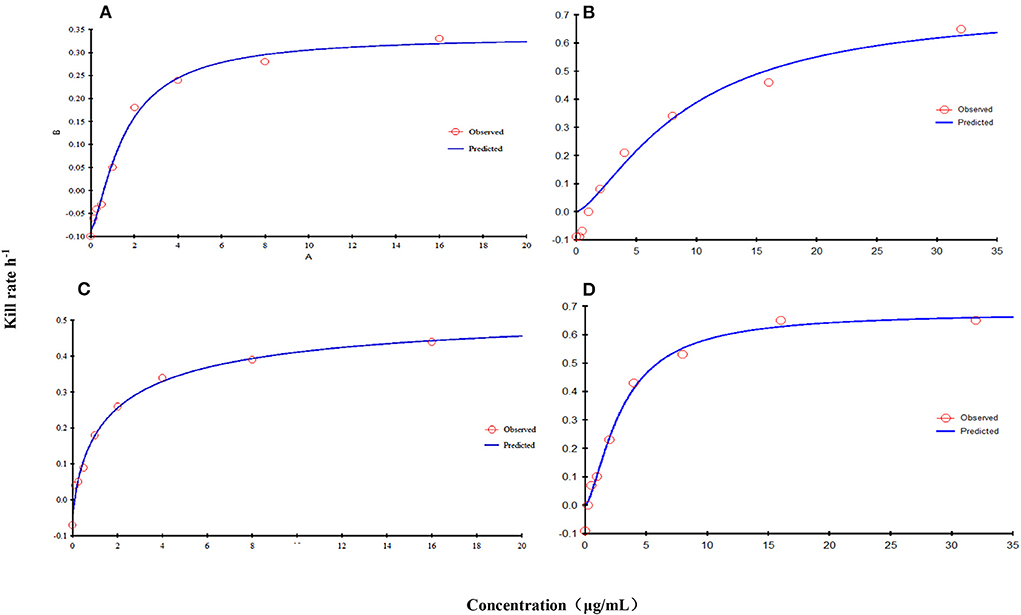
Figure 2. Relationship between the kill rate and concentration obtained from the Emax model at 0–9 h [FF alone: (A), CVCC3952; (B), CVCC3857; FF in combination with DOX: (C), CVCC3952; (D), CVCC3857).
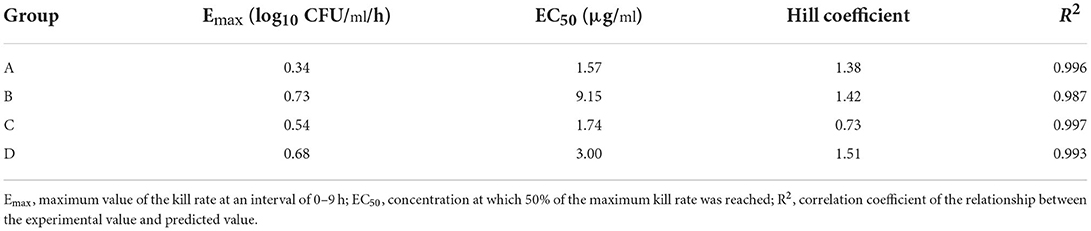
Table 2. Estimation of the kill-rate parameter derived from the Emax model which fitted the data from the in vitro static time–kill curve at an interval of 0–9 h.
PK in the in vitro dynamic model
To simulate the antibiotic exposure obtained by various treatment doses, six dose groups were designed based on clinical studies. The concentration–time curves of the in vitro dynamic model for FF are shown in Figure 3. Data were analyzed according to a one-compartment model by WinNonlin. The half-life was calculated to be 4.995 ± 0.25 h. AUC24h and Cmax after each drug administration was 17.54–145.56 μg h/ml and 2.52–19.13 μg/ml, respectively.
PK/PD modeling of FF alone against RA
Figure 4 shows the antibacterial efficacy of FF alone against RA at six simulated dosing regimens: escalating doses of FF resulted in marked killing of the CVCC3857 strain of RA. The bacterial count decreased by 0.63 log10CFU/ml and 1.60 log10CFU/ml, respectively, at the FF doses of 2 and 4 mg/L within 24 h. If the dose was ≥8 mg/L, then FF showed bactericidal activity against the CVCC3857 strain of RA, and a reduction of 4.88 log10CFU/ml was reached at 8 mg/L within 24 h. However, for the RA38 strain with a MIC of 4 μg/ml for FF, a bactericidal effect was not observed at any dose within 24 h. The maximum reduction was 2.99 log10CFU/ml.
The Emax relationship of the three PK/PD parameters vs. the antibacterial effect of FF used alone against the CVCC3857 and RA38 strains of RA are shown in Figure 5. The R2 of AUC24h/MIC, Cmax/MIC, and %T >MIC with an antibacterial effect was 0.991, 0.989, and 0.994, respectively. The AUC24h/MIC, Cmax/MIC, and %T >MIC for eliciting a reduction in 3 log10CFU/ml was 40.10 h, 5.43, and 58.71, respectively.
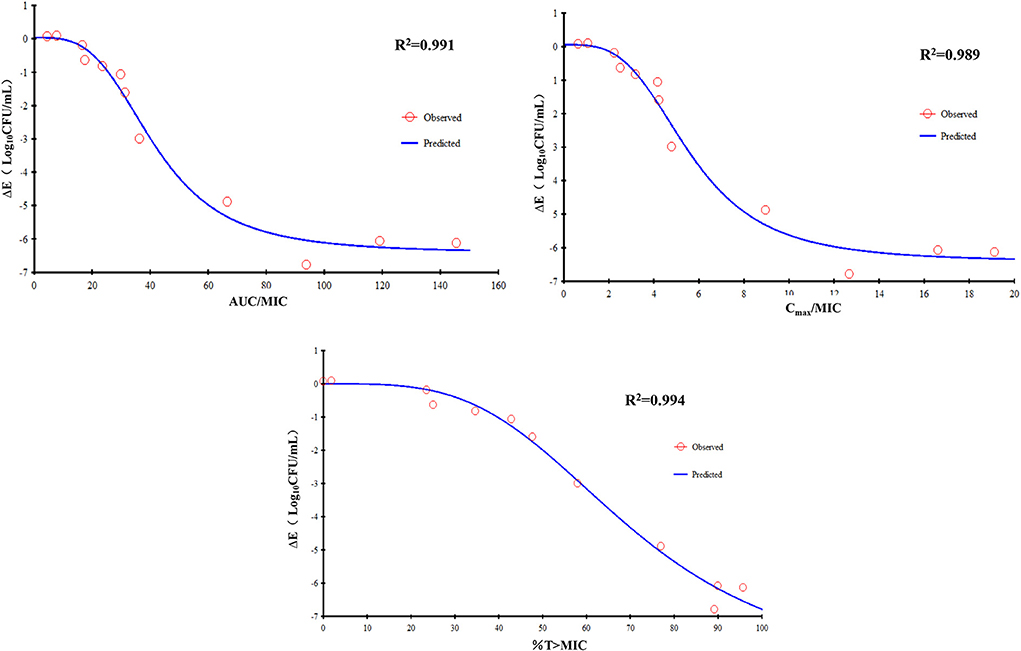
Figure 5. Emax relationships for the three PK/PD parameters of florfenicol used alone vs. the antibacterial effect.
PK/PD modeling of FF in combination with DOX against RA
The dynamic time–kill curves of DOX in combination with FF against RA strains are shown in Figure 6. Growth control, the dose of FF alone at 20 mg/L, and DOX alone at a constant concentration of 0.5 MIC were included in all tests. Marked reductions were documented in the presence of DOX at 0.5 MIC within 24 h: a reduction of 4.31 log10CFU/ml was produced using FF (2 mg/L) against the CVCC3857 strain. Bactericidal activity was reached at FF ≥8 mg/L against the RA38 strain. For RA11, RA17, RA4, and RA24 strains, the maximum reduction of bacteria (in log10CFU/ml) was 6.91, 6.91, 4.53, and 4.97, respectively, at FF ≥16 mg/L.
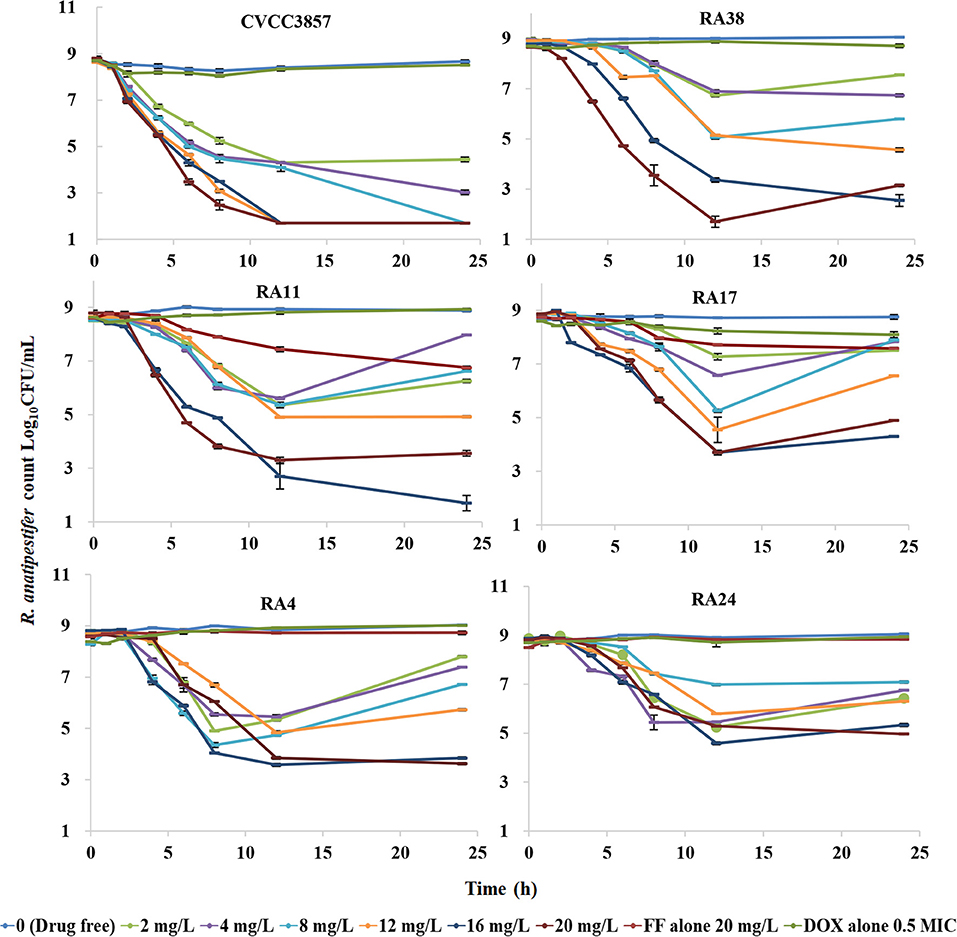
Figure 6. Dynamic time–kill curves of florfenicol in combination with doxycycline against Riemerella anatipestifer.
The data for FF combined with DOX against six RA strains were fitted to the sigmoidal Emax model. The PK/PD parameters that correlated most closely to efficacy were AUC24h/MIC (R2 = 0.861) and Cmax/MIC (R2 = 0.864; Figure 7). The relationship between antibacterial efficacy and PK/PD parameters was assessed by the sigmoidal Emax model, and the obtained parameters of E0, Emax, EC50, and Hill coefficient are presented in Table 3. The magnitude of AUC24h/MIC and Cmax/MIC required for bacterial killing of at 3 log10CFU/ml was 34.84 h and 4.74, respectively.
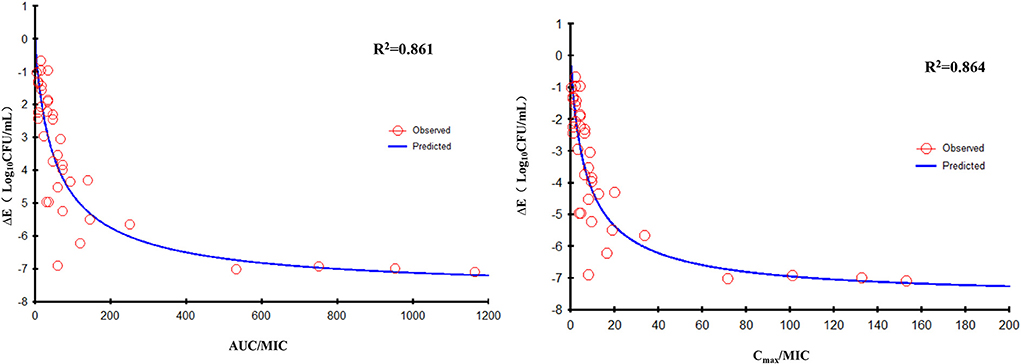
Figure 7. Emax relationships for the three PK/PD parameters of florfenicol in combination with doxycycline vs. the antibacterial effect of different isolates.
Discussion
Widespread use of FF has led to changes in the susceptibility of RA to FF. The MIC of RA strains (n = 66) isolated in Taiwan ranged from ≤ 0.5 to 16 μg/ml, and nine strains possessed the FF-resistant gene floR with a MIC ≥4 μg/ml (9). One study indicated the MIC90 of RA isolates (n = 103) obtained from ducks to be 8 μg/ml during 2008 and 2010 in Southern China (25). The MIC50 and MIC90 of RA isolates (n = 105) from sick ducks or sick geese was 1 and 4 μg/ml, respectively, in Guangdong Province (China), and the MIC of insolates ranged from 0.125 to 16 μg/ml (26). In the present study, the MIC of FF for seven RA strains ranged from 0.5 to 16 μg/ml. The strains selected in our study are important for guiding drug therapy against RA. Similar to other reported pathogens, synergistic and additive effects were observed in the checkerboard assay of FF and DOX against RA. The degree of reduction in the MIC for FF could reach 87.5% (1/8 MIC) upon combination with DOX at 0.5 MIC.
Static time–kill curves demonstrated FF to have a significant effect against RA. At a dose of 30 mg/kg, a Cmax (in μg/ml) of FF in duck plasma of 18.91, 17.86, and 13.88 has been reported in separate studies (27–29). Considering the FF concentration in animals and the MIC of strains, the CVCC3952 and CVCC3857 strains were selected for the static time–kill test. The concentration series was set to 0–32 MIC. A bactericidal effect was desirable if the FF concentration was 1 MIC combined with a DOX concentration of 0.5 MIC. The kill rate of FF in the presence of DOX at 0 h to 9 h was higher than the kill rate of FF alone deduced from the parameters in Table 2. The antibacterial activity of FF against RA improved from the aspects of efficacy and efficacy upon combination with DOX. A certain degree of regrowth was observed in the monotherapy regimens of FF against the CVCC3952 strain at 12–24 h (Figure 1A). This problem could be overcome by combining FF with DOX (Figure 1C). In addition, the drug-tolerant persistence of RA was eliminated by combining two or three antimicrobial agents (21).
We adopted a one-compartment open model to simulate the PK of ducks to study the effect of dynamic drug concentrations against RA. Due to the rapid absorption of FF following extravascular administration, absorption was not considered in this model. Seven strains with different susceptibility to FF were tested in in vitro models to ensure that our results were robust. Although immune factors in animals have not been considered, studies using in vitro models have allowed examination of a direct relationship between dosing regimens and bacteriological effects. The in vitro dynamic time–kill curve characterized the activity of the drug against bacteria preliminarily. Then, data were fitted to the sigmoidal Emax model to obtain specific PK/PD parameters, and the parameter value corresponding to a certain antibacterial effect was calculated by the PK/PD index obtained. Furthermore, PK/PD interactions were analyzed to determine the dependence of the concentration and/or time of the activities (30).
Antibacterial efficacy was characterized in in vitro dynamic time–kill curves. If dose ≥8 mg/L, continuous interactions of FF alone resulted in a bactericidal effect for the CVCC3857 strain of RA (MIC = 1 μg/ml; Figure 4A). The values of the PK/PD parameters AUC24h/MIC, Cmax/MIC, or %T >MIC are indicators for a drug to achieve an antibacterial effect. An increased MIC due to reduced susceptibility and emergence of resistance affect PK/PD parameters, thereby causing treatment failure (31). For the RA38 strain (MIC = 4 μg/ml), a bactericidal effect was not achieved at the maximum administered dose of FF monotherapy (20 mg/L). Similar results were observed for other RA strains with a MIC ≥4 μg/ml in the present study. The effect of FF against RA was enhanced in the presence of DOX. For the CVCC3857 strain, a reduction of 4.31 log10CFU/ml was elicited at a FF dose of 2 mg/L in the presence of DOX compared with a reduction of 0.63 log10CFU/ml using FF alone within 24 h. Marked reductions were observed in all strains at different doses of FF administered along with constant infusion of DOX at 0.5 MIC. The combination of FF with DOX reduced the bacterial burden ≥4.53 log10CFU/ml from the initial inoculum for the RA38, RA11, RA17, RA4, and RA24 strains with MICs ≥4 μg/ml. Drug combinations delay development of bacterial resistance, enhance antibacterial activity, and treat the infections caused by drug-resistant bacteria.
According to the bactericidal properties of drugs on bacteria, drugs can be divided into time-dependent drugs and concentration-dependent drugs. If the antibiotic concentration is increased, concentration-dependent drugs lead to faster killing, whereas the kill rate of time-dependent drugs might remain constant. In the present study, static time–kill curves showed that a higher concentration of antibiotic elicited a more robust antibacterial effect at 0–9 h. However, at 24 h of the test, bacterial counts dropped to the limit of detection in time–kill studies and dynamic studies if the concentration was >4 MIC or >8 mg/L, respectively. The correlation coefficient of AUC24 h/MIC, Cmax/MIC, and %T >MIC with an antibacterial effect was 0.991, 0.989, and 0.994, respectively. Whether the mechanism of action of FF against RA was driven by concentration or time is not known. When FF was combined with DOX, fitting of the Emax model showed that the effect on RA was concentration-dependent and driven by AUC24h/MIC and Cmax/MIC. The AUC24h/MIC, Cmax/MIC, and %T >MIC of FF alone for eliciting a reduction of 3 log10CFU/ml was 40.10 h, 5.43, and 58.71, respectively. For strains with a high MIC that could not be treated with FF alone, the value of AUC24h/MIC, and Cmax/MIC required to produce a reduction of 3 log10CFU/ml was 34.84 h and 4.74 in the presence of DOX at 0.5 MIC, respectively. Hence, combination therapy of FF and DOX with a high MIC was efficacious against the infections caused by RA. Possibly, the presence of DOX reduced the MIC of FF and thereby improved the AUC/MIC ratio.
An appropriate PK/PD index can aid prediction of antibacterial efficacy: %T >MIC for a time-dependent drug or AUC24h/MIC for a concentration-dependent drug, but Cmax/MIC is not considered by the European Committee on Antimicrobial Susceptibility Testing (32). Studies have demonstrated that the killing action of FF on the respiratory-tract pathogens of cows and pigs is concentration-dependent and driven by AUC24h/MIC. The AUC24h/MIC for a reduction of 3 log10 has been reported to be 44.02 h for Streptococcus suis, 37.3 h for P. multocida, and 58.4 h for A. pleuropneumoniae in pigs (13, 33), whereas 26.6 h for M. haemolytica and 18.1 h for P. multocida has been reported for calves (16). The AUC24h/MIC for eliciting a reduction of 3 log10CFU/ml on RA was 40.10 h in our study. In the studies mentioned above, FF had a long t1/2β in pigs and calves. The AUC24h/MIC is the most appropriate index if t1/2β is long. Hence, a single FF dose of 40 mg/kg should elicit a more robust bactericidal effect than a dosing regimen of two doses of 20 mg/kg at an interval of 48 h against the respiratory pathogens in calves (34). The best-fitted PK/PD index can be dependent upon the t1/2β of the drug. It has been shown that the f AUC/MIC and f T >MIC have a similar predictive capacity for β-lactams, with a preference for f T >MIC if t1/2β is relatively short to the dosing interval and f AUC/MIC if t1/2β is relatively long to the dosing interval (35, 36). Thus, the selection and magnitude of PK/PD indices differ among animals with a varying capacity to eliminate a drug (20). This is the probably the case for FF with a longer t1/2β sufficient for T >MIC to suppress regrowth of the main population, so AUC24h/MIC is the best predictor in pigs and calves. In the present study, the correlation coefficient of AUC24 h/MIC and %T >MIC with an antibacterial effect was 0.991 and 0.994, respectively. Also, %T >MIC was as suitable as AUC24h/MIC as the PK/PD index for predicting bacterial killing. Alternatively, a shorter interval should be considered in ducks due to the short t1/2β of FF.
Conclusions
Synergistic and additive effects were observed in the checkerboard assay of FF and DOX against RA. The antibacterial activity of FF against RA was improved from the aspects of efficacy and efficacy in combination with DOX. Due to a short t1/2β, the obvious concentration-dependent characteristics of FF against RA were not observed. The parameters of AUC24h/MIC, Cmax/MIC, and %T >MIC of FF for eliciting a reduction of 3 log10CFU/ml was 40.10 h, 5.43, and 58.71, respectively. The effect of FF against RA was enhanced by combination with DOX; the AUC24h/MIC and Cmax/MIC required to elicit a reduction of 3 log10CFU/ml was 34.84 h with a MIC ≤ 16 μg/ml for RA strains. These data provide a novel approach for combating RA infections.
Data availability statement
The original contributions presented in the study are included in the article/supplementary material, further inquiries can be directed to the corresponding author.
Author contributions
HD and HZ conceived and designed the experiments, revised the manuscript, and supervision of the entire project. HZ, YH, JY, and XL performed the experiments. HZ analyzed data and wrote the manuscript. All authors have read and approved the final manuscript.
Funding
This work was supported by the National Natural Science Foundation of China (Grant No. 31972733).
Conflict of interest
The authors declare that the research was conducted in the absence of any commercial or financial relationships that could be construed as a potential conflict of interest.
Publisher's note
All claims expressed in this article are solely those of the authors and do not necessarily represent those of their affiliated organizations, or those of the publisher, the editors and the reviewers. Any product that may be evaluated in this article, or claim that may be made by its manufacturer, is not guaranteed or endorsed by the publisher.
References
1. Gyuris É, Wehmann E, Czeibert K, Magyar T. Antimicrobial susceptibility of Riemerella anatipestifer strains isolated from geese and ducks in Hungary. Acta Vet Hung. (2017) 65:153–65. doi: 10.1556/004.2017.016
2. Li T, Shan M, He J, Wang X, Wang S, Tian M, et al. Riemerella anatipestifer M949_0459 gene is responsible for the bacterial resistance to tigecycline. Oncotarget. (2017) 8:96615–26. doi: 10.18632/oncotarget.19633
3. Pathanasophon P, Phuektes P, Tanticharoenyos T, Narongsak W, Sawada T. A potential new serotype of Riemerella anatipestifer isolated from ducks in Thailand. Avian Pathol. (2002) 31:267–70. doi: 10.1080/03079450220136576
4. Chang CF, Lin WH, Yeh TM, Chiang TS, Chang YF. Antimicrobial susceptibility of Riemerella anatipestifer isolated from ducks and the efficacy of ceftiofur treatment. J Vet Diagn Invest. (2003) 15:26–9. doi: 10.1177/104063870301500106
5. Qiu Z, Cao C, Qu Y, Lu Y, Sun M, Zhang Y, et al. In vivo activity of cefquinome against Riemerella anatipestifer using the pericarditis model in the duck. J Vet Pharmacol Ther. (2016) 39:299–304. doi: 10.1111/jvp.12271
6. Turbahn A, De Jackel SC, Greuel E, De Jong A, Froyman R, Kaleta EF. Dose response study of enrofloxacin against Riemerella anatipestifer septicaemia in Muscovy and Pekin ducklings. Avian Pathol. (1997) 26:791–802. doi: 10.1080/03079459708419253
7. Chang FF, Chen CC, Wang SH, Chen CL. Epidemiology and antibiogram of Riemerella Anatipestifer isolated from waterfowl slaughterhouses in Taiwan. J Vet Res. (2019) 63:79–86. doi: 10.2478/jvetres-2019-0003
8. Zhu DK, Luo HY, Liu MF, Zhao XX, Jia RY, Chen S, et al. Various profiles of tet genes addition to tet(X) in Riemerella anatipestifer isolates from ducks in China. Front Microbiol. (2018) 9:585. doi: 10.3389/fmicb.2018.00585
9. Chen YP, Lee SH, Chou CH, Tsai HJ. Detection of florfenicol resistance genes in Riemerella anatipestifer isolated from ducks and geese. Vet Microbiol. (2012) 154:325–31. doi: 10.1016/j.vetmic.2011.07.012
10. Li R, Jiang Y, Peng K, Wang Y, Wang M, Liu Y, et al. Phenotypic and genomic analysis reveals Riemerella anatipestifer as the potential reservoir of tet(X) variants. J Antimicrob Chemother. (2022) 77:374–80. doi: 10.1093/jac/dkab409
11. Rattanapanadda P, Kuo HC, Vickroy TW, Sung CH, Rairat T, Lin TL, et al. In vitro and in vivo synergistic effects of florfenicol and thiamphenicol in combination against swine Actinobacillus pleuropneumoniae and Pasteurella multocida. Front Microbiol. (2019) 10:2430. doi: 10.3389/fmicb.2019.02430
12. Abu-Basha E A, Gharaibeh SM, Thabet AM. In vitro susceptibility of resistant Escherichia coli field isolates to antimicrobial combinations. J Appl Poult Res. (2012) 21:595–602. doi: 10.3382/japr.2011-00500
13. Dorey L, Pelligand L, Cheng Z, Lees P. Pharmacokinetic/pharmacodynamic integration and modelling of florfenicol for the pig pneumonia pathogens Actinobacillus pleuropneumoniae and Pasteurella multocida. PLoS ONE. (2017) 12:e0177568. doi: 10.1371/journal.pone.0177568
14. el-Banna HA. Pharmacokinetics of florfenicol in normal and Pasteurella-infected Muscovy ducks. Br Poult Sci. (1998) 39:492–6. doi: 10.1080/00071669888656
15. Elgeddawy SA, Shaheen HM, El-Sayed YS, Abd Elaziz M, Darwish A, Samak D, et al. Effects of the dietary inclusion of a probiotic or prebiotic on florfenicol pharmacokinetic profile in broiler chicken. J Anim Physiol Anim Nutr. (2020) 104:549–57. doi: 10.1111/jpn.13317
16. Sidhu P, Rassouli A, Illambas J, Potter T, Pelligand L, Rycroft A, et al. Pharmacokinetic-pharmacodynamic integration and modelling of florfenicol in calves. J Vet Pharmacol Ther. (2014) 37:231–42. doi: 10.1111/jvp.12093
17. Tikhomirov M, Pozniak B, Smutkiewicz A, Switała M. Pharmacokinetics of florfenicol and thiamphenicol after single oral and intravenous, as well as multiple oral administrations to geese. Br Poult Sci. (2021) 62:25–31. doi: 10.1080/00071668.2020.1824290
18. Yang F, Sun N, Zhao ZS, Wang GY, Wang MF. Pharmacokinetics of doxycycline after a single intravenous, oral or intramuscular dose in Muscovy ducks (Cairina moschata). Br Poult Sci. (2015) 56:137–42. doi: 10.1080/00071668.2014.989488
19. Li J, Hao H, Cheng G, Wang X, Ahmed S, Shabbir MAB, et al. The effects of different enrofloxacin dosages on clinical efficacy and resistance development in chickens experimentally infected with Salmonella Typhimurium. Sci Rep. (2017) 7:11676. doi: 10.1038/s41598-017-12294-7
20. Nielsen EI, Friberg LE. Pharmacokinetic-pharmacodynamic modeling of antibacterial drugs. Pharmacol Rev. (2013) 65:1053–90. doi: 10.1124/pr.111.005769
21. Tang T, Wu Y, Lin H, Li Y, Zuo H, Gao Q, et al. The drug tolerant persisters of Riemerella anatipestifer can be eradicated by a combination of two or three antibiotics. BMC Microbiol. (2018) 18:137. doi: 10.1186/s12866-018-1303-8
22. Odds FC. Synergy, antagonism, and what the chequerboard puts between them. J Antimicrob Chemother. (2003) 52:1. doi: 10.1093/jac/dkg301
23. Kebriaei R, Rice SA, Stamper KC, Rybak MJ. Dalbavancin alone and in combination with ceftaroline against four different phenotypes of Staphylococcus aureus in a simulated pharmacodynamic/pharmacokinetic model. Antimicrob Agents Chemother. (2019) 63:e01743-18. doi: 10.1128/AAC.01743-18
24. Xiao X, Sun J, Chen Y, Huang RJ, Huang T, Qiao GG, et al. In vitro dynamic pharmacokinetic/pharmacodynamic (PK/PD) modeling and PK/PD cutoff of cefquinome against Haemophilus parasuis. BMC Vet Res. (2015) 11:33. doi: 10.1186/s12917-015-0343-7
25. Sun N, Liu JH, Yang F, Lin DC Li GH, Chen ZL, et al. Molecular characterization of the antimicrobial resistance of Riemerella anatipestifer isolated from ducks. Vet Microbiol. (2012) 158:376–83. doi: 10.1016/j.vetmic.2012.03.005
26. Li Y, Zhang Y, Ding H, Mei X, Liu W, Zeng J, et al. In vitro susceptibility of four antimicrobials against Riemerella anatipestifer isolates: a comparison of minimum inhibitory concentrations and mutant prevention concentrations for ceftiofur, cefquinome, florfenicol, and tilmicosin. BMC Vet Res. (2016) 12:250. doi: 10.1186/s12917-016-0796-3
27. Lan W, Xiao X, Jiang Y, Jiang L, Zhao X, Yu Z, et al. Comparative pharmacokinetics of florfenicol in healthy and Pasteurella multocida-infected Gaoyou ducks. J Vet Pharmacol Ther. (2019) 42:355–60. doi: 10.1111/jvp.12761
28. Tikhomirov M, Pozniak B, Smutkiewicz A, Switała M. Pharmacokinetics of florfenicol and thiamphenicol in ducks. J Vet Pharmacol Ther. (2019) 42:116–20. doi: 10.1111/jvp.12714
29. Xiao X, Lan W, Zhao Y, Li R, Liu Y, Liu J, et al. In vivo pharmacokinetic and pharmacodynamic (PK/PD) modeling and establishment of the PK/PD cutoff of florfenicol against Pasteurella multocida in ducks. Front Microbiol. (2020) 11:616685. doi: 10.3389/fmicb.2020.616685
30. Zhang H, Mao C, Li J, Huang Z, Gu X, Shen X, et al. pharmacokinetic/pharmacodynamic integration of doxycycline against Mycoplasma hyopneumoniae in an in vitro model. Front Pharmacol. (2019) 10:1088. doi: 10.3389/fphar.2019.01088
31. Dudley MN, Ambrose PG. Pharmacodynamics in the study of drug resistance and establishing in vitro susceptibility breakpoints: ready for prime time. Curr Opin Microbiol. (2000) 3:515–21. doi: 10.1016/S1369-5274(00)00132-6
32. Toutain PL, Sidhu PK, Lees P, Rassouli A, Pelligand L. VetCAST Method for determination of the pharmacokinetic-pharmacodynamic cut-off values of a long-acting formulation of florfenicol to support clinical breakpoints for florfenicol antimicrobial susceptibility testing in cattle. Front Microbiol. (2019) 10:1310. doi: 10.3389/fmicb.2019.01310
33. Lei Z, Liu Q, Yang S, Yang B, Khaliq H, Li K, et al. PK-PD integration modeling and cutoff value of florfenicol against Streptococcus suis in Pigs. Front Pharmacol. (2018) 9:2. doi: 10.3389/fphar.2018.00002
34. Pelligand L, Lees P, Sidhu PK, Toutain PL. Semi-mechanistic modeling of florfenicol time-kill curves and in silico dose fractionation for calf respiratory pathogens. Front Microbiol. (2019) 10:1237. doi: 10.3389/fmicb.2019.01237
35. Kristoffersson AN, David-Pierson P, Parrott NJ, Kuhlmann O, Lave T, Friberg LE, et al. Simulation-based evaluation of PK/PD indices for meropenem across patient groups and experimental designs. Pharm Res. (2016) 33:1115–25. doi: 10.1007/s11095-016-1856-x
Keywords: florfenicol, doxycycline, combination therapy, Riemerella anatipestifer, PK/PD model
Citation: Zhang H, Huang Y, Yu J, Liu X and Ding H (2022) PK/PD integration of florfenicol alone and in combination with doxycycline against Riemerella anatipestifer. Front. Vet. Sci. 9:975673. doi: 10.3389/fvets.2022.975673
Received: 22 June 2022; Accepted: 19 August 2022;
Published: 08 September 2022.
Edited by:
Arturo Anadón, Complutense University of Madrid, SpainCopyright © 2022 Zhang, Huang, Yu, Liu and Ding. This is an open-access article distributed under the terms of the Creative Commons Attribution License (CC BY). The use, distribution or reproduction in other forums is permitted, provided the original author(s) and the copyright owner(s) are credited and that the original publication in this journal is cited, in accordance with accepted academic practice. No use, distribution or reproduction is permitted which does not comply with these terms.
*Correspondence: Huanzhong Ding, aHpkaW5nQHNjYXUuZWR1LmNu